- 1Guangdong Provincial Key Laboratory of Utilization and Conservation of Food and Medicinal Resources in Northern Region, College of Biology and Agriculture, Shaoguan University, Shaoguan, China
- 2Guangdong Provincial Engineering and Technology Research Center of Special Fruit and Vegetables in Northern Region, Engineering and Technology Research Center of Shaoguan Horticulture in Shaoguan University, Shaoguan University, Shaoguan, China
- 3College of Horticulture, South China Agricultural University, Guangzhou, China
- 4Shaoguan Engineering and Technology Research Center of Leaf Mustard, Shaoguan University, Shaoguan, China
- 5Mordern Seed Industry Research Institute of Renhua Danxia, Shaoguan, China
Both selenium (Se) and anthocyanins are crucial for maintaining human health. Preharvest Se treatments could promote anthocyanin biosynthesis and augment Se levels in vegetables, helping to combat Se deficiencies in dietary intake. However, it remains unknown whether preharvest Se treatment could balance growth and anthocyanin biosynthesis in plants and what the appropriate treatment concentration is. In this study, preharvest treatments with sodium selenite at varying concentrations (0, 5, 10, and 30 mg/kg) affect the growth and nutritional quality of purple leaf mustard (Brassica juncea) with abundant anthocyanins. Lower Se concentrations (≤10 mg/kg) of preharvest treatments enhanced photosynthesis, facilitated root system development, consequently elevated the biomass. Conversely, higher Se levels (≥30 mg/kg) reduced photosynthesis and biomass. The dosage-dependent effects of Se treatments were corroborated through seedlings cultivated in hydroponic conditions. Moreover, nearly all Se treatments elevated the contents of various nutrients in leaf mustard, particularly anthocyanin and organic se. These results suggest an overall enhancement in nutritional quality of leaf mustard plants. Furthermore, the application of 10 mg/kg Se significantly enhanced the activity of phenylalanine ammonia-lyase and upregulated the expression of 12 genes pivotal for anthocyanin biosynthesis, further demonstrating the fortified effects of Se enrichment on anthocyanins in leaf mustard. Low-level Se treatments resulted in heightened antioxidant activity (APX, CAT, and POD), mitigating reactive oxygen species induced by increasing Se content in tissues. The enhanced antioxidant activities may be beneficial for the normal growth of leaf mustard under Se stress conditions. In conclusion, our study demonstrated preharvest Se treatment at 10 mg/kg could balance the growth and anthocyanin biosynthesis in purple leaf mustard. This study offers valuable insights into anthocyanin fortification through Se enrichment methods in agricultural practices, ensuring that such fortification does not compromise leafy vegetable yield.
Introduction
Selenium (Se) is a metalloid trace element that naturally occurs in nature, and is essential for maintaining human health (1). Deficiency of Se can negatively impact the human immune system, as well as increase the risk of cancers, inflammatory diseases, and neurodegenerative disorders (2, 3). Fortunately, Se deficiency in the human body can be addressed through the consumption of Se-enriched foods. Generally, organic Se species, such as Se-containing amino acids including selenocystine, Se-methylselenocysteine, and selenomethionine, have greater bioactivities and lower toxicity compared to inorganic Se for the human body (4). However, the process of enriching foods with organic Se is relatively difficult and expensive in practice. Plants have the ability to absorb both organic and inorganic Se from the soil and can convert inorganic Se to organic forms within their bodies (5). Therefore, Se-enriched agricultural products serve as an important source for individuals to fulfill their Se requirements and preserve optimal health.
However, Se deficiency in soil is a global issue (6), which poses significant challenges to agricultural productivity and human health. For instance, it has been reported that approximately 72% of the land area in China lacks Se (7). As a result, it is necessary to externally apply Se in order to enhance its availability in the soil and ultimately increase the Se levels in agricultural products. It has been reported that the application of Se in the soil has both positive and negative effects on plants. Low doses of Se treatments can enhance plant growth and resistance against various biotic and abiotic stresses (8). On the other hand, excessive accumulation of Se in plants can impede growth, lead to the generation of reactive oxygen species (ROS), damage the photosynthesis system, disrupt nutrient absorption and transport, and ultimately cause genotoxicity in plants (9). For instance, low-level (5 μM) Se treatment could promote the growth of arsenic (As)-hyperaccumulator Pteris vittata under normal and As stress conditions (10). However, high Se concentrations (≥24 μM) reduced photosynthetic efficiency and antioxidant enzyme activity, disturbed carbon and nitrogen metabolism in apple plants (11). Although the effects of Se on plant growth have been extensively documented, more investigations are needed, particularly in those characteristic vegetable species with high content of bioactive compounds such as anthocyanin, to guide production practices due to plant species diversity.
Se application not only affects the growth and development of plants, but also enhances the nutritional quality by inducing the biosynthesis of secondary metabolites (12). The contents of secondary metabolites including phenolic compounds, carotenoids, flavonoids, proteins, glycosides, glutathione and vitamins were significantly increased after Se supplementation (13–15). Among them, anthocyanins are water-soluble pigments, garnering significant attention for their numerous health benefits in agricultural products (16), especially in fresh fruit and vegetables. Previous studies have demonstrated that anthocyanins possess potent antioxidant, anti-inflammatory, anticancer, antidiabetic, antihyperlipidemic, and antiulcer properties (17–19). Moreover, they have been associated with improved cardiovascular health, enhanced immune function, and the prevention of age-related diseases (20, 21). Therefore, it can be reasonably inferred that enhancing anthocyanins could amplify the beneficial biological activities of pigmented fruit and vegetables.
Recent studies have shown that Se supplement in soils can stimulate anthocyanin biosynthesis and accumulation during grain development in several crop species (22). It has been reported that Se treatment could promote anthocyanin accumulation in radish sprouts (Raphanus sativus) by enhancing photosynthesis and sucrose transport (23). In wheat seeds, Se biofortification increased the contents of Se and anthocyanin, and genes responsible for anthocyanin biosynthesis were significantly upregulated after Se treatment (24). Pigmented wheat cultivars exhibited higher Se efficiency absorption and the same transcription factors, such as 2R-MYB and bHLH, control both the Se flow direction and anthocyanin biosynthesis (25). Notably, anthocyanins are also responsible for the vibrant colors observed in plants, which typically range from red to purple and blue (26). The anthocyanins in fruit and vegetables not only enhances consumers’ appeal but also brings significant economic benefits (23). While fruit, seeds, and flowers of plants are reproductive organs and the development does not depend the photosynthesis for energy production, anthocyanin fortification by Se treatment in these organs does not result in a significant decrease in biomass. However, excessive accumulation of anthocyanins in vegetative organs, such as leaves, can intensify leaf coloration, potentially reducing photosynthetic efficiency, and thus leading to a delayed growth (27). Hence, it is essential to investigate whether and how Se-induced anthocyanin biosynthesis in leaves impacts the growth and yield of anthocyanin-enriched vegetables.
Brassica vegetables are one of the most widely consumed horticultural crops worldwide, known for their diverse array of nutrients (28). In the past few years, our research team has successfully developed a novel purple leaf mustard (Brassica juncea) variety named “Zifei,” which has abundant anthocyanins in the leaf. Furthermore, it has been demonstrated that anthocyanins in Brassica vegetable leaf are mainly accumulated at the adaxial epidermis of leaves (29), and the accumulation of anthocyanins in leaf mustard leaves results in reduced photosynthetic efficiency and biomass (30). Therefore, it is of great interest to investigate whether preharvest Se treatment could balance growth and anthocyanin biosynthesis and what the appropriate concentration is in leafy vegetables. Therefore, the main objectives of this study are: (a) to examine the changes in phenotype of purple leaf mustard when exposed to different concentrations of Se treatments before harvest; (b) to assess the influence of Se treatment on the nutritional quality of purple leaf mustard, especially regarding anthocyanin biosynthesis. The results of this study enriched the anticipated relationships between Se biofortification and anthocyanin biosynthesis in plants.
Materials and methods
Leaf mustard cultivation and sodium selenite treatment
The seeds of leaf mustard (‘Zifei’, a novel hybrid cultivar with high anthocyanin content in leaves) were initially sown in a seedling tray containing a mixture of peat and perlite in a 1:1 ratio. After 14 days of germination, seedlings having consistent growth were transplanted into square plates measuring 8 × 8 cm (height × width). For the investigation of preharvest sodium selenite treatment effects, about 40-d-old leaf mustard plants were employed. The seedlings were irrigated with the 1‰ (m/v) Hyponex solution (Chembase, Japan) and cultivated in an artificial climate room maintained at a temperature of 25°C with a 12-h light (1.5 × 104 ± 250 lx)/dark cycle (31). Different concentrations of sodium selenite (0, 5, 10, and 30 mg/kg on a dry weight basis of substrates) were administered to the substrates 7 days before harvest. Each treatment group consisted of 48 plants, with three replications, each comprising 16 plants. Subsequently, the leaf mustards were harvested to assess the impacts of preharvest Se treatments on the growth and nutritional quality.
Analysis of biomass and root system of leaf mustard plants
The fresh and dry weight of different parts (leaf and root) of each individual plant were measured separately using an electronic scale. Prior to determining the dry weight, the seedlings were dried until a constant weight was achieved. The shoot diameter (at the junction between the leaf and root) of the leaf mustard was assessed using a Vernier caliper. The root system of the leaf mustard was scanned and analyzed using a MICROTEK root analysis scanner (MRS-9600 TFU2L, China) (32), and the data included root length, projected area of root system, and root tip number.
Measurement of photosynthetic parameters
At 7 days of Se treatment, the 3rd – 4th main stem leaves from the bottom of the plant were selected for measurements of photosynthetic parameters (Pn, net photosynthetic rate; Tr, transpiration rate; C leaf, stomatal conductance; CO2 int., intercellular CO2 concentration) and chlorophyll fluorescence parameters (Fo, Fv, Fm, Fv/Fm) using a portable photosynthesis system (Yaxin-1105, China). Before the measurements of chlorophyll fluorescence parameters, leaf mustard seedlings were held at dark environment for 20 min for dark adaptation. Measurements were taken between 9:00 and 11:30 a.m., and repeated three times for each leaf.
Relative chlorophyll contents (SPAD) in leaves was determined using a portable plant nutrient analyzer (TYS-4N, China) (33). To extract chlorophyll and carotenoid pigments, 1.0 g of fresh samples was soaked in 95% ethanol for 48 h. The mixture was then centrifuged at 11,000 × g at 4°C for 10 min. The supernatants were then analyzed at 665, 649 and 470 nm using a UV spectrophotometer (T2602, YOKE Instrument, China) to measure the amounts of chlorophylls and carotenoids (34).
Determination of leaf color and total anthocyanin content (TAC) in mustard plant leaves
The color parameters (L*, a*, b* and ΔE) on the obverse side of leaf were measured with a CR-400 Chroma meter (KONICA MINOLTA, Japan). Total anthocyanin in leaves was extracted with methanol-HCl method. In total, 1.0 g of fresh samples were soaked in 5 mL of 1% (v/v) methanol-HCl for 24 h in dark environment. The absorbance of extract was concurrently measured at 530, 620, and 650 nm. Total anthocyanin content (TAC) was quantified according to the following formula: TAC = (OD530 − OD620) − 0.1 × (OD650 − OD620) (35).
Assay of total Se contents in mustard plant leaves and roots
Total Se contents in leaves and roots of leaf mustard seedlings were determined according to the China National Standard (GB 5009.93–2017). In total, 0.5 g of dry samples were ground into powder and digested with 10 mL of mixture of nitric acid and perchloric acid (10:1). The digested solution was reconstituted to 10 mL with 100 g/L potassium ferricyanide solution. Total Se contents were determined using an atomic fluorescence spectrometer (HGF-V9, Haiguang Instrument, China) (36). The result was expressed as milligram per kilogram dry weight (mg/kg DW).
Determination of total soluble solids (TSS), glutathione (GSH), and total soluble protein (TSP) contents
For TSS determinations, 100 g of fresh samples from three independent plants were homogenized and filtered. The 0.2 mL of filtrates were used to measure TSS contents using a handheld digital refractometer (Atago, Japan). GSH contents in leaf mustard plants were determined using a test kit (Sangon Biotech, China) following the manufacturer’s instructions (37).
The TSP contents were determined with the Coomassie brilliant blue (CBB) method (38). Briefly, 1.0 g of leaf samples was homogenized into 5 mL of 0.5 mM phosphate buffer (pH 7.0). The homogenates were centrifuged at 11,000 × g at 4°C for 10 min. For the measurement of TSP content, 0.2 mL of the supernatants were mixed with 5 mL of 0.1 g/L CBB and reacted at room temperature for 15 min. The absorbance was recorded at 595 nm.
Determination of ascorbic acid, free amino acid and total phenolic contents
For this, 1.0 g of fresh samples was homogenized with 5 mL of 10 mmol/L trichloroacetic acid. The homogenates were centrifuged at 11,000 × g for 10 min and then were used to measure ascorbic acid content (AAC) according to the methods described by Tan et al. (39). Briefly, 1.0 mL of supernatants, 1 mL of 10 mmol/L trichloroacetic acid, 1 mL of ethanol, 0.5 mL of 0.4% (v/v) phosphoric acid, 1 mL of 5 g/L 4,7-Diphenyl-4,10-Phenanthroline and 0.5 mL of 0.2 mmol/L FeCl3 were mixed in a tube. The absorbance of mixtures was monitored at 543 nm.
Free amino acid (FAA) content was determined using the hydrated ninhydrin method as described by Liu et al. (11). In total, 1.0 g of leaf samples were homogenized with 5 mL of ultrapure water and subjected to a boiling water bath for 20 min. The resulting homogenates were filtered to obtain the crushed extracts for FAA analysis. The reaction system consisted of 3 mL, which included 0.5 mL of the crushed extracts, 2 mL of 5 mmol/L cyanate buffer, and 0.5 mL of 10 mmol/L ninhydrin. The mixed solutions were allowed to react at room temperature for 1 h, and the absorbance was measured at 570 nm.
Total phenolic content (TPC) was extracted and quantified using a modified version of the method previously used by Yuan et al. (40). In summary, 1.0 g of leaf samples were homogenized with 5 mL of 1% (v/v) HCl-methanol, extracting at 25°C for 2 h. The extracts were then centrifuged at 4°C at 11,000 × g for 10 min. For the analysis, 0.5 mL of the supernatants, 1.5 mL of 1 mol/L sodium carbonate, and 1.0 mL of Folin–Ciocalteu reagent were sequentially added into a new tube, mixed thoroughly and incubated at 25°C for 1 h in dark. The absorbance of the resulting solution was measured at 765 nm. TPC was calculated with a standard curve generated from varying concentrations of gallic acid.
qRT-PCR analysis
Total RNA of mustard plants was extracted using a RNAprep extraction Kit (DP441, Tiangen, China) according to the kit instructions. RNA integrity and concentration were checked using a Nano Photometer (Nano 300, ALL SHENG, China). The cDNA was synthesized using a cDNA Synthesis Kit (11123ES60, Yeasen, China) following the instructions. Quantitative real-time PCR (qRT-PCR) was performed using a real-time PCR system (CFX Opus 384, Bio-Rad, United States) and a qPCR analysis kit (11201ES03, Yeasen, China) (41). The primer sequences used for qRT-PCR analysis were listed in Supplementary Table S1.
Measurement of hydrogen peroxide and malondialdehyde content in leaf mustard plants
Hydrogen peroxide (H2O2) and alondialdehyde (MDA) in leaf mustard samples were extracted with normal saline. For the extraction of crude extracts, 1.0 g of leaf samples was homogenized with 5 mL of normal saline. The extracts were then centrifuged at 4°C at 11,000 × g for 10 min and used for H2O2 and MDA content measurements. The levels of H2O2 and MDA were quantified using commercial kits, following the instructions provided by the manufacturer (42).
Enzyme activity determination in leaf mustard plants
As for the preparation of crude enzymes, 1.0 g of samples were homogenized in 5 mL of 0.5 mM phosphate buffer (pH 7.0) containing 1% PVP. The homogenates were then centrifuged at 11,000 × g, 4°C for 15 min, and the supernatants were collected for enzyme activity measurements.
For phenylalanine ammonia-lyase (PAL) activity measurement, 0.5 mL of each solution including supernatants, 5 mmol/L dithiothreitol and 20 mmol/L L-phenylalanine were first mixed and added up to a total volume of 3 mL with 0.1 mmol/L sodium borate (pH 8.8). The mixed solutions were incubated at room temperature (25°C) for 2 h, and the absorbance of the reaction was determined at 290 nm.
The activities of peroxidase (POD) and catalase (CAT) were measured according to the methods introduced by Wang et al. (42). The POD activity was assayed by measuring the oxidation rate of guaiacol at 470 nm, while the CAT activity was determined by monitoring the decomposition rate of hydrogen peroxide at 240 nm. Additionally, the ascorbate peroxidase (APX) activity was measured using both ascorbic acid and hydrogen peroxide as substrates at 290 nm (37).
Phenotype analysis of leaf mustard seedlings under Se stress condition
To confirm the effects of sodium selenite treatments on the growth of leaf mustard, a separate experiment was conducted using seedlings as the experimental material (Supplementary Figure S1). Seedlings were planted in a 1‰ (m/v) Hyponex solution containing sodium selenite, with final concentrations of 0, 5, 10, and 30 mg/L. The seeds were sown and incubated in 15 cm diameter culture dishes for 7 days at 25°C under 12-h light/dark cycles. The germination rate (%) was evaluated 2 days after sowing, while fresh weight, hypocotyl length, and relative electrolyte leakage (REL) of the seedlings were measured after 7 days of exposure to Se stress. Each treatment group consisted of three replicates of 60 seedlings for phenotypic analysis.
To determine the REL, three seedlings from each treatment were immersed into 25 mL of distilled water at room temperature for 2 h. The electrical conductivity (EC) was measured using a handheld conductance bridge (DDB-303A, INESA, China). Total EC was measured again after boiling the tube for 30 min, and REL was expressed as a percentage of the total EC (37).
Reactive oxygen species (ROS) staining in leaf mustard seedlings under Se stress conditions
To investigate the effects of varying concentrations of Se treatments on ROS contents in seedlings, leaf mustard seeds were incubated in different concentrations of Se solutions (0, 5, 10, and 30 mg/L) for 7 days. The whole seedlings were used to stain the levels of two major ROS, H2O2 and superoxide anion (O2-.). H2O2 and O2-. in seedlings were stained with the nitro-blue tetrazolium (NBT) and 3,3’-Diaminobenzidine (DAB) reagents (43).
Statistical analysis
The experiment was conducted using a random design, and all values were reported as mean ± standard deviation (SD) with a sample size of 3 (n = 3). Statistically significant differences among treatments were compared by the student’s t-test and/or one-way ANOVA in the SPSS software (version 22.0), with a significant level set at p < 0.05. To avoid Type I errors, the Bonferroni correction was considered for conducting the significance analysis. Statistical differences were indicated using different letters.
Results
Effects of varying concentrations of Se treatments on the biomass of leaf mustard plants
As shown in Table 1, the growth of purple leaf mustard was affected by varying concentrations of sodium selenite treatments. The shoot diameters, as well as the fresh and dry weight of roots, leaves and the whole plant of leaf mustard exhibited a similar trend following the various Se treatments. As the Se concentration increased gradually, the values also increased before declining (Table 1). Treatment with 5 mg/kg Se slightly enhanced shoot diameters and the fresh and dry weights of the plant, though there was no significant difference compared to the control (0 mg/kg). On the other hand, the 10 mg/kg Se treatment led to significant increases in biomass, while the 30 mg/kg Se treatment resulted in a significant reduction in leaf mustard biomass compared to the control. These results suggest that an appropriate concentration of Se treatment can enhance the growth of leaf mustard, indicating that preharvest Se application has the potential to improve purple leaf mustard yield.

Table 1. The biomass of leaf mustard affected by different concentrations of sodium selenite preharvest treatments.
Root development plays a vital role in plant growth, prompting us to examine the effects of Se treatment on root system. As anticipated, the 10 mg/kg Se treatment notably increased root length, projected area of root system, and root tip number, whereas the 30 mg/kg Se treatment decreased these parameters compared to the control (Table 1).
Effects of varying concentrations of Se treatments on the photosynthesis of mustard plant leaves
Four major photosynthetic parameters, including net photosynthetic rate (Pn), transpiration rate (Tr), stomatal conductance (C leaf) and intercellular CO2 (CO2 int), were measured in the leaves to evaluate the effects of preharvest Se treatment on photosynthesis. The photosynthetic parameters of purple leaf mustard were influenced by varying concentrations of Se treatments. With increasing Se treatment concentration, the values of Pn, Tr, and C leaf exhibited a pattern of initially increasing and then decreasing, while the intercellular CO2 (CO2 int) showed the opposite trend (Figures 1A–D). The Se treatment at a concentration of 10 mg/kg resulted in the highest values for Pn, Tr, and C leaf, which were 111.21, 47.56, and 67.76% higher than the control, respectively. Additionally, the values of CO2 int. for the 10 mg/kg Se treatment were significantly lower than those of the control. In contrast, the Pn values for the 30 mg/kg Se treatment were significantly lower compared to the control, while the CO2 int. values for the 30 mg/kg Se treatment were significantly higher (Figures 1A–D). These results, in conjunction with other literatures, indicate that an appropriate Se treatment could enhance photosynthetic efficiency, while high concentrations of Se treatment may lead to photo inhibition in the leaves (11).
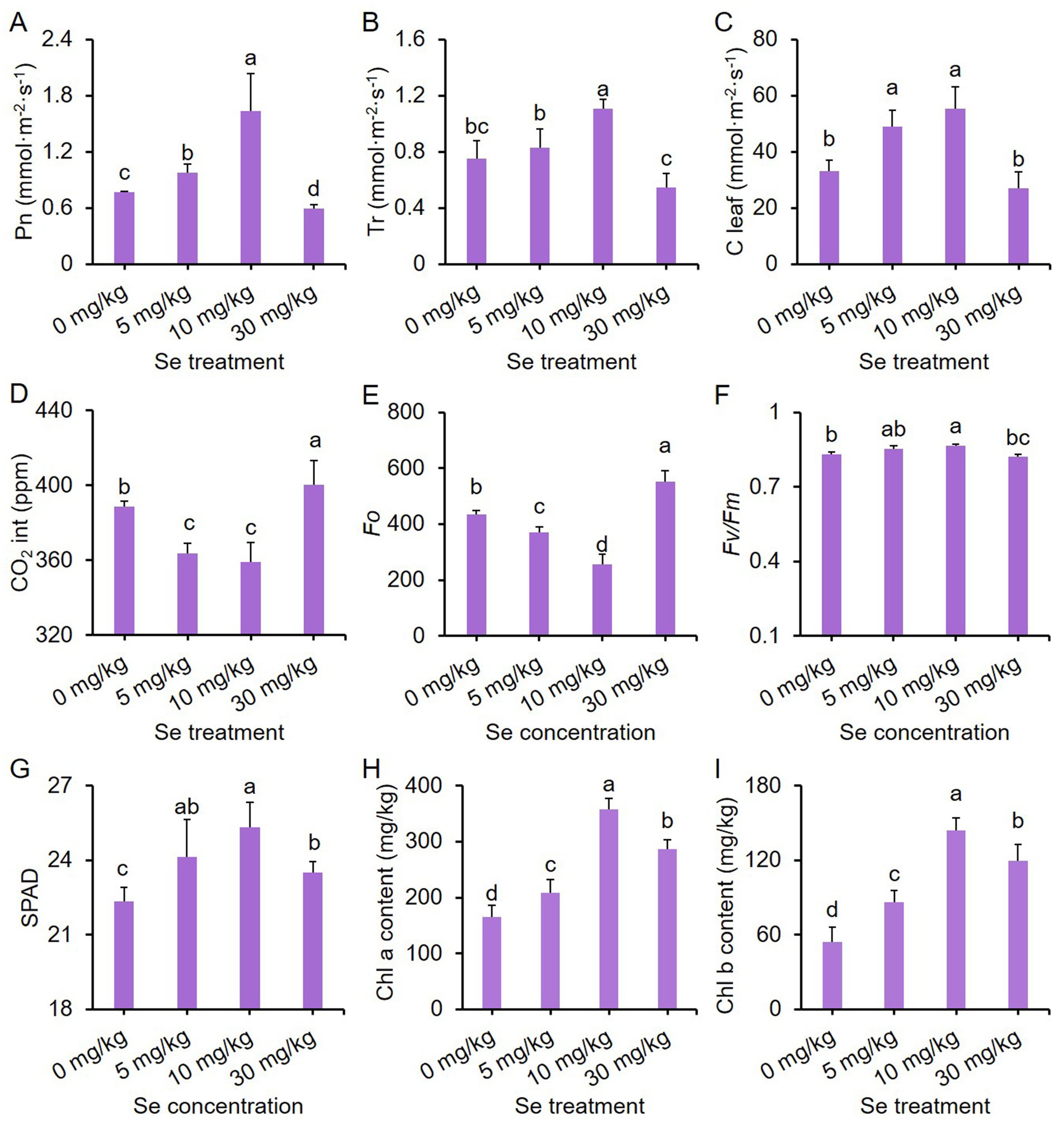
Figure 1. Effects of preharvest Se treatments with different concentrations on the photosynthesis of purple leaf mustard plant. (A) Net photosynthetic rate (Pn); (B) transpiration rate (Tr); (C) stomatal conductance (C leaf); (D) intercellular CO2 (CO2 int); (E) chlorophyll fluorescence parameter Fo; (F) chlorophyll fluorescence parameter Fv/Fm; (G) relative chlorophyll content (SPAD); (H) chlorophyll a content; (I) chlorophyll b content. Different concentrations of sodium selenite (0, 5, 10, and 30 mg/kg) were added to the soil at 7 days before harvest. The data is presented as means ± standard error from three replicates. Statistical differences (p < 0.05) are denoted by different letters above the bars.
In order to delve deeper into the impact of Se on the photosynthesis of leaf mustard, we also analyzed chlorophyll fluorescence parameters and chlorophyll pigment contents. The parameter Fo represents the minimum fluorescence yield, indicating the baseline fluorescence level when all PSII reaction centers are open. On the other hand, the parameter Fv/Fm signifies the maximum quantum efficiency of PSII, with higher values indicating enhanced photosynthetic efficiency (44). Fo values showed a trend of decreasing and then increasing with rising Se concentration, with the 10 mg/kg Se treatment displaying the lowest Fo value (Figure 1E). On the other hand, Fv/Fm exhibited a completely opposite trend to Fo under varying Se treatments (Figure 1F). Nevertheless, all Se treatments significantly boosted chlorophyll pigment contents compared to the control, including relative chlorophyll content (SPAD), chlorophyll a and chlorophyll b contents, with the Se treatment at 10 mg/kg concentration yielding the highest chlorophyll contents (Figures 1G–I). These results suggest that the influence of Se treatment on purple leaf mustard growth is possibly associated with the efficiency of light utilization, rather than a reduction in the biosynthesis of chlorophyll pigments in purple leaf mustard.
Se treatments deepen the color of purple leaf mustard plant
To investigate the impact of Se treatment on the color of purple leaf mustard plants, we examined the color of the leaf surface and the levels of chromogenic substances, including anthocyanin and carotenoids. Within color parameters, L* signifies the lightness of a color, while a* and b* gauge its position on the green-red and blue-yellow axes, respectively. In addition, ΔE denotes the overall color difference (40).
Both L* and a* values declined with increasing Se concentration, while b* and ΔE values had the opposite trend. Se treatments at concentrations higher than 5 mg/kg notably decreased L* and a* values but increased b* and ΔE values compared to the control (Figures 2A–D). In comparison to the control, all Se treatments exhibited significantly higher total anthocyanin content (TAC), with the content increasing as the Se concentration rose (Figure 2E). Furthermore, Se treatments at 10 and 30 mg/kg concentrations led to higher carotenoid content relative to the control (Figure 2F). These results collectively suggest that Se treatment may promote the accumulation of pigments, thereby intensifying the coloration.
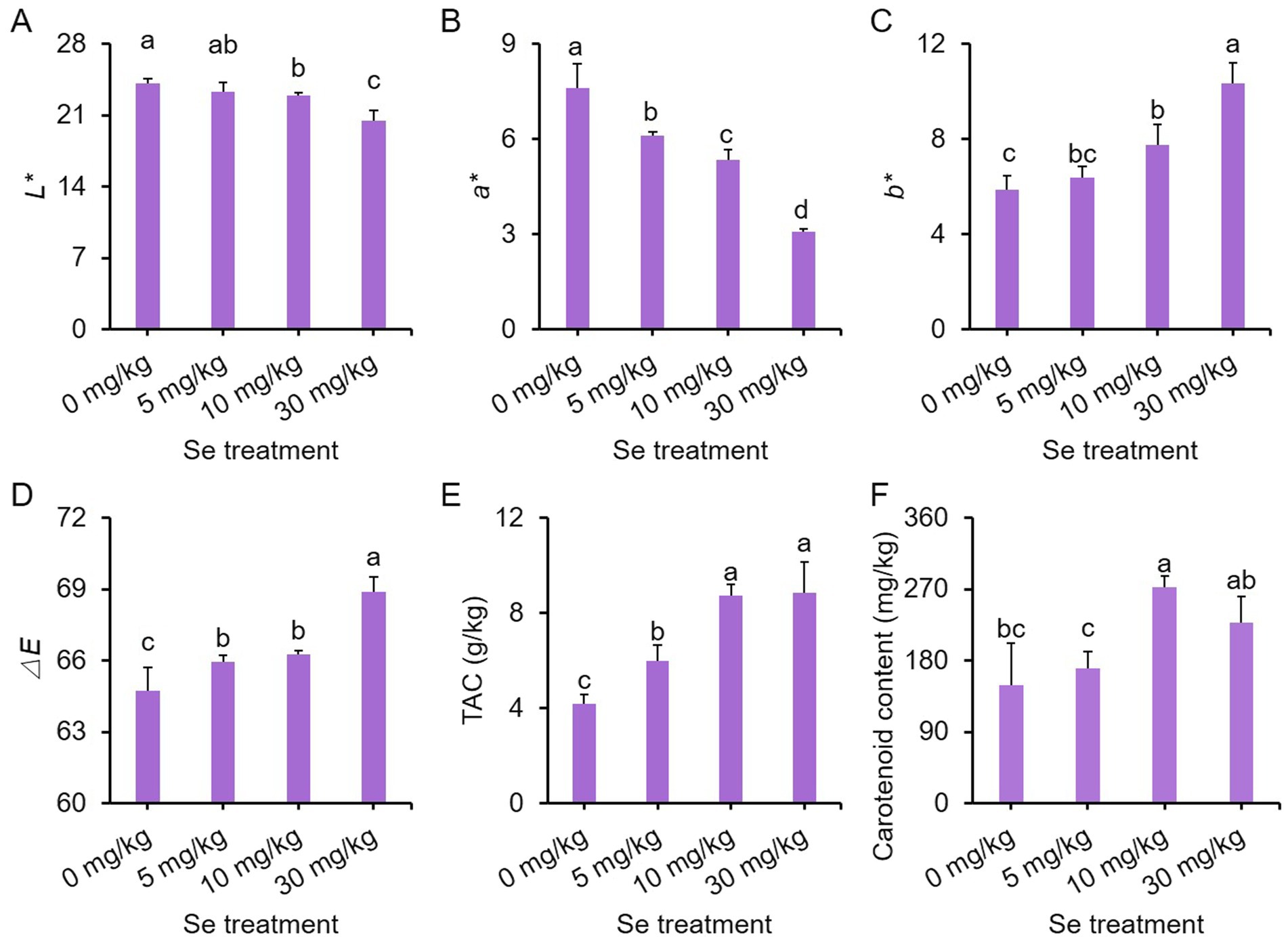
Figure 2. Effects of preharvest Se treatments with different concentrations on leaf surface color of purple leaf mustard plants. (A) L*; (B) a*; (C) b*; (D) chromatism ΔE; (E) total anthocyanin content (TAC); (F) carotenoid content. Different concentrations of sodium selenite (0, 5, 10, and 30 mg/kg) were added to the soil at 7 days before harvest. The data is presented as means ± standard error from three replicates. Statistical differences (p < 0.05) are denoted by different letters above the bars.
We also assessed the ratio of total chlorophyll to TAC to investigate whether the buildup of pigments, particularly dark anthocyanin, impacts the photosynthesis of the leaf mustard plants. All Se treatments enhanced total chlorophyll contents, with the 10 mg/kg Se treatment displaying the highest content (Supplementary Figure S2A). However, only the 10 mg/kg Se treatment showed a higher ratio of total chlorophyll to TAC compared to the other Se treatments and the control (Supplementary Figure S2B). These results imply that the heightened pigment levels may indeed influence photosynthesis.
Se treatments improve the nutritional quality of leaf mustard plant
To assess the ability of purple leaf mustard to enrich Se, total Se levels in both leaves and roots were determined. Total Se contents in both leaves and roots increased with the concentration of Se treatment, with roots accumulating more Se (Figures 3A–C), indicating a high enrichment efficiency of purple leaf mustard for Se.
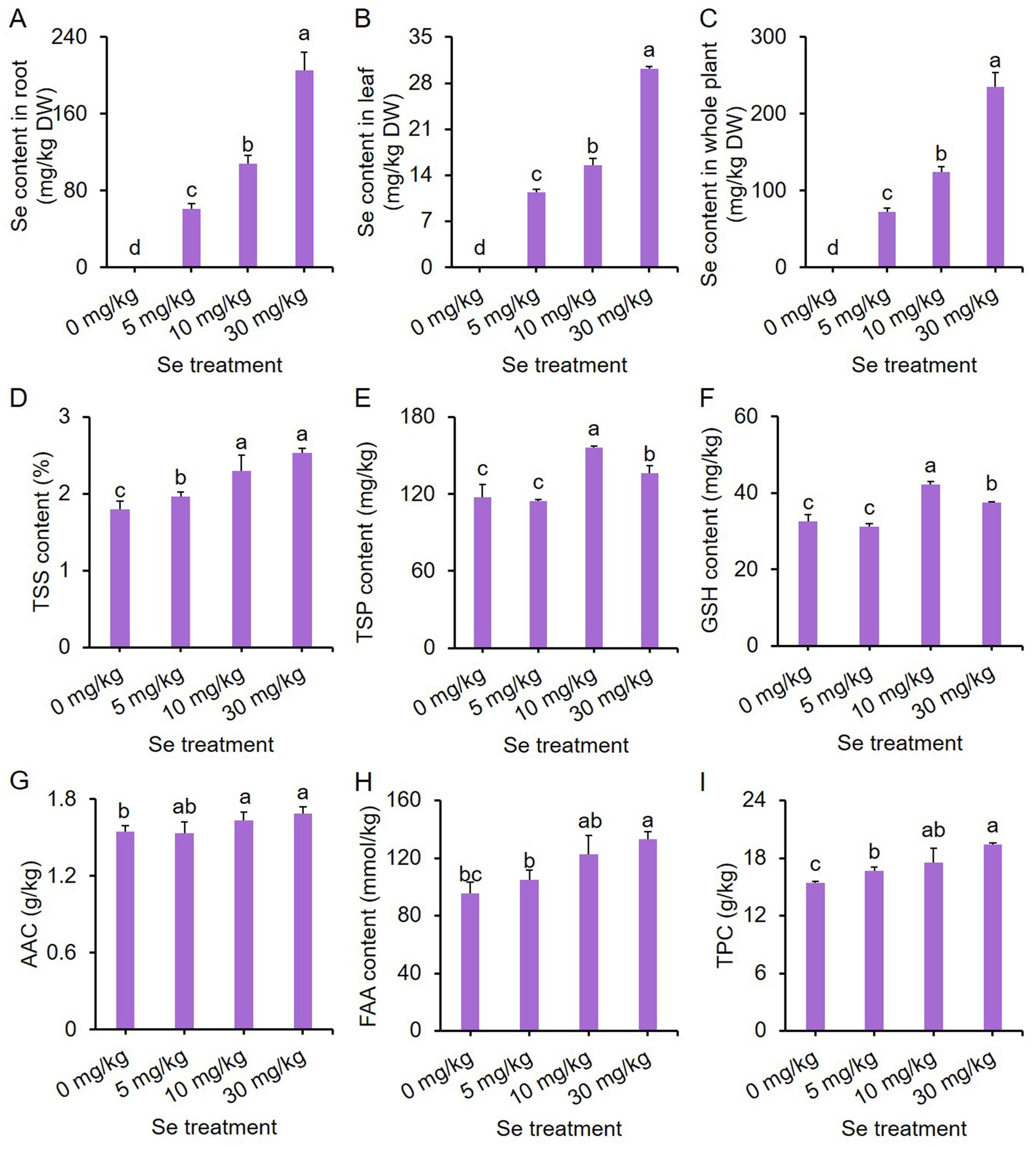
Figure 3. Effects of preharvest Se treatments with different concentrations on nutritional quality of purple leaf mustard plant. (A) Total selenium (Se) content in root; (B) total Se content in leaf; (C) total Se content in the whole plant; (D) total soluble solid (TSS) content; (E) total soluble protein (TSP) content; (F) glutathione (GSH) content; (G) ascorbic acid content (AAC); (H) free amino acid (FAA) content; (I) total phenolic content (TPC). Different concentrations of sodium selenite (0, 5, 10, and 30 mg/kg) were added to the soil at 7 days before harvest. The data is presented as means ± standard error from three replicates. Statistical differences (p < 0.05) are denoted by different letters above the bars.
Furthermore, the total soluble solid (TSS) content increased with increasing Se treatment concentrations, with all Se treatments showing higher TSS content compared to the control (Figure 3D). Different Se treatment intensities elicited similar trends in total soluble protein (TSP) and glutathione (GSH) contents (Figures 3E,F). Both the 10 and 30 mg/kg Se treatments resulted in significantly higher TSP and GSH contents, with the 10 mg/kg Se treatment exhibiting the highest content.
For ascorbic acid content (AAC) and free amino acid (FAA) content, only the 10 mg/kg and 30 mg/kg Se treatments significantly enhanced AAC and FAA content as compared to the control (Figures 3G,H). In contrast, all Se treatments resulted in a significantly higher total phenolic content (TPC) than the control (Figure 3I). These results clearly suggest that preharvest Se treatment could improve the nutritional quality of leaf mustard.
Se treatment upregulates the expression of key genes in anthocyanin biosynthesis pathway
Anthocyanin is synthesized through the phenylpropanoid pathway (45). As Se treatments intensified the purple color and enhanced total anthocyanin content (TAC) in purple leaf mustard, we investigated the impact of Se treatment on protein activity and gene expression of key enzymes responsible for anthocyanin biosynthesis. A simplified pathway for anthocyanin biosynthesis in plants is illustrated in Figure 4A.
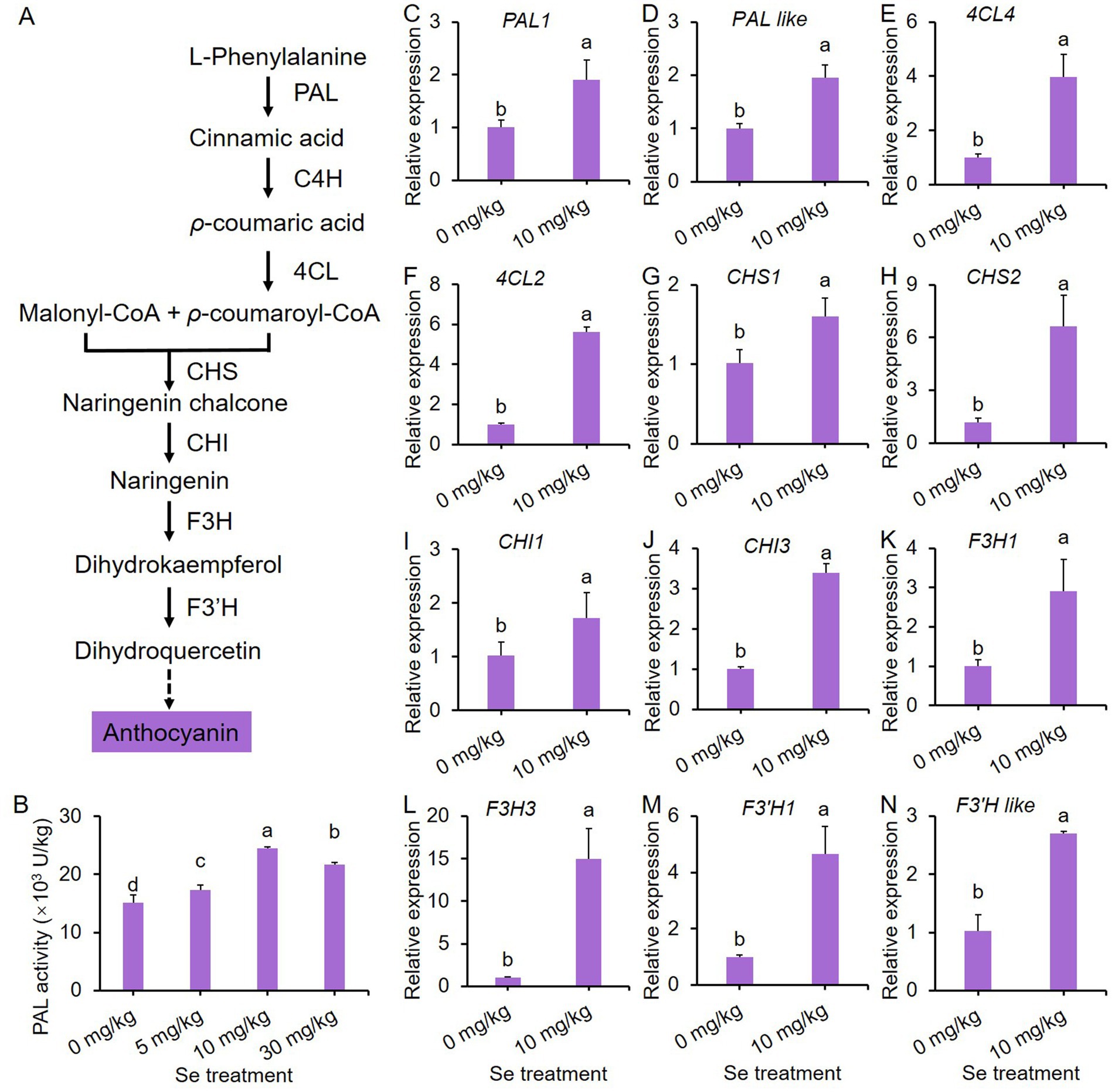
Figure 4. 10 mg/kg Se treatment induces the biosynthesis of anthocyanin through the phenylpropanoid pathway in purple leaf mustard plant. (A) A simplified pathway for anthocyanin biosynthesis; (B) phenylalanine ammonia-lyase (PAL) activity; (C–N) the gene expression profiles of key enzymes responsible for anthocyanin biosynthesis in the phenylpropanoid pathway. Different concentrations of sodium selenite (0, 5, 10, and 30 mg/kg) were added to the soil at 7 days before harvest. The data is presented as means ± standard error from three replicates. Statistical differences (p < 0.05) are denoted by different letters above the bars.
In Figure 4B, it is evident that Se treatments increased PAL activity, which is the initial enzyme in the anthocyanin biosynthesis pathway, compared to the control. Furthermore, the Se treatment at 10 mg/kg exhibited the highest PAL activity. Consequently, this concentration of Se treatment was chosen as a representative to study the effects of Se treatments on gene expression in the phenylpropanoid pathway.
A total of 14 representative genes were selected to analyze their expression profiles. As depicted in Figures 4C–N, the expression of almost all genes was significantly up-regulated by Se treatment, with the exception of two C4H genes (Supplementary Figure S3). These results show that Se treatment stimulates anthocyanin biosynthesis by inducing the gene expression of key enzymes in the phenylpropanoid pathway.
Effects of varying concentrations of Se treatments on ROS metabolism in leaf mustard plants
Antioxidant enzymes are crucial for supporting plant growth under abiotic stress and play essential roles in maintaining a balance between the production and breakdown of reactive oxygen species (ROS) (46). As such, we conducted an investigation to examine the impact of Se treatment on ROS metabolism in leaf mustard plants.
The levels of hydrogen peroxide (H2O2) and malondialdehyde (MDA) increased with the concentration of Se treatment. All Se treatments showed significantly higher H2O2 contents compared to the control (Figure 5A), while only the 10 and 30 mg/kg Se treatments resulted in elevated MDA contents (Figure 5B). These results suggest that Se treatment induces oxidative stress in purple leaf mustard.
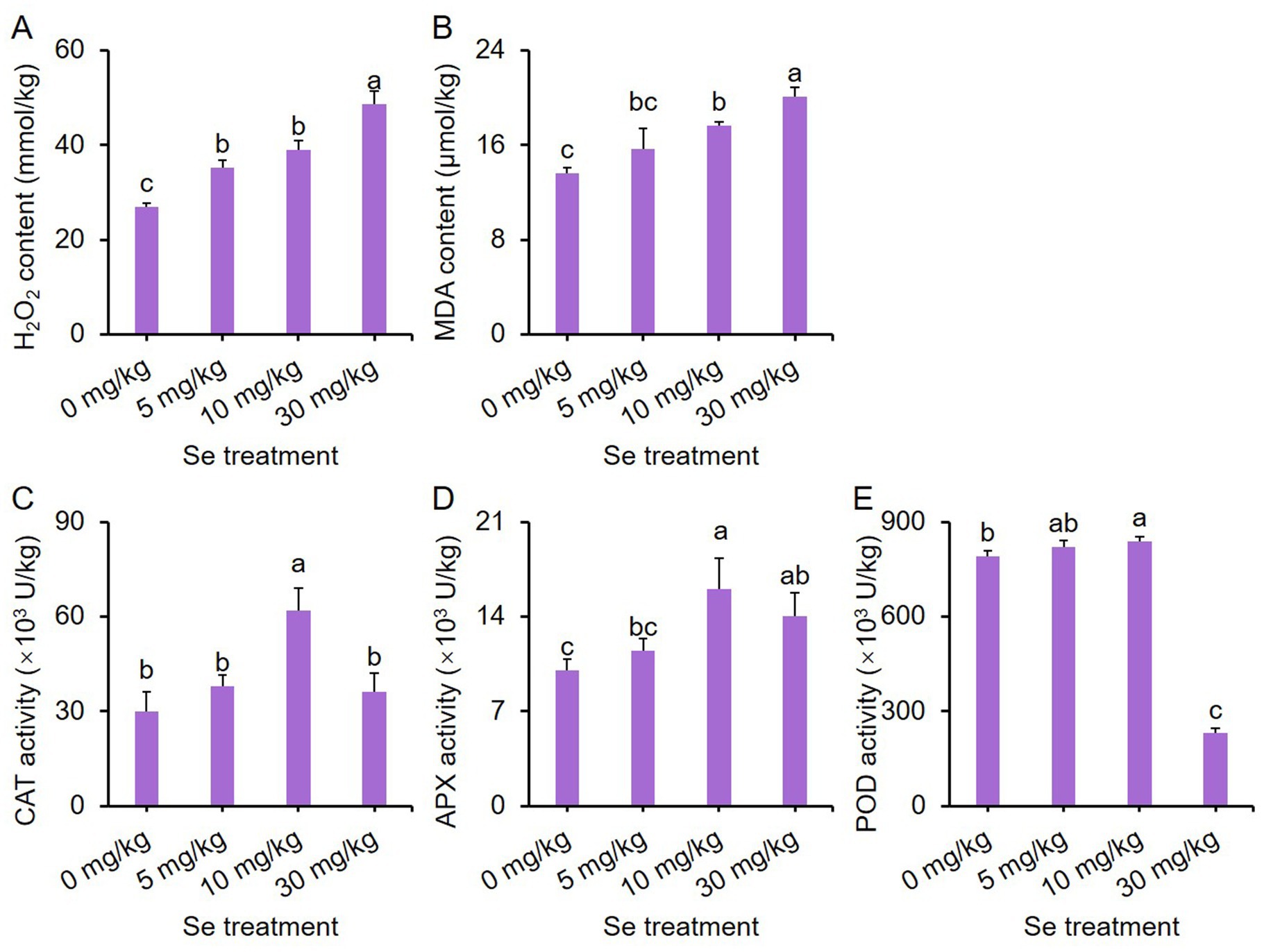
Figure 5. Effects of preharvest Se treatments with different concentrations on reactive oxygen species (ROS) metabolism in purple leaf mustard plants. (A) Hydrogen peroxide (H2O2) content; (B) malondialdehyde (MDA) content; (C) catalase (CAT) activity; (D) ascorbate peroxidase (APX) activity; (E) peroxidase (POD) activity. Different concentrations of sodium selenite (0, 5, 10, and 30 mg/kg) were added to the soil 7 days before harvest. The data is presented as means ± standard error from three replicates. Statistical differences (p < 0.05) are denoted by different letters above the bars.
In terms of catalase (CAT) activity, only the 10 mg/kg Se treatment led to enhanced activity (Figure 5C). The ascorbate peroxidase (APX) activity for both the 10 and 30 mg/kg Se treatments was notably higher than that of the other treatments, with the 10 mg/kg Se treatment displaying the highest APX activity (Figure 5D). Additionally, the peroxidase (POD) activity was highest with the 10 mg/kg Se treatment and lowest with the 30 mg/kg Se treatment (Figure 5E). These results indicate that Se treatment can influence the activity of antioxidant enzymes.
Effects of varying concentrations of Se treatments on the growth performance of leaf mustard seedlings
The application manner of Se may affect plant growth. The addition of optimal concentration of Se element in soil has been shown to improve the growth and nutritional quality of leaf mustard plants in this study. To assess whether Se treatment yields consistent effects on leaf mustard under different cultivation methods, an additional experiment was conducted using seedlings in a hydroponic system.
The growth performance of seedlings subjected to different intensities of Se stress treatments (final concentrations of Se at 0, 5, 10, and 30 mg/L) is depicted in Supplementary Figure S4, revealing that the 30 mg/L Se treatment led to noticeably smaller biomass for the seedlings. Se concentrations below 30 mg/L did not hinder seed germination rates, whereas the 30 mg/L Se treatment significantly reduced germination rates (Figure 6A).
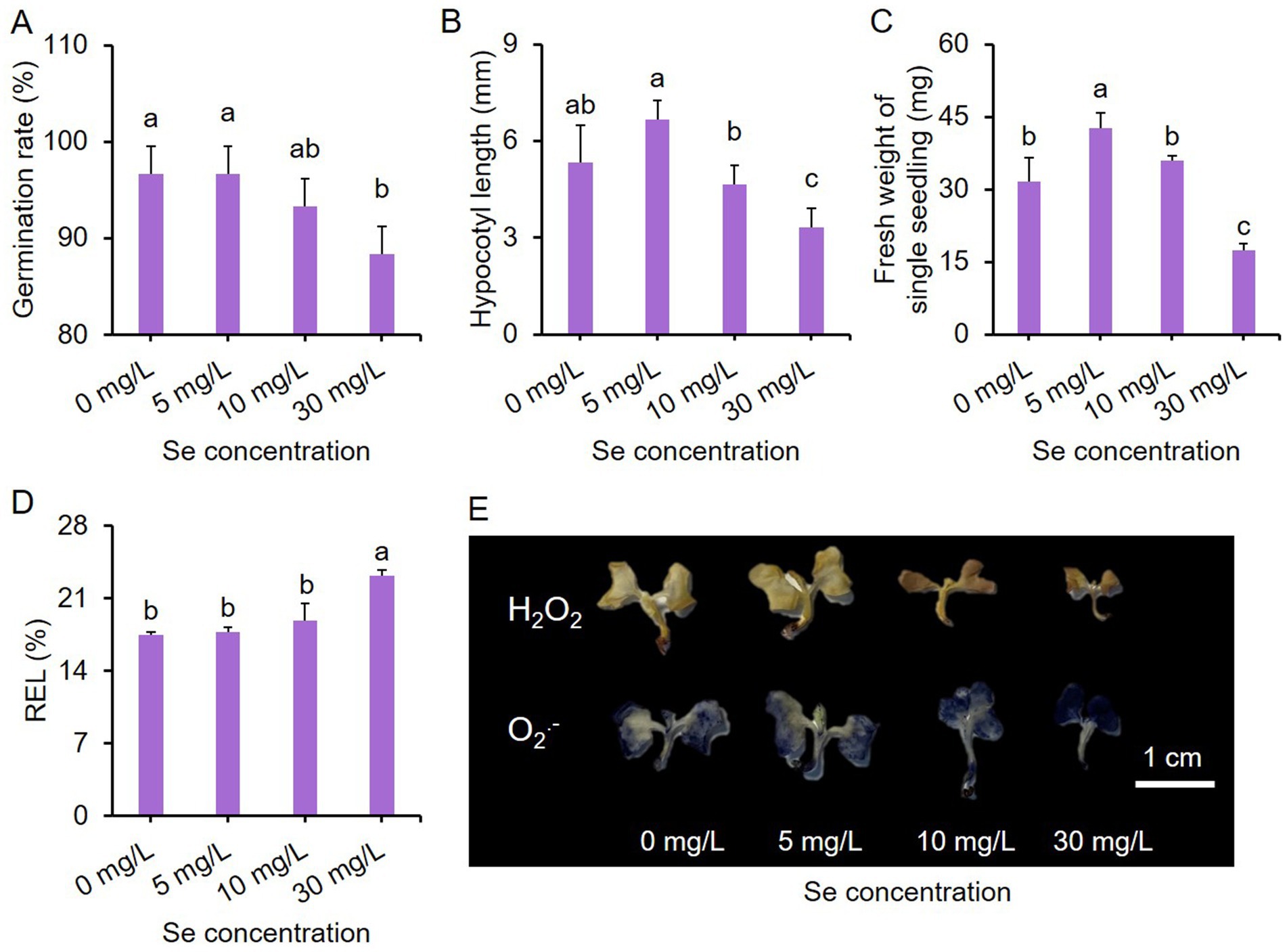
Figure 6. Effects of Se stress on the phenotype of purple leaf mustard young seedlings. (A) Germination rate of seeds under Se stress conditions; (B) hypocotyl length of seedlings; (C) fresh weight of single seedling; (D) relative electrolyte leakage (REL) of seedlings under Se stress conditions; (E) hydrogen peroxide (H2O2) and superoxide anion (O2-.) staining in seedlings. Seedlings were incubated in different concentrations of sodium selenite solutions (0, 5, 10, and 30 mg/L) for 7 days. The data is presented as means ± standard error from three replicates. Statistical differences (p < 0.05) are denoted by different letters above the bars.
The impact of different concentrations of Se treatments on hypocotyl length (Figure 6B) and fresh weight of single seedling (Figure 6C) showed similar trends overall. In comparison to the control, the 5 mg/L Se treatment enhanced hypocotyl length and fresh weight, while the 30 mg/L Se treatment decreased them. The hypocotyl length and fresh weight of the 10 mg/L Se treatment were comparable to those of the control. Notably, only the 30 mg/L Se treatment resulted in a significantly higher relative electrolyte leakage (REL) compared to the other treatments (Figure 6D).
H2O2 and O2.- are two primary ROS in plants during abiotic stress (47). We also analyzed the accumulation of H2O2 and O2.- in seedlings exposed to Se stress conditions. Low concentrations of Se treatments led to a slight increase in the levels of these two ROS, while the 30 mg/L Se treatment resulted in higher ROS accumulation (shown by a darker color) compared to the control group (Figure 6E). These results together suggest that the developmental stage of the plant may also influence Se sensitivity, with young seedlings being more sensitive to Se stress. Further investigations are required to compare Se enrichment efficacy of distinct application methods.
Discussion and conclusion
Both Se and anthocyanins play essential roles in promoting human health (48, 49). For instance, Se exhibits a broad spectrum of pleiotropic effects, including antioxidant and anti-inflammatory properties, as well as its role in the production of active thyroid hormones (50). Similarly, anthocyanins possess potent oxygen radical scavenging abilities and are known to contribute to the delay of aging in humans (19). Plants have the ability to absorb Se from the soil, making the cultivation of vegetables in Se-rich soils a viable and safe strategy for biofortifying the nutritional quality of food with this essential nutrient. In this study, sodium selenite was applied to the roots of purple leaf mustard, leading to a significant increase in Se enrichment in tissues, and Se contents increased with increasing treatment concentrations (Figures 3A–C). More importantly, Se treatment markedly boosted the anthocyanin content in leaf mustard, resulting in a deepening color on the leaf surface (Figure 2). These results suggest that preharvest Se treatment not only enhances the levels of Se and anthocyanins in vegetable tissues, but also improves the visual appeal of leaf mustard leaves, making them more attractive to consumers. Anthocyanins are primarily produced through the phenylpropanoid pathway, with their biosynthesis relying on the activity and gene expression of several key enzymes such as PAL (51). A widely targeted metabolome analysis revealed that preharvest application of Se significantly enhanced the contents of several phenolic acids, and meanwhile upregulated the expression of key genes in the phenylpropanoid pathway such as C4H, COMT, CHS, and FLS (36). In this study, Se treatment enhanced PAL activity and notably upregulated the expression of 12 genes (including PALs, 4CLs, CHSs, CHIs, F3Hs and F3’H3) responsible for anthocyanin biosynthesis in leaf mustard (Figure 4), demonstrating positive roles of Se treatment on anthocyanin biosynthesis at gene level. These results further suggest that Se treatment promotes anthocyanin biosynthesis by directly activating the expression of genes responsible for the process.
The TSS, GSH, ascorbic acid, FAA, phenolics and carotenoids are essential nutrients that offer significant health benefits (52, 53). In this study, Se treatments were found to increase the levels of TSS, GSH, ascorbic acid, FAA, total phenolic compounds and soluble proteins in purple leaf mustard (Figures 2, 3), indicating that, with the exception of Se and anthocyanins, preharvest Se treatment also positively influenced the levels of other nutrients. These results align with similar results observed in pepper fruit (54) and radish sprouts (23). Besides their nutritional benefits, TSS, FAA and soluble proteins serve as crucial osmotic substances in plants (55), while GSH and ascorbic acid functions as important non-enzymatic antioxidants (56). It could be reasonably speculated that the evaluated these substances can help protect leaf mustard from oxidative damage induced by Se stress.
Although Se is not considered essential for plants, it is recognized as a beneficial element that can promote plant growth (57). However, many studies have shown that the beneficial effects of Se application are constrained by its concentration. For instance, in apple plants, lower Se treatments (6, 9, and 12 μM) have been shown to enhance plant growth, whereas higher concentrations of Se (>12 μM) inhibit growth, particularly in root development (11). In our current study, lower Se concentrations, particularly at 10 mg/kg or 5 mg/L, significantly enhanced the growth of leaf mustard plants and seedlings. However, higher Se concentrations (30 mg/kg or 30 mg/L) markedly hindered plant (Table 1) and seedling growth (Figure 6). Similar results have been observed in cabbage, another leafy vegetable of the Brassica family (15). These results suggest that the impact of Se preharvest treatment on the growth of leaf mustard is dependent on the treated concentration, with only an appropriate concentration leading to growth promotion. Furthermore, many factors, including growth conditions, influence the growth and Se absorption in plants (58). In this study, leaf mustard seedlings were grown in a growth container with strictly controlled relative humidity, light, and temperature. Further investigations are necessary to clarify how and whether growth conditions affect the growth and Se absorption in purple leaf mustard under Se stress conditions.
Plant growth and development under abiotic stress conditions are closely associated with the antioxidant system (59). In Se-enriched plants, Se could induce cellular ROS and thus influence cell death via the apoptosis mechanism at toxic concentrations (6). In this study, a high Se concentration (30 mg/kg or 30 mg/L) resulted in significantly higher H2O2 and MDA contents (Figure 5), as well as REL and O2.- (Figure 6), indicating that a high Se concentration triggered oxidative damages on mustard tissues. This was attributed to the failure of ROS scavenging, as the 30 mg/kg Se treatment significantly reduced the activities of several antioxidant enzymes including APX, CAT and POD (Figure 5). Of greater importance, the 10 mg/kg Se treatment simultaneously enhanced antioxidant enzyme activity but had lower H2O2 and MDA contents compared to the high Se concentration, and promoted growth. This suggests that the enhanced antioxidant activity could promptly scavenge ROS, thereby avoiding Se-triggered oxidative damage and guaranteeing normal growth. This further highlights the importance of the antioxidant system for leaf mustard under Se stress. These results are consistent with results observed in different plant species, such as cabbage (60) and mushroom (61), further demonstrating the dual effects of Se treatment on antioxidant enzymes. In addition to triggering oxidative stress, H2O2 also serves as a crucial signaling molecule in plant stress responses and has the potential to stimulate antioxidant activity through feedback mechanisms (62, 63). The elevated levels of H2O2 observed in low concentrations of Se treatments (Figures 5A, 6E) may play a role in inducing antioxidant activity.
In addition to the antioxidant system, plant biomass primarily relies on photosynthesis (64). Previous studies have shown that Se treatment has dual effects on plant photosynthesis (23). In purple leaf mustard here, low levels of Se treatments enhanced photosynthetic parameters, increased chlorophyll pigment content, and improved chlorophyll fluorescence (Figure 1). A significant linear correlation between Se content and photosynthesis rate, as well as chlorophyll fluorescence, was observed in rice following Se treatment (65). It was observed that the 30 mg/kg Se treatment did not reduce total chlorophyll content or chlorophyll fluorescence (Fv/Fm) compared to the control (Figure 1). These results suggest that Se application can enhance the rate of photosynthesis in purple leaf mustard by promoting chlorophyll production, resulting in increased photosynthetic activity. However, the 30 mg/kg Se treatment led to a decrease in biomass compared to the control group (Table 1). Additionally, only the 10 mg/kg Se treatment enhanced the ratio of total chlorophyll and anthocyanin content, while the 30 mg/kg Se treatment significantly reduced this ratio compared to the control (Supplementary Figure S2). Since anthocyanins are primarily accumulated in the epidermal cells of Brassica vegetable leaves (29), and high Se concentrations also increased anthocyanin content (Figure 2E), the ratio of chlorophyll to anthocyanin in leaves appears to affect photosynthetic efficiency. This may explain why high concentration of Se treatment reduced the photosynthesis rate without reducing chlorophyll content.
In conclusion, preharvest Se treatment with varying concentrations had distinct effects on the growth and nutritional quality of leaf mustard. Treatment at 10 mg/kg balanced the growth and anthocyanin biosynthesis, suggesting the application of Se preharvest at a proper concentration might be a promising method for anthocyanin fortification in fresh food products. Based on the results and discussion presented above, we proposed a model to suggest how suitable concentration of Se preharvest treatment balances the growth and the nutritional quality of purple leaf mustard (Figure 7). A 10 mg/kg Se treatment boosted growth by improving photosynthetic efficiency and increased the contents of key nutrients, particularly anthocyanins, by upregulating the expression of key genes responsive for their biosynthesis. Moreover, Se treatment enhanced antioxidant activities, which might play a crucial role in protecting plants from oxidative stress and improving plant health and quality. Overall, our study is the first time for studying the roles of Se in balancing the growth and anthocyanin biosynthesis in plants.
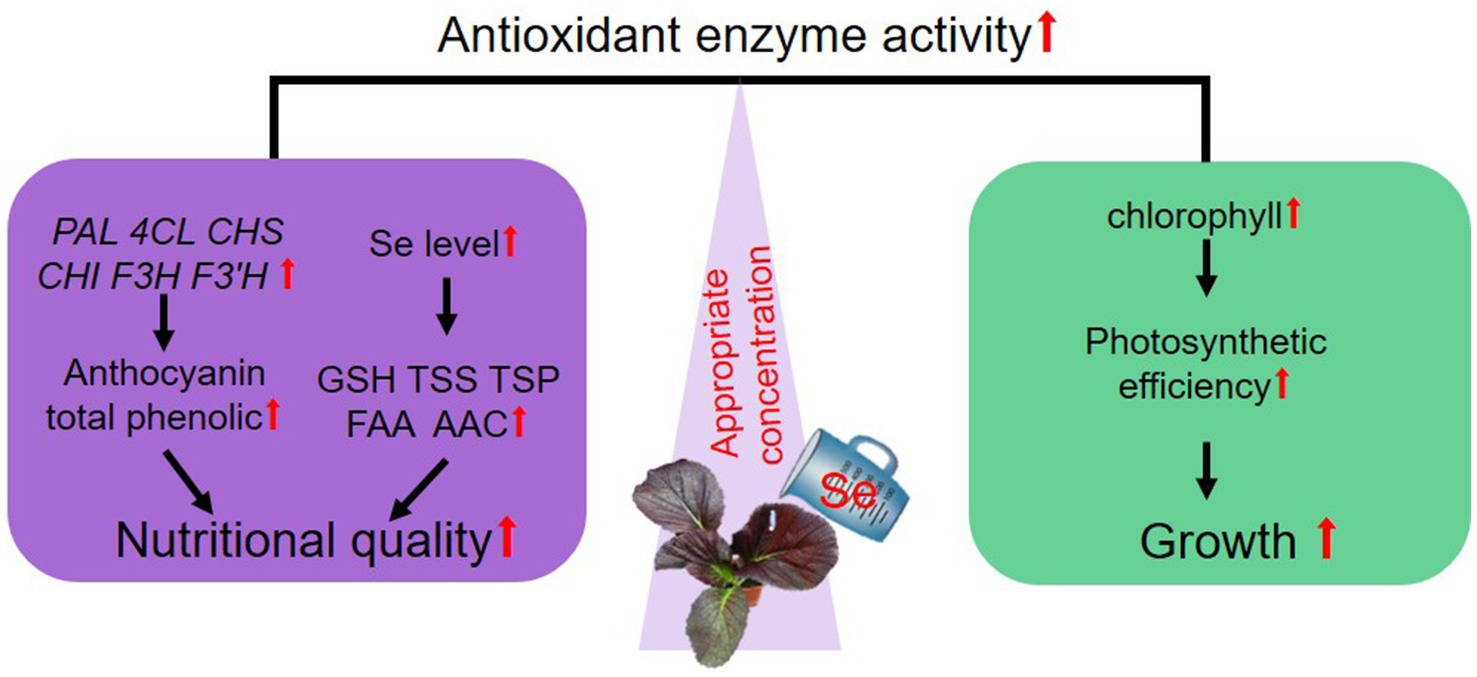
Figure 7. A proposed model illustrating how suitable Se treatment balances the growth and nutritional quality of purple leaf mustard. “↑” denotes a reinforcement or heightened impact by Se treatment.
Data availability statement
The original contributions presented in the study are included in the article/Supplementary material, further inquiries can be directed to the corresponding authors.
Author contributions
BW: Conceptualization, Writing – review & editing. XY: Data curation, Investigation, Methodology, Writing – original draft. GW: Writing – original draft. Y-nZ: Writing – original draft. R-cZ: Writing – original draft. H-mF: Writing – review & editing. H-bL: Writing – review & editing.
Funding
The author(s) declare that financial support was received for the research, authorship, and/or publication of this article. This study was financially supported by the Shaoguan Science and Technology Plan Project (No. 220725116272795), Strategic Special Funds for Guangdong Rural Revitalization (No. 210903164532210) and Key Research Platform and Project of Guangdong Province of Educational Department (No. 2024GCZX006).
Conflict of interest
The authors declare that the research was conducted in the absence of any commercial or financial relationships that could be construed as a potential conflict of interest.
Publisher’s note
All claims expressed in this article are solely those of the authors and do not necessarily represent those of their affiliated organizations, or those of the publisher, the editors and the reviewers. Any product that may be evaluated in this article, or claim that may be made by its manufacturer, is not guaranteed or endorsed by the publisher.
Supplementary material
The Supplementary material for this article can be found online at: https://www.frontiersin.org/articles/10.3389/fnut.2024.1447084/full#supplementary-material
References
1. Kieliszek, M, and Serrano Sandoval, SN. The importance of selenium in food enrichment processes. A comprehensive review. J Trace Elem Med Biol. (2023) 79:127260. doi: 10.1016/j.jtemb.2023.127260
2. Avery, JC, and Hoffmann, PR. Selenium, selenoproteins, and immunity. Nutrients. (2018) 10:1203. doi: 10.3390/nu10091203
3. Huang, Z, Rose, AH, and Hoffmann, PR. The role of selenium in inflammation and immunity: from molecular mechanisms to therapeutic opportunities. Antioxid Redox Signal. (2012) 16:705–43. doi: 10.1089/ars.2011.4145
4. Gandin, V, Khalkar, P, Braude, J, and Fernandes, AP. Organic selenium compounds as potential chemotherapeutic agents for improved cancer treatment. Free Radic Biol Med. (2018) 127:80–97. doi: 10.1016/j.freeradbiomed.2018.05.001
5. Wang, Z, Huang, W, and Pang, F. Selenium in soil-plant-microbe: a review. Bull Environ Contam Toxicol. (2022) 108:167–81. doi: 10.1007/s00128-021-03386-2
6. Tangjaidee, P, Swedlund, P, Xiang, J, Yin, H, and Quek, SY. Selenium-enriched plant foods: selenium accumulation, speciation, and health functionality. Front Nutr. (2023) 9:962312. doi: 10.3389/fnut.2022.962312
7. Zhou, XB, Li, YY, and Lai, F. Effects of different water management on absorption and accumulation of selenium in rice. Saudi J Biol Sci. (2018) 25:1178–82. doi: 10.1016/j.sjbs.2017.10.017
8. Rizwan, M, Ali, S, Zia-Ur-Rehman, M, Rinklebe, J, Tsang, DCW, Tack, FMG, et al. Effects of selenium on the uptake of toxic trace elements by crop plants: a review. Crit Rev Env Sci Tec. (2020) 51:2531–66. doi: 10.1080/10643389.2020.1796566
9. Natasha, SM, Niazi, NK, Khalid, S, Murtaza, B, Bibi, I, and Rashid, MI. A critical review of selenium biogeochemical behavior in soil-plant system with an inference to human health. Environ Pollut. (2018) 234:915–34. doi: 10.1016/j.envpol.2017.12.019
10. Dai, ZH, Ding, S, Chen, JY, Han, R, Cao, Y, Liu, X, et al. Selenate increased plant growth and arsenic uptake in as-hyperaccumulator Pteris vittata via glutathione-enhanced arsenic reduction and translocation. J Hazard Mater. (2022) 424:127581. doi: 10.1016/j.jhazmat.2021.127581
11. Liu, CL, Zhou, GJ, Qin, HH, Guan, YF, Wang, TY, Ni, W, et al. Metabolomics combined with physiology and transcriptomics reveal key metabolic pathway responses in apple plants exposure to different selenium concentrations. J Hazard Mater. (2024) 464:132953. doi: 10.1016/j.jhazmat.2023.132953
12. Du, B, Luo, HW, He, LX, Zhang, LH, Liu, YF, Mo, ZW, et al. Rice seed priming with sodium selenate: effects on germination, seedling growth, and biochemical attributes. Sci Rep. (2019) 9:4311. doi: 10.1038/s41598-019-40849-3
13. Rao, S, Gou, Y, Yu, T, Cong, X, Gui, J, Zhu, Z, et al. Effects of selenate on se, flavonoid, and glucosinolate in broccoli florets by combined transcriptome and metabolome analyses. Food Res Int. (2021) 146:110463. doi: 10.1016/j.foodres.2021.110463
14. Wang, HJ, Chen, Y, Wang, ZL, Yuan, YH, and Yue, TL. Integrated analysis of transcriptome, proteome and non-targeted metabolome for exploring the mechanism of selenium biotransformation in Pichia kudriavzevii. Food Biosci. (2024) 61:104958. doi: 10.1016/j.fbio.2024.104958
15. Yang, XY, Liao, XL, Yu, L, Rao, S, Chen, QW, Zhu, ZZ, et al. Combined metabolome and transcriptome analysis reveal the mechanism of selenate influence on the growth and quality of cabbage (Brassica oleracea var. capitata L.). Food Res Int. (2022) 156:111135. doi: 10.1016/j.foodres.2022.111135
16. Fang, J . Bioavailability of anthocyanins. Drug Metab Rev. (2014) 46:508–20. doi: 10.3109/03602532.2014.978080
17. Bowen-Forbes, CS, Zhang, Y, and Nair, MG. Anthocyanin content, antioxidant, anti-inflammatory and anticancer properties of blackberry and raspberry fruits. J Food Compos. (2010) 23:554–60. doi: 10.1016/j.jfca.2009.08.012
18. Putta, S, Yarla, NS, Peluso, I, Tiwari, DK, Reddy, GV, Giri, PV, et al. Anthocyanins: multi-target agents for prevention and therapy of chronic diseases. Curr Pharm Des. (2017) 23:6321–46. doi: 10.2174/1381612823666170519151801
19. Wang, H, Cao, G, and Prior, RL. Oxygen radical absorbing capacity of anthocyanins. J Agric Food Chem. (1997) 45:304–9. doi: 10.1021/jf960421t
20. He, JR, Ye, SX, Correia, P, Fernandes, I, Zhang, R, Wu, MC, et al. Dietary polyglycosylated anthocyanins, the smart option? A comprehensive review on their health benefits and technological applications. Compr Rev Food Sci Food Saf. (2022) 21:3096–128. doi: 10.1111/1541-4337.12970
21. Kalt, W, Cassidy, A, Howard, LR, Krikorian, R, Stull, AJ, Tremblay, F, et al. Recent research on the health benefits of blueberries and their anthocyanins. Adv Nutr. (2020) 11:224–36. doi: 10.1093/advances/nmz065
22. Zhang, FJ, Li, XY, Wu, QQ, Lu, P, Kang, QF, Zhao, MY, et al. Selenium application enhances the accumulation of flavones and anthocyanins in bread wheat (Triticum aestivum L.) grains. J Agric Food Chem. (2022) 70:13431–44. doi: 10.1021/acs.jafc.2c04868
23. Chen, JH, Chen, H, Wang, HX, Zhan, JY, Yuan, XX, Cui, J, et al. Selenium treatment promotes anthocyanin accumulation in radish sprouts (Raphanus sativus L.) by its regulation of photosynthesis and sucrose transport. Food Res Int. (2023) 165:112551. doi: 10.1016/j.foodres.2023.112551
24. Xia, Q, Shui, Y, Zhi, H, Ali, A, Yang, ZP, and Gao, Z. Exogeneous selenium enhances anthocyanin synthesis during grain development of colored-grain wheat. Plant Physiol Biochem. (2023) 200:107742. doi: 10.1016/j.plaphy.2023.107742
25. Pu, Z, Wei, G, Liu, Z, Chen, L, Guo, H, Li, Y, et al. Selenium and anthocyanins share the same transcription factors R2R3MYB and bHLH in wheat. Food Chem. (2021) 356:129699. doi: 10.1016/j.foodchem.2021.129699
26. Piccolo, EL, Landi, M, Massai, R, Remorini, D, and Guidi, L. Girled-induced anthocyanin accumulation in red-leafed Prunus cerasifera: effect on photosynthesis, photoprotection and sugar metabolism. Plant Sci. (2020) 294:110456. doi: 10.1016/j.plantsci.2020.110456
27. Hughes, NM, Neufeld, HS, and Burkey, KO. Functional role of anthocyanins in high-light winter leaves of the evergreen herb Galax urceolata. New Phytol. (2005) 168:575–87. doi: 10.1111/j.1469-8137.2005.01546.x
28. Kapusta-Duch, J, Kopeć, A, Piatkowska, E, Borczak, B, and Leszczyńska, T. The beneficial effects of Brassica vegetables on human health. Rocz Panstw Zakl Hig. (2012) 63:389–95.
29. Li, HB, Du, Y, Zhang, JK, Feng, HM, Liu, JG, Yang, GL, et al. Unraveling the mechanism of purple leaf formation in brassica napus by integrated metabolome and transcriptome analyses. Front Plant Sci. (2022) 13:945553. doi: 10.3389/fpls.2022.945553
30. Di, H, Zhao, Y, Zhou, A, Chen, Z, Ma, J, Liu, D, et al. Integrated metabolome and transcriptome analysis revealed color formation in purple leaf mustard (Brassica juncea). Sci Hortic. (2024) 337:113526. doi: 10.1016/j.scienta.2024.113526
31. Peng, MM, Chen, ZS, Zhang, L, Wang, YJ, Zhu, SJ, and Wang, G. Preharvest application of sodium nitroprusside alleviates yellowing of Chinese flowering cabbage via modulating chlorophyll metabolism and suppressing ROS accumulation. J Agric Food Chem. (2023) 71:9280–90. doi: 10.1021/acs.jafc.3c00630
32. Wang, B, Lin, LN, Yuan, X, Zhu, YN, Wang, YK, Li, DL, et al. Low-level cadmium exposure induced hormesis in peppermint young plant by constantly activating antioxidant activity based on physiological and transcriptomic analyses. Front Plant Sci. (2023) 14:1088285. doi: 10.3389/fpls.2023.1088285
33. Wang, B, Wang, YK, Yuan, X, Jiang, YY, Zhu, YN, Kang, XM, et al. Comparative transcriptomic analysis provides key genetic resources in clove basil (Ocimum gratissimum) under cadmium stress. Front Genet. (2023) 14:1224140. doi: 10.3389/fgene.2023.1224140
34. Lichtenthaler, HK . Chlorophylls and carotenoids: pigments of photosynthetic biomembranes. Meth Enzym. (1987) 148:350–82. doi: 10.1016/0076-6879(87)48036-1
35. An, JP, Liu, X, Li, HH, You, CX, Wang, XF, and Hao, YJ. Apple RING E3 ligase MdMIEL1 inhibits anthocyanin accumulation by ubiquitinating and degrading MdMYB1 protein. Plant Cell Physiol. (2017) 58:1953–62. doi: 10.1093/pcp/pcx129
36. Chen, ZS, Zhang, L, Peng, MM, Zhu, SJ, and Wang, G. Preharvest application of selenite enhances the quality of Chinese flowering cabbage during storage via regulating the ascorbate-glutathione cycle and phenylpropanoid metabolisms. Food Res Int. (2023) 163:112229. doi: 10.1016/j.foodres.2022.112229
37. Wang, B, and Zhu, SJ. Pre-storage cold acclimation maintained quality of cold-stored cucumber through differentially and orderly activating ROS scavengers. Postharvest Biol Tec. (2017) 129:1–8. doi: 10.1016/j.postharvbio.2017.03.001
38. Snyder, JC, and Desborough, SL. Rapid estimation of potato tuber total protein content with coomassie brilliant blue G-250. Theor Appl Genet. (1978) 52:135–9. doi: 10.1007/BF00264747
39. Tan, XL, Zhao, YT, Shan, W, Kuang, JF, Lu, WJ, Su, XG, et al. Melatonin delays leaf senescence of postharvest Chinese flowering cabbage through ROS homeostasis. Food Res Int. (2020) 138:109790. doi: 10.1016/j.foodres.2020.109790
40. Yuan, X, Tang, BL, Wang, YK, Jiang, YY, He, JM, Wang, G, et al. Inhibitory effects of peppermint extracts on the browning of cold-stored fresh-cut taro and the phenolic compounds in extracts. Front Sustain Food Syst. (2023) 7:1191396. doi: 10.3389/fsufs.2023.1191396
41. Wang, YK, Ye, H, Lin, W, Wang, G, Luo, T, He, JM, et al. Cinnamic acid application inhibits the browning of cold-stored taro slices by maintaining membrane function, reducing flavonoid biosynthesis and enhancing glutathione metabolism. Postharvest Biol. Tec. (2024) 218:113180. doi: 10.1016/j.postharvbio.2024.113180
42. Wang, B, Huang, YY, Zhang, ZM, Xiao, YH, and Xie, J. Ferulic acid treatment maintains the quality of fresh-cut taro (Colocasia esculenta) during cold storage. Front Nut. (2022) 9:884844. doi: 10.3389/fnut.2022.884844
43. Wang, B, Wang, G, and Zhu, SJ. DNA damage inducible protein 1 is involved in cold adaption of harvested cucumber fruit. Front Plant Sci. (2020) 10:1723. doi: 10.3389/fpls.2019.01723
44. Banks, JM . Continuous excitation chlorophyll fluorescence parameters: a review for practitioners. Tree Physiol. (2017) 37:1128–36. doi: 10.1093/treephys/tpx059
45. Sunil, L, and Shetty, NP. Biosynthesis and regulation of anthocyanin pathway genes. Appl Microbiol Biotechnol. (2022) 106:1783–98. doi: 10.1007/s00253-022-11835-z
46. Nadarajah, KK . ROS homeostasis in abiotic stress tolerance in plants. Int J Mol Sci. (2020) 21:5208. doi: 10.3390/ijms21155208
47. Mittler, R, Zandalinas, SI, Fichman, Y, and Van, BF. Reactive oxygen species signalling in plant stress responses. Nat Rev Mol Cell Biol. (2022) 23:663–79. doi: 10.1038/s41580-022-00499-2
48. Qu, SS, Wang, G, Li, MM, Yu, WT, and Zhu, SJ. LcNAC90 transcription factor regulates biosynthesis of anthocyanin in harvested litchi in response to ABA and GA3. Postharvest Biol. Tec. (2022) 194:112109. doi: 10.1016/j.postharvbio.2022.112109
49. Zhu, YG, Pilon-Smits, EA, Zhao, FJ, Williams, PN, and Meharg, AA. Selenium in higher plants: understanding mechanisms for biofortification and phytoremediation. Trends Plant Sci. (2009) 14:436–42. doi: 10.1016/j.tplants.2009.06.006
50. Rayman, MP . Selenium and human health. Lancet. (2012) 379:1256–68. doi: 10.1016/S0140-6736(11)61452-9
51. McInnis, S, Clemens, S, and Kermode, AR. The ornamental variety, Japanese striped corn, contains high anthocyanin levels and PAL specific activity: establishing the potential for development of an oral therapeutic. Plant Cell Rep. (2009) 28:503–15. doi: 10.1007/s00299-008-0650-6
52. Langi, P, Kiokias, S, Varzakas, T, and Proestos, C. Carotenoids: from plants to food and feed industries. Methods Mol Biol. (2018) 1852:57–71. doi: 10.1007/978-1-4939-8742-9_3
53. Wu, J, Qu, LB, Li, ZH, Zhao, LP, Sun, YQ, and Yang, R. Light-responsive benzobisthiazole as oxidase mimic for rapid determination of glutathione in food and vegetable. Food Chem. (2023) 427:136672. doi: 10.1016/j.foodchem.2023.136672
54. Li, D, Zhou, CR, Zhang, JB, An, QS, Wu, YL, Li, JQ, et al. Nanoselenium foliar applications enhance the nutrient quality of pepper by activating the capsaicinoid synthetic pathway. J Agric Food Chem. (2020) 68:9888–95. doi: 10.1021/acs.jafc.0c03044
55. Luo, H, Riu, M, Ryu, CM, and Yu, JM. Volatile organic compounds emitted by Burkholderia pyrrocinia CNUC9 trigger induced systemic salt tolerance in Arabidopsis thaliana. Front Microbiol. (2022) 13:1050901. doi: 10.3389/fmicb.2022.1050901
56. Frendo, P, Baldacci-Cresp, F, Benyamina, SM, and Puppo, A. Glutathione and plant response to the biotic environment. Free Radic Biol Med. (2013) 65:724–30. doi: 10.1016/j.freeradbiomed.2013.07.035
57. Zhu, SM, Liang, YL, Gao, DK, An, XJ, and Kong, FC. Spraying foliar selenium fertilizer on quality of table grape (Vitis vinifera L.) from different source varieties. Sci Hortic. (2017) 218:87–94. doi: 10.1016/j.scienta.2017.02.025
58. Zhou, X, Yang, J, Kronzucker, HJ, and Shi, W. Selenium biofortification and interaction with other elements in plants: a review. Front Plant Sci. (2020) 11:586421. doi: 10.3389/fpls.2020.586421
59. Gill, SS, and Tuteja, N. Reactive oxygen species and antioxidant machinery in abiotic stress tolerance in crop plants. Plant Physiol Biochem. (2010) 48:909–30. doi: 10.1016/j.plaphy.2010.08.016
60. Liao, XL, Rao, S, Yu, T, Zhu, ZZ, Yang, XY, Xue, H, et al. Selenium yeast promoted the se accumulation, nutrient quality and antioxidant system of cabbage (Brassica oleracea var L.). Plant Signal Behav. (2021) 16:1907042. doi: 10.1080/15592324.2021.1907042
61. Zhao, CH, Li, JH, Chen, N, Bai, HJ, Qu, LH, Yang, Y, et al. Antioxidant activity and transcriptomic analysis of se-enriched golden oyster mushroom Pleurotus citrinopileatus (Agaricomycetes). Int J Med Mushrooms. (2020) 22:755–62. doi: 10.1615/IntJMedMushrooms.2020035403
62. Das, CK, Srivastava, G, Dubey, A, Verma, S, Saxena, M, Roy, M, et al. The seed stimulant effect of nano iron pyrite is compromised by nano cerium oxide: regulation by the trace ionic species generated in the aqueous suspension of iron pyrite. RSC Adv. (2016) 6:67029–38. doi: 10.1039/C6RA15584G
63. Qureshi, MK, Gawroński, P, Munir, S, Jindal, S, and Kerchev, P. Hydrogen peroxide-induced stress acclimation in plants. Cell Mol Life Sci. (2022) 79:129. doi: 10.1007/s00018-022-04156-x
64. Yiotis, C, McElwain, JC, and Osborne, BA. Enhancing the productivity of ryegrass at elevated CO2 is dependent on tillering and leaf area development rather than leaf-level photosynthesis. J Exp Bot. (2021) 72:1962–77. doi: 10.1093/jxb/eraa584
Keywords: preharvest treatment, selenium enrichment, leaf mustard, anthocyanin fortification, growth promotion
Citation: Wang B, Yuan X, Wang G, Zhu Y-n, Zhou R-c, Feng H-m and Li H-b (2024) Preharvest sodium selenite treatments affect the growth and enhance nutritional quality of purple leaf mustard with abundant anthocyanin. Front. Nutr. 11:1447084. doi: 10.3389/fnut.2024.1447084
Edited by:
Rachida Ouaabou, Abdelmalek Essaadi University, MoroccoReviewed by:
Razieh Farzad, University of Florida, United StatesSaad Farouk, Mansoura University, Egypt
Copyright © 2024 Wang, Yuan, Wang, Zhu, Zhou, Feng and Li. This is an open-access article distributed under the terms of the Creative Commons Attribution License (CC BY). The use, distribution or reproduction in other forums is permitted, provided the original author(s) and the copyright owner(s) are credited and that the original publication in this journal is cited, in accordance with accepted academic practice. No use, distribution or reproduction is permitted which does not comply with these terms.
*Correspondence: Bin Wang, Yl93YW5nQHNndS5lZHUuY24=; Hui-min Feng, MzQ4NjAxNzY2M0BxcS5jb20=; Hai-bo Li, TGloYWktYm9AMTYzLmNvbQ==
†These authors have contributed equally to this work and share first authorship