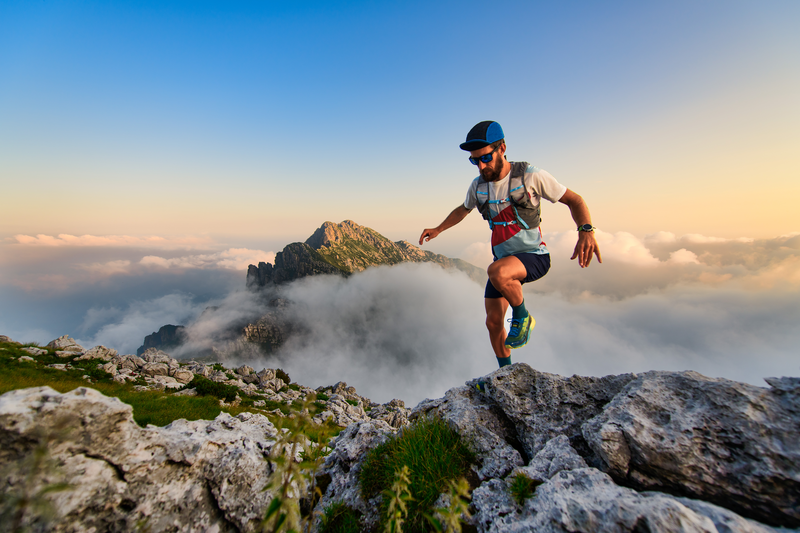
94% of researchers rate our articles as excellent or good
Learn more about the work of our research integrity team to safeguard the quality of each article we publish.
Find out more
ORIGINAL RESEARCH article
Front. Nutr.
Sec. Nutritional Immunology
Volume 12 - 2025 | doi: 10.3389/fnut.2025.1550880
This article is part of the Research Topic Health Effects of Natural Compounds from Plant View all 4 articles
The final, formatted version of the article will be published soon.
You have multiple emails registered with Frontiers:
Please enter your email address:
If you already have an account, please login
You don't have a Frontiers account ? You can register here
Functional constipation is a common gastrointestinal health problem among the ageing people. Dietary fiber supplementation is widely recognized as a first-line strategy for constipation. However, the effectiveness of dietary fiber in practical applications is still not satisfactory, and dietary fibers from different sources are believed to possess varying physiological activities in alleviating constipation.Burdock root is a vegetable rich in dietary fiber. In this study, functional constipation in the aged rats was induced by loperamide. 3 mg/kg•bw•day and 1.5 mg/kg•bw•day doses of dietary fiber from burdock root were used to intervene on functional constipation in aged rats. It was found that dietary fiber from burdock root increased intestinal motility to ameliorate functional constipation in ageing rats, along with potential mechanisms including repairing intestinal barrier, regulating intestinal hormones, anti-inflammation and anti-oxidation. Our findings provide a theoretical basis for the potential mechanism of burdock root dietary fiber to ameliorate functional constipation, it is expected to be a natural functional food to against functional constipation in the ageing.
Keywords: Dietary Fiber, Burdock root, Functional constipation, the Ageing, Nutritional strategy
Received: 03 Jan 2025; Accepted: 07 Mar 2025.
Copyright: © 2025 Mo, Ma, Li, Song, Song and Wang. This is an open-access article distributed under the terms of the Creative Commons Attribution License (CC BY). The use, distribution or reproduction in other forums is permitted, provided the original author(s) or licensor are credited and that the original publication in this journal is cited, in accordance with accepted academic practice. No use, distribution or reproduction is permitted which does not comply with these terms.
* Correspondence:
Jiangfeng Song, Jiangsu Academy of Agricultural Sciences (JAAS), Nanjing, 210014, Jiangsu Province, China
Qiqi Song, Suzhou Vocational Health College, Suzhou, Jiangsu Province, China
Ling Wang, The First Affiliated Hospital of Soochow University, Suzhou, 215006, Jiangsu Province, China
Disclaimer: All claims expressed in this article are solely those of the authors and do not necessarily represent those of their affiliated organizations, or those of the publisher, the editors and the reviewers. Any product that may be evaluated in this article or claim that may be made by its manufacturer is not guaranteed or endorsed by the publisher.
Research integrity at Frontiers
Learn more about the work of our research integrity team to safeguard the quality of each article we publish.