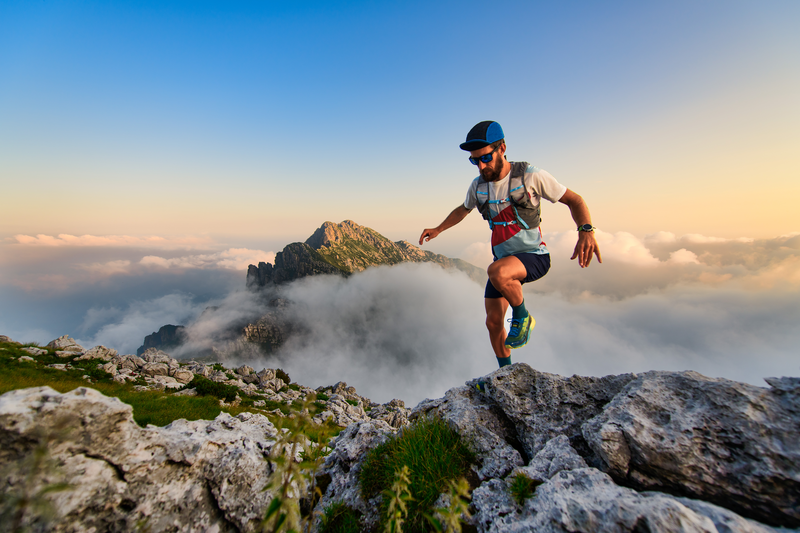
94% of researchers rate our articles as excellent or good
Learn more about the work of our research integrity team to safeguard the quality of each article we publish.
Find out more
ORIGINAL RESEARCH article
Front. Nutr.
Sec. Nutrition and Metabolism
Volume 12 - 2025 | doi: 10.3389/fnut.2025.1500600
This article is part of the Research Topic Maternal and Infant Nutrition: Impact on Breast Milk, Infant Gut Microbiota and Health Development View all articles
The final, formatted version of the article will be published soon.
You have multiple emails registered with Frontiers:
Please enter your email address:
If you already have an account, please login
You don't have a Frontiers account ? You can register here
Nutritional programming is a manipulation of fetal and neonatal development through maternal feeding. In humans and pigs, maternal yeast supplementation was demonstrated as a promising approach to positively to modulate newborns' health. This study aimed to investigate the effects of Saccharomyces cerevisiae var. boulardii CNCM I-1079 (SB) supplementation in pregnant and lactating bitches on the newborns' early growth rate (EGR, between birth and two days of life), metabolic profiles, and the association between both of them. A total of 17 female dogs and their 81 puppies were included. From day 28 of gestation until the end of the study, bitches were divided into two groups, one of which received orally 1.3´10 9 colony forming units of live yeast per day. Puppies from mothers receiving the live yeast were defined as the SB group (n = 40) and the others were defined as the placebo group (n =4 1). For each puppy, EGR was calculated, and blood and urine samples were collected at D2 for metabolome analysis using liquid chromatography-mass spectrometry (LCMS). Puppies from the SB group presented higher EGR compared with the placebo group (12 % vs. 7 %; p = 0.049). According to the Sparse Partial Least Squares Discriminant Analysis (sPLS-DA), both urine and serum metabolome profiles were significantly different between the two groups with a total of 29 discriminating metabolites in urine and serum. Fourteen of them were implicated in the nitrogen metabolism pathway including, gamma-aminobutyrate, 3-methyl-l-histidine and xanthosine (less abundant in SB compared with placebo group, all p < 0.05), adenine, aspartate and proline (more abundant in SB compared with placebo group, all p < 0.05). Metabolic pathways pointed to proline synthesis, a crucial component in collagen synthesis and osteoarticular system development. Urinary proline abundance was positively correlated with EGR (r = 0.45; p < 0.001). These findings highlight the potential benefits of maternal supplementation with SB promoting early neonatal growth, essential for the neonatal survival, through nitrogen metabolism orientation.
Keywords: Nutritional programming, early growth, Proline, Metabolome, newborn dog, dog, yeast
Received: 23 Sep 2024; Accepted: 11 Feb 2025.
Copyright: © 2025 Bendahmane, Garrigues, Apper, Mugnier, Svilar, Martin, Chastant, Meynadier and Mila. This is an open-access article distributed under the terms of the Creative Commons Attribution License (CC BY). The use, distribution or reproduction in other forums is permitted, provided the original author(s) or licensor are credited and that the original publication in this journal is cited, in accordance with accepted academic practice. No use, distribution or reproduction is permitted which does not comply with these terms.
* Correspondence:
Ilyas Bendahmane, NeoCare, ENVT, Université de Toulouse, Toulouse, France
Disclaimer: All claims expressed in this article are solely those of the authors and do not necessarily represent those of their affiliated organizations, or those of the publisher, the editors and the reviewers. Any product that may be evaluated in this article or claim that may be made by its manufacturer is not guaranteed or endorsed by the publisher.
Research integrity at Frontiers
Learn more about the work of our research integrity team to safeguard the quality of each article we publish.