- 1Gansu Engineering Technology Research Center for Microalgae, Hexi University, Zhangye, China
- 2College of Life Sciences, Yantai University, Yantai, China
Spirulina is capable of using light energy and fixing carbon dioxide to synthesize a spectrum of organic substances, including proteins, polysaccharides, and unsaturated fatty acids, making it one of the most coveted food resources for humanity. Conventionally, Spirulina products are formulated into algal powder tablets or capsules. However, the processing and preparation of these products, involving screw pump feeding, extrusion, high-speed automation, and high-temperature dewatering, often result in the rupture of cell filaments, cell fragmentation, and the unfortunate loss of vital nutrients. In contrast, fresh Spirulina, cultivated within a closed photobioreactor and transformed into an edible delight through harvesting, washing, filtering, and sterilizing, presents a refreshing taste and odor. It is gradually earning acceptance as a novel health food among the general public. This review delves into the manufacturing processes of fresh Spirulina, analyzes its nutritional advantages over conventional algal powder, and ultimately prospects the avenues for fresh Spirulina’s application in modern food processing. The aim is to provide valuable references for the research and development of new microalgal products and to propel the food applications of microalgae forward.
1 Introduction
Spirulina, also known as Arthrospira, is a type of blue-green algae that has been consumed by humans for centuries. Historical records reveal its use as a food source by the Aztecs and other Mesoamerican cultures dating back to the 16th century. Traditionally, it was harvested from the alkaline waters of Lake Texcoco in Mexico and transformed into dried cakes for consumption (1, 2). Today, Spirulina stands as a prominent economic microalgae species, produced extensively worldwide, and has become a favored dietary supplement among health-conscious individuals. This remarkable algae species is abundant in high-quality plant proteins, polysaccharides, carotenoids, phycocyanin, vitamins, minerals, and other trace elements (3). Research has uncovered a multitude of health benefits associated with Spirulina, including its ability to lower blood lipids, resist oxidation, combat infection, cancer, radiation, and aging, while also enhancing the body’s immune system. Consequently, Spirulina presents itself as an exceptional candidate for the creation of high-quality dietary supplements and nutraceutical products (4, 5).
Currently, Spirulina is commercially produced in diverse locations worldwide, including Hawaii, Mexico, Thailand, China, and India. The cultivation process entails growing the algae in shallow water and constantly agitating the water to ensure optimal sunlight exposure and nutrient absorption (4). Once harvested, Spirulina can be transformed into various forms such as dry powder, flakes, tablets, and capsules, making it a versatile dietary supplement. However, in the conventional production process, after harvesting and spray drying, the fresh biomass undergoes high temperatures, leading to significant nutritional losses. This includes protein denaturation, pigment oxidation, and Maillard reactions between sugar and amine, ultimately resulting in reduced nutritional value. Specifically, carotenoids and phycocyanin levels decrease by over 20%, and other heat-sensitive biological enzyme active substances also experience substantial losses (6, 7).
Therefore, the living and fresh Spirulina presents itself as an exceptional alternative to the currently available dry powder products in the market, particularly appealing to consumers who are increasingly mindful of their nutrition. The fresh Spirulina is primarily cultivated in closed photobioreactor, eliminating the need for spray drying and thus fully preserving its nutrient composition and bioactive substances. Its refreshing and odorless taste further enhances its appeal to the public (8). China has emerged as a leader in the design and development of fresh Spirulina, solidifying its position as the world’s largest producer. Concurrently, other major algae-producing countries have also demonstrated remarkable efforts and achievements, having developed a diverse range of fresh Spirulina products (9, 10). However, a comprehensive understanding of the nutritional value and application potential of fresh Spirulina remains untapped. This review aims to compare fresh Spirulina with traditional Spirulina products, analyze its nutritional advantages, and summarize its application and development prospects in food processing. By doing so, it lays a foundation for the research of related products and the advancement of the microalgal industry.
2 The production processes differences between fresh Spirulina and traditional product
The two forms of Spirulina products, fresh Spirulina and Spirulina powder (or tablets and pressed candies made from processed Spirulina powder), exhibit notable differences in production process, preservation, and nutritional composition. Traditional Spirulina products are derived from artificially large-scale cultivated Spirulina platensis or Spirulina maxima, undergoing harvest, leaching, rinsing, and drying (1). Fresh Spirulina, on the other hand, refers to concentrated slurry obtained by simply cleaning and processing fresh Spirulina cells harvested from closed photobioreactors. It can be consumed directly or added to food (8, 11) (Figure 1).
2.1 Cultivation systems
Currently, the dry powder is mostly produced using open raceway pond systems, such as those employed by Earthrise Nutritionals, Cyanotech Corporation in the United States, and Neptunus Corporation in China (Figures 2A,B). The Chinese scientists and entrepreneurs have innovatively invented greenhouse raceway pond technology, which represents the most critical technological breakthrough in the industrialization of Spirulina production (Figures 2C,D). This technology significantly extends the annual production period and has directly led to a leap in the biomass of Spirulina in China (12). These cultivation systems utilize natural lighting and employs paddle wheel mechanisms to drive liquid flow and mixing, ensuring uniform light exposure for the Spirulina. Due to its fully or partially open environment, the production process of Spirulina is susceptible to external environmental influences such as rainwater, dust, bacteria, and zooplankton. These contaminants may potentially impact the quality and safety of Spirulina.
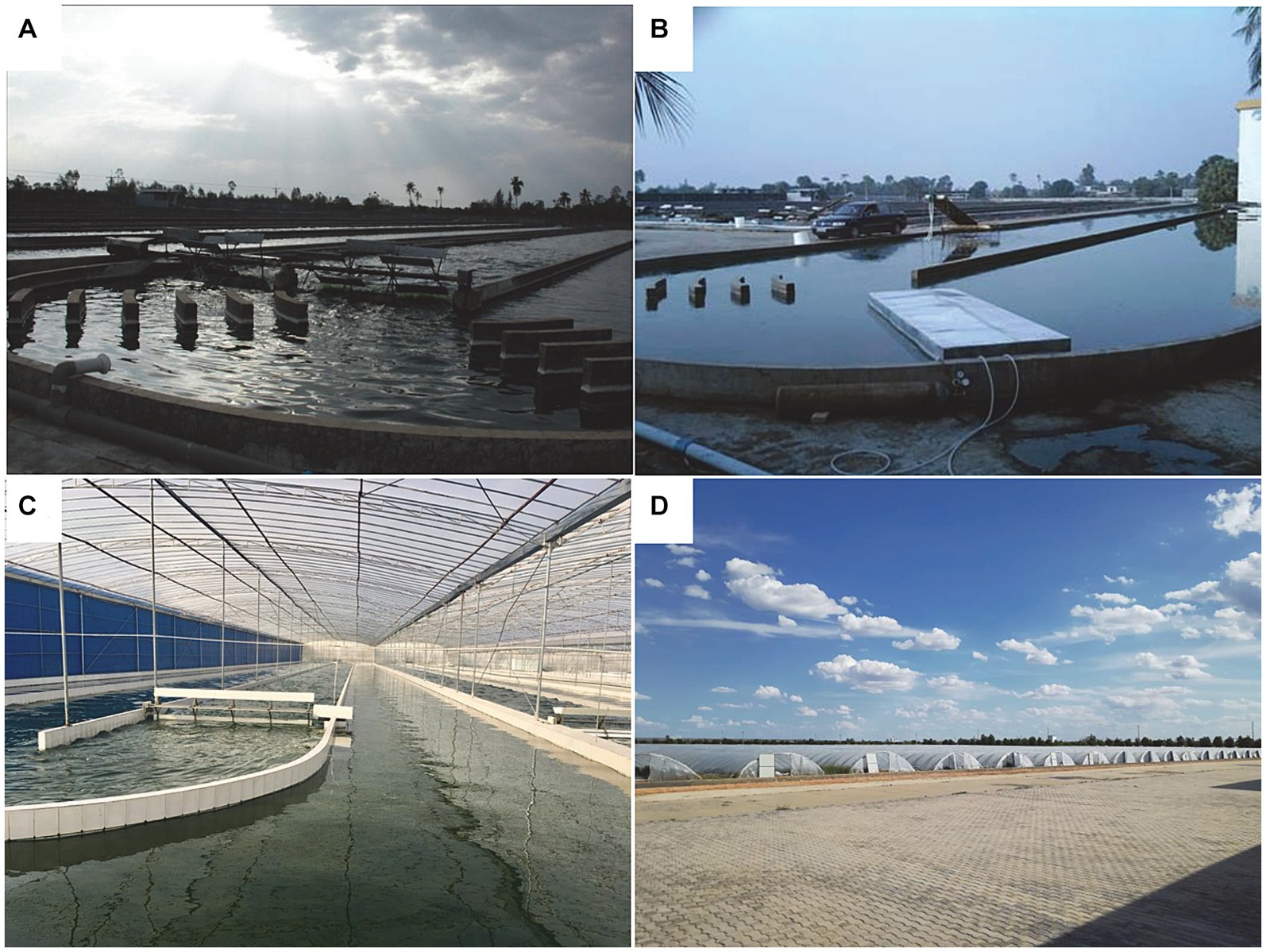
Figure 2. Microalgae large-scale cultivation. (A) Raceway pond for Spirulina production in Sanya. (B) Anti-leakage equipment for CO2 supplementation. Raceway pond in greenhouse in Inner Mongolia greenhouse interior (C) and exterior (D).
The production of fresh Spirulina usually adopts full-enclosed photobioreactors that are ideal for controlling the growth environment. In a fully enclosed reactor, light intensity, temperature, nutrient supply, and gas composition can be adjusted to maximize the productivity and quality of the microalgal biomass. Moreover, strict aseptic operations and equipment disinfection measures inside the reactor are required to ensure sterility. The filtration of air is also crucial for aseptic operation, generally achieved through high-efficiency filters and air disinfection equipment (10, 13).
Photobioreactors are usually composed of vertically or horizontally combined pipes (made of borosilicate or organic glass). In the reactor system with horizontal pipelines, the circulation of the culture medium is driven by pumps. The mixed gas with a high content of CO2 is introduced intermittently or in the pH-feedback-style in the gas–liquid exchange unit to supplement the carbon source for Spirulina growth. Reactors with vertical pipes have a relatively large diameter, usually between 30 and 60 cm. Some light sources of reactors are located inside the cylinder, and others are placed around the reactor to enhance the photosynthesis of microalgal cells. This type of reactor has a high ventilation intensity, requiring extremely rigorous air sterility (Figure 3).
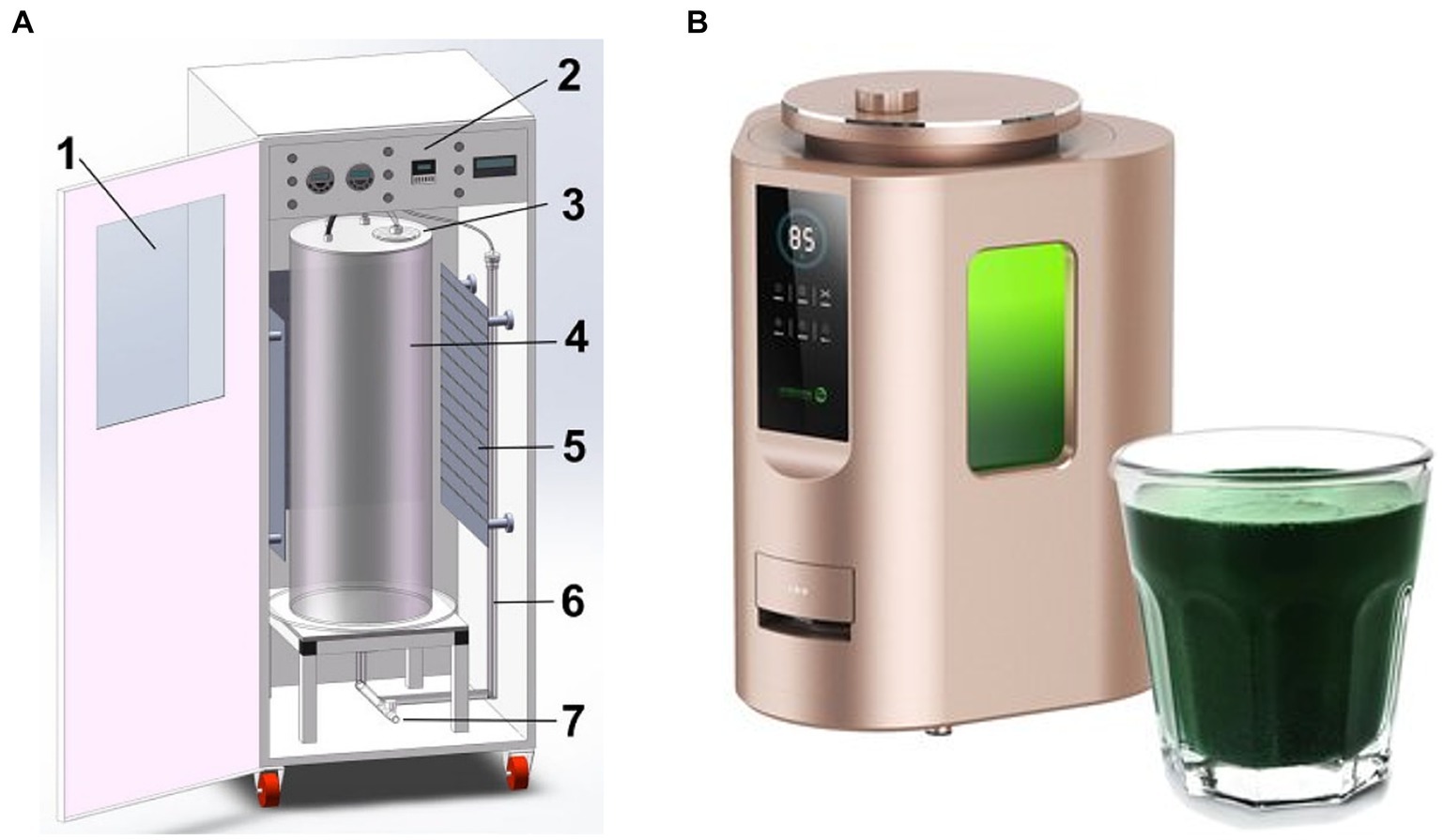
Figure 3. Schematic diagram of column bioreactor for fresh Spirulina production (A, 1: observatory window and front door; 2: controlling panel; 3: reactor upper cover; 4: reactor column; 5: LED light source panel; 6: aeration pipe; 7: water tap) and commercially available equipment (B).
Fresh Spirulina and its production facilities have potentially vital applications in life support systems, especially in enclosed environments, such as space stations and submarines (14, 15). During growing, Spirulina utilizes light energy to absorb CO2 and release oxygen, which is instrumental in maintaining good air quality in enclosed environments such as space stations. Furthermore, Spirulina can provide abundant nutrients, including proteins, vitamins, and minerals, preventing individuals in enclosed environments from nutritional deficiencies, especially vitamin deficiencies. In summary, fresh Spirulina can serve as a nutritious food source, contributing to resource cycling and the self-sustaining of ecosystems in long-term hermetic missions.
2.2 Hygiene and quality problems
Large-scale cultivation of Spirulina typically involves outdoor open ponds driven by paddle wheel mechanisms. These systems are susceptible to contamination by pathogenic microorganisms, including bacteria, fungi, viruses and predators, such as protozoa, rotifers, copepods and cladocerans. Indeed, contamination poses a significant challenge, as it has the potential to substantially diminish the quality of Spirulina products and may potentially lead to foodborne illnesses. In addition, the contamination also reduces the growth rate and the overall biomass productivity. Thus, effective biosecurity and quality control measures during the production process are crucial for preventing biological contamination and ensuring product safety and quality (9).
The methods to control contamination during the cultivation of Spirulina mainly include chemical and physical methods, such as adding antibiotics, ammonium salts (16), as well as filtration or foam flotation (17). To guarantee safety and long-term shelf-life of the dietary supplement, the fresh biomass harvested from open pond is usually hot or spray dried at temperatures above 100°C. Nevertheless, due to contamination during the cultivation process, some bacterial or fungal toxins may remain in the dried Spirulina powder, or the ash content of the Spirulina dry powder may increase. According to the Chinese National Standard (GB/T 16919–2022 General Quality Rules for Edible Spirulina Powder), edible Spirulina dry powder must not be detected with mycotoxins, and its ash content should be less than 7%.
The hygienic quality of fresh Spirulina primarily relies on the sterilization efficacy of the culture medium and equipment, pollution control techniques during cultivation, and non-thermal sterilization methods after filtration and concentration (9). Fresh Spirulina is typically cultivated using purified mineral water or pure water supplemented with nutrient salts as the culture medium. The cultivation equipment is primarily made of high-borosilicate glass or stainless steel that can withstand high-temperature sterilization. Due to the adoption of a fully closed cultivation system, clean air filtration ensures minimal microbial growth. According to the “Fresh Spirulina” group standard (QMZWZ [2022] No. 5) issued by the Qingdao Microalgae Industry Association, the total bacterial count in fresh Spirulina for direct consumption should be less than 10,000 CFU/g, and harmful bacteria such as Salmonella and Staphylococcus aureus should not be detected. When stored in a light-blocking, well-packaged condition at −18°C (±2°C), fresh Spirulina has a shelf life of 12 months, while homemade products should be consumed promptly.
2.3 Downstream processing
Spirulina is typically dried after harvesting to facilitate its storage and incorporation into food. The drying of Spirulina accounts for approximately 30% of the total production cost, and the traditional methods employed to dry fresh Spirulina include spray drying, freeze drying, solar drying, convective hot air drying, and spouted bed drying (18). Subsequently, after re-dissolution, the algal powder presents evident breakage and damage under the microscope. In contrast, the algal filaments of fresh Spirulina are intact, without obvious breakage, damage, or leakage of ingredients (Figure 4).
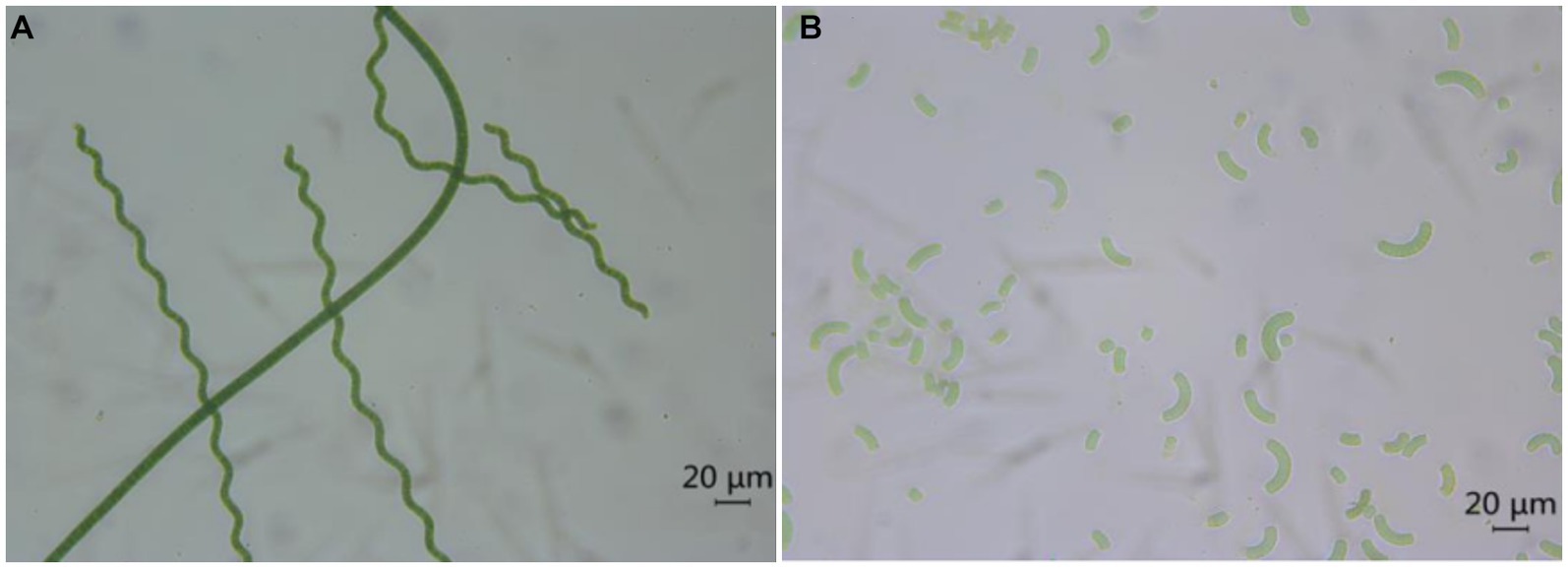
Figure 4. Optical photos of fresh Spirulina (A) and redissolved Spirulina powder (B) under a microscope.
Currently, the primary decontamination methods for fresh Spirulina involve non-thermal sterilization technologies such as pulsed electric field (PEF), ultrasonication, ozonation and cold plasma (19, 20). Within this array of approaches, each method exhibits unique strengths and limitations (as outlined in Table 1), thereby contributing to the ongoing quest for an optimal decontamination solution for Spirulina biomass (9). While preserving nutritional and sensory attributes, these techniques avoid the drawbacks of traditional thermal methods. Cold plasma, in particular, stands out for its versatility, efficacy, and ability to maintain Spirulina’s nutritional excellence. However, challenges persist, including equipment costs and the need for further research to optimize its application at large scales. By carefully selecting the appropriate method, the industry can produce safer and higher-quality fresh Spirulina products.
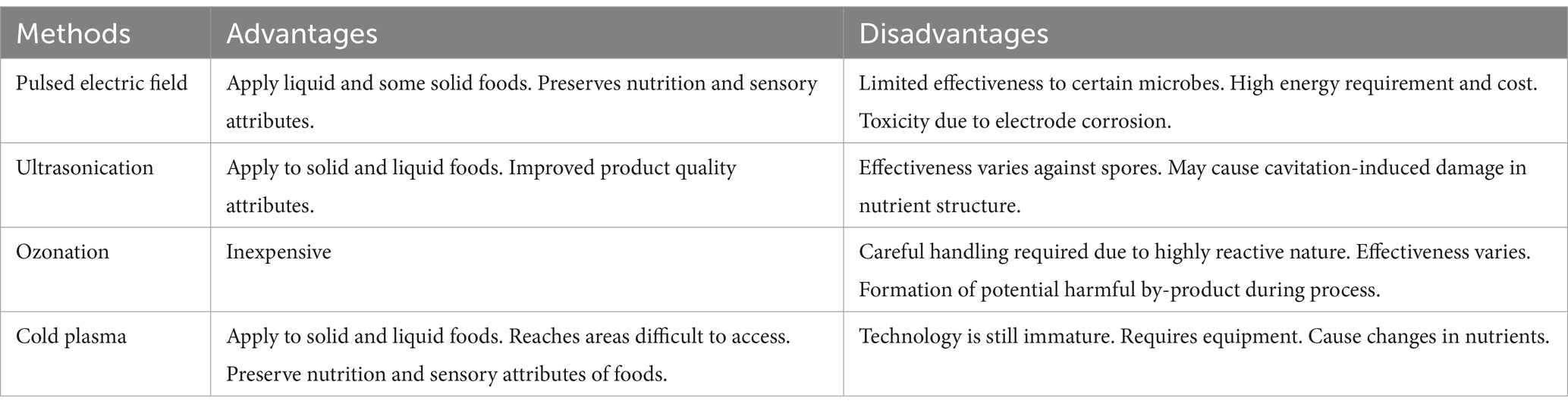
Table 1. Comparison of major advantages and disadvantages of non-thermal techniques for fresh Spirulina.
2.4 Nutrient loss caused by conventional drying method
The dehydration and drying processes of Spirulina powder may induce the loss of some nutritional components. In particular, some thermal-sensitive nutrients, such as vitamins and enzymes, may degrade or denature during processing. By comparison, without intense processing, more natural nutrients are retained in fresh Spirulina. The nutritional components affected by processing means include but are not limited to the following four categories.
2.4.1 Loss of heat-sensitive nutrients
Spirulina contains various heat-sensitive nutrients, such as vitamins C and B vitamins. Because of the high temperature during drying (the spray drying temperature is usually 180–200°C for inlet air and 80–90°C for outlet air), these nutrients are partially decomposed or volatilized, declining their content in Spirulina powder (18). Regarding B vitamins, the total content of B1, B2, niacin, and B6 in fresh Spirulina is 275 mg/kg (on a dry basis), while that in Spirulina powder is 216 mg/kg (on a dry basis), lost by about 20% (21).
2.4.2 Abated protein and enzyme activity
Spirulina is rich in proteins and enzyme substances, some of which are critical for human health. However, the high temperature in the drying process may cause the denaturation of some proteins and loss of enzyme activity, affecting the content and activity of these nutrients in Spirulina powder. The activity of superoxide dismutase is not detected in Spirulina powder, while its activity unit is 167.7 U/g in fresh Spirulina. In an experiment of feeding mice with fresh Spirulina and dried algae powder, the serum content of SOD with anti-lipid peroxidation function in the fresh Spirulina group is significantly higher than in the algae powder group, indicating that fresh Spirulina has a superior effect on the body’s antioxidant function (22).
2.4.3 Oxidation and degradation of lipids
Spirulina consists of abundant unsaturated fatty acids and other lipid substances, possessing substantial nutritional value and health functions (23). However, the drying process brings about the oxidation and degradation of some lipids, diminishing the content of unsaturated fatty acids in Spirulina powder. For instance, eicosapentaenoic acid plays a positive role in lowering blood lipids; docosahexaenoic acid is a major nutrient for the growth and maintenance of nervous system cells and an essential fatty acid that makes up the brain and retina, commonly known as “brain gold.” They are barely detected in Spirulina powder, while in fresh Spirulina, their concentrations reach 4.2 mg/100 g (algal slurry) and 1.9 mg/100 g (algal slurry), respectively (24).
2.4.4 Loss of active ingredients
Some active ingredients in Spirulina, such as polysaccharides and antioxidants, may be impacted by drying treatment. High temperatures and the drying process can cause the denaturation or deactivation of some active ingredients, thereby affecting their content and biological activity in Spirulina powder. Phycocyanin is a natural blue pigment with an outstanding antioxidant capacity and an active protein abundant in Spirulina. After drying at a high temperature of 200°C, the total content of phycocyanin and allophycocyanin in Spirulina powder drops by nearly 20% (6, 25).
3 Nutritional advantages and health benefits of fresh Spirulina
3.1 Amino acid composition and nutritional evaluation of fresh Spirulina
Fresh Spirulina boasts a protein content of no less than 5.5 g per 100 g, surpassing human milk by 6-fold, cow’s milk by 2-fold, and even outpacing goat’s milk by 14%. Moreover, it is abundant in various nutrients essential for a balanced, modern diet, as outlined in Table 2 (2, 9).
The protein content of fresh Spirulina encompasses a broad spectrum of essential amino acids, making it a valuable source of plant-based protein (26). Its supply of essential amino acids is nearly identical to the recommended nutritional composition pattern for humans by the Food and Agriculture Organization of the United Nations and the World Health Organization. Compared with natural cow’s milk, fresh Spirulina may exhibit varying levels and ratios of specific essential amino acids. For example, it is abundant in lysine and isoleucine and relatively deficient in histidine and tryptophan. However, fresh Spirulina stands out as a nutrient-rich, low-fat, and low-cholesterol food alternative to cow’s milk. Moreover, it serves as a superior protein source for vegetarians and lactose-intolerant individuals due to its enhanced digestibility and absorption rates (27).
Overall, the amino acid composition and supply of fresh Spirulina make it a meritorious source of protein, especially for vegetarians and those seeking various protein intakes. Its amino acid composition is more comprehensive than that of natural milk, enhancing its nutritional value.
3.2 Content and unique health functions of phycocyanin
Phycocyanin is one of the most active ingredients in fresh Spirulina and has a strong antioxidant ability. Its content in Spirulina varies with the variety, growth conditions, and harvest timing, typically accounting for 2–10% of total proteins in Spirulina (28). However, the drying process can result in a loss of phycocyanin. Particularly, high temperatures and prolonged intense light may lead to partial denaturation or degradation of phycocyanin (7, 25). In contrast, the complete cell structure in fresh Spirulina can effectively diminish the wastage of phycocyanin as a natural barrier. The efficacy and application areas of phycocyanin include but are not limited to the following types.
3.2.1 Antioxidant
Phycocyanin helps neutralize free radicals in the body and reduce cellular oxidative stress to prevent multiple chronic diseases. When alleviating the side effects of the anticancer drug doxorubicin, phycocyanin can downgrade the expression of the apoptotic gene Bax and the release of cytochrome C in myocardial cells, thereby lowering the oxidative damage of doxorubicin to myocardial cells and abating the risk of triggering primary cardiomyopathy and congestive heart disease (29). Moreover, phycocyanin can serve as an antioxidant in food, health products, and cosmetics.
3.2.2 Cancer treatment
Phycocyanin has shown certain potential in cancer treatment. Some studies suggested that it might constrain tumor growth and spread and alleviate the side effects of radiotherapy and chemotherapy. Phycocyanin can inhibit the proliferation of breast cancer cell MCF-7 in vivo and in vitro and promote cell differentiation and apoptosis (30). Additionally, phycocyanin can suppress the proliferation of ovarian cancer cell SKOV-3 by mediating vascular endothelial growth factor (VEGF) and the signaling pathway of oncogene p53 (31). It can enhance the efficiency of cancer cell therapy by reducing the expression of multidrug-resistant gene proteins. The above findings provide important research directions for the treatment of multiple malignant tumors, new ideas for exploring targeted, safe, and efficient tumor treatments, and broad market prospects for the development and application of fresh Spirulina.
3.2.3 Immune regulation
Phycocyanin is believed to have a specific immune regulatory effect, which can improve the body’s immune function, strengthen resistance, and abate the risk of potential autoimmune diseases. Liu et al. revealed that after treating allergic mice with phycocyanin, the levels of IgE and histamine in the mice decreased, and the release of cytokines IL-4 and IL-13 was downregulated, verifying that phycocyanin can reduce allergic reactions in mice through its immunomodulatory activity (32). Chang et al. confirmed that phycocyanin could decline cellular endocytosis and elevate the expression of costimulatory molecules (33).
3.2.4 Anti-inflammation
Inflammation is a dynamic feed-back of the autoimmune system, which can promote the recovery of damaged tissues, eliminate aging and diseased cells, and protect oneself from external invasion of viruses and bacteria. However, excessive inflammation attacks the tissues and cells of important organs while clearing pathogens, leading to pathological changes and disrupting health (34, 35). Phycocyanin can directly restrain inflammation. The action modes include TLR and KF- κB pathways. Meanwhile, it can indirectly curb inflammation by acting on signaling pathways such as oxidation and apoptosis, thereby reducing tissue damage (Figure 5) (36). A recent study discovered that the phycocyanin peptide segment obtained by the complex enzyme action of phycocyanin could inhibit oxidative stress and inflammatory response induced by TGF-β1 to protect the lung (37).
Phycocyanin exhibits potential application value in multiple fields. In recent years, researchers have conducted a series of in vitro, mouse, and clinical experiments (Table 3). However, the formulation and dosage of its compatibility therapy still need to be improved based on clinical research. As a new food resource, fresh Spirulina can furthest preserve the biological activity of phycocyanin. Consuming it based on the recommended amount of the dietary nutrition tower and food standards is expected to exert a preventive effect to a certain degree.
3.3 Composition and nutrition values of Spirulina cell wall
The cell wall of Spirulina belongs to a type of plant cell wall, and it is a kind of crude fiber. Simultaneously, it has the characteristics of the gram-negative bacterial cell wall. It is mostly composed of peptidoglycan and contains pectin, mucopolysaccharides, cell wall acid, diaminohexanoic acid, and cellulose (38, 39). Polysaccharides in the cell wall of Spirulina (such as peptidoglycans and hemicellulose) can be considered prebiotics. After resisting digestion by stomach acid and trypsin, they reach the large intestine and provide nutrients for the growth of probiotics. These probiotics maintain a balance of intestinal microflora, enhance the immune system, mitigate intestinal inflammation, and promote food digestion (40, 41). Moreover, cell wall polysaccharides possess the characteristics of dietary fiber, which can scale up fecal volume, improve bowel movements, and alleviate constipation problems. Additionally, dietary fiber is conducive to controlling blood sugar and cholesterol levels. The cell wall of Spirulina is composed of some trace elements, such as calcium, magnesium, and zinc. These elements are released by the cell wall in the intestine and absorbed by the human body (42).
3.4 Glucosylglycerol and its health effects
Discovered in Japanese traditional fermented food (sake), glucosylglycerol (GG) is a type of glycoside compound formed by the reaction and linking between glycerol molecules and glucose molecules through glycosidase. GG has been reported to have various health benefits, such as treating allergic respiratory diseases, protecting conjunctiva, lowering blood sugar, and curbing visceral fat accumulation (43, 44). Luo et al. cultivated Spirulina under high salt stress, obtained Spirulina containing GG, and recovered part of phycocyanin after extracting GG, making full use of raw materials (43, 45). Fresh Spirulina cells can be cultured under high osmotic pressure and stored after concentration. Mixing Spirulina with water at a specific ratio and allowing it to settle for a while can result in an extracted liquid rich in GG for consumption. This process expands the application prospects of fresh Spirulina (Table 4).
4 Application of fresh Spirulina in food processing
Fresh Spirulina is extensively applied in various types of food processing. It lends nutritional value and special taste to food. Adding fresh Spirulina to noodles and bread can elevate their protein content, increase nutritional sources for vegetarians, and give cooked wheaten food a distinctive color and taste (46). Fresh Spirulina can also be added to cookies and cakes, endowing these desserts with a natural green color. For various sports, hypoglycemic, and flavored drinks, fresh Spirulina can incorporate nutritional value into these functional products and provide additional vitamins and minerals (42). Spirulina tea is a combination of Spirulina and tea and has both the unique nutritional value of Spirulina and the fresh taste of tea, making it a healthy and delicious beverage (47). Moreover, fresh Spirulina can be added to ice creams, fruit juices, and seasonings, rendering them special colors and flavors to meet consumers’ needs.
4.1 Spirulina pasta
Spirulina has a high content of proteins, vitamins, and minerals. The addition of Spirulina can significantly improve the nutritional value of pasta, which is especially essential for vegetarians and those who need to increase their protein intake. Adding Spirulina can alter the structure and characteristics of food (48). For example, increasing proteins and polysaccharides can advance the elasticity and taste of pasta, making it softer and more elastic. Furthermore, Spirulina can augment the viscosity of noodles and improve the extensibility of dough, contributing to the operability and processing performance during pasta making.
In the manufacturing process of microalgae noodles, Spirulina is usually mixed with flour to make the dough, which is then compressed, cut, or rolled to form the shape of the noodles. To ensure the texture, taste, and nutritional balance of the noodles, the addition (dry basis) is normally between 0.1 and 1.0% of the flour (49, 50).
Bread is a most common cereal staple in Europe and America. Adding fresh Spirulina to bread can enhance the content of vitamins, minerals, and other nutrients. Moreover, algae play a water retention role in bread, which can postpone the starch aging of bread and extend its shelf life. In the production of microalgae bread, the fermentation process and baking temperature are crucial to retain the nutritional components of Spirulina without altering the taste and flavor of the bread. Table 5 and show the raw materials, formula, and end product of a kind of microalgae bread developed by the Gansu Microalgae Technology Innovation Center.
4.2 Spirulina beverage
Fresh Spirulina is widely adopted in microalgae nutritional and healthy beverages, especially in sports, hypoglycemic, and flavored drinks. However, fresh Spirulina has a grassy smell, which may be unacceptable for some consumers (51, 52). Some measures can be adopted to mask or mitigate this smell to improve the taste and acceptance of products, for example, using natural spices, such as vanilla, lemon, orange peel, and mint. They can augment the complexity of flavored beverages, making them tastier. Meanwhile, Spirulina can be combined with flavor components, such as fruit juices, plant berries, and nut sauces, to cover the grassy smell and heighten flavor diversity. In addition, the addition of Spirulina should be adjusted to moderate the taste of the product and assuage the perception of the grassy smell.
4.3 Other food applications
Spirulina dairy products are crafted by incorporating fresh, deodorized Spirulina into the dairy manufacturing process at a precise ratio. To illustrate, fresh Spirulina is enriched with yeast and a carbon source (glucose), undergoing fermentation to eliminate any odor, followed by pH adjustment. This process yields a blue treatment liquid abundant in phycocyanin. In formulating regular milk, 25% of this vibrant blue liquid is seamlessly integrated. With the addition of protein stabilizers and subsequent pasteurization, the Spirulina milk is born. A probiotic Spirulina yogurt, rich in carotenoids, is prepared using fresh Spirulina, devoid of any food additives such as stabilizers or acidity regulators (53, 54). Remarkably, the fresh Spirulina exhibited accelerated growth of L. acidophilus during fermentation and sustained its viability even during storage. These methods serve as a versatile foundation for the formulation of a multitude of innovative products.
5 Conclusion
Fresh Spirulina is a novel product form distinct from dried Spirulina powder, offering notable advantages in terms of nutritional composition and content, as well as functional effects. Compared to natural cow’s milk, fresh Spirulina contains a more comprehensive amino acid profile, elevating its significance as a valuable source of protein. Additionally, its low-fat and lactose-desensitizing properties broaden the potential applications of fresh Spirulina. The functional components of fresh Spirulina, represented by phycocyanin, functional crude fiber, and glucosylglycerol, exhibit promising efficacy in improving immunity and antioxidant capacity and maintaining intestinal health, garnering considerable attention and acclaim from scientists and international organizations worldwide. Consequently, fresh Spirulina is increasingly incorporated into diverse food production endeavors. In order to fully leverage the nutritional advantages of fresh Spirulina, expanding theoretical and applied research is essential in areas such as production reactors, cold sterilization, preservation process, and flavor blending.
Author contributions
GL: Conceptualization, Formal analysis, Funding acquisition, Methodology, Supervision, Visualization, Writing – original draft, Writing – review & editing. HL: Formal analysis, Software, Writing – original draft. SY: Data curation, Investigation, Writing – original draft. ZS: Conceptualization, Data curation, Investigation, Supervision, Writing – original draft, Writing – review & editing. LS: Writing – original draft, Writing – review & editing. LW: Resources, Writing – original draft.
Funding
The author(s) declare that financial support was received for the research, authorship, and/or publication of this article. This work was supported in part by grants from Gansu Province Higher Education Institutions Industrial Support Project (2020C-25), and Gansu Province Science and Technology Program Project ([2021] No. 12).
Conflict of interest
The authors declare that the research was conducted in the absence of any commercial or financial relationships that could be construed as a potential conflict of interest.
Publisher’s note
All claims expressed in this article are solely those of the authors and do not necessarily represent those of their affiliated organizations, or those of the publisher, the editors and the reviewers. Any product that may be evaluated in this article, or claim that may be made by its manufacturer, is not guaranteed or endorsed by the publisher.
References
1. Soni, RA, Sudhakar, K, and Rana, RS. Spirulina-from growth to nutritional product: a review. Trends Food Sci Technol. (2017) 69:157–71. doi: 10.1016/j.tifs.2017.09.010
3. Afroz, S, and Singh, R. Cultivation of super food–Spirulina (blue-green algae): an agribusiness outlook. J Food Sci Rep. (2021) 2:34–40.
4. Lafarga, T, Sánchez-Zurano, A, Villaró, S, Morillas-España, A, and Acién, G. Industrial production of Spirulina as a protein source for bioactive peptide generation. Trends Food Sci Technol. (2021) 116:176–85. doi: 10.1016/j.tifs.2021.07.018
5. Lafarga, T, Fernández-Sevilla, JM, González-López, C, and Acién-Fernández, FG. Spirulina for the food and functional food industries. Food Res Int. (2020) 137:109356. doi: 10.1016/j.foodres.2020.109356
6. Silva, NC, Graton, IS, Duarte, CR, and Barrozo, MAS. Effects of infrared and microwave radiation on the bioactive compounds of microalga Spirulina platensis after continuous and intermittent drying. Molecules. (2023) 28:5963. doi: 10.3390/molecules28165963
7. Silva, JPS, Veloso, CRR, de Souza Barrozo, MA, and Vieira, LGM. Indirect solar drying of Spirulina platensis and the effect of operating conditions on product quality. Algal Res. (2021) 60:102521. doi: 10.1016/j.algal.2021.102521
8. Agustini, TW, Suzery, M, Sutrisnanto, D, Ma’ruf, WF, and Hadiyanto,. Comparative study of bioactive substances extracted from fresh and dried Spirulina sp. Procedia Environ Sci. (2015) 23:282–9. doi: 10.1016/j.proenv.2015.01.042
9. Bumandalai, O, Bayliss, KL, and Moheimani, NR. Innovative processes for combating contaminants in fresh Spirulina. Algal Res. (2024) 78:103397. doi: 10.1016/j.algal.2024.103397
10. Zhang, S, Chen, F, Pang, H, Gao, Y, Wen, Y, and Wang, G. Observation of Spirulina platensis cultivation in a prototype household bubble column photobioreactor during 107 days. Biotechnol Biotechnol Equip. (2021) 35:1669–79. doi: 10.1080/13102818.2021.2003246
11. Akao, PK, Cohen-Yaniv, V, Peretz, R, Kinel-Tahan, Y, Yehoshua, Y, and Mamane, H. Effect of ozonation on Spirulina platensis filaments by dynamic imaging particle analysis. Biomass Bioenergy. (2019) 127:105247. doi: 10.1016/j.biombioe.2019.05.016
12. Lu, Y-M, Xiang, W-Z, and Wen, Y-H. Spirulina (Arthrospira) industry in Inner Mongolia of China: current status and prospects. J Appl Phycol. (2011) 23:265–9. doi: 10.1007/s10811-010-9552-4
13. Assunção, J, and Malcata, FX. Enclosed “non-conventional” photobioreactors for microalga production: a review. Algal Res. (2020) 52:102107. doi: 10.1016/j.algal.2020.102107
14. Wagner, I, Steinweg, C, and Posten, C. Mono- and dichromatic LED illumination leads to enhanced growth and energy conversion for high-efficiency cultivation of microalgae for application in space. Biotechnol J. (2016) 11:1060–71. doi: 10.1002/biot.201500357
15. Relitti, F, Ogrinc, N, Giani, M, Cerino, F, Smodlaka Tankovic, M, Baricevic, A, et al. Stable carbon isotopes of phytoplankton as a tool to monitor anthropogenic CO2 submarine leakages. Water. (2020) 12:3573. doi: 10.3390/w12123573
16. Molina, D, de Carvalho, JC, Júnior, AIM, Faulds, C, Bertrand, E, and Soccol, CR. Biological contamination and its chemical control in microalgal mass cultures. Appl Microbiol Biotechnol. (2019) 103:9345–58. doi: 10.1007/s00253-019-10193-7
17. Molina-Grima, E, García-Camacho, F, Acién-Fernández, FG, Sánchez-Mirón, A, Plouviez, M, Shene, C, et al. Pathogens and predators impacting commercial production of microalgae and cyanobacteria. Biotechnol Adv. (2022) 55:107884. doi: 10.1016/j.biotechadv.2021.107884
18. Ma, Z, Ahmed, F, Yuan, B, and Zhang, W. Fresh living Arthrospira as dietary supplements: current status and challenges. Trends Food Sci Technol. (2019) 88:439–44. doi: 10.1016/j.tifs.2019.04.010
19. Hart, A, Anumudu, C, Onyeaka, H, and Miri, T. Application of supercritical fluid carbon dioxide in improving food shelf-life and safety by inactivating spores: a review. J Food Sci Technol. (2022) 59:417–28. doi: 10.1007/s13197-021-05022-7
20. Fernandes, FAN, and Rodrigues, S. Cold plasma processing on fruits and fruit juices: a review on the effects of plasma on nutritional quality. Processes (2021) 9:2098. doi: 10.3390/pr9122098
21. López-Rodríguez, A, Mayorga, J, Flaig, D, Fuentes, G, Cotabarren, J, Obregón, WD, et al. Comparison of two strains of the edible cyanobacteria Arthrospira: biochemical characterization and antioxidant properties. Food Biosci. (2021) 42:101144. doi: 10.1016/j.fbio.2021.101144
22. Stunda-Zujeva, A, Berele, M, Lece, A, and Šķesters, A. Comparison of antioxidant activity in various Spirulina containing products and factors affecting it. Sci Rep. (2023) 13:4529. doi: 10.1038/s41598-023-31732-3
23. Chen, C, Tang, T, Shi, Q, Zhou, Z, and Fan, J. The potential and challenge of microalgae as promising future food sources. Trends Food Sci Technol. (2022) 126:99–112. doi: 10.1016/j.tifs.2022.06.016
24. Dıraman, H, Koru, E, and Dibeklioğlu, H. Fatty acid profile of Spirulina platensis used as a food supplement. Israeli J Aquacult Bamidgeh. (2009) 61:134–42. doi: 10.46989/001c.20548
25. Demarco, M, Moraes, JO, Ferrari, MC, Neves, FF, Laurindo, JB, and Tribuzi, G. Production of Spirulina (Arthrospira platensis) powder by innovative and traditional drying techniques. J Food Process Eng. (2022) 45:e13919. doi: 10.1111/jfpe.13919
26. Clément, G, Giddey, C, and Menzi, R. Amino acid composition and nutritive value of the alga Spirulina maxima. J Sci Food Agric. (1967) 18:497–501.
27. Atallah, AA, Morsy, OM, and Gemiel, DG. Characterization of functional low-fat yogurt enriched with whey protein concentrate, ca-caseinate and Spirulina. Int J Food Prop. (2020) 23:1678–91. doi: 10.1080/10942912.2020.1823409
28. Pagels, F, Guedes, AC, Amaro, HM, Kijjoa, A, and Vasconcelos, V. Phycobiliproteins from cyanobacteria: chemistry and biotechnological applications. Biotechnol Adv. (2019) 37:422–43. doi: 10.1016/j.biotechadv.2019.02.010
29. Khan, M, Varadharaj, S, Shobha, JC, Naidu, MU, Parinandi, NL, Kutala, VK, et al. C-phycocyanin ameliorates doxorubicin-induced oxidative stress and apoptosis in adult rat cardiomyocytes. J Cardiovasc Pharmacol. (2006) 47:9–20. doi: 10.1097/01.fjc.0000191520.48404.27
30. Li, B, Chu, X, Gao, M, and Li, W. Apoptotic mechanism of MCF-7 breast cells in vivo and in vitro induced by photodynamic therapy with C-phycocyanin. Acta Biochim Biophys Sin. (2010) 42:80–9. doi: 10.1093/abbs/gmp104
31. Pan, R, Lu, R, Zhang, Y, Zhu, M, Zhu, W, Yang, R, et al. Spirulina phycocyanin induces differential protein expression and apoptosis in SKOV-3 cells. Int J Biol Macromol. (2015) 81:951–9. doi: 10.1016/j.ijbiomac.2015.09.039
32. Liu, Q, Wang, Y, Cao, M, Pan, T, Yang, Y, Mao, H, et al. Anti-allergic activity of R-phycocyanin from Porphyra haitanensis in antigen-sensitized mice and mast cells. Int Immunopharmacol. (2015) 25:465–73. doi: 10.1016/j.intimp.2015.02.032
33. Chang, CJ, Yang, YH, Liang, YC, Chiu, CJ, Chu, KH, Chou, HN, et al. A novel phycobiliprotein alleviates allergic airway inflammation by modulating immune responses. Am J Respir Crit Care Med. (2011) 183:15–25. doi: 10.1164/rccm.201001-0009OC
34. Baht, GS, and Grol, MW. The immune system and inflammation in musculoskeletal health, aging, and disease. Front Immunol. (2023) 14:1218118. doi: 10.3389/fimmu.2023.1218118
35. Liu, R, Qin, S, and Li, W. Phycocyanin: anti-inflammatory effect and mechanism. Biomed Pharmacother. (2022) 153:113362. doi: 10.1016/j.biopha.2022.113362
36. Campbell, NK, Fitzgerald, HK, and Dunne, A. Regulation of inflammation by the antioxidant haem oxygenase 1. Nat Rev Immunol. (2021) 21:411–25. doi: 10.1038/s41577-020-00491-x
37. Xie, Y, Li, W, Zhu, L, Zhai, S, Qin, S, and Du, Z. Effects of phycocyanin in modulating the intestinal microbiota of mice. MicrobiologyOpen. (2019) 8:e00825. doi: 10.1002/mbo3.825
38. Chen, W, Xu, J, Yu, Q, Yuan, Z, Kong, X, Sun, Y, et al. Structural insights reveal the effective Spirulina platensis cell wall dissociation methods for multi-output recovery. Bioresour Technol. (2020) 300:122628. doi: 10.1016/j.biortech.2019.122628
39. Linsong, H, Wangsun, C, Xiaoying, K, Yongming, S, Gaixiu, Y, Lianhua, L, et al. A study of the solvent-free algae Cell-Wall dissociation approach for multi-output recovery. J Biobaased Mater Bioenergy. (2022) 16:452–8. doi: 10.1166/jbmb.2022.2194
40. Ma, H, Xiong, H, Zhu, X, Ji, C, Xue, J, Li, R, et al. Polysaccharide from Spirulina platensis ameliorates diphenoxylate-induced constipation symptoms in mice. Int J Biol Macromol. (2019) 133:1090–101. doi: 10.1016/j.ijbiomac.2019.04.209
41. Camacho, F, Macedo, A, and Malcata, F. Potential industrial applications and commercialization of microalgae in the functional food and feed industries: a short review. Mar Drugs. (2019) 17:312. doi: 10.3390/md17060312
42. Akbarnezhad, M, Mehrgan, MS, Kamali, A, and Baboli, M. Effects of microelements (Fe, cu, Zn) on growth and pigment contents of Arthrospira (Spirulina) platensis. Iran J Fish Sci. (2020) 19:653–68. doi: 10.22092/IJFS.2019.120614
43. Luo, Q, Duan, Y, and Lu, X. Biological sources, metabolism, and production of glucosylglycerols, a group of natural glucosides of biotechnological interest. Biotechnol Adv. (2022) 59:107964. doi: 10.1016/j.biotechadv.2022.107964
44. Takenaka, F, Uchiyama, H, and Imamura, T. Identification of α-D-Glucosylglycerol in sake. Biosci Biotechnol Biochem. (2020) 64:378–85. doi: 10.1271/bbb.64.378
45. Lu, XF, Wu, XF, and Luo, Q. Qingdao Institute of Biomass Energy and Bioprocess Technology, Chinese Academy of Sciences. A method for co-preparing algal peptides with glycerol glucoside and phycocyanin. Chinese Patent. (2023):CN202310672213.8.
46. Bazarnova, J, Nilova, L, Trukhina, E, Bernavskaya, M, Smyatskaya, Y, and Aktar, T. Use of microalgae biomass for fortification of food products from grain. Food Secur. (2023) 10:3018. doi: 10.3390/foods10123018
47. Sun, S, Zhao, Y, Wang, L, Tan, Y, Shi, Y, Sedjoah, R-CA-A, et al. Ultrasound-assisted extraction of bound phenolic compounds from the residue of Apocynum venetum tea and their antioxidant activities. Food Biosci. (2022) 47:101646. doi: 10.1016/j.fbio.2022.101646
48. Fradique, M, Batista, AP, Nunes, MC, Gouveia, L, Bandarra, NM, and Raymundo, A. Incorporation of Chlorella vulgaris and Spirulina maxima biomass in pasta products. Part 1: preparation and evaluation. J Sci Food Agric. (2010) 90:1656–64. doi: 10.1002/jsfa.3999
49. Saharan, V, and Jood, S. Effect of storage on Spirulina platensis powder supplemented breads. J Food Sci Technol. (2021) 58:978–84. doi: 10.1007/s13197-020-04612-1
50. Fradinho, P, Niccolai, A, Soares, R, Rodolfi, L, Biondi, N, Tredici, MR, et al. Effect of Arthrospira platensis (Spirulina) incorporation on the rheological and bioactive properties of gluten-free fresh pasta. Algal Res. (2020) 45:101743. doi: 10.1016/j.algal.2019.101743
51. Dan-xia, W. Study on the processing of sphauericepus gracilis Spirulina healthy beverage. Agricult Food Sci. (2004) 25:121–3.
52. Zamri, NAS, Kamaruding, NA, and Shaharuddin, S. Ameliorative effects of microencapsulated Spirulina platensis in beverage; physicochemical, simulated release, and organoleptic properties. Nutr Food Sci. (2023) 53:1279–92. doi: 10.1108/NFS-02-2023-0041
53. Patel, P, Jethani, H, Radha, C, Vijayendra, SVN, Mudliar, SN, Sarada, R, et al. Development of a carotenoid enriched probiotic yogurt from fresh biomass of Spirulina and its characterization. J Food Sci Technol. (2019) 56:3721–31. doi: 10.1007/s13197-019-03844-0
54. Garofalo, C, Norici, A, Mollo, L, Osimani, A, and Aquilanti, L. Fermentation of microalgal biomass for innovative food production. Microorganism. (2020) 10:2069. doi: 10.3390/microorganisms10102069
55. Lu, L, Li, W, Sun, C, Kang, S, Li, J, Luo, X, et al. Phycocyanin ameliorates radiation-induced acute intestinal toxicity by regulating the effect of the gut microbiota on the TLR4/Myd88/NF-κB pathway. J Parenter Enter Nutr. (2020) 44:1308–17. doi: 10.1002/jpen.1744
56. Fan, Y. The roles of CD59 gene in anti-atherosclerotic effect of C-phycocyanin. Immunol J. (2013) 24:305–9.
57. Li, B, Xu, Y-J, Chu, X-M, Gao, M-H, Wang, X-H, Nie, S-M, et al. Molecular mechanism of inhibitory effects of CD59 gene on atherosclerosis in ApoE (−/−) mice. Immunol Lett. (2013) 156:68–81. doi: 10.1016/j.imlet.2013.09.025
58. Singh, KN, Hasan, SS, Kumar, J, Raj, I, Pathan, AA, Parmar, A, et al. Crystal structure and interaction of phycocyanin with secretase: a putative therapy for Alzheimer's disease. CNS Neurol Disord Drug Targets. (2014) 13:691–8. doi: 10.2174/1871527313666140228114456
59. Pavón-Fuentes, N, Marín-Prida, J, Llópiz-Arzuaga, A, Falcón-Cama, V, Campos-Mojena, R, Cervantes-Llanos, M, et al. Phycocyanobilin reduces brain injury after endothelin-1- induced focal cerebral ischaemia. Clin Exp Pharmacol Physiol. (2020) 47:383–92. doi: 10.1111/1440-1681.13214
60. Pak, W, Takayama, F, Mine, M, Nakamoto, K, Kodo, Y, Mankura, M, et al. Anti-oxidative and anti-inflammatory effects of Spirulina on rat model of non-alcoholic steatohepatitis. J Clin Biochem Nutr. (2012) 51:227–34. doi: 10.3164/jcbn.12-18
61. Ma, P, Huang, R, Jiang, J, Ding, Y, Li, T, and Ou, Y. Potential use of C-phycocyanin in non-alcoholic fatty liver disease. Biochem Biophys Res Commun. (2020) 526:906–12.
62. Xia, D, Liu, B, Xin, W, Liu, T, Sun, J, Liu, N, et al. Protective effects of C-phycocyanin on alcohol-induced subacute liver injury in mice. J Appl Phycol. (2016) 28:765–72. doi: 10.1007/s10811-015-0677-3
63. Xie, Y, Li, W, Lu, C, Zhu, L, Qin, S, and Du, Z. The effects of phycocyanin on bleomycin-induced pulmonary fibrosis and the intestinal microbiota in C57BL/6 mice. Appl Microbiol Biotechnol. (2019) 103:8559–69. doi: 10.1007/s00253-019-10018-7
Keywords: fresh spirulina, photobioreactor, nutritional merits, food processing, phycocyanin
Citation: Luo G, Liu H, Yang S, Sun Z, Sun L and Wang L (2024) Manufacturing processes, additional nutritional value and versatile food applications of fresh microalgae Spirulina. Front. Nutr. 11:1455553. doi: 10.3389/fnut.2024.1455553
Edited by:
Jian Li, Harbin Engineering University, ChinaReviewed by:
Baosheng Ge, China University of Petroleum, ChinaSu Chern Foo, Monash University Malaysia, Malaysia
Copyright © 2024 Luo, Liu, Yang, Sun, Sun and Wang. This is an open-access article distributed under the terms of the Creative Commons Attribution License (CC BY). The use, distribution or reproduction in other forums is permitted, provided the original author(s) and the copyright owner(s) are credited and that the original publication in this journal is cited, in accordance with accepted academic practice. No use, distribution or reproduction is permitted which does not comply with these terms.
*Correspondence: Zhongliang Sun, emxzdW5AeXR1LmVkdS5jbg==