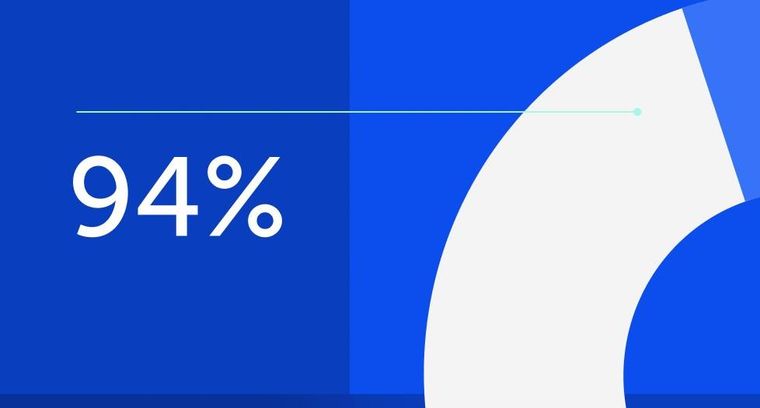
94% of researchers rate our articles as excellent or good
Learn more about the work of our research integrity team to safeguard the quality of each article we publish.
Find out more
ORIGINAL RESEARCH article
Front. Nutr., 27 March 2025
Sec. Nutrition and Metabolism
Volume 12 - 2025 | https://doi.org/10.3389/fnut.2025.1529332
This article is part of the Research TopicNutrition, Inflammation and Oxidative Stress in Obstetrics and GynecologyView all 12 articles
Objective: This study sought to investigate the association between metabolic syndrome (MetS) and Composite Dietary Antioxidant Index (CDAI) in females, with the goal of informing evidence-based prevention and clinical management strategies for MetS.
Methods: The 2011–2016 National Health and Nutrition Examination Survey (NHANES) recruited a total of 2,790 female participants and screened 1,562 participants for estrogen non-deficiency. The diagnosis of MetS was based on criteria set by the National Cholesterol Education Program-Adult Treatment Panel III. The CDAI was calculated according to the intake of 10 dietary antioxidants. Multivariable logistic regression was performed to investigate the relationship between the CDAI and MetS in females. We also performed restricted cubic splines, two-piecewise linear regression, and subgroup analysis in further analysis.
Results: Our analyses demonstrated a significant inverse association between the Composite Dietary Antioxidant Index (CDAI) and metabolic syndrome (MetS) prevalence in females. Restricted cubic spline analysis indicated a linear dose–response relationship (p for linearity = 0.029), with two-piecewise linear regression analysis revealed an inflection point at 1.99. Below 1.99, each unit increase in the CDAI was associated with a 2% reduction in the risk of MetS in females; above 1.99, the risk reduction was 1%. Participants without MetS were significantly younger than those with MetS (43.49 ± 16.04 vs. 54.77 ± 15.52 years, p < 0.001). Notably, estrogen levels also were negatively correlated with the prevalence of MetS. Subgroup analysis revealed that the relationship between the CDAI and MetS remained consistent across all subgroups.
Conclusion: In the female population, CDAI levels exhibited an inverse relationship with the prevalence of metabolic syndrome, and estrogen levels demonstrated a negative correlation with its incidence.
Metabolic syndrome (MetS) is a condition characterized by a clustering of metabolic risk factors. It is defined by the World Health Organization as a pathological condition characterized by abdominal obesity, insulin resistance, hypertension, and hyperlipidemia (1). Given its high prevalence and severe consequences, MetS has become a global problem, placing a huge economic burden on societies and health systems in the future (2–5).
However, women with MetS bear a greater health burden. Studies show that females have a higher prevalence of MetS than men (5), and female patients tend to experience more severe symptoms than males (6, 7). Previous research suggests that MetS in middle-aged females may contribute to or exacerbate pain, sleep disturbances, sexual dysfunction, and mood alterations, likely due to aging and chronic inflammation (8). A systematic review and meta-analysis further revealed that MetS in females is associated with an increased risk of several malignancies, including endometrial, pancreatic, and breast cancers. The strongest associations were seen in sex-specific cancers like endometrial cancer and postmenopausal breast cancer (6). Remarkably, postmenopausal females with MetS have a twofold higher risk of developing breast cancer than those without MetS (8).
Evidence indicates that females with MetS experience more severe symptoms than men, possibly due to sex-related hormonal factors. Estrogen plays a crucial role in modulating inflammation and metabolic homeostasis in females (9, 10). It regulates insulin resistance (11), energy metabolism (12), and lipid metabolism (13, 14). The hormone exhibits dual functionality in oxidative stress regulation, providing antioxidant protection through receptor-mediated mechanisms (15), while also orchestrating cellular defense systems against oxidative damage (16). Pathologically, the chronic inflammation and oxidative stress characteristic of MetS are well-established contributors to carcinogenesis (8, 15). Recent studies suggest that ERα-mediated mitochondrial energy regulation represents a key pathway underlying estrogen’s metabolic protective effects (16). However, the postmenopausal decline in estrogen levels promotes macrophage infiltration in adipose tissue, worsening insulin resistance via TNF-α/IL-6-JNK pathway activation (17).
Estrogen fluctuations, mediated through cyclic variations in nuclear receptor ERRα-regulated mitochondrial biogenesis (18), constitute an inherent physiological characteristic in females. Targeting the menopausal decline in estrogen through dual strategies—suppressing NADPH oxidase activity to mitigate oxidative stress while enhancing endogenous antioxidant defenses (e.g., superoxide dismutase (SOD) and catalase)—may effectively reduce the risk of MetS (19). Therefore, antioxidant protection may yield significant benefits for females with MetS, offering greater preventive value than treatment alone. Mechanistically, oxidative stress drives MetS pathogenesis via NF-κB-mediated inflammation (20), insulin signaling disruption, and adipocyte dysfunction (21). Several studies have confirmed the role of dietary antioxidants in counteracting oxidative stress (22–25). For instance, serum levels of carotenoids, particularly α and β-carotene, as well as retinyl esters, exhibit an inversely association with MetS (26). Additionally, abnormal vitamin A metabolism contributes to damage and plays a key role in MetS in a gut microbiota-dependent manner (27) while dietary vitamin E levels are inversely correlated with MetS (28, 29).
Therefore, mitigating oxidative stress may represent a viable preventive and therapeutic approach for managing metabolic syndrome (MetS) in female populations. Notably, while previous investigations predominantly focused on isolated antioxidant components, emerging evidence underscores the critical role of holistic dietary patterns in modulating oxidative-inflammatory pathways (30). The Comprehensive Dietary Antioxidant Index (CDAI), a validated metric reflecting synergistic antioxidant capacity, was developed based on its cumulative inhibitory effects on pro-inflammatory mediators, including tumor necrosis factor-alpha (TNF-α) and interleukin-1 beta (IL-1β). Its integrates quantitative assessments of multiple dietary antioxidants—vitamins A, C, and E, alongside manganese (Mn), selenium (Se), and zinc (Zn), etc., thereby providing a comprehensive characterization of individual antioxidant profiles (31), and has been applied in several studies (32–34).
Despite emerging evidence suggesting a non-linear association between MetS and the CDAI, critical knowledge gaps remain (35). Existing studies only found sex-specific differences in association between MetS and CDAI (36), and the stratified regression analyses indicate CDAI-MetS associations may be unique to female populations. However, the biological mechanisms underlying this sexual dimorphism, particularly the potential mediating role of estrogen in modulating antioxidant-metabolic interactions, remain inadequately explored. Since antioxidant supplements have shown no effect on MetS prevention, this study focused on dietary antioxidants (excluding supplements) to better reflect real-world nutritional exposures. In summary, this study aimed to quantify the CDAI-MetS association in females and investigate the role of estrogen in this relationship.
All participant information and relevant data for this study were obtained from the National Health and Nutrition Examination Survey (NHANES) database, which is conducted by the National Center for Health Statistics, a division of the Centers for Disease Control and Prevention. For this study, we downloaded consecutive datasets from 2011 to 2016 (Figure 1), which were analyzed to accurately assess the relationship between a healthy CDAI index and MetS.
The NHANES database collects participants’ food intake over two consecutive days using 24-h dietary recall interviews. The first interview was conducted face to face, and the second was done over the phone 3–10 days later. The CDAI for all participants was calculated using the method recommended by Wright (Equation 1), incorporating 10 vitamins and minerals from food sources (vitamins A, C, E, selenium, zinc, alpha-carotene, beta-carotene, lycopene, lutein, and zeaxanthin, with lutein and zeaxanthin counting as a single metric). The intake of each antioxidant was standardized by subtracting the mean intake and then dividing by the standard deviation (where x represents the intake of individual dietary antioxidants and represents the average intake of each component; SD is the standard deviation of the mean).
In this study, we used the National Cholesterol Education Program-Adult Treatment Panel III (NCEP-ATP III) for the diagnosis of MetS. The NCEP-ATP III criteria are based on five measures: abdominal obesity, elevated triglycerides, lowered high-density lipoprotein (HDL) cholesterol, elevated blood pressure, and elevated fasting blood glucose. MetS is diagnosed when three or more of the following five criteria are met: (1) waist circumference ≥102 cm in men or ≥88 cm in females; (2) serum triglyceride ≥150 mg/dL; (3) serum HDL cholesterol <40 mg/dL in men or <50 mg/dL in females; (4) fasting blood glucose ≥100 mg/dL or use of hypoglycemic drugs; (5) blood pressure ≥130/85 mmHg or receiving relevant medication.
To assess the impact of potential confounders, several important covariates were selected, including age, race and ethnicity, education level, marital status, drinking, smoking, body mass index (BMI), diabetes, and hypertension. Categorical variables included race and ethnicity (Non-Hispanic White people, Non-Hispanic Black people, Mexican American, and Other), education level (High school, Above high school, and Other), marital status (Married, Unmarried, and Other), drinking (No/Unknown, Yes), smoking (No/Unknown, Yes), diabetes (No/Unknown, Yes/Borderline), and hypertension (No/Unknown, Yes).
Demographic and clinical characteristics of participants were collected, with continuous variables described as (mean ± SD) and categorical variables expressed as number and percentage. Chi-squared tests were used to compare baseline characteristics of categorical variables. Logistic regression models were applied to investigate the relationship between CDAI and MetS in females. Model 1 was a rough model without no adjustment for any covariates. Model 2 was further adjusted for age, race and ethnicity, and education level. Model 3 was a fully adjusted model, further adjusting for alcohol consumption, smoking status, BMI, diabetes, and hypertension. To verify the robustness of the study results, a sensitivity analysis (Model 4) was conducted by excluding diabetes and hypertension to assess the impact of adjusting for these variables on the association between CDAI and MetS. A restricted cubic spline was then performed to explore the nonlinear relationship between the CDAI and MetS in females, and two-piecewise linear regression was used to calculate the inflection point. Finally, subgroup analysis was conducted to confirm the consistency and stability of the study results in each subgroup. All statistical analyses were conducted using R software version 4.2.3.1 Two-sided p < 0.05 was considered statistically significant.
In total, 2,790 female participants were included in this study and grouped according to the quartile distribution of CDAI. Significant trends were observed in race and ethnicity, education level, marital status, smoking status, and BMI with changes in CDAI quartiles. Additionally, the prevalence of MetS exhibited a significant downward trend with increased the CDAI quartiles (p < 0.001) (Table 1).
Table 1. Baseline analyses based on CDAI quartiles for general female adult population, NHANES 2011–2016.
Multivariate logistic regression models were established to examine the relationship between the CDAI and MetS. Three models were constructed. Model 1 was not adjusted for any covariates. Model 2 was adjusted for age, race and ethnicity, and education level. Model 3 was further adjusted for alcohol consumption, smoking status, BMI, diabetes, and hypertension. In both Models 1 and 2, the CDAI was associated with a reduced prevalence of MetS, whether treated as a continuous or categorical variable. Even after adjusting for all confounding variables in Model 3, the protective effect of the CDAI (continuous) on MetS remained significant (odds ratio [OR] [95% confidence interval CI] = 0.96 [0.94, 0.99], p = 0.009). Compared with Q1, the CDAI in Q4 was associated with a 28% reduction in the prevalence of MetS (p = 0.017; Table 2). In the sensitivity analysis (Model 4, excluding diabetes and hypertension), the negative association between CDAI and MetS remained statistically significant (OR [95% CI] = 0.97 [0.94, 0.99], p = 0.009). The effect estimates for CDAI quartiles were comparable to those in Model 3, indicating that the association between CDAI and MetS remains robust regardless of whether diabetes and hypertension are adjusted for. In sum, the higher CDAI was a protective factor in MetS.
Table 2. Multivariable-adjusted logistic regression analysis of the relationship between CDAI and prevalence of MetS in American adult women, NHANES 2011–2016.
The relationship between components of the CDAI and MetS was also examined. After adjusting for all confounders, no components (vitamins A, C, E, selenium, zinc, and carotenoids: α-carotene, β-carotene, lycopene, lutein, and zeaxanthin) were independently associated with the presence of MetS (p > 0.05; Table 3).
Table 3. Relationship between individual dietary antioxidants and prevalence of MetS in American adult women, NHANES 2011–2016.
A restricted cubic spline was used to analyze whether there was a nonlinear correlation between the CDAI and MetS. After adjusting for age, race and ethnicity, education level, alcohol consumption, smoking status, BMI, diabetes, and hypertension, the results showed that the relationship between the CDAI and MetS was linear (p for linearity = 0.0292, p nonlinearity = 0.569) (Figure 2).
The threshold effect of the CDAI on MetS in females was further analyzed using two-piecewise linear regression. The results showed that the inflection point of two-piecewise linear regression was 1.99, with a significant correlation between the CDAI and MetS in females (p < 0.05). When the CDAI was below 1.99, the risk of MetS in females decreased by 2% for each unit increase; with CDAI scores above 1.99, the risk reduction was 1% (Figure 3 and Table 4).
The results of subgroup analysis showed that the relationship between the CDAI and MetS was consistent and stable across all subgroups (Figure 4). None of the factors significantly affected the association (p > 0.05). Moreover, this association was found in all subgroups except for non-Hispanic Black people, participants with more than a high school education, unmarried participants, those with borderline or diagnosed diabetes, and participants with hypertension.
In total, 1,562 participants (mean age: 47.23 years) with data for estrogen levels were screened from the 2,790 included participants. We explored whether there was a relationship between estrogen and MetS. In total, 559 participants were diagnosed with MetS, resulting in a prevalence rate of 35.79% (Table 5). The results indicated that females without MetS were younger (43.49 ± 16.04 years) and had lower BMI (28.29 ± 7.23 kg/m2) than those who had MetS (age: 54.77 ± 15.52 years, BMI: 34.37 ± 7.57 kg/m2). Additionally, females without MetS had higher CDAI scores. When estrogen was divided into tertiles, there was a significant downward trend in the prevalence of MetS with increasing estrogen tertiles (p < 0.001; Figure 5).
Table 5. Demographic and clinical characteristics of general female adult population (with estrogen) in the United States.
To investigate the role of estrogen in the association between CDAI and the risk of MetS in females, we performed further logistic regression analyses. Model 1 showed that both high levels of CDAI and high levels of estrogen were protective against MetS (OR [95% CI] 0.58 [0.42, 0.78], p < 0.001; OR [95% CI] = 0.30 [0.22, 0.38], p < 0.001, respectively) (Figure 6A). After adjusting for all covariates in Model 2, the protective effect of estrogen remained significant (OR [95% CI] 0.63 [0.43, 0.93], p = 0.021) (Figure 6B).
Figure 6. Role of estrogen in the association between CDAI and risk of MetS in women. (A) Model 1: Logistic regression analysis of CDAI and estrogen. (B) Model 2: Model 1 with additional covariates, including age, race and ethnicity, education, marital status, smoking, drinking, diabetes, and hypertension.
Global epidemiological data reveal a striking female preponderance in MetS, affecting over 500 million females worldwide (4, 5, 37). This disparity may stem from estrogen’s role in redox regulation, as estrogen deficiency elevates oxidative stress and inflammation—canonical drivers of metabolic dysregulation (38–40). More critically, estrogen deficiency disrupts the estrogen-antioxidant crosstalk, a novel mechanism identified in this study. Our investigation elucidates a critical interplay between CDAI and estrogenic status in modulating MetS risk among females. We identified a robust inverse association between CDAI and MetS prevalence, characterized by a biphasic dose–response relationship with an inflection point at CDAI = 1.99. Crucially, estrogen status significantly modified this relationship, with higher estrogen levels enhancing the protective capacity of CDAI. These findings underscore the necessity of optimizing antioxidant intake during premenopausal phase to maximize metabolic protection.
MetS is characterized by a heightened pro-oxidative and pro-inflammation (41). Oxidative stress arises owing to an imbalance between the synthesis of antioxidants and pro-oxidants, causing harm to tissues and organs. It primarily results from excessive levels of reactive oxygen species (ROS), which cause damage to damage macromolecules such as DNA, lipids, proteins, and carbohydrates (42, 43). As an extrinsic factor, diet can affect the plasma redox status by reducing ROS and reactive nitrogen species (44). The CDAI serves as an integrative measure of dietary antioxidant vitamins/minerals, indicating the antioxidant potential of individual dietary sources. We analyzed both the individual components of the CDAI and the index as a whole. In separate analyses, no single dietary component was significantly associated with MetS prevalence (p > 0.05), a finding that contrasts with previous studies (45). This may be due to the differences in the cohort samples of our study, which was conducted on females only, and previous population-wide studies. However, when considering the CDAI as a whole, we observed a significant downward trend in MetS prevalence across increasing CDAI quartiles (p < 0.001). The risk of MetS in females decreased significantly with higher CDAI levels, and females in the highest quartile (OR [95% CI] = 0.62[0.49, 0.77], p < 0.001) of the CDAI had an approximately 28% lower risk of MetS than those in the lowest quartile. This is consistent with previous population-wide studies, and such an association was also present in female patients (35, 36, 44). Our analyses revealed a nonlinear association between the CDAI and MetS, with an inflection point identified at 1.99 through threshold effect modeling. Below this critical threshold, each unit increase in CDAI conferred a 2.0% absolute risk reduction (p < 0.001), whereas supra-threshold increments attenuated this protective to 1.0% per unit (p = 0.001), suggesting a biological ceiling effect of dietary antioxidants. In previous studies, a dose–response trend similar to that in our study was observed (36). Therefore, we hypothesized that a high intake of dietary antioxidants and phytochemicals may reduce the risk of developing MetS in females because the combined intake of dietary antioxidants reduces oxidative stress. Intake of exogenous antioxidants can improve patient quality of life by preventing oxidative imbalance and maintaining a stable biochemical redox state (46), thereby avoiding the deleterious effects of chronic oxidative stress in the human body (47). According to a previous study, daily intake of antioxidants can enhance antioxidant defense and mitigate oxidative stress by increasing plasma antioxidant levels (48).
Oxidative stress exhibits sex-related differences, with estrogen conferring protection against its detrimental effects in females (49, 50). This hormonal regulation plays a pivotal role in modulating the protective association between dietary antioxidants (assessed via CDAI) and MetS in females. Estrogen regulates redox homeostasis through multiple molecular mechanisms, including: upregulating the expression of endogenous antioxidant enzymes (51), binding to mitochondrial estrogen receptors to enhance antioxidant defense (52), and suppressing reactive oxygen species (ROS) generation by inhibiting NADPH oxidase activity (52). Epidemiological studies indicate that the prevalence of MetS increases with age (53), a phenomenon largely driven by age-related exacerbation of oxidative stress driven by either excessive ROS production or impaired antioxidant systems (54).
In our study, the mean age of females without MetS was 43.49 ± 16.04 years, significantly lower than that of females with MetS (54.77 ± 15.52 years; p < 0.001). This age disparity aligns with epidemiological evidence showing a higher prevalence of MetS in postmenopausal populations (53, 55). Mechanistically, menopause typically occurs between 45 and 55 years (55), and the subsequent decline in estrogen levels exacerbates oxidative stress and systemic inflammation (38, 39). Estrogen deficiency disrupts redox homeostasis, leading to progressive accumulation of oxidative damage markers (56, 57), which in turn promotes endothelial dysfunction—a key contributor of insulin resistance, hypertension, and dyslipidemia (45, 57). These pathological alterations collectively drive MetS development in aging females.
Meanwhile, our study confirmed this finding, demonstrating a significant downward trend in MetS prevalence with increasing estrogen levels. Regression analysis further revealed that high levels of estrogen exerted a protective effect against MetS (OR[95% CI] = 0.30[0.22, 0.38], p < 0.001). This protective role may be attributed to the loss of estrogen is associated with a diminished defense against oxidative stress (58). The oxidative stress has a pivotal role in some components of MetS, including abdominal obesity, hypertension, insulin resistance, and dyslipidemia (44, 59–61).
This was the first large-sample study to investigate the association between the CDAI and MetS in females, while also considered the role of estrogen. Our findings provide epidemiological evidence supporting the implementation of preemptive nutritional strategies aimed at optimizing composite dietary antioxidant optimization during the estrogen depletion phase (specifically the premenopausal transition window) as a prophylactic measure against MetS development. A public health priority for the prevention of disease is consuming an optimal diet that can reduce or suppress inflammation owing to its composition and thereby modulate the risk of various diseases (62). As a new paradigm in the prevention and treatment of MetS, dietary interventions should include advice on antioxidant-rich diets given by nutritional professionals as well as increased promotion of these diets and specific antioxidant dietary modifications for menopausal females. Increased dietary intake of vitamins A, C, and E together with selenium, zinc, and carotenoid-rich foods may improve the current status of MetS among females globally.
This study benefits from methodological rigor through utilization of the nationally representative NHANES sampling framework and comprehensive adjustments for established demographic, anthropometric, and metabolic covariates, enhancing internal validity. Notwithstanding these strengths, several methodological constraints warrant consideration: (1) The observational cross-sectional design precludes temporal sequence determination and causal inference; (2) Sex-specific analytic focus limits external validity to male populations; (3) The moderate sample size relative to population-level epidemiological standards constrains statistical power for detecting modest effect sizes; (4) Potential residual confounding persists despite multivariable adjustments, including unmeasured lifestyle determinants (e.g., chrononutrition patterns, physical activity gradients) and epigenetic regulatory mechanisms influencing redox homeostasis.
The datasets presented in this study can be found in online repositories. The names of the repository/repositories and accession number(s) can be found in the article/supplementary material.
The studies involving humans were approved by National Center for Health Statistics. The studies were conducted in accordance with the local legislation and institutional requirements. The participants provided their written informed consent to participate in this study. Written informed consent was obtained from the individual(s) for the publication of any potentially identifiable images or data included in this article.
WL: Conceptualization, Data curation, Formal analysis, Investigation, Methodology, Project administration, Software, Supervision, Validation, Writing – original draft, Writing – review & editing. YX: Conceptualization, Data curation, Formal analysis, Investigation, Methodology, Project administration, Software, Supervision, Validation, Writing – original draft, Writing – review & editing. LX: Investigation, Methodology, Project administration, Supervision, Writing – original draft, Writing – review & editing. KL: Formal analysis, Methodology, Project administration, Resources, Validation, Writing – original draft, Writing – review & editing. QL: Conceptualization, Funding acquisition, Investigation, Project administration, Resources, Visualization, Writing – original draft, Writing – review & editing.
The author(s) declare that financial support was received for the research and/or publication of this article. This work was supported by 2024 Sichuan Provincial Science and Technology Achievement Transfer and Transformation Guidance Program (No. 24ZHSF0072) and 2025 Sichuan Provincial Key Research and Development Program (No. 25QYCX0359), Sichuan, China.
We thank LetPub (www.letpub.com.cn) for its linguistic assistance during the preparation of this manuscript.
The authors declare that the research was conducted in the absence of any commercial or financial relationships that could be construed as a potential conflict of interest.
The author(s) declare that no Gen AI was used in the creation of this manuscript.
All claims expressed in this article are solely those of the authors and do not necessarily represent those of their affiliated organizations, or those of the publisher, the editors and the reviewers. Any product that may be evaluated in this article, or claim that may be made by its manufacturer, is not guaranteed or endorsed by the publisher.
1. Huang, PL. A comprehensive definition for metabolic syndrome. Dis Model Mech. (2009) 2:231–7. doi: 10.1242/dmm.001180
2. Gupta, A, and Gupta, V. Metabolic syndrome: what are the risks for humans? Biosci Trends. (2010) 4:204–12.
3. Cano-Ibáñez, N, Gea, A, Martínez-González, MA, Salas-Salvadó, J, Corella, D, Zomeño, MD, et al. Dietary diversity and nutritional adequacy among an older Spanish population with metabolic syndrome in the Predimed-plus study: a cross-sectional analysis. Nutrients. (2019) 11:958. doi: 10.3390/nu11050958
4. Saklayen, MG. The global epidemic of the metabolic syndrome. Curr Hypertens Rep. (2018) 20:12. doi: 10.1007/s11906-018-0812-z
5. Delavari, A, Forouzanfar, MH, Alikhani, S, Sharifian, A, and Kelishadi, R. First nationwide study of the prevalence of the metabolic syndrome and optimal cutoff points of waist circumference in the Middle East: the national survey of risk factors for noncommunicable diseases of Iran. Diabetes Care. (2009) 32:1092–7. doi: 10.2337/dc08-1800
6. Esposito, K, Chiodini, P, Colao, A, Lenzi, A, and Giugliano, D. Metabolic syndrome and risk of cancer: a systematic review and meta-analysis. Diabetes Care. (2012) 35:2402–11. doi: 10.2337/dc12-0336
7. Min, SH, Yang, Q, Min, SW, Ledbetter, L, Docherty, SL, Im, EO, et al. Are there differences in symptoms experienced by midlife climacteric women with and without metabolic syndrome? A scoping review. Women's Health (Lond Engl). (2022) 18:17455057221083817. doi: 10.1177/17455057221083817
8. Palmiero, P, Maiello, M, Cecere, A, and Ciccone, MM. Metabolic syndrome and breast cancer: a dangerous association for postmenopausal women. Acta Biomed. (2021) 92:e2021177. doi: 10.23750/abm.v92i3.11335
9. Monteiro, R, Teixeira, D, and Calhau, C. Estrogen signaling in metabolic inflammation. Mediat Inflamm. (2014) 2014:615917:1–20. doi: 10.1155/2014/615917
10. De Rooij, SR, Nijpels, G, Nilsson, PM, Nolan, JJ, Gabriel, R, Bobbioni-Harsch, E, et al. Low-grade chronic inflammation in the relationship between insulin sensitivity and cardiovascular disease (Risc) population: associations with insulin resistance and cardiometabolic risk profile. Diabetes Care. (2009) 32:1295–301. doi: 10.2337/dc08-1795
11. De Paoli, M, Zakharia, A, and Werstuck, GH. The role of estrogen in insulin resistance: a review of clinical and preclinical data. Am J Pathol. (2021) 191:1490–8. doi: 10.1016/j.ajpath.2021.05.011
12. Mauvais-Jarvis, F, Clegg, DJ, and Hevener, AL. The role of estrogens in control of energy balance and glucose homeostasis. Endocr Rev. (2013) 34:309–38. doi: 10.1210/er.2012-1055
13. Katzer, K, Hill, JL, Mciver, KB, and Foster, MT. Lipedema and the potential role of estrogen in excessive adipose tissue accumulation. Int J Mol Sci. (2021) 22:11720. doi: 10.3390/ijms222111720
14. Ko, SH, and Kim, HS. Menopause-associated lipid metabolic disorders and foods beneficial for postmenopausal women. Nutrients. (2020) 12:202. doi: 10.3390/nu12010202
15. Mendonça, FM, de Sousa, FR, Barbosa, AL, Martins, SC, Araújo, RL, Soares, R, et al. Metabolic syndrome and risk of cancer: which link? Metab Clin Exp. (2015) 64:182–9. doi: 10.1016/j.metabol.2014.10.008
16. Liu, Y, Dong, Y, Jiang, Y, Han, S, Liu, X, Xu, X, et al. Caloric restriction prevents inheritance of polycystic ovary syndrome through oocyte-mediated DNA methylation reprogramming. Cell Metab. (2025). ahead of print. doi: 10.1016/j.cmet.2025.01.014
17. Zhang, Y, Hu, S, Han, S, Liu, C, Liang, X, Li, Y, et al. Transgenerational inheritance of diabetes susceptibility in male offspring with maternal androgen exposure. Cell Discov. (2025) 11:14. doi: 10.1038/s41421-025-00769-1
18. Klinge, CM. Estrogens regulate life and death in mitochondria. J Bioenerg Biomembr. (2017) 49:307–24. doi: 10.1007/s10863-017-9704-1
19. Reis, J, Gorgulla, C, Massari, M, Marchese, S, Valente, S, Noce, B, et al. Targeting ROS production through inhibition of NADPH oxidases. Nat Chem Biol. (2023) 19:1540–50. doi: 10.1038/s41589-023-01457-5
20. Vona, R, Gambardella, L, Cittadini, C, Straface, E, and Pietraforte, D. Biomarkers of oxidative stress in metabolic syndrome and associated diseases. Oxidative Med Cell Longev. (2019) 2019:1–19. doi: 10.1155/2019/8267234
21. Franco, C, Sciatti, E, Favero, G, Bonomini, F, Vizzardi, E, and Rezzani, R. Essential hypertension and oxidative stress: novel future perspectives. Int J Mol Sci. (2022) 23:14489. doi: 10.3390/ijms232214489
22. Panchal, SK, Wanyonyi, S, and Brown, L. Selenium, vanadium, and chromium as micronutrients to improve metabolic syndrome. Curr Hypertens Rep. (2017) 19:10. doi: 10.1007/s11906-017-0701-x
23. Retondario, A, Fernandes, R, Rockenbach, G, Alves, MA, Bricarello, LP, Trindade, EBSM, et al. Selenium intake and metabolic syndrome: a systematic review. Clin Nutr (Edinburgh, Scotland). (2019) 38:603–14. doi: 10.1016/j.clnu.2018.02.021
24. Santa, K, Kumazawa, Y, and Nagaoka, I. Prevention of metabolic syndrome by phytochemicals and vitamin D. Int J Mol Sci. (2023) 24:2627. doi: 10.3390/ijms24032627
25. Liao, S, Omage, SO, Börmel, L, Kluge, S, Schubert, M, Wallert, M, et al. Vitamin E and metabolic health: relevance of interactions with other micronutrients. Antioxidants (Basel, Switzerland). (2022) 11:1785. doi: 10.3390/antiox11091785
26. Beydoun, MA, Chen, X, Jha, K, Beydoun, HA, Zonderman, AB, and Canas, JA. Carotenoids, vitamin A, and their association with the metabolic syndrome: a systematic review and meta-analysis. Nutr Rev. (2019) 77:32–45. doi: 10.1093/nutrit/nuy044
27. Zhang, T, Sun, P, Geng, Q, Fan, H, Gong, Y, Hu, Y, et al. Disrupted spermatogenesis in a metabolic syndrome model: the role of vitamin A metabolism in the gut-testis axis. Gut. (2022) 71:78–87. doi: 10.1136/gutjnl-2020-323347
28. Guo, H, Ding, J, Liu, Q, Li, Y, Liang, J, and Zhang, Y. Vitamin C and metabolic syndrome: a meta-analysis of observational studies. Front Nutr. (2021) 8:728880. doi: 10.3389/fnut.2021.728880
29. Schleicher, RL, Carroll, MD, Ford, ES, and Lacher, DA. Serum vitamin C and the prevalence of vitamin C deficiency in the United States: 2003-2004 National Health and Nutrition Examination Survey (NHANES). Am J Clin Nutr. (2009) 90:1252–63. doi: 10.3945/ajcn.2008.27016
30. Cespedes, EM, and Hu, FB. Dietary patterns: from nutritional epidemiologic analysis to national guidelines. Am J Clin Nutr. (2015) 101:899–900. doi: 10.3945/ajcn.115.110213
31. Yu, YC, Paragomi, P, Wang, R, Jin, A, Schoen, RE, Sheng, LT, et al. Composite dietary antioxidant index and the risk of colorectal cancer: findings from the Singapore Chinese health study. Int J Cancer. (2022) 150:1599–608. doi: 10.1002/ijc.33925
32. Wu, M, Si, J, Liu, Y, Kang, L, and Xu, B. Association between composite dietary antioxidant index and hypertension: insights from NHANES. Clin Exp Hypertens. (2023) 45:2233712. doi: 10.1080/10641963.2023.2233712
33. Wright, ME, Mayne, ST, Stolzenberg-Solomon, RZ, Li, Z, Pietinen, P, Taylor, PR, et al. Development of a comprehensive dietary antioxidant index and application to lung cancer risk in a cohort of male smokers. Am J Epidemiol. (2004) 160:68–76. doi: 10.1093/aje/kwh173
34. Zhao, L, Sun, Y, Cao, R, Wu, X, Huang, T, and Peng, W. Non-linear association between composite dietary antioxidant index and depression. Front Public Health. (2022) 10:988727. doi: 10.3389/fpubh.2022.988727
35. Liao, ZY, Xiao, MH, She, Q, and Xiong, BQ. Association between the composite dietary antioxidant index and metabolic syndrome: evidence from NHANES 2003-2018. Eur Rev Med Pharmacol Sci. (2024) 28:1513–23. doi: 10.26355/eurrev_202402_35481
36. Zhou, Q, Zhou, L, Chen, X, Chen, Q, and Hao, L. Composite dietary antioxidant index is associated with reduced prevalence of metabolic syndrome but not mortality in metabolic syndrome: results from NHANES 2001-2018. Prev Med Rep. (2024) 41:102704. doi: 10.1016/j.pmedr.2024.102704
37. Chew, NWS, Ng, CH, Tan, DJH, Kong, G, Lin, C, Chin, YH, et al. The global burden of metabolic disease: data from 2000 to 2019. Cell Metab. (2023) 35:414–28.e3. doi: 10.1016/j.cmet.2023.02.003
38. Pu, D, Tan, R, Yu, Q, and Wu, J. Metabolic syndrome in menopause and associated factors: a meta-analysis. Climacteric. (2017) 20:583–91. doi: 10.1080/13697137.2017.1386649
39. Janssen, I, Powell, LH, Crawford, S, Lasley, B, and Sutton-Tyrrell, K. Menopause and the metabolic syndrome: the study of women's health across the nation. Arch Intern Med. (2008) 168:1568–75. doi: 10.1001/archinte.168.14.1568
40. Masenga, SK, Kabwe, LS, Chakulya, M, and Kirabo, A. Mechanisms of oxidative stress in metabolic syndrome. Int J Mol Sci. (2023) 24:7898. doi: 10.3390/ijms24097898
41. Hutcheson, R, and Rocic, P. The metabolic syndrome, oxidative stress, environment, and cardiovascular disease: the great exploration. Exp Diabetes Res. (2012) 2012:271028:1–13. doi: 10.1155/2012/271028
42. Sanz, RL, García Menéndez, S, Inserra, F, Ferder, L, and Manucha, W. Sodium-glucose cotransporter-2 inhibitors protect tissues via cellular and mitochondrial pathways: experimental and clinical evidence. World J Exp Med. (2024) 14:91519. doi: 10.5493/wjem.v14.i2.91519
43. Roumeliotis, S, Roumeliotis, A, Dounousi, E, Eleftheriadis, T, and Liakopoulos, V. Dietary antioxidant supplements and uric acid in chronic kidney disease: a review. Nutrients. (2019) 11:1911. doi: 10.3390/nu11081911
44. Thomas, MS, Huang, L, Garcia, C, Sakaki, JR, Blesso, CN, Chun, OK, et al. The effects of eggs in a plant-based diet on oxidative stress and inflammation in metabolic syndrome. Nutrients. (2022) 14:2548. doi: 10.3390/nu14122548
45. Čolak, E, and Pap, D. The role of oxidative stress in the development of obesity and obesity-related metabolic disorders. J Med Biochem. (2021) 40:1–9. doi: 10.5937/jomb0-24652
46. Demmig-Adams, B, and Adams, WW 3rd. Antioxidants in photosynthesis and human nutrition. Science (New York, NY). (2002) 298:2149–53. doi: 10.1126/science.1078002
47. Liakopoulos, V, Roumeliotis, S, Bozikas, A, Eleftheriadis, T, and Dounousi, E. Antioxidant supplementation in renal replacement therapy patients: is there evidence? Oxidative Med Cell Longev. (2019) 2019:1–23. doi: 10.1155/2019/9109473
48. Daneshzad, E, Keshavarz, SA, Qorbani, M, Larijani, B, and Azadbakht, L. Dietary total antioxidant capacity and its association with sleep, stress, anxiety, and depression score: a cross-sectional study among diabetic women. Clin Nutr Espen. (2020) 37:187–94. doi: 10.1016/j.clnesp.2020.03.002
49. Borrás, C, Gambini, J, Gómez-Cabrera, MC, Sastre, J, Pallardó, FV, Mann, GE, et al. 17beta-oestradiol up-regulates longevity-related, antioxidant enzyme expression via the Erk1 and Erk2[Mapk]/NfkappaB cascade. Aging Cell. (2005) 4:113–8. doi: 10.1111/j.1474-9726.2005.00151.x
50. Borrás, C, Sastre, J, García-Sala, D, Lloret, A, Pallardó, FV, and Viña, J. Mitochondria from females exhibit higher antioxidant gene expression and lower oxidative damage than males. Free Radic Biol Med. (2003) 34:546–52. doi: 10.1016/S0891-5849(02)01356-4
51. Ulas, M, and Cay, M. The effects of 17beta-estradiol and vitamin E treatments on oxidative stress and antioxidant levels in brain cortex of diabetic ovariectomized rats. Acta Physiol Hung. (2010) 97:208–15. doi: 10.1556/APhysiol.97.2010.2.7
52. Díaz, A, López-Grueso, R, Gambini, J, Monleón, D, Mas-Bargues, C, Abdelaziz, KM, et al. Sex differences in age-associated type 2 diabetes in rats-role of estrogens and oxidative stress. Oxidative Med Cell Longev. (2019) 2019:1–13. doi: 10.1155/2019/6734836
53. Hildrum, B, Mykletun, A, Hole, T, Midthjell, K, and Dahl, AA. Age-specific prevalence of the metabolic syndrome defined by the international diabetes federation and the national cholesterol education program: the Norwegian hunt 2 study. BMC Public Health. (2007) 7:220. doi: 10.1186/1471-2458-7-220
54. Geng, Q, Gao, H, Yang, R, Guo, K, and Miao, D. Pyrroloquinoline Quinone prevents estrogen deficiency-induced osteoporosis by inhibiting oxidative stress and osteocyte senescence. Int J Biol Sci. (2019) 15:58–68. doi: 10.7150/ijbs.25783
55. Takahashi, TA, and Johnson, KM. Menopause. Med Clin North Am. (2015) 99:521–34. doi: 10.1016/j.mcna.2015.01.006
56. Padgett, LE, Broniowska, KA, Hansen, PA, Corbett, JA, and Tse, HM. The role of reactive oxygen species and proinflammatory cytokines in type 1 diabetes pathogenesis. Ann N Y Acad Sci. (2013) 1281:16–35. doi: 10.1111/j.1749-6632.2012.06826.x
57. Forrester, SJ, Kikuchi, DS, Hernandes, MS, Xu, Q, and Griendling, KK. Reactive oxygen species in metabolic and inflammatory signaling. Circ Res. (2018) 122:877–902. doi: 10.1161/CIRCRESAHA.117.311401
58. Manolagas, SC. From estrogen-centric to aging and oxidative stress: a revised perspective of the pathogenesis of osteoporosis. Endocr Rev. (2010) 31:266–300. doi: 10.1210/er.2009-0024
59. Wilkinson, MJ, Manoogian, ENC, Zadourian, A, Lo, H, Fakhouri, S, Shoghi, A, et al. Ten-hour time-restricted eating reduces weight, blood pressure, and atherogenic lipids in patients with metabolic syndrome. Cell Metab. (2020) 31:92–104.e5. doi: 10.1016/j.cmet.2019.11.004
60. Dieli-Conwright, CM, Courneya, KS, Demark-Wahnefried, W, Sami, N, Lee, K, Buchanan, TA, et al. Effects of aerobic and resistance exercise on metabolic syndrome, sarcopenic obesity, and circulating biomarkers in overweight or obese survivors of breast cancer: a randomized controlled trial. J Clin Oncol. (2018) 36:875–83. doi: 10.1200/JCO.2017.75.7526
61. Jeong, J, and Suh, YJ. Association between serum uric acid and metabolic syndrome in Koreans. J Korean Med Sci. (2019) 34:e307. doi: 10.3346/jkms.2019.34.e307
Keywords: NHANES, metabolic syndrome, CDAI, estrogen, females
Citation: Liu W, Xu Y, Xiao L, Li K and Liu Q (2025) Composite dietary antioxidant index is associated with the prevalence of metabolic syndrome in females: results from NHANES 2011–2016. Front. Nutr. 12:1529332. doi: 10.3389/fnut.2025.1529332
Received: 16 November 2024; Accepted: 13 March 2025;
Published: 27 March 2025.
Edited by:
Justyna Opydo-Szymaczek, Poznan University of Medical Sciences, PolandReviewed by:
Qian Zhu, Guangdong Provincial People's Hospital, ChinaCopyright © 2025 Liu, Xu, Xiao, Li and Liu. This is an open-access article distributed under the terms of the Creative Commons Attribution License (CC BY). The use, distribution or reproduction in other forums is permitted, provided the original author(s) and the copyright owner(s) are credited and that the original publication in this journal is cited, in accordance with accepted academic practice. No use, distribution or reproduction is permitted which does not comply with these terms.
*Correspondence: Ke Li, Y29saW5saWtlQDE2My5jb20=; Qiang Liu, c2Zzcm15eWtqa0AxNjMuY29t
†These authors have contributed equally to this work and share first authorship
Disclaimer: All claims expressed in this article are solely those of the authors and do not necessarily represent those of their affiliated organizations, or those of the publisher, the editors and the reviewers. Any product that may be evaluated in this article or claim that may be made by its manufacturer is not guaranteed or endorsed by the publisher.
Research integrity at Frontiers
Learn more about the work of our research integrity team to safeguard the quality of each article we publish.