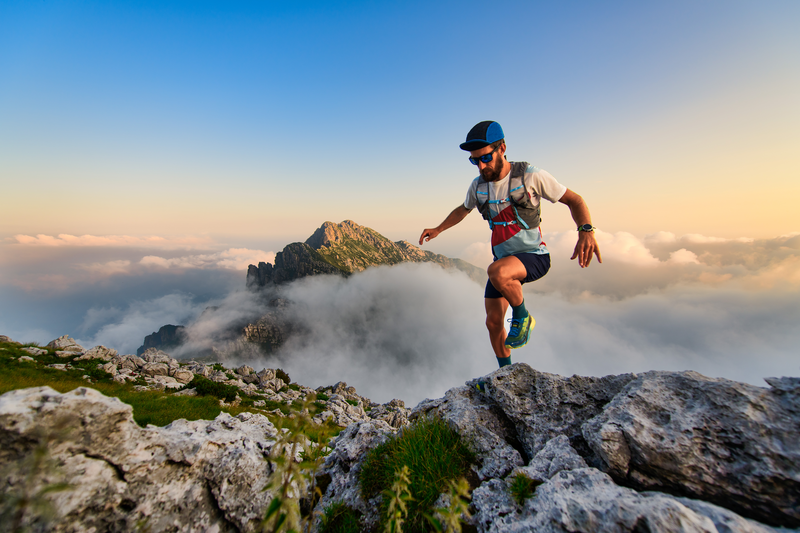
94% of researchers rate our articles as excellent or good
Learn more about the work of our research integrity team to safeguard the quality of each article we publish.
Find out more
ORIGINAL RESEARCH article
Front. Nutr.
Sec. Clinical Nutrition
Volume 12 - 2025 | doi: 10.3389/fnut.2025.1528677
The final, formatted version of the article will be published soon.
You have multiple emails registered with Frontiers:
Please enter your email address:
If you already have an account, please login
You don't have a Frontiers account ? You can register here
AbstractBackground: Previous studies have confirmed the relationship between gut microbiota and sleep disorders, characterized by the persistent inability to achieve adequate sleep, with dietary composition playing a key role in maintaining microbiota homeostasis. Our study aims to explore the relationship between the newly proposed Dietary Index for Gut Microbiota (DI-GM) and sleep disorders, as well as whether the Dietary Inflammatory Index (DII) mediates this relationship.Methods: This study is based on data from 30,406 participants in the National Health and Nutrition Examination Survey (NHANES) from 2005 to 2018, a cross-sectional survey that represents the U.S. adult population. We used multivariable logistic regression models to examine the relationship between DI-GM and sleep disorders. Subgroup interaction analyses were conducted to assess the stability of the results. Mediation analysis was employed to explore the effect of the Dietary Inflammatory Index (DII) on the relationship between DI-GM and sleep disorders.Results: The DI-GM score was significantly negatively correlated with sleep disorders. After adjusting for covariates, each unit increase in DI-GM was associated with a 5% reduction in the prevalence of sleep disorders (p < 0.001). Additionally, there was a trend toward a decrease in the prevalence of sleep disorders with increasing DI-GM (trend p < 0.05). Dose-response curve analysis revealed a linear relationship between DI-GM and sleep disorders, with higher DI-GM scores being associated with lower prevalence of sleep disorders. DII was positively correlated with sleep disorders (p < 0.001) and decreased as DI-GM increased (β = -0.37, p < 0.001). Mediation analysis showed that DII significantly mediated the relationship between DI-GM and sleep disorders, with a mediation proportion of 27.36% (p < 0.001).Conclusions: The results of this study indicate that the DI-GM score was significantly negatively correlated with sleep disorders. A higher DI-GM score is associated with a lower incidence of sleep disorders, while the DII significantly mediated the relationship between DI-GM and sleep disorders. Specifically, an increase in DII may attenuate the protective effect of DI-GM on sleep disorders.
Keywords: DI-GM, sleep disorder, DiI, NHANES, Cross-sectional study;
Received: 15 Nov 2024; Accepted: 28 Feb 2025.
Copyright: © 2025 ying, Pan and Shen. This is an open-access article distributed under the terms of the Creative Commons Attribution License (CC BY). The use, distribution or reproduction in other forums is permitted, provided the original author(s) or licensor are credited and that the original publication in this journal is cited, in accordance with accepted academic practice. No use, distribution or reproduction is permitted which does not comply with these terms.
* Correspondence:
Fei Xiao Shen, Department of Otorhinolaryngology, Children' Hospital of Nanjing Medical UniversityUniversity, nanjing,jiangsu province, China
Disclaimer: All claims expressed in this article are solely those of the authors and do not necessarily represent those of their affiliated organizations, or those of the publisher, the editors and the reviewers. Any product that may be evaluated in this article or claim that may be made by its manufacturer is not guaranteed or endorsed by the publisher.
Research integrity at Frontiers
Learn more about the work of our research integrity team to safeguard the quality of each article we publish.