- 1Department of Pedodontics, Affiliated Stomatology Hospital of Jinzhou Medical University, Jinzhou, China
- 2Collaborative Innovation Center for Health Promotion of Children and Adolescents of Jinzhou Medical University, Jinzhou, China
- 3Department of Micro-endodontics, Affiliated Stomatology Hospital of Jinzhou Medical University, Jinzhou, China
- 4Department of Prosthetics, Affiliated Stomatology Hospital of Jinzhou Medical University, Jinzhou, China
Introduction: A complicated scenario where “multiple disease threats coexist and multiple health influencing factors are intertwined” is demonstrated by the fact that dental caries, obesity myopia and scoliosis have emerged as global public health issues. The problem of diseases co-existing in living things can be resolved by using probiotics. Lactobacillus plantarum, has gained attention recently due to its probiotic properties, useful traits, and potential medical applications.
Objective: Examining the anti-obesity and anti-caries effects of L. plantarum ATCC 14917 on dental caries and obese rat models caused by a high-fat and high-sugar diet is the aim of this study.
Method: In vitro, we assessed the L. plantarum strain’s probiotic properties, such as its antibacterial activity and ability to build biofilms, to determine its ability to inhibit Streptococcus mutans. Prior to the in vivo experiment, the subsist test for L. plantarum ATCC 14917 was carried out by mimicking its capacity to lower blood sugar and blood lipid levels as well as its tolerance to gastrointestinal disorders. In order to assess the health promotion effect of L. plantarum in vivo. Three-week-old rats were fed a high-sugar, high-fat diet for 8 weeks. They were split into three groups: the control group (Control), the caries and obesity group (CA _OB) and the caries and obesity treated with L. plantarum ATCC14917 group (LP). L. plantarum ATCC 14917 was applied during the experiment, and the associated indices were then thoroughly assessed. These included the use of Mirco-CT to calculate the enamel volume, the staining of liver and fat cell sections, serological analysis, and 16S rRNA sequencing of feces.
Results: It was proved that the L. plantarum could inhibit the proliferation of S. mutans and remove dental plaque biofilm in time, which showed the remarkable effects of anti-caries in vitro. The demineralization rate of enamel decreased by 44.10% due to the inhibition of acid production by pathogenic bacteria. Moreover, In intestinal and stomach juice simulations, L. plantarum has a high survival rate. The characteristics of bacterial activity in a wide range of pH could degrade triglycerides and glucose in vitro smoothly. The LP group demonstrated it by reducing animal weight, serum biochemical indices, and HE-stained adipocytes as compared to the CA_OB group. 16S rRNA sequencing data showed that a high-fat and high-sugar diet induced the imbalance of intestinal flora, which showed an increase in microbial abundance, including unclassified_o__Clostridia_UCG-014, unclassified_f__Oscillospiraceae, Turicibacter, unclassified_f__Lachnospiraceae, Clostridium_sensu_stricto_1. After the intervention of L. plantarum, the number of Lactobacillus, Limosilactobacillus, unclassified_f__Muribaculaceae, Blautia, Faecalibaculum increased significantly.
Conclusion: Therefore, L. plantarum ATCC 14917 performed the potential of reducing tooth decay and controlling weight gain by a single strain. Support the management of dental caries and obesity, and establish a foundation for future functional food research and development.
Highlights
• L. plantarum ATCC 14917 could treat dental caries and obesity at the same time.
• L. plantarum ATCC 14917 degraded glucose and triglycerides well in vitro.
• L. plantarum ATCC 14917 recovered the intestinal flora imbalance.
1 Introduction
Dental caries and obesity are considered to be chronic, highly prevalent and multifactorial diseases, which are harmful to the life and health of children and adolescents (1–3). Streptococcus mutans is the main pathogenic bacteria of dental caries. Because of its excellent ability to make full use of sucrose to synthesize extracellular glucan and intracellular polysaccharide, which can promote adhesion to form dental plaque biofilm and provide sufficient energy for itself (4). Moreover, acidification of the microenvironment in biofilm leads to demineralization of enamel and decay (5). Obesity is caused by a combination of biology and environment variables, which are characterized by excessive adipose tissue, dysfunction and changes of intestinal microbiota (6, 7).
Recent years have seen a lot of emphasis focused on the connection between dental caries and obesity, and various studies have examined this relationship (8, 9). Older children and adolescents who are obese have a higher risk of developing dental caries, and there is a significant positive correlation between dental caries and weight gain (10–12). Reasonable mechanisms have been put out for the increasing prevalence and or severity of caries in overweight or obesity individuals. One explanation is that poor eating habits and excessive carbohydrate intake are the primary causes (13–15). Additionally, because obesity is a chronic inflammatory illness, decreased salivary flow can exacerbate oral discomfort (16). As a result, obese children may be more susceptible to dental caries (17, 18). Obesity and dental cavities are associated with parents’ poor income and educational attainment (19). Children who are overweight or obese have a higher risk of dental cavities (20, 21). As a result, the association between dental caries and obesity is assessed across a range of social and environmental contexts, as well as across cultural, dietary, and obesity-causing behaviors.
Excessive carbohydrate intake will not only promote the occurrence of dental caries, but also accumulate fat (22). Recent studies have unveiled the existence of the oral-intestinal axis, highlighting significant relevance among specific genes, their metabolites, and the development of dental caries and obesity, which may be the potential target of intervention (23–25). The chronic usage of antibiotics has been shown to disrupt the delicate equilibrium of both oral and intestinal ecosystems, potentially leading to an increase in bacterial resistance (26). Consequently, there is a pressing need for the development and implementation of safer and more effective preventive strategies for caries and obesity.
Probiotic therapy emerges as a promising solution, as it can help restore the balance of oral microbiota, thereby preventing and treating a range of oral health issues, including caries and periodontology (27). Among these probiotics, Lactobacillus plantarum stands out as a facultative heterotrophic fermentation organism with unique regulatory properties (28). It has been found to address disturbances in glucose and lipid metabolism that may arise from diets high in fats and sugars (29). Its beneficial effects may involve different mechanisms, including improving blood glucose and blood lipid metabolism, regulating intestinal microbiota (30).
By using the agar diffusion method and crystal violet staining method, L. plantarum of antibacterial activity and antibacterial membrane capacity against S. mutans were detected and its aggregation activity was investigated. We evaluated the strain’s probiotic characteristics, including L. plantarum for tolerance in the gastrointestinal tract and its ability to consume fat and sugar. In obese rats with dental caries caused by a high-sugar and high-fat diet, the gut microbial composition and demineralized enamel volume were observed using 16S rRNA and Micro-CT. This research of purpose was to assess the inhibitory effect of L. plantarum on dental caries and obesity. It provide a fresh perspective on the use of probiotics to manage illnesses, and support as a foundation for the development of functional foods.
2 Materials and methods
2.1 Bacterium and supernatant preparation
Lactobacillus plantarum (L. plantarum ATCC 14917, CICC, China) and S. mutans (S. mutans ATCC 25175, CGMCC, China) were grown in DeMan-Rogosa-Sharpe (MRS) medium (Hopebio., Ltd., Qingdao, China) and Brain-Heart-Infusion (BHI) medium (Hopebio., Ltd., Qingdao, China) respectively. All strains were fostered at 37°C for 16–24 h, and were adjusted to OD600 = 1, respectively. The bacterial culture was centrifuged and filtered to extract the supernatant of L. plantarum.
2.2 Antimicrobial property of Lactobacillus in vitro
2.2.1 Lactobacillus bacteriostatic test
Lactobacillus plantarum culture solution was added to an Oxford cup and the growth inhibition diameter was measured after 24 h of culture with MRS liquid culture medium as control. Absorption method was partially modified to test antibacterial activity of L. plantarum. L. plantarum supernate an MRS medium were mixed into 100 μL of S. mutans culture solution, respectively. When the inhibition ratio is less than two after 18 to 24 h of culture, the sample demonstrated good antibacterial activity. The bacteriostatic value was obtained by Equation 1:
where A represented the bacteriostatic value, C1 the control group’s average colony forming units (CFU), and T1 the experimental group’s average CFU.
2.2.2 Streptococcus mutans in supernatant of Lactobacillus plantarum
Supernatant of Lactobacillus was to handle S. mutans. A final concentration of 12.5 to 100% (v/v) was employed, with MRS liquid medium serving as the control. Every 4 hours, 100 microliters of various concentrations of culture fluid were collected and put in a 96-well plate to monitor the growth conditions. The ELISA Reader was used to measure the OD value at 600 nm. An ELISA reader was used to measure optical density at 600 nm.
2.2.3 Formation and removal of biofilm
To evaluate the biofilm formation and removal, crystal violet staining was employed, utilizing various concentrations of the supernatant ranging from 0 to 100% (v/v), with MRS liquid medium serving as the control. Following a 24 h culture period, it was washed with PBS for 3 times and a 15 min methanol fixation. 0.1% crystal violet was utilized for staining, followed by the dissolution of 33% glacial acetic acid. The optical densities were again measured at 600 nm to assess the effectiveness of the different concentrations on biofilm dynamics.
By adding 50% (v/v) L. plantarum supernatant with the same quantity at various time intervals (0 h, 6 h, 12 h, and 24 h), which was used to observe the production and removal of biofilm. MRS medium was used as the control (12 h and 24 h). Staining and determination steps are the same as above, and the biofilm reduction rate is obtained by Equation 2:
Once a biofilm had formed after 24 h of culture, a dyeing working solution was made by diluting PI and N0 fluorescent dye with NaCl solution (Shanghai Beibo Biotechnology Co., Ltd.). After gently rinsing the side wall three times with NaCl and draining, add 200 μL of the dyeing working solution and let it sit in the dark for fifteen minutes. After washing the bacteria once with NaCl solution, resuspend them by adding the proper amount of NaCl solution. The red signal is received through the red channel, and the green signal is received through the green channel. Look through a fluorescent microscope and snap pictures.
2.2.4 Auto-aggregation and co-aggregation
Specific modifications to the previously established methods were applied. Adjust the concentration of L. plantarum solution to OD600 = 0.6 ± 0.05, and the concentration of S. mutans solution to OD600 = 0.5 ± 0.05, and the bacteria separately and in a mixed manner. The absorbance of the upper liquid were measured at various time intervals. The self-polymerization and co-polymerization ability of strains at different times were judged, respectively. The Auto-aggregation ability was obtained by Equation 3:
A0 refers to the absorbance measured at 0 h, while At represents absorbance values taken at 2, 4, 6, and 24 h.
The Co-aggregation ability was obtained by Equation 4:
Where Ax is the absorbance of 0 h L. plantarum, Ay is the absorbance of 0 h S. mutans, Amix is the absorbance of the mixture at the 2, 4, 6, and 24 h time points.
2.3 Evaluation of digestive environment tolerance
2.3.1 Tolerance to acid and bile salt
Lactobacillus plantarum was inoculated into MRS medium with pH of 3.0 and 0.3% (w/v) bile salt (Beijing Solarbio Science & Technology Co., Ltd.), respectively. The MRS culture medium served as the control. Following incubation for 3 and 24 h, the results were analyzed by evaluating the optical density at a wavelength of 600 nm. The survival rate was obtained by Equation 5:
2.3.2 Tolerance of gastric juice and intestinal juice
Lactobacillus plantarum suspension was inoculated into artificial gastric juice (Shanghai yuanye Bio-Technology Co., Ltd.) and artificial intestine juice, respectively. The survival rate was obtained by Equation 5.
2.4 Oral and intestinal correlation
2.4.1 The capability of Lactobacillus to scavenge glucose
Lactobacillus plantarum and S. mutans were inoculated in MRS and BHI broth containing 10% glucose. After being diluted to the same concentration (OD600 = 1). The glucose content after 24 h was measured by the glucose kit method (Nanjing Jiancheng Bioengineering Company, China), and the solution without bacterium was used as control. The content rate of glucose was obtained from Equation 6:
2.4.2 The ability of Lactobacillus plantarum to scavenge triglycerides
A 0.5% (v/v) inoculum of both L. plantarum and S. mutans cultures were introduced into Triglyceride-MRS and Triglyceride-BHI media, incubated at 37°C for 24 h. The triglyceride content was measured utilizing the triglyceride kit method, both with and without bacteria for comparative analysisthe triglyceride content ratio is calculated according to Equation 6.
2.5 In vivo effects of Lactobacillus plantarum
2.5.1 Animal model establishment
All procedures were conducted at the Animal Experimental Center of Jinzhou Medical University, with the animal studies receiving prior examination and approval from the Animal Ethics Committee of the same institution. The approval agreement number of animal program is 240,139. Male Sprague Dawley rats of 3-week-old were purchased and housed under controlled temperature (20°C ± 5°C) and Temperature (50% ± 10%) conditions. Rats were randomly allocated into three groups, each containing three rats. The control group (Control) was given conventional feed and sterile water, while the caries and obesity group (CA_OB) and caries and obesity treated with L. plantarum ATCC14917 group (LP) were given a high-fat and high-sugar feed and 5% sucrose water. The 56-day experiment included a 0–3 day antibiotic interference period during which ampicillin (0.5 μg/mL) inhibited the oral flora of the rats. The colonization time of S. mutans is 4–8 days. Before each use, a bacterial solution (108 CFU/mL) was made using BHI culture medium. Each rat was anesthetized by inhalation. Using sterile cotton swabs, dip the S. mutans suspension until it is saturated. Then, in turn, daub in the respective group’s teeth, tongue, and oral mucosa, remaining at each location for 15 s. Using a blunt syringe, rinse the rat’s mouth, making sure the solution reaches every area of the mouth and plays its full part. The experimental solution was 1 mL/tube. Rats handled once daily in the morning and evening. The LP group was given 2 × 108 CFU/mL L. plantarum bacterial solution orally from day 14 to day 56. Until the completion of the trial, L. plantarum was administered four times per week. To ensure microbial colonization, refrain from eating or drinking for 30 min prior to and following the inoculation. The same experimenter always performed the procedure. Every week, the rats’ weight was recorded. The animals were put down at the conclusion of the experiment, and samples were gathered.
2.5.2 Micro-CT observation
Mandibular specimens were scanned by Micro-CT [PINGSENG Healthcare (Kunshan) Inc.]. The enamel was separated from the mandible with a fixed threshold and the volume of enamel was calculated to evaluate the dental caries. The demineralization rate of enamel was obtained from Equation 7:
Where V0 was the volume of enamel in control group, Vt was the volume of enamel in LP group, and Vm was the volume of enamel in CA_OB group.
2.5.3 Analysis of serum biochemistry
Serum was extracted by centrifuging blood samples at 5000 × g for 10 min after the animals were put under anesthesia. The serum was stored at-80°C until further analysis.
Utilizing the assay kits, levels of Total triglyceride (TG), Total cholesterol (T-CHO), High-density lipoprotein-cholesterol (HDL), Low-density lipoprotein-cholesterol (LDL), and Blood glucose were evaluated (Nanjing Jiancheng Bioengineering Company, China).
2.5.4 Analysis of histology and staining
Hematoxylin and eosin (H&E) staining was performed on the rat liver and epididymis adipose tissues. Rat liver and epididymis fatty tissue was cleaned with regular saline, fixed for 24 h in a 10% paraformaldehyde solution, and the excess fixative was rinsed off with tap water. Following ethanol gradient dehydration, the tissue was embedded in paraffin and cut using a microtome into slices that were 4–5 microns thick. Following hematoxylin–eosin staining, followed by photography to document the findings.
2.5.5 16S rRNA gene sequencing of intestinal flora
Fresh feces from each rat were collected on the 57th day under aseptic conditions on the ultra-clean workstation, put in a 5/mL freezing tube, kept in the refrigerated at-80°C, and then delivered to the firm for testing. The FastPure Stool DNA Isolation Kit (MJYH, shanghai, China) was used to extract the total DNA. The hypervariable region V3–V4 of the bacterial 16S rRNA gene were amplified with primer pairs 338F (5’-ACTCCTACGGGAGGCAGCAG-3′) and 806R (5’-GGACTACHVGGGTWTCTAAT-3′). Identification, purification and quantification of PCR products were carried out. The sequencing was based on the platform of Shanghai Maggie Biomedical Technology Co., Ltd.
2.5.6 Statistical analysis
The statistical program GraphPad Prism 8.0.2 was utilized for the mapping and analysis. To analyze the differences among the groups, a one-way analysis of variance was utilized, with a significance level set at p < 0.05 to indicate noteworthy differences. Data were expressed as mean ± standard deviation, and each experimental condition was replicated three times to ensure reliability and consistency in the results obtained.
3 Results
3.1 Function of Lactobacillus plantarum in vitro
3.1.1 Antibacterial ability
Lactobacillus plantarum had a substantial inhibitory effect on S. mutans growth, and it measured 18.85 ± 1.05 mm in diameter (Figure 1A). Lactobacillus exhibited a 2.24 antibiosis activity to S. mutans. The aforementioned findings demonstrated that Lactobacillus had a potent antibacterial impact (Figures 1B,C).
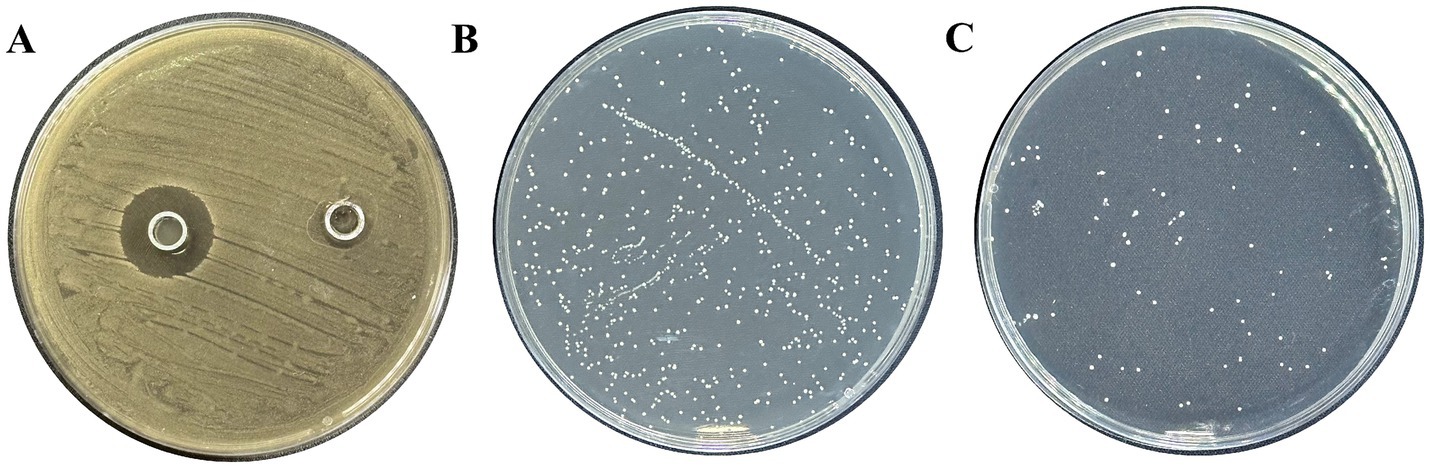
Figure 1. Inhibition of L. plantarum on S. mutans. (A) Inhibitory zone of L. plantarum on S. mutans, (B) CFU of S. mutans by MRS, (C) CFU of S. mutans by L. plantarum supernate. Data are represented as means ± standard deviation (SD). There were three biological duplicates (n = 3).
3.1.2 Determination of bacteriostatic curve
The bacteriostatic effects of the five groups of L. plantarum supernate started to take effect at 4 h, with the corresponding bacteriostatic rates being 60.90, 72.30, 79.18, 79.83, and 80.62%. The bacteriostatic efficacy of L. plantarum supernatant at concentrations of 12.5 and 25% declined with time, from 8 to 24 h (p < 0.05). Nonetheless, L. plantarum supernate still plays a good bacteriostatic role at concentrations of 50 to 100% (v/v), and the bacteriostatic rate was higher than 79% (p > 0.05) (Figure 2).
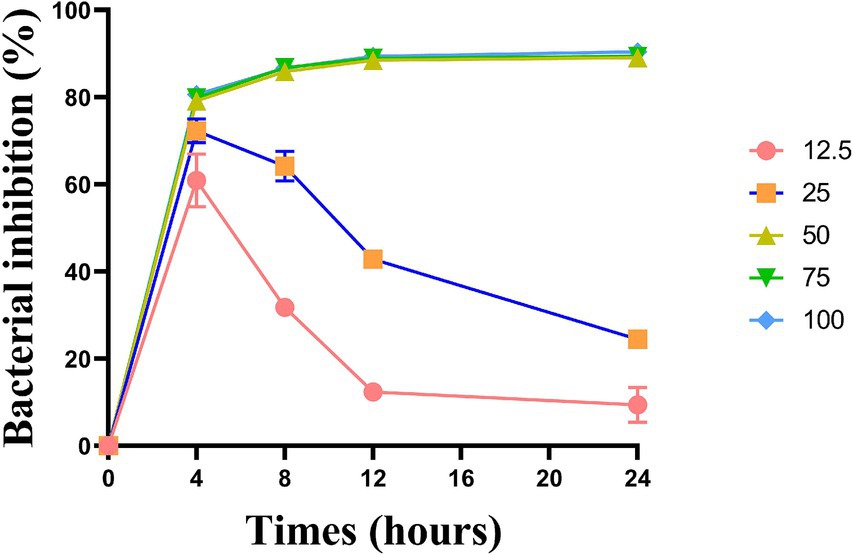
Figure 2. Inhibitory effect of L. plantarum supernate with concentration ranging from 12.5 to 100% (v/v) on the growth curve of S. mutans. Values are means ± SD. There were three biological duplicates (n = 3).
3.1.3 Proportional distribution of crystal violet biofilm and live/dead fluorescent staining
Studies have proved the influence of Lactobacillus on the biofilm of S. mutans within 24 h. L. plantarum supernate (0, 12.5, 25, 50, 75, 100%) exhibited varying degrees of inhibitory effects on S. mutans biofilm. The corresponding inhibitory rates were 15.91, 66, 80.79, and 83.99% (p > 0.05). The biofilm formation process is characterized by four crucial time points: the initial bacterial adhesion occurring at 0 h, initial bacterial colonization at 6 h, early biofilm development at 12 h, and the maturation of the biofilm at 24 h (Figure 3).
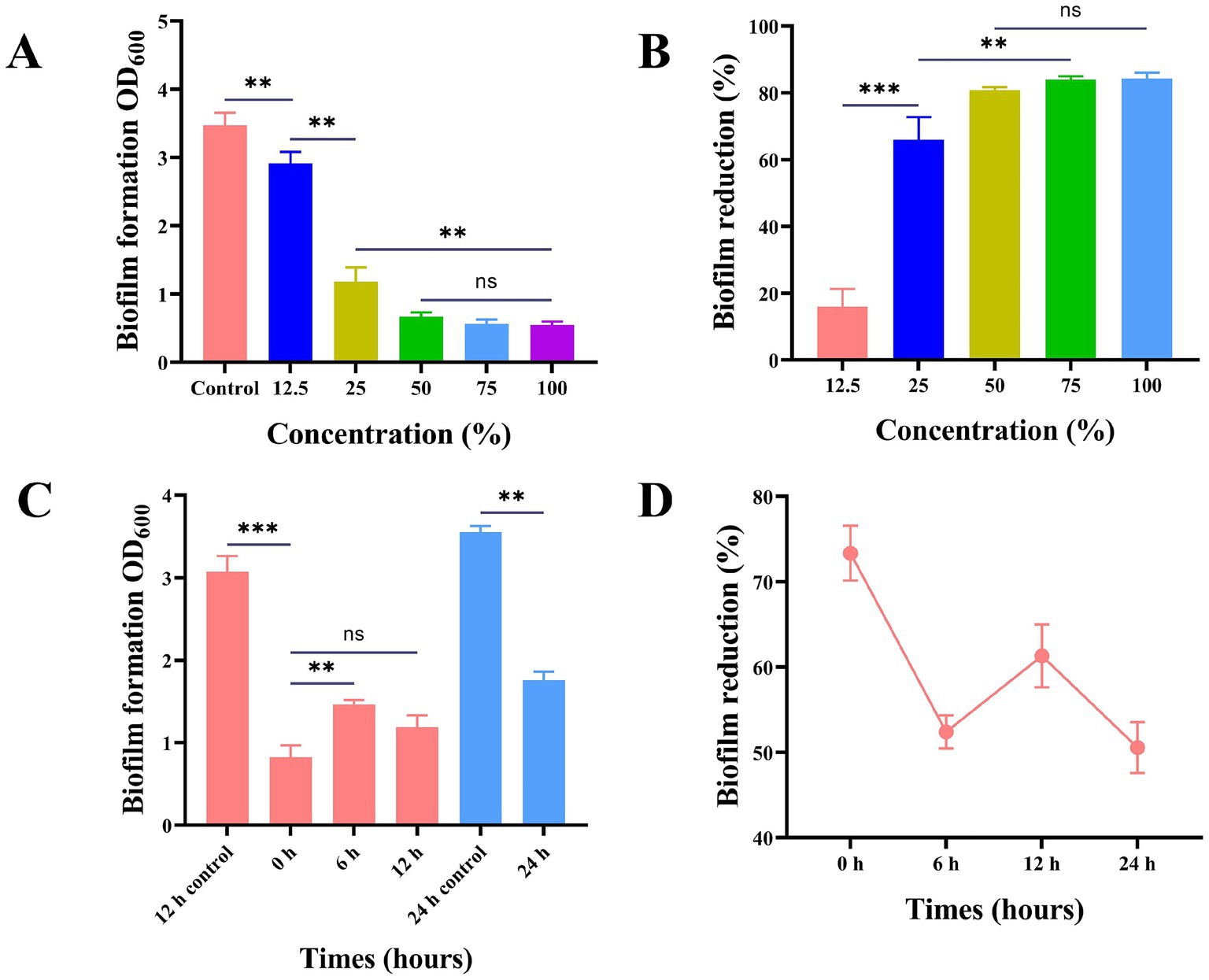
Figure 3. Effect of supernatant of L. plantarum on biofilm of S. mutans. (A) The anti-S. mutans biofilm effect of the L. plantarum supernate with the concentration ranging from 12.5 to 100% (v/v), (B) the reduction rate of biofilm of S. mutans, (C) the effect of adding L. plantarum supernate at different time points on the biomass of S. mutans, (D) Reduction rate of biofilm of S. mutans at various moments in time. Values are means ± SD. There were three biological duplicates (n = 3). * p < 0.05, ** p < 0.01, *** p < 0.001.
In addition, with the intervention of L. plantarum, fluorescent staining was also used to measure the biofilm activity of S. mutans. After 24 h of culture, it was found that the colonies in the control group covered the whole field of vision, and there was no gap in the middle. The membrane was a dense network structure, mainly with green fluorescence and scattered with red fluorescence (Figure 4A). Live bacteria decreased gradually, the block structure decreased, the biofilm became sparse gradually, the red part increased, and the activity of the biofilm was obviously inhibited (Figure 4B).
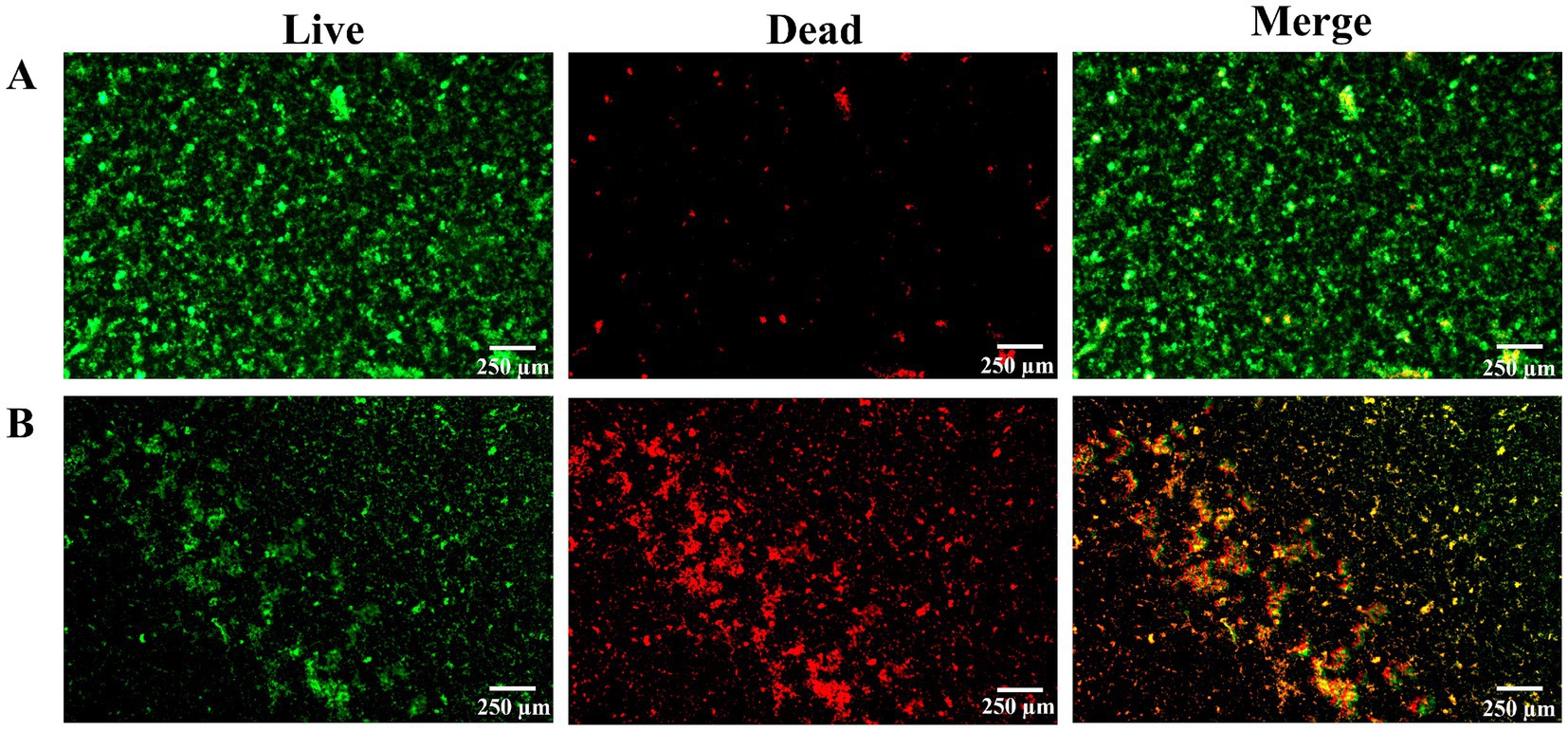
Figure 4. After 24 h of culture, observe the proportion of live and dead bacteria in biofilm by fluorescence staining for 30 min. (A) The control group, (B) the treatment group. Note: green was the live bacteria, red was the dead bacteria, and the superimposed color of the dead bacteria and the live bacteria was yellow or orange. There were three biological duplicates (n = 3).
3.1.4 Auto-aggregation and co-aggregation
With time, L. plantarum ATCC 14917’s capacity for self-aggregation grew. The auto-aggregation rates of the strain were observed over various time intervals: 2 h, 4 h, 6 h, and 24 h, yielding rates of 22.62, 31.56, 39.32, and 70.91%, respectively. At 2 hours, the co-aggregation ability was minimal; by 4 hours, it had clearly increased. The strain under test started to agglutinate with S. mutans, according to the data, and after 24 h, the rate of cross-agglutination had reached 48.32% (Figure 5).
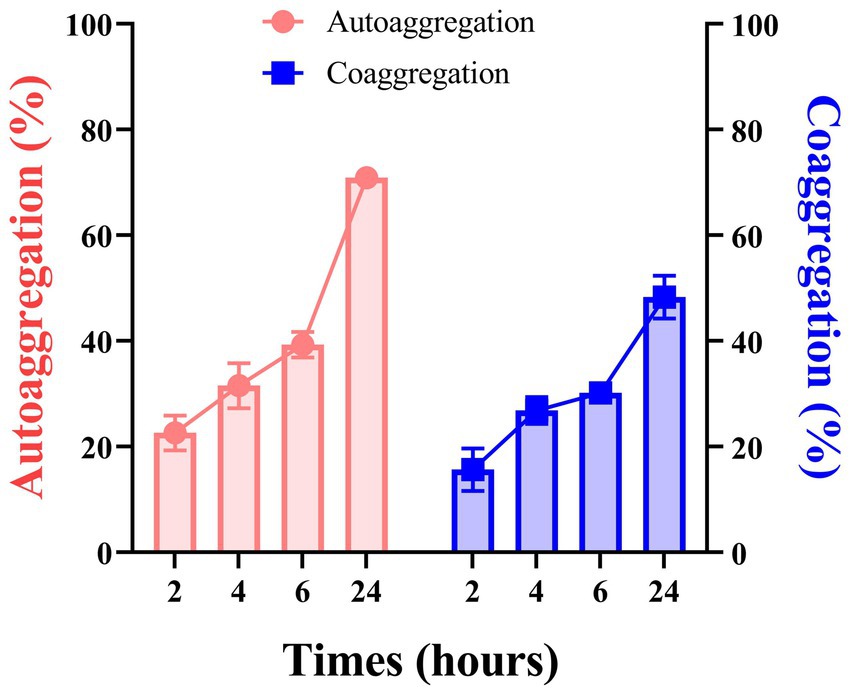
Figure 5. The auto-aggregation and co-aggregation ability of L. plantarum at 2 h, 4 h, 6 h, and 24 h. Data are represented as means ± SD. There were three biological duplicates (n = 3).
3.1.5 Tolerance of the digestive environment
The properties of probiotics were evaluated using the average intestinal bile salt content, which was 0.3% (w/v). Under conditions of low pH, the survival rate of L. plantarum decreased significantly, falling from 73.20 to 15.18%. For the duration ranging from three to twenty-four hours in a medium containing 0.3% bile salts, the survival rate remained consistently near 80% (Table 1).
The number of viable bacteria decreased by 0.68 and 0.3 after 3 hours, respectively. Lactic acid bacteria have a strong ability to adapt to the tough environment of the gastrointestinal tract, as evidenced by the fact that even while their development was limited, a constant survival rate could still be ensured (Table 2).
3.1.6 Determination of triglyceride and glucose
Bacteria require glucose as their primary growth substrate, and different strains exhibit diverse abilities to metabolize it. The amount of glucose content was found to be 32.54 and 236.13% in the culture medium, and the rate of lowering blood sugar and the increasing blood sugar are 67.46 and 136.13%, respectively (Figure 6A). On the other hand, the triglyceride content in the Triglyceride-MRS and Triglyceride-BHI media was 63.84 and 254.50%, respectively, and the lipid-lowering and fat-increasing rates were 36.16 and 154.50% (Figure 6B). The data above demonstrated the strain’s strong probiotic qualities.
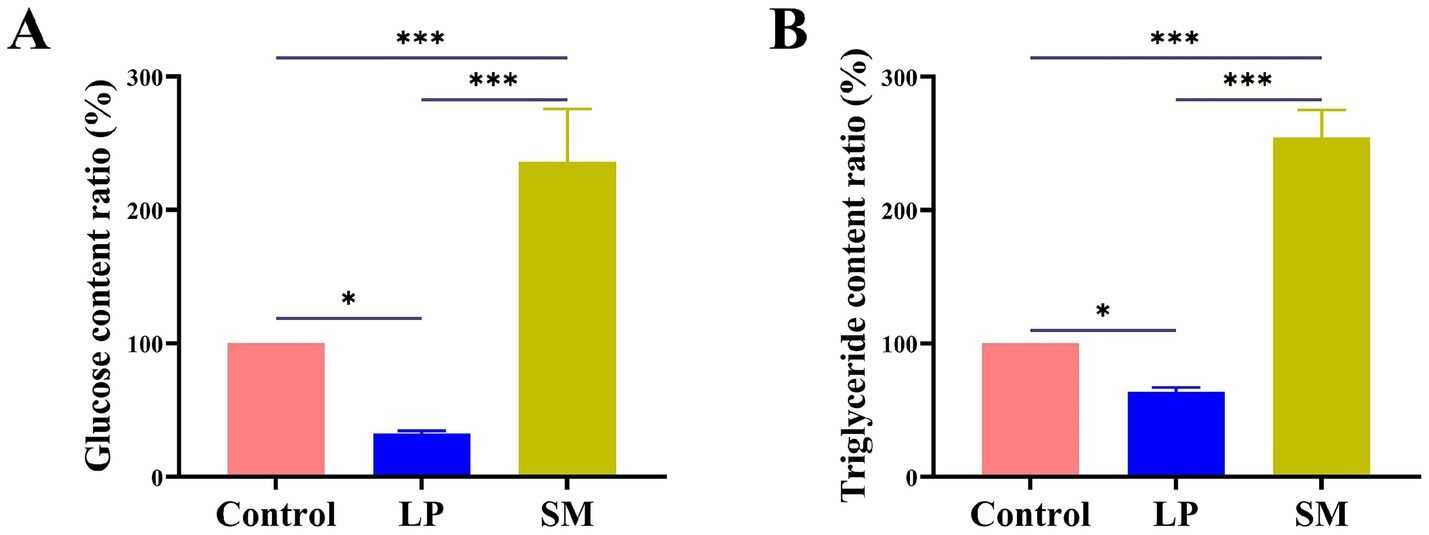
Figure 6. (A) Effects of L. plantarum and S. mutans on glucose, (B) effects of L. plantarm and S. mutans on triglyceride. Values are means ± SD. There were three biological duplicates (n = 3). * p < 0.05, ** p < 0.01, *** p < 0.001.
3.1.7 Micro-CT observation
Rat molar enamel volumes were computed for every experimental group. The model demonstrating the establishment of caries is further validated by the considerable amount of demineralized enamel found in the CA_OB group when compared to the Control group. The volume of demineralized enamel in LP group is similar to that in Control group. In addition, the demineralization rate of enamel of the LP group is similar to that of the Control group. The above results indicate that L. plantarum has an obvious inhibitory effect on dental caries in animal models (Figure 7).
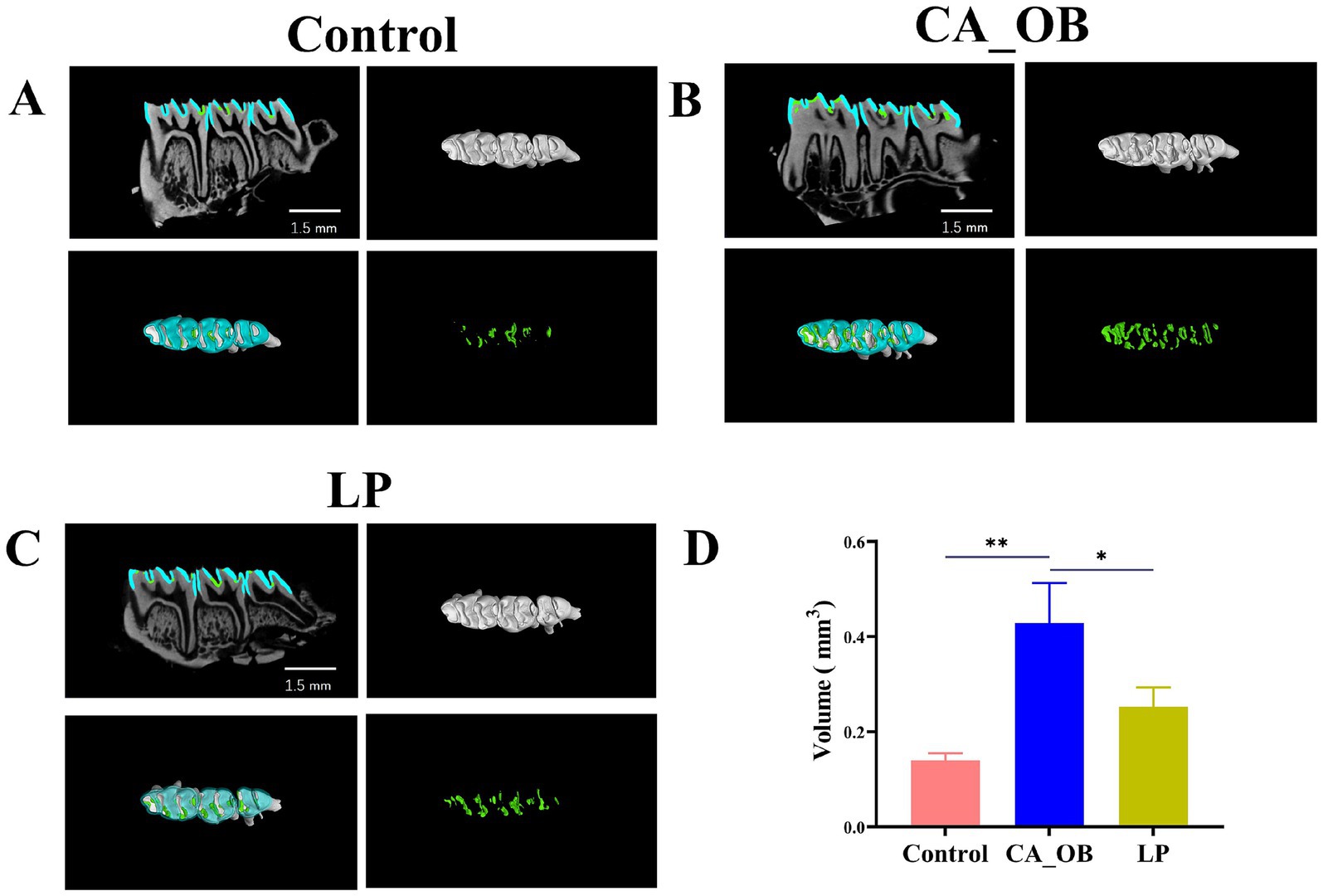
Figure 7. To observe the development of dental caries in rats. (A–C) The left maxillary tooth’s 3D microcomputer tomography pictures show demineralized enamel (cyan), two-dimensional axial and demineralized enamel (green), (D) demineralized enamel. Data are represented as means ± SD. There were three biological duplicates (n = 3). * p < 0.05, ** p < 0.01, *** p < 0.001.
3.1.8 Assessment of body weight and serum indices in rats
After an eight-week feeding period, a clear change in weight was noted among the rats in the LP group, with the CA_OB group displaying a much greater weight than their Control counterparts. Rats within the LP group showed significantly lower weights compared to those in the CA_OB group (Figure 8A). Compared with the Control group, the serum TG, T-CHO, LDL-C and Blood glucose levels in the CA_OB group were significantly increased, while the serum HDL-C level was significantly decreased, indicating that the blood lipid metabolism in CA_OB group was abnormal. However, the LP group significantly inhibited the lipid parameters in rat serum (Figures 8B–F).
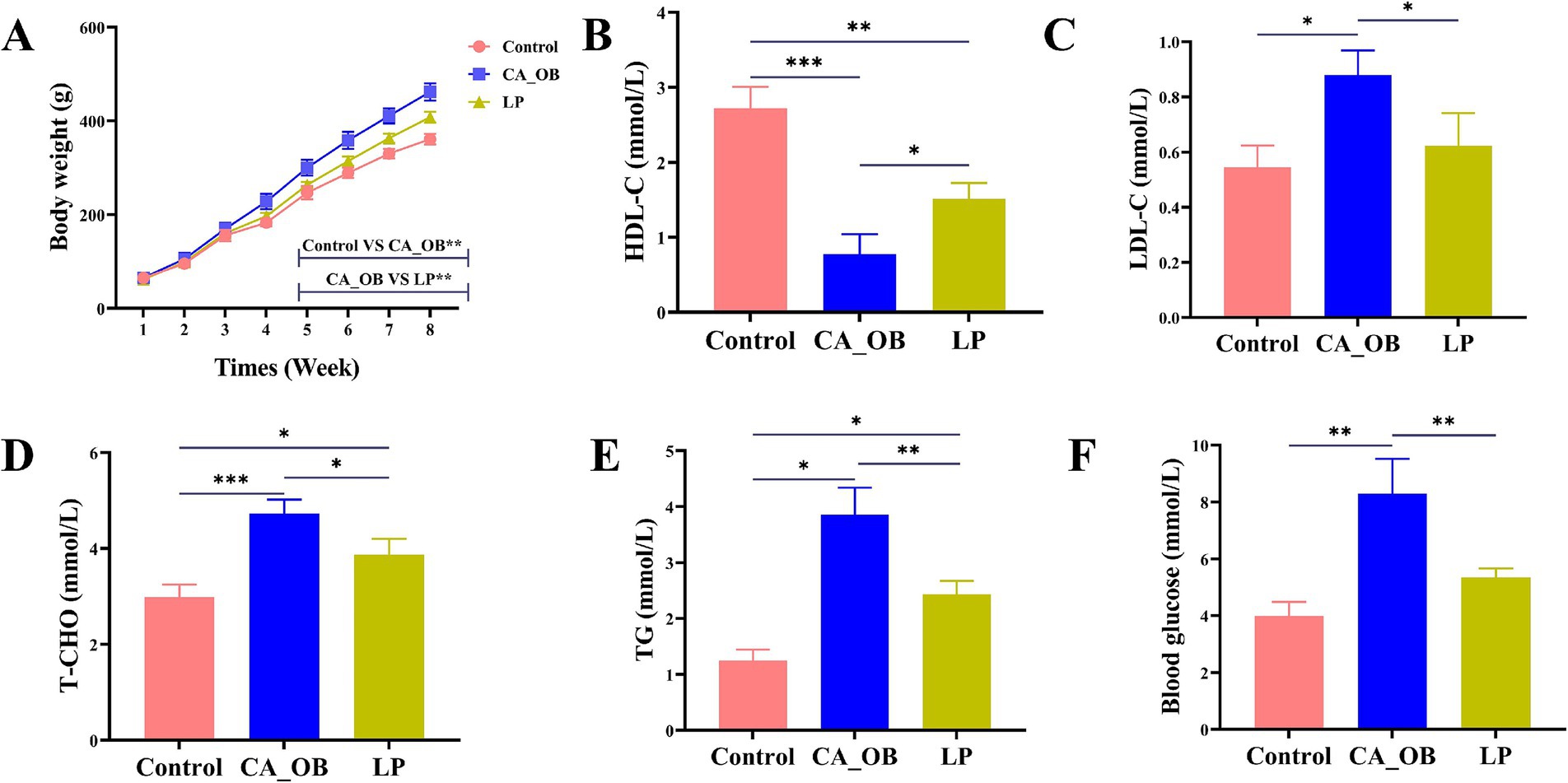
Figure 8. Rat weights and serum indices in relation to L. plantarum. (A) Change of rat weights within 8 weeks, (B) HDL, (C) LDL, (D) T-CHO, (E) TG, and (F) Blood glucose. Values are means ± SD. There were three biological duplicates (n = 3). * p < 0.05, ** p < 0.01, *** p < 0.001.
3.1.9 H&E staining sections of rat liver and fat
The results indicated no significant pathological changes in the liver tissue of rats from the Control group. In the CA_OB group, liver cells were obviously swollen, filled with fat vacuoles of different sizes, and inflammatory cells were obviously infiltrated. Rats from the LP group had far fewer fat vacuoles in their liver tissue, although there were still some localized inflammatory reactions (Figure 9A). The CA_OB group’s fat cells were noticeably larger and there were less cells in the same field of vision when compared to the group on a regular diet. Rat epididymal adipocyte hypertrophy may be inhibited following treatment with L. plantarum, suggesting that probiotic supplementation has a beneficial impact on lipogenesis (Figure 9B).
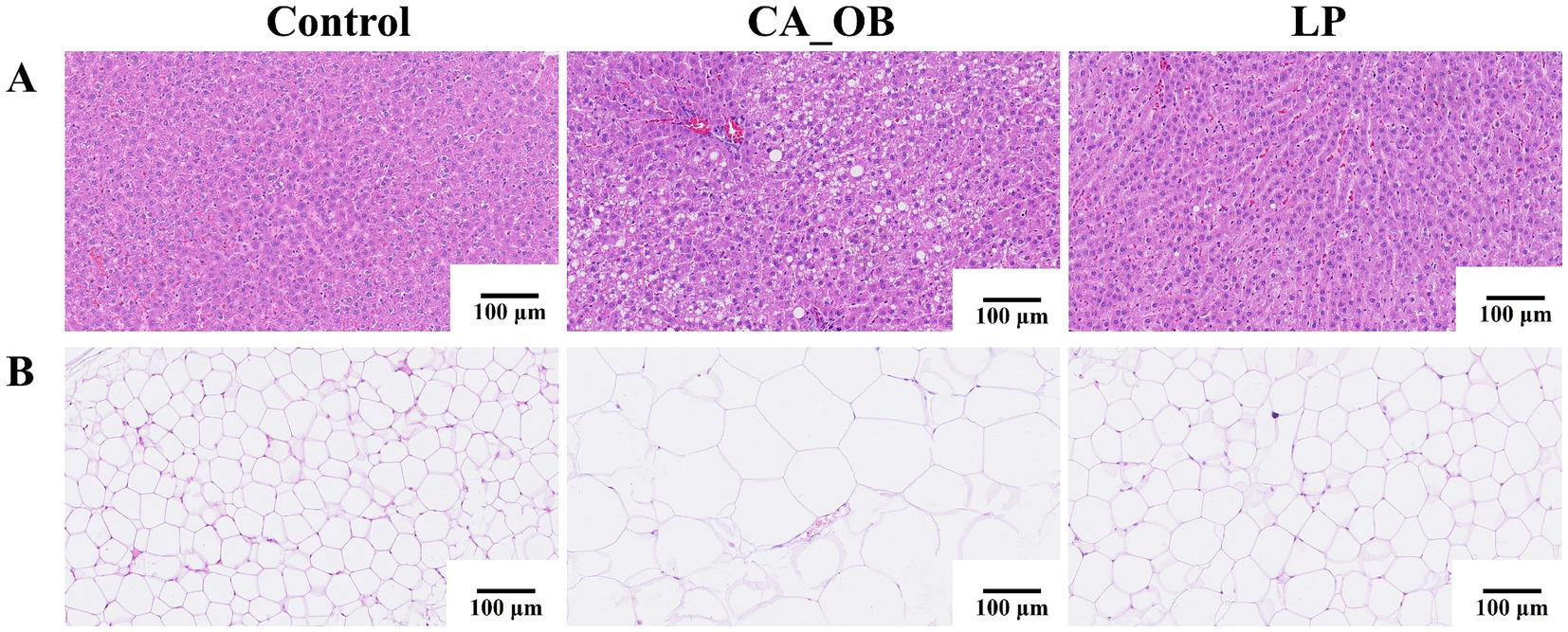
Figure 9. When rats are given L. plantarum, it can prevent their blood lipid levels from rising. (A) The morphological change of liver tissue in rats by H&E staining, (B) the change of epididymal adipose tissue in rats by H&E staining. Data are represented as means ± SD. There were three biological duplicates (n = 3).
3.1.10 16S rRNA gene sequencing of intestinal flora
To assess the diversity of bacterial communities, the dilution curve was primarily constructed using the Alpha diversity index from samples taken at various sequencing depths. The Shannon index is an important metric that tends to smooth out the dilution curve, serving as a reflection of the diversity present within microbial communities. This index indicates that the data volume gathered from each group is sufficient to meet the sequencing requirements (Figure 10A). Results from the Principal Coordinate Analysis highlighted that the contributions of Principal Component 1 and Principal Component 2 exceeded 60%, allowing for a comprehensive representation of the community composition. The LP group was similar to the Control group at the OTU level, and the CA_OB group and the LP group could be distinguished more clearly (Figure 10B). The PCoA data revealed that the LP group and the Control group nearly completely overlapped at the genus level, further supporting the idea that lactic acid bacteria might govern the gut flora (Figure 10C). To evaluate whether the differences between groups were statistically significant, we employed supervised partial least squares analysis. The findings from this analysis clearly illustrated a notable separation between the groups at both the OTU level and the genus level (Figures 10D,E). The ANOSIM analysis further showed the significant differences between groups (Figure 10F).
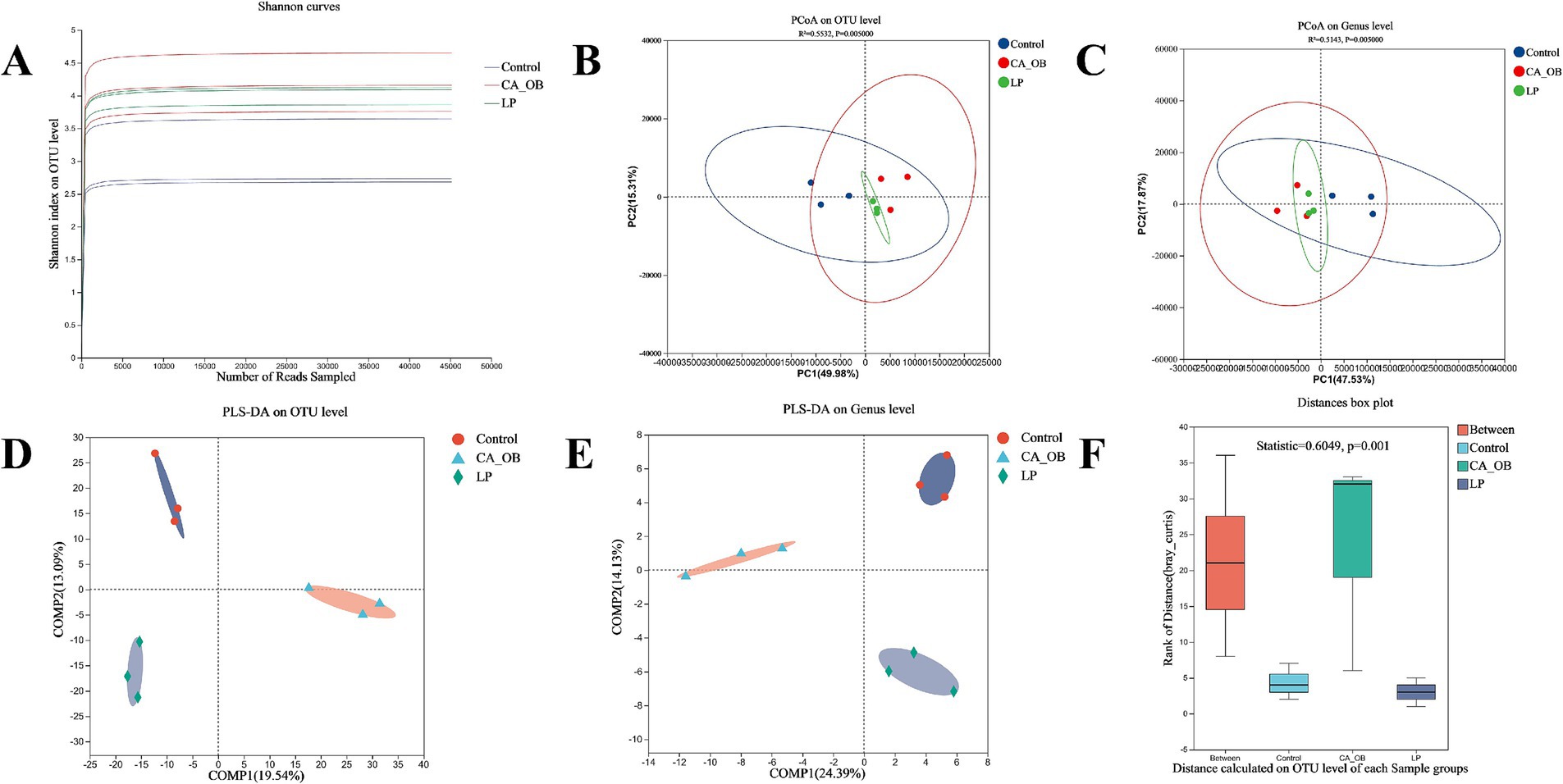
Figure 10. Effect of adding L. plantarum on the diversity of intestinal flora. (A) Shannon curves, (B) PCoA plot of the intestinal flora on OTU level, (C) PCoA on Genus level, (D) PLS-DA on OTU level, (E) PLS-DA on Genus level, (F) Beta diversity analysis of ANOSIM analyze on OTU level. There were three biological duplicates (n = 3). Principal coordinate analysis (PCoA); Partial least-squares discriminant analysis (PLS-DA); Analysis of similarities (ANOSIM); Operational taxonomic unit (OTU).
Intestinal microbiota may shield the host against pathogens that cause intestinal disorders in addition to enhancing nutrition absorption and preserving immunity. On the genus of level, the LP group increased relative abundance of Lactobacillus, Limosilactobacillus, unclassified_f__Muribaculaceae, Blautia, Faecalibaculum. The LP group led to the decrease of the abundance of pathogenic bacteria, including unclassified_o__Clostridia_UCG-014, unclassified_f__Oscillospiraceae, Turicibacter, unclassified_f__Lachnospiraceae, Clostridium_sensu_stricto_1 (Figure 11A).
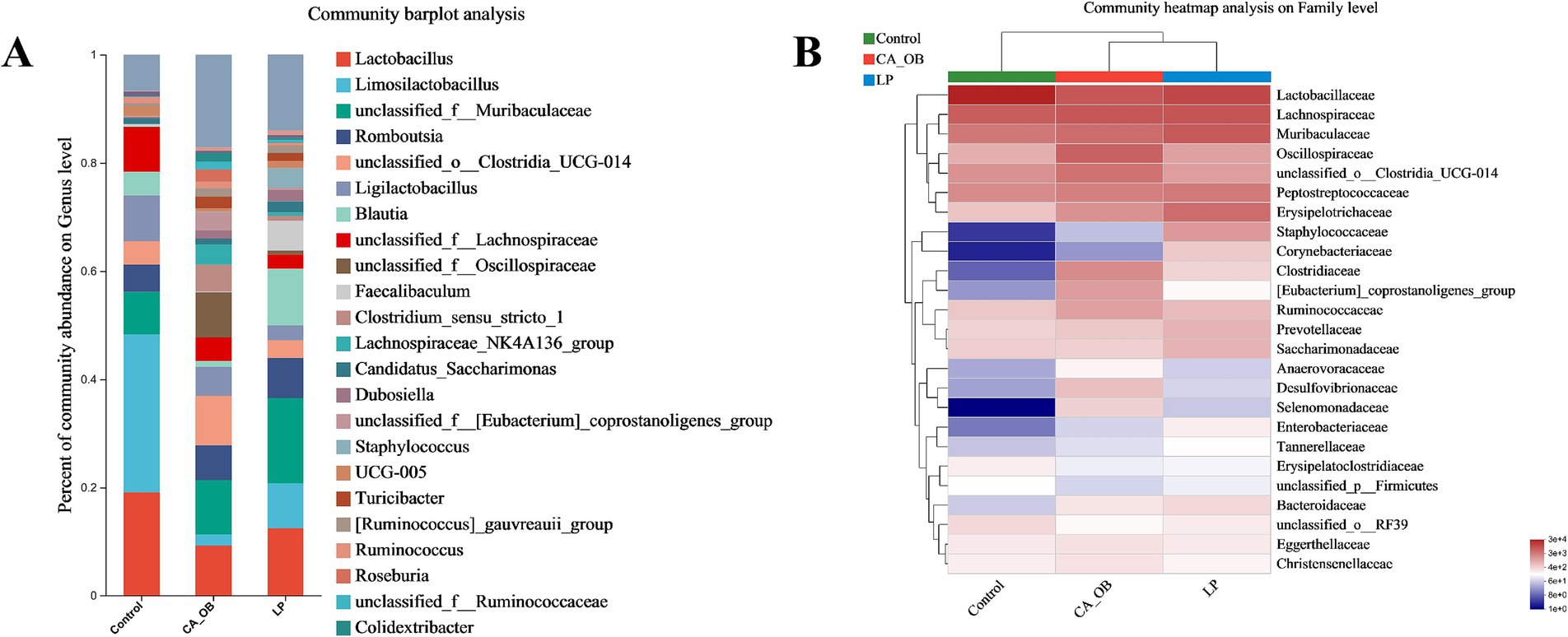
Figure 11. At different classification levels, the species composition and relative abundance of various species were counted. (A) Comparative genus-level abundance of the gut flora, (B) family-level gut flora’s relative abundance. There were three biological duplicates (n = 3).
On the family of level, the three groups of bacteria were mainly the following: Lactobacillaceae, Lachnospiraceae, Muribaculaceae, Oscillospiraceae, unclassified_o__Clostridia_UCG-014, Peptostreptococcaceae, Erysipelotrichaceae (Figure 11B).
This analysis illuminated the communities or species that exhibited significant variation across all taxonomic levels, including phylum, class, order, family, and genus. It was evident that the relative importance of microbial biomarkers escalated in tandem with increasing Linear Discriminant Analysis (LDA) scores. Compared with the Control group, in the CA_OB group, g__unclassified_f__[Eubacterium]_coprostanoligenes_group, f__Selenomonadaceae, g__unclassified_f__Oscillospiraceae, g__Quinella, g__unclassified_f__Desulfovibrionaceae. Contrasting to the CA_OB group, The profusion of g__Phascolarctobacterium, f__Acidaminococcaceae, g__Elusimicrobium and c__Elusimicrobia within the LP group was markedly elevated (Figures 12A,B). The degree of microbial ecological imbalance was measured using the microbial dysbiosis index. The degree of microbial imbalance increases with increasing value. In comparison to the Control group, the CA_OB group had a considerably higher microbial dysbiosis index. The microbial dysbiosis index clearly decreased following L. plantarum intervention, suggesting that L. plantarum may control the intestinal flora of CA_OB rats (Figure 12C).
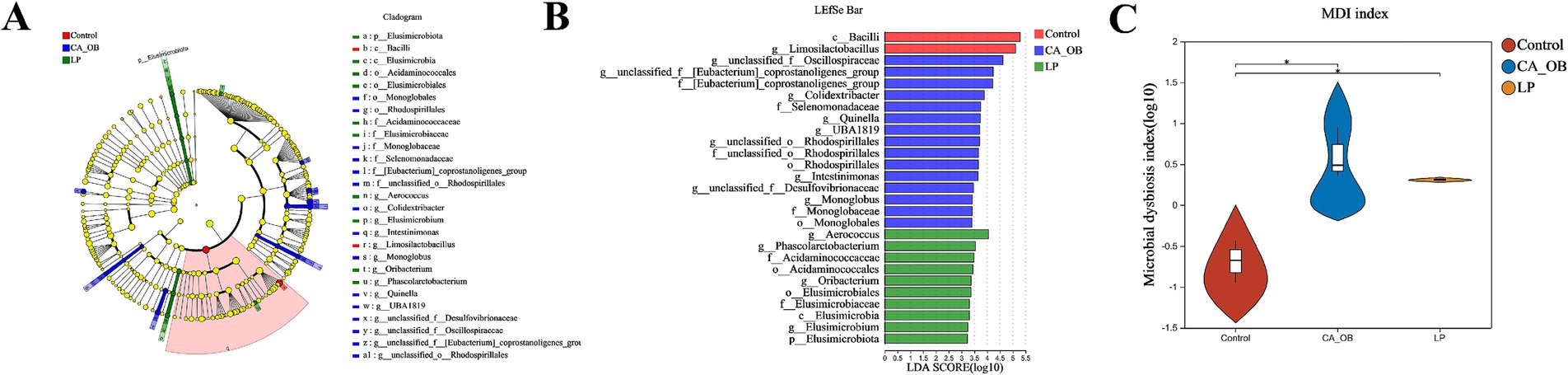
Figure 12. Analysis on interspecific differences of intestinal flora. (A,B) The LEfse multi-level species differences was conducted, (C) the microbial dysbiosis index in different groups. There were three biological duplicates (n = 3). Linear discriminant analysis effect size (LEfse).
Phylogenetic Investigation of Communities by Reconstruction of Unobserved States (PICRUSt2), Kyoto Encyclopedia of Genes and Genomes (KEGG), Evolutionary Genealogy of Genes: Non-supervised Orthologous Groups (EggNOG).
Bacterial colony function is largely associated with the metabolism pathway, according to the 16S rRNA sequencing data and KEGG functional predictions (Figure 13A). We could further demonstrate that bacteria are related to global and overview maps, carbohydrate metabolism, and amino acid metabolism by combining 16S rRNA sequencing data with KEGG data information of Pathway Level 2 (Figure 13B). Based on the 16S rRNA data and the PICRUSt2 channel display of EggNOG and KEGG databases, we predicted the biological functions of bacteria. 16S rRNA sequencing data combined with KEGG data information of Pathway Level 3, we could further confirm that bacteria are related to purine metabolism, peptidoglycan biosynthesis, oxidative phosphorylation, amino acid metabolism, streptomycin biosynthesis, nitrogen metabolism, taurine and hypotaurine metabolism (Figure 13C). In an effort to delve deeper into the functional roles of bacteria, we utilized the EggNOG database. Predictions indicated that the primary functions of the bacteria were closely associated with metabolic processes. These processes predominantly involved translation, the structure and biogenesis of ribosomes, as well as the transport and metabolism of amino acids and carbohydrates (Figure 13D).
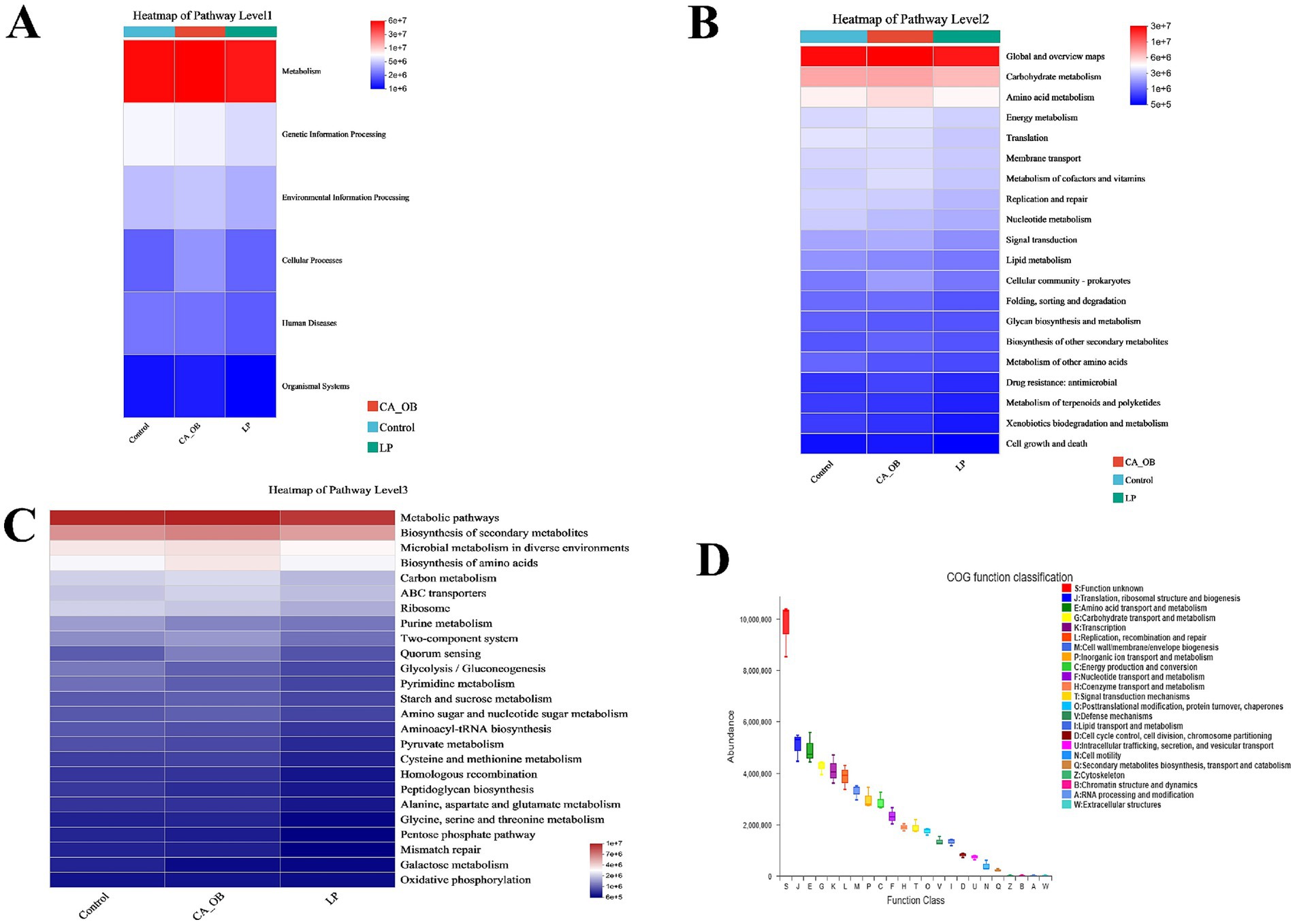
Figure 13. Prediction and analysis of intestinal flora function. (A–C) The function of PICRUSt2 in conjunction with the KEGG database to anticipate intestinal microorganisms showing result of the KEGG pathway at Level l (A), Level 2 (B) and Level 3 (C), (D) PICRUSt2 is combined with the EggNOG database to forecast the function of intestinal microorganisms. There were three biological duplicates (n = 3).
At the genus level, Limosilactobacillus was negatively connected with associated with obesity variables (TC, TG, LDL, Blood Glucose), while Clostridium_sensu_stricto_1, Turicibacter, unclassified_f__Oscillospiraceae, Colidextribacter, Quinella, Desulfovibrio were positively linked to some relating to obesity factors (Figure 14A). At the family level, Lactobacillaceae, unclassified_p__Firmicutes had a negative correlation with linked to obesity indexes, while Streptococcaceae, Desulfovibrionaceae, Clostridiaceae, Oscillospiraceae, Selenomonadaceae, UCG-010 were found to have a positive correlation with obesity measures (Figure 14B).
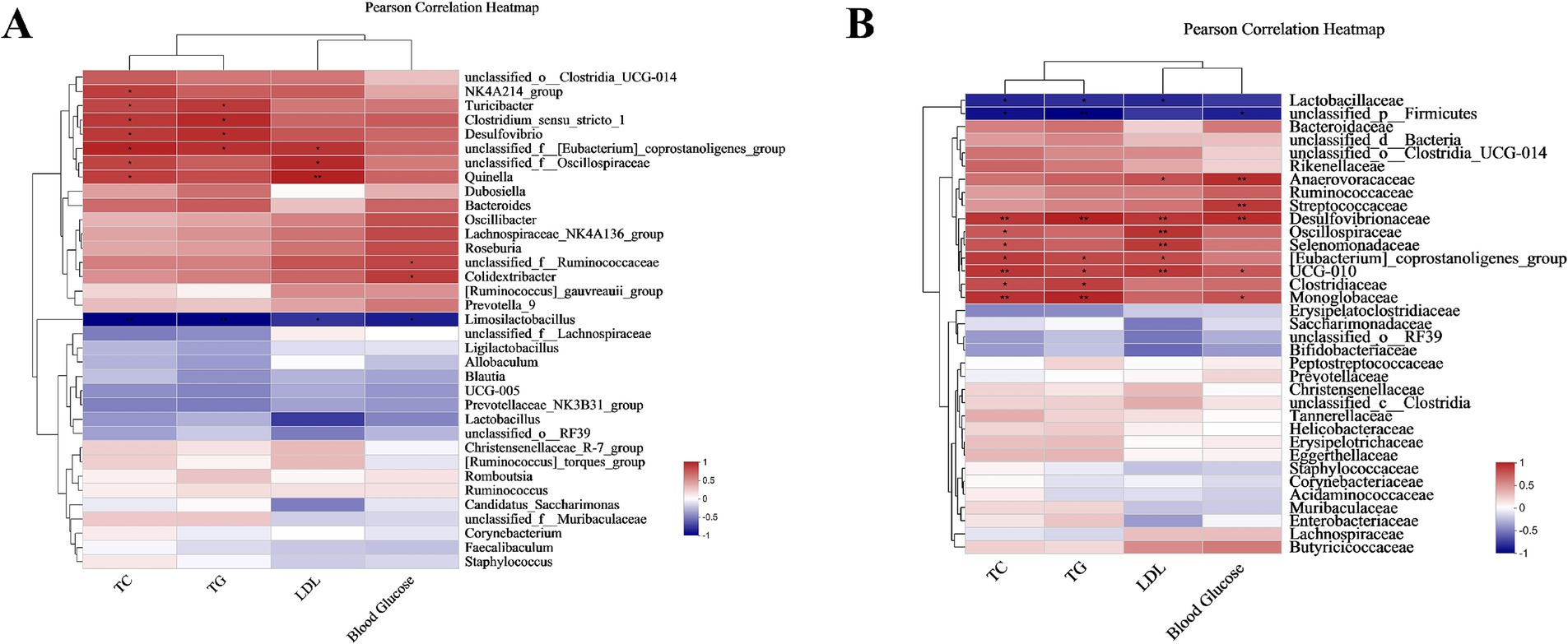
Figure 14. Pearson correlation between lipid metabolism index and microbiota. (A) Pearson correlation between the genus and lipid metabolism index. (B) Pearson correlation between the family and lipid metabolism index. Correlations were represented with a red color indicating positive relationships and blue indicating negative ones. There were three biological duplicates (n = 3). * p < 0.05, ** p < 0.01, *** p < 0.001.
4 Discussion
There is an urgent need for novel approaches to the prevention and management of dental caries and obesity, two disorders that have a negative impact on one’s physical and well-being due to their rising prevalence. One promising candidate is L. plantarum, a genus of Lactobacillus that has recently been reclassified and is noted for its extensive metabolic diversity (31, 32). Prior studies showed that L. plantarum ATCC14917 inhibited the growth of S. mutans, which causes dental caries, and broke down the structure of harmful biofilm (33). Research findings suggest that incorporating L. plantarum into health regimens may help mitigate the onset of conditions such as atherosclerosis and non-alcoholic fatty liver disease (34, 35). However, it was little known whether Lactobacillus will affect the treatment of clinical diseases with dental caries and obesity.
Biofilm was formed by microbial communities to withstand different environments and shield bacterial cells with tenacious adherence (36). The results showed that the yield was the lowest at 0 h, and the regulation effect on biofilm formation of S. mutans was better at 12 h, and early intervention had a better influence on biofilm formation. It was speculated that the antibacterial products in the supernatant of L. plantarum played a role and affected the initial adhesion and colonization of pathogenic bacteria. In the later stage of biofilm formation, L. plantarum supernate was still effective on the original mature S. mutans biofilm, which was similar to the previously reported results (37). A number of in vitro parameters, such as continuous human digestion, gastric juice and bile production, and pathogen resistance, can be used to assess the health advantages of Lactobacillus (38). L. plantarum has a high viable count in intestinal and simulated artificial gastric juice, which is in line with other study findings and suggests that the strain may withstand the hostile conditions of the gastrointestinal tract and thrive (39).
Probiotics’ ability to self-aggregate is typically what allows them to adhere to mucous membranes and epithelial cells’ surfaces (40). According to the current study, L. plantarum ATCC 14917’s capacity for self-aggregation grew over time. Through the processes of saliva ingestion, L. plantarum forms co-aggregates with pathogenic bacteria. This process effectively reduces the pathogenic bacteria that float within the oral cavity, thereby minimizing the potential for these harmful microorganisms to colonize the oral environment (41). The experimental results show that the strains to be tested have a higher copolymerization ability in the early stage (4 h) and a lower copolymerization ability in the late stage (24 h), which may be because the strains and determination times differ.
Dental caries was a typical biofilm-induced disease (42). Microorganisms present in the oral cavity are a crucial factor in the development of dental caries; however, they alone are insufficient, as the creation of cariogenic biofilm relies on the host’s dietary habits (43). It was noted that unlike S. mutans, none of the tested strains of Lactobacillus demonstrated a meaningful increase in biofilm formation and did not produce water-insoluble sticky extracellular polysaccharides under similar growth conditions when cultured in sucrose-enriched medium compared to when they were grown in a medium lacking sucrose (44, 45). Thus, we hypothesize that it might be because S. mutans continuously produces sugar using sucrose as a substrate. Conversely, L. plantarum, categorized as a heterotrophic bacterium, is limited to consuming carbohydrates and not able to make sugar, aligning with the in vitro experimental findings that showcased its anti-caries properties.
The oral cavity is an important passage into the human body (46). The first stage of digestion involves the mechanical breakdown of food by the teeth, which, in conjunction with saliva, forms a cohesive mass that subsequently progresses into the gastrointestinal tract (47). Thus, it seemed sense that probiotics would affect the microbial flora of the mouth cavity in the same way as they affect the intestinal system (48). It has been revealed that among the overweight population, the number of Firmicutes increased while the number of Bacteroides declined (49). S. mutans belongs to Firmicutes (50). The formation of fats is directly linked to the fermentation and absorption of polysaccharides produced by gut microorganisms through a balanced diet (51). Consequently, we conjectured that S. mutans would promote fat accumulation while L. plantarum might inhibit it. These findings suggest that the Lactobacillus may prevent obesity and tooth cavities from developing.
By creating an animal model, the function of L. plantarum ATCC 14917 in vivo was further confirmed. With particular emphasis on the density of hard tissue, Micro-CT was suggested as a trustworthy technique for determining the volume of tooth hard tissue (52). Consequently, the mandibular enamel volume was computed in this study. The results show that L. plantarum played a significant anti-caries role.
Furthermore, prolonged consumption of high-fat and high-sugar diets can significantly alter the diversity and composition of microbial communities within the gut, as well as modify the intestinal environment (53). Probiotics have the potential to directly alleviate diseases by modulating the ratio of good to harmful microorganisms within the microbial flora (54). Unclassified_f__Muribaculaceae belongs to Bacteroides S24-7, which usually exists in the intestinal microbiota of healthy mice (55). The findings of this study indicate that gymnosperms classified within the CA_OB group exhibited a lower abundance compared to those in the LP group. Moreover, the unclassified_f__Muribaculaceae was shown to play a significant role in regulating body weight, as well as in the degradation of food items and polysaccharides (56). This family is also responsible for the production of short-chain fatty acids (SCFAs), which include important metabolites such as succinic acid and propionic acid (57). In the context of clinical research, Lactobacillus and Bifidobacterium are among the most frequently employed probiotic strains. Lactobacillus can alter the synthesis pathway of lipids and carbohydrates, convert sugar to lactic acid (58).
Both unclassified_f__Ruminococcaceae and Ligilactobacillus have beneficial effects on the composition of intestinal microbiota, preserving the steady state of the intestinal milieu and emerging as important elements of the microbiota of healthy individuals (59, 60). Unclassified_f__Ruminococcaceae is one of the most effective bacterial genera for decomposing carbohydrates (61). It is a member of the Ruminococcaceae family of bacteria, multiple of which are known to make butyrate (62). SCFAs was found to be produced by the unclassified_f__[Eubacterium]_coprostanoligenes_group (63). It is positively correlated with fecal butyrate content. On a high-fat diet, it has been found that unclassified_f__[Eubacterium]_coprostanoligenes_group have a lipid-lowering impact (64). Limosilactobacillus can help with weight management, liver disease relief, increasing intestinal integrity, immunological regulation, and improving glucose homeostasis (65). Another lactic acid-producing bacterium, Escherichia coli, was found in the fecal samples, showing no significant difference across other groups. Its presence has been associated with anti-inflammatory properties and the enhancement of the intestinal barrier (66). The phylum Firmicutes includes the genus Blautia, which is recognized for its potential in mitigating intestinal inflammation (67). This inflammatory response has been linked to the regulation of G-protein coupled receptors, specifically GPR41 and GPR43 (68).
In the CA_OB group, the abundance of unclassified_o__Clostridia_UCG-014 and unclassified_f__Oscillospiraceae increased, and this was positively connected with serum indices. A conditional pathogen linked to numerous illnesses is unclassified_o__Clostridia_UCG-014 (69). Furthermore, it is believed that unclassified_f__Oscillospiraceae is connected to liver disorders (70) and damage (71). The CA_OB group exhibited a significantly higher abundance of Clostridium_sensu_stricto_1 and Turicibacte in comparison to the LP group. These genera contain significant pathogenic bacteria that, through causing toxins that lead to intestinal infections and encouraging chronic inflammation, contribute to the obesity process (72, 73). A type of bacteria found in the digestive tract called unclassified_f__Lachnospiraceae is linked to an increase in lipid levels and fat mass (74). Glucose and lipid metabolism are positively correlated with unclassified_f__Lachnospiraceae (75). Desulfovibrionaceae is a type of sulfate-reducing bacteria that produces more H2S (76). Inflammation results from H2S’s reduction of disulfide bonds in the mucus network, which breaks the mucus barrier and exposes epithelial cells to toxins and germs (77). According to some research, a probiotic-rich diet can reduce the quantity of Desulfovibrionaceae in obese people (78). The above results showed that L. plantarum could adjust the richness of other flora and the composition of intestinal flora, and play different functions.
5 Conclusion
This investigation also delved into the impacts of L. plantarum on both intestinal and oral health. The results demonstrated impressive antibacterial and anti-obesity effects of L. plantarum against the oral pathogen S. mutans in vitro. Through the use of Micro-CT imaging, the study confirmed a significant disparity in the incidence of dental caries between the LP and CA_OB groups. Furthermore, its strain improved fat accumulation in rat liver and markedly regulated the abnormality of lipid metabolism. Consequently, it was anticipated that L. plantarum ATCC 14917 would find applications in the medical and healthcare fields to help maintain the integrity of the intestinal barrier and the equilibrium of intestinal flora.
Data availability statement
The datasets presented in this article are not readily available because due to laboratory policies and confidentiality agreements, raw data cannot be provided. Requests to access the datasets should be directed to Xiaopeng Yang, ODI5MTA5MjNAcXEuY29t.
Ethics statement
The animal studies were approved by Experimental Animal Center of Jinzhou Medical University. The studies were conducted in accordance with the local legislation and institutional requirements. Written informed consent was obtained from the owners for the participation of their animals in this study.
Author contributions
WY: Writing – original draft, Formal analysis, Investigation. MJ: Conceptualization, Formal analysis, Writing – review & editing. BC: Software, Writing – review & editing. KJ: Data curation, Writing – review & editing. NM: Methodology, Writing – review & editing. YL: Validation, Writing – review & editing. MW: Visualization, Writing – review & editing. MB: Validation, Writing – review & editing. CW: Funding acquisition, Project administration, Supervision, Writing – review & editing. XY: Supervision, Conceptualization, Resources, Writing – review & editing.
Funding
The author(s) declare that financial support was received for the research, authorship, and/or publication of this article. This work was financially supported by the Applied Basic Research Program of Liaoning Province (2023JH2/101700071, 2022JH2/101300033), National Natural Science Foundation of China (U21A2074, 62375115), and Education Department Science and Technology Innovation team of Liaoning province (LJ222410160037).
Conflict of interest
The authors declare that the research was conducted in the absence of any commercial or financial relationships that could be construed as a potential conflict of interest.
Generative AI statement
The author(s) declare that no Generative AI was used in the creation of this manuscript.
Publisher’s note
All claims expressed in this article are solely those of the authors and do not necessarily represent those of their affiliated organizations, or those of the publisher, the editors and the reviewers. Any product that may be evaluated in this article, or claim that may be made by its manufacturer, is not guaranteed or endorsed by the publisher.
References
1. Shi, J, Wang, Q, Ruan, G, Chen, Y, Zhao, M, Shi, D, et al. Efficacy of probiotics against dental caries in children: a systematic review and meta-analysis. Crit Rev Food Sci Nutr. (2023) 63:9977–94. doi: 10.1080/10408398.2022.2077693
2. Wen, PYF, Chen, MX, Zhong, YJ, Dong, QQ, and Wong, HM. Global burden and inequality of dental caries, 1990 to 2019. J Dent Res. (2022) 101:392–9. doi: 10.1177/00220345211056247
3. Aron-Wisnewsky, J, Warmbrunn, MV, Nieuwdorp, M, and Clément, K. Metabolism and metabolic disorders and the microbiome: the intestinal microbiota associated with obesity, lipid metabolism, and metabolic health-pathophysiology and therapeutic strategies. Gastroenterology. (2021) 160:573–99. doi: 10.1053/j.gastro.2020.10.057
4. Peng, X, Cheng, L, You, Y, Tang, C, Ren, B, Li, Y, et al. Oral microbiota in human systematic diseases. Int J Oral Sci. (2022) 14:14. doi: 10.1038/s41368-022-00163-7
5. Bowen, WH, Burne, RA, Wu, H, and Koo, H. Oral biofilms: pathogens, matrix, and Polymicrobial interactions in microenvironments. Trends Microbiol. (2018) 26:229–42. doi: 10.1016/j.tim.2017.09.008
6. Chien, SC, Chandramouli, C, Lo, CI, Lin, CF, Sung, KT, Huang, WH, et al. Associations of obesity and malnutrition with cardiac remodeling and cardiovascular outcomes in Asian adults: a cohort study. PLoS Med. (2021) 18:e1003661. doi: 10.1371/journal.pmed.1003661
7. Zhang, Q, Bai, Y, Wang, W, Li, J, Zhang, L, Tang, Y, et al. Role of herbal medicine and gut microbiota in the prevention and treatment of obesity. J Ethnopharmacol. (2023) 305:116127. doi: 10.1016/j.jep.2022.116127
8. Manohar, N, Hayen, A, and Arora, A. Obesity and dental caries in early childhood: a systematic review protocol. JBI Evid Synth. (2020) 18:135–45. doi: 10.11124/JBISRIR-D-19-00058
9. Garrocho-Rangel, A, Martínez-López, I, Butrón-Téllez Girón, C, Pozos-Guillén, A, and Zavala-Alonso, V. Obesity/overweight and dental caries experience in children and adolescents: an umbrella review. Eur Arch Paediatr Dent. (2024) 25:779–89. doi: 10.1007/s40368-024-00920-8
10. Li, LW, Wong, HM, and McGrath, CP. Longitudinal association between obesity and dental caries in adolescents. J Pediatr. (2017) 189:149–154.e5. doi: 10.1016/j.jpeds.2017.06.050
11. Qadri, G, Alkilzy, M, Feng, YS, and Splieth, C. Overweight and dental caries: the association among German children. Int J Paediatr Dent. (2015) 25:174–82. doi: 10.1111/ipd.12110
12. Basha, S, Mohamed, RN, Swamy, HS, Ramamurthy, PH, and Sexena, V. Caries incidence among obese adolescents: a 3-year prospective study. Oral Health Prev Dent. (2017) 15:65–71. doi: 10.3290/j.ohpd.a37715
13. Rizzardi, KF, Indiani, C, Mattos-Graner, RO, de Sousa, ET, Nobre-Dos-Santos, M, and Parisotto, TM. Firmicutes levels in the mouth reflect the gut condition with respect to obesity and early childhood caries. Front Cell Infect Microbiol. (2021) 11:593734. doi: 10.3389/fcimb.2021.593734
14. Khattak, O, Iqbal, A, Chaudhary, FA, Syed, J, Alsharari, T, Vundavalli, S, et al. Evaluating a linkage between obesity and the occurrence of dental caries among school going children in Sakaka, Al Jouf, Kingdom of Saudi Arabia. PeerJ. (2022) 10:e13582. doi: 10.7717/peerj.13582
15. Ashour, NA, Ashour, AA, and Basha, S. Association between body mass index and dental caries among special care female children in Makkah City. Ann Saudi Med. (2018) 38:28–35. doi: 10.5144/0256-4947.2017.31.12.1515
16. Leme, L, Rizzardi, KF, Santos, IB, and Parisotto, TM. Exploring the relationship between salivary levels of TNF-α, Lactobacillus acidophilus, Lactobacillus gasseri, obesity, and caries in early childhood. Pathogens. (2022) 11. doi: 10.3390/pathogens11050579
17. Manohar, N, Hayen, A, Fahey, P, and Arora, A. Obesity and dental caries in early childhood: a systematic review and meta-analyses. Obes Rev. (2020) 21:e12960. doi: 10.1111/obr.12960
18. Bakhoda, MR, Haghighat Lari, MM, Khosravi, G, Khademi, Z, Abbasi, F, and Milajerdi, A. Childhood obesity in relation to risk of dental caries: a cumulative and dose-response systematic review and meta-analysis. BMC Oral Health. (2024) 24:966. doi: 10.1186/s12903-024-04733-5
19. Kumar, S, Kroon, J, Lalloo, R, Kulkarni, S, and Johnson, NW. Relationship between body mass index and dental caries in children, and the influence of socio-economic status. Int Dent J. (2017) 67:91–7. doi: 10.1111/idj.12259
20. Baker, SR, Foster Page, L, Thomson, WM, Broomhead, T, Bekes, K, Benson, PE, et al. Structural determinants and Children's Oral health: a cross-National Study. J Dent Res. (2018) 97:1129–36. doi: 10.1177/0022034518767401
21. Ballo, L, Arheiam, A, and Marhazlinda, J. Determinants of caries experience and the impact on the OHRQOL of 6-year-old Libyan children: a cross-sectional survey. BMC Oral Health. (2021) 21:320. doi: 10.1186/s12903-021-01681-2
22. Su, Y, Ren, J, Zhang, J, Zheng, J, Zhang, Q, Tian, Y, et al. Lactobacillus paracasei JY062 alleviates glucolipid metabolism disorders via the Adipoinsular Axis and gut microbiota. Nutrients. (2024) 16. doi: 10.3390/nu16020267
23. Bao, J, Li, L, Zhang, Y, Wang, M, Chen, F, Ge, S, et al. Periodontitis may induce gut microbiota dysbiosis via salivary microbiota. Int J Oral Sci. (2022) 14:32. doi: 10.1038/s41368-022-00183-3
24. Lu, Y, Li, Z, and Peng, X. Regulatory effects of oral microbe on intestinal microbiota and the illness. Front Cell Infect Microbiol. (2023) 13:1093967. doi: 10.3389/fcimb.2023.1093967
25. Mohamed, RN, Basha, S, Al-Thomali, Y, AlZahrani, FS, Ashour, AA, and Almutair, NE. Association between early childhood caries and obesity among preschool children. Oral Health Prev Dent. (2022) 20:113–8. doi: 10.3290/j.ohpd.b2805445
26. Wu, H, Ma, Y, Peng, X, Qiu, W, Kong, L, Ren, B, et al. Antibiotic-induced dysbiosis of the rat oral and gut microbiota and resistance to Salmonella. Arch Oral Biol. (2020) 114:104730. doi: 10.1016/j.archoralbio.2020.104730
27. Homayouni Rad, A, Pourjafar, H, and Mirzakhani, E. A comprehensive review of the application of probiotics and postbiotics in oral health. Front Cell Infect Microbiol. (2023) 13:1120995. doi: 10.3389/fcimb.2023.1120995
28. Echegaray, N, Yilmaz, B, Sharma, H, Kumar, M, Pateiro, M, Ozogul, F, et al. A novel approach to Lactiplantibacillus plantarum: from probiotic properties to the omics insights. Microbiol Res. (2023) 268:127289. doi: 10.1016/j.micres.2022.127289
29. Li, P, He, X, Feng, E, Wei, J, Tu, H, and Chen, T. Lactobacillus acidophilus JYLA-126 ameliorates obesity-associated metabolic disorders by positively regulating the AMPK signaling pathway through the gut-liver Axis. Probiotics Antimicrob Proteins. (2023). doi: 10.1007/s12602-023-10190-3
30. Garcia-Gonzalez, N, Battista, N, Prete, R, and Corsetti, A. Health-promoting role of Lactiplantibacillus plantarum isolated from fermented foods. Microorganisms. (2021) 9. doi: 10.3390/microorganisms9020349
31. Reuben, RC, Roy, PC, Sarkar, SL, Rubayet Ul Alam, ASM, and Jahid, IK. Characterization and evaluation of lactic acid bacteria from indigenous raw milk for potential probiotic properties. J Dairy Sci. (2020) 103:1223–37. doi: 10.3168/jds.2019-17092
32. Yilmaz, B, Bangar, SP, Echegaray, N, Suri, S, Tomasevic, I, Manuel Lorenzo, J, et al. The impacts of Lactiplantibacillus plantarum on the functional properties of fermented foods: A review of current knowledge. Microorganisms. (2022) 10. doi: 10.3390/microorganisms10040826
33. Zeng, Y, Fadaak, A, Alomeir, N, Wu, TT, Rustchenko, E, Qing, S, et al. Lactobacillus plantarum Disrupts S. mutans-C. Albicans cross-kingdom biofilms. Front Cell Infect Microbiol. (2022) 12:872012. doi: 10.3389/fcimb.2022.872012
34. Hassan, A, Din, AU, Zhu, Y, Zhang, K, Li, T, Wang, Y, et al. Anti-atherosclerotic effects of Lactobacillus plantarum ATCC 14917 in ApoE(−/−) mice through modulation of proinflammatory cytokines and oxidative stress. Appl Microbiol Biotechnol. (2020) 104:6337–50. doi: 10.1007/s00253-020-10693-x
35. Wen, X, Liu, H, Luo, X, Lui, L, Fan, J, Xing, Y, et al. Supplementation of Lactobacillus plantarum ATCC14917 mitigates non-alcoholic fatty liver disease in high-fat-diet-fed rats. Front Microbiol. (2023) 14:1146672. doi: 10.3389/fmicb.2023.1146672
36. Li, J, Wang, H, Li, N, Zhang, Y, Lü, X, and Liu, B. Antibiotic susceptibility and biofilm-forming ability of Veillonella strains. Anaerobe. (2022) 78:102667. doi: 10.1016/j.anaerobe.2022.102667
37. Wasfi, R, Abd El-Rahman, OA, Zafer, MM, and Ashour, HM. Probiotic Lactobacillus sp. inhibit growth, biofilm formation and gene expression of caries-inducing Streptococcus mutans. J Cell Mol Med. (2018) 22:1972–83. doi: 10.1111/jcmm.13496
38. Liu, C, Han, F, Cong, L, Sun, T, Menghe, B, and Liu, W. Evaluation of tolerance to artificial gastroenteric juice and fermentation characteristics of Lactobacillus strains isolated from human. Food Sci Nutr. (2022) 10:227–38. doi: 10.1002/fsn3.2662
39. Vougiouklaki, D, Tsironi, T, Tsantes, AG, Tsakali, E, Van Impe, JFM, and Houhoula, D. Probiotic properties and antioxidant activity in vitro of lactic acid Bacteria. Microorganisms. (2023) 11. doi: 10.3390/microorganisms11051264
40. Beldarrain-Iznaga, T, Villalobos-Carvajal, R, Sevillano-Armesto, E, and Leiva-Vega, J. Functional properties of Lactobacillus casei C24 improved by microencapsulation using multilayer double emulsion. Food Res Int. (2021) 141:110136. doi: 10.1016/j.foodres.2021.110136
41. Fan, S, Xue, T, Bai, B, Bo, T, and Zhang, J. Probiotic properties including the antioxidant and hypoglycemic ability of lactic acid Bacteria from fermented grains of Chinese baijiu. Food Secur. (2022) 11. doi: 10.3390/foods11213476
42. Chu, W, Tang, H, Dong, Z, Hou, A, Qiu, R, Xu, X, et al. A multifunctional polymeric coating with self-adsorbed, antifouling and in situ remineralization properties for caries management. J Mater Chem B. (2023) 11:5151–62. doi: 10.1039/D3TB00377A
43. Sedghi, L, DiMassa, V, Harrington, A, Lynch, SV, and Kapila, YL. The oral microbiome: role of key organisms and complex networks in oral health and disease. Periodontol 2000. (2021) 87:107–31. doi: 10.1111/prd.12393
44. Fitzgerald, RJ, Adams, BO, Fitzgerald, DB, and Knox, KW. Cariogenicity of human plaque lactobacilli in gnotobiotic rats. J Dent Res. (1981) 60:919–26. doi: 10.1177/00220345810600051201
45. Fitzgerald, RJ, Fitzgerald, DB, Adams, BO, and Duany, LF. Cariogenicity of human oral lactobacilli in hamsters. J Dent Res. (1980) 59:832–7. doi: 10.1177/00220345800590051501
46. Kitamoto, S, Nagao-Kitamoto, H, Jiao, Y, Gillilland, MG 3rd, Hayashi, A, Imai, J, et al. The Intermucosal connection between the mouth and gut in commensal Pathobiont-driven colitis. Cell. (2020) 182:447–462.e14. doi: 10.1016/j.cell.2020.05.048
47. Olsen, I, and Yamazaki, K. Can oral bacteria affect the microbiome of the gut? J Oral Microbiol. (2019) 11:1586422. doi: 10.1080/20002297.2019.1586422
48. Burton, JP, Wescombe, PA, Macklaim, JM, Chai, MH, Macdonald, K, Hale, JD, et al. Persistence of the oral probiotic Streptococcus salivarius M18 is dose dependent and megaplasmid transfer can augment their bacteriocin production and adhesion characteristics. PLoS One. (2013) 8:e65991. doi: 10.1371/journal.pone.0065991
49. Li, J, Wang, M, Ma, S, Jin, Z, Yin, H, and Yang, S. Association of gastrointestinal microbiome and obesity with gestational diabetes mellitus-an updated globally based review of the high-quality literatures. Nutr Diabetes. (2024) 14:31. doi: 10.1038/s41387-024-00291-5
50. Michels, N, Zouiouich, S, Vanderbauwhede, B, Vanacker, J, Indave Ruiz, BI, and Huybrechts, I. Human microbiome and metabolic health: An overview of systematic reviews. Obes Rev. (2022) 23:e13409. doi: 10.1111/obr.13409
51. Vetrani, C, Di Nisio, A, Paschou, SA, Barrea, L, Muscogiuri, G, Graziadio, C, et al. R. On behalf of the obesity programs of nutrition education, G. Assessment opera, from gut microbiota through Low-grade inflammation to obesity: key players and potential targets. Nutrients. (2022) 14. doi: 10.3390/nu14102103
52. Oliveira, LB, Massignan, C, Oenning, AC, Rovaris, K, Bolan, M, Porporatti, AL, et al. Validity of micro-CT for in vitro caries detection: a systematic review and meta-analysis. Dentomaxillofac Radiol. (2020) 49:20190347. doi: 10.1259/dmfr.20190347
53. Alarcón Yempén, RE, Venzel, R, Paulino Campos, MC, de Oliveira, LP, Lins, RVD, Pessoni, AM, et al. Gut microbiota: a potential therapeutic target for management of diabetic retinopathy? Life Sci. (2021) 286:120060. doi: 10.1016/j.lfs.2021.120060
54. Guo, M, Liu, H, Yu, Y, Zhu, X, Xie, H, Wei, C, et al. Lactobacillus rhamnosus GG ameliorates osteoporosis in ovariectomized rats by regulating the Th17/Treg balance and gut microbiota structure. Gut Microbes. (2023) 15:2190304. doi: 10.1080/19490976.2023.2190304
55. Chung, YW, Gwak, HJ, Moon, S, Rho, M, and Ryu, JH. Functional dynamics of bacterial species in the mouse gut microbiome revealed by metagenomic and metatranscriptomic analyses. PLoS One. (2020) 15:e0227886. doi: 10.1371/journal.pone.0227886
56. Lee, PS, Teng, CY, Kalyanam, N, Ho, CT, and Pan, MH. Garcinol reduces obesity in high-fat-diet-fed mice by modulating gut microbiota composition. Mol Nutr Food Res. (2019) 63:e1800390. doi: 10.1002/mnfr.201800390
57. Zhou, Y, Zhang, Y, Qian, Y, Tang, L, Zhou, T, Xie, Y, et al. Ziyuglycoside II attenuated OVX mice bone loss via inflammatory responses and regulation of gut microbiota and SCFAs. Int Immunopharmacol. (2024) 132:112027. doi: 10.1016/j.intimp.2024.112027
58. Vemuri, R, Gundamaraju, R, Shinde, T, Perera, AP, Basheer, W, Southam, B, et al. Lactobacillus acidophilus DDS-1 modulates intestinal-specific microbiota, short-chain fatty acid and immunological profiles in aging mice. Nutrients. (2019) 11. doi: 10.3390/nu11061297
59. Lee, G, You, HJ, Bajaj, JS, Joo, SK, Yu, J, Park, S, et al. Distinct signatures of gut microbiome and metabolites associated with significant fibrosis in non-obese NAFLD. Nat Commun. (2020) 11:4982. doi: 10.1038/s41467-020-18754-5
60. Liu, Y, Zhong, X, Lin, S, Xu, H, Liang, X, Wang, Y, et al. Limosilactobacillus reuteri and caffeoylquinic acid synergistically promote adipose browning and ameliorate obesity-associated disorders. Microbiome. (2022) 10:226. doi: 10.1186/s40168-022-01430-9
61. Kubasova, T, Kollarcikova, M, Crhanova, M, Karasova, D, Cejkova, D, Sebkova, A, et al. Gut anaerobes capable of chicken Caecum colonisation. Microorganisms. (2019) 7. doi: 10.3390/microorganisms7120597
62. Kim, YJ, Jung, DH, and Park, CS. Important roles of Ruminococcaceae in the human intestine for resistant starch utilization. Food Sci Biotechnol. (2024) 33:2009–19. doi: 10.1007/s10068-024-01621-0
63. Zheng, DW, Li, RQ, An, JX, Xie, TQ, Han, ZY, Xu, R, et al. Prebiotics-encapsulated probiotic spores regulate gut microbiota and suppress Colon Cancer. Adv Mater. (2020) 32:e2004529. doi: 10.1002/adma.202004529
64. Wei, W, Jiang, W, Tian, Z, Wu, H, Ning, H, Yan, G, et al. Streptococcus and g. Eubacterium_coprostanoligenes_group combined with sphingosine to modulate the serum dyslipidemia in high-fat diet mice. Clin Nutr. (2021) 40:4234–45. doi: 10.1016/j.clnu.2021.01.031
65. Luo, Z, Chen, A, Xie, A, Liu, X, Jiang, S, and Yu, R. Limosilactobacillus reuteri in immunomodulation: molecular mechanisms and potential applications. Front Immunol. (2023) 14:1228754. doi: 10.3389/fimmu.2023.1228754
66. Vallianou, N, Stratigou, T, Christodoulatos, GS, and Dalamaga, M. Understanding the role of the gut microbiome and microbial metabolites in obesity and obesity-associated metabolic disorders: current evidence and perspectives. Curr Obes Rep. (2019) 8:317–32. doi: 10.1007/s13679-019-00352-2
67. Hosomi, K, Saito, M, Park, J, Murakami, H, Shibata, N, Ando, M, et al. Oral administration of Blautia wexlerae ameliorates obesity and type 2 diabetes via metabolic remodeling of the gut microbiota. Nat Commun. (2022) 13:4477. doi: 10.1038/s41467-022-32015-7
68. Ozato, N, Saito, S, Yamaguchi, T, Katashima, M, Tokuda, I, Sawada, K, et al. Blautia genus associated with visceral fat accumulation in adults 20–76 years of age. NPJ Biofilms Microbiomes. (2019) 5:28. doi: 10.1038/s41522-019-0101-x
69. Wells, CL, and Wilkins, TD. Clostridia: sporeforming anaerobic bacilli. In: S Baron, editor. Medical microbiology. Galveston (TX): University of Texas Medical Branch at Galveston (1996)
70. Low, L, Suleiman, K, Shamdas, M, Bassilious, K, Poonit, N, Rossiter, AE, et al. Gut Dysbiosis in ocular mucous membrane pemphigoid. Front Cell Infect Microbiol. (2022) 12:780354. doi: 10.3389/fcimb.2022.780354
71. Wang, C, Ma, C, Fu, K, Gong, LH, Zhang, YF, Zhou, HL, et al. Phillygenin attenuates carbon tetrachloride-induced liver fibrosis via modulating inflammation and gut microbiota. Front Pharmacol. (2021) 12:756924. doi: 10.3389/fphar.2021.756924
72. Yang, WY, Lee, Y, Lu, H, Chou, CH, and Wang, C. Analysis of gut microbiota and the effect of lauric acid against necrotic enteritis in Clostridium perfringens and Eimeria side-by-side challenge model. PLoS One. (2019) 14:e0205784. doi: 10.1371/journal.pone.0205784
73. Ye, J, Ma, J, Rozi, P, Kong, L, Zhou, J, Luo, Y, et al. The polysaccharides from seeds of Glycyrrhiza uralensis ameliorate metabolic disorders and restructure gut microbiota in type 2 diabetic mice. Int J Biol Macromol. (2024) 264:130622. doi: 10.1016/j.ijbiomac.2024.130622
74. Micioni Di Bonaventura, MV, Coman, MM, Tomassoni, D, Micioni Di Bonaventura, E, Botticelli, L, Gabrielli, MG, et al. Supplementation with Lactiplantibacillus plantarum IMC 510 modifies microbiota composition and prevents body weight gain induced by cafeteria diet in rats. Int J Mol Sci. (2021) 22. doi: 10.3390/ijms222011171
75. Vacca, M, Celano, G, Calabrese, FM, Portincasa, P, Gobbetti, M, and De Angelis, M. The controversial role of human gut Lachnospiraceae. Microorganisms. (2020) 8. doi: 10.3390/microorganisms8040573
76. Baffert, C, Kpebe, A, Avilan, L, and Brugna, M. Hydrogenases and H(2) metabolism in sulfate-reducing bacteria of the Desulfovibrio genus. Adv Microb Physiol. (2019) 74:143–89. doi: 10.1016/bs.ampbs.2019.03.001
77. Ijssennagger, N, van der Meer, R, and van Mil, SWC. Sulfide as a mucus barrier-breaker in inflammatory bowel disease? Trends Mol Med. (2016) 22:190–9. doi: 10.1016/j.molmed.2016.01.002
Keywords: Lactobacillus plantarum , Streptococcus mutans , dental caries, obesity, gut microbiota
Citation: Yang W, Jiang M, Chen B, Jiang K, Ma N, Li Y, Wang M, Bao M, Wang C and Yang X (2024) Study the effect of Lactobacillus plantarum ATCC 14917 for caries prevention and anti-obesity. Front. Nutr. 11:1511660. doi: 10.3389/fnut.2024.1511660
Edited by:
Marcella Reale, University of Studies G. d’Annunzio Chieti and Pescara, ItalyReviewed by:
Alberto Finamore, Council for Agricultural Research and Agricultural Economy Analysis | CREA, ItalyZipeng Jiang, Zhejiang University, China
Copyright © 2024 Yang, Jiang, Chen, Jiang, Ma, Li, Wang, Bao, Wang and Yang. This is an open-access article distributed under the terms of the Creative Commons Attribution License (CC BY). The use, distribution or reproduction in other forums is permitted, provided the original author(s) and the copyright owner(s) are credited and that the original publication in this journal is cited, in accordance with accepted academic practice. No use, distribution or reproduction is permitted which does not comply with these terms.
*Correspondence: Xiaopeng Yang, eWFuZ3hwQGp6bXUuZWR1LmNu; Chengyue Wang, d2FuZ2N5QGp6bXUuZWR1LmNu
†These authors have contributed equally to this work