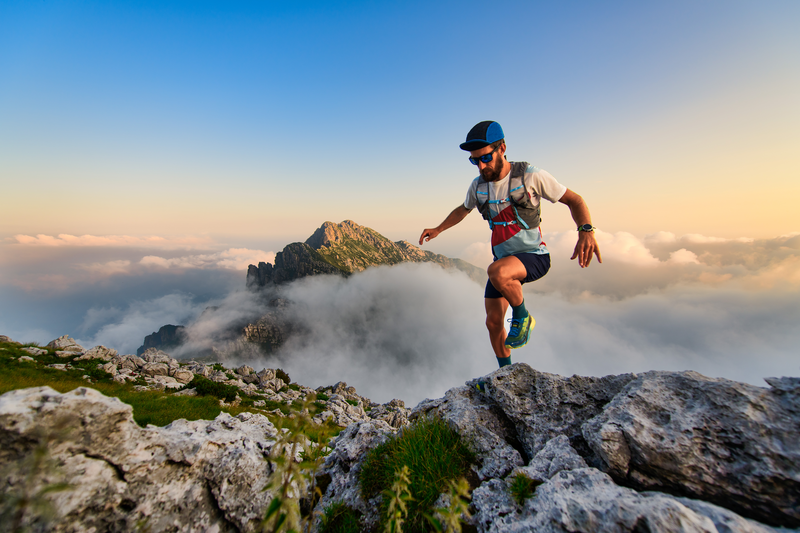
94% of researchers rate our articles as excellent or good
Learn more about the work of our research integrity team to safeguard the quality of each article we publish.
Find out more
MINI REVIEW article
Front. Nutr.
Sec. Nutrition, Psychology and Brain Health
Volume 12 - 2025 | doi: 10.3389/fnut.2025.1492306
This article is part of the Research Topic Ketogenic Metabolic Therapy as a Treatment for Mental Health Disorders View all 14 articles
The final, formatted version of the article will be published soon.
You have multiple emails registered with Frontiers:
Please enter your email address:
If you already have an account, please login
You don't have a Frontiers account ? You can register here
Adhering to the ketogenic diet can reduce or stop seizures, even when other treatments fail, via mechanism(s) distinct from other available therapies. These results have led to interest in the diet for treating conditions such as Alzheimer's disease, depression and schizophrenia. Evidence points to the neuromodulator adenosine as a key mechanism underlying therapeutic benefits of a ketogenic diet. Adenosine represents a unique and direct link among cell energy, neuronal activity, and gene expression. The importance of the dopaminergic system is established in addiction, as are the challenges of modulating the dopamine system directly. A mediator that could antagonize dopamine’s effects would be useful, and adenosine is such a mediator due to its function and location. Studies report that the ketogenic diet improves cognition, sociability, and perseverative behaviors, and might improve depression. Many of the translational opportunities based on the ketogenic diet/adenosine link have come to the fore, including addiction, autism spectrum disorder, painful conditions, and a range of hyperdopaminergic disorders.
Keywords: Ketogenic Diet, Adenosine, Dopamine, Psychiatric disorder, Addiction
Received: 06 Sep 2024; Accepted: 11 Feb 2025.
Copyright: © 2025 Ruskin, Martinez and Masino. This is an open-access article distributed under the terms of the Creative Commons Attribution License (CC BY). The use, distribution or reproduction in other forums is permitted, provided the original author(s) or licensor are credited and that the original publication in this journal is cited, in accordance with accepted academic practice. No use, distribution or reproduction is permitted which does not comply with these terms.
* Correspondence:
David Ruskin, Trinity College, Hartford, CT, United States
Susan A Masino, Trinity College, Hartford, CT, United States
Disclaimer: All claims expressed in this article are solely those of the authors and do not necessarily represent those of their affiliated organizations, or those of the publisher, the editors and the reviewers. Any product that may be evaluated in this article or claim that may be made by its manufacturer is not guaranteed or endorsed by the publisher.
Research integrity at Frontiers
Learn more about the work of our research integrity team to safeguard the quality of each article we publish.