- 1Yantai Key Laboratory of Special Medical Food, School of Food and Bioengineering, Yantai Institute of Technology, Yantai, Shandong, China
- 2College of Pharmacy, Binzhou Medical College, Yantai, Shandong, China
- 3Department of Medical Records Management, Yantai Affiliated Hospital of Binzhou Medical University, Yantai, Shandong, China
Polyphenols, as subordinate metabolites of plants, have demonstrated significant antibacterial, anti-inflammatory, and antioxidant action in scientific learn. These compounds exert their effects through various mechanisms, containing interference with microbial cell structures, rule of host immune responses, and neutralization of free radicals. This multifaceted activity positions polyphenols as promising candidates for maintaining human health and treating related diseases. Notably, in the context of escalating antibiotic resistance, the antibacterial properties of polyphenols offer innovative avenues for the development of new therapeutic agents. Additionally, their anti-inflammatory and antioxidant effects hold substantial potential for treating inflammatory diseases and mitigating the aging process. This review aims to summarize the latest findings on the biological activities of polyphenols, highlighting their mechanisms of action and potential applications in health and disease management. Furthermore, optimizing polyphenol extraction methods aligns with the goals of sustainable and green processing, reducing environmental impact while enhancing food safety and extending shelf life. Employing advanced analytical techniques, such as spectroscopy and chromatography, can ensure the accurate evaluation of polyphenol content and efficacy. These efforts collectively contribute to the ongoing improvement of food processing practices and product quality, promoting a healthier and more sustainable future in the food industry.
1 Introduction
Polyphenols, which are bioactive compounds synthesized by plants, can be found in many plant structures, from fruits and flowers to seeds, leaves, and woody sections. Polyphenols are highly diverse in their structure and function, with over 8,000 identified phenolic structures ranging from simple phenolic acids to complex, highly polymerized substances such as tannins (1). Polyphenols are characterized by their distinctive structural feature of one or more aromatic rings containing hydroxyl groups and their derivatives. Polyphenols can be classified into several main classes based on the strength of their phenolic ring structure, including phenolic acids, flavonoids, stilbenes, phenolic alcohols, and lignans (2).
The health benefits of polyphenols have garnered significant attention, particularly their antibacterial, anti-inflammatory, and antioxidant properties. These compounds are increasingly linked to clean label products, which appeal to consumers seeking healthier food options (3). The growing consumer preference for natural food additives over synthetic ones, driven by concerns about the potential carcinogenicity of synthetic compounds, has encouraged the food industry to explore natural alternatives (4). Consequently, research on polyphenols is on the rise due to the abundance of plant species in nature, which results in a vast quantity and widespread distribution of these compounds (2, 5). As a result, it is impractical to exhaustively cover every type of plant polyphenol. Despite significant progress in understanding polyphenols, challenges persist in optimizing their extraction and elucidating their pharmacological activities, owing to their immense diversity.
The synthesis, accumulation, and stability of polyphenols in plants are governed by a complex interplay of internal and external factors (Table 1A), these factors significantly impact the polyphenol content, highlighting the need for optimized cultivation, harvesting, and processing methods. Despite advancements, challenges in standardizing polyphenol extraction and maintaining stability persist due to their diverse nature. Addressing these challenges is crucial for improving polyphenols’ health benefits, particularly in replacing synthetic antioxidants in food products, necessitating further research into genetic mechanisms and innovative agricultural technologies. A holistic approach that integrates genetic, environmental, and agricultural factors is essential for maximizing the benefits of polyphenols. This will not only improve their application in the food industry but also enhance their contribution to human health. The objective of this review is to enhance the understanding of polyphenols and illustrate advancements in related disciplines. This article aims to provide an overview of the antibacterial, anti-inflammatory, and antioxidant properties of polyphenols, as well as the factors influencing their content and stability. By consolidating recent research findings, this review seeks to offer valuable insights for future studies and practical applications in health science and food processing technology.
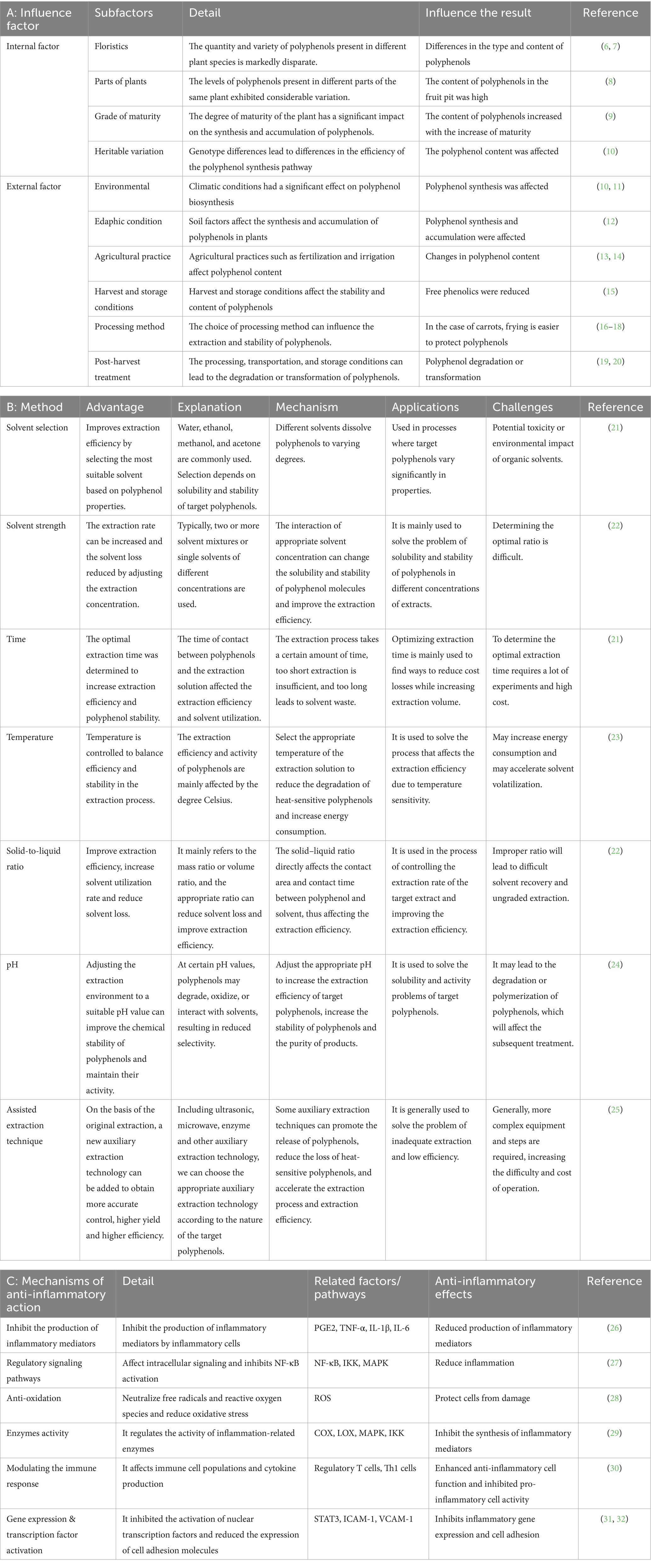
Table 1. Comprehensive insights into polyphenol content and stability in plants, extraction optimization, and anti-inflammatory mechanisms: (A) factors influencing polyphenol content and stability in plants; (B) optimization methods for polyphenol extraction: advantages, mechanisms, applications, and challenges; (C) mechanisms of anti-inflammatory action of polyphenols: pathways, factors, and effects.
2 Extraction process and determination method
2.1 The extraction technology of polyphenols
The extraction technology of polyphenols can be broadly categorized into traditional and modern techniques (Table 1B). Traditional methods, such as impregnation, percolation, and Soxhlet extraction, are commonly employed in phytochemical analysis due to their straightforward operational characteristics. However, these methods often involve lengthy processing times, high costs, and the use of environmentally unfriendly solvents, which are significant drawbacks (33).
In contrast, modern extraction technologies, including ultrasound-assisted extraction, microwave-assisted extraction, and supercritical fluid extraction, provide notable enhancements in efficiency compared to traditional methods. These advanced techniques minimize both the extraction time and solvent usage, making the process more sustainable and cost-effective (34). These modern methods are crucial for enhancing productivity, improving the quality and quantity of the output, and reducing costs and environmental impact.
Efficiently optimizing the polyphenol extraction process is crucial for enhancing productivity, improving the quality and quantity of the output, and reducing costs and environmental impact. Key aspects to consider during optimization include the specific types of polyphenols, raw material characteristics, extraction costs, the intended application of the final product, and the overall ecological consequences (35). Conducting laboratory-scale optimization followed by validation for scaled production is crucial to determine the most suitable extraction methods for specific applications. By thoroughly evaluating these factors (Table 1C), researchers can significantly improve the effectiveness of polyphenol extraction, ensuring the process is scientifically rigorous and practically viable. This approach allows for the development of optimized extraction protocols that maximize polyphenol yield and quality while being cost-effective and environmentally sustainable.
3 Biological activity
3.1 Antibacterial activity
Polyphenols exhibit various antibacterial mechanisms (Figure 1A), closely tied to their structure, chemical composition, and lipophilicity (36). These compounds can damage bacterial cell membranes, impairing their structure and function (37). For instance, polyphenols can interact with bacterial cell membranes, leading to structural damage and leakage of cellular contents, which is facilitated by the hydroxyl groups in their structure that form hydrogen bonds with the membrane (38). Studies have shown that this interaction is proportional to the number of hydrogen bond donors, and the presence of alkyl groups in the aromatic ring enhances the antibacterial effect by producing phenoxy free radicals (39, 40). Polyphenols also disrupt bacterial intercellular communication systems, known as quorum sensing (QS), which regulate phenotypic traits in a density-dependent manner. Rose tea extract, for instance, significantly inhibits QS and reduces the motility of Escherichia coli and Pseudomonas aeruginosa (41). Tannic acid, ellagic acid, and epigallocatechin gallate (EGCG) specifically inhibit N-acyl-homoserine lactones (AHLs)-mediated QS in Gram-negative bacteria, blocking bacterial signaling and coordinating activities critical for infection and survival (42).
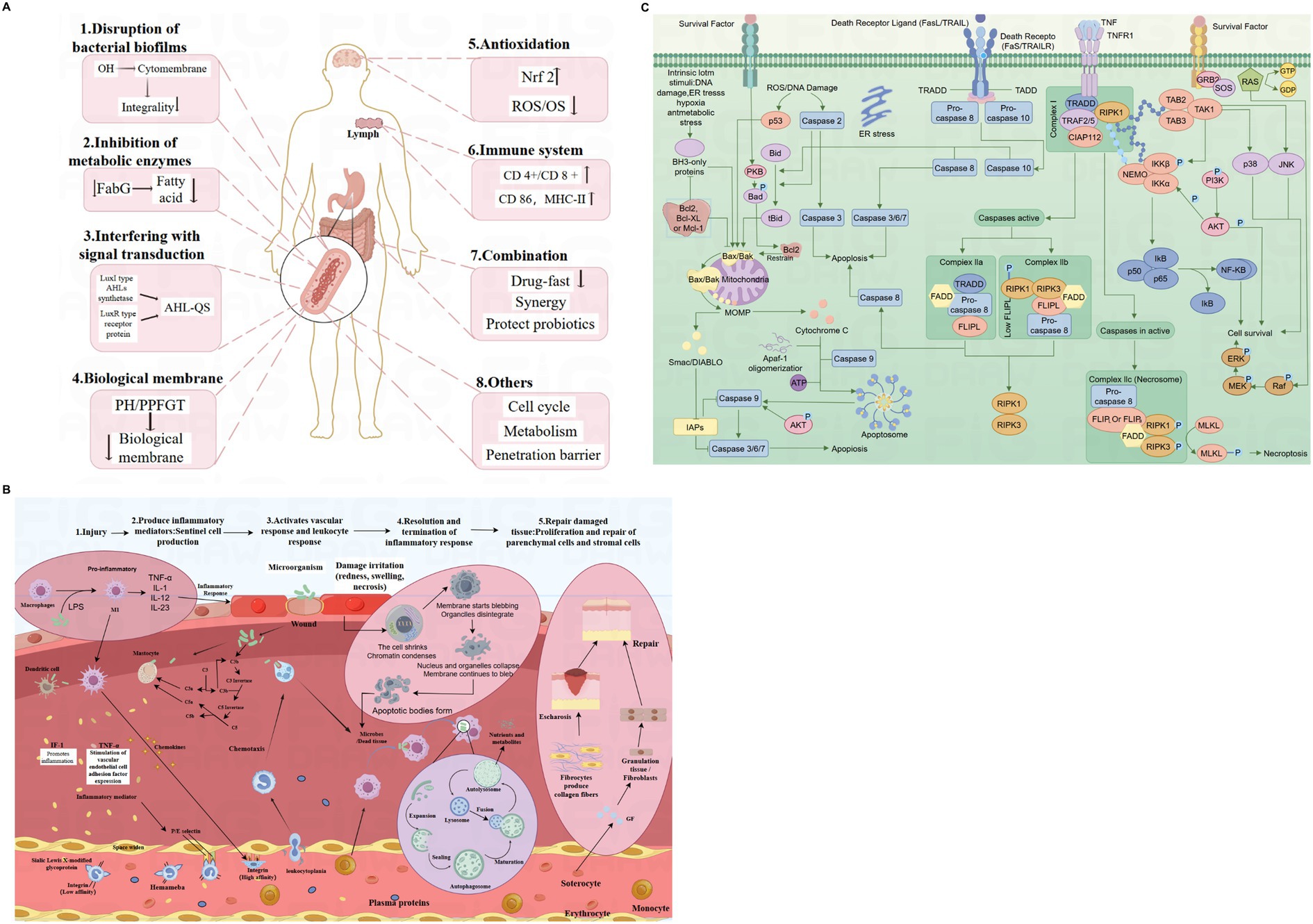
Figure 1. Mechanisms of polyphenols in biological systems: antibacterial mechanisms of polyphenols: disruption of biofilms, enzyme inhibition, signal transduction interference, and immune modulation (A). Cellular and molecular mechanisms of inflammation and tissue repair (B). Mechanisms of mitochondrial oxidative stress and its impact on apoptosis and survival pathways (C).
Furthermore, polyphenols possess significant anti-biofilm properties (43). Biofilms are structural communities of microbial cells encased in extracellular polysaccharides, providing a protective barrier against host immune responses and antibiotics. Polyphenols inhibit biofilm formation without affecting bacterial cell growth, thus reducing the protective effects of the biofilm and decreasing bacterial resistance to antibiotics (37). This has been demonstrated in studies using Padma heptaplex (PH) and polyphenol extracts from green tea (PPFGT), where therapeutic effects were enhanced (44).
Polyphenols also inhibit key enzymes involved in bacterial growth and metabolism. For example, tea polyphenols affect the membrane proteins of P. aeruginosa by inhibiting enzymes in the tricarboxylic acid cycle, fatty acid biosynthesis, protein biosynthesis, and DNA metabolism (45). Catechins inhibit the antibacterial activity of DNA gyrase, while apigenin inhibits both DNA gyrase and hydroxyacyl-acyl carrier protein dehydratase activity (46).
Moreover, polyphenols can decrease bacterial outer membrane permeability, leading to the loss of chemiosmotic control and cell death. The effectiveness of polyphenols as antimicrobial agents depends on factors such as chemical structure, concentration, bacterial species, and environmental conditions, with varied efficacy in laboratory versus food settings (47). Despite these variables, polyphenols offer significant advantages in antibacterial food packaging by inhibiting microbial growth with minimal impact on food quality. The diversity of plant-derived polyphenols reduces the likelihood of contributing to antimicrobial resistance and may even reverse antibiotic resistance, thereby reducing human exposure to resistant bacteria (48). This positions polyphenols as promising natural alternatives to synthetic antioxidants in food products, addressing consumer concerns about the carcinogenicity of synthetic compounds and enhancing the safety and quality of food.
3.2 Anti-inflammatory effect
Inflammation is the body’s defensive response to injury or infection, involving several stages (Figure 1B). Various injurious agents, such as viruses, bacteria, and microorganisms, damage tissues or cells, prompting nearby sentinel cells (macrophages, mast cells, dendritic cells) to recognize these harmful agents or necrotic materials (49). This recognition triggers the production of inflammatory mediators, initiating vascular responses and leukocyte reactions. Consequently, leukocytes and plasma proteins exude from the blood circulation surrounding the injury site. These white blood cells and plasma proteins then arrive at the site of damage, where they work to dilute, neutralize, and eliminate harmful substances. Phagocytes engulf apoptotic tissues and cells, producing growth factors (GF) and cytokines that stimulate fibroblasts and stromal cell proteins, thereby accelerating the repair of injured sites. Additionally, in response to inflammatory mediators, monocytes in the blood differentiate into macrophages, supplementing the inflammatory response process.
Many diseases are inextricably linked to inflammation, including cancer, type II diabetes, obesity, arthritis, and neurodegenerative diseases (50). Polyphenols can mitigate inflammatory responses through various pathways. For instance, they inhibit the expression of proinflammatory genes (30), reduce the production of inflammatory mediators, and suppress the activity of enzymes involved in inflammatory processes, such as cyclooxygenase, lipoxygenase, MAPK (mitogen-activated protein kinase), and IKK (κ kinase inhibitor) (29, 51, 52). Table 1C summarizes the anti-inflammatory mechanisms of polyphenols.
Polyphenols inhibit the production of inflammatory mediators by inflammatory cells, such as macrophages and monocytes, including prostaglandin E2 (PGE2), tumor necrosis factor α (TNF-α), interleukin (IL-1β, IL-6, etc.), and other cytokines (26). This action on macrophages plays a crucial role in the inflammatory response. Furthermore, polyphenols regulate intracellular signaling pathways by inhibiting the activation of NF-κB (nuclear factor κB), a key transcription factor that regulates the expression of inflammatory genes involved in cellular immunity, inflammation, stress, proliferation, and apoptosis (27). They inhibit NF-κB activation and reduce the expression of inflammatory genes by affecting IKK activation, regulating oxidant levels, or interfering with the binding of NF-κB to DNA (53). Polyphenols also modulate MAPK signaling and arachidonic acid signaling to reduce the inflammatory response at various levels, including blocking the release of TNF-α (53).
Additionally, the antioxidant properties of polyphenols help neutralize free radicals and reactive oxygen species (ROS) produced during inflammation, which cause cellular damage. By eliminating these harmful substances, polyphenols alleviate oxidative stress and the associated inflammatory responses (28). The anti-inflammatory mechanisms of polyphenols render them promising therapeutic agents for treating and the treatment and prevention of inflammatory diseases. However, the anti-inflammatory effect of polyphenols may differ depending on various factors, including their chemical structure, dose, mode of administration, and individual differences (54). Future research should focus on identifying the most effective polyphenols and their optimal dosages.
3.3 Antioxidant performance
Additionally, polyphenols play a crucial role in enhancing the host immune system. Macrophages, which are pivotal in the immune response, perform functions such as phagocytosis, antigen presentation, and immune regulation. Resveratrol, for instance, has been shown to enhance macrophage phagocytic activity and antigen-presenting capability, elevate the expression of CD86 and MHC-II, activate the TLR4/NF-κB/JNK signaling pathway, and increase the proportion of CD4+ and CD8+ T cells in peripheral blood (55, 56), thereby amplifying the immune response. Lymphocytes contribute to immunity by producing antibodies via B cells, which neutralize or mark bacteria for destruction. T cells not only bolster the immune response and directly kill infected cells but also differentiate into memory cells, providing long-term immune protection. This establishes a specific and enduring immune defense against bacterial infections.
Chemically synthesized antioxidants such as butylated hydroxyanisole (BHA), butylated hydroxytoluene (BHT), propyl gallate (PG), and tert-butylhydroquinone (TBHQ) are commonly utilized (57). However, the extensive use of these synthetic antioxidants has raised concerns about their potential adverse effects. High doses of chemically synthesized antioxidants have been shown to cause DNA damage, endocrine disruption, and carcinogenic effects (58–60). For example, BHA can interfere with endocrinology, induce cell cycle arrest, and affect male fertility (61). Similarly, BHT has been linked to effects on mitochondrial and endoplasmic reticulum homeostasis, stalling the cell cycle (62). Moreover, both BHA and BHT have been associated with direct carcinogenic effects (63).
Given these concerns, there is a growing need to identify natural antioxidants that can serve as viable alternatives to traditional synthetic antioxidants. The process of fat oxidation, for example, commences when hydrogen atoms of an unsaturated fatty acid (RH) are removed by an active molecules (such as the hydroxyl radical •OH), forming an alkyl radical (R•). This alkyl radical subsequently forms a peroxy radical (ROO•), which removes hydrogen atoms from another fatty acid molecule, resulting in the formation of new alkyl radicals and lipid peroxides (ROOH). The two free radicals eventually interact to form a non-free radical stable molecule, such as a dimer or polymer, thus concluding the oxidation process (57). The addition of antioxidants interferes with this process, retarding oxidation.
The process of protein oxidation is similar to lipid oxidation, but the free radicals formed in the initial stage are protein radicals, which undergo further oxidative reactions to ultimately form non-radical products (57). The final product of protein oxidation can be a carbon–carbon cross-linked derivative (64, 65). The distinction between lipid and protein oxidation is also reflected in the resulting oxidation products. Lipid oxidation can alter taste and odor, whereas protein oxidation can affect juice, tenderness, and color.
Figure 1C shows some of the pathways of apoptosis and survival. During apoptosis, the cell first undergoes a reduction in size, coalescence of genetic material, and eventual breakdown into apoptotic vesicles, which are ultimately removed by phagocytes. Several natural products have demonstrated significant antioxidant potential. Studies show that many vegetables and fruits, such as sorghum (66), rosehips (67), olive oil and olives (68), grape seeds, cherries, berries, pomegranates, parsley, artichokes, and kale (69), as well as whole grains (such as rice) (70), and catechins and theaflavins in tea, possess considerable antioxidant properties. Some studies have explored the potential of these compounds for food preservation, yielding encouraging results. For instance, rosemary, which is rich in caffeic acid, rosmarinic acid, flavonoids, and phenolic diterpenes, has shown the highest antioxidant and antibacterial effects in experiments with cottage cheese (71). Additionally, rosemary extract demonstrated similar protein antioxidant properties to BHT in the preservation of refrigerated pig liver (72). Guava leaf extract, when incorporated into fresh pork sausage, inhibited fat oxidation compared to a control group with BHT added (73). Similarly, adding bee pollen to sausages stored at low temperatures for a month significantly reduced lipid peroxidation (74).
However, certain limitations exist, such as the instability of astaxanthin and carotene upon exposure to light, which diminishes their antioxidant efficacy (75, 76). The antioxidant capacity of polyphenols is attributed to the presence of hydroxyl groups in their structure, with the number and position of these groups being crucial determinants of their antioxidant potential. The antioxidant effect of polyphenols is primarily realized through several mechanisms. Polyphenols engage in free radical scavenging by directly reacting with free radicals, including oxygen radicals (ROS), nitrogen radicals (RNS), and carbon-centered radicals. They neutralize these free radicals through the donation of hydrogen atoms (hydrogen atom transfer, HAT) or electrons (electron transfer, ET), thereby preventing the chain reactions initiated by these radicals (77). Polyphenols possess multiple hydroxyl groups that enable them to form stable chelates with transition metal ions, thereby reducing the generation of free radicals catalyzed by these metals. For instance, curcumin is capable of chelating metal ions such as Cu2+ and Fe2+ (53), while quercetin chelates ferrous ions (78).
Polyphenols are also capable of inhibiting the activity of specific oxidases, such as lipoxygenase (LOX) and cyclooxygenase (COX) (79). These enzymes are responsible for catalyzing the production of active oxides during inflammation and oxidative stress, which in turn generate inflammatory mediators like leukotrienes and nitric oxide (79). Polyphenols exert their effects by enhancing the antioxidant enzyme system, wherein they activate or upregulate the activity of intracellular antioxidant enzymes such as superoxide dismutase (SOD), glutathione peroxidase (GPx), and catalase (CAT). These enzymes are pivotal in the detoxification of reactive oxygen species (ROS). For example, curcumin has been demonstrated to augment the activity of SOD, GPx, and catalase, consequently mitigating peroxide toxicity (80).
These antioxidant mechanisms act in concert to render polyphenols compelling candidates for the prevention and treatment of oxidative stress-related diseases. Nevertheless, the antioxidant effect of polyphenols is susceptible to modulation by a number of factors, including their chemical structure, concentration, presence form, bioavailability, and other variables. Polyphenols can be employed as antioxidants due to their antioxidant properties. For instance, resveratrol has been demonstrated to play a role in protecting cellular components from oxidative damage and reducing the risk of various degenerative diseases (81).
The figure effectively illustrates the potential of polyphenols in therapeutic applications, including antibacterial treatments, anti-inflammatory interventions, and protection against oxidative stress-related diseases. Future research should focus on identifying specific polyphenol compounds that are most effective in these roles and exploring their bioavailability and efficacy in clinical settings. Understanding the synergies between different polyphenols and their combined effects on these pathways could also open new avenues for developing polyphenol-based therapeutics.
4 Conclusion
Optimizing the extraction processes of polyphenols to enhance efficiency, yield, and quality while reducing environmental impact is aligned with the principles of sustainable and green processing. The challenges of incorporating polyphenols into food products must be addressed with a focus on food safety, sensory quality, and compliance with health standards. Sustainable practices in food processing, including the use of renewable resources, energy-efficient technologies, and waste reduction techniques, are essential for maximizing the benefits of polyphenols. Additionally, advanced analytical techniques such as spectroscopy, chromatography, and molecular diagnostics are crucial for accurately evaluating polyphenol content and efficacy.
This review emphasizes the imperative for sustained research into the intricate mechanisms of polyphenols. Innovation in the application of polyphenols can be driven by fostering cooperation and knowledge sharing among academics, scientists, and industrial experts. This collaborative effort is aimed at promoting the sustainability of advanced food processing methods, ensuring continuous improvement in both processing techniques and product quality, and embracing the principles of the green revolution. Overall, plant polyphenols have the potential to transform the food industry by enhancing the safety, traceability, and authenticity of food products, while facilitating sustainable processing methods and improving food quality assessment.
Author contributions
SS: Writing – original draft, Validation, Software, Methodology, Investigation, Conceptualization. ZL: Formal analysis, Writing – review & editing, Validation, Software, Methodology, Investigation. ML: Writing – review & editing, Validation, Software, Resources, Methodology. NG: Writing – review & editing, Validation, Supervision, Software, Resources. XW: Writing – review & editing, Project administration, Funding acquisition, Conceptualization.
Funding
The author(s) declare that no financial support was received for the research, authorship, and/or publication of this article.
Acknowledgments
We acknowledge the valuable assistance provided by the Key Laboratory of Special Medical of Yantai. Additionally, we would like to thank the Food Safety and Sensory Quality Academic Team (2023XSTD03) of Yantai Institute of Technology for their support and collaboration.
Conflict of interest
The authors declare that the research was conducted in the absence of any commercial or financial relationships that could be construed as a potential conflict of interest.
Publisher’s note
All claims expressed in this article are solely those of the authors and do not necessarily represent those of their affiliated organizations, or those of the publisher, the editors and the reviewers. Any product that may be evaluated in this article, or claim that may be made by its manufacturer, is not guaranteed or endorsed by the publisher.
References
1. Dai, J, and Mumper, RJ. Plant phenolics: extraction, analysis and their antioxidant and anticancer properties. Molecules. (2010) 15:7313–52. doi: 10.3390/molecules15107313
2. Abbas, M, Saeed, F, Anjum, FM, Afzaal, M, Tufail, T, Bashir, MS, et al. Natural polyphenols: an overview. Int J Food Prop. (2016) 20:1689–99. doi: 10.1080/10942912.2016.1220393
3. Nikmaram, N, Budaraju, S, Barba, FJ, Lorenzo, JM, Cox, RB, Mallikarjunan, K, et al. Application of plant extracts to improve the shelf-life, nutritional and health-related properties of ready-to-eat meat products. Meat Sci. (2018) 145:245–55. doi: 10.1016/j.meatsci.2018.06.031
4. Tomadoni, B, Viacava, G, Cassani, L, Moreira, MR, and Ponce, A. Novel biopreservatives to enhance the safety and quality of strawberry juice. J Food Sci Technol. (2015) 53:281–92. doi: 10.1007/s13197-015-2068-9
5. Tsao, R. Chemistry and biochemistry of dietary polyphenols. Nutrients. (2010) 2:1231–46. doi: 10.3390/nu2121231
6. Fu, L, Xu, BT, Xu, XR, Qin, XS, Gan, RY, and Li, HB. Antioxidant capacities and total phenolic contents of 56 wild fruits from South China. Molecules. (2010) 15:8602–17. doi: 10.3390/molecules15128602
7. Lia, A-N, Lia, S, Lia, H-B, Xua, D-P, Xub, X-R, and Chenc, F. Total phenolic contents and antioxidant capacities of 51 edible and wild flowers. J Funct Foods. (2014) 6:319–30. doi: 10.1016/j.jff.2013.10.022
8. Lebaka, VR, Wee, YJ, Ye, W, and Korivi, M. Nutritional composition and bioactive compounds in three different parts of mango fruit. Int J Environ Res Public Health. (2021) 18:741. doi: 10.3390/ijerph18020741
9. Bouaziz, M, Chamkha, M, and Sayadi, S. Comparative study on phenolic content and antioxidant activity during maturation of the olive cultivar Chemlali from Tunisia. J Agric Food Chem. (2004) 52:5476–81. doi: 10.1021/jf0497004
10. Andre, CM, Oufir, M, Hoffmann, L, Hausman, J-F, Rogez, H, Larondelle, Y, et al. Influence of environment and genotype on polyphenol compounds and in vitroantioxidant capacity of native Andean potatoes (Solanum tuberosum L.). J Food Compos Anal. (2009) 22:517–24. doi: 10.1016/j.jfca.2008.11.010
11. Aurora, G-R, Desamparados, SM, Marta, LG, and Giuseppe, F. Phenolic and volatile compounds of extra virgin olive oil (Olea europaea L. Cv Cornicabra) with regard to fruit ripening and irrigation management. J Agric Food Chem. (2006) 54:7130–6. doi: 10.1021/jf060798r
12. Tabart, J, Kevers, C, Pincemail, J, Defraigne, J-O, and Dommes, J. Antioxidant capacity of black currant varies with organ, season, and cultivar. J Agric Food Chem. (2006) 54:6271–6. doi: 10.1021/jf061112y
13. Nour, V, Trandafir, I, and Cosmulescu, S. Antioxidant capacity, phenolic compounds and minerals content of blackcurrant (Ribes nigrum L.) leaves as influenced by harvesting date and extraction method. Ind Crop Prod. (2014) 53:133–9. doi: 10.1016/j.indcrop.2013.12.022
14. Heimler, D, Romani, A, and Ieri, F. Plant polyphenol content, soil fertilization and agricultural management: a review. Eur Food Res Technol. (2017) 243:1107–15. doi: 10.1007/s00217-016-2826-6
15. Mullen, W, Stewart, AJ, Lean, MEJ, Gardner, P, Duthie, GG, and Crozier, A. Effect of freezing and storage on the phenolics, ellagitannins, flavonoids, and antioxidant capacity of red raspberries. J Agric Food Chem. (2002) 50:5197–201. doi: 10.1021/jf020141f
16. Cristiana, M, Emma, C, Attilio, V, Vincenzo, F, and Nicoletta, P. Effects of different cooking methods on nutritional and physicochemical characteristics of selected vegetables. J Agric Food Chem. (2008) 56:139–47. doi: 10.1021/jf072304b
17. Ferracane, R, Pellegrini, N, Visconti, A, Graziani, G, Chiavaro, E, Miglio, C, et al. Effects of different cooking methods on antioxidant profile, antioxidant capacity, and physical characteristics of artichoke. J Agric Food Chem. (2008) 56:8601–8. doi: 10.1021/jf800408w
18. Li, A-N, Li, S, Zhang, Y-J, Xu, X-R, Chen, Y-M, and Li, H-B. Resources and biological activities of natural polyphenols. Nutrients. (2014) 6:6020–47. doi: 10.3390/nu6126020
19. Dong, H, Wang, X, Huang, J, and Xing, J. Effects of post-harvest stigmasterol treatment on quality-related parameters and antioxidant enzymes of green asparagus (Asparagus officinalis L.). Food Addit Contam Part A. (2016) 33:1785–92. doi: 10.1080/19440049.2016.1241896
20. Giordano, M, Bertolino, M, Belviso, S, Ghirardello, D, and Zeppa, G. Effects of species, post-harvest treatment, and roasting on fibre, volatile compounds, and polyphenol contents in coffee silverskin. Food Secur. (2022) 11:3132. doi: 10.3390/foods11193132
21. Lapornik, B, Prošek, M, and Golc Wondra, A. Comparison of extracts prepared from plant by-products using different solvents and extraction time. J Food Eng. (2005) 71:214–22. doi: 10.1016/j.jfoodeng.2004.10.036
22. Sabaragamuwa, R, and Perera, CO. Total triterpenes, polyphenols, flavonoids, and antioxidant activity of bioactive phytochemicals of Centella asiatica by different extraction techniques. Food Secur. (2023) 12:3972. doi: 10.3390/foods12213972
23. Park, M, and Lee, K-G. Effect of roasting temperature and time on volatile compounds, total polyphenols, total flavonoids, and lignan of omija (Schisandra chinensis Baillon) fruit extract. Food Chem. (2021) 338:127836. doi: 10.1016/j.foodchem.2020.127836
24. Kim, J, and Choe, E. Effect of the pH on the lipid oxidation and polyphenols of soybean oil-in-water emulsion with added peppermint (Mentha piperita) extract in the presence and absence of iron. Food Sci Biotechnol. (2018) 27:1285–92. doi: 10.1007/s10068-018-0324-2
25. Tang, WQ, Li, DC, Lv, YX, and Jiang, JG. Extraction and removal of caffeine from green tea by ultrasonic-enhanced supercritical fluid. J Food Sci. (2010) 75:C363–8. doi: 10.1111/j.1750-3841.2010.01604.x
26. González, R, Ballester, I, López-Posadas, R, Suárez, MD, Zarzuelo, A, Martínez-Augustin, O, et al. Effects of flavonoids and other polyphenols on inflammation. Crit Rev Food Sci Nutr. (2011) 51:331–62. doi: 10.1080/10408390903584094
27. Bitler, CM, Viale, TM, Damaj, B, and Crea, R. Hydrolyzed olive vegetation water in mice has anti-inflammatory activity. J Nutr. (2005) 135:1475–9. doi: 10.1093/jn/135.6.1475
28. Zorov, DB, Juhaszova, M, and Sollott, SJ. Mitochondrial reactive oxygen species (ROS) and ROS-induced ROS release. Physiol Rev. (2014) 94:909–50. doi: 10.1152/physrev.00026.2013
29. Bae, J-S. Role of high mobility group box 1 in inflammatory disease: focus on sepsis. Arch Pharm Res. (2012) 35:1511–23. doi: 10.1007/s12272-012-0901-5
30. Karasawa, K, Uzuhashi, Y, Hirota, M, and Otani, H. A matured fruit extract of date palm tree (Phoenix dactylifera L.) stimulates the cellular immune system in mice. J Agric Food Chem. (2011) 59:11287–93. doi: 10.1021/jf2029225
31. Capiralla, H, Vingtdeux, V, Venkatesh, J, Dreses-Werringloer, U, Zhao, HT, Davies, P, et al. Identification of potent small-molecule inhibitors of STAT3 with anti-inflammatory properties in RAW 264.7 macrophages. FEBS J. (2012) 279:3791–9. doi: 10.1111/j.1742-4658.2012.08739.x
32. Gresele, P, Cerletti, C, Guglielmini, G, Pignatelli, P, de Gaetano, G, and Violi, F. Effects of resveratrol and other wine polyphenols on vascular function: an update. J Nutr Biochem. (2011) 22:201–11. doi: 10.1016/j.jnutbio.2010.07.004
33. Xiaokang, W, Lyng, JG, Brunton, NP, Cody, L, Jacquier, J-C, Harrison, SM, et al. Monitoring the effect of different microwave extraction parameters on the recovery of polyphenols from shiitake mushrooms: comparison with hot-water and organic-solvent extractions. Biotechnol Rep. (2020) 27:e00504. doi: 10.1016/j.btre.2020.e00504
34. Umaña, M, Eim, V, Garau, C, Rosselló, C, and Simal, S. Ultrasound-assisted extraction of ergosterol and antioxidant components from mushroom by-products and the attainment of a β-glucan rich residue. Food Chem. (2020) 332:127390. doi: 10.1016/j.foodchem.2020.127390
35. Rocchetti, G, Blasi, F, Montesano, D, Ghisoni, S, Marcotullio, MC, Sabatini, S, et al. Impact of conventional/non-conventional extraction methods on the untargeted phenolic profile of Moringa oleifera leaves. Food Res Int. (2019) 115:319–27. doi: 10.1016/j.foodres.2018.11.046
36. Pauli, A, and Knobloch, K. Inhibitory effects of essential oil components on growth of food-contaminating fungi. Z Lebensm Unters Forsch. (1987) 185:10–3. doi: 10.1007/bf01083332
37. Ma, Y, Zhao, L, Gao, M, and Loor, JJ. Tea polyphenols protect bovine mammary epithelial cells from hydrogen peroxide-induced oxidative damage in vitro. J Anim Sci. (2018) 96:4159–4172. doi: 10.1093/jas/sky278
38. Gyawali, R, and Ibrahim, SA. Natural products as antimicrobial agents. Food Control. (2014) 46:412–429. doi: 10.1016/j.foodcont.2014.05.047
39. Deans, SG, and Simpson, EJ. Antioxidants from Salvia officinalis. In: Sage. CRC Press (2000) 199–206.
40. Ultee, A, Bennik, MHJ, and Moezelaar, R. The phenolic hydroxyl group of carvacrol is essential for action against the food-borne pathogen Bacillus cereus. Appl Environ Microbiol. (2002) 68:1561–1568. doi: 10.1128/AEM.68.4.1561-1568.2002
41. Zhang, J, Rui, X, Wang, L, Guan, Y, Sun, X, and Dong, M. Polyphenolic extract from Rosa rugosa tea inhibits bacterial quorum sensing and biofilm formation. Food Control. (2014) 42:125–31. doi: 10.1016/j.foodcont.2014.02.001
42. Huber, B, Riedel, K, Köthe, M, Givskov, M, Molin, S, and Eberl, L. Genetic analysis of functions involved in the late stages of biofilm development in Burkholderia cepacia H111. Mol Microbiol. (2002) 46:411–426. doi: 10.1046/j.1365-2958.2002.03182.x
43. Dong, G, Liu, H, Yu, X, Zhang, X, Lu, H, Zhou, T, et al. Antimicrobial and anti-biofilm activity of tannic acid against Staphylococcus aureus. Nat Prod Res. (2017) 32:2225–8. doi: 10.1080/14786419.2017.1366485
44. Farkash, Y, Feldman, M, Ginsburg, I, Steinberg, D, and Shalish, M. Polyphenols inhibit Candida albicans and Streptococcus mutans biofilm formation. Dent J. (2019) 7:42. doi: 10.3390/dj7020042
45. Yi, S-m, Zhu, J-l, Fu, L-l, and Li, J-r. Tea polyphenols inhibit Pseudomonas aeruginosa through damage to the cell membrane. Int J Food Microbiol. (2010) 144:111–117. doi: 10.1016/j.ijfoodmicro.2010.09.005
46. Gradišar, H, Pristovšek, P, Plaper, A, and Jerala, R. Green tea catechins inhibit bacterial DNA gyrase by interaction with its ATP binding site. J Med Chem. (2007) 50:264–271. doi: 10.1021/jm060817o
47. Huang, T, Qian, Y, Wei, J, and Zhou, C. Polymeric antimicrobial food packaging and its applications. Polymers. (2019) 11:560. doi: 10.3390/polym11030560
48. Gupta, PD, and Birdi, TJ. Development of botanicals to combat antibiotic resistance. J Ayurveda Integr Med. (2017) 8:266–75. doi: 10.1016/j.jaim.2017.05.004
49. Dalli, J, Serhan, C, and Gordon, S. Macrophage proresolving mediators—the when and where. Microbiol Spectrum. (2016) 4. doi: 10.1128/microbiolspec.MCHD-0001-2014
50. Bengmark, S. Acute and “chronic” phase reaction—a mother of disease. Clin Nutr. (2004) 23:1256–66. doi: 10.1016/j.clnu.2004.07.016
51. Leiherer, A, Mündlein, A, and Drexel, H. Phytochemicals and their impact on adipose tissue inflammation and diabetes. Vasc Pharmacol. (2013) 58:3–20. doi: 10.1016/j.vph.2012.09.002
52. Siddiqui, AM, Cui, X, Wu, R, Dong, W, Zhou, M, Hu, M, et al. The anti-inflammatory effect of curcumin in an experimental model of sepsis is mediated by up-regulation of peroxisome proliferator-activated receptor-γ*. Crit Care Med. (2006) 34:1874–82. doi: 10.1097/01.Ccm.0000221921.71300.Bf
53. Yahfoufi, N, Alsadi, N, Jambi, M, and Matar, C. The immunomodulatory and anti-inflammatory role of polyphenols. Nutrients. (2018) 10:1618. doi: 10.3390/nu10111618
54. Dinarello, CA. Anti-inflammatory agents: present and future. Cell. (2010) 140:935–50. doi: 10.1016/j.cell.2010.02.043
55. Arruvito, L, Sanz, M, Banham, AH, and Fainboim, L. Expansion of CD4+ CD25+ and FOXP3+ regulatory T cells during the follicular phase of the menstrual cycle: implications for human reproduction. J Immunol Res. (2007) 178:2572–2578. doi: 10.4049/jimmunol.178.4.2572
56. Chen, M, Chen, X, Song, X, Muhammad, A, Jia, R, Zou, Y, et al. The immune-adjuvant activity and the mechanism of resveratrol on pseudorabies virus vaccine in a mouse model. Int Immunopharmacol. (2019) 76:105876. doi: 10.1016/j.intimp.2019.105876
57. Gutiérrez-del-Río, I, López-Ibáñez, S, Magadán-Corpas, P, Fernández-Calleja, L, Pérez-Valero, Á, Tuñón-Granda, M, et al. Terpenoids and polyphenols as natural antioxidant agents in food preservation. Antioxidants. (2021) 10:1264. doi: 10.3390/antiox10081264
58. Baran, A, Yildirim, S, Ghosigharehaghaji, A, Bolat, İ, Sulukan, E, and Ceyhun, SB. An approach to evaluating the potential teratogenic and neurotoxic mechanism of BHA based on apoptosis induced by oxidative stress in zebrafish embryo (Danio rerio). Hum Exp Toxicol. (2020) 40:425–38. doi: 10.1177/0960327120952140
59. Eskandani, M, Hamishehkar, H, and Ezzati Nazhad Dolatabadi, J. Cytotoxicity and DNA damage properties of tert-butylhydroquinone (TBHQ) food additive. Food Chem. (2014) 153:315–20. doi: 10.1016/j.foodchem.2013.12.087
60. Vandghanooni, S, Forouharmehr, A, Eskandani, M, Barzegari, A, Kafil, V, Kashanian, S, et al. Cytotoxicity and DNA fragmentation properties of butylated hydroxyanisole. DNA Cell Biol. (2013) 32:98–103. doi: 10.1089/dna.2012.1946
61. Ham, J, Lim, W, You, S, and Song, G. Butylated hydroxyanisole induces testicular dysfunction in mouse testis cells by dysregulating calcium homeostasis and stimulating endoplasmic reticulum stress. Sci Total Environ. (2020) 702:134775. doi: 10.1016/j.scitotenv.2019.134775
62. Ham, J, Lim, W, Whang, K-Y, and Song, G. Butylated hydroxytoluene induces dysregulation of calcium homeostasis and endoplasmic reticulum stress resulting in mouse Leydig cell death. Environ Pollut. (2020) 256:113421. doi: 10.1016/j.envpol.2019.113421
63. Bauer, AK, and Dwyer-Nield, LD. Two-stage 3-methylcholanthrene and butylated hydroxytoluene-induced lung carcinogenesis in mice. Methods Cell Biol. (2021) 163:153–73. doi: 10.1016/bs.mcb.2020.07.003
64. Cunha, LCM, Monteiro, MLG, Lorenzo, JM, Munekata, PES, Muchenje, V, de Carvalho, FAL, et al. Natural antioxidants in processing and storage stability of sheep and goat meat products. Food Res Int. (2018) 111:379–90. doi: 10.1016/j.foodres.2018.05.041
65. Ribeiro, JS, Santos, MJMC, Silva, LKR, Pereira, LCL, Santos, IA, da Silva Lannes, SC, et al. Natural antioxidants used in meat products: a brief review. Meat Sci. (2019) 148:181–8. doi: 10.1016/j.meatsci.2018.10.016
66. Ortiz-Cruz, RA, Ramírez-Wong, B, Ledesma-Osuna, AI, Torres-Chávez, PI, Sánchez-Machado, DI, Montaño-Leyva, B, et al. Effect of extrusion processing conditions on the phenolic compound content and antioxidant capacity of Sorghum (Sorghum bicolor (L.) Moench) bran. Plant Foods Hum Nutr. (2020) 75:252–7. doi: 10.1007/s11130-020-00810-6
67. Koczka, N, Stefanovits-Bányai, É, and Ombódi, A. Total polyphenol content and antioxidant capacity of rosehips of some Rosa species. Medicines. (2018) 5:84. doi: 10.3390/medicines5030084
68. Peršurić, Ž, Saftić Martinović, L, Zengin, G, Šarolić, M, and Kraljević Pavelić, S. Characterization of phenolic and triacylglycerol compounds in the olive oil by-product pâté and assay of its antioxidant and enzyme inhibition activity. LWT. (2020) 125:109225. doi: 10.1016/j.lwt.2020.109225
69. Ruiz-Torralba, A, Guerra-Hernández, EJ, and García-Villanova, B. Antioxidant capacity, polyphenol content and contribution to dietary intake of 52 fruits sold in Spain. CyTA J Food. (2018) 16:1131–8. doi: 10.1080/19476337.2018.1517828
70. Ding, C, Liu, Q, Li, P, Pei, Y, Tao, T, Wang, Y, et al. Distribution and quantitative analysis of phenolic compounds in fractions of Japonica and Indica rice. Food Chem. (2019) 274:384–91. doi: 10.1016/j.foodchem.2018.09.011
71. Hashemi, SMB, Amininezhad, R, Shirzadinezhad, E, Farahani, M, and Yousefabad, SHA. The antimicrobial and antioxidant effects of Citrus aurantium L. flowers (Bahar Narang) extract in traditional yoghurt stew during refrigerated storage. J Food Saf. (2015) 36:153–61. doi: 10.1111/jfs.12222
72. Estévez, M, Ventanas, S, and Cava, R. Effect of natural and synthetic antioxidants on protein oxidation and colour and texture changes in refrigerated stored porcine liver pâté. Meat Sci. (2006) 74:396–403. doi: 10.1016/j.meatsci.2006.04.010
73. Tran, TTT, Ton, NMN, Nguyen, TT, Le, VVM, Sajeev, D, Schilling, MW, et al. Application of natural antioxidant extract from guava leaves (Psidium guajava L.) in fresh pork sausage. Meat Sci. (2020) 165:108106. doi: 10.1016/j.meatsci.2020.108106
74. Almeida, JDF, Reis, ASD, Heldt, LFS, Pereira, D, Bianchin, M, Moura, CD, et al. Lyophilized bee pollen extract: a natural antioxidant source to prevent lipid oxidation in refrigerated sausages. LWT Food Sci Technol. (2017) 76:299–305. doi: 10.1016/j.lwt.2016.06.017
75. Carocho, M, Morales, P, and Ferreira, ICFR. Antioxidants: reviewing the chemistry, food applications, legislation and role as preservatives. Trends Food Sci Technol. (2018) 71:107–20. doi: 10.1016/j.tifs.2017.11.008
76. de Oliveira, VS, Ferreira, FS, Cople, MCR, Labre, TDS, Augusta, IM, Gamallo, OD, et al. Use of natural antioxidants in the inhibition of cholesterol oxidation: a review. Compr Rev Food Sci Food Saf. (2018) 17:1465–83. doi: 10.1111/1541-4337.12386
77. de Lima Cherubim, DJ, Buzanello Martins, CV, Oliveira Fariña, L, and da Silva de Lucca, RA. Polyphenols as natural antioxidants in cosmetics applications. J Cosmet Dermatol. (2019) 19:33–7. doi: 10.1111/jocd.13093
78. Heim, KE, Tagliaferro, AR, and Bobilya, DJ. Flavonoid antioxidants: chemistry, metabolism and structure-activity relationships. J Nutr Biochem. (2002) 13:572–84. doi: 10.1016/s0955-2863(02)00208-5
79. Cheon, BS, Kim, YH, Son, KS, Chang, HW, Kang, SS, and Kim, HP. Effects of prenylated flavonoids and biflavonoids on lipopolysaccharide-induced nitric oxide production from the mouse macrophage cell line RAW 264.7. Planta Med. (2000) 66:596–600. doi: 10.1055/s-2000-8621
80. Sporn, MB, and Liby, KT. NRF2 and cancer: the good, the bad and the importance of context. Nat Rev Cancer. (2012) 12:564–71. doi: 10.1038/nrc3278
Keywords: bioactive constituents, biological activity, extraction methods, sustainable processing, mechanisms
Citation: Sun S, Liu Z, Lin M, Gao N and Wang X (2024) Polyphenols in health and food processing: antibacterial, anti-inflammatory, and antioxidant insights. Front. Nutr. 11:1456730. doi: 10.3389/fnut.2024.1456730
Edited by:
Luca Rastrelli, University of Salerno, ItalyReviewed by:
Corina Predescu, University of Agronomic Sciences and Veterinary Medicine, RomaniaCopyright © 2024 Sun, Liu, Lin, Gao and Wang. This is an open-access article distributed under the terms of the Creative Commons Attribution License (CC BY). The use, distribution or reproduction in other forums is permitted, provided the original author(s) and the copyright owner(s) are credited and that the original publication in this journal is cited, in accordance with accepted academic practice. No use, distribution or reproduction is permitted which does not comply with these terms.
*Correspondence: Xiaojie Wang, d3hqMTAzMDRAMTI2LmNvbQ==