- 1Graduate School of Medicine, Science and Technology, Shinshu University, Nagano, Japan
- 2Food Technology Department, Nagano Prefecture General Industrial Technology Center, Nagano, Japan
- 3Food and Feed Immunology Group, Laboratory of Animal Food Function, Graduate School of Agricultural Science, Tohoku University, Sendai, Japan
- 4Department of Gastroenterology and Hepatology, Fujita Health University, Toyoake, Japan
- 5Department of Medical Research on Prebiotics and Probiotics, Fujita Health University, Toyoake, Japan
- 6BIOSIS Lab. Co., Ltd., Aichi, Japan
- 7Institute for Aqua Regeneration, Shinshu University, Nagano, Japan
- 8Department of Biomolecular Innovation, Institute for Biomedical Sciences, Shinshu University, Nagano, Japan
Koji amazake, which is made from rice and rice koji (a product of Aspergillus oryzae), is a traditional Japanese beverage that has glucose as its main component. It also contains isomaltose, which has been reported to have various functionalities related to gut health. In the present study, we attempted to produce amazake with a higher concentration of isomaltose without using any additives by focusing on the saccharification step of rice koji production as a means of creating new value for amazake. Two types of rice koji that were obtained at different fermentation time points were used, and we changed the saccharification process from the usual one step of saccharification to two steps of saccharification using a different type of rice koji for each step. The amazake made by double saccharification (DSA) contained 20 times more isomaltose than the commercial amazake products. In an in vivo study, oral administration of the DSA modified the cecal microbiota in mice. Moreover, changes were seen in the abundances of several gut microorganisms, such as Anaerotignum lactatifermentans, Muribaculum intestinale, and Parabacteroides merdae. These findings indicate that our novel method may be useful for producing amazake with a high isomaltose content that may have health benefits in humans.
1 Introduction
Washoku, which means traditional Japanese cuisine, was registered in 2013 on the intangible cultural heritage list by the United Nations Educational, Scientific, and Cultural Organization. As the Japanese government has emphasized, the health benefits of washoku have been gaining much attention not only in Japan, but also around the world (1). Washoku often contains many and various fermented foods (2). Most Japanese fermented foods contain koji, which is a grain, such as rice, wheat, and/or soybeans, fermented with a non-pathogenic fungus, i.e., Aspergillus oryzae or Aspergillus luchuensis (3); these fungi produce enzymes for the saccharification of the starch present in the grains (4).
Amazake is a drink that is one of the simplest traditional Japanese fermented products prepared using koji. There are two types of amazake that differ depending on the raw material used: koji amazake and sake-cake amazake. Koji amazake is made from rice and rice koji (A. oryzae products), and its manufacturing process consists of two main steps: preparation of the rice koji and saccharification. To make rice koji, brown rice is polished into white rice, gently washed in water, steeped, and steamed. The spores of A. oryzae are then inoculated onto the steamed rice. Next, the rice koji is mixed with water, and placed in a tank set at 50°C to 60°C for the saccharification (5).
The main component of koji amazake is glucose, which is produced from rice by breaking down rice starch; this is performed by an amylase secreted by A. oryzae. As a result, koji amazake mainly contains glucose rather than sucrose and fructose, which are found in many other beverages. Furthermore, various oligosaccharides are produced by transglycosylation, such as maltose (Glc(α1-4)Glc) and isomaltose (Glc(α1-6)Glc) (5). In particular, isomaltose has been gaining much attention as an alternative to sucrose due to its potential health benefits, such as its low glycemic index and slow hydrolysis (6–11), and probiotic potential (12, 13).
Accordingly, we believe that amazake with a high concentration of isomaltose may have more health benefits for humans and increase the value of amazake on the market. In a previous investigation to improve the quality of koji amazake, Oguro et al. (14) studied the relationships between temperature, saccharification, and oligosaccharide production, including isomaltose; they reported that modifications to the saccharification conditions resulted in only small changes to the concentration of isomaltose.
In this study, we focused on the rice koji-making process to find a way to increase the value of amazake, and established a method that significantly increased the isomaltose concentration in amazake. In the production of amazake, it is common to use one type of rice koji and to perform one saccharification step. However, in this study, we used two types of rice koji that were obtained at different fermentation time points, and saccharification was carried out in two steps using the two types of obtained rice koji, one for each saccharification step. The isomaltose concentration in the amazake produced using double saccharification (double-saccharification amazake; DSA) was increased as a result, which is a novel finding. Furthermore, administration of the DSA to mice induced changes in the gut microbiota. Here, we introduce our new two-step saccharification method for producing DSA, and report on the potential of DSA to confer health benefits, which is expected to increase the value of amazake.
2 Materials and methods
2.1 Starter koji
The starter koji used in this study (koji No. 1 and No. 2) were purchased from Akita Konno Co., Ltd. (Akita, Japan). Koji No. 1 is often used for making bean koji, and koji No. 2 is specifically used for making rice koji for sake. The starter koji strains were stored at 4°C until used for the experiments.
2.2 Preparation of rice koji and saccharification for making amazake
To examine the effects of different fungal strains on saccharification and the fermentation time, we separately used two types of starter koji (koji No. 1 and No. 2) to make rice koji. For making rice koji, we used Akita Komachi rice that was produced in 2023. The rice was gently washed in water, steeped overnight, and steamed for 1 h at normal pressure. Subsequently, the steamed rice was cooled to approximately 35°C, mixed with the koji (0.35 g/kg), and fermented in a bath maintained at 32°C during fermentation (for 42 h). At 18 and 25 h of fermentation, the rice koji samples were mixed by hand to break up the clumps that had formed and as a method to help decrease the temperature to the appropriate temperature range. The temperature of the rice koji was controlled during the fermentation process as follows: the temperature was 30°C at the start, then it was gradually increased to 38°C by 18 h, at which point the sample was mixed, then kept at 33°C until 25 h, at which point the sample was mixed again, and kept at 30°C until 42 h. The rice koji was sampled at 18, 25, and 42 h with the samples prepared using starter koji No. 1 referred to as samples X-1, Y-1, and Z-1, respectively, and those prepared using starter koji No. 2 referred to as samples X-2, Y-2, and Z-2, respectively. These samples were stored at-30°C until used in the experiments. For the experiments, 50 g of the obtained X, Y, or Z was mixed with an equal amount of water and incubated at 50°C for 6 h for the saccharification to make amazake.
2.3 Saccharification
For saccharification with the addition of maltose, 10 g of maltose was dissolved in 40 g of water. Subsequently, 40 g of each sample was mixed with the maltose solution and incubated at 50°C for 6 h, then sampled for the analysis of the sugar levels.
The DSA was prepared using the rice koji samples X-1 and Z-2 as follows: 50 g of sample X-1 was mixed with 50 g of water and incubated at 50°C for 6 or 24 h; subsequently, the obtained saccharification sample (60 g) was mixed with sample Z-2 (40 g) and incubated at 50°C for 6 or 30 h.
2.4 Analysis of the sugar concentrations in amazake
In addition to the DSA prepared in this study, commercial amazake products were also examined. For the glucose analysis, the amazake samples were diluted two-fold with distilled water and centrifuged at 1,870 × g for 10 min at 4°C. Aliquots of the obtained supernatants were diluted and analyzed for glucose using a glucose analyzer GA05 (A&T Corporation, Kanagawa, Tokyo). The amount of maltose or isomaltose in each sample was analyzed by high-performance liquid chromatography (HPLC) using a WATERS1525 (Nihon Waters K.K., Tokyo, Japan). In brief, 2 g of sample and 2 mL of 50% ethanol were mixed and sonicated for 30 min. After extracting the saccharides from each sample, the supernatant that was obtained by centrifugation at 1,870 × g for 10 min at 4°C was filtered through a 0.45-μm filter to remove the impurities. Then, each sample was analyzed by HPLC with a Shodex Asahipak NH2P-50 4E column (4.6 mm I.D. × 150 mm) at 35°C to quantify the maltose or isomaltose. The eluent was 70% acetonitrile, and the flow rate was set at 0.8 mL/min. The sugars in the samples were detected with a Refractive Index Detector.
2.5 Determination of the nutrient composition of DSA
Determination of the nutrient composition of the DSA was outsourced to LSI Medience Corporation (Tokyo, Japan). One-hundred grams of DSA was prepared as described above, and samples were sent to LSI Medience Corporation.
2.6 Mice and oral administration of the DSA
C57BL/6 mice (7 weeks old, female) were purchased from Japan SLC (Shizuoka, Japan). The mice were housed under controlled temperature and light conditions with ad libitum access to a standard diet (MF; Oriental Yeast Co., Ltd., Tokyo, Japan) and sterile water. The mice were acclimatized for a week, then randomly allocated into two experimental groups (N = 6 each): a phosphate-buffered saline (PBS) control group and a DSA group. Each mouse was orally administered PBS or a homogenate of DSA (200 μL/mouse) for 14 consecutive days (Days 1 to 14); the homogenate of DSA was prepared by centrifugation of the DSA at 1,400 × g for 5 min. On day 14, the mice were euthanized, and the cecal contents were collected.
2.7 16S rRNA gene sequencing
DNA was extracted from the cecal content samples using a fecal collection kit, and the 16S V3–V4 region of the DNA was amplified according to the methods of a previous report (15). The DNA was sequenced using the Illumina MiSeq platform (Illumina, San Diego, CA, USA) and a MiSeq Reagent Kit v3 (Illumina). Quantitative Insights into Microbial Ecology version 2 (Qiime2) bacterial flora analysis software (16) was used for microbiota analysis. Qiime2view was used to analyze the diversity and changes in the intestinal flora at the family and species levels based on the resulting qzv files. The α-diversity was analyzed using the observed features (amplicon sequence variants; ASVs) and Shannon diversity. The β-diversity was analyzed using the Jaccard and Bray-Curtis dissimilarities. In addition, multiple comparison tests using the Tukey–Kramer method were performed to analyze the intestinal bacteria that were significantly different between the DSA and PBS groups at the species level.
2.8 Statistical analysis
Statistical analysis was performed using Prism software (version 7; GraphPad Software, San Diego, CA, USA). Outliers were identified using the ROUT method (Q = 2%) and omitted before further statistical analysis. Statistically significant differences were assessed by two-tailed ordinary one-way analysis of variance followed by Tukey’s multiple comparisons test when statistical significance (p < 0.05) was indicated. Results are presented as the mean ± standard error (SE).
3 Results
3.1 The effects of double saccharification on the concentration of isomaltose in amazake
Figure 1 shows a scheme of the manufacturing steps for DSA. The nutritional information on the DSA is shown in Table 1. The rice koji was sampled at three different time points, i.e., at 18 h (samples X), 25 h (samples Y), and 42 h (samples Z) (Figure 1A). The concentrations of glucose, maltose, and isomaltose in each sample were measured to examine the relationship between the fermentation time and the concentration of each saccharide. The concentration of glucose was the lowest in samples X (18-h time point) regardless of the type of koji used (koji No. 1 or No. 2; Figure 2A). The concentration of maltose was highest in samples X (Figure 2B). The concentration of isomaltose did not differ significantly between the samples, but it was slightly higher in sample X-1 than in the other samples (Figure 2C). Considering that isomaltose is mostly formed from maltose through the transglycosylation activity of α-glucosidase, a saccharification test was performed with the addition of maltose to examine which sample had the highest transglycosylation activity. The concentration of isomaltose increased when maltose was added, and was highest in samples Z from the longest time point, especially in sample Z-2 (Figure 2D). To confirm which sample had the highest maltose content and to examine how the concentration of maltose depended on the time of saccharification, sample X-1 was saccharified for 24 h. The concentration of maltose increased until 6 h, and remained stable thereafter. In contrast, the concentration of glucose continued to increase until 24 h (Figure 2E). A second saccharification test was conducted to examine how the time of the first saccharification influenced the concentration of isomaltose in amazake samples produced using sample X-1 saccharified for 6 or 24 h. In both amazake samples, the level of isomaltose was approximately 20% at 30 h after the start of the second fermentation (Figure 2F). When the concentration of isomaltose in the DSA was compared to those in commercial products, we found that the DSA contained significantly more (almost 20 times more) isomaltose than the commercial products (Figure 2G).
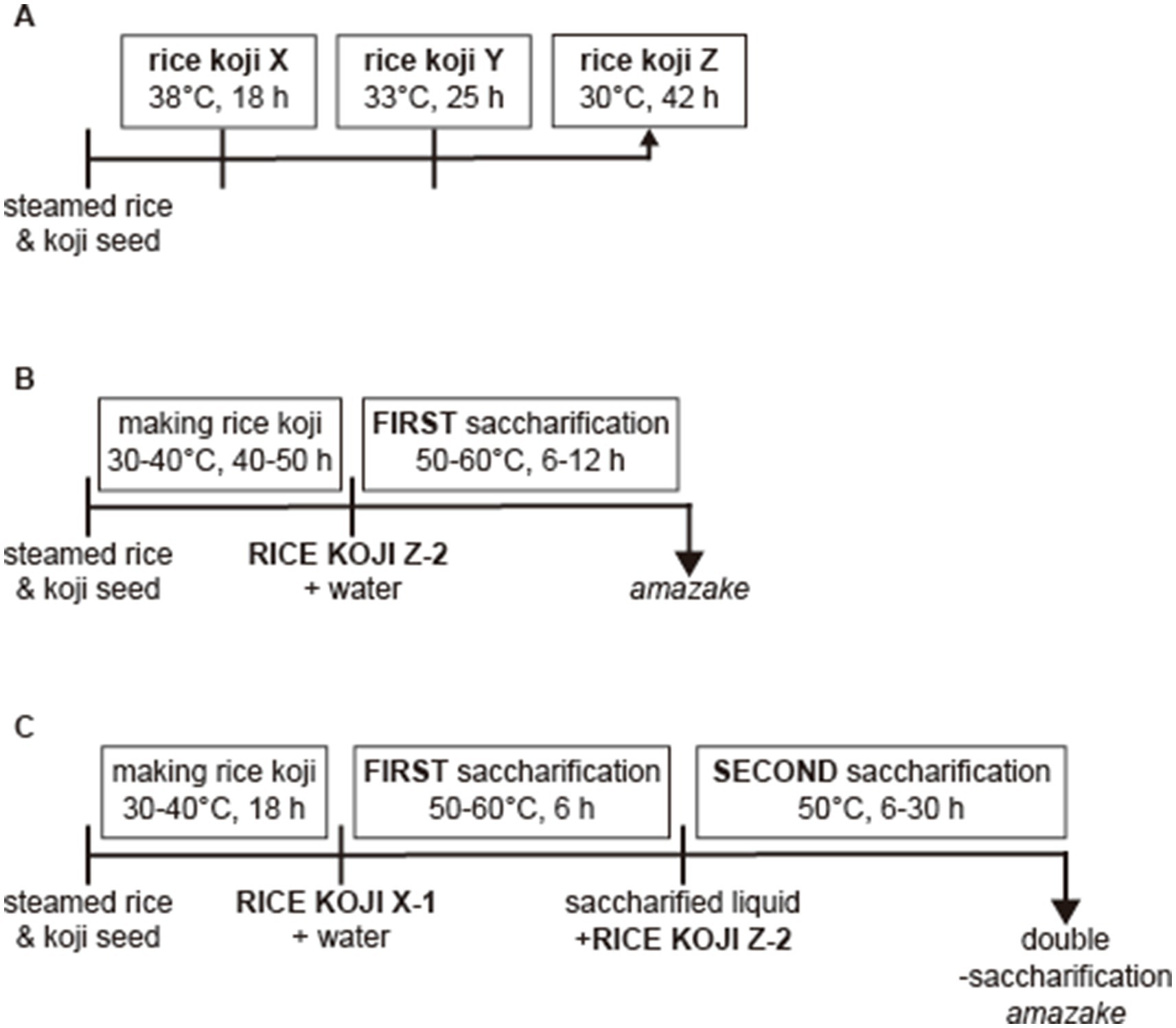
Figure 1. Scheme of the manufacturing of amazake. The schedule for rice koji sampling (A), the manufacturing steps for amazake (B), and for DSA (C).
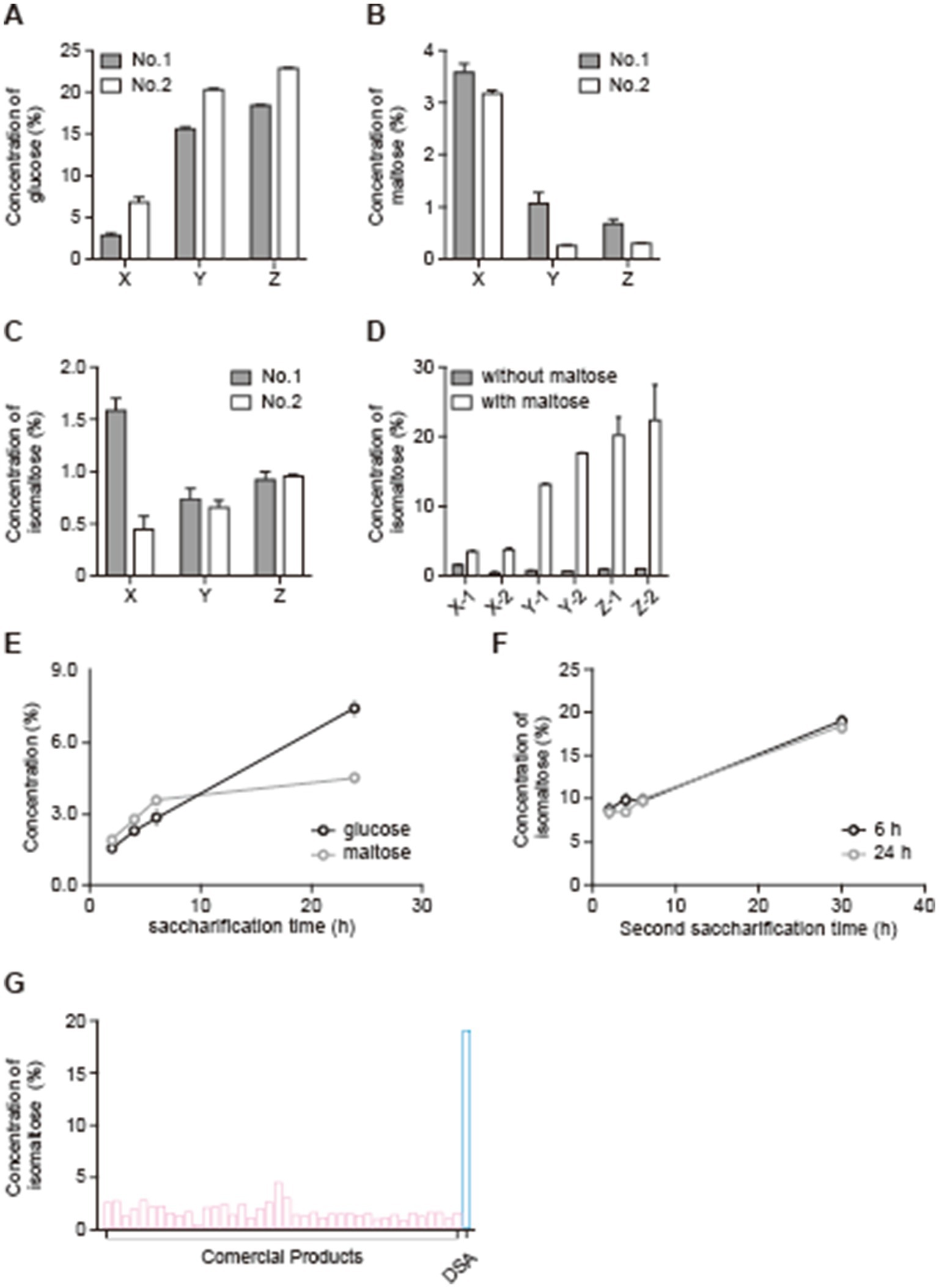
Figure 2. Variations in the concentration of each sugar due to the use of different starter koji and fermentation times, and increases in the isomaltose concentration in amazake due to double saccharification. Concentrations of glucose (A), maltose (B), and isomaltose (C) during koji production, concentrations of isomaltose during koji production with maltose addition (D), concentrations of glucose and maltose during the first fermentation (E), concentration of isomaltose during the second fermentation (F) using DSA, and comparison of isomaltose concentration in commercial amazake with that in DSA-produced amazake (G). Data are shown as the mean ± SE. *p < 0.05.
3.2 DSA altered the diversity of the cecal microbiota
After 2 weeks of oral administration of PBS or DSA in mice, the cecal contents were collected and analyzed by next-generation sequencing, and the α-diversity was examined. There was a significant difference in the observed features between the PBS and DSA groups (Figure 3A), while the Shannon diversity index showed no significant increase in the α-diversity in the two groups (Figure 3B). Figures 3C,D show the Bray-Curtis and Jaccard β-diversity analysis results for the mouse cecal contents. The principal coordinate analysis results showed that the oral administration of DSA altered the gut microbiota (Figures 3C,D). The results of the taxonomic analysis are presented in Figure 4A as a bar plot (via QIIME2) showing the bacterial composition (at the family level) of the cecal content in each animal of each group. The significance of differences was investigated. The differences in the gut microbiota due to the administration of DSA were examined by linear discriminant analysis (LDA) at the species level from the LDA effect size (LEfSe) analysis at the species level. Three bacteria were extracted (Figure 4B), and a comparison of the relative abundances showed that the abundance of Anaerotignum lactatifermentans was decreased, and those of Muribaculum intestinale, and Parabacteroides merdae were increased in the DSA group when compared to the PBS group (Figures 4C,D).
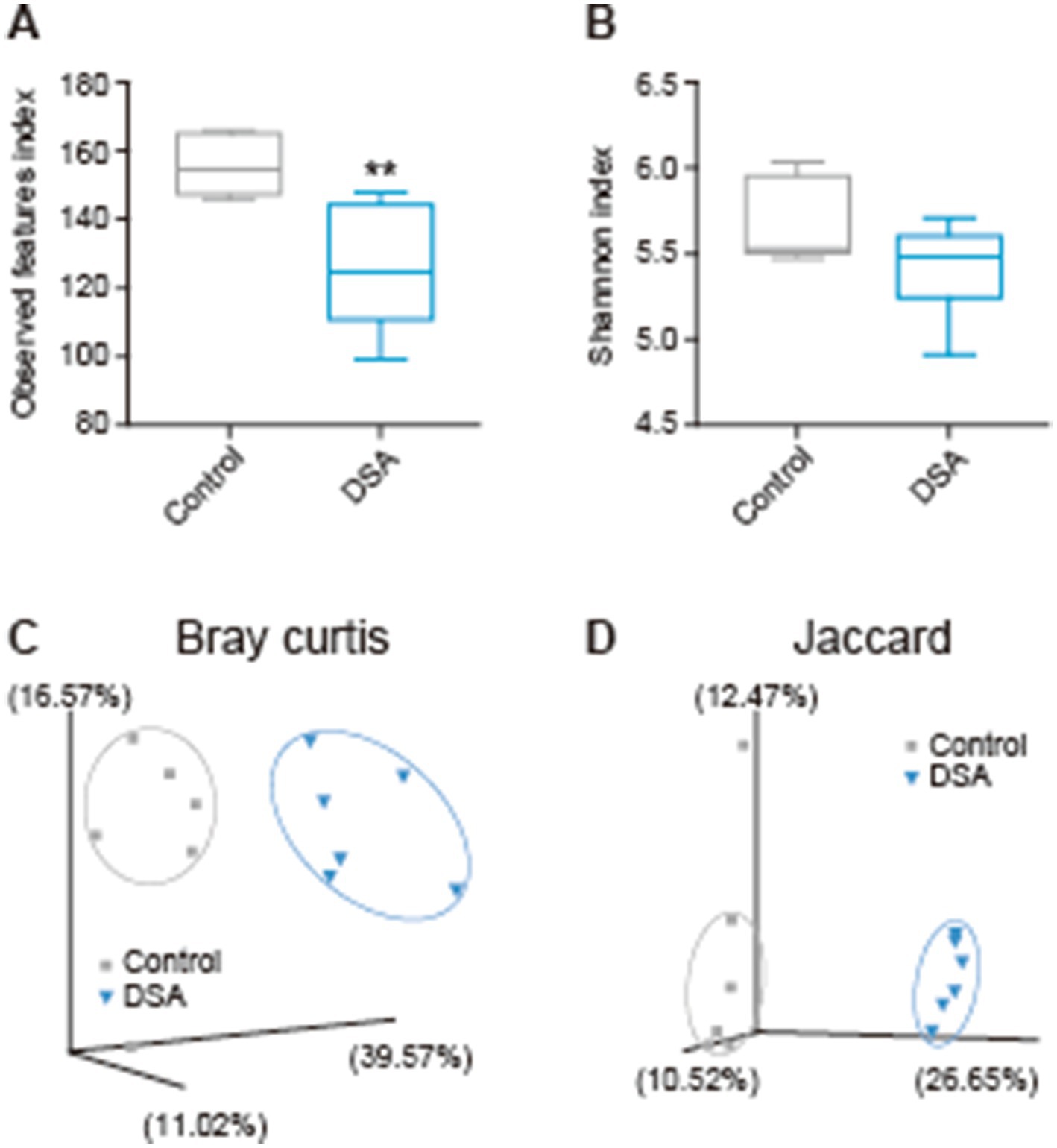
Figure 3. Variations in the diversity of the intestinal microbiota in mice administered DSA. Observed features index (A), Shannon index (B), and β-diversity analyzed using Bray-Curtis (C) and Jaccard (D) dissimilarities. N = 6. Data are shown as the mean ± SE. **p < 0.01.
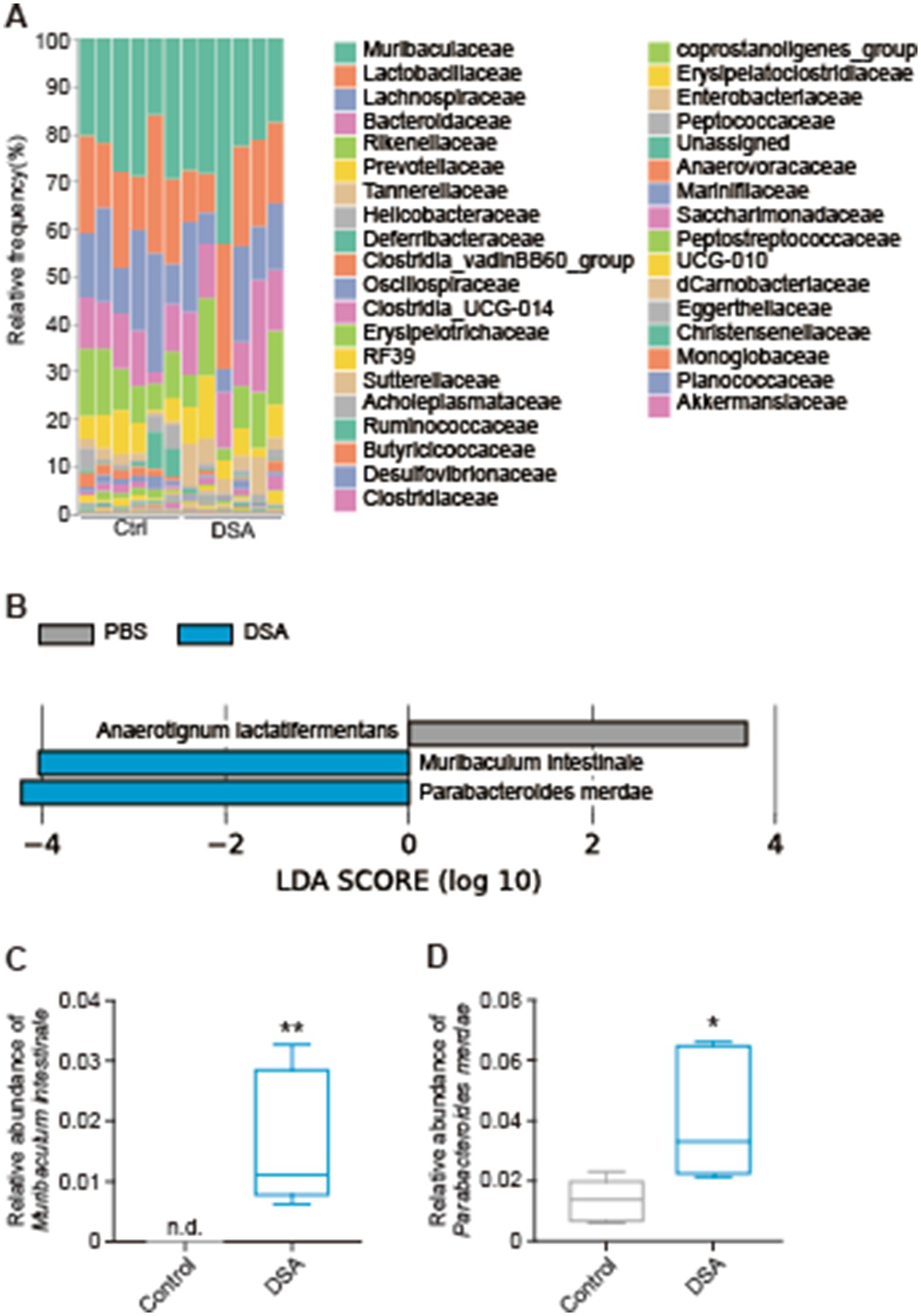
Figure 4. Composition of the gut microbiota and the relative abundances of several bacteria affected by DSA administration. A bar plot (A), bacteria with significant differences in the ASVs at the species level (B), the relative abundances of M. intestinale (C) and P. merdae (D). N = 6. Data are shown as the mean ± SE. *p < 0.05, **p < 0.01.
4 Discussion
It is already known that the concentration of isomaltose in amazake is not affected by the temperature or the time of saccharification (14). Therefore, in this study, we focused on the rice koji-making process, which is an important step in manufacturing amazake, for increasing the concentration of isomaltose.
In the present study, we used a common rice koji production method: rice koji production was completed in 42 h with mixing to break up clumps at 18 h and 25 h, and the temperature conditions were strictly controlled. However, numerous other methods also exist, and they differ between sake or miso breweries and stores. In the present study, the rice koji obtained at 18, 25, and 42 h was used as rice koji samples X, Y, and Z, respectively, and the concentrations of glucose, maltose, and isomaltose were measured after 6 h of saccharification (Figures 2A–C). When we compared all samples, the glucose concentration was lowest in the sample from the earliest time point in the rice koji making process, which were samples X. However, the concentration of maltose was the highest in samples X. Glucose is the main component in amazake, and it is derived from rice starch. Rice starch is first broken down by the α-amylase and glucoamylase secreted by koji mold, resulting in maltose and glucose (5). The produced maltose is also broken down to glucose by the α-glucosidase secreted by koji mold. Therefore, the concentrations of these saccharides were affected by the degree of mycelial growth and the amounts of enzymes present. Indeed, samples X showed the lowest glucose level and the highest maltose level, likely because the activity of maltase, which degrades maltose, was low. In fact, the activity of α-glucosidase and maltase has been shown to increase with the fermentation time (17, 18); thus, it is likely that the activity of these enzymes was lower in samples X since the fermentation time was shorter than for samples Y and Z. However, we did not examine the enzyme activities in the present study, and they will need to be analyzed in the future to confirm this. In addition, the concentration of isomaltose was similar in all samples, although it was slightly higher in sample X-1. It is known that isomaltose is commonly synthesized from maltose and maltooligosaccharides through hydrolytic and transfer reactions by α-glucosidase with transglycosylation activity, especially when maltose is present at a high concentration (19). In other words, a low concentration of maltose in samples may cause the maltose to become glucose through a hydrolytic reaction.
According to these results and the fact that a high concentration of maltose is needed to produce isomaltose, we conducted a saccharification test with the addition of maltose. We found that the addition of maltose increased the concentration of isomaltose (Figure 2D). Moreover, it has been reported that the production of enzymes in koji increases with the fermentation time (17), which might explain why samples Z showed the highest concentration of isomaltose: samples Z likely contained the most α-glucosidase and possibly the highest transglycosylation activity.
In the previous investigation, it was revealed that a high concentration of maltose and the transglycosylation activity of α-glucosidase were necessary for increasing the content of isomaltose in amazake. Thus, we considered that it may be possible to increase the isomaltose content of amazake without the use of additives if two saccharification steps were conducted using sample X-1 for the first step and sample Z-2 for the second step (Figures 1, 2). Regardless of the first saccharification time (6 or 24 h), the effect of the second saccharification using sample Z-2 provided a significantly higher concentration of isomaltose when compared to that of the commercial amazake products. The reason no difference was seen between the 6 and 24 h time points in the first saccharification is likely because the concentration of maltose was similar in the two samples (Figures 2E,F).
In conclusion, we succeeded in finding a new method of amazake production for increasing the concentration of isomaltose without the use of additives. Our amazake contained approximately 20 times more isomaltose than the commercial products (Figure 2G). However, the concentration of essential enzymes, such as α-amylase, glucoamylase, and α-glucosidase, as well as their transglycosylation activities, were not checked when the two saccharification steps were performed. Also, in this study, sample X-1 was used for the first saccharification and sample Z-2 was used for the second saccharification, but the details of how the two kinds of koji samples interact remain unclear. Therefore, further experiments are needed in the future.
Since isomaltose is widely expected to be a prebiotic (30), we performed an in vivo experiment to test the effects of DSA administration in mice. No differences were seen in the Shannon index between the two groups. In addition, the β-diversity showed significantly different clusters. Although the DSA had little influence on the species diversity within a group, it modified the structure of the bacterial clusters in the DSA group when compared to the PBS group (Figure 3). Moreover, the abundances of three types of bacteria were found to have changed significantly due to the administration of DSA, namely, A. lactatifermentans, M. intestinale, and P. merdae (Figure 4). M. intestinale is highly associated with dietary habits, and there are some studies showing that the consumption of a western diet and cafeteria foods may decrease its abundance in the gut (20, 21). M. intestinale is a glycan-degrading and butyrate-producing bacterium, and it has been reported that the abundance of M. intestinale increased or recovered with improvements of obesity-and intestinal inflammatory disease-related factors that were affected by high-fat diets and dextran sulfate sodium administration (22, 23). Thus, the consumption of DSA may be useful and effective for promoting a stable and healthy intestinal environment. The abundance of M. intestinale may have increased in the gut of mice that were administered DSA due to the fact that it is a glycan-degrading bacterium and DSA is considered to have more sugar chains because it contains more isomaltose than the commercial products. On the other hand, P. merdae has been isolated from human feces, and has been reported to be associated with several diseases, such as hypertension, polycystic ovary syndrome, cancer, and obesity (24). For example, while P. merdae was found in increased abundance in people with hypertension (25) and polycystic ovary syndrome (26), an increase in the abundance of P. merdae in the gut has been reported to contribute to the treatment of obesity-related cardiovascular disease. This effect was due to the ability of P. merdae to catabolism branched-chain amino acids, mediated by the porA gene (27). In other words, DSA intake may contribute to the prevention of lifestyle-related obesity and related metabolic diseases by increasing the abundances of M. intestinale and P. merdae in the gut. Interestingly, not only has P. merdae been shown to be more abundant in the gut of those in the 90-to 99-year-old group in East China (28), but Muribaculaceae species, including M. intestinale, have also been reported to be associated with longevity (29). Thus, while we believe that habitual DSA consumption would confer continual health benefits, a more detailed understanding of the function of the bacterial strain altered by DSA administration requires further investigation.
In the present study, we introduced a novel method of producing koji amazake that contains a high concentration of isomaltose. We focused on the rice koji-making process instead of the saccharification step, and prepared two types of rice koji that were obtained at different time points and were subsequently used in two saccharification steps; this method resulted in an increased concentration of isomaltose. Furthermore, the administration of DSA increased the abundances of the obesity-and longevity-related organisms P. merdae and M. intestinale, which can degrade glycan. These results suggest that DSA consumption may confer health benefits and help maintain a healthy intestinal environment.
Data availability statement
The datasets presented in this study can be found in online repositories. The names of the repository/repositories and accession number(s) can be found in the article/supplementary material.
Ethics statement
The animal study was approved by the Committee for Animal Experiments of Shinshu University. The study was conducted in accordance with the local legislation and institutional requirements.
Author contributions
AM: Data curation, Investigation, Validation, Visualization, Writing – original draft, Writing – review & editing. AS: Data curation, Investigation, Validation, Writing – original draft. FN: Data curation, Investigation, Writing – original draft. TF: Writing – review & editing. TT: Writing – review & editing. JT: Data curation, Investigation, Writing – review & editing. TS: Funding acquisition, Methodology, Project administration, Supervision, Visualization, Writing – original draft, Writing – review & editing.
Funding
The author(s) declare that financial support was received for the research, authorship, and/or publication of this article. This work was supported by the Education and Research Support Fund from Shinshu University (2019-2023) to TS.
Acknowledgments
We thank Ms. Chikako Miyazaki of the Institute for Biomedical Sciences, Shinshu University, for technical support. We also thank the Research Center for Support to Advanced Science and Shinshu University for the use of their facilities.
Conflict of interest
Tadashi Fujii and Takumi Tochio are members of BIOSIS Lab. Co., Ltd.
The remaining authors declare that the research was conducted in the absence of any commercial or financial relationships that could be construed as a potential conflict of interest.
Publisher’s note
All claims expressed in this article are solely those of the authors and do not necessarily represent those of their affiliated organizations, or those of the publisher, the editors and the reviewers. Any product that may be evaluated in this article, or claim that may be made by its manufacturer, is not guaranteed or endorsed by the publisher.
References
1. Yatsuya, H, and Tsugane, S. What constitutes healthiness of Washoku or Japanese diet? Eur J Clin Nutr. (2021) 75:863–4. doi: 10.1038/s41430-021-00872-y
2. Hamajima, H, Matsunaga, H, Fujukawa, A, Sato, T, Mitsutake, S, Yanagita, T, et al. Japanese traditional dietary fungus koji aspergillus oryzae functions as a prebiotic for Blautia coccoides through glycosylceramide: Japanese dietary fungus koji is a new prebiotic. Springerplus. (2016) 5:1321. doi: 10.1186/s40064-016-2950-6
3. Machida, M, Asai, K, Sano, M, Tanaka, T, Kumagai, T, Terai, G, et al. Genome sequencing and analysis of aspergillus oryzae. Nature. (2005) 438:1157–61. doi: 10.1038/nature04300
4. Kitagaki, H, and Kitamoto, K. Breeding research on sake yeasts in Japan: history, recent technological advances, and future perspectives. Annu Rev Food Sci Technol. (2013) 4:215–35. doi: 10.1146/annurev-food-030212-182545
5. Kurahashi, A. Ingredients, functionality, and safety of the Japanese traditional sweet drink Amazake. J Fungi. (2021) 7:469. doi: 10.3390/jof7060469
6. Holub, I, Gostner, A, Theis, S, Nosek, L, Kudlich, T, Melcher, R, et al. Novel findings on the metabolic effects of the low glycaemic carbohydrate isomaltulose (Palatinose™). Brit J Nutr. (2010) 103:1730–7. doi: 10.1017/S0007114509993874
7. Hwang, D, Park, HR, Lee, SJ, Kim, HW, Kim, JH, and Shin, KS. Oral administration of palatinose vs sucrose improves hyperglycemia in normal C57BL/6J mice. Nutr Res. (2018) 59:44–52. doi: 10.1016/j.nutres.2018.06.010
8. König, D, Theis, S, Kozianowski, G, and Berg, A. Postprandial substrate use in overweight subjects with the metabolic syndrome after isomaltulose (Palatinose™) ingestion. Nutrition. (2012) 28:651–6. doi: 10.1016/j.nut.2011.09.019
9. Konig, D, Zdzieblik, D, Holz, A, Theis, S, and Gollhofer, A. Substrate utilization and cycling performance following Palatinose ingestion: a randomized, double-blind Controlled. Trial Nutr. (2016) 8:390. doi: 10.3390/nu8070390
10. Mateo-Gallego, R, Perez-Calahorra, S, Lamiquiz-Moneo, I, Marco-Benedi, V, Bea, AM, Fumanal, AJ, et al. Effect of an alcohol-free beer enriched with isomaltulose and a resistant dextrin on insulin resistance in diabetic patients with overweight or obesity. Clin Nutr. (2020) 39:475–83. doi: 10.1016/j.clnu.2019.02.025
11. Sokolowska, E, Sadowska, A, Sawicka, D, Kotulska-Bablinska, I, and Car, H. A head-to-head comparison review of biological and toxicological studies of isomaltulose, d-tagatose, and trehalose on glycemic control. Crit Rev Food Sci. (2022) 62:5679–704. doi: 10.1080/10408398.2021.1895057
12. Su, HH, Xu, RY, Yang, ZD, Guo, YS, Gao, JY, Mo, LZ, et al. Green synthesis of isomaltulose from cane molasses by an immobilized recombinant Escherichia coli strain and its prebiotic activity. LWT Food Sci Technol. (2021) 143:111054. doi: 10.1016/j.lwt.2021.111054
13. Yang, ZD, Guo, YS, Huang, JS, Gao, YF, Peng, F, Xu, RY, et al. Isomaltulose exhibits prebiotic activity, and modulates gut microbiota, the production of short chain fatty acids, and secondary bile acids in rats. Molecules. (2021) 26:464. doi: 10.3390/molecules26092464
14. Oguro, Y, Nakamura, A, and Kurahashi, A. Effect of temperature on saccharification and oligosaccharide production efficiency in koji amazake. J Biosci Bioeng. (2019) 127:570–4. doi: 10.1016/j.jbiosc.2018.10.007
15. Takahashi, H, Fujii, T, Yamakawa, S, Yamada, C, Fujiki, K, Kondo, N, et al. Combined oral intake of short and long fructans alters the gut microbiota in food allergy model mice and contributes to food allergy prevention. BMC Microbiol. (2023) 23:266. doi: 10.1186/s12866-023-03021-6
16. Bolyen, E, Rideout, JR, Dillon, MR, Bokulich, NA, Abnet, CC, Al-Ghalith, GA, et al. Author correction: reproducible, interactive, scalable and extensible microbiome data science using QIIME 2. Nat Biotechnol. (2019) 37:1091. doi: 10.1038/s41587-019-0252-6
17. Lee, H, Lee, S, Kyung, S, Ryu, J, Kang, S, Park, M, et al. Metabolite profiling and anti-aging activity of Rice Koji fermented with aspergillus oryzae and aspergillus cristatus: a comparative study. Meta. (2021) 11:524. doi: 10.3390/metabo11080524
18. Sun, X, Zhang, J, Fan, ZH, Xiao, P, Li, F, Liu, HQ, et al. MAL62 overexpression enhances uridine diphosphoglucose-dependent trehalose synthesis and glycerol metabolism for cryoprotection of baker's yeast in lean dough. Microb Cell Factories. (2020) 19:196. doi: 10.1186/s12934-020-01454-6
19. Tiangpook, S, Nhim, S, Prangthip, P, Pason, P, Tachaapaikoon, C, Ratanakhanokchai, K, et al. Production of a series of long-chain Isomaltooligosaccharides from maltose by Bacillus subtilis AP-1 and associated prebiotic properties. Food Secur. (2023) 12:1499. doi: 10.3390/foods12071499
20. Leigh, SJ, Kaakoush, NO, Bertoldo, MJ, Westbrook, RF, and Morris, MJ. Intermittent cafeteria diet identifies fecal microbiome changes as a predictor of spatial recognition memory impairment in female rats. Transl Psychiatry. (2020) 10:734. doi: 10.1038/s41398-020-0734-9
21. McNamara, MP, Singleton, JM, Cadney, MD, Ruegger, PM, Borneman, J, and Garland, T. Early-life effects of juvenile Western diet and exercise on adult gut microbiome composition in mice. J Exp Biol. (2021) 224:239699. doi: 10.1242/jeb.239699
22. Lee, SH, Seo, D, Lee, KH, Park, SJ, Park, S, Kim, H, et al. Biometabolites of Citrus unshiu Peel enhance intestinal permeability and Alter gut commensal Bacteria. Nutrients. (2023) 15:319. doi: 10.3390/nu15020319
23. Medina-Larqué, AS, Rodríguez-Daza, MC, Roquim, M, Dudonné, S, Pilon, G, Levy, É, et al. Cranberry polyphenols and agave agavins impact gut immune response and microbiota composition while improving gut barrier function, inflammation, and glucose metabolism in mice fed an obesogenic diet. Front Immunol. (2022) 13:871080. doi: 10.3389/fimmu.2022.871080
24. Cui, Y, Zhang, L, Wang, X, Yi, Y, Shan, Y, Liu, B, et al. Roles of intestinal Parabacteroides in human health and diseases. FEMS Microbiol Lett. (2022) 369:fnac072. doi: 10.1093/femsle/fnac072
25. Yan, Q, Gu, Y, Li, X, Yang, W, Jia, L, Chen, C, et al. Alterations of the gut microbiome in hypertension. Front Cell Infect Microbiol. (2017) 7:381. doi: 10.3389/fcimb.2017.00381
26. Chu, W, Han, Q, Xu, J, Wang, J, Sun, Y, Li, W, et al. Metagenomic analysis identified microbiome alterations and pathological association between intestinal microbiota and polycystic ovary syndrome. Fertil Steril. (2020) 113:e1284:1286–1298.e4. doi: 10.1016/j.fertnstert.2020.01.027
27. Qiao, S, Liu, C, Sun, L, Wang, T, Dai, H, Wang, K, et al. Publisher correction: gut Parabacteroides merdae protects against cardiovascular damage by enhancing branched-chain amino acid catabolism. Nat Metab. (2023) 5:184. doi: 10.1038/s42255-023-00740-y
28. Wang, N, Li, R, Lin, H, Fu, C, Wang, X, Zhang, Y, et al. Enriched taxa were found among the gut microbiota of centenarians in East China. PLoS One. (2019) 14:e0222763. doi: 10.1371/journal.pone.0222763
29. Sibai, M, Altuntas, E, Yildirim, B, Ozturk, G, Yildirim, S, and Demircan, T. Microbiome and longevity: high abundance of longevity-linked Muribaculaceae in the gut of the long-living rodent Spalax leucodon. OMICS. (2020) 24:592–601. doi: 10.1089/omi.2020.0116
Keywords: koji amazake, Duncaniella, double saccharification, gut microbiota, mouse, Isomaltose, Muribaculum
Citation: Murakami A, Saito A, Namai F, Fujii T, Tochio T, Toida J and Shimosato T (2024) Koji amazake produced by double saccharification contains more isomaltose and modifies the gut microbiota in mice. Front. Nutr. 11:1489912. doi: 10.3389/fnut.2024.1489912
Edited by:
John Gieng, San Jose State University, United StatesReviewed by:
Richard Ansah Herman, Jiangsu University of Science and Technology, ChinaMelliana Christina Jonathan, Independent Researcher, Jakarta, Indonesia
Copyright © 2024 Murakami, Saito, Namai, Fujii, Tochio, Toida and Shimosato. This is an open-access article distributed under the terms of the Creative Commons Attribution License (CC BY). The use, distribution or reproduction in other forums is permitted, provided the original author(s) and the copyright owner(s) are credited and that the original publication in this journal is cited, in accordance with accepted academic practice. No use, distribution or reproduction is permitted which does not comply with these terms.
*Correspondence: Takeshi Shimosato, c2hpbW90QHNoaW5zaHUtdS5hYy5qcA==
†These authors have contributed equally to this work