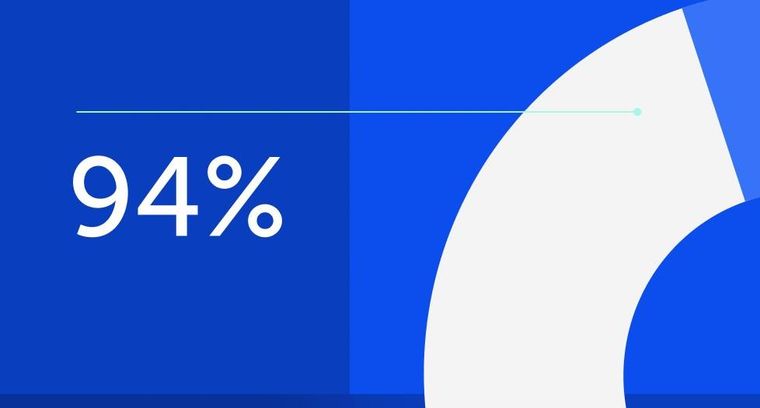
94% of researchers rate our articles as excellent or good
Learn more about the work of our research integrity team to safeguard the quality of each article we publish.
Find out more
ORIGINAL RESEARCH article
Front. Nutr., 28 March 2025
Sec. Nutrition and Metabolism
Volume 12 - 2025 | https://doi.org/10.3389/fnut.2025.1527207
This article is part of the Research TopicDietary Habits in Liver Health and Disease: Preclinical and Clinical StudiesView all 14 articles
Objective: This study examines the link between serum manganese (Mn) levels and non-alcoholic fatty liver disease (NAFLD), with a focus on gender differences.
Methods: Utilizing data from the NHANES 2017–2018, we included participants aged 18 and older, excluding those without ultrasonic liver assessment, serum Mn data, or with hepatitis or significant alcohol use. The final analysis comprised 4,294 individuals, with 2,708 in the NAFLD group and 1,586 in the non-NAFLD group. Serum Mn was quantified via inductively coupled plasma mass spectrometry. We compared demographic and health-related variables between groups using appropriate statistical tests and categorized participants into quartiles based on Mn levels. Multivariate logistic regression and spline regression analyses were conducted to evaluate the association between serum Mn and NAFLD risk by gender.
Results: Serum Mn was significantly elevated in the NAFLD group compared to non-NAFLD individuals (9.06 vs. 9.33 μg/L, Z = 2.815, p = 0.005). After adjustments, males in the third Mn quartile showed a higher NAFLD risk (OR = 1.575; 95% CI: 1.193–2.087), while females in the fourth quartile also had increased risk (OR = 1.725; 95% CI: 1.313–2.269), both compared to the first quartile (p < 0.01). A positive dose–response relationship was found for both genders (P for trend <0.01), with nonlinear associations in males (P for nonlinearity <0.01) and linear associations in females (P for nonlinearity = 0.818). Significant interactions with ethnicity in males and hypertension in females were also noted.
Conclusion: Higher serum Mn levels are significantly associated with increased NAFLD risk in both genders, highlighting the need for gender-specific considerations in future studies and clinical practices.
Non-alcoholic fatty liver disease (NAFLD) is a clinical-pathological syndrome characterized by diffuse macrovesicular fatty degeneration and lipid accumulation in hepatocytes, primarily affecting the liver lobule. Unlike other liver diseases, NAFLD is not attributable to alcohol consumption or other clearly hepatotoxic factors (1). Epidemiological surveys have highlighted the growing prevalence of NAFLD, which stands at 25.24% globally, making it the most common chronic liver condition worldwide (2). The increasing incidence of NAFLD is closely linked to rising obesity rates, influenced by improved living standards, dietary changes, sedentary lifestyles, and environmental contaminants, which have established etiologic roles with strong sex-dimorphism (3, 4). Projections suggest that by 2030, the number of NAFLD cases will escalate by 21%, reaching 100.9 million from 83.1 million in 2015 (5). If left unmanaged, NAFLD can progress to more severe liver conditions, such as fibrosis, cirrhosis, and hepatocellular carcinoma (6).
Manganese (Mn) is a trace element with toxic potential, which enters the body mainly through the gastrointestinal tract from sources such as dietary intake, including the consumption of vegetables and fruits contaminated with fungicides such as maneb and mancozeb, as well as other environmental exposures (7). Mn is crucial for the clearance of reactive oxygen species (ROS) from mitochondrial oxidative stress, primarily through its role in the enzyme manganese superoxide dismutase (Mn-SOD). The C47T polymorphism in the SOD2 gene, which affects Mn-SOD’s mitochondrial targeting and activity, has been identified as an independent risk factor for advanced fibrosis in NAFLD (8). Although the exact pathogenesis of NAFLD remains elusive, the involvement of ROS, oxidative stress, inflammation, and fatty acid metabolism imbalances are key contributing factors (9). Elevated serum Mn levels can exacerbate NAFLD progression by influencing fat accumulation, lipogenesis, insulin resistance, oxidative stress, and inflammation (10).
With the modernization of agriculture and industry, metal pollution has intensified, leading to a growing concern about the impact of various metals on health. Studies have demonstrated significant associations between NAFLD and exposures to metals such as cadmium (11) and arsenic (12), among others (13). Despite the lack of safe and effective treatments for NAFLD, research on the correlation between trace metal Mn and NAFLD is still limited. Notably, serum Mn levels exhibit significant gender differences, with females showing higher levels than males (14).
However, it is important to note that serum Mn levels may not be fully predictive of internal contamination. Studies suggest that matrices like hair could provide a more accurate assessment of Mn exposure due to its longer retention time and less fluctuation compared to serum levels (14). This limitation should be considered when interpreting the results of this study.
This study aims to analyze these gender differences separately, exploring the correlation between serum Mn levels and the risk of NAFLD progression in both males and females. By doing so, the study seeks to enhance the understanding of NAFLD pathogenesis from different perspectives and identify potential biomarkers for its development. This could ultimately aid in formulating effective prevention strategies at both the individual and population levels.
The National Health and Nutrition Examination Survey (NHANES) is a comprehensive cross-sectional study aimed at evaluating the health and nutritional status of the U.S. population (15). Conducted every 2 years, NHANES gathers extensive data encompassing demographic, lifestyle, health, and nutritional information from participants. The NHANES public database can be accessed at NHANES CDC.1 For this investigation, we utilized data from the 2017–2018 NHANES cycle. Our inclusion criteria focused on registered participants aged 18 years and older, yielding an initial cohort of 5,856 individuals. We applied several exclusion criteria: (1) absence of liver ultrasound transient elastography results (n = 253); (2) diagnosis of hepatitis B or C (n = 85); (3) significant alcohol intake (men >30 g/day, women >20 g/day) (n = 478); (4) missing critical laboratory data such as alanine aminotransferase (ALT) and aspartate aminotransferase (AST) (n = 489); and (5) unavailability of serum manganese (Mn) levels (n = 257). Ultimately, a total of 4,294 participants were retained for analysis.
Liver ultrasound transient elastography serves as a non-invasive, objective method for diagnosing non-alcoholic fatty liver disease (NAFLD), noted for its robust sensitivity and specificity in population studies (16). The Controlled Attenuation Parameter (CAP) is a key indicator for NAFLD detection, with performance comparable to that of liver biopsy, which is considered the gold standard. A diagnosis of NAFLD is established with a CAP value of 223 dB/m or above, while excluding individuals with hepatitis B, hepatitis C, autoimmune liver disorders, and significant alcohol use (men >30 g/day, women >20 g/day) (17).
Serum manganese levels were measured at the Environmental Health Sciences Laboratory of the National Center for Environmental Health using inductively coupled plasma dynamic reaction cell mass spectrometry. This process adheres to rigorous quality control standards (18). Normal serum Mn concentrations typically range from 4 to 15 μg/L. (19) In this study, the detection threshold for serum Mn was set at 0.990 μg/L, with any values below this limit substituted with the detection limit divided by the square root of 2.
We conducted statistical analyses using R version 4.2.2. For data that exhibited skewed distributions, results are presented as medians (M) with interquartile ranges (P25, P75), and comparisons were performed using the Wilcoxon rank-sum test. Categorical variables were represented as counts and percentages, with differences assessed via the chi-square (χ2) test. To explore the association between serum Mn levels and NAFLD, we employed multivariate adjusted logistic regression models. Serum Mn levels were analyzed both as continuous and categorical variables, stratified into quartiles with the first quartile serving as the reference group. Odds ratios (OR) and 95% confidence intervals (CI) were computed across three modeling approaches: Model 1, which included no adjustments; Model 2, which adjusted for demographic factors such as age, ethnicity, education, marital status, Family-to-Poverty Ratio (FMPIR), and Body Mass Index (BMI); and Model 3, which further adjusted for health-related factors including smoking, alcohol consumption, diabetes, hypertension, and hyperlipidemia.
We also utilized restricted cubic spline regression to examine non-linear relationships between serum Mn levels and NAFLD, visualizing the dose–response association. Additionally, subgroup analyses were performed, categorizing participants by age, ethnicity, education, marital status, FMPIR, smoking status, alcohol consumption, and history of diabetes, hypertension, and hyperlipidemia. Interaction terms were incorporated into our models, and likelihood ratio tests were conducted to assess the presence of interactions, thereby uncovering potential variations in the relationship between serum Mn levels and NAFLD. A p-value of less than 0.05 was deemed statistically significant.
The study comprised 4,294 participants, divided into 2,708 individuals with NAFLD and 1,586 without NAFLD. Notable differences in demographic and clinical features were evident between these groups. Participants with NAFLD were significantly older and included a higher proportion of males, Mexican Americans, individuals with high school education or less, and those with a history of smoking more than 100 cigarettes in their lifetime. Additionally, the NAFLD group had more married individuals or those living with a partner, and a larger proportion fell within the FMPIR range of 1.30 to 3.50 and had a BMI of 30 or higher.
Clinically, the NAFLD group exhibited elevated levels of systolic and diastolic blood pressure, HOMA-IR, waist circumference, triglycerides, ALT, AST, GGT, fasting glucose, CRP, HbA1c, and CAP, along with reduced levels of HDL-C. The prevalence of diabetes, hypertension, and hyperlipidemia was also significantly higher in the NAFLD group (p < 0.01). Furthermore, serum manganese (Mn) levels were higher in the NAFLD group compared to the non-NAFLD group. Detailed demographic and clinical data are presented in Table 1.
To determine the association between serum Mn levels and NAFLD, multivariate logistic regression models were employed, stratified by gender. Serum Mn was assessed both as a continuous variable and across quartiles.
For males, each quartile increase in serum Mn was associated with a 25.20% (OR = 1.252, 95% CI: 1.097–1.429), 17.70% (OR = 1.177, 95% CI: 1.020–1.358), and 18.90% (OR = 1.189, 95% CI: 1.028–1.375) higher risk of NAFLD in models 1, 2, and 3, respectively. When analyzed by quartiles, in model 3, the risk of NAFLD increased by 40.70% (OR = 1.407, 95% CI: 1.097–1.807) and 57.50% (OR = 1.575, 95% CI: 1.193–2.087) in the Q2 and Q3 groups compared to the lowest Mn group (Q1).
For females, similar trends were observed. The risk of NAFLD increased by 12.90% (OR = 1.129, 95% CI: 1.018–1.252), 26.60% (OR = 1.266, 95% CI: 1.128–1.421), and 32.40% (OR = 1.324, 95% CI: 1.176–1.491) per quartile increase in serum Mn in models 1, 2, and 3, respectively. When comparing quartiles in model 3, the risk of NAFLD was higher by 30.60% (OR = 1.306, 95% CI: 1.006–1.696), 44.40% (OR = 1.444, 95% CI: 1.109–1.882), and 72.50% (OR = 1.725, 95% CI: 1.313–2.269) in the Q2, Q3, and Q4 groups, respectively, relative to the lowest Mn group (Q1).
These findings highlight a significant association between elevated serum Mn levels and increased risk of NAFLD, with varying degrees of risk observed across different quartiles of Mn concentration. Detailed logistic regression results are provided in Tables 2, 3.
After adjusting for variables such as age, ethnicity, education, marital status, FMPIR, BMI, smoking, alcohol consumption, diabetes, hypertension, and hyperlipidemia, we employed restricted cubic spline regression analysis to examine the relationship between serum manganese (Mn) levels and the risk of non-alcoholic fatty liver disease (NAFLD) by gender.
In males, a positive dose–response relationship was observed between serum Mn levels and the risk of NAFLD (p < 0.01), with a significant non-linear component (P for nonlinearity <0.01). Specifically, serum Mn levels below 8.747 μg/L were protective against NAFLD, with the protective effect diminishing as Mn levels increased. Between 8.747 μg/L and 10.909 μg/L, serum Mn levels were associated with an increased risk of NAFLD, and this risk continued to rise with higher levels. Beyond 10.909 μg/L, no significant association with NAFLD risk was detected.
In females, the positive dose–response relationship between serum Mn levels and NAFLD risk was also significant (p < 0.01), but the relationship was linear (P for nonlinearity = 0.818). Serum Mn levels below 9.850 μg/L were protective, with the protective effect declining as levels increased. When serum Mn levels exceeded 9.850 μg/L, the risk of NAFLD increased significantly.
Figures 1, 2 provide a detailed illustration of these relationships.
In males, significant interactions were found between serum Mn levels and both ethnicity (p = 0.011) and education (p = 0.020). For non-Hispanic Black males, each quartile increase in serum Mn was associated with a 52.90% increase in the risk of NAFLD (OR = 1.529; 95% CI: 1.164–2.008). Additionally, males with education below high school experienced a 70.30% increase in NAFLD risk (OR = 1.703; 95% CI: 1.233–2.351), with these differences being statistically significant (p < 0.01).
In females, a significant interaction was observed between serum Mn levels and hypertension status (p = 0.006). Hypertensive females experienced a 65.80% increase in NAFLD risk (OR = 1.658; 95% CI: 1.153–2.386) with each quartile increase in serum Mn. Conversely, non-hypertensive females had a 31.40% increase in NAFLD risk (OR = 1.314; 95% CI: 1.143–1.511), with both interactions showing statistically significant differences (p < 0.01).
Tables 4, 5 provide detailed results of these subgroup analyses.
Based on the NHANES 2017–2018 survey data, this study found that serum Mn levels in the NAFLD group were significantly higher than those in the control group (p = 0.005). After constructing multivariate logistic regression models and adjusting for confounders, serum Mn levels were positively associated with NAFLD in both males and females (male Q3 vs. Q1: OR = 1.575, 95% CI: 1.193–2.087; female Q4 vs. Q1: OR = 1.725, 95% CI: 1.313–2.267).
The restricted cubic spline regression revealed a nonlinear dose–response relationship in males, where Mn acted protectively at lower concentrations (≤8.747 μg/L) but transitioned to a risk factor at intermediate levels (8.747–10.909 μg/L). In contrast, females exhibited a linear relationship, with Mn becoming a risk factor above 9.850 μg/L. These gender-specific patterns may stem from differences in Mn metabolism, hormonal influences, or genetic factors. For instance, estrogen has been shown to modulate Mn homeostasis by affecting transporters like SLC30A10, which regulates Mn excretion (20). Additionally, males may experience higher oxidative stress due to lower baseline antioxidant enzyme activity (e.g., Mn-SOD), amplifying Mn’s toxic effects at intermediate levels (21).
The gender disparity in dose–response relationships could also arise from differences in body composition (e.g., higher lean mass in males altering Mn distribution) or sex-specific expression of Mn-binding proteins (22).
Mechanistically, Mn’s dual role—as a nutrient and toxin—may explain the observed thresholds. At physiological levels, Mn supports mitochondrial function and antioxidant defense via Mn-SOD (23). However, beyond optimal levels, Mn’s toxicity may outweigh its beneficial effects, contributing to the pathogenesis of NAFLD through mechanisms such as increased oxidative stress and inflammation (24, 25).
Notably, conflicting findings from prior studies warrant discussion. While animal models report lower hepatic Mn levels in NAFLD (26), this discrepancy may reflect species-specific Mn metabolism or compensatory mechanisms in chronic disease. Serum Mn levels may not fully correlate with tissue accumulation in advanced NAFLD, as hepatic damage could impair Mn storage or increase systemic release (23). Furthermore, human studies using serum Mn (27) versus tissue-specific measurements (26) may yield divergent results. For example, serum Mn elevation in NAFLD could indicate dysregulated excretion (e.g., via bile) rather than tissue overload (28), a hypothesis requiring validation through paired serum and liver biopsy studies.
Subgroup analyses highlighted interactions between Mn and sociodemographic/clinical factors. In males, ethnicity and education modified Mn-NAFLD associations, possibly due to environmental or occupational Mn exposure disparities (e.g., non-Hispanic Black individuals facing higher industrial pollution). Hypertensive females exhibited stronger Mn-NAFLD links, suggesting shared pathways between Mn toxicity, oxidative stress, and endothelial dysfunction.
Limitations of this cross-sectional study preclude causal inferences. While serum Mn levels are a practical biomarker, they may not fully reflect hepatic Mn accumulation or long-term exposure. Prospective cohort studies with repeated Mn measurements and tissue-level data (e.g., liver biopsies) are needed to clarify causality. Additionally, confounding by unmeasured factors (e.g., dietary Mn intake, genetic polymorphisms in Mn transporters) and recall bias in self-reported covariates (e.g., alcohol use) may influence results.
In conclusion, this study underscores serum Mn as a potential risk factor for NAFLD, with gender-specific thresholds and mechanisms. Future research should prioritize elucidating Mn’s tissue-specific dynamics, longitudinal associations, and molecular pathways in NAFLD pathogenesis. Clinically, monitoring serum Mn in high-risk populations (e.g., industrial workers) and addressing gender-specific risk profiles could enhance NAFLD prevention strategies.
The original contributions presented in the study are included in the article/supplementary material, further inquiries can be directed to the corresponding author.
The studies involving humans were approved by the Ethics Committee of Beijing Chao-Yang Hospital. All Patients and their families participated voluntarily and signed informed consent forms, and the study was performed in accordance with the Helsinki II declaration. Informed consent was obtained from all the study subjects before enrollment. The studies were conducted in accordance with the local legislation and institutional requirements. The participants provided their written informed consent to participate in this study. Written informed consent was obtained from the individual(s) for the publication of any potentially identifiable images or data included in this article.
ZH: Conceptualization, Investigation, Writing – original draft. YZ: Methodology, Supervision, Writing – original draft. HT: Conceptualization, Formal analysis, Methodology, Writing – review & editing.
The author(s) declare that no financial support was received for the research and/or publication of this article.
The authors declare that the research was conducted in the absence of any commercial or financial relationships that could be construed as a potential conflict of interest.
The author(s) declare that no Gen AI was used in the creation of this manuscript.
All claims expressed in this article are solely those of the authors and do not necessarily represent those of their affiliated organizations, or those of the publisher, the editors and the reviewers. Any product that may be evaluated in this article, or claim that may be made by its manufacturer, is not guaranteed or endorsed by the publisher.
1. Byrne, CD, and Targher, G. NAFLD: a multisystem disease. J Hepatol. (2015) 62:S47–64. doi: 10.1016/j.jhep.2014.12.012
2. Younossi, ZM, Koenig, AB, Abdelatif, D, Fazel, Y, Henry, L, and Wymer, M. Global epidemiology of nonalcoholic fatty liver disease-Meta-analytic assessment of prevalence, incidence, and outcomes. Hepatology. (2016) 64:73–84. doi: 10.1002/hep.28431
3. Della Torre, S. Non-alcoholic fatty liver disease as a canonical example of metabolic inflammatory-based liver disease showing a sex-specific prevalence: relevance of estrogen signaling. Front Endocrinol. (2020) 11:572490. doi: 10.3389/fendo.2020.572490
4. Le Magueresse-Battistoni, B. Endocrine disrupting chemicals and metabolic disorders in the liver: what if we also looked at the female side? Chemosphere. (2021) 268:129212. doi: 10.1016/j.chemosphere.2020.129212
5. Estes, C, and Razavi, H. Modeling the epidemic of nonalcoholic fatty liver disease demonstrates an exponential increase in burden of disease. Hepatology. (2018) 67:123–33. doi: 10.1002/hep.29466
6. Argo, CK, and Caldwell, SH. Epidemiology and natural history of non-alcoholic steatohepatitis. Clin Liver Dis. (2009) 13:511–31. doi: 10.1016/j.cld.2009.07.005
7. Li, L, and Yang, X. The essential element manganese, oxidative stress, and metabolic diseases: links and interactions. Oxidative Med Cell Longev. (2018) 2018:7580707. doi: 10.1155/2018/7580707
8. Al-Serri, A, Anstee, QM, Valenti, L, Nobili, V, Leathart, JB, Dongiovanni, P, et al. The SOD2 C47T polymorphism influences NAFLD fibrosis severity: evidence from case-control and intra-familial allele association studies. J Hepatol. (2012) 56:448–54. doi: 10.1016/j.jhep.2011.05.029
9. Mota, M, Banini, BA, Cazanave, SC, and Sanyal, AJ. Molecular mechanisms of lipotoxicity and glucotoxicity in nonalcoholic fatty liver disease. Metab Clin Exp. (2016) 65:1049–61. doi: 10.1016/j.metabol.2016.02.014
10. Rezazadeh, A, and Yazdanparast, R. Prevention of nonalcoholic steatohepatitis in rats by two manganese-salen complexes. Iran Biomed J. (2014) 18:41–8. doi: 10.6091/ibj.1201.2013
11. Hyder, O, Chung, M, Cosgrove, D, Herman, JM, Li, Z, Firoozmand, A, et al. Cadmium exposure and liver disease among US adults. J Gastrointest Surg. (2013) 17:1265–73. doi: 10.1007/s11605-013-2210-9
12. Tan, M, Schmidt, RH, Beier, JI, Watson, WH, Zhong, H, States, JC, et al. Chronic subhepatotoxic exposure to arsenic enhances hepatic injury caused by high fat diet in mice. Toxicol Appl Pharmacol. (2011) 257:356–64. doi: 10.1016/j.taap.2011.09.019
13. Cave, M, Appana, S, Patel, M, Falkner, KC, McClain, CJ, and Brock, G. Polychlorinated biphenyls, lead, and mercury are associated with liver disease in American adults: NHANES 2003-2004. Environ Health Perspect. (2010) 118:1735–42. doi: 10.1289/ehp.1002720
14. O'Neal, SL, and Zheng, W. Manganese toxicity upon overexposure: a decade in review. Curr Environ Health Rep. (2015) 2:315–28. doi: 10.1007/s40572-015-0056-x
15. Johnson, CL, Paulose-Ram, R, Ogden, CL, Carroll, MD, Kruszon-Moran, D, Dohrmann, SM, et al. National health and nutrition examination survey: analytic guidelines, 1999-2010 In: Vital and health statistics Series 2, Data evaluation and methods research, 161 (2013). 1–24.
16. Zhang, K, Nulali, J, Zhang, C, Chen, Y, Cheng, J, Shi, X, et al. The association between serum vitamin a and NAFLD among US adults varied in different BMI groups: a cross-sectional study. Food Funct. (2023) 14:836–44. doi: 10.1039/D2FO02204D
17. Peng, H, Pan, L, Ran, S, Wang, M, Huang, S, Zhao, M, et al. Prediction of MAFLD and NAFLD using different screening indexes: a cross-sectional study in U.S. adults. Front Endocrinol. (2023) 14:1083032. doi: 10.3389/fendo.2023.1083032
18. Wang, X, Seo, YA, and Park, SK. Serum selenium and non-alcoholic fatty liver disease (NAFLD) in U.S. adults: National Health and nutrition examination survey (NHANES) 2011-2016. Environ Res. (2021) 197:111190. doi: 10.1016/j.envres.2021.111190
19. Williams, M, Todd, GD, Roney, N, Crawford, J, Coles, C, McClure, PR, et al. Agency for Toxic Substances and Disease Registry (ATSDR) toxicological profiles In: Toxicological profile for manganese. Atlanta (GA): Agency for Toxic Substances and Disease Registry (US) (2012)
20. Kochhar, S, Jacobs, DM, Ramadan, Z, Berruex, F, Fuerholz, A, and Fay, LB. Probing gender-specific metabolism differences in humans by nuclear magnetic resonance-based metabonomics. Anal Biochem. (2006) 352:274–81. doi: 10.1016/j.ab.2006.02.033
21. Sabato, V, Ebo, DG, Koppelman, SJ, Jayasena, S, Luykx, D, Schepens, E, et al. Allergenicity attributes of different peanut market types. Food and chemical toxicology: an international journal published for the British industrial biological research association 2016. Food Chem Toxicol. (2016) 92:256. doi: 10.1016/j.fct.2016.03.019
22. Marchetti, A, and Orlando, M. A cold-active esterase enhances mesophilic properties through Mn (2+) binding. FEBS J. (2023) 290:2394–411. doi: 10.1111/febs.16661
23. Tang, S, Luo, S, Wu, Z, and Su, J. Association between blood heavy metal exposure levels and risk of metabolic dysfunction associated fatty liver disease in adults: 2015-2020 NHANES large cross-sectional study. Front Public Health. (2024) 12:1280163. doi: 10.3389/fpubh.2024.1280163
24. Santamaria, AB. Manganese exposure, essentiality & toxicity. Indian J Med Res. (2008) 128:484–500.
25. Horning, KJ, Caito, SW, Tipps, KG, Bowman, AB, and Aschner, M. Manganese is essential for neuronal health. Annu Rev Nutr. (2015) 35:71–108. doi: 10.1146/annurev-nutr-071714-034419
26. Gatiatulina, ER, Popova, EV, Polyakova, VS, Skalnaya, AA, Agletdinov, EF, Nikonorov, AA, et al. Evaluation of tissue metal and trace element content in a rat model of non-alcoholic fatty liver disease using ICP-DRC-MS. J Trace Elem Med Biol. (2017) 39:91–9. doi: 10.1016/j.jtemb.2016.08.007
27. Wan, H, Jiang, Y, Yang, J, Ma, Q, Liu, L, Peng, L, et al. Sex-specific associations of the urinary fourteen-metal mixture with NAFLD and liver fibrosis among US adults: a nationally representative study. Ecotoxicol Environ Saf. (2022) 248:114306. doi: 10.1016/j.ecoenv.2022.114306
Keywords: serum manganese, non-alcoholic fatty liver disease, transient elastography of liver, body mass index, hypertension, ethnicity, gender
Citation: He Z, Zhao Y and Tang H (2025) Serum manganese and its association with non-alcoholic fatty liver disease: findings from NHANES. Front. Nutr. 12:1527207. doi: 10.3389/fnut.2025.1527207
Received: 13 November 2024; Accepted: 10 March 2025;
Published: 28 March 2025.
Edited by:
Evelyn Nunes Goulart Da Silva Pereira, Oswaldo Cruz Foundation (Fiocruz), BrazilReviewed by:
Brigitte Le Magueresse-Battistoni, INSERM U1060 Laboratoire de Recherche en Cardiovasculaire, Métabolisme, diabétologie et Nutrition, FranceCopyright © 2025 He, Zhao and Tang. This is an open-access article distributed under the terms of the Creative Commons Attribution License (CC BY). The use, distribution or reproduction in other forums is permitted, provided the original author(s) and the copyright owner(s) are credited and that the original publication in this journal is cited, in accordance with accepted academic practice. No use, distribution or reproduction is permitted which does not comply with these terms.
*Correspondence: Hua Tang, MTMxMjA0MzEyNjZAMTYzLmNvbQ==
Disclaimer: All claims expressed in this article are solely those of the authors and do not necessarily represent those of their affiliated organizations, or those of the publisher, the editors and the reviewers. Any product that may be evaluated in this article or claim that may be made by its manufacturer is not guaranteed or endorsed by the publisher.
Research integrity at Frontiers
Learn more about the work of our research integrity team to safeguard the quality of each article we publish.