- 1Department of Food and Nutrition, College of Community Science, G.B. Pant University of Agriculture and Technology, Pantnagar, Uttarakhand, India
- 2Department of Food Science and Technology, College of Agriculture, G.B. Pant University of Agriculture and Technology, Pantnagar, Uttarakhand, India
- 3Department of Processing and Food Engineering, MCAET, Acharya Narendra Dev University of Agriculture and Technology, Ayodhya, Uttar Pradesh, India
- 4Division of Crop Improvement, ICAR-VPKAS, Almora, Uttarakhand, India
Objective: The present study assessed the impact of different bio-processing treatments on the in vitro protein digestibility (IVPD) and inhibitory properties against α-amylase and α-glucosidase of whole grains viz. black wheat (BW), barnyard millet (BM), and black soybean (BS) using at initial optimized conditions including soaking and germination.
Method: Nutritional analysis of grains was done using standard AOAC methods and in vitro models were used for assessing the anti-diabetic properties.
Results: Nutritional analysis of the grains revealed significant differences in various parameters. BS having higher levels of moisture, ash, crude protein, crude fat, and physiological energy value compared to BM and BW. Optimization of soaking (0–24 h) and germination (0–72 h) times showed significant improvements in in-vitro protein digestibility (IVPD), trypsin inhibitory activity (TIU/mg), and glycemic index (GI) across all samples. Considering the management of hyperglycemia, BW and BM do not require any prior processing to be utilized for the development of food products. However, for BS, soaking followed by germination for 48 h proved to be an effective processing, that resulted in an IVPD of 68.706% and a GI of 51.03, with a TIU/mg of 23.166. Soaking reduced α-amylase and α-glucosidase inhibition, while germination increased inhibitory activity significantly (p < 0.05).
Conclusion: These findings highlighted the potential benefits of incorporating BW, BM, and BS into the diet for enhanced nutrient intake and better management of hyperglycemia considering the GI and inhibitory activity of α-amylase, α-glucosidase, and trypsin enzymes.
1 Introduction
In the realm of nutrition, whole grains (WG) stand out as vital components of a healthy diet. Comprising the outer bran, germ, and inner endosperm, these grains are abundant in dietary fiber, antioxidants, and essential micronutrients (1). Various researches including human trials and prospective cohort studies increasingly highlights the role of whole grains in reducing fat mass, increasing resting metabolic rate, promoting negative energy balance, increasing insulin sensitivity, managing hyperglycemia, improving the lipid profile, and reducing systemic inflammation (1, 2).
Diet plays a crucial role in managing hyperglycemia. Key dietary factors, including the content of carbohydrates, fiber, and protein, can significantly influence the glycemic index (GI) and insulin secretion (3). The GI is a measure that ranks foods based on how quickly glucose is released into the bloodstream after consumption (3, 4). Foods are categorized into high-GI (> 70), intermediate-GI (55–70), and low-GI (< 55) based on their effects on blood glucose levels (5). Incorporating low-GI foods into daily diets can be particularly beneficial especially for the management of blood glucose levels (6). For instance, pigmented grains like black wheat (BW), millets such as barnyard millet (BM), and legumes like black soybean (BS) can help regulate postprandial insulin and glucose levels. These foods fall under low GI (<55) category among other grains that are part of their respective classes of cereals, millets and legumes. This cause only moderate or low increases in glucose after intake, followed by a gradual return to baseline levels, thereby aiding in maintaining stable glucose levels (7). Supporting this, a recent network meta-analysis of 56 trials demonstrated that low-GI diets significantly reduced both glycated hemoglobin (HbA1c) and fasting blood glucose (FBG) compared to control diets (8).
Another approach to controlling postprandial glycemia involves slowing down carbohydrate digestion. This can be achieved by inhibiting the activity of enzymes like α-amylase and α-glucosidase, which are responsible for breaking down starches into glucose (9, 10). α-Amylases, found in the salivary glands and pancreas, break down starches into disaccharides and oligosaccharides. These are further converted into glucose by α-glucosidases located in the small intestine. By inhibiting these enzymes, the rate of starch digestion slows, which can help manage postprandial hyperglycemia.
However, managing diabetes is not solely about dietary components. The structural properties of raw materials, the types of processing methods used, and the structure of the final food products can all impact the GI and insulin secretion (3). Common processing techniques such as soaking and germination induce a range of qualitative changes in raw foods, affecting their physical properties, nutritional composition, and starch characteristics. Despite their significance, there is limited data on how these processing methods affect low-GI foods. Also, while studies have explored the benefits of bio-processing treatments like germination and soaking on individual cereals and legumes, limited research exists on their combined impact on nutrient digestibility and enzyme inhibitory activity in diverse genotypes.
Therefore, to address this gap, the present study focuses on optimizing the processing parameters and examining how soaking and germination impact the nutritional and anti-diabetic properties of low-GI foods like BW, BM, and BS. Also, combined impact on nutrient digestibility and enzyme inhibitory activity in diverse genotypes such as BW, BM and BS was studied in the present research. This bridges that gap by providing genotype-specific insights into bio-processing benefits. Through this research, we aim to enhance our understanding of how processing methods can be leveraged to improve the management of diabetes and contribute to better health outcomes.
2 Materials and methods
2.1 Raw materials
BW line [NABIMG-11-Black (BW/2* PBW621; IC0620916; INGR17003), a wheat (Triticum aestivum) germplasm with black grain color] was procured from the local market of Uchaiti village, Moradabad, Uttar Pradesh, India. BM (VL172) and BS (VL Bhat 201) were procured from Vivekananda Parvatiya Krishi Anusandhan Sansthan (VPKAS), Almora, Uttarakhand, India. The glucose assay kit was purchased from Megazyme International Ireland Ltd. (Wicklow, Ireland). All other analytical grade reagents were purchased from Sisco Research Laboratories Pvt. Ltd. (SRL), Mumbai, India.
2.2 Cleaning and processing
All extraneous materials, including broken seeds, dust, stones, and fragments of metal and glass, were removed manually from the grains. BW, BM and BS were adjusted to a moisture content of 10 ± 1%. Samples were soaked at 30 ± 5°C (RH > 80%) and weighed after a gap of every 1 h until consistent weight gain was observed. Germination was carried out at 30 ± 5°C (RH > 80%) until a non-significant increase in sprout length was observed. Soaked and germinated samples were dried at 40 ± 5°C for 24 h. The samples were ground to make flour using a mixer grinder (INALSA, MG Compact Lx, B00ID6GEVM, Tuareg Marketing Pvt. Ltd., Noida, Uttar Pradesh) and stored in airtight containers till further analysis. Figure 1 depicts the schematic depiction of the study design.
2.3 Nutritional analysis
The proximate composition of samples was analyzed using standard methods AOAC (11). In vitro protein digestibility was assessed by employing Akeson and Stahmann (12) method.
2.4 Anti-diabetic properties
The GI of grains was calculated in vitro by Goni et al. (4), and was expressed as the predicted glycemic index (pGI). The hydrolysis index (HI) was calculated by using the trapezoid rule to compute the area under the hydrolysis curves (AUC, 0–180 min). The percentage relationship between the AUC for the test food and the reference food (glucose) was used to calculate the HI. The technique described by Wongsa et al. (10) and Wang et al. (9) was employed to estimate the inhibitory activity of α-amylase and α-glucosidase.
2.5 Anti-nutritional properties
Trypsin inhibitor activity (TIA) is its ability to inhibit the caesinolytic activity of trypsin was determined by the method of Liu (13) and results were expressed as percent trypsin inhibition.
2.6 Statistical analysis
IBM SPSS Statistics 27.0 software, IBM, United States, was used for statistical analysis, and the data was reported as mean ± standard error (SE). Significant differences between means were found using analysis of variance (ANOVA) and post-hoc Duncan multiple range test (DMRT) at p < 0.05.
3 Results
3.1 Proximate composition
Nutritional analysis of samples BW, BM, and BS revealed significant differences (p < 0.05) in various parameters, shedding light on their respective nutritional qualities (Table 1). All samples stand out with notably good levels of ash, crude protein, crude fat, and physiological energy value (PEV). This suggested that these foods potentially offer a richer source of essential nutrients (both macro- and micro-nutrients) and energy. Additionally, BW and BM showcased higher carbohydrate content and lower crude fat in comparison to BS as shown in Table 1.
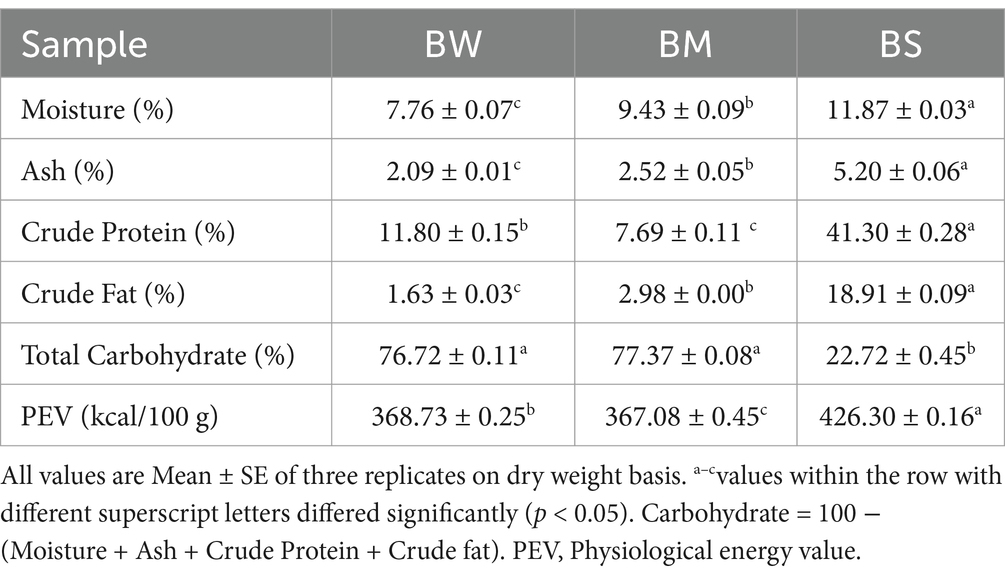
Table 1. Proximate composition of selected grains (black wheat, BW; barnyard millet, BM; black soybean, BS; % dw basis).
3.2 Optimization of soaking and germination time
The relationship between soaking time and the weight gain of grains across three samples: BM, BS, and BW has been illustrated in Table 2. Initially, at 0 h of soaking, all samples start with a uniform weight of 50 g. As soaking time increased, there was a consistent trend of weight gain observed across all samples. However, BM exhibits the slowest rate of weight gain, while BS and BW showed comparatively higher rates, resulting in significant differences (p < 0.05) in weight gain between the samples. This disparity persisted throughout the soaking period, with BM consistently lagging behind BS and BW. Notably, beyond 8 h of soaking, the rate of weight gain tends to plateau for all samples, indicating that after 8, 12, and 16 h the weight of samples showed insignificant changes. Based on these observations, the optimum soaking time (OS) for BM, BS, and BW to achieve maximal weight gain was found to be around 12, 16, and 11 h where the weight was increased by 31, 130, and 57% respectively, beyond which was a insignificant change in weight.
Germination was carried out for 72 h and was optimized based on sprout length (Figure 2). The data illustrates the relationship between germination time and sprout length across three samples: BW, BM, and BS. As the germination time increases, there are noticeable changes in sprout length for each sample. Initially, at 0 h, all samples show negligible sprout growth. However, after 12 h, BS exhibited significant (p < 0.05) sprout growth (6.00 ± 0.58 mm), while BM and BW remained relatively unchanged. By 24 h, all samples displayed varying degrees of sprout elongation, with BS exhibiting the longest sprouts (17.25 ± 0.14 mm), followed by BW (2.50 ± 0.00 mm) and BM (0.77 ± 0.02 mm). This trend continued up to 48 h, with BS consistently producing the longest sprouts (23.17 ± 0.44 mm), followed by BM (5.83 ± 0.17 mm) and BW (12.83 ± 0.17 mm). Notably, the rate of sprout growth stabilized after 48 h, as indicated by marginal changes in sprout length from 48 to 72 h across all samples. Adverse quality changes were noticed in the sprouts after 48 h of germination among all samples. These observations suggested that while germination time positively correlates with sprout length, there is a plateauing effect beyond a certain duration. Considering the above findings, the optimum germination time for all three samples was observed to be 48 h. However, these findings underscore the dynamic relationship between germination time and sprout growth, highlighting the potential for optimizing germination conditions to enhance sprout length, which can have implications for the improved nutritional quality and commercial viability of sprouted products.
3.3 Effect of processing on in vitro protein digestibility
Impact of various processing methods on in vitro protein digestibility (IVPD) across three different samples viz., BW, BM, and BS has been illustrated in Figure 3. The results of the Duncan Multiple Range Test (DMRT) revealed that processing showed significant variations in IVPD at p < 0.05 (Table 3). Initially, untreated samples exhibited relatively lower IVPD values, with BS recording the lowest digestibility compared to BM and BW. However, after processing there was a notable improvement in IVPD across all samples. Soaking for 8 to 16 h lead to incremental increase in digestibility, with BM showing the highest enhancement after 12 h of soaking. Subsequent germination periods of 24 to 48 h resulted in significant (p < 0.05) enhancement in IVPD for all samples, with BM demonstrating the highest overall digestibility values. In the present study, protein digestibility improved significantly post-germination. Digestibility increased by 11.4% in NABIMG-11 (BW), 21.7% in VL 172 (BM), and 35.87% in VL Bhat 201 (BS; p < 0.05).
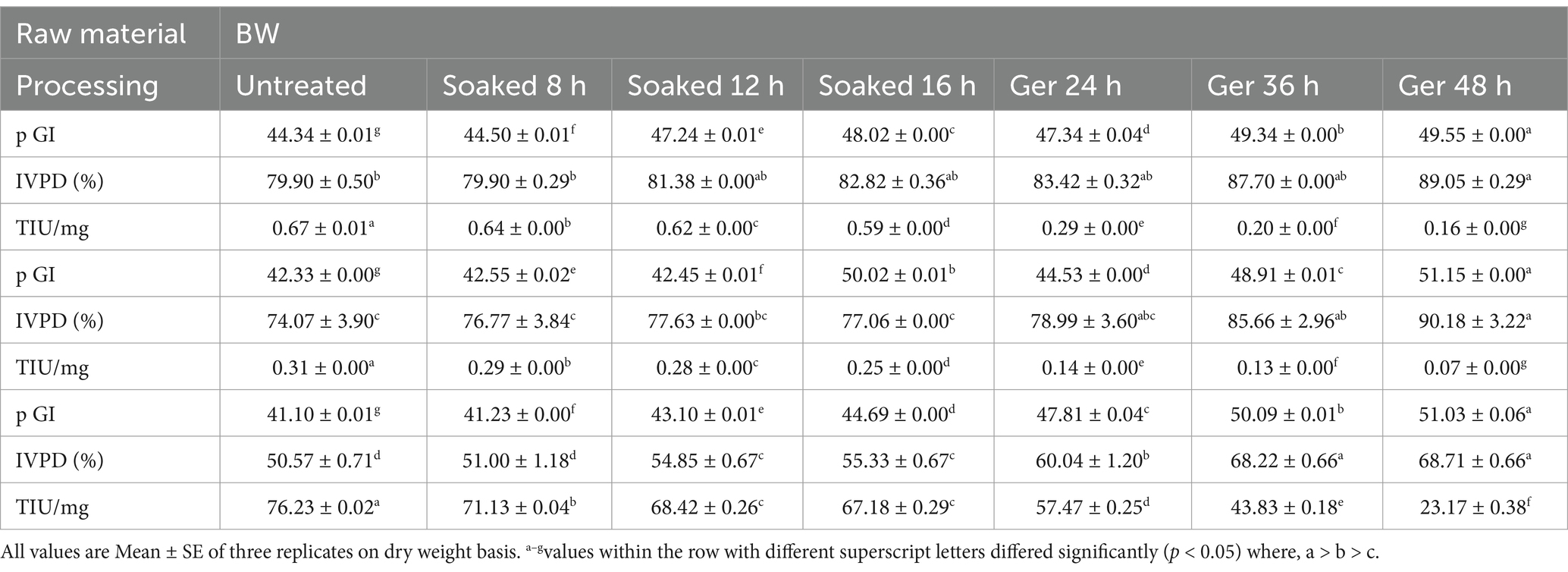
Table 3. Effect of processing on predicted glycemic index (pGI), in vitro protein digestibility (IVPD) and trypsin inhibitory units (TIU) of selected grains (black wheat, BW; barnyard millet, BM; black soybean, BS; % dw basis).
3.4 Effect of processing on GI
The pGI of grains differed significantly (p < 0.05) as a result of the processing of all three samples: BW, BM, and BS (Figure 4). Initially, untreated samples exhibited relatively low pGI values across all samples, with slight variation among them. Upon processing, there was a discernible trend of increasing pGI observed across all samples (Table 3). Soaking for 8 to 16 h led to marginal changes in pGI values, with fluctuations observed in all samples. However, more significant alterations were evident following germination periods of 24 to 48 h, with pGI values showing a substantial increase. Notably, BM displayed the most pronounced increase in pGI after 48 h of germination. BS showed a significant increase in pGI with the highest value of 51.03 after 48 h germination. When soaking prolonged to 16 h, BW and BM showed a significant increase in pGI (p < 0.05) however, when grains germinated for 10 and 11 h respectively, pGI showed a significant decline at 24 h germination, which was then gradually increased with increased in germination time.
3.5 Effect of processing on enzyme inhibitory activity
Alpha-amylase (1:2000 U/mg) inhibitory activity differed significantly (p < 0.05) in processed samples (BW, BM, and BS; Figure 5a). Soaking of grains reduced the α-amylase inhibition by 5, 18, and 26% for BM, BW, and BS, respectively. During germination, α-amylase inhibitory activity increased significantly (p < 0.05) with the increase in germination time. α-glucosidase (100 U/mg) inhibitory activity showed similar trends where soaking significantly reduced the α-glucosidase inhibition by 16, 29, and 17% for BM, BW, and BS, respectively, (Figure 5b). Whereas, germination significantly (p < 0.05) increased the inhibitory activity (α-amylase and α-glucosidase) with the increase in germination time by 17.06 and 11.8% for BW;11.8and 3.16% for BM (p < 0.01) respectively.
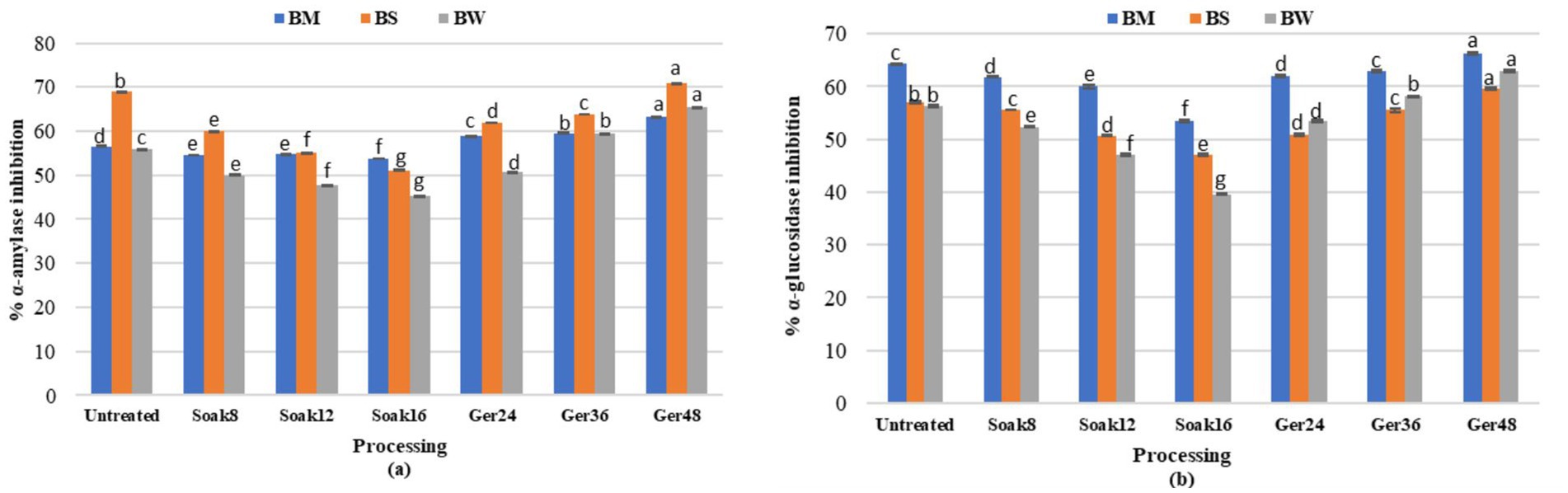
Figure 5. Effect of processing on (a) a-amylase and (b) a-glucosidase inhibitory activity of BW, BM and BS.
3.6 Effect of processing on trypsin inhibitory activity
The impact of various processing methods on trypsin inhibitory units (TIU) in three different samples: BW, BM, and BS has been presented in Figure 6. Initially, untreated samples exhibited relatively high TIU values across all samples, with BS recording the highest inhibitory activity compared to BM and BW. Processing impacted a decrease in TIU values across all samples (Table 3). Soaking for 8 to 16 h led to gradual reductions in TIU, with a notable decrease of 19, 12, and 12% in BM, BW, and BS, respectively. Subsequent germination periods of 24 to 48 h resulted in a significant (p < 0.05) decrease in TIU by 77, 70, and 77% for BM, BW, and BS, respectively, with BM showing the most pronounced reduction in trypsin inhibitory activity.
3.7 Selection of processing method considering management of hyperglycemia
Opting for a suitable processing method is crucial for maximizing nutritional quality while minimizing glycemic response and trypsin inhibitory activity of the three low GI samples: BW, BM, and BS. BIS (14) standards, stated that the TIU/mg should be less than 75 for legume flour (soybean) that will be used in the development of products that require further cooking. Talking of the quality of protein in terms of its digestibility, Nosworthy et al. (15) reported that pulses with more than 70% protein digestibility are considered good in digestibility. As for the GI, it should be less than 55 to fall under the low-GI category (5). Therefore, considering these goals the processing method selected for the raw material was, BM (unprocessed), BS (germinated 48 h), and BW (unprocessed) as shown in Table 3. However, all three grains fall under the low GI category with GI < 55 before any bio-processing and even after the application of a bio-processing treatment that not only improved the protein digestibility but still showed lower GI.
4 Discussion
Dietary management plays a crucial role in maintaining blood glucose levels in diabetic individuals. This can be managed through slowing down rate of carbohydrate digestion by incorporating hydrolyzing enzymes (α-amylase and α-glucosidase) inhibitors or low GI foods in the diet or both. However, prior processing is essential to improve its nutritional and functional properties that affect the physical properties, nutritional composition, starch characteristics, cooking qualities, etc. Therefore, it is pivotal to understand those changes during processing to attain the maximum nutritional benefits from food.
4.1 Proximate composition
Proximate composition of raw grains emphasized the potential benefits of incorporating BW, BM, and BS individually or in combination into the diet for enhanced and balanced nutrient intake. All samples showed moisture content <12% which is indicative of their good shelf-life as increased moisture levels may contribute to reduced shelf-life due to greater susceptibility to microbial spoilage and oxidative degradation (16). Ash content in all samples was good, which denotes the mineral content of the food and consists of major elements such as Na, K, Ca, and Mg, and trace elements such as Fe, Zn, and Cu (7). Increased mineral concentration was linked, indirectly, to increased hygroscopicity through interactions between minerals carbohydrates, and water (17). Similar to other research, BW and BS showed a higher content of protein that represents the good quality of protein in terms of its amino acid composition (7, 18). Further exploration into the specific nutritional constituents of BW, BM, and BS could provide valuable insights into their potential dietary applications associated with various health conditions.
4.2 Optimization of soaking and germination time
Soaking increased weight gain due to water imbibition by grains leading to increased water absorption capacity during soaking which might be due to changes in total protein structure and loosening of starch polymers during soaking (19). Another explanation for weight gain after soaking may be because of the rise in the protein content which possesses both hydrophilic and hydrophobic nature and so may interact with both water and oil in foods, and ultimately accountable for enhancement in water absorption (20). However, further scientific discussion regarding the mechanisms underlying the saturation of grain absorption capacity and the potential implications for industrial processes and nutritional enhancement strategies is required. Germination time of grains positively correlated with sprout length. Many researchers studied the optimum germination time from 12 to 72 h (21, 22), some continued germination until the length of sprouts reaches 2/3rd of the length of seeds (23) associated with the specific grain type. However, in the present study, there observed a plateauing effect beyond a certain duration along with spoilage signs were observed in the samples on germination beyond 48 h. Studies reported that germination for a shorter duration is more effective for reducing antinutritional factors and preserving their chemical composition, thus improving their potential as a high-quality raw material for plant-based foods (24). These findings underscore the dynamic relationship between soaking-weight gain, and germination time-sprout growth, highlighting the need for optimizing soaking and germination conditions for BW, BM, and BS, which can have implications for the utilization of these grains in the development of value-added food products.
4.3 Effect of processing on in vitro protein digestibility
Processing like soaking and germination improved the digestibility of protein for all grains (p < 0.05). Similar observations were observed for cowpea and lentils (25); finger millet (26), and maize (22). These findings emphasized the efficacy of soaking and germination processes in enhancing protein digestibility, potentially attributed to enzymatic activities and structural modifications during processing (27).
Enhanced protein digestibility suggested enhanced biological value of proteins, which could be attributed to lower levels of antinutrients (28), the partial solubilization that occurs during seed sprouting, indicated by higher levels of water-soluble proteins and free amino acids in the sprouted seeds, and the proteolysis of complex protein structures into more digestible forms (22, 29). The improved digestibility, as observed in this study, may contribute to better protein and carbohydrate absorption, leading to enhanced satiety and reduced gastrointestinal discomfort. Enhanced protein digestibility can support muscle maintenance and metabolic health, while improved starch breakdown can minimize bloating and digestive issues, particularly for individual’s sensitivities to complex carbohydrates (29). However, the variations in digestibility among samples emphasize the importance of considering intrinsic factors such as grain type and composition in optimizing processing methods for improved protein bioavailability. Further exploration into the underlying mechanisms driving protein digestibility enhancements during processing could provide valuable insights for developing tailored strategies to maximize the nutritional benefits of grain-based products.
4.4 Effect of processing on GI
Findings suggested that processing methods, particularly prolonged germination durations, can significantly impact the glycemic response of grains (p < 0.05). The observed rise in pGI may be attributed to enzymatic activities during processing, which can potentially break down complex carbohydrates into simpler sugars (30, 31), leading to faster glucose release upon digestion. According to some studies, these circumstances also lead to both the leaching of bioactive components and increased enzyme activity (32). Additionally, variations in pGI among samples highlight the importance of considering grain type and processing conditions in managing glycemic responses, particularly for individuals with conditions like diabetes. In addition to dietary considerations, variations in the raw material’s structural qualities, the way food is processed, and the end product’s structure can all impact GI and insulin secretion (3). High GI of food resulted in rapid absorption of carbohydrates that evoked high postprandial blood glucose levels and insulin concentrations which lead to the development of insulin resistance and the incidence of T2D (31). Though, it is evident that even after prior processing, all the grains still fell under the low GI category (pGI < 55), further investigation into the underlying mechanisms driving changes in pGI during processing could yield valuable insights for optimizing grain processing techniques to mitigate adverse glycemic effects while maximizing nutritional benefits and establish their potential as a functional food.
4.5 Effect of processing on enzyme inhibitory activity
Enzyme inhibitory activity was affected significantly during processing. Soaking resulted in declined inhibition which aligns with previous research demonstrating that soaking reduces α-amylase and α-glucosidase activities (p < 0.05) (33). This decline in activity might be attributed to the leaching out of anthocyanin components that are responsible for imparting the α-amylase inhibitory activity (33, 34). However, the current study revealed that the grains, especially the germinated form, exhibited inhibitory activity against porcine pancreatic α-amylase and α-glucosidase because they had higher polyphenolic indexes (35), which are linked to the inhibition of hydrolyzing enzymes in a dose-dependent manner (36). According to recent research, phenolic compounds may bind to amino acid residues at the active sites of digestive enzymes through hydrogen bonding, which prevents the digestive enzymes from catalyzing the catalytic reaction on carbohydrates. This explains how phenolic compounds inhibit the activities of digestive enzymes (37, 38). Furthermore, these phenolic acids can bind to the active protein pocket of α-glucosidase, which decreases glucose absorption by favoring dissipation of the Na + electrochemical gradient. This is the mechanism that propels the build-up of active glucose and subsequent glucose transport (39), as a result of which they are recognized to reduce the absorption of glucose and control postprandial hyperglycemia (36), suggesting that they may be used therapeutically to treat diabetes mellitus. Though the in vitro demonstration of the inhibitory impact of the grain extracts has been demonstrated, these results suggest that the extracts may be able to reduce the starch hydrolysis-induced rise in postprandial glucose.
4.6 Effect of processing on trypsin inhibitory activity
Processing reduced the anti-nutritional factors, particularly prolonged germination durations, effectively reduced trypsin inhibitory activity in grains (p < 0.05) and thereby reduced the levels of anti-nutrients present. This reduction in TIU was comparable to the reduction observed in soybean (40), mung bean (41) and finger millet (22). The two factors responsible for this decrease in TIA are soaking in water, which causes soluble substances, such as trypsin inhibitors, to leach into the soaking water, and activating endogenous protease, which is related to trypsin inhibitor and is sensitive to temperature, water content, and other environmental factors (42). Activation of proteases resulted in the breakdown of proteins, including trypsin inhibitors, into smaller peptides and amino acids. This increased enzymatic activity during germination, leading to the degradation of trypsin inhibitors (22). Thus, the mechanism of endogenous proteases linked to trypsin inhibitor levels can be studied further. Additionally, variations in TIU reduction among samples highlight the importance of considering grain type and processing conditions in managing trypsin inhibitory activity, particularly for enhancing the nutritional quality and digestibility of grain-based products for maximizing nutritional benefits.
4.7 Selection of processing method considering management of hyperglycemia
Considering the requirements for the management of diabetes through dietary approaches, it was crucial to optimize the processing parameters as well as understand the effect of processing on the nutritional quality so that the end-product should impart maximum digestibility of protein with a minimum release of glucose into the bloodstream, along with minimum anti-nutrients to hinder nutrient bioavailability. Based on the standard values where IVPD should be more than 70% (15), GI should be less than 55 (5) and TIU should be less than 75 (14), it was observed that BW and BM do not require any prior processing to be utilized for the development of food products. However, for BS soaking followed by germination for 48 h proved to be an effective processing, that resulted in an IVPD of 68.706% and a GI of 51.03, with a TIU/mg of 23.166. While the TIU/mg value is slightly higher than the specified threshold, it remains within acceptable limits, considering the significant improvement in IVPD and GI. This suggests a potential benefit for managing postprandial glucose responses, making these bio-processed grains suitable for diabetic and pre-diabetic populations. Also, the global dietary landscape is increasingly shifting toward low-GI wholegrain foods due to their benefits in managing diabetes, promoting weight control, and supporting metabolic health (3). The observed reductions in GI and enzyme activity inhibition suggest that BW, BM, and BS could be formulated into functional foods targeting diabetic and pre-diabetic populations. This aligns with the growing consumer preference for functional foods that regulate postprandial glucose levels having potential applications including low-GI goods, fortified protein products, and diabetic-friendly meal alternatives, addressing the rising global incidence of metabolic disorders. Additionally, such techniques align with clean-label food trends by naturally improving food quality without synthetic additives (35).
5 Conclusion
The nutritional profiles of BW, BM, and BS are distinct, highlighting the potential benefits of incorporating these grains individually or in combination into the diet for enhanced and balanced nutrient intake. Optimizing soaking and germination times improved protein digestibility, GI, and, trypsin inhibitory activity, particularly across all samples. Considering the management of hyperglycemia, the selected processing methods for the raw materials were BM (unprocessed), BS (germinated 48 h), and BW (unprocessed), align with established standards and offer balanced nutritional benefits, highlighting the importance of tailored techniques for optimizing grain nutritional benefits. Soaking reduced α-amylase and α-glucosidase inhibition, while germination increased inhibitory activity.
Further exploration into the underlying mechanisms driving these changes during processing could inform more efficient grain processing techniques and enhance the nutritional quality of grain-based products.
Data availability statement
The original contributions presented in the study are included in the article/supplementary material, further inquiries can be directed to the corresponding author.
Author contributions
KG: Conceptualization, Data curation, Formal analysis, Investigation, Writing – original draft, Writing – review & editing. AK: Formal analysis, Methodology, Resources, Supervision, Writing – review & editing. AD: Supervision, Writing – review & editing. SS: Supervision, Writing – review & editing. NS: Supervision, Writing – review & editing. DJ: Resources, Supervision, Writing – review & editing. AB: Resources, Supervision, Writing – review & editing. PG: Writing – review & editing.
Funding
The author(s) declare that no financial support was received for the research and/or publication of this article.
Acknowledgments
The authors appreciate the help of the Department of Food Science and Nutrition, G.B. Pant University of Agriculture and Technology, Pantnagar, for providing the necessary facilities to carry out the research work. Also, appreciate ICAR-VPKAS, Almora for providing the raw material resources to carry out the study.
Conflict of interest
The authors declare that the research was conducted in the absence of any commercial or financial relationships that could be construed as a potential conflict of interest.
The reviewing editor SPS declared a shared affiliation with the author DJ at the time of review.
Generative AI statement
The author(s) declare that no Gen AI was used in the creation of this manuscript.
Publisher’s note
All claims expressed in this article are solely those of the authors and do not necessarily represent those of their affiliated organizations, or those of the publisher, the editors and the reviewers. Any product that may be evaluated in this article, or claim that may be made by its manufacturer, is not guaranteed or endorsed by the publisher.
References
1. Ghanbari-Gohari, F, Mousavi, SM, and Esmaillzadeh, A. Consumption of whole grains and risk of type 2 diabetes: a comprehensive systematic review and dose–response meta-analysis of prospective cohort studies. Food Sci Nutr. (2022) 10:1950–60. doi: 10.1002/fsn3.2811
2. Hu, Y, Ding, M, Sampson, L, Willett, WC, Manson, JE, Wang, M, et al. Intake of whole grain foods and risk of type 2 diabetes: results from three prospective cohort studies. BMJ. (2020) 370:m2206. doi: 10.1136/bmj.m2206
3. Nelson, K, Stojanovska, L, Vasiljevic, T, and Mathai, M. Germinated grains: a superior whole grain functional food? Can J Physiol Pharmacol. (2013) 91:429–41. doi: 10.1139/cjpp-2012-0351
4. Goñi, I, Garcia-Alonso, A, and Saura-Calixto, F. A starch hydrolysis procedure to estimate glycemic index. Nutr Res. (1997) 17:427–37. doi: 10.1016/S0271-5317(97)00010-9
5. Eleazu, CO. The concept of low glycemic index and glycemic load foods as panacea for type 2 diabetes mellitus; prospects, challenges and solutions. Afr Health Sci. (2016) 16:468–79. doi: 10.4314/ahs.v16i2.15
6. WHO (2017). Healthy eating habits 2 for patients with diabetes. Available online at: https://iris.who.int/bitstream/handle/10665/254746/9789290618072-diab-mod4-eng.pdf?sequence=11&isAllowed=y (Accessed September 10, 2024).
7. Goel, K, Kushwaha, A, Dutta, A, Sharma, SK, Shahi, NC, and Kumar, A. Nutritional quality of black wheat flatbread: a therapeutic alternative for diabetes management. Annals of Phytomedicine. (2024) 13:1–10. doi: 10.54085/ap.2024.13.1.88
8. Schwingshackl, L, Chaimani, A, Hoffmann, G, Schwedhelm, C, and Boeing, H. A network meta-analysis on the comparative efficacy of different dietary approaches on glycaemic control in patients with type 2 diabetes mellitus. Eur J Epidemiol. (2018) 33:157–70. doi: 10.1007/s10654-017-0352-x
9. Wang, T, Li, X, Zhou, B, Li, H, Zeng, J, and Gao, W. Anti-diabetic activity in type 2 diabetic mice and α-glucosidase inhibitory, antioxidant and anti-inflammatory potential of chemically profiled pear peel and pulp extracts (Pyrus spp.). J Funct Foods. (2015) 13:276–88. doi: 10.1016/j.jff.2014.12.049
10. Wongsa, P, Chaiwarith, J, Voranitikul, J, Chaiwongkhajorn, J, Rattanapanone, N, and Lanberg, R. Identification of phenolic compounds in colored rice and their inhibitory potential against α-amylase. Chiang Mai J Sci. (2019) 46:672–82.
11. AOAC. Official methods of analysis of the Association of Official Analytical Chemists. 19th ed. Washington, USA: AOAC International (2012).
12. Akeson, WR, and Stahmann, MA. A pepsin pancreatin digest index of protein quality evaluation. J Nutr. (1964) 83:257–61. doi: 10.1093/jn/83.3.257
13. Liu, K. Soybean trypsin inhibitor assay: further improvement of the standard method approved and reapproved by American oil chemists’ society and American Association of Cereal Chemists International. J Am Oil Chem Soc. (2019) 96:635–45. doi: 10.1002/aocs.12205
14. BIS. New Delhi, India. IS 7837 (1975): Edible full-fat soya flour [FAD 16: Food grains, starches and ready to eat foods]. New Delhi: BIS (1975). 19 p.
15. Nosworthy, MG, Neufeld, J, Frohlich, P, Young, G, Malcolmson, L, and House, JD. Determination of the protein qality of cooked Canadian pulses. Food Sci Nutrit. (2017) 5:896–903. doi: 10.1002/fsn3.473
16. Ojo, OG, Akintayo, RO, Faleye, A, Shittu, TA, and Adeola, AA. Moisture-based shelf life estimation of ambient-stored grain flours. FUTA J Res Sci. (2017) 13:473–82.
17. St-Onge, L, Kwong, E, Sabsabi, M, and Vadas, EB. Quantitative analysis of pharmaceutical products by laser-induced breakdown spectroscopy. Spectrochim Acta B At Spectrosc. (2002) 57:1131–40. doi: 10.1016/S0584-8547(02)00062-9
18. Tian, SQ, Chen, ZC, and Wei, YC. Measurement of colour-grained wheat nutrient compounds and the application of combination technology in dough. J Cereal Sci. (2018) 83:63–7. doi: 10.1016/j.jcs.2018.07.018
19. Kajihausa, OE, Fasasi, RA, and Atolagbe, YM. Effect of different soaking time and boiling on the proximate composition and functional properties of sprouted sesame seed flour. Nigerian Food J. (2014) 32:8–15. doi: 10.1016/S0189-7241(15)30112-0
20. Chandra, S, Singh, S, and Kumari, D. Evaluation of functional properties of composite flours and sensorial attributes of composite flour biscuits. J Food Sci Technol. (2015) 52:3681–8. doi: 10.1007/s13197-014-1427-2
21. Sharma, S, Saxena, DC, and Riar, CS. Analysing the effect of germination on phenolics, dietary fibres, minerals and γ-amino butyric acid contents of barnyard millet (Echinochloa frumentaceae). Food Biosci. (2016) 13:60–8. doi: 10.1016/j.fbio.2015.12.007
22. Mbithi-Mwikya, S, Van Camp, J, Yiru, Y, and Huyghebaert, A. Nutrient and antinutrient changes in finger millet (Eleusine coracan) during sprouting. LWT-Food Sci Technol. (2000) 33:9–14. doi: 10.1006/fstl.1999.0605
23. Razavi, SN, Hojjatoleslamy, M, Molavi, H, and Boroujeni, LS. The effect of germinated lentil flour on the physicochemical and organoleptic characteristics of Sangak bread. J Culin Sci Technol. (2022) 20:253–65. doi: 10.1080/15428052.2020.1840473
24. Wintersohle, C, Arnold, SJ, Geis, HM, Keutgen, F, Etzbach, L, and Schweiggert-Weisz, U. Impact of short-term germination on dehulling efficiency, enzymatic activities, and chemical composition of mung bean seeds (Vigna radiata L.). Future Foods. (2024) 10:100416. doi: 10.1016/j.fufo.2024.100416
25. Ghavidel, RA, and Prakash, J. The impact of germination and dehulling on nutrients, antinutrients, in vitro iron and calcium bioavailability and in vitro starch and protein digestibility of some legume seeds. LWT-Food Sci Technol. (2007) 40:1292–9. doi: 10.1016/j.lwt.2006.08.002
26. Ongol, MP, Niyonzima, E, Gisanura, I, and Vasanthakaalam, H. Effect of germination and fermentation on nutrients in maize flour. Pakistan J Food Sci. (2013) 23:183–8.
27. Wu, J, Zhou, Q, Zhou, C, Cheng, KW, and Wang, M. Strategies to promote the dietary use of pigeon pea (Cajanus cajan L.) for human nutrition and health. Food Front. (2024) 5:1014–30. doi: 10.1002/fft2.381
28. Sharma, N, and Niranjan, K. Foxtail millet: properties, processing, health benefits, and uses. Food Rev Int. (2018) 34:329–63. doi: 10.1080/87559129.2017.1290103
29. Nkhata, SG, Ayua, E, Kamau, EH, and Shingiro, JB. Fermentation and germination improve nutritional value of cereals and legumes through activation of endogenous enzymes. Food Sci Nutrit. (2018) 6:2446–58. doi: 10.1002/fsn3.846
30. Srujana, MNS, Kumari, BA, Maheswari, KU, Devi, KS, Lakshmi, VV, and Suneetha, WJ. Glycemic index profiling of germinated quinoa (Chenopodium quinoa wild). J Pharmacognosy Phytochem. (2017) 6:2634–6.
31. Lappi, J, Selinheimo, E, Schwab, U, Katina, K, Lehtinen, P, Mykkänen, H, et al. Sourdough fermentation of wholemeal wheat bread increases solubility of arabinoxylan and protein and decreases postprandial glucose and insulin responses. J Cereal Sci. (2010) 51:152–8. doi: 10.1016/j.jcs.2009.11.006
32. Matella, NJ, Dolan, KD, Stoeckle, AW, Bennink, MR, Lee, YS, and Uebersax, MA. Use of hydration, germination, and α-galactosidase treatments to reduce oligosaccharides in dry beans. J Food Sci. (2005) 70:C203–7. doi: 10.1111/j.1365-2621.2005.tb07126.x
33. Shi, L, Mu, K, Arntfield, SD, and Nickerson, MT. Changes in levels of enzyme inhibitors during soaking and cooking for pulses available in Canada. J Food Sci Technol. (2017) 54:1014–22. doi: 10.1007/s13197-017-2519-6
34. Vadivel, V, and Pugalenthi, M. Effect of various processing methods on the levels of antinutritional constituents and protein digestibility of Mucuna pruriens (L.) DC. Var. utilis (wall. Ex Wight) baker ex Burck (velvet bean) seeds. J Food Biochem. (2008) 32:795–812. doi: 10.1111/j.1745-4514.2008.00199.x
35. Pradeep, PM, and Sreerama, YN. Impact of processing on the phenolic profiles of small millets: evaluation of their antioxidant and enzyme inhibitory properties associated with hyperglycemia. Food Chem. (2015) 169:455–63. doi: 10.1016/j.foodchem.2014.08.010
36. Shobana, S, Sreerama, YN, and Malleshi, NG. Composition and enzyme inhibitory properties of finger millet (Eleusine coracana L.) seed coat phenolics: mode of inhibition of α-glucosidase and pancreatic amylase. Food Chem. (2009) 115:1268–73. doi: 10.1016/j.foodchem.2009.01.042
37. Hua, F, Zhou, P, Wu, HY, Chu, GX, Xie, ZW, and Bao, GH. Inhibition of α-glucosidase and α-amylase by flavonoid glycosides from Lu'an GuaPian tea: molecular docking and interaction mechanism. Food Funct. (2018) 9:4173–83. doi: 10.1039/c8fo00562a
38. Ofosu, FK, Elahi, F, Daliri, EBM, Chelliah, R, Ham, HJ, Kim, JH, et al. Phenolic profile, antioxidant, and antidiabetic potential exerted by millet grain varieties. Antioxidants. (2020) 9:254. doi: 10.3390/antiox9030254
39. Welsch, CA, Lachance, PA, and Wasserman, BP. Dietary phenolic compounds: inhibition of Na+−dependent D-glucose uptake in rat intestinal brush border membrane vesicles. J Nutr. (1989) 119:1698–704. doi: 10.1093/jn/119.11.1698
40. Zahir, M, Fogliano, V, and Capuano, E. Soybean germination limits the role of cell wall integrity in controlling protein physicochemical changes during cooking and improves protein digestibility. Food Res Int. (2021) 143:110254. doi: 10.1016/j.foodres.2021.110254
41. Mubarak, AE. Nutritional composition and antinutritional factors of mung bean seeds (Phaseolus aureus) as affected by some home traditional processes. Food Chem. (2005) 89:489–95. doi: 10.1016/j.foodchem.2004.01.007
Keywords: diabetes, soaking, germination, glycemic index, enzyme inhibitory activity, protein digestibility
Citation: Goel K, Kushwaha A, Dutta A, Sharma SK, Shahi NC, Joshi DC, Bhartiya A and Gupta P (2025) Impact of bio-processing treatments on the nutritional and anti-diabetic enzyme inhibitory properties of black wheat, barnyard millet, and black soybean. Front. Nutr. 12:1554993. doi: 10.3389/fnut.2025.1554993
Edited by:
Marco Montemurro, National Research Council (CNR), ItalyReviewed by:
Sumer Pal Singh, Indian Agricultural Research Institute (ICAR), IndiaAjmer Singh Grewal, Guru Gobind Singh College of Pharmacy, India
Jie Tu, Jiangsu University of Science and Technology, China
Copyright © 2025 Goel, Kushwaha, Dutta, Sharma, Shahi, Joshi, Bhartiya and Gupta. This is an open-access article distributed under the terms of the Creative Commons Attribution License (CC BY). The use, distribution or reproduction in other forums is permitted, provided the original author(s) and the copyright owner(s) are credited and that the original publication in this journal is cited, in accordance with accepted academic practice. No use, distribution or reproduction is permitted which does not comply with these terms.
*Correspondence: Krati Goel, Z29lbGtyYXRpLjIyQGdtYWlsLmNvbQ==