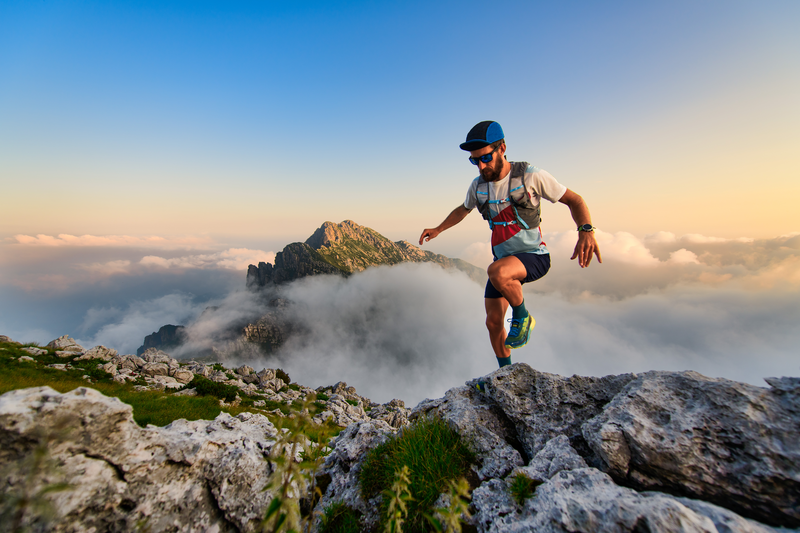
95% of researchers rate our articles as excellent or good
Learn more about the work of our research integrity team to safeguard the quality of each article we publish.
Find out more
REVIEW article
Front. Nutr. , 01 April 2025
Sec. Nutrition and Sustainable Diets
Volume 12 - 2025 | https://doi.org/10.3389/fnut.2025.1476672
Pregnancy is a period characterized by extensive physiological changes in both the mother and fetus. During this period, the nutritional status of the mother has a profound and irreversible impact on her health and the growth and development of the fetus. The fetus depends exclusively on the mother and drives nutrients through the placenta. Therefore, mothers must be provided with a well-balanced diet that is adequate in both macro- and micronutrients. Most pregnant women generally manage to get adequate macronutrients; however, many women fail to get micronutrients up to the recommended dietary allowance. Micronutrients such as vitamins and minerals are necessary for preventing congenital abnormalities and the optimal development of the brain and body of the fetus. Their inadequacy can lead to complications like anemia, hypertension, pre-eclampsia, maternal and fetal hypothyroidism, premature infants, intrauterine growth restriction, stillbirth, and other negative pregnancy outcomes. New studies recommend the use of prenatal micronutrient supplements to prevent birth defects and health issues caused by deficiencies in folic acid, iron, iodine, and calcium during pregnancy. This is especially important in developing nations where deficiencies are prevalent. Also while using these supplements, their upper limits (UL) must be considered to avoid overload. In this review, we provide an overview of the four most critical micronutrients during pregnancy: iron, folic acid, iodine, and calcium. We provide insight into their sources, RDAs, deficiency consequences, and the need for supplementation while considering the risk of micronutrient overload. To maximize the potential benefits while minimizing the risk of nutrient overload, although knowledge gaps remain.
Pregnancy is a period of rapid and extensive physiological changes that increase maternal nutritional requirements (1, 2). The first 1,000 days of a newborn’s life (from gestation to second birthday) are considered the “golden opportunity” for determining their health in life (3). Therefore, the nutritional status of the mother before and during pregnancy has a significant and irreversible impact on the growth of infants including birth weight, as well as the mother’s health (4). Adequate macronutrients and micronutrients are mandatory to promote healthy gestation. Their deficiency can trigger a state of biological competition between the mother and infant (5), leading to complications such as anemia and hypertension, as well as impaired function, growth, and development in the infant. In addition to negative pregnancy outcomes, micronutrient deficiencies can also affect adulthood, intergenerational health, morbidity, and infant mortality (6, 7).
Every 2 minutes, a woman dies during pregnancy or childbirth, revealing alarming setbacks for women’s health in recent years. Among the most essential micronutrients, the four most vital ones are (1) iron, (2) folic acid/B9, (3) calcium, and (4) iodine (see graphical abstract). Iron is the most critical nutrient in the body because it helps in the production of hemoglobin and myoglobin and the metabolism of proteins (8–10). Insufficient iron intake can cause anemia and iron deficiency anemia. Globally, iron deficiency is affecting about 45 million pregnant women (11–13). That is why the idea that iron supplementation should be universal during pregnancy is under discussion (14–16). However, iron supplementation can also cause some gastrointestinal problems, like nausea (17). Folic acid is involved in the development of neural tube and protein metabolism and prevents the development of birth defects in the fetus, pre-eclampsia, spontaneous miscarriage, and placental anomalies in the mother (18–21). Despite the benefits of folic acid supplementation, its overconsumption can cause some adverse effects like increased insulin resistance and low levels of adiponectin in children, leading to childhood obesity (22). Adequate calcium intake is crucial for fetal bone development and reducing risks of preeclampsia and preterm delivery, which is why its intestinal absorption doubles in pregnancy (23–25). Hypocalcemia is not very common during pregnancy but occurs due to hypoparathyroidism and extremely low dietary calcium intake (26, 27). On the other hand, excessive calcium supplementation can lead to hypercalcemia and kidney stone risk (28, 29). Iodine is essential for thyroid hormone production, particularly during pregnancy due to increased maternal thyroid demand, a higher glomerular filtration rate, and fetal needs (30–35). According to the International Council for Control of Iodine Deficiency Disorders (ICCIDD), and the World Health Organization (WHO), the iodine requirement for pregnant and lactating women is 250 μg/day. However, excessive iodine intake can lead to thyroid disorders in both the mother and fetus, posing risks such as hypothyroidism, goiter, and negative pregnancy outcomes, including macrosomia (36–38). Therefore, women should consume micronutrient dosages according to their own country’s recommendations throughout gestation to support a healthy pregnancy and fetal growth (Table 1).
Keeping in view the above-given importance of these micronutrients, it is critical to have a deeper understanding of pregnancy nutritional requirements. Therefore, this review provides insights into sources, bioavailability, Recommended Dietary Allowance (RDA), causes and consequences of deficiency, and the need for supplementation while considering the danger of micronutrient overload of the four most vital nutrients during pregnancy: iron, folic acid, iodine, and calcium to assess and improve pregnant women’s health status.
In adult individuals, the amount of iron is about 35 to 45 mg per kg of body weight (39–41).
Iron plays a crucial role in the synthesis of myoglobin (in muscle tissue) and hemoglobin. Hemoglobin is used for the transportation of oxygen from the lungs to the tissues through red blood cells (8–10, 39). During pregnancy, iron requirements increase more than twice, particularly in the 2nd and 3rd trimesters. Consequently, the iron RDA for pregnant women is up to 27 mg per day (42–46). Mothers require an increased intake of iron during pregnancy due to three primary factors. Firstly, an increase in the amount of maternal blood and plasma; secondly, a baby demands iron for its current metabolic activities and stores iron reserves for the next 6 months after birth as breastmilk is low in iron; and lastly, the placenta needs a significant amount of iron because of its accelerated metabolic functioning (9, 41, 47–49). Moreover, the daily use of iron during pregnancy is associated with a reduced risk of low birth weight (50). The total iron requirement during pregnancy is expected to be 1,040 mg, with 300 mg for the fetus, 50 mg for the placenta, 450 mg for the increase of maternal red cell mass, and 240 mg for basal iron loss (51).
There are two different sources of dietary iron heme and no heme. Heme represents 40% of total iron in animal foods and exhibits a bioavailability range of 15 to 40% in the human body, indicating their greater ability to be absorbed and utilized (52). Moreover, non-heme iron is derived from plant-derived sources. Its bioavailability ranges from 1 to 15% and is more susceptible to alteration by various constituents present in food (53). Overall, the most well-known animal source of iron is the liver of the Bovidae family, which includes calf liver as well as liver from pigs, sheep, horses, and ducks. Other animal sources with great iron amounts are the kidney, the brewer’s yeast, and meats (54, 55).
Iron can only be absorbed when it is ferrous (Fe2+) or bound to a protein like heme. In the proximal duodenum, the low pH of gastric acid allows a ferric reductase enzyme, duodenal cytochrome B (Dcytb), to convert insoluble ferric (Fe3+) into absorbable ferrous ions (Fe2+). In enterocytes, iron can be stored as ferritin or transported through the basolateral membrane and into circulation as ferroportin (56) as shown in Figure 1. The bioavailability of dietary iron is not optimal due to potential interactions with other nutrients in food, such as calcium (present in milk-based products like cheese), polyphenols (in caffeine-containing foods like tea and coffee), phytate (in whole-grain-containing products, e.g., bread), and oxalic acid (in beetroot), which decreases iron absorption (57, 58). Conversely, several nutrients, such as fructose, copper, vitamin A, beta-carotene, and vitamin C, have been found to boost the absorption of iron (59). Among these options, vitamin C is the most promising for enhancing the absorption of iron (60). The effect of vitamin C has been proven to be amount-dependent (61, 62) and can increase the absorption of iron only when both nutrients are consumed together (63). Moreover, it has been reported taking 500 mg of ascorbic acid with food increases the absorption of iron six times, but ascorbic acid taken 4–8 h before is less effective (64). Despite stability issues of vitamin C during food preparation, adding vitamin C to the diet appears to be a good way to increase iron consumption (65). However, some studies indicate that the addition of ascorbic acid to the whole diet has no significant impact on increasing iron absorption because of meal composition and the whole food matrix in the diet as shown in Figure 2 (66–69). Furthermore, in order to maximize absorption of oral iron supplements, women should take it on an empty stomach, 1 h before meals, with a source of Vitamin C (ascorbic acid) such as orange. Mothers should also avoid taking other medications such as antacids at the same time because they may impair absorption (70).
Iron insufficiency is a prevalent global issue, impacting over 2 billion individuals. This includes approximately 30 % of pregnant women in developed nations, while this prevalence is even higher in underdeveloped nations (8). Women of reproductive age, especially vegans or vegetarians, obese women, those suffering from inflammatory bowel disease (IBD), and endurance athletes are at a higher risk of iron deficiency. Iron insufficiency is the predominant cause of anemia during pregnancy, which affects around 45 million pregnant women globally. Iron deficiency anemia (IDA) is a condition characterized by low levels of hemoglobin. As defined by the WHO, hemoglobin concentrations below 110 g per liter during the 1st and 3rd trimesters or below 105 g per liter during the 2nd trimester are indicative of IDA (11–13, 71–76).
During pregnancy, iron deficiency anemia has adverse effects on both the mother’s and fetus’ well-being. In the fetus, ID and/or IDA have been associated with the risk of premature infants, low birth weight (LBW), or small for gestational age (SGA) infants, and even increased morbidity and fetal death (9, 15, 77). Mothers with iron deficiency might experience difficulties with breathing, fainting, fatigue, palpitations, and sleep disturbances (47–49).
In 2012, the Global Nutrition Target 2025 aimed for a 50% reduction in the prevalence of anemia among women of the reproductive age group (78) Unfortunately, according to the 2021 Global Nutrition Report, there has been limited global progress toward meeting the target. On the contrary, a projected estimate based on the current trends details an expected prevalence in 2025 of more than double the set target level (31.2% rather than 14.3%) (79).
There are several biomarkers available by which we can detect iron deficiency. Hemoglobin levels have been utilized as a means of assessing iron status for centuries, particularly in low-resource nations, due to its simplicity and affordability. Nevertheless, it is deficient in detecting the initial stage of iron insufficiency (80). There are several other iron-specific biomarkers, including serum ferritin, total iron binding capacity saturation (%TSAT), and hepcidin, which can specifically differentiate iron deficiency anemia (IDA) from other causes of anemia (81). All these biomarkers have their pros and cons. as you can see in Table 2.
Nutritional surveys in the United Kingdom and Norway showed that women’s dietary patterns change little with pregnancy (82, 83). Moreover, only 30 percent of dietary iron can be effectively absorbed even in ideal circumstances, relying solely on dietary iron is not practical for meeting the increased iron requirements during pregnancy. WHO recommends pregnant women to take 30 mg to 60 mg of elemental iron and 400 μg (0.4 mg) of folic acid supplement to prevent maternal anemia, puerperal sepsis, low birth weight, and preterm birth. The equivalent of 60 mg of elemental iron is 300 mg ferrous sulfate heptahydrate, 180 mg ferrous fumarate or 500 mg of ferrous gluconate (84). Preventative iron supplementation during pregnancy leads to a significant reduction of 70% in maternal anemia. Hence, pregnant women should consider the utilization of iron supplements, the appropriate dosage of which should be determined based on the prevalence of maternal anemia within their specific geographic area (14–16). When iron insufficiency is more than 40% of the population WHO recommends 60 mg of daily oral elemental iron and when it is less than 40% WHO recommends a lesser dose of 30 mg daily (85). There are 17 trials (N = 24,023) on maternal iron supplementation conducted by the US Preventive Services Task Force. Iron supplementation reduced the risk of maternal iron deficiency anemia at term (4 trials, n = 2,230, 8.6% vs. 19.8%) and maternal iron deficiency at term (6 trials, n = 2,361, 46% vs. 70%) as compared to placebo or no iron supplement. But were no statistically significant differences in maternal quality of life and maternal health compared with placebo or no supplementation (86). Furthermore, one meta-analysis indicates that intermittent oral iron supplementation with a dose of 120 mg/day is more effective than daily oral iron supplementation with a dose of 60 mg/day in increasing hemoglobin levels among pregnant women, with lesser side effects. Crucially, this approach is linked with a significant reduction in adverse effects related to iron supplementation. That is why intermittent oral iron supplementation is recommended for those individuals who are not able to adhere to the daily regime due to adverse events (87).
However, women with severe anemia (hemoglobin 8.5 g/dL or ferritin 30 ug/L) should use intravenous iron, which appears to be an effective and more secure alternative because oral iron therapy could trigger adverse reactions in them. A meta-analysis has also confirmed that women receiving iron through IV reach their targeted Hb levels more often and quickly with fewer side effects The well-tolerated nature of intravenous iron (IV) is attributed to the existence of type II iron complexes (88).
Due to the higher prevalence of iron deficiency policymakers are discussing whether universal iron supplementation should be adopted as a policy for pregnant women. In this regard, the Office of Dietary Supplements (ODS) of the National Institutes of Health (NIH) recently organized a seminar to evaluate the requirement for iron supplementation treatment among expecting women who already have enough iron (89–91). Hence, further research is needed, before the implementation of this policy (14–16, 92).
There are other interventions to address iron deficiency including iron fortification of staple foods, including wheat, maize, and rice according to the dietary habits of the affected population group is regarded as the most cost-effective long-term approach to reduce the prevalence of anemia especially in low-income countries (93, 94). The final possible strategy for anemia management is nutritional counseling. According to the systematic review, a study in which only counseling was used turned out to be effective in anemia prevention and management (95). The World Health Organization advises pregnant women to get counseling about healthy eating and keeping physically active during pregnancy (85).
Moreover, iron supplementation can cause some undesirable effects, like oral supplementation, which may induce some common gastrointestinal symptoms, including vomiting, bloating, abdominal pain, diarrhea, the presence of blackish or tarry stools, and constipation (17), while the administration of intravenous (IV) iron can lead to adverse effects such as injection site irritation, skin discoloration, general discomfort, and a metallic taste (73).
One study indicated that there is an increase in the risk of gestational diabetes as a result of iron supplementation in non-anemic women. Zhang et al. concluded in a prospective study that more than 30 mg of elemental oral iron daily for more than 3 months periconceptionally increased the risk of gestational diabetes (96); however, meta-analyses have shown that this is not the case (97). Overall, Women should consume individualized iron supplementation doses based on their geographic region anemia prevalence throughout the pregnancy to enhance iron levels and outcomes may serve as a financially effective intervention. This approach would help limit the risks associated with iron excess and its potential adverse effects (42–44, 74).
Folate, also referred to as vitamin B9, represents both its natural and synthetic form., Folate plays a vital role in various physiological processes. These processes comprise the production of nucleotides, the repair and methylation of DNA, and protein metabolism (e.g., the breakdown of homocysteine). Pregnant women require 5 to 10 times more folate compared to nonpregnant women due to increased fetal development, placental growth, uterine expansion, and higher blood volume (18–21). The recommended intake value for folate is 500–600 μg/d for pregnant women in different countries (98).
Hence, it is crucial to ensure that the recommended daily intake of folate for pregnant women must be fulfilled either through dietary sources or through supplementation throughout pregnancy (99). Natural sources of folate include a variety of food items, like green leafy vegetables (e.g., asparagus and broccoli), citrus fruit, legumes, yeast, lima beans, and organ meats (e.g., beef liver) (100, 101).
To be absorbed, folates must be converted enzymatically into folate monoglutamates by folate reductase in the jejunal mucosa. In the liver, folate monoglutamate is converted to dihydrofolate (by an enzyme called dihydrofolate synthase) and to tetrahydrofolate (by an enzyme called dihydrofolate reductase). Tetrahydrofolate is converted into 5,10-methylenetetrahydrofolate by serine hydroxymethyltransferase 5 indicated in Figure 1 (102). The absorption of folate from dietary sources is not efficient as 50 to 75 percent of the folate content is lost during the production and processing of food. Folate insufficiency can also arise due to diminished absorption or metabolism, as well as greater requirements or utilization of folate. In this regard, it has been observed that folic acid exhibits a twofold higher ability for absorption in comparison to folate. It has been found that folic acid supplements show 85% bioavailability when consumed with a meal and 100% bioavailability on an empty stomach (101, 103). Moreover, the effect of the food matrix and the type and amount of folic acid consumed also influence folic acid bioavailability as shown in Figure 2 (104). To account for the bioavailability difference between folate and folic acid, scientists developed a way to compare the two, known as daily folate equivalents (DFE). DFEs adjust for the nearly 50 percent lower bioavailability of food folate compared with that of folic acid: 1 μg of dietary folate equivalent = 0.6 μg of folic acid from fortified food or as a supplement taken with meals = 1 μg of food folate = 0.5 μg of a supplement taken on an empty stomach (105).
Pregnant women may exhibit a greater vulnerability to folate insufficiency because of their elevated physiological demand for folate, as epigenetic processes are observed to take place in both the placenta and the fetus, and certain hormones (estrogen and progesterone) exhibit increased concentrations, which in turn reduce the absorption of folate during pregnancy (73, 106, 107). Women of fertile age, especially in underdeveloped countries, are susceptible to folic acid insufficiency (108), particularly where malaria and sickle-cell anemia are common (109), as well as those with inflammatory bowel disease who use alcohol or tobacco or who use antifolates, anti-epileptics, and anti-inflammatory drugs (IBD) (101, 103).
Insufficient folic acid during pregnancy has the potential to adversely affect both maternal and fetal health. In the developing fetus, inadequate folic acid can cause many irreversible adverse effects, such as congenital disorders, fetal growth restriction, and premature infants. Congenital anomalies, sometimes also referred to as birth defects, have the potential to impact many organ systems within the human body. Congenital heart abnormalities (CHDs) and neural tube defects (NTDs) are the predominant classifications of congenital anomalies. Neural tube defects (NTDs) include two unique congenital malformations, specifically spina bifida and anencephaly. Spina bifida is a congenital condition characterized by improper growth of the spinal cord, whereas anencephaly is a congenital anomaly characterized by the lack of brain and cranial structures in a neonate. Both illnesses have a high mortality rate. In mothers, insufficient levels of folic acid can lead to serious difficulties, such as the occurrence of pre-eclampsia, spontaneous miscarriage, and various placental malformations, including abruption (110–113).
There are two indications utilized in the assessment of folate deficiency: serum folate concentrations and red blood cell (RBC) folate concentrations. Serum folate concentrations are indicative of recent food intake, while red blood cell (RBC) folate concentrations reflect the body’s stores of folate. Serum or plasma folate levels below 4 nanograms per milliliter or 10 nanomoles per Liter and red blood cell folate levels below 151 nanograms per milliliter (ng/mL; or 340 nanomoles per liter, nmol/L) are indicative (114).
Meta-analysis and systematic review have indicated a significant association between maternal folic acid intake and the risk of congenital anomalies. In particular, children whose mothers took periconceptional folic acid supplementation were 77% less likely to have congenital defects (115). WHO recommends 400 μg (0.4 mg) of folic acid as early as possible during pregnancy (ideally before conception) to prevent neural tube defects (84). Both dosage and timing are crucial factors to consider in folic acid supplementation. This is due to the neural tube closing on day 28 following conception, a period during which pregnancy may not yet be diagnosed as 41% of pregnancies worldwide are unintended. Hence, the initiation of folic acid treatment beyond the first month of pregnancy fails to offer sufficient efficacy in the prevention of neural tube defects (114, 116–118). To address this issue some governments adopted mandatory folic acid fortification of cereal products, mainly flour. Folic acid fortification would prevent approximately 40–50% of NTD cases by raising average plasma folate levels from baseline levels of approximately 5 ng/mL to, say, 20 ng/mL. If the level was at 40 ng/mL, which is achieved by 4 mg of folic acid per day, it would be almost 80% (119). In 2,000 Chile introduced 0.22 milligrams of folic acid per 100 grams of wheat. By doing so the average daily folic acid consumption increased by 0.43 mg a day, resulting in a 43% reduction in NTD risk (120). But after 9 years Chile reduced the fortification level to 0.18 milligrams per 100 gram wheat flour due to theoretical safety concerns and this resulted in a 10% increase in the NTD (121). Moreover, after mandatory folic acid fortification in the United States and Canada, the occurrence of some NTD declined by 28% (122) and 46% (123). However, two studies by Dorise and Beringer found that only 20% (124) and 55% (125) of pregnant women in Australia met the folate recommendations from diet alone (including natural food folate and fortified foods), respectively. The United Nations International Multiple Micronutrient Antenatal Preparation (UNIMMAP) is a well-known multiple micronutrient formula containing 15 vitamins and minerals, containing iron and folic acid in recommended dosages. An analysis conducted by World Health Organization (WHO) finds that UNIMMAP-MMS lowers the risk of small for gestational age (SGA) and low birth weight (LBW) (126). An additional individual patient data (IPD) meta-analysis also finds MMS(multiple micronutrient supplements) lower the risk of stillbirth and preterm birth (127). Overall, food alone (even fortified food) is usually not enough for women to meet their daily folate needs, so supplements are often recommended.
Folate is regarded as non-toxic due to its water-soluble nature, which enables it to be eliminated from the body by the urinary system (128). Nevertheless, the consumption of folic acid over 1,000 mg/day has been associated with several negative consequences, such as an elevated risk of specific types of cancer, including breast, prostate, and colon cancer (42–44, 101, 129–131). Increased folic acid may also conceal b12 insufficiency, which contributes to megaloblastic anemia and neuropathy Additionally, it has the potential to induce zinc malabsorption, leading to subsequent deficits in immunological, neurological, and gastrointestinal functionality (42–44, 129, 131–133).
Several studies have identified a correlation between periconceptional folic acid (FA) supplementation and increased rates of twin pregnancies and miscarriages. Multiple births are linked to an increased risk of a few prenatal problems as well as infant morbidity and mortality (134). Moreover, folic acid supplementation does not affect the birth weight (135). WHO also recommends multivitamin supplements instead of iron-folic acid supplements alone to improve birth weight (74). Nevertheless, on the balance of benefits and risks, adequate folate intake through supplements and diet is advised for all women during preconception and pregnancy (109) (Table 3).
Table 3. The table represents a summary of key details on iron, folic acid, calcium, and iodine for pregnant women.
Calcium makes up 1 to 2% of total body mass and is the most abundant mineral in the body. It is vital for various physiological mechanisms as well as reactions such as bone formation, muscle contracting, hormone, and enzyme activity, and is a crucial intracellular component that maintains cell membranes and serves an imperative part in the functioning of nerve cells (136–138). Calcium demands increase during gestation as women lose stored calcium as a result of fetal skeleton development. Especially during the third trimester, when maximal calcium accretion occurs for rapid mineralizing of the fetal skeleton. Increased intestinal calcium absorption, renal calcium conservation, and mobilization of calcium from the maternal skeleton are the three potential calcium sources to assist fetal bone accretion (74, 133, 139, 140).
Calcium that is lost during gestation can only be substituted by dietary calcium consumption, foods fortified with either organic or inorganic calcium, and dietary supplements. Calcium citrate and calcium carbonate make up the largest proportion of calcium dietary supplements (133, 141). Milk, dairy goods, green leafy vegetables, fish with soft bones (e.g., sardines), and legumes are the richest dietary sources of calcium. For vegans, nuts, dried fruits, fortified soy milk, and soy products like tofu are excellent substitute dietary options for calcium (142).
There are two general mechanisms by which ingested calcium is absorbed by mammalian small intestines: transcellular active transport in the duodenum and upper jejunum, and paracellular passive transport throughout the intestine (143, 144). Cow’s milk and its derivatives are the highest-quality and most bioavailable sources of calcium. The calcium bioavailability of other animals’ milk, soy milk, and certain vegetables is comparable with that of cow’s milk. Some foods might have higher concentrations of calcium, yet their bioavailability fluctuates, as phytates and oxalates are absorbed less efficiently than carbohydrates. Vitamin D, carbohydrates, particularly lactose from milk and dairy products, L-lysine, L-arginine and ascorbic acid (vitamin C) have a synergistic impact on the uptake of calcium, whereas fiber-rich foods, fats, phosphates, protein (not completely understood), caffeine, and alcohol have an opposing effect as shown in Figure 2 (143, 145).
Calcium requirements range between 1,000 and 1,200 mg/day (146, 147). There is a rise in calcium needs during gestation which can be met via dietary intake however, taking calcium supplements at doses between 300 mg and 2,000 mg per day has been suggested (136). From the start of the 20th week of gestation until the end of pregnancy, pregnant women are recommended to get 1.5–2.0 g of mineral calcium per day if they are at elevated risk or in conditions where there is insufficient dietary intake of calcium. The total everyday amount should be split into three doses, ideally during meals (137, 148).
A complete prenatal calcium supplementation program that was piloted in the Dailekh area of Nepal was assessed in one study. In order to distribute and advise pregnant women on the use of calcium through government antenatal care (ANC) programs, the initiative trained medical professionals and community health volunteers. 94.6% of pregnant women in the district were covered by the calcium distribution through ANC, according to a survey of 1,240 recent postpartum women. To receive the entire 150-day course of calcium supplements, the majority of women (more than 80%) attended ANC early. Pregnant women reported high levels of acceptability, compliance (79.5% consumed the entire allowance), and coverage; almost all said they would be ready to recommend the calcium and use it in future pregnancies. The program also maintained consistent calcium availability, suggesting this universal free supplementation model can be successfully scaled up in other parts of Nepal (149).
It should be noted that adequate vitamin D status [circulating level of 25(OH)D being >80 nmol] in the body is critical for the regulation of intestinal calcium absorption and bone homeostasis. Also, vitamin K is necessary for the activation of Gla proteins which are also responsible for the regulation of calcium in the body (150–152).
Serum calcium, parathyroid hormone (PTH), and 1,25(OH)2D levels are essential biomarkers for determining calcium levels in the human system. 2.15–2.50 mmoL/L is considered to be the standard range for serum calcium. A rise in the concentrations of PTH and 1,25(OH)2D suggests that the body needs greater calcium absorption (153–156).
In 2011, about 51% of the global population, or approximately 3.5 billion individuals, were vulnerable to calcium deficiency, with 90% of this population residing in Africa and Asia. Even in High-Income nations, several demographics fail to comply with the recommendations (157, 158). Calcium deficiency during gestation can impact both the mother and fetus. Maternal calcium shortages during gestation have the potential to cause long-lasting impacts, such as insulin resistance in offspring. It is possible through the modification of many metabolic characteristics and the epigenetic control of gene expression (159). Additionally, it can result in pre-eclampsia, pregnancy-induced hypertension in mothers, and a considerable loss of bone minerals in the developing fetus (160–163).
There are several strategies that can be used to assist increase calcium intake. These include encouraging the intake of foods that are naturally high in calcium, fortifying staple foods, producing more calcium-containing crops through biofortification, and employing food processing methods that can increase calcium content or bioavailability. Policymakers who want to increase calcium intake can use these interventions. It is highly recommended that the coverage and health effects of such treatments be tracked and evaluated (164).
Women who ingest minimal to no milk or dairy products, vegans, high-performance athletes, Asian women having inadequate vitamin D status, those who take a high fiber diet, have allergies to cow’s milk or are lactose intolerant, have conditions like hypochlorhydric stomach, or have celiac disease are at risk of being calcium deficient (142, 158, 165–167). Additionally, a meta-analysis has demonstrated that in Low and middle-income countries, the dietary calcium intake during pregnancy is very low, unlike the High-income countries (25, 168). Low calcium intakes across Asian, African, and Latin American countries have been reported in a review (169, 170). The average intake of calcium in low- and middle -income countries was found to be 648 mg/day, whereas that from high-income countries was 948 mg/day (171, 172).
Like deficiency, overconsumption of calcium can also seriously affect both maternal and fetal health. For pregnant women between the ages of 19 and 50, the tolerable upper intake level (UL) of calcium is 2,500 mg. Calcium overconsumption can cause hypercalcemia (rare), which can lead to vascular and soft tissue calcification, nephrolithiasis, hypercalciuria (which also happens naturally during gestation), and other major maternal, fetal, and neonatal problems (24, 173, 174).
Iodine is a trace mineral that accounts for 0.00004% of the total human weight and is most concentrated in the thyroid gland, muscle, and numerous endocrine tissues (175). In thyroid glands, iodine serves as vital for the biogenesis of thyroid hormones that are necessary for the optimum functioning of almost all tissues and perform a crucial role in the regulation of growth, development, differentiation, and metabolic processes, significantly influencing the body’s metabolic activity and utilization of oxygen. Within the uterus, they are essential for fetal growth and differentiation (176, 177). Since there is an upsurge in hormone demands commencing in the initial 13 weeks of pregnancy, pregnant women experience dramatic changes in thyroid function and a hike in iodine needs (31, 178).
The primary source of iodine for humans is diet, although certain populations obtain it from water. Seaweed, marine-sourced fish and shellfish, eggs (particularly fortified), dairy products, livestock, and iodized salt are iodine-rich food sources. However certain categories of fish and seafood pose an elevated risk of contamination with parasitic organisms, pathogens, and toxic substances. Safe processing and proper handling can reduce this risk (179–185).
There are few studies regarding the bioavailability of iodine from diet, and these imply that the actual bioavailable quantity tends to be less than what is consumed. Upon being converted to iodide, the gastrointestinal tract swiftly absorbs iodine from food (186). Upon entering the circulation, iodide is absorbed in appropriate amounts by the thyroid gland and excreted in most amounts in the urine (187). However, the absorption efficacy by the gastrointestinal tract of consumed iodine is regarded as high (greater than 90%) as shown in Figure 1 (188). Seaweed contains abundant iodine concentrations. In vitro studies evaluating iodine absorption in different seaweeds have found that 49–82% of the iodine can be absorbed during digestion in the gut (189). Pasteurization has been shown to reduce the iodine level in milk, while sterilization has no impact on the iodine concentrations in milk (179–183). It has been shown that goitrogens in cruciferous vegetables and soy products, can interfere with the synthesis of thyroid hormones in a variety of ways, primarily by inhibiting the utilization of iodine as indicated in Figure 2 (190).
As a result of maternal thyroid stimulation, iodine requirements increase by around 50 percent during pregnancy, leaving both the mother and the developing fetus susceptible (181, 191). The 2007 recommendations of the World Health Organization (WHO), the United Nations Children’s Fund (UNICEF), and the International Council for the Control of Iodine Deficiency (ICCIDD) recommend a daily iodine consumption of 250 mcg during gestation (192). In iodine-deficient regions, controlled studies have shown that iodine supplemental intake before or throughout early gestation eradicates emerging instances of cretinism, increases birth weight, and mitigates the incidence of perinatal and infant fatalities. Also, it enhances developmental outcomes in youngsters by 10 to 20% (193).
A randomized, double-blind trial in Sweden found that providing a daily 150 μg iodine supplement to mildly iodine-deficient pregnant women improved their iodine status. The intervention group reached iodine sufficiency, with median urinary iodine concentrations (UICs) of 139 μg/L and 136 μg/L in the second and third trimesters, respectively. The intervention group also exhibited higher median UICs and lower median thyroglobulin levels compared to controls, without affecting other thyroid markers or neonatal outcomes (194).
There are two primary methods for administering supplemental iodine: daily administration of potassium iodide or yearly administration of a gradually released iodine formulation like iodized oil (195). Because the thyroid is capable of storing iodine, it appears that iodine before pregnancy is as essential as, if not more essential than, iodine during gestation (196). A cohort study found that lower iodine status during pregnancy and postpartum was associated with lower TSH and higher fT3 and fT4 concentrations. Initiating iodine-containing supplements before and during pregnancy was associated with more optimal thyroid function compared to no supplement use. These results support the importance of optimizing iodine intake before pregnancy (197). Iodine supplemental intake in the form of multivitamins potassium iodide containing 150 μg of iodine is prescribed as early as the onset of pregnancy and even sooner in planned pregnancies (198–201).
In 2009, Australia introduced mandatory iodized salt fortification in bread to address mild iodine deficiency. A study analyzed the program’s impact on iodine intake using 2011–2012 national dietary data, finding that the fortification effectively achieved adequate iodine levels across socioeconomic and geographic groups, with significant benefits for women of childbearing age and children (202).
Serum iodine concentration (SIC) and thyroglobulin (Tg) are important iodine metabolism biomarkers. Urinary iodine is also an accurate marker for recent iodine consumption as urinary iodine excretion indicates over 90 percent of dietary iodine intake in a constant state (203–205). For Median urinary iodine concentration (mUIC), in 2007, WHO, UNICEF, and ICCIDD updated the epidemiological guidelines of iodine levels for women during pregnancy and suggested the general public median UIC value as the assessing marker having an adequate level range of 150–249 g/L (206, 207). Serum levels of thyroid hormones are measured to evaluate the thyroid’s functioning and iodine status. Total T4 (TT4) determination is preferable over free T4 (FT4) determination, particularly during the final stages of gestation. The adjustment of the TT4 in the gestational period by a factor of 1.5 relative to nonpregnant benchmarks gives a practical FT4 measurement. For actual practice, the nonpregnant baseline range of 4.5–12.5 g/dL will change to 6.75–18.75 g/dL (208, 209).
Nearly 2 billion individuals worldwide are suffering from iodine deficiency (ID), with roughly 50 million exhibiting medical symptoms (210). Overall, 53% of the pregnant population has insufficient iodine intake (211). The insufficiency of iodine is widespread in the majority of countries. Among these countries, Africa and Southeast Asia are the most affected (212). Iodine deficiency also places some developed countries at risk, including continental Europe, the United States, Australia, the Republic of Ireland, and the United Kingdom (213). For women of reproductive age, appropriate iodine levels are crucial. It must be taken into account that not only severe prenatal deficient levels of iodine but also minimal to significant shortfalls are all linked to negative effects arising in the progeny (214).
As soon as the daily intake of iodine declines below 100 μg, iodine deficiency during gestation gets usually serious (195). Iodine deficiency during pregnancy may cause low thyroid hormone levels in both mother and fetus, leading to birth complications and impaired brain development in the baby, increasing the risk of perinatal death (215–218). Additionally, there is mounting evidence that iodine deficiency in pregnancy has an adverse and irreversible effect on the neurocognitive development of the embryo, particularly when the shortage occurs during the first trimester (219–222). A cohort study investigated the impact of maternal iodine status on offspring cognitive outcomes. Mother–child pairs from the Avon Longitudinal Study of Parents and Children (ALSPAC) cohort were examined by assessing urinary iodine content and creatinine to adjust for urine volume in preserved samples from 1,040 first-trimester pregnant women. Women were chosen based on having a singleton pregnancy and the availability of both a urine sample from the first trimester (defined as ≤13 weeks’ gestation; median 10 weeks [IQR 9–12]) and an intelligence quotient (IQ) assessment of the offspring at 8 years of age. The iodine-to-creatinine ratios in women were classified as <150 μg/g (deficient) or ≥ 150 μg/g (adequate) according to WHO pregnancy standards.
Mild-to-moderate iodine deficiency was observed in the cohort (median urinary iodine: 91.1 μg/L). Maternal iodine-to-creatinine ratios <150 μg/g were associated with increased odds of low verbal IQ, reading accuracy, and comprehension scores in children. A dose–response relationship was evident, with cognitive outcomes worsening as iodine levels decreased. Results suggested that even mild-to-moderate iodine deficiency during pregnancy may adversely affect children’s cognitive development, highlighting the importance of adequate iodine nutrition in pregnancy (223).
However, no effects were found on preterm birth, low birth weight, and hypertensive disorders among euthyroid pregnant women with different urinary iodine concentrations (UIC). Another systematic review confirmed no effects of maternal UIC on anthropometric measures of new born. Moreover, no link was found between maternal urinary iodine and the brain morphology of the infant (224–226).
Deficiency of iodine can result from restricted salt intake as a preventative measure against numerous noncommunicable diseases, a Paleolithic or low salicylate diet, an eating pattern with generally reduced dairy intake, an excessive intake of industrial foods that may not have iodized salt, or any poorly balanced diet (214). Due to the limited iodine quantity found in plant-based foods, vegans and vegetarians are also vulnerable to iodine deficiency (227). Moreover, smoking and iodine exhibited a statistically significant negative interaction (228).
Likewise, excessive iodine consumption during the gestational period may result in adverse outcomes. The upper intake level of iodine is 1,100 mcg/day (85, 229). There is a potential for adverse consequences associated with excessive consumption of iodine during pregnancy, and the suggested UI of iodine consumption is disputed. Ingestion of iodine supplementary products, water, diet, or medication containing an excessive amount of iodine, as well as exposure to objects having iodine, like radiological contrast material and disinfecting agents, might result in more than iodine. Hyperthyroidism, hypothyroidism, an increased risk of subclinical hypothyroidism, and an elevated risk of isolated hypothyroxinemia may result from abundant iodine (36–38). Along with causing fetal hypothyroidism and goiter and being a distinct risk factor for macrosomia, too much iodine consumption may be associated with additional negative pregnancy outcomes (230–232) (Table 2).
Pregnancy is the most crucial stage in a woman’s life, and diet during this period determines the health of the mother and newborn. Ideal micronutrient intake is often neglected during this time, as the major focus is often on the increased calorie requirements. Micronutrient deficiencies can lead to some serious health issues for the mother and fetus and also increase the fetal mortality risk. This review covers all aspects related to the consumption of four major micronutrients iron, folic acid, iodine, and calcium during pregnancy including their dietary sources and factors that affect their bioavailability. Ideally, their requirements should be met by dietary sources but their increased requirements during pregnancy demand other means, like the use of supplements. These supplements must be given according to the individual needs and RDAs set by the WHO. Because not only deficiency but also overconsumption has serious health implications. This emphasizes the need for a thorough nutritional assessment of pregnant women which helps decide whether there is a need to take supplements or whether the requirements can be fulfilled by dietary sources.
This review helps the readers to understand the importance of sufficient micro-nutrient intake for a healthy gestation period. To increase awareness, there must be educational programs and training for not only pregnant women but all women of reproductive age on maintaining the ideal body weight and eating for a healthy future generation. More programs should be designed for populations that are at risk of deficiency, like introducing supplemented or fortified foods. Cost, access, and availability are important factors to be considered before implementing an intervention in underdeveloped or developing countries.
There is a lack of studies with multiple micronutrient supplements that evaluate their combined effects, including synergistic and antagonistic interactions between them as well as the underlying mechanisms of these interactions. Furthermore, for pregnant women taking certain medications like blood-thinning medicines, we need to study in detail the effects of medicines on micronutrients’ bioavailability and other pregnancy outcomes. It is also crucial to do research on pre-conceptional diet and set RDAs for women trying for conception. Moreover, samples used in these studies should be alike to avoid variations in results due to various factors like the age of the mother at the time of conception, weight before and during pregnancy, gap between successive pregnancies, multiple pregnancies, presence of any infertility syndrome like polycystic ovarian syndrome and family history of congenital disorders. It is also required to study twin or triplet pregnancies separately to set RDAs for women having multiple gestation. Hence, to date, there are a lot of knowledge gaps to cover to gain a proper understanding of micronutrient intake during pregnancy.
NF: Conceptualization, Data curation, Formal analysis, Writing – original draft, Writing – review & editing. SY: Conceptualization, Data curation, Project administration, Writing – original draft, Writing – review & editing. LR: Conceptualization, Data curation, Investigation, Writing – original draft. AI: Data curation, Investigation, Software, Validation, Writing – original draft, Writing – review & editing. MI: Data curation, Software, Writing – review & editing. ZB: Investigation, Data curation, Writing – review & editing. AS: Writing – review & editing, Validation. HN: Writing – review & editing, Data curation, Validation. WS: Writing – review & editing, Software. MA: Data curation, Software, Writing – review & editing. ZK: Investigation, Software, Writing – review & editing. FA-A: Writing – review & editing, Project administration. QS: Writing – review & editing, Supervision, Resources. YM: Project administration, Resources, Supervision, Validation, Writing – original draft, Writing – review & editing.
The author(s) declare that financial support was received for the research and/or publication of this article. The research was funded by Deanship of Scientific Research at King Faisal University under project number KFU241765.
The authors declare that the research was conducted in the absence of any commercial or financial relationships that could be construed as a potential conflict of interest.
All claims expressed in this article are solely those of the authors and do not necessarily represent those of their affiliated organizations, or those of the publisher, the editors and the reviewers. Any product that may be evaluated in this article, or claim that may be made by its manufacturer, is not guaranteed or endorsed by the publisher.
1. Bader, RA, Bader, ME, Rose, DJ, and Braunwald, E. Hemodynamics at rest and during exercise in normal pregnancy as studied by cardiac catheterization. J Clin Invest. (1955) 34:1524–36. doi: 10.1172/JCI103205
2. Pipkin, F, Chamberlain, G, and Steer, P. Maternal physiology in pregnancy In: G Chamberlain, PJ Steer, editors. Turnbulls obstetrics. third ed. Churchil, Livingstone, Sydney, Toronto: Churchill Livingstone (2001). 71–91.
3. Cusick, SE, and Georgieff, MK. The role of nutrition in brain development: the golden opportunity of the “first 1000 days”. J Pediatr. (2016) 175:16–21. doi: 10.1016/j.jpeds.2016.05.013
4. Grieger, JA, Grzeskowiak, LE, and Clifton, VL. Preconception dietary patterns in human pregnancies are associated with preterm delivery. J Nutr. (2014) 144:1075–80. doi: 10.3945/jn.114.190686
5. King, JC. The risk of maternal nutritional depletion and poor outcomes increases in early or closely spaced pregnancies. J Nutr. (2003) 133:1732S–6S. doi: 10.1093/jn/133.5.1732S
6. Anderson, AS. Pregnancy as a time for dietary change? Proc Nutr Soc. (2001) 60:497–504. doi: 10.1079/PNS2001113
7. Srivastava, S, Mehrotra, P, Srivastava, S, and Siddiqui, M. Some essential elements in maternal and cord blood in relation to birth weight and gestational age of the baby. Biol Trace Elem Res. (2002) 86:97–105. doi: 10.1385/BTER:86:2:097
8. Beard, JL. Effectiveness and strategies of iron supplementation during pregnancy. Am J Clin Nutr. (2000) 71:1288S–94S. doi: 10.1093/ajcn/71.5.1288s
9. Cairo, G, Bernuzzi, F, and Recalcati, S. A precious metal: Iron, an essential nutrient for all cells. Genes Nutr. (2006) 1:25–39. doi: 10.1007/BF02829934
10. Gupta, CP. Role of Iron (Fe) in body. IOSR J Applied Chem (IOSR-JAC). (2014) 7:38–46. doi: 10.9790/5736-071123846
11. Yadav, P, Das, P, Mirdha, BR, Gupta, SD, Bhatnagar, S, Pandey, RM, et al. Current spectrum of malabsorption syndrome in adults in India. Indian J Gastroenterol. (2011) 30:22–8. doi: 10.1007/s12664-011-0081-0
12. Pasricha, S-RS, Flecknoe-Brown, SC, Allen, KJ, Gibson, PR, McMahon, LP, Olynyk, JK, et al. Diagnosis and management of iron deficiency anaemia: a clinical update. Med J Aust. (2010) 193:9–525. doi: 10.5694/j.1326-5377.2010.tb04038.x
13. Bayraktar, UD. Treatment of iron deficiency anemia associated with gastrointestinal tract diseases. World J Gastroenterol. (2010) 16:2720. doi: 10.3748/wjg.v16.i22.2720
14. Fogelholm, M. New Nordic nutrition recommendations are here. Food Nutr Res. (2013) 57:22903. doi: 10.3402/fnr.v57i0.22903
15. Peña-Rosas, JP, De-Regil, LM, Garcia-Casal, MN, and Dowswell, T. Daily oral iron supplementation during pregnancy. Cochrane Database Syst Rev. (2015) 2015:1–527. doi: 10.1002/14651858.CD004736.pub5
16. Laganà, AS, Costabile, L, Filati, P, Noventa, M, Vitagliano, A, and D'Anna, R. Effects of micronised dispersible ferric pyrophosphate combined with alpha-lactalbumin in pregnant women affected by iron deficiency anemia: results from a prospective, double-blind, randomized controlled trial. Eur Rev Med Pharmacol Sci. (2018) 22:3602–8. doi: 10.26355/eurrev_201806_15187
17. Tolkien, Z, Stecher, L, Mander, AP, Pereira, DIA, and Powell, JJ. Ferrous sulfate supplementation causes significant gastrointestinal side-effects in adults: a systematic review and meta-analysis. PLoS One. (2015) 10:e0117383. doi: 10.1371/journal.pone.0117383
18. Antony, AC. In utero physiology: role of folic acid in nutrient delivery and fetal development. Am J Clin Nutr. (2007) 85:598S–603S. doi: 10.1093/ajcn/85.2.598S
19. Czeizel, AE, and Dudás, I. Prevention of the first occurrence of neural-tube defects by Periconceptional vitamin supplementation. N Engl J Med. (1992) 327:1832–5. doi: 10.1056/NEJM199212243272602
20. Kim, JM, Hong, K, Lee, JH, Lee, S, and Chang, N. Effect of folate deficiency on placental DNA methylation in hyperhomocysteinemic rats. J Nutr Biochem. (2009) 20:172–6. doi: 10.1016/j.jnutbio.2008.01.010
21. Tamura, T, and Picciano, MF. Folate and human reproduction. Am J Clin Nutr. (2006) 83:993–1016. doi: 10.1093/ajcn/83.5.993
22. Popkin, BM, and CM, D. The obesity epidemic is a worldwide phenomenon. Nutr Rev. (1998) 56:106–14. doi: 10.1111/j.1753-4887.1998.tb01722.x
23. Villar, J, Abdel-Aleem, H, Merialdi, M, Mathai, M, Ali, MM, Zavaleta, N, et al. World Health Organization randomized trial of calcium supplementation among low calcium intake pregnant women. Am J Obstet Gynecol. (2006) 194:639–49. doi: 10.1016/j.ajog.2006.01.068
24. Hacker, AN, Fung, EB, and King, JC. Role of calcium during pregnancy: maternal and fetal needs. Nutr Rev. (2012) 70:397–409. doi: 10.1111/j.1753-4887.2012.00491.x
25. Hofmeyr, GJ, Lawrie, TA, Atallah, Á, and Torloni, MR. Calcium supplementation during pregnancy for preventing hypertensive disorders and related problems. Cochrane Database Syst Rev. (2018) 2018. doi: 10.1002/14651858.CD001059.pub5
26. Stabile, I, Chard, T, and Grudzinkas, G. Clinical obstetrics and gynaecology. London, UK: Springer Science & Business Media (2012).
27. Mahadevan, S, Kumaravel, V, and Bharath, RJ. Metabolism, Calcium and bone disorders in pregnancy. Indian J Endocrinol Metabolism. (2012) 16:358–63. doi: 10.4103/2230-8210.95665
28. Smith, C, Kristensen, C, Davis, M, and Abraham, P. An evaluation of the physicochemical risk for renal stone disease during pregnancy. Clin Nephrol. (2001) 55:205–11.
29. Del Valle, HB, Yaktine, AL, Taylor, CL, and Ross, AC. Dietary reference intakes for calcium and vitamin D. Washington, DC, United States: National Academies Press (2011).
30. Yarrington, C, and Pearce, EN. Iodine and pregnancy. J Thyroid Res. (2011) 2011:934104:1–8. doi: 10.4061/2011/934104
31. Glinoer, D. Pregnancy and iodine. New York, United States: Mary Ann Liebert, Inc; (2001), 11, 471–481.
32. Hershman, JM. Physiological and pathological aspects of the effect of human chorionic gonadotropin on the thyroid. Best Pract Res Clin Endocrinol Metab. (2004) 18:249–65. doi: 10.1016/j.beem.2004.03.010
33. Dafnis, E, and Sabatini, S. The effect of pregnancy on renal function: physiology and pathophysiology. Am J Med Sci. (1992) 303:184–205. doi: 10.1097/00000441-199203000-00011
34. de Escobar, GM, Obregón, MJ, and del Rey, FE. Iodine deficiency and brain development in the first half of pregnancy. Public Health Nutr. (2007) 10:1554–70. doi: 10.1017/S1368980007360928
35. Pearce, EN, Leung, AM, Blount, BC, Bazrafshan, HR, He, X, Pino, S, et al. Breast milk iodine and perchlorate concentrations in lactating Boston-area women. J Clin Endocrinol Metab. (2007) 92:1673–7. doi: 10.1210/jc.2006-2738
36. Leung, AM, and Braverman, LE. Consequences of excess iodine. Nature Rev Endocrinol: Nature Publishing Group. (2014) 10:136–42. doi: 10.1038/nrendo.2013.251
37. Shi, X, Han, C, Li, C, Mao, J, Wang, W, Xie, X, et al. Optimal and safe upper limits of iodine intake for early pregnancy in iodine-sufficient regions: a cross-sectional study of 7190 pregnant women in China. J Clin Endocrinol Metab. (2015) 100:1630–8. doi: 10.1210/jc.2014-3704
38. Zhao, W, Li, X, Xia, X, Gao, Z, and Han, C. Iodine nutrition during pregnancy: past, present, and future. Biol Trace Elem Res. (2019) 188:196–207. doi: 10.1007/s12011-018-1502-z
39. Andrews, NC. The iron transporter DMT1. Int J Biochem Cell Biol. (1999) 31:991–4. doi: 10.1016/S1357-2725(99)00065-5
40. Cumming, RLC. Disorders of iron metabolism. Practitioner. (1978) 221:184–275. doi: 10.1016/B978-0-12-161909-1.50012-8
41. Fisher, AL, and Nemeth, E. Iron homeostasis during pregnancy. Am J Clin Nutr. (2017) 106:1567S–74S. doi: 10.3945/ajcn.117.155812
43. Kominiarek, MA, and Rajan, P. Nutrition recommendations in pregnancy and lactation. Med Clin North Am. (2016) 100:1199–215. doi: 10.1016/j.mcna.2016.06.004
44. Medeiros, DM In: JJ Otten, JP Hellwig, and LD Meyers, editors. Dietary reference intakes: The essential guide to nutrient requirements. Washington, DC: The National Academies Press (2006)
45. Bothwell, TH. Iron requirements in pregnancy and strategies to meet them. Am J Clin Nutr. (2000) 72:257S–64S. doi: 10.1093/ajcn/72.1.257S
46. Viteri, FE. The consequences of iron deficiency and anemia in pregnancy. Adv Exp Med Biol. (1994) 352:127–39. doi: 10.1007/978-1-4899-2575-6_10
47. Oski, FA, and Naiman, JL. The hematologic aspects of the maternal-fetal... - Google scholar. Philadelphia, PA, USA: W.B. Saunders Company. (1982).
48. Cao, C. Reviews KOObN, undefined, pregnancy and iron homeostasis: an update. KO O'brien Nutrition Rev. (2013) 71:35–51. doi: 10.1111/j.1753-4887.2012.00550.x
49. McArdle, HJ, and Gambling, L, “Iron deficiency during pregnancy: the consequences for placental function and fetal outcome.” Cambridge org HJ McArdle, L Gambling, C. Kennedy. Proceedings of the Nutrition Society, 2014•cambridgeorg. (2012).
50. Haider, BA, Olofin, I, Wang, M, Spiegelman, D, Ezzati, M, and Fawzi, WW. Anaemia, prenatal iron use, and risk of adverse pregnancy outcomes: systematic review and meta-analysis. BMJ. (2013) 346:346. doi: 10.1136/bmj.f3443
51. EFSA Panel on Dietetic Products N, Allergies. Scientific opinion on dietary reference values for energy. EFSA J. (2013) 11:1-112. doi: 10.2903/j.efsa.2013.3005
52. Lombardi-Boccia, L, and Lucarini, LD. Meat and meat products consumption in Italy: contribution to trace elements, heme iron and selected B vitamins supply. Int J Vitam Nutr Res. (2004) 74:247–51. doi: 10.1024/0300-9831.74.4.247
53. Hunt, JR. Bioavailability of iron, zinc, and other trace minerals from vegetarian diets. Am J Clin Nutr. (2003) 78:633S–9S. doi: 10.1093/ajcn/78.3.633S
54. Luca, S, Laura, C, Maurizio, M, Claudio, M, Pierluigi, P, Angela, P, et al. LARN Livelli di Assunzione di Riferimento di Nutrienti ed energia per la popolazione italiana. IV Revisione. (2014)
55. Carruba, M, and Bracale, R. 2. AMBITO. Esami di stato 2011–2014: tracce, soluzioni e commenti critici (vol 2). Rome, Italy: Edizioni Studium. (2020).
56. Ems, T, St Lucia, K, and Huecker, MR. Biochemistry, iron absorption. United States: StatPearls Publishing LLC. (2017).
57. SandstroÈm, B. Micronutrient interactions: effects on absorption and bioavailability. Br J Nutr. (2001) 85:S181–5. doi: 10.1079/BJN2000312
58. Hurrell, R, and Egli, I. Iron bioavailability and dietary reference values1234. Am J Clin Nutr. (2010) 91:1461S–7S. doi: 10.3945/ajcn.2010.28674F
59. Briguglio, M, Hrelia, S, Malaguti, M, Lombardi, G, Riso, P, Porrini, M, et al. The central role of iron in human nutrition: From folk to contemporary medicine, vol. 12. Switzerland: MDPI AG. (2020).
60. Lynch, SR, and Stoltzfus, RJ. Iron and ascorbic acid: proposed fortification levels and recommended Iron compounds. J Nutr. (2003) 133:2978S–84S. doi: 10.1093/jn/133.9.2978S
61. Mao, X, and Yao, G. Effect of vitamin C supplementations on iron deficiency anemia in Chinese children. Biomed Environ Sci: BES. (1992) 5:125–9.
62. Davidsson, L, Walczyk, T, Morris, A, and Hurrell, RF. Influence of ascorbic acid on iron absorption from an iron-fortified, chocolate-flavored milk drink in Jamaican children. Am J Clin Nutr. (1998) 67:873–7. doi: 10.1093/ajcn/67.5.873
63. Cook, JD, and Reddy, MB. Effect of ascorbic acid intake on nonheme-iron absorption from a complete diet. Am J Clin Nutr. (2001) 73:93–8. doi: 10.1093/ajcn/73.1.93
64. Cook, JD, and Monsen, ER. Vitamin C, the common cold, and iron absorption. Am J Clin Nutr. (1977) 30:235–41. doi: 10.1093/ajcn/30.2.235
65. Cori, TO. Enhancers of iron absorption: ascorbic acid and other organic acids. Int J Vitam Nutr Res. (2004) 74:403–19. doi: 10.1024/0300-9831.74.6.403
66. Cook, JD, Watson, SS, Simpson, KM, Lipschitz, DA, and Skikne, BS. The effect of high ascorbic acid supplementation on body iron stores. United States: Elsevier Inc. (1984), 64, 721–726.
67. Malone, H, Kevany, J, Scott, J, O’Broin, S, and O’Connor, G. Ascorbic acid supplementation: its effects on body iron stores and white blood cells. Ir J Med Sci. (1986) 155:74–9. doi: 10.1007/BF02940053
68. Hunt, JR, Mullen, LM, Lykken, GI, Gallagher, SK, and Nielsen, FH. Ascorbic acid: effect on ongoing iron absorption and status in iron-depleted young women. Am J Clin Nutr. (1990) 51:649–55. doi: 10.1093/ajcn/51.4.649
69. Hunt, JR, Gallagher, SK, and Johnson, L. Effect of ascorbic acid on apparent iron absorption by women with low iron stores. Am J Clin Nutr. (1994) 59:1381–5. doi: 10.1093/ajcn/59.6.1381
70. Pavord, S, Myers, B, Robinson, S, Allard, S, Strong, J, Oppenheimer, C, et al. UK guidelines on the management of iron deficiency in pregnancy. Br J Haematol. (2012) 156:588–600. doi: 10.1111/j.1365-2141.2011.09012.x
71. McLean, E, Cogswell, M, Egli, I, Wojdyla, D, and de Benoist, B. Worldwide prevalence of anaemia, WHO vitamin and mineral nutrition information system, 1993–2005. Public Health Nutr. (2009) 12:444. doi: 10.1017/S1368980008002401
72. Organization WH. Haemoglobin concentrations for the diagnosis of anaemia and assessment of severity. Geneva: World Health Organization. (2011).
73. Sifakis, S, and Pharmakides, G. Anemia in Pregnancy. Ann N Y Acad Sci. (2000) 900:125–36. doi: 10.1111/j.1749-6632.2000.tb06223.x
74. Parisi, F, di Bartolo, I, Savasi, VM, and Cetin, I. Micronutrient supplementation in pregnancy: Who, what and how much? Obstetric Med. (2019) 12:5–13. doi: 10.1177/1753495X18769213
75. Hawk, SN, Englehardt, KG, and Small, C. Risks of iron deficiency among vegetarian college women. Health. (2012) 4:113–9. doi: 10.4236/health.2012.43018
76. Unicef, U, and WHO, U. WHO: Iron deficiency anaemia: assessment, prevention, and control. A guide for programme managers. Geneva, Switzerland: World Health Organization (WHO) and UNICEF. (2001).
77. Domellöf, M, Braegger, C, Campoy, C, Colomb, V, Decsi, T, Fewtrell, M, et al. Iron requirements of infants and toddlers. J Pediatr Gastroenterol Nutr. (2014) 58:119–29. doi: 10.1097/MPG.0000000000000206
78. Organization WH. Global nutrition targets 2025: Stunting policy brief. Geneva, Switzerland: World Health Organization (2014).
79. Di Cesare, M, Ghosh, S, Osendarp, S, and Mozaffarian, D. A world free from malnutrition: An assessment of progress towards the global nutrition targets. Global Nutrit Report: The state of global nutrition. (2021):20–34.
80. Georgieff, MK. Iron deficiency in pregnancy. Am J Obstet Gynecol. (2020) 223:516–24. doi: 10.1016/j.ajog.2020.03.006
81. Demikhov, VG, Morshchakova, EF, Demikhova, EV, Isakova, OV, Turchannikova, EV, and Ayrapetova, VA. Iron deficiency in pregnancy. Pediatric Hematol/Oncol Immunopathol. (2002) 1:21–3.
82. Trygg, K, Lund-Larsen, K, Sandstad, B, Hoffman, HJ, Jacobsen, G, and Bakketeig, LS. Do pregnant smokers eat differently from pregnant non-smokers? Paediatr Perinat Epidemiol. (1995) 9:307–19. doi: 10.1111/j.1365-3016.1995.tb00147.x
83. Crozier, SR, Robinson, SM, Godfrey, KM, Cooper, C, and Inskip, HM. Women’s dietary patterns change little from before to during pregnancy. J Nutr. (2009) 139:1956–63. doi: 10.3945/jn.109.109579
84. World Health Organization. Daily iron and folic acid supplementation during pregnancy. Geneva, Switzerland: WHO (2024).
85. Organization WH. WHO recommendations on antenatal care for a positive pregnancy experience. Geneva, Switzerland: World Health Organization (2016).
86. Nicholson, WK, Silverstein, M, Wong, JB, Chelmow, D, Coker, TR, Davis, EM, et al. Screening and supplementation for iron deficiency and iron deficiency anemia during pregnancy: US preventive services task force recommendation statement. JAMA. (2024) 332:906–13. doi: 10.1001/jama.2024.15196
87. Banerjee, A, Athalye, S, Shingade, P, Khargekar, V, Mahajan, N, Madkaikar, M, et al. Efficacy of daily versus intermittent oral iron supplementation for prevention of anaemia among pregnant women: a systematic review and meta-analysis. Eclinicalmedicine. (2024) 74:102742. doi: 10.1016/j.eclinm.2024.102742
88. Govindappagari, S, and Burwick, RM. Treatment of iron deficiency anemia in pregnancy with intravenous versus oral iron: systematic review and meta-analysis. Am J Perinatol. (2019) 36:366–76. doi: 10.1055/s-0038-1668555
89. Siu, AL. Screening for iron deficiency anemia and iron supplementation in pregnant women to improve maternal health and birth outcomes: U.S. preventive services task force recommendation statement. Ann Intern Med. (2015) 163:529–36. doi: 10.7326/M15-1707
90. Brannon, PM, Stover, PJ, and Taylor, CL. Integrating themes, evidence gaps, and research needs identified by workshop on iron screening and supplementation in iron-replete pregnant women and young children. Am J Clin Nutr. (2017) 106:1703S–12S. doi: 10.3945/ajcn.117.156083
91. Dewey, KG, and Oaks, BM. U-shaped curve for risk associated with maternal hemoglobin, iron status, or iron supplementation. Am J Clin Nutr. (2017) 106:1694S–702S. doi: 10.3945/ajcn.117.156075
92. Zhang, C, and Rawal, S. Dietary iron intake, iron status, and gestational diabetes. Am J Clin Nutr. (2017) 106:1672S–80S. doi: 10.3945/ajcn.117.156034
93. Uauy, R, Hertrampf, E, and Reddy, M. Iron fortification of foods: overcoming technical and practical barriers. J Nutr. (2002) 132:849S–52S. doi: 10.1093/jn/132.4.849S
94. Hurrell, RF. Preventing iron deficiency through food fortification. Nutr Rev. (1997) 55:210–22. doi: 10.1111/j.1753-4887.1997.tb01608.x
95. Shivalli, S, Srivastava, RK, and Singh, GP. Trials of improved practices (TIPs) to enhance the dietary and iron-folate intake during pregnancy-a quasi experimental study among rural pregnant women of Varanasi, India. PLoS One. (2015) 10:e0137735. doi: 10.1371/journal.pone.0137735
96. Zhang, Y, Xu, S, Zhong, C, Li, Q, Wu, M, Zhang, G, et al. Periconceptional iron supplementation and risk of gestational diabetes mellitus: a prospective cohort study. Diabetes Res Clin Pract. (2021) 176:108853. doi: 10.1016/j.diabres.2021.108853
97. Petry, CJ. Iron supplementation in pregnancy and risk of gestational diabetes: a narrative review. Nutrients. (2022) 14:4791. doi: 10.3390/nu14224791
98. Gomes, S, Lopes, C, and Pinto, E. Folate and folic acid in the periconceptional period: recommendations from official health organizations in thirty-six countries worldwide and WHO. Public Health Nutr. (2016) 19:176–89. doi: 10.1017/S1368980015000555
100. Suitor, CW, and Bailey, LB. Dietary Folate equivalents. J Am Diet Assoc. (2000) 100:88–94. doi: 10.1016/S0002-8223(00)00027-4
101. Institute of Medicine Standing Committee on the scientific evaluation of dietary reference I, its panel on Folate OBV, choline. Dietary reference intakes for thiamin, riboflavin, niacin, vitamin B6, Folate, vitamin B12, pantothenic acid, biotin, and choline. Washington, DC: National Academies Press. (1998).
102. Balion, C, and Kapur, B. Clinical utility of serum and red blood cell analysis. Clin Lab News. (2011) 37:8–10.
103. Ross, AC, Caballero, B, Cousins, RJ, and Tucker, KL. Modern nutrition in health and disease. USA: Jones & Bartlett Learning. (2020).
104. Brouwer, IA, van Dusseldorp, M, West, CE, and Steegers-Theunissen, RP. Bioavailability and bioefficacy of folate and folic acid in man. Nutr Res Rev. (2001) 14:267–94. doi: 10.1079/NRR200126
105. Institute of Medicine (IOM). Dietary reference intakes for thiamin, riboflavin, niacin, vitamin B6, folate, vitamin B12, pantothenic acid, biotin, and choline. Washington, DC, USA: National Academies Press (US). (2000).
106. Cetin, I, Berti, C, and Calabrese, S. Role of micronutrients in the periconceptional period. Hum Reprod Update. (2010) 16:80–95. doi: 10.1093/humupd/dmp025
107. McMillen, IC, Maclaughlin, SM, Muhlhausler, BS, Gentili, S, Duffield, JL, and Morrison, JL. Developmental origins of adult health and disease: the role of periconceptional and foetal nutrition. Wiley Online Library. Malden, USA: Blackwell Publishing Inc. (2008);102:82–9.
108. Rain, JD, Blot, I, and Tchernia, G. Folic acid deficiency in developing nations. Folates and Cobalamins. (1989):171–7. doi: 10.1007/978-3-642-74364-1_13
109. De-Regil, LM, Peña-Rosas, JP, Fernández-Gaxiola, AC, and Rayco-Solon, P. Effects and safety of periconceptional oral folate supplementation for preventing birth defects. Cochrane Database Syst Rev. (2015) 2015. doi: 10.1002/14651858.CD007950.pub3
110. Forges, T, Monnier-Barbarino, P, Alberto, JM, Guéant-Rodriguez, RM, Daval, JL, and Guéant, JL. Impact of folate and homocysteine metabolism on human reproductive health. Hum Reprod Update. (2007) 13:225–38. doi: 10.1093/humupd/dml063
111. Ray, JG, and Laskin, CA. Folic acid and Homocyst(e)ine metabolic defects and the risk of placental abruption, pre-eclampsia and spontaneous pregnancy loss: a systematic review. Placenta. (1999) 20:519–29. doi: 10.1053/plac.1999.0417
112. Steegers-Theunissen, RP, Van Iersel, CA, Peer, PG, Nelen, WL, and Steegers, EA. Hyperhomocysteinemia, pregnancy complications, and the timing of investigation. Obstet Gynecol. (2004) 104:336–43. doi: 10.1097/01.AOG.0000129955.47943.2a
113. Bajaj, AD. A self-study on identification of etiology of Anemia in pregnancy. Indian J Obstetrics Gynecol Res. (2022) 9:537–40. doi: 10.18231/j.ijogr.2022.102
114. VMNIS. Micronutrient Indicators Serum and red blood cell folate concentrations for assessing folate status in populations. Geneva, Switzerland: World Health Organization (WHO) (2015).
115. Moges, N, Sisay Chanie, E, Anteneh, RM, Zemene, MA, Gebeyehu, AA, Belete, MA, et al. The effect of folic acid intake on congenital anomalies. A systematic review and meta-analysis. Frontiers. Pediatrics. (2024) 12:1386846. doi: 10.3389/fped.2024.1386846
116. Caudill, MA. Folate bioavailability: implications for establishing dietary recommendations and optimizing status. Am J Clin Nutr. (2010) 91:1455S–60S. doi: 10.3945/ajcn.2010.28674E
117. de Benoist, B. Conclusions of a WHO technical consultation on folate and vitamin B12 deficiencies. Food Nutr Bull. (2008) 29:S238–44. doi: 10.1177/15648265080292S129
118. Singh, S, Sedgh, G, and Hussain, R. Unintended pregnancy: worldwide levels, trends, and outcomes. Stud Fam Plan. (2010) 41:241–50. doi: 10.1111/j.1728-4465.2010.00250.x
119. Wald, NJ. Folic acid and neural tube defects: discovery, debate and the need for policy change. J Med Screen. (2022) 29:138–46. doi: 10.1177/09691413221102321
120. Hertrampf, E, and Cortés, F. National food-fortification program with folic acid in Chile. Food Nutr Bull. (2008) 29:S231–7. doi: 10.1177/15648265080292S128
121. Pardo, R, Vilca, M, Villarroel, L, Davalji, T, Obrycki, JF, Mazumdar, M, et al. Neural tube defects prevalence does not increase after modification of the folic acid fortification program in Chile. Birth Defects Res. (2022) 114:259–66. doi: 10.1002/bdr2.1994
122. Williams, LJ, Rasmussen, SA, Flores, A, Kirby, RS, and Edmonds, LD. Decline in the prevalence of spina bifida and anencephaly by race/ethnicity: 1995–2002. Pediatrics. (2005) 116:580–6. doi: 10.1542/peds.2005-0592
123. De Wals, P, Tairou, F, Van Allen, MI, Uh, S-H, Lowry, RB, Sibbald, B, et al. Reduction in neural-tube defects after folic acid fortification in Canada. N Engl J Med. (2007) 357:135–42. doi: 10.1056/NEJMoa067103
124. Dorise, B, Byth, K, McGee, T, Wood, A, and Blumenthal, C. A low intensity dietary intervention for reducing excessive gestational weight gain in an overweight and obese pregnant cohort. Eating and Weight Disord-Stud Anorexia, Bulimia and Obesity. (2020) 25:257–63. doi: 10.1007/s40519-018-0566-2
125. Beringer, M, Schumacher, T, Keogh, L, Sutherland, K, Knox, P, Herden, J, et al. Nutritional adequacy and the role of supplements in the diets of indigenous Australian women during pregnancy. Midwifery. (2021) 93:102886. doi: 10.1016/j.midw.2020.102886
126. Organization WH. WHO antenatal care recommendations for a positive pregnancy experience. Nutritional interventions update: Multiple micronutrient supplements during pregnancy. Geneva, Switzerland: World Health Organization (2020).
127. Smith, ER, Shankar, AH, Wu, LS, Aboud, S, Adu-Afarwuah, S, Ali, H, et al. Modifiers of the effect of maternal multiple micronutrient supplementation on stillbirth, birth outcomes, and infant mortality: a meta-analysis of individual patient data from 17 randomised trials in low-income and middle-income countries. Lancet Glob Health. (2017) 5:e1090–100. doi: 10.1016/S2214-109X(17)30371-6
128. Patel, KR. The adverse effects of an excessive folic acid intake. Eur J Clin Nutr. (2016) 71:159–63. doi: 10.1038/ejcn.2016.194
129. Charles, D, Ness, AR, Campbell, D, Smith, GD, and Hall, MH. Taking folate in pregnancy and risk of maternal breast cancer. BMJ. (2004) 329:1375–6. doi: 10.1136/bmj.329.7479.1375
130. Heart Outcomes Prevention Evaluation (HOPE) 2. Homocysteine lowering with folic acid and B vitamins in vascular disease. N Engl J Med. (2006) 354:1567–77. doi: 10.1056/NEJMoa060900
131. Figueiredo, JC, Grau, MV, Haile, RW, Sandler, RS, Summers, RW, Bresalier, RS, et al. Folic acid and risk of prostate Cancer: results from a randomized clinical trial. JNCI J Natl Cancer Inst. (2009) 101:432–5. doi: 10.1093/jnci/djp019
132. Stabler, SP, and Allen, RH. Vitamin B12 deficiency as a worldwide problem. Annu Rev Nutr. (2004) 24:299–326. doi: 10.1146/annurev.nutr.24.012003.132440
133. Darwish, AM, Mohamad, SN, Gamal Al-Din, HR, Elsayed, YA, and Ahmad, SI. Prevalence and predictors of deficient dietary calcium intake during the third trimester of pregnancy: the experience of a developing country. J Obstet Gynaecol Res. (2009) 35:106–12. doi: 10.1111/j.1447-0756.2008.00879.x
134. Bailey, LB, and Berry, RJ. Folic acid supplementation and the occurrence of congenital heart defects, orofacial clefts, multiple births, and miscarriage. Am J Clin Nutr. (2005) 81:1213S–7S. doi: 10.1093/ajcn/81.5.1213
135. Saccone, G, and Berghella, V. Folic acid supplementation in pregnancy to prevent preterm birth: a systematic review and meta-analysis of randomized controlled trials. Eur J Obstet Gynecol Reprod Biol. (2016) 199:76–81. doi: 10.1016/j.ejogrb.2016.01.042
136. Buppasiri, P, Lumbiganon, P, Thinkhamrop, J, Ngamjarus, C, Laopaiboon, M, and Medley, N. Calcium supplementation (other than for preventing or treating hypertension) for improving pregnancy and infant outcomes. Cochrane database of systematic reviews: John Wiley and Sons Ltd. (2015) 2015:CD007079. doi: 10.1002/14651858.CD007079.pub3
137. Kumar, A, and Kaur, S. Calcium: A Nutrient in Pregnancy. J Obstetrics Gynecol India: Federation of Obstetric and Gynecol Societies of India. (2017) 67:313–8. doi: 10.1007/s13224-017-1007-2
138. Thomas, M, and Weisman, SM. Calcium supplementation during pregnancy and lactation: effects on the mother and the fetus. Am J Obstet Gynecol. (2006) 194:937–45. doi: 10.1016/j.ajog.2005.05.032
139. Kalkwarf, HJ, and Specker, BL. Bone mineral changes during pregnancy and lactation. Endocrine. (2002) 17:49–54. doi: 10.1385/ENDO:17:1:49
140. Ritchie, LD, Fung, EB, Halloran, BP, Turnlund, JR, Van Loan, MD, Cann, CE, et al. A longitudinal study of calcium homeostasis during human pregnancy and lactation and after resumption of menses. Am J Clin Nutr. (1998) 67:693–701. doi: 10.1093/ajcn/67.4.693
141. Buchowski, MS. Calcium in the context of dietary sources and metabolism. Calcium: Chemistry, analysis, function and effects. Cambridge, UK: Royal Society of Chemistry (RSC) (2015). p. 3–20.
142. Williamson, CS. Nutrition in pregnancy. Nutr Bull. (2006) 31:28–59. doi: 10.1111/j.1467-3010.2006.00541.x
143. Bronner, F, Pansu, D, and Stein, WD. An analysis of intestinal calcium transport across the rat intestine. American journal of physiology-gastrointestinal and liver. Physiology. (1986) 250:G561–9. doi: 10.1152/ajpgi.1986.250.5.G561
144. Johnson, LR. Physiology of the gastrointestinal tract. Amsterdam, Netherlands, and Boston, USA: Elsevier (2006).
145. Buzinaro, EF, Almeida, RNA, and Mazeto, GMFS. Biodisponibilidade do cálcio dietético. Arquivos Brasileiros de Endocrinologia & Metabologia. (2006) 50:852–61. doi: 10.1590/S0004-27302006000500005
146. Ayoubi, JM, Hirt, R, Badiou, W, Hininger-Favier, I, Favier, M, Zraik-Ayoubi, F, et al. Nutrition et femme enceinte. EMC - Obstétrique. (2012) 7:1–13. doi: 10.1016/S0246-0335(12)70039-X
147. World Health O. WHO recommendations on antenatal care for a positive pregnancy experience. Geneva, Switzerland: World Health Organization (WHO) (2016).
148. World Health O. WHO recommendations for prevention and treatment of pre-eclampsia and eclampsia. Geneva, Switzerland: World Health Organization (WHO) (2011).
149. Thapa, K, Sanghvi, H, Rawlins, B, Karki, YB, Regmi, K, Aryal, S, et al. Coverage, compliance, acceptability and feasibility of a program to prevent pre-eclampsia and eclampsia through calcium supplementation for pregnant women: an operations research study in one district of Nepal. BMC Pregnancy Childbirth. (2016) 16:1–10. doi: 10.1186/s12884-016-1033-6
150. Fleet, JC. Vitamin D-mediated regulation of intestinal calcium absorption. Nutrients. (2022) 14:3351. doi: 10.3390/nu14163351
151. Hollis, BW. Circulating 25-hydroxyvitamin D levels indicative of vitamin D sufficiency: implications for establishing a new effective dietary intake recommendation for vitamin D. J Nutr. (2005) 135:317–22. doi: 10.1093/jn/135.2.317
152. Berkner, KL, and Runge, KW. Vitamin K-dependent protein activation: normal gamma-glutamyl carboxylation and disruption in disease. Int J Mol Sci. (2022) 23:5759. doi: 10.3390/ijms23105759
153. Liu, J, Han, P, Wu, J, Gong, J, and Tian, D. Prevalence and predictive value of hypocalcemia in severe COVID-19 patients. J Infect Public Health. (2020) 13:1224–8. doi: 10.1016/j.jiph.2020.05.029
154. Voulgaridou, G, Papadopoulou, SK, Detopoulou, P, Tsoumana, D, Giaginis, C, Kondyli, FS, et al. Vitamin D and calcium in osteoporosis, and the role of bone turnover markers: a narrative review of recent data from RCTs. Diseases. (2023) 11:29. doi: 10.3390/diseases11010029
155. Tsuprykov, O, Elitok, S, Buse, C, Chu, C, Krämer, BK, and Hocher, B. Opposite correlation of 25-hydroxy-vitamin D-and 1, 25-dihydroxy-vitamin D-metabolites with gestational age, bone-and lipid-biomarkers in pregnant women. Sci Rep. (2021) 11:1923. doi: 10.1038/s41598-021-81452-9
156. Tinawi, M. Disorders of calcium metabolism: hypocalcemia and hypercalcemia. Cureus. (2021) 13. doi: 10.7759/cureus.12420
157. Kumssa, DB, Joy, EJM, Ander, EL, Watts, MJ, Young, SD, Walker, S, et al. Dietary calcium and zinc deficiency risks are decreasing but remain prevalent. Sci Rep. (2015) 5:5. doi: 10.1038/srep10974
158. Shlisky, J, Mandlik, R, Askari, S, Abrams, S, Belizan, JM, Bourassa, MW, et al. Calcium deficiency worldwide: prevalence of inadequate intakes and associated health outcomes. Ann N Y Acad Sci. (2022) 1512:10–28. doi: 10.1111/nyas.14758
159. Takaya, J. Calcium-deficiency during pregnancy affects insulin resistance in offspring. Int J Molecular Sci: MDPI. (2021) 22. doi: 10.3390/ijms22137008
160. Kovacs, CS, and Kronenberg, HM. Maternal-fetal calcium and bone metabolism during pregnancy, puerperium, and lactation*. Endocr Rev. (1997) 18:832–72.
161. Belizán, J, Villar, J, and Repke, J. The relationship between calcium intake and pregnancy-induced hypertension: up-to-date evidence. Am J Obstet Gynecol. (1988) 158:898–902. doi: 10.1016/0002-9378(88)90091-9
162. Malas Naser, O, and Shurideh, ZM. Does serum calcium in pre-eclampsia and normal pregnancy differ? Saudi Med J. (2001) 22:868–71.
163. Sukonpan, K, and Phupong, V. Serum calcium and serum magnesium in normal and preeclamptic pregnancy. Arch Gynecol Obstet. (2005) 273:12–6. doi: 10.1007/s00404-004-0672-4
164. Bourassa, MW, Abrams, SA, Belizán, JM, Boy, E, Cormick, G, Quijano, CD, et al. Interventions to improve calcium intake through foods in populations with low intake. Ann N Y Acad Sci. (2022) 1511:40–58. doi: 10.1111/nyas.14743
165. Abrudan, IM, Kelemen, B, Girbovan, AM, Abrudan, CV, Crisan, C, Sur, G, et al. The diagnosis of patients with celiac disease and calcium deficie. Int J Celiac Dis. (2016) 4:16–8. doi: 10.12691/ijcd-4-1-3
166. Graham, M, Clark, C, Scherer, A, Ratner, M, and Keen, C. An analysis of the nutritional adequacy of mass-marketed vegan recipes. Cureus. (2023). 15. doi: 10.7759/cureus.37131
167. Sipponen, P, and Härkönen, M. Hypochlorhydric stomach: a risk condition for calcium malabsorption and osteoporosis? Scand J Gastroenterol. (2010) 45:133–8. doi: 10.3109/00365520903434117
168. Cormick, G, Betrán, A, Romero, I, Lombardo, C, Gülmezoglu, A, Ciapponi, A, et al. Global inequities in dietary calcium intake during pregnancy: a systematic review and meta-analysis. BJOG Int J Obstet Gynaecol. (2019) 126:444–56. doi: 10.1111/1471-0528.15512
169. Merialdi, M, Mathai, M, Ngoc, N, Purwar, M, Campodonico, L, Abdel-Aleem, H, et al. World Health Organization systematic review of the literature and multinational nutritional survey of calcium intake during pregnancy. Fetal and Maternal Med Rev. (2005) 16:97–121. doi: 10.1017/S0965539505001506
170. Lee, SE, Talegawkar, SA, Merialdi, M, and Caulfield, LE. Dietary intakes of women during pregnancy in low-and middle-income countries. Public Health Nutr. (2013) 16:1340–53. doi: 10.1017/S1368980012004417
171. Meyer, F, Amaechi, BT, Fabritius, H-O, and Enax, J. Overview of calcium phosphates used in biomimetic oral care. Open Dent J. (2018) 12:406–23. doi: 10.2174/1874210601812010406
172. Bauer, DC. Calcium supplements and fracture prevention. N Engl J Med. (2013) 369:1537–43. doi: 10.1056/NEJMcp1210380
173. Institute of M. Dietary reference intakes for calcium and vitamin D. Washington, D.C.: National Academies Press (2011).
174. Rey, E, Jacob, CE, Koolian, M, and Morin, F. Hypercalcemia in pregnancy – a multifaceted challenge: case reports and literature review. Clin Case Reports. (2016) 4:1001–8. doi: 10.1002/ccr3.646
175. Kramer, GH. Retention of iodine in the body: biological half-life of iodine in the human body In: VR Preedy, GN Burrow, RR Watson, editors. Comprehensive handbook of iodine: Nutritional, biochemical, pathological and therapeutic aspects. Amsterdam, Netherlands and Boston, USA: Elsevier (2009). 184–91.
176. Dev, N, Sankar, J, and Vinay, MV. Functions of thyroid hormones. Thyroid disorders: Basic science and clinical practice. Cham, Switzerland: Springer International Publishing; (2016). p. 11–25.
177. Harding, KB, Peña-Rosas, JP, Webster, AC, Yap, CMY, Payne, BA, Ota, E, et al. Iodine supplementation for women during the preconception, pregnancy and postpartum period. Cochrane Database Syst Rev. (2017) 2017:CD011761. doi: 10.1002/14651858.CD011761.pub2
178. Glinoer, D. The regulation of thyroid function in pregnancy: pathways of endocrine adaptation from physiology to pathology. Endocr Rev. (1997) 18:404–33. doi: 10.1210/edrv.18.3.0300
179. Fuge, R, and Johnson, CC. Iodine and human health, the role of environmental geochemistry and diet, a review. Appl Geochem. (2015) 63:282–302. doi: 10.1016/j.apgeochem.2015.09.013
180. Lee, SM, Lewis, J, Buss, DH, Holcombe, GD, and Lawrance, PR. Iodine in British foods and diets. Br J Nutr. (1994) 72:435–46. doi: 10.1079/BJN19940045
181. Obican, SG, Jahnke, GD, Soldin, OP, and Scialli, AR. Teratology public affairs committee position paper: iodine deficiency in pregnancy. Birth Defects Res Part A - Clin Molecular Teratol. (2012) 94:677–82. doi: 10.1002/bdra.23051
182. Pearce, EN. National Trends in iodine nutrition: is everyone getting enough? Thyroid. (2007) 17:823–7. doi: 10.1089/thy.2007.0102
183. Shapira, N. Modified egg as a nutritional supplement during peak BRAIN development: A new target for fortification. Nutr Health. (2009) 20:107–18. doi: 10.1177/026010600902000203
184. Samanta, M, and Choudhary, P. Safety of fish and seafood In: RL Singh and S Mondal, editors. Food safety and human health. London, United Kingdom: Academic Press (2019). 169–87.
185. Reames, E. Nutritional benefits of seafood. Mississippi, USA: Southern Regional Aquaculture Center (SRAC). (2012).
186. Glinoer, D. The importance of iodine nutrition during pregnancy. Public Health Nutr. (2007). 10:1542–1546. doi: 10.1017/S1368980007360886
187. IOM (Institute of Medicine). Dietary reference intakes for vitamin a, vitamin K, arsenic, boron, chromium, copper, iodine, iron, manganese, molybdenum, nickel, silicon, vanadium, and zinc. US: National Academies Press (2002).
188. Products EPoD, Efsa Panel on Dietetic Products N, Allergies. Scientific opinion on dietary reference values for iodine. EFSA J. (2014) 12. doi: 10.2903/j.efsa.2014.3660
189. Domínguez-González, MR, Chiocchetti, GM, Herbello-Hermelo, P, Vélez, D, Devesa, V, and Bermejo-Barrera, P. Evaluation of iodine bioavailability in seaweed using in vitro methods. J Agric Food Chem. (2017) 65:8435–42. doi: 10.1021/acs.jafc.7b02151
190. Felker, P, Bunch, R, and Leung, AM. Concentrations of thiocyanate and goitrin in human plasma, their precursor concentrations in brassica vegetables, and associated potential risk for hypothyroidism. Nutr Rev. (2016) 74:248–58. doi: 10.1093/nutrit/nuv110
191. Jouanne, M, Oddoux, S, Noël, A, and Voisin-Chiret, AS. Nutrient requirements during pregnancy and lactation. Nutrients. (2021) 13:1–17. doi: 10.3390/nu13020692
192. World Health O. Assessment of iodine deficiency disorders and monitoring their elimination: A guide for programme managers. Third ed. Geneva, Switzerland: World Health Organization (WHO) (2007).
193. Zimmermann, MB. The importance of adequate iodine during pregnancy and infancy. World Rev Nutr Diet. (2016) 115:118–24. doi: 10.1159/000442078
194. Manousou, S, Eggertsen, R, Hulthén, L, and Filipsson, NH. A randomized, double-blind study of iodine supplementation during pregnancy in Sweden: pilot evaluation of maternal iodine status and thyroid function. Eur J Nutr. (2021) 60:3411–22. doi: 10.1007/s00394-021-02515-1
195. Andersson, M, De Benoist, B, Delange, F, and Zupan, J. Prevention and control of iodine deficiency in pregnant and lactating women and in children less than 2-years-old: Conclusions and recommendations of the technical consultation. Public health nutrition. Cambridge: Cambridge University Press (2007).
196. Bath, SC, editor. “The effect of iodine deficiency during pregnancy on child development.” Proceedings of the nutrition society; (2019). Cambridge University Press.
197. Næss, S, Markhus, MW, Strand, TA, Kjellevold, M, Dahl, L, Stokland, A-EM, et al. Iodine nutrition and iodine supplement initiation in association with thyroid function in mildly-to-moderately iodine-deficient pregnant and postpartum women. J Nutr. (2021) 151:3187–96. doi: 10.1093/jn/nxab224
198. Marco, A, Vicente, A, Castro, E, Eva Perez, C, Rodríguez, O, Merchan, MA, et al. Patterns of iodine intake and urinary iodine concentrations during pregnancy and blood thyroid-stimulating hormone concentrations in the newborn progeny. Thyroid. (2010) 20:1295–9. doi: 10.1089/thy.2010.0046
199. Smyth, PPA, Burns, R, Huang, RJ, Hoffman, T, Mullan, K, Graham, U, et al. Does iodine gas released from seaweed contribute to dietary iodine intake? Environ Geochem Health. (2011) 33:389–97. doi: 10.1007/s10653-011-9384-4
200. Stagnaro-Green, A, Abalovich, M, Alexander, E, Azizi, F, Mestman, J, Negro, R, et al. Guidelines of the American Thyroid Association for the diagnosis and management of thyroid disease during pregnancy and postpartum. Thyroid. (2011) 21:1081–125. doi: 10.1089/thy.2011.0087
201. Trumbo, P, Yates, AA, Schlicker, S, and Poos, M. Dietary reference intakes. J Am Diet Assoc. (2001) 101:294–301. doi: 10.1016/S0002-8223(01)00078-5
202. Charlton, K, Probst, Y, and Kiene, G. Dietary iodine intake of the Australian population after introduction of a mandatory iodine fortification programme. Nutrients. (2016) 8:701. doi: 10.3390/nu8110701
203. Li, X, Tu, P, Gu, S, Mo, Z, Wu, L, Xing, M, et al. Serum iodine as a potential individual iodine status biomarker: a cohort study of mild iodine deficient pregnant women in China. Nutrients. (2023) 15:3555. doi: 10.3390/nu15163555
204. Hlucny, K, Alexander, BM, Gerow, K, and Larson-Meyer, DE. Reflection of dietary iodine in the 24 h urinary iodine concentration, serum iodine and thyroglobulin as biomarkers of iodine status: a pilot study. Nutrients. (2021) 13:2520. doi: 10.3390/nu13082520
205. Jiang, Y, Gu, S, Mo, Z, Li, X, Liu, C, Li, Y, et al. Thyroglobulin as a sensitive biomarker of iodine status in mildly and moderately iodine-deficient pregnant women. Biol Trace Elem Res. (2024) 202:3923–31. doi: 10.1007/s12011-023-03986-5
206. Organization WH. Assessment of iodine deficiency disorders and monitoring their elimination: A guide for programme managers. Geneva, Switzerland: World Health Organization (2007).
207. Bertinato, J. Iodine nutrition: Disorders, monitoring and policies. Adv Food Nutr Res. (2021) 96:365–415. doi: 10.1016/bs.afnr.2021.01.004
208. Lee, RH, Spencer, CA, Mestman, JH, Miller, EA, Petrovic, I, Braverman, LE, et al. Free T4 immunoassays are flawed during pregnancy. Am J Obstet Gynecol. (2009) 200:260.e1–6. doi: 10.1016/j.ajog.2008.10.042
209. Pramanik, S, Mukhopadhyay, P, and Ghosh, S. Total T4 rise in pregnancy: a relook? Thyroid. Demogr Res. (2020) 13:1–3. doi: 10.1186/s13044-020-00088-5
210. Biban, BG, and Lichiardopol, C. Iodine deficiency, still a global problem? Curr Health Sci J. (2017) 43:103–11. doi: 10.12865/CHSJ.43.02.01
211. Patriota, ES, Lima, IC, Nilson, EA, Franceschini, SC, Goncalves, VS, and Pizato, N. Prevalence of insufficient iodine intake in pregnancy worldwide: a systematic review and meta-analysis. Eur J Clin Nutr. (2022) 76:703–15. doi: 10.1038/s41430-021-01006-0
212. Simpong, DL. Addressing the challenge of iodine deficiency in developing countries. MOJ. Public Health. (2017) 5:89–91. doi: 10.15406/mojph.2017.05.00129
213. Zimmermann, MB, editor. “Symposium on 'Geographical and geological influences on nutrition' - iodine deficiency in industrialised countries.” Proceedings of the Nutrition Society; (2010).
214. Krela-Kaźmierczak, I, Czarnywojtek, A, Skoracka, K, Rychter, AM, Ratajczak, AE, Szymczak-Tomczak, A, et al. Is there an ideal diet to protect against iodine deficiency? Nutrients: MDPI AG. (2021) 13:1–15. doi: 10.3390/nu13020513
215. Hetzel, B. Iodine deficiency disorders (IDD) and their eradication. Lancet. (1983) 322:1126–9. doi: 10.1016/S0140-6736(83)90636-0
216. Negro, R, Soldin, OP, Obregon, MJ, and Stagnaro-Green, A. Hypothyroxinemia and pregnancy. Endocr Pract. (2011):422–9. doi: 10.4158/EP10309.RA
217. Zimmermann, MB. The effects of iodine deficiency in pregnancy and infancy. Paediatr Perinat Epidemiol. (2012) 26:108–17. doi: 10.1111/j.1365-3016.2012.01275.x
218. Zimmermann, MB, Jooste, PL, and Pandav, CS. Iodine-deficiency disorders. Lancet. (2008) 372:1251–62. doi: 10.1016/S0140-6736(08)61005-3
219. de Escobar, GM, Obregón, MJ, and Escobar del Rey, F. Maternal thyroid hormones early in prenancy and fetal brain development. Best Pract Res Clin Endocrinol Metab. (2004) 18:225–48. doi: 10.1016/j.beem.2004.03.012
220. Morreale De Escobar, G, Maria, M, Js, Jes’u, Js, Obrego’n, Obrego’n, O, Escobar, F, et al. Clinical perspective is neuropsychological development related to maternal hypothyroidism or to maternal hypothyroxinemia?*. USA: The Journal of Clinical Endocrinology & Metabolism. (2000).
221. Sánchez-Vega, J, del Rey, FE, Fariñas-Seijas, H, and de Escobar, GM. Inadequate iodine nutrition of pregnant women from Extremadura (Spain). Eur J Endocrinol. (2008) 159:439–45. doi: 10.1530/EJE-08-0309
222. Van Vliet, G, and Polak, M. Thyroid gland development and function. Basel: Elsevier GmbH. (2007).
223. Bath, SC, Steer, CD, Golding, J, Emmett, P, and Rayman, MP. Effect of inadequate iodine status in UK pregnant women on cognitive outcomes in their children: results from the Avon longitudinal study of parents and children (ALSPAC). Lancet. (2013) 382:331–7. doi: 10.1016/S0140-6736(13)60436-5
224. Nazarpour, S, Ramezani Tehrani, F, Behboudi-Gandevani, S, Bidhendi Yarandi, R, and Azizi, F. Maternal urinary iodine concentration and pregnancy outcomes in euthyroid pregnant women: a systematic review and meta-analysis. Biol Trace Elem Res. (2020) 197:411–20. doi: 10.1007/s12011-019-02020-x
225. Nazeri, P, Shab-Bidar, S, Pearce, EN, and Shariat, M. Do maternal urinary iodine concentration or thyroid hormones within the normal range during pregnancy affect growth parameters at birth? A systematic review and meta-analysis. Nutr Rev. (2020) 78:747–63. doi: 10.1093/nutrit/nuz105
226. Mulder, TA, Korevaar, TIM, Peeters, RP, van Herwaarden, AE, de Rijke, YB, White, T, et al. Urinary iodine concentrations in pregnant women and offspring brain morphology. Thyroid. (2021) 31:964–72. doi: 10.1089/thy.2020.0582
227. Fields, C, and Borak, J. Iodine deficiency in vegetarian and vegan diets: evidence-based review of the World's literature on iodine content in vegetarian diets. Comprehensive handbook of iodine: Nutritional, biochemical, pathological and therapeutic Aspects. (2009):521–31. doi: 10.1016/B978-0-12-374135-6.00054-6
228. Cho, NH, Choi, HS, Kim, KW, Kim, HL, Lee, SY, Choi, SH, et al. Interaction between cigarette smoking and iodine intake and their impact on thyroid function: original article. Clin Endocrinol. (2010) 73:264–70. doi: 10.1111/j.1365-2265.2010.03790.x
229. Benjamin, C, Lindsay, A, and Andrew, P. Encyclopedia of human nutrition. Second Amsterdam, Netherlands: Elsevier/Academic Press. ed (2005).
230. Martino, E, Bartalena, L, Bogazzi, F, Braverman, LE, and Martino, E. Effects of amiodarone administration during pregnancy on neonatal thyroid function and subsequent neurodevelopment. J Endocrinol Investig. (2001) 24:116–30. doi: 10.1007/BF03343825
231. Theodoropoulos, T, Braverman, LE, and Vagenakis, AG. Iodide-induced hypothyroidism: a potential Hazard during perinatal life. Science. (1979) 205:502–3. doi: 10.1126/science.451615
232. Xiao, Y, Sun, H, Li, C, Li, Y, Peng, S, Fan, C, et al. Effect of iodine nutrition on pregnancy outcomes in an iodine-sufficient area in China. Biol Trace Elem Res. (2018) 182:231–7. doi: 10.1007/s12011-017-1101-4
233. National institute of health office. Office of Dietary Supplements - Iron: NIH; (2024). Available from: https://ods.od.nih.gov/factsheets/Iron-HealthProfessional/ (Accessed November 27, 2024).
234. U.S. Department of Health and Human Services. Office of dietary supplements - folate: NIH; (2022). Available from: https://ods.od.nih.gov/factsheets/Folate-HealthProfessional/#en2 (Accessed November 27, 2024).
235. National institute of Health Office. Office of Dietary Supplements - calcium: NIH; (2024). Available from: https://ods.od.nih.gov/factsheets/Calcium-HealthProfessional/ (Accessed November 27, 2024).
236. National institute of Health Office. Office of Dietary Supplements - iodine: NIH; (2024). Available from: https://ods.od.nih.gov/factsheets/Iodine-HealthProfessional%20/#:~:text=To%20accommodate%20increased%20iodine%20needs,pregnancy%20and%20lactation%20%5B3%5D (Accessed November 27, 2024).
237. Government of Canada. Dietary reference intakes tables: Reference values for elements: Government of Canada; (2023). Available from: https://www.canada.ca/en/health-canada/services/food-nutrition/healthy-eating/dietary-reference-intakes/tables/reference-values-elements.html. (Accessed November 27, 2024).
238. National Health and Medical Research Council. Iron: NHMRC; (2006). Available from: https://www.eatforhealth.gov.au/nutrient-reference-values/nutrients/iron. (Accessed December 10, 2024).
239. National Health and Medical Research Council. Folate: NHMRC; (2006). Available from: https://www.eatforhealth.gov.au/nutrient-reference-values/nutrients/folate. (Accessed December 10, 2024).
240. National Health and Medical Research Council. Calcium: NHMRC; (2006). Available from: https://www.eatforhealth.gov.au/nutrient-reference-values/nutrients/calcium (Accessed December 10, 2024).
241. National Health and Medical Research Council. Iodine: NHMRC; (2006). Available from: https://www.eatforhealth.gov.au/nutrient-reference-values/nutrients/iodine (Accessed December 10, 2024).
243. e.V. DGfE. Referenzwerte für die Nährstoffzufuhr: Calcium. Bonn: Deutsche Gesellschaft für Ernährung e.V (2013).
244. für die Nährstoffzufuhr D-CR. Deutsche Gesellschaft für Ernährung, Österreichische Gesellschaft für Ernährung, Schweizerische Gesellschaft für Ernährungsforschung. Frankfurt am Main: Schweizerische Vereinigung für Ernährung (2000).
245. Blomhoff, R, Andersen, R, Arnesen, EK, Christensen, JJ, Eneroth, H, Erkkola, M, et al. Nordic nutrition recommendations 2023: Integrating environmental. Copenhagen: Aspects: Nordic Council of Ministers (2023).
246. Indian Council of Medical Research. ICMR-NIN expert group on nutrient requirement for Indians, recommended dietary allowances (RDA) and estimated average requirements (EAR) - 2020. Hyderabad, India: ICMR (2020).
247. Marangoni, F, Cetin, I, Verduci, E, Canzone, G, Giovannini, M, Scollo, P, et al. Maternal diet and nutrient requirements in pregnancy and breastfeeding. An Italian Consensus Document Nutrients. (2016) 8:629. doi: 10.3390/nu8100629
248. EFSA Panel on Dietetic Products N, Allergies. Scientific opinion on dietary reference values for calcium. EFSA J. (2015) 13:4101. doi: 10.2903/j.efsa.2015.4101
249. Martin, A. Les apports nutritionnels conseillés pour la population française. Reproduction Nutrit Develop (France). (2001) 41:119–28. doi: 10.1051/rnd:2001100
250. Philpott, CC, and Jadhav, S. The ins and outs of iron: escorting iron through the mammalian cytosol. Free Radic Biol Med. (2019) 133:112–7. doi: 10.1016/j.freeradbiomed.2018.10.411
251. Cappellini, MD, Musallam, KM, and Taher, AT. Iron deficiency anaemia revisited. J Intern Med. (2020) 287:153–70. doi: 10.1111/joim.13004
252. Rusch, JA, van der Westhuizen, DJ, Gill, RS, and Louw, VJ. Diagnosing iron deficiency: controversies and novel metrics. Best Pract Res Clin Anaesthesiol. (2023) 37:451–67. doi: 10.1016/j.bpa.2023.11.001
253. Dignass, A, Farrag, K, and Stein, J. Limitations of serum ferritin in diagnosing iron deficiency in inflammatory conditions. Int J Chronic Dis. (2018) 2018:1–11. doi: 10.1155/2018/9394060
254. Coffey, R, and Ganz, T. Iron homeostasis: an anthropocentric perspective. J Biol Chem. (2017) 292:12727–34. doi: 10.1074/jbc.R117.781823
255. Zaman, B, Rasool, S, Jasim, S, and Abdulah, D. Hepcidin as a diagnostic biomarker of iron deficiency anemia during pregnancy. J Matern Fetal Neonatal Med. (2021) 34:1288–96. doi: 10.1080/14767058.2019.1635112
256. Konz, T, Montes-Bayón, M, and Vaulont, S. Hepcidin quantification: methods and utility in diagnosis. Metallomics. (2014) 6:1583–90. doi: 10.1039/C4MT00063C
257. Allen, LH. Anemia and iron deficiency: effects on pregnancy outcome. Am J Clin Nutr. (2000) 71:1280S–4S. doi: 10.1093/ajcn/71.5.1280s
258. Allen, LH. Biological mechanisms that might underlie Iron's effects on fetal growth and preterm birth. J Nutr. (2001) 131:581S–9S. doi: 10.1093/jn/131.2.581S
Keywords: pregnancy, micronutrients, supplementation, fetal growth, Iron, folic acid, calcium, iodine
Citation: Fatima N, Yaqoob S, Rana L, Imtiaz A, Iqbal MJ, Bashir Z, Shaukat A, Naveed H, Sultan W, Afzal M, Kashif Z, Al-Asmari F, Shen Q and Ma Y (2025) Micro-nutrient sufficiency in mothers and babies: management of deficiencies while avoiding overload during pregnancy. Front. Nutr. 12:1476672. doi: 10.3389/fnut.2025.1476672
Received: 06 August 2024; Accepted: 13 January 2025;
Published: 01 April 2025.
Edited by:
Rakesh Bhardwaj, Indian Council of Agricultural Research (ICAR), IndiaReviewed by:
Mian Anjum Murtaza, University of Sargodha, PakistanCopyright © 2025 Fatima, Yaqoob, Rana, Imtiaz, Iqbal, Bashir, Shaukat, Naveed, Sultan, Afzal, Kashif, Al-Asmari, Shen and Ma. This is an open-access article distributed under the terms of the Creative Commons Attribution License (CC BY). The use, distribution or reproduction in other forums is permitted, provided the original author(s) and the copyright owner(s) are credited and that the original publication in this journal is cited, in accordance with accepted academic practice. No use, distribution or reproduction is permitted which does not comply with these terms.
*Correspondence: Yongkun Ma, bWF5b25na3VuQHVqcy5lZHUuY24=
†These authors have contributed equally to this work
Disclaimer: All claims expressed in this article are solely those of the authors and do not necessarily represent those of their affiliated organizations, or those of the publisher, the editors and the reviewers. Any product that may be evaluated in this article or claim that may be made by its manufacturer is not guaranteed or endorsed by the publisher.
Research integrity at Frontiers
Learn more about the work of our research integrity team to safeguard the quality of each article we publish.