- 1NITTE (Deemed to be University), Department of Food Safety and Nutrition, Nitte University Centre for Science Education and Research (NUCSER), Mangalore, Karnataka, India
- 2Department of Traditional Foods and Applied Nutrition, Central Food Technological Research Institute (CFTRI), Mysore, Karnataka, India
- 3Freeze Drying and Animal Product Technology Division, Defence Food Research Laboratory, DRDO, Ministry of Defence, Mysore, Karnataka, India
Background: Protein hydrolysates derived from food sources contains enormous number of peptides which are composed of amino acid possessess various bioactive properties. However, the use of protein hydrolysates as a nutraceutical is hindered due to their unpleasant flavour. The study aims to enhance the biological activity and palatability of protein hydrolysates.
Methodology: In the present study, soybean protein hydrolysate (SPH) was prepared using alcalase for 4 h (control). Modification of hydrolysis (MPH) was carried out by reiterating the hydrolysis of the supernatant obtained after 2 h of hydrolysis using an enzyme to 50% of alcalase during each successive hydrolysis. Samples were characterised by their physio-chemical and functional properties. Furthermore, the effect of modification on the protein digestibility and bitterness intensity using e-tongue was studied. The suppressive effect on retrogradation of corn starch was analysed using texture profile analysis.
Results: The results demonstrated increased protein content by 1.6 and 1.9% in MPH compared to SPH and UNH, respectively. MPH showed 1.5- and 1.6-fold higher DH% than SPH before and after gastrointestinal digestion (p < 0.05). A decrease in molecular weight was found in the order of UNH > SPH > MPH. Nevertheless, MPH displayed significantly higher functional properties (p ≤ 0.05). The hardness of retrograded corn starch was significantly reduced in the MPH (1.21N) than SPH (1.55 N) and UNH (1.81N) compared to control (1.71N) during 7-day storage at 4°C (p ≤ 0.05). E-tongue analysis of MPH showed a 4-fold reduction in bitterness than SPH.
Conclusion: Modification of hydrolysis of soybean has demonstrated its significance in improved DH% functional properties and palatability. In addition, improved protein digestibility with promising benefits in deferral action on retrogradation of starch over the traditional process of hydrolysis was observed. The outcome of this study contributes to the potential utilisation of MPH as an ingredient in the formulation of nutraceutical products.
1 Introduction
Soybean (Glycine max) is a good source of protein, dietary fibre, vitamins, and minerals. It has various industrial applications including extraction of edible oil or used as bio-diesels and bio-plastic materials. Protein hydrolysates are the fractions of protein that are formed during the hydrolysis of protein. These protein hydrolysates have proven to have bioactive (antioxidant, anticancer, antihypertensive, antimicrobials, etc.) and functional properties [water holding, oil holding, foam forming, emulsification, etc.), which are commonly used in animal feed and have limited use in food formulations due to low palatability (1–4). Numerous studies have been carried out on the preparation of soybean protein hydrolysates and their bioactive properties (5–7)]. The major challenge to using it as a nutraceutical food is the formation of bitter flavour during hydrolysis. Typically, hydrophobic amino acids such as glycine, alanine, valine, leucine, isoleucine, proline, phenylalanine, methionine, and tryptophan are known to contribute to bitterness in protein hydrolysates (8). Nevertheless, the bitterness of protein hydrolysates is also associated with the degree of hydrolysis (DH%), molecular weight, position of proline residues, type of enzymes used, and amino acid sequence in a peptide (9). Despite its high biological value, these problems hinder the usage of protein hydrolysates as a major constituent in a product (7, 8, 10, 11). Researchers and food industries have continued to work on improving the taste, odour, and overall acceptability of protein hydrolysates and alternative protein sources, and production methods are being explored to address sustainability and cost concerns (7, 8, 10). Thus, implementing the novel approach to produce the protein hydrolysates with the minimum process is an area of interest. Studies have been conducted on protein hydrolysates used as food additives in a high-starch gluten-free diet to improve its nutritional composition (12, 13). In our study, protein hydrolysates from soybeans were prepared by modifying the conventional enzymatic hydrolysis and studied for their characteristic features and functional properties. In addition, the incorporation of MPH into high-calorie-based corn starch is to examine its suitability in deferment of retrogradation in gelatinised corn starch. High-starch products often face severe retrogradation during cold storage which extremely affects the quality and shortens the shelf life of the products. There are studies demonstrating a combination of rice starch with ionic (xanthan gum) or non-ionic (guar gum) hydrocolloids exhibits a significant way to minimise the side effect of starch retrogradation (14, 15). Therefore, the present study intends to modify the conventional hydrolysis process to enhance the sensory qualities, functional properties, and digestibility of soybean protein hydrolysates.
2 Materials and methods
2.1 Materials
Soybean (Glycine max) was obtained from the local market in Mangaluru, Karnataka, India.
2.2 Reagents
Proteolytic enzymes such as alcalase (Bacillus licheniformis) (2.972 U/mL activity) were purchased from Sigma-Aldrich. Sodium hydroxide, solvents, digestive enzymes, and other chemicals used in the study were of analytical grade.
3 Methodology
3.1 Preparation of soybean protein hydrolysate (SPH)
Soybean protein hydrolysates were prepared according to Yathisha et al. (16). In brief, 500 g of dried soybean (14.01% moisture content) was soaked in potable water for 20 min followed by homogenisation with 1,000 mL of water. Inactivation of endogenous enzymes was carried out by heating the mixture at 90°C for 20 min. The homogenate was allowed to cool to 55°C, and the pH was adjusted to 8.5. Hydrolysis was carried out with 2% (w/v) alcalase for 4 h. The process was terminated at 90°C for 20 min followed by rapid cooling (4°C). The supernatant was separated and filtered after centrifuging at 10,000 rpm for 20 min. The filtrate was lyophilised and stored at −20°C for further analysis.
3.2 Modification of soybean protein hydrolysates (MPHs)
Modification of preparation of the protein hydrolysates was carried out by splitting the hydrolysis time and enzyme concentration by 50%. The process of the preparation of SPH (control) and MPH (Modified) is given in Figure 1A. MPH was prepared by adding 1% of alcalase enzyme (v/w) to the homogenate (pH 8.5) and incubating at 55°C for 2 h followed by centrifuging at 10,000 rpm for 20 min (Phase-I). The process was repeated for 2 h with the remaining 1% of alcalase (Phase-II). The supernatant was separated and filtered after centrifuging at 10,000 rpm for 20 min. The filtrate was lyophilised and stored at −20°C for further analysis.
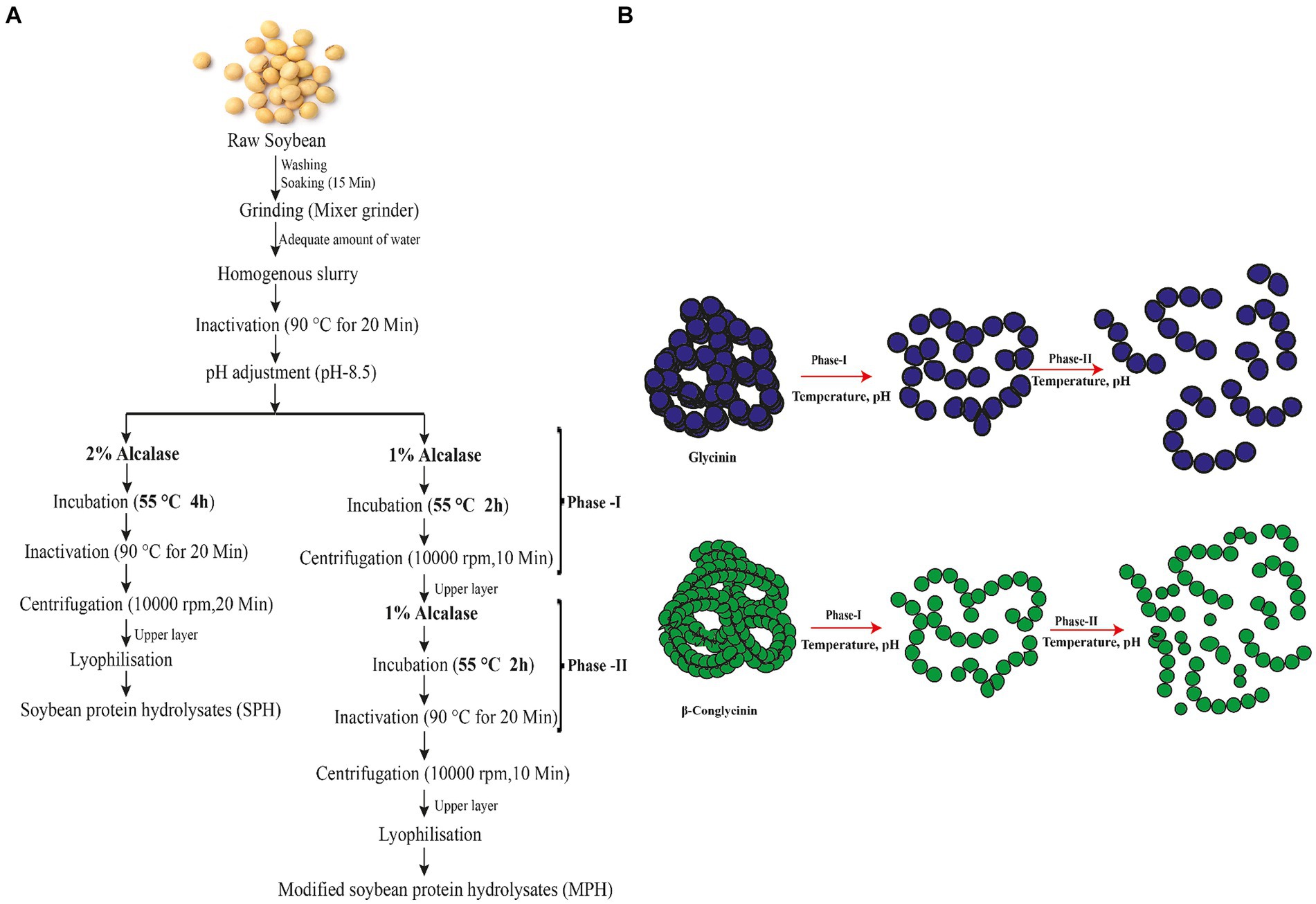
Figure 1. Preparation of soybean protein hydrolysates (SPHs) and modified protein hydrolysates (MPHs). (A) Enzymatic hydrolysis of protein from soybean is prepared by using alcalase for 4 h. In the conventional method, hydrolysis was performed for 4 h with 2% alcalase to produce soybean protein hydrolysis (SPH) which serves as a control. Modified hydrolysis of soybean (MPH) was performed by splitting the hydrolysis time and enzyme concentration into Phase-I and Phase-II. (B) Possible mechanism of generation of peptides during the preparation of modified protein hydrolysates (MPHs). During the hydrolysis of soybean, the main constituents of soya protein (glycinin and β-conglycinin) unfold at first and break down into peptides with varied chain lengths by the action of alcalase on hydrolysis. In Phase-I, the large trimeric and hexameric structure unfolds and forms polypeptides with varied MW. In Phase-II, the polypeptides formed in Phase-I are hydrolysed further which break down the polypeptides into smaller MW peptides.
3.3 Degree of hydrolysis (DH%)
The DH% was measured by estimating the soluble nitrogen using trichloroacetic acid (TCA). In brief, protein hydrolysates (5 mg/mL) and 50 μL of 10% TCA were added and incubated at room temperature for 15 min followed by centrifugation at 5000 rpm for 10 min. The supernatant was estimated for the protein content (16). The degree of hydrolysis was calculated using the following formula:
3.4 Characterization of soybean protein hydrolysates
3.4.1 Molecular weight distribution by SDS-PAGE
Distribution of MW in protein hydrolysates was carried out according to the method followed by Idowu et al. (17), with slight modifications. A stacking gel of 5% and a running gel composition of 20% were used to run the electrophoresis. In brief, 50 mg/mL of protein hydrolysates were prepared in phosphate buffer and mixed well. The solution was then centrifuged at 8500 g for 15 min at 4°C using a cold centrifuge. The supernatant was mixed with sample buffer (0.125 M Tris–HCl, pH 6.8 containing 4% SDS with β-mercaptoethanol) and 20% glycerol at a ratio of 1:1 (v/v). Then, 30 μL of the sample was loaded onto the gel. The electrophoresis was initially run at 60 MV for 30 min followed by 90 MV for 60 min. Furthermore, the gels were fixed and stained with 0.05% (w/v), Coomassive brilliant blue R-250 in 15% methanol and 5% acetic acid and de-stained using 30% methanol and 10% acetic acid. The low-range molecular mass markers (3 to 197 kDa) were used to identify the MW of the hydrolysates.
3.4.2 Amino acid composition by LC–MS
The amino acid content in SPH and MPH was estimated according to Vashishth et al. (18), with slight modifications. In brief, 100 mg of UNH, SPH, and MPH were treated with 1 mL of 6 N HCl in a separate 25 mL flask and incubated at 60°C for 24 h. Furthermore, the sample was diluted with 20 mL of HPLC grade water. A known amount of aliquot was dried and dissolved in 1 mL of 0.1 N HCl. The sample was derivatised using 5, 1, and 1 μL of borate buffer, o-phthalaldehyde (OPA), and sample, respectively. Then, 8 μL of FMOC (9-fluronitrilemethylchloroformate) reagent was added to the above mixture. The derivatised sample was analysed using an HPLC system (Waters RP-HPLC system Model-1525) with a C18 column (250 mm X 4.6 mm, no. OHSO1521) and equipped with a photodiode-array detector (PDA). The mobile phases A (2.72 g of sodium acetate trihydrate and 180 μL trimethylamine, pH 7.2 in 1000 mL water) and B [water, methanol, and acetonitrile (20:40:40) with 2.72 g sodium acetate trihydrate] were used at a flow rate of 0.45–1.0 mL/min at 338 nm for 30 min.
3.4.3 Particle size distribution
The particle size of the samples was determined using the laser diffraction particle size analyser (SALD-2101 SHIMADZU, Japan) equipped with Microtrac particle size analysis software (19).
3.4.4 Water activity
The water activity (aw) of the sample was measured using the water activity meter (Aqualab Pawkit Meter Food, USA). In brief, 0.5 g of the sample was placed on the plates and inserted into the system (19).
3.4.5 Color measurements
A colour measuring system (Model CM3500D, Minolta spectrophotometer, Minolta Co., Ltd., Japan) was used to determine the Chroma and Hue of the samples. The results were expressed in L*, a*, and b* values (20).
3.5 Evaluation of bitterness intensity by e-tongue analysis
The bitterness evaluation of protein hydrolysates was carried out using an Electronic-Tongue (Auto-sampler, Model: ASTREE, αM.O.S Toulouse, France) as explained by Singh et al. (21). In brief, 250 mg of SPH and MPH were dissolved in 25 mL of distilled water, vortexed until fully dissolved, and then filtered through Whatman NO.1 filter paper for analysis. Different concentrations of standard caffeine (0–0.020 M) were used as a standard. The standard curve was plotted against bitterness measured values v/s reference (Figure 2A) for the bitterness in the samples.
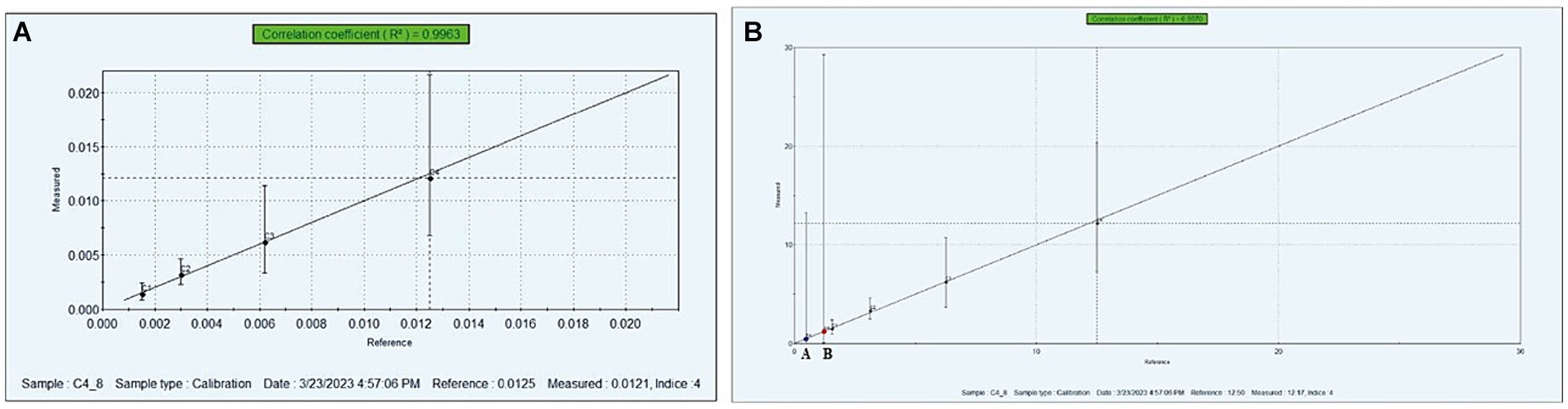
Figure 2. Calibration curve of standard caffeine obtained by e-tongue analysis (A), bitterness intensity of SPH and MPH (B). (A) Modified soybean protein hydrolysate (MPH), (B) soybean protein hydrolysate (SPH).
3.6 Functional properties
3.6.1 Solubility
The solubility of SPH and MPH was estimated at varied ranges of pH (2, 4, 6, 8, 10, and 12) as explained by Yathisha et al. (16). Next, a 200-mg sample was dissolved in 10 mL of distilled water. The pH was adjusted using 1 N HCl and 1 N NaOH. The mixture was stirred continuously at 37°C for 30 min and then centrifuged at 8000 rpm for 10 min. The supernatant was estimated for soluble proteins using Lowry’s method and calculated using the following equation.
3.6.2 Oil-holding capacity (OHC)
The OHC of SPH and MPH was determined according to Leni et al. (22). In brief, 100 mg of samples were taken in a pre-weighed vial, and 1 mL of sunflower oil was added. The samples were vortexed for 1 min and incubated for 30 min at room temperature. The mixture was centrifuged at 13,600 g at 25°C for 10 min (Eppendorf Germany 5810R). The final weight was taken after decanting the oil upside down (45° angle), and the OHC capacity was calculated as follows:
3.6.3 Hardness of retrograded corn starch using TPA
The mixture of 10 g of corn starch in 100 mL of deionised water and 1 g of samples and guar gum was heated at 95°C for 30 min in a shaking water bath. The starch was then allowed to cool for 1 h. The gelatinised samples were prepared into a rectangle shape (2.7 cm 170 × 2.7 cm × 2 cm) and stored at 4°C. Each sample was compressed thrice using a TA-XT plus texture analyser (TA Instruments, USA) equipped with a cylindrical probe (36 mm diameter) at 1 mm/s, and the deformation level was 30% of 173 the original sample height (13).
3.6.4 In vitro gastrointestinal (GI) digestion
The effect of GI digestion on the protein digestibility of samples was performed according to the method given by Sanjukta et al. (23), with slight modifications. The GI digestion was carried out initially using 4% pepsin (2 h) at pH 2 followed by 4% pancreatin (4 h) at pH 7.5 at 37°C. The enzyme activity was stopped by heating at 90°C for 10 min. The digesta was centrifuged and lyophilised to estimate the DH%.
3.7 Safety evaluation of SPH and MPH (in vitro and in vivo)
In vitro cell proliferation was measured by using RAW264.7 macrophage cell lines as described by Saisavoey et al. (24), with slight modifications. In brief, 1 × 104 cells were seeded in 96-well plates and incubated for 48 h. The cells are then treated with SPH and MPH at different concentrations (20–100 μg) for 48 h. Then, 5 μL of 50 μg/mL of MTT was added to each well and incubated for 4 h. The supernatant in each well was removed, and 100 μL of DMSO (dimethyl sulphoxide) was added. Absorbance was read at 595 nm using a multiplate reader, and cell viability was calculated using the following formula:
In vivo toxicity of MPH was evaluated using male Wistar rats approved by the Institutional Animal Ethics Committee (NGSMIPS/IAEC/JUNE-2020/207). The acute toxicity of MPH was assessed as per the guidelines (25). Six male Wistar rats (150–170 kg B.W) were divided into two groups (N = 3) caged separately and provided adequate diet and potable water with a 12-h day and night cycle. Before the study began, the animals were fasted overnight from food, and the test group was dosed orally with 2000 mg/kg BW of MPH, whereas the control group received the same amount of distilled water. Each rat was observed carefully for 14 days for any behavioural changes and lethality. After behavioural studies, the animals were sacrificed, and vital organs (heart, kidney, lungs, and liver) were collected and examined for histopathology (26).
3.8 Statistical analysis
All data represent the mean value ± SD of three independent measurements. The comparison between the two groups was carried out using two-way and one-way ANOVA. GraphPad Prism software version 8.3.0 was used to analyse statistical significance at a p-value of ≤0.05.
4 Results
4.1 Modified method for preparation of protein hydrolysis (MPH)
Process modification of preparation of protein hydrolysate was carried out to decrease the MW and reduce the bitterness in the sample. MPH was prepared by hydrolysing with alcalase to obtain SPH with higher nutritional and functional properties. The modification of traditional protein hydrolysate preparation avails the already hydrolysed proteins into further breakdown to release shorter chain length peptides with medium or low molecular weight peptides in it. The preparation of MPH and the possible mechanism for the generation of protein hydrolysates with lower MW peptides are shown in Figure 1.
MPH showed significantly higher protein content (68.86%) compared to SPH (58.36%) and UNH (34.62%). Nevertheless, modification resulted in reduced fat content in MPH to 10.65% from SPH which had fat content of 22.41%. These results demonstrate that a decrease in the fat content and an increase in the protein content can increase the biological activity of MPH along with stability in terms of long-term storage.
4.2 Degree of hydrolysis (DH%)
DH of the protein hydrolysates determines the extent of breaking down of the parent protein by the proteolytic enzymes. The DH% of all the samples is tabulated in Table 1. It was observed that the modification of soybean protein hydrolysates significantly increased the DH% than the control and unhydrolysed soybean protein (p < 0.05). MPH showed 3.3-fold higher than UNH and 1.5-fold higher than SPH. These results demonstrate that modification could increase the DH of the protein hydrolysates which intend to exhibit various biological and functional properties of the hydrolysates.
4.3 Molecular weight distribution by SDS-PAGE
The molecular weight (MW) distribution was illustrated using electrophoresis by SDS-PAGE (Figure 3). The MW of the SPH and MPH ranged between >3 kDa and 38 kDa. The bands in the gel showed that unhydrolysed soybean (UNH) showed bands up to 62 kDa indicating the high molecular proteins in it. On the other hand, SPH showed bands up to 28 kDa exhibiting a reduction in the molecular weight on hydrolysis. MPH showed thicker bands at 3 kDa and showed bands only up to 15 kDa. These results revealed that the modification of the hydrolysis process could efficiently decrease the molecular weights of MPH than SPH resulting in the formation of lower molecular weight peptides.
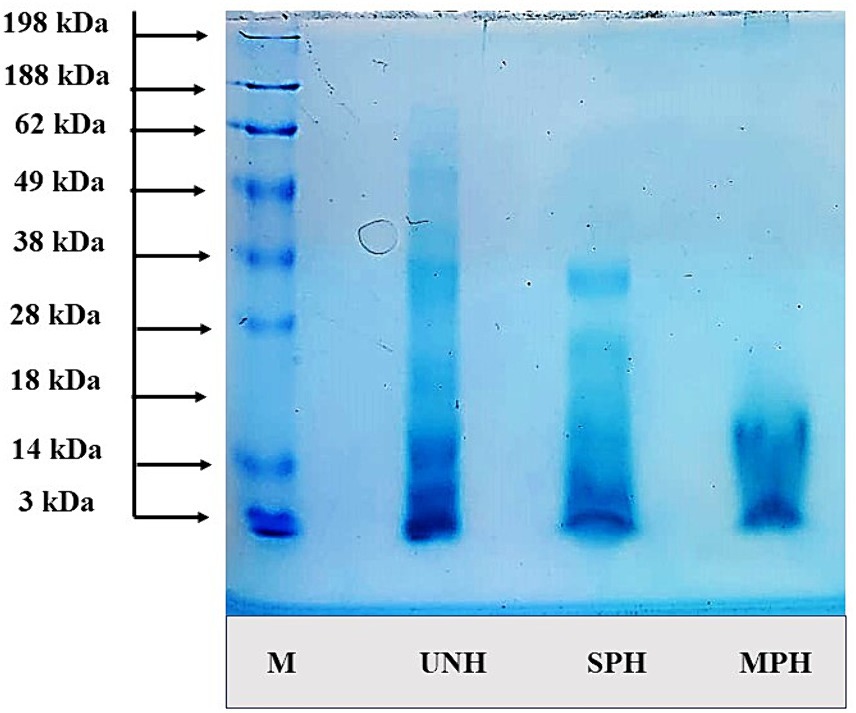
Figure 3. Molecular weight distribution of samples using SDS-PAGE. UNH, unhydrolysed soybean; SPH, soybean protein hydrolysate; MPH, modified protein hydrolysate.
4.4 Physio-chemical characterization
4.4.1 Amino acid composition of protein hydrolysates
Amino acid analysis was carried out to investigate the effect of the modified method on the residual amino acid contents of the sample. Amino acid compositions of SPH and MPH are given in Table 2. Among all the essential amino acids, arginine was found abundant in both SPH and MPH (19.90 and 11.79 mg/100 g, respectively). However, it was observed that there was a reduction in the amino acid content in MPH than SPH. This could be because of the degradation of amino acids during the hydrolysis process or thermal inactivation. Nevertheless, all the amino acid contents were found to be reduced in MPH. Tyrosine was absent in both SPH and MPH, whereas valine was found to be degraded in MPH.
4.4.2 Particle size distribution of protein hydrolysates
Particle size analysis of protein hydrolysates demonstrates the structural dimensions of the powders. The percentage particle size of the samples is shown in Table 1. In the present study, the particle size of the UNH, SPH, and MPH ranged between 0.1 and 1,000 μm. It was observed that MPH had a higher of 28.62% of particles ranging between 0 and 100 μm, whereas SPH and UNH had 20.81 and 17.31%, respectively, which are statistically significant (p ≤ 0.05). Both SPH and MPH had 80% of the particle size within 500 μm, whereas UNH showed 69.9%.
4.4.3 Water activity (aw) of protein hydrolysates
To determine the stability and shelf life of the dried samples from microbial and autolysis, aw was analysed. In the present study, UNH, SPH, and MPH were analysed for their water activity (Table 1). The aw of MPH was found to be significantly decreased compared to SPH and MPH (p ≤ 0.05), whereas a non-significant difference was observed between SPH and UNH. The aw was found to be in the order of MPH < SPH < UNH.
4.4.4 Color measurement of protein hydrolysates
The colour measurement was carried out to determine the hue and chroma of the hydrolysates. The results of colour measurements are shown in Table 1. L* values of UNH, SPH, and MPH showed 77.95, 73.83, and 72.99, respectively, whereas all the samples showed negative for a* and positive for b*. The results of L*, a*, and b* indicate the hydrolysates are light white with yellowish green in colour.
4.5 Evaluation of bitterness using e-tongue
The bitter taste in the protein hydrolysates is one major shortcoming to explore as it is a functional ingredient in nutraceutical food formulations. In the present study, the bitterness intensity of SPH and MPH was carried out using e-tongue. The bitterness concentration of the SPH and MPH is shown in Figure 2B. From the graph, it was observed that the bitterness concentration of SPH and MPH was found to be 0.48 and 0.12 mM/100 g, respectively. The results of this study revealed that the modification of soybean protein hydrolysates exhibited a 4-fold lesser bitterness concentration than the SPH.
4.6 Functional properties
4.6.1 Oil-holding capacity (OHC) and solubility
In the present study, the samples were evaluated for their OHC and solubility before and after hydrolysis. The OHC of all the samples is shown in Table 1. From the table, it was observed that the unhydrolysed soybean protein had higher OHC (4.74 g of oil/g), whereas SPH and MPH had 3.34 and 3.27 oil/g of hydrolysates, respectively. The OHC between SPH and MPH is statistically non-significant (p > 0.05). Solubility (%) in different pH (2–12) of the UNH, SPH, and MPH is shown in Figure 4. UNH showed a solubility (%) maximum of 21.41% at pH 2 and least at pH 6 (6.55%). SPH and MPH showed higher solubility (87.96 and 97.79%) at pH 2 and lower solubility (78.56 and 79.63%) at pH 6.
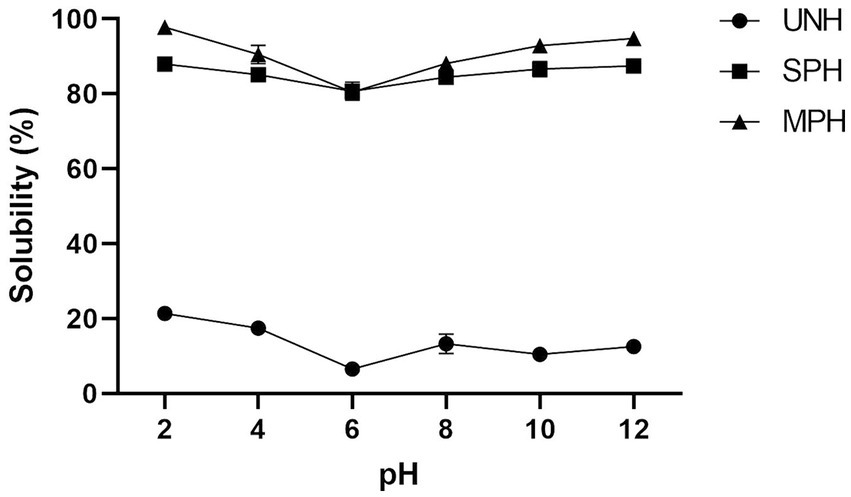
Figure 4. Solubility (%) of UNH, SPH, and MPH at different pH. UNH, unhydrolysed soybean; SPH, soybean protein hydrolysate; MPH, modified protein hydrolysate.
4.6.2 Hardness of retrograded corn starch using texture profile analysis (TPA)
The effect of samples in reducing the hardness of retrograded corn starch was evaluated using texture profile analysis (TPA). The TPA of retrograded corn starch during the 0th and 7th day of storage is given in Table 3. The hardness of the gels incorporated with MPH showed significantly lower hardness than other samples. As observed in the table, the hardness of gelatinised corn starch (control) evidently increased (p ≤ 0.05) from 0.64 to 1.73 N during the course of storage from 0 to 7 days at 4°C, whereas the addition of 1% MPH showed an increase from 0.24 to 1.21 N. Nevertheless, other parameters such as fracturability, cohesiveness, and gumminess were also found to be altered by the addition of UNH, SPH, and MPH.
4.7 Effect of modification of soybean hydrolysis on protein digestibility
In the present study, the protein digestibility of all the samples was determined by GI digestion followed by estimating the DH% (Figure 5). The results showed that the protein digestibility was found to be higher in MPH than in SPH (p < 0.05). All the samples showed significantly increased DH% upon GI digestion. On the other hand, GI-digested MPH showed 1.61-fold higher DH than GI-digested SPH and 2.57-fold higher than UNH. These findings illustrate the importance of modification on increased protein digestibility of MPH than others.
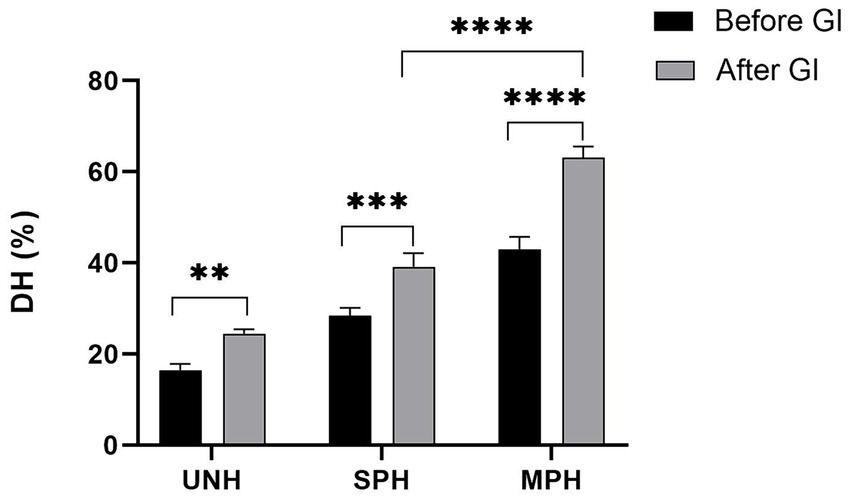
Figure 5. Effect of gastrointestinal digestion on the degree of hydrolysis of UNH, SPH, and MPH. UNH, unhydrolysed soybean; SPH, soybean protein hydrolysate; MPH, modified protein hydrolysate. *Statistical significance at p ≤ 0.05. **Statistical significance at p ≤ 0.01. ***Statistical significance at p ≤ 0.001. ****Statistical significance at p ≤ 0.0001.
4.8 Safety evaluation of SPH and MPH
The SPH and MPH were assessed for their cell proliferation ability using RAW264.7 macrophage cell lines. The cell proliferation was found to be 115 and 118% in SPH and MPH, respectively. The results indicate that both SPH and MPH have higher LC50 concentrations and are likely to be non-toxic. This result was further confirmed by in vivo acute toxicity assay. The in vivo toxicity using male Wistar rats was assessed to examine any behavioural, neurological, and histological changes. No changes were observed in the neurological functions or behaviours such as aggressiveness, alertness, touch response, and motor activity, following the oral administration of MPH. The histopathological observation of vital organs using eosin staining is shown in Figure 6. From the histopathology results, it was found that there were no changes in the function as per the histological study.
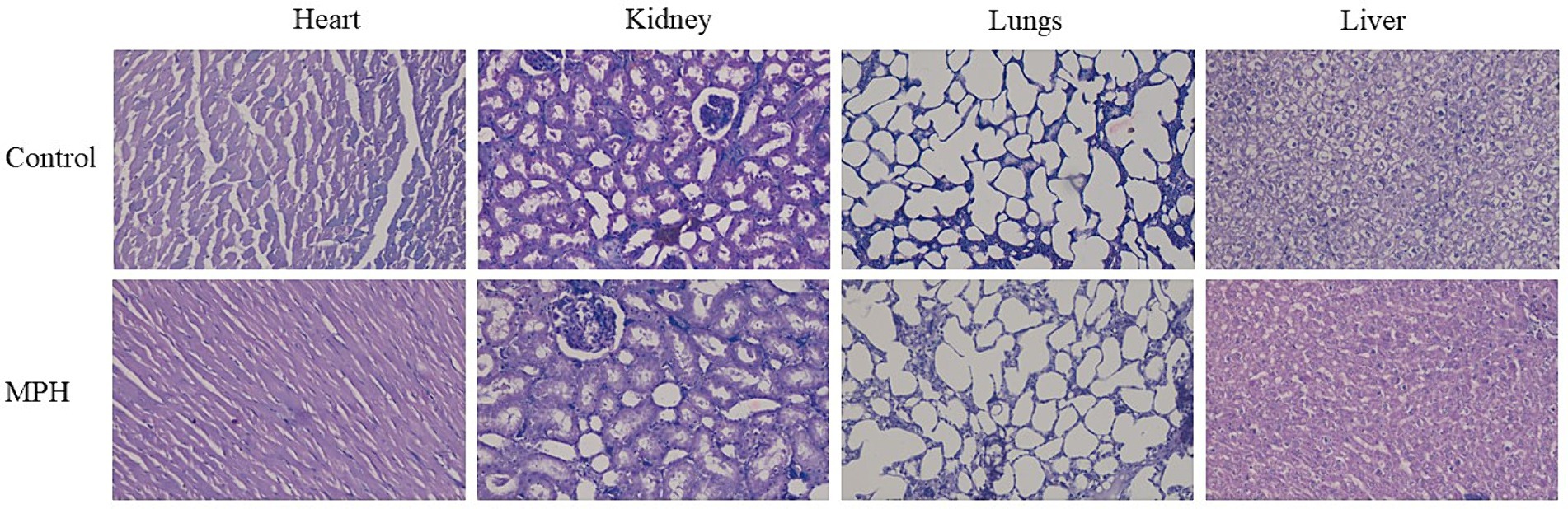
Figure 6. Histopathological examination of heart and lungs of Wistar rats dosed with MPH at 2000 mg/kg B.W. MPH, modified soybean protein hydrolysate.
5 Discussion
5.1 Physicochemical properties of soybean protein hydrolysate before and after modification
Protein hydrolysates are comprised of active peptides and exhibit therapeutic potential against lifestyle disorders (27). Scientific investigations underscore their bioactive properties and enhanced functional attributes (4, 5, 28–30). Despite their promising role in nutraceutical foods, their practical application is currently constrained primarily by production costs, alterations during digestion, and suboptimal palatability (2, 8). Reports indicate that protein hydrolysates with higher DH% and peptides of lower MW often exhibit favourable functional and bioactive properties (4, 31, 32). Our study focussed to enhance the palatability and functional characteristics of protein hydrolysates through modified hydrolysis processes, specifically by adjusting time and enzyme concentration in two phases. The study aims to reduce the bitterness and improve the functional properties of soya protein hydrolysate.
Proteolytic enzymes play a vital role in generating protein hydrolysates, with the DH% indicating the extent of the enzyme-substrate reaction (33). The increased DH% in MPH is likely due to hydrolysed oligo-peptides from Phase-I undergoing further hydrolysis into smaller peptides in Phase-II, without interference from unhydrolysed native proteins. Alcalase is known for its broad substrate specificity, which is an endo-peptidase that cleaves hydrophobic amino acids such as tryptophan, phenylalanine, leucine, isoleucine, valine, and methionine residues (34). Yathisha et al. (4) reported that hydrolysis with alcalase yielded a higher DH (38%) in ribbon fish protein hydrolysates compared to other enzymes such as flavourzyme and papain (12 and 18%, respectively). The MW distribution of protein showed bands ranging between 3 and 15 kDa in the MPH sample, while SPH and UNH exhibited wider ranges. Similar changes in MW were observed in Sacha inchi (Plukenetia volubilis) protein hydrolysates reported by Rawdkuen et al. (35) using papain and Calotropis proteases. Suresh (36) noted an increase in bands in the lower MW region with higher enzyme concentration and hydrolysis time. These results are also supported by the particle size of the SPH and MPH which showed that 78.4% of the particles have 0–500 μm size. Studies have reported the fact that protein hydrolysate showing lower MW peptides possesses higher functional and bioactive properties (2, 16, 37).
The development of bitterness in the hydrolysis of protein is a major challenge in food industries to be used as a functional ingredient (7). According to Fan et al. (38), the presence of hydrophobic amino acids in the protein hydrolysates, such as phenylalanine, proline, leucine, arginine, valine, lysine, and histidine, can be attributed to the bitterness. The author also states that hydrolysis with alkaline protease produced hydrolysates with the highest bitterness. In our study, modification of protein hydrolysate preparation using alcalase exhibited effectiveness in reducing the bitterness, showing 4-fold lesser than SPH. Correspondingly, MPH showed reduced hydrophobic amino acids such as proline, phenylalanine, leucine, isoleucine, and complete destruction of valine when compared to SPH. These findings corroborate with the results reported by Newman et al. (39) and Fan et al. (38). The author states that prolonging hydrolysis time beyond a specific point results in a decrease in bitterness due to the eventual hydrolysis of the bitter peptides. Changing the proteolytic enzymes can also reduce the bitterness in the hydrolysates (11). Studies have also reported that other treatments with activated carbon, extraction with alcohol, use of cyclodextrins, chromatographic separation, hydrolysing further with the exopeptidase, and use of butanol can also aid in de-bittering (8, 10). Another study by Zhang et al. (7) stated that soya protein hydrolysates with alcalase and cross-linking with trans-glutaminase (TGase) could reduce the bitter-active peptides lowering bitterness and improving the overall flavour. However, these methods are also reported to affect protein hydrolysate yield, altering the structure and bioactive properties.
5.2 Effect of modification on functional properties and protein digestibility of soybean protein hydrolysates
Functional properties are the physio-chemical properties of proteins that influence their behavior during processing, handling, and consumption. Hydrolysis of protein using proteolytic enzymes produces peptides of different chain lengths and free amino acids which modifies the properties of native proteins such as solubility, oil-holding capacity, swelling capacity, foaming, and emulsifying capacities. Generally, protein hydrolysates with higher DH increase the ionisation of amino and carboxyl side chains of amino acid residues increasing the hydrophilicity. Hydrolysis develops interactions between the hydrophobic peptides and the remaining intact protein in hydrolysates causing a decrease in hydrophobicity of the protein surface, thereby increasing their solubility (40). Similar results were observed, where UNH had the least water solubility at different pH ranges. Conversely, SPH and MPH showed higher solubility in the acid and alkaline media whereas solubility was found to be decreased in the low acidic to neutral pH. The decrease in solubility of approximately 5.0–6.0 pH is due to the general isoelectric point for seed proteins (41). The solubility of walnut protein hydrolysates was found to be increased as the hydrolysis time increased (42). These results were consistent with the findings of Mokni Ghribi et al. (43) in chickpea protein hydrolysates using alcalase. Horax et al. (40) reported maximum solubility (74.5% at pH 3) of soybean protein hydrolysates (40). In the present study, the OHC of the samples was observed to decrease accordingly as the DH% increased. However, the OHC of SPH and MPH was observed to be non-significant (p ≤ 0.05). These findings are similar to the study carried out with cherry kernel protein concentrate and hydrolysates by Cingöz and Yildirim (44). The author reports that the OHC of the hydrolysates is not solely dependent on the hydrolysis or DH but may be due to the hydrolysis process which might reduce the oil-trapping surfaces. Similar results were also reported by Guan et al. (45) in which the OHC was found to be decreased in the trypsin hydrolysed oat bran protein compared to untreated protein. These findings also throw light on the modification of hydrolysis in improving the functional properties over the traditional process. Retrogradation is a phenomenon in which the gelatinised starch hardens over a period of time by the re-association of amylose and amylopectin removing water molecules from it (13). In our study, the influence of MPH on the hardness of gelatinised starch showed a significant reduction in hardness even after storing for 7 days at 4°C. Our results also correlate with the findings of Hu et al. (12) and Luo et al. (13), in which addition of whey protein and grass carp protein hydrolysates decreased the hardness of the gelatinised rice starch. In the present study, the MPH showed a higher tendency to reduce the hardness of the corn starch over SPH and UNH. The inhibition of the formation of hydrogen bonds among the starch chain is because of the interaction between the smaller peptides with the starch molecules and their formation of the starch–protein complex (13). These findings also support our findings of decreased hardness of retrograded starch.
Protein digestibility is one of the important characteristics which determines the bioavailability of protein hydrolysates. In the present study, the protein digestibility of all the samples was assessed by GI digestion followed by estimating the DH%. The increase in the DH% indicates a large number of peptide bonds being cleaved during GI digestion, which implies an increase in protein digestibility (46). The author also reported that modifications in the protein structures during processing (hydrolysis) may expose more peptide bonds for cleaving during GI digestion (47). Our results also corroborate with these studies as the protein digestibility was found to be in the order of MPH > SPH > UNH. Studies also suggest that lower molecular weight peptides are more likely to be absorbed in the body than high molecular weight peptides. Our previous review also supports the fact that GI digestion can alter the molecular weight of the peptides and have both positive and negative effects on absorption and bioactive properties (2, 37). Studies have also proven that increased DH% shows higher bioactive and functional properties (4, 16, 48). Hence, the increased DH% after GI digestion can improve the bioaccessibility of protein for absorption in the intestine. On the other hand, the effect of GI digestion on the bioactive properties needs to be studied to depict its efficiency on the bioactive properties of MPH. Hence, the use of MPH as a high protein constituent makes them a prominent food additive. The influence of protein hydrolysates on high-calorie food can be investigated to understand their functional attributes. Liu et al. (49) studied the suitability of soy protein hydrolysates as a fat replacer in ice cream. These findings also provide additional application of MPH in promoting its use as a food additive for food processing industries.
5.3 Safety evaluation of soybean protein hydrolysates
SPH and MPH exhibited higher cell proliferation in normal cell lines. This could be because the essential amino acids in both SPH and MPH would promote cell growth by increasing the cell metabolic activity in the test group. Similar results were also obtained in the in vivo toxicity assay in which the test group rats did not show any behavioural changes. The histopathological examination also proved that there were no significant pathological changes among the test group and control group. This might be because of the presence of essential amino acids in SPH and MPH that could promote cell growth in in vitro and harmless effects in in vivo. Other studies have also suggested that protein hydrolysates are likely to be non-toxic and can be consumed without causing any major side effects (24, 26). According to Umayaparvathi et al. (50), protein hydrolysates and isolated peptides exhibit cytotoxicity in cancer cells, but on the other hand, they are also non-toxic in normal cell lines. This could be because of the selective cell proliferation behaviour of the bioactive compounds.
6 Conclusion
The study aimed to investigate the functional properties, palatability, and protein digestibility of MPH over SPH and UNH. Despite various methods employed for de-bittering protein hydrolysates, it often incurs high costs, reduced yield, and alterations in properties. Contrary to these challenges, our study demonstrated that MPH maintained functional group stability, enhanced functional properties, and reduced bitterness intensity without any additional process. The outcomes of the study emphasise the promising functional properties of MPH and its potential in the food and pharmaceutical industries, providing valuable insights for improving properties with reduced bitterness. The cause of high functionality and decreased bitterness needs to be studied in detail. Furthermore, the findings can have applications in the broader food industry, potentially influencing the development of new and improved food formulations.
Data availability statement
The raw data supporting the conclusions of this article will be made available by the authors, without undue reservation.
Ethics statement
Ethical approval was not required for the studies on humans in accordance with the local legislation and institutional requirements because only commercially available established cell lines were used. The animal study was approved by NGSM Institute of Pharmaceutical Sciences, Deralakatte, Mangalore. The study was conducted in accordance with the local legislation and institutional requirements.
Author contributions
NJ: Conceptualization, Data curation, Formal analysis, Methodology, Validation, Writing – original draft, Writing – review & editing. SA: Writing – original draft. MN: Writing – original draft. SY: Writing – original draft. BM: Conceptualization, Funding acquisition, Investigation, Methodology, Project administration, Resources, Supervision, Validation, Visualization, Writing – original draft, Writing – review & editing.
Funding
The author(s) declare that financial support was received for the research, authorship, and/or publication of this article. This study was funded by Nitte (Deemed to be University), Nitte University Centre for Science Education and Research Indian Council of Medical Research (ICMR).
Acknowledgments
The authors thank Anirban Chakraborty, Director, Nitte University Centre for Science Education and Research (NUCSER), Nitte (Deemed to be University) for his constant support. The authors also extend their gratitude to Iddya Karunasagar and Indrani Karunasagar, DST-NUTEC, Nitte (Deemed to be University). The authors are indebted to Nitte (DU) for providing the facilities. The authors extend their sincere gratitude to the Indian Council of Medical Research (ICMR) for the financial support.
Conflict of interest
The authors declare that the research was conducted in the absence of any commercial or financial relationships that could be construed as a potential conflict of interest.
Publisher’s note
All claims expressed in this article are solely those of the authors and do not necessarily represent those of their affiliated organizations, or those of the publisher, the editors and the reviewers. Any product that may be evaluated in this article, or claim that may be made by its manufacturer, is not guaranteed or endorsed by the publisher.
References
1. Lassissi, TA, Hettiarachchy, NS, Rayaprolu, SJ, Kannan, A, and Davis, M. Functional properties and Angiotensin-I converting enzyme inhibitory activity of soy – whey proteins and fractions. FRIN. (2014) 64:598–602. doi: 10.1016/j.foodres.2014.07.015
2. Jogi, N, Yathisha, UG, and Bhat, I. Antihypertensive activity of orally consumed ACE-I inhibitory peptides. Crit Rev Food Sci Nutr. (2021) 62:8986–99. doi: 10.1080/10408398.2021.1938508
3. Verma, AK, Chatli, MK, Kumar, P, and Mehta, N. Antioxidant and antimicrobial activity of porcine liver hydrolysate in meat emulsion and their influence on Physico-chemical and color deterioration during refrigeration storage. J Food Sci. (2019) 84:1844–53. doi: 10.1111/1750-3841.14683
4. Yathisha, UG, Karunasagar, I, and Mamatha, BS. Bioactivity and functional properties of protein hydrolysate from muscle and visceral waste of ribbon fish (Lepturacanthus savala). Extracted Three Differ Proteolytic Enzymes. (2021) 11:363–79. doi: 10.1080/22311866.2021.1953399
5. Chatterjee, C, Gleddie, S, and Xiao, CW. Soybean bioactive peptides and their functional properties. Nutrients. (2018) 10:8–11. doi: 10.3390/nu10091211
6. Hanafi, MA, Hashim, SN, Chay, SY, Ebrahimpour, A, Zarei, M, Muhammad, K, et al. High angiotensin-I converting enzyme (ACE) inhibitory activity of Alcalase-digested green soybean (Glycine max) hydrolysates. Food Res Int. (2018) 106:589–97. doi: 10.1016/j.foodres.2018.01.030
7. Zhang, Q, Cheng, Z, Wang, Y, Zheng, S, Wang, Y, and Fu, L. Combining Alcalase hydrolysis and transglutaminase-cross-linking improved bitterness and techno-functional properties of hypoallergenic soybean protein hydrolysates through structural modifications. LWT. (2021) 151:112096. doi: 10.1016/j.lwt.2021.112096
8. Idowu, AT, and Benjakul, S. Bitterness of fish protein hydrolysate and its debittering prospects. J Food Biochem. (2019) 43:e12978–10. doi: 10.1111/jfbc.12978
9. Yarnpakdee, S, Benjakul, S, Kristinsson, HG, and Kishimura, H. Antioxidant and sensory properties of protein hydrolysate derived from Nile tilapia (Oreochromis niloticus) by one- and two-step hydrolysis. J Food Sci Technol. (2015) 52:3336–49. doi: 10.1007/s13197-014-1394-7
10. Daukšas, E, Šližyte, R, Rustad, T, and Storrø, I. Bitterness in fish protein hydrolysates and methods for removal. J. Aquatic Food Product Technol. (2004) 13:101–14. doi: 10.1300/J030v13n02_09
11. Humiski, LM, and Aluko, RE. Physicochemical and bitterness properties of enzymatic pea protein hydrolysates. J Food Sci. (2007) 72:S605–11. doi: 10.1111/j.1750-3841.2007.00475.x
12. Hu, Y, He, C, Zhang, M, Zhang, L, Xiong, H, and Zhao, Q. Inhibition from whey protein hydrolysate on the retrogradation of gelatinized rice starch. Food Hydrocoll. (2020) 108:105840. doi: 10.1016/j.foodhyd.2020.105840
13. Luo, Y, Niu, L, Zhang, S, and Xiao, J. Effect of grass carp skin protein hydrolysates on the Retrogradation tendency of gelatinized Rice starch in the presence of non-ionic and ionic hydrocolloids during cold storage. Starch/Staerke. (2019) 71:1–29. doi: 10.1002/star.201800260
14. Samutsri, W, and Suphantharika, M. Effect of salts on pasting, thermal, and rheological properties of rice starch in the presence of non-ionic and ionic hydrocolloids. Carbohydr Polym. (2012) 87:1559–68. doi: 10.1016/j.carbpol.2011.09.055
15. Tang, M, Hong, Y, Gu, Z, Zhang, Y, and Cai, X. The effect of xanthan on short and long-term retrogradation of rice starch. Starch/Staerke. (2013) 65:702–8. doi: 10.1002/star.201200170
16. Yathisha, UG, Vaidya, S, and Sheshappa, MB. Functional properties of protein Hydrolyzate from ribbon fish (Lepturacanthus Savala) as prepared by enzymatic hydrolysis. Int J Food Prop. (2022) 25:187–203. doi: 10.1080/10942912.2022.2027964
17. Idowu, AT, Benjakul, S, Sinthusamran, S, Sookchoo, P, and Kishimura, H. Protein hydrolysate from salmon frames: production, characteristics and antioxidative activity. J Food Biochem. (2018) 43:e12734–12. doi: 10.1111/jfbc.12734
18. Vashishth, R, Semwal, AD, Naika, M, Sharma, GK, and Kumar, R. Influence of cooking methods on antinutritional factors, oligosaccharides and protein quality of underutilized legume Macrotyloma uniflorum. Food Res Int. (2021) 143:110299. doi: 10.1016/j.foodres.2021.110299
19. Suryabhan, P, Lohith, K, and Anu-Appaiah, KA. Sucrose and sorbitol supplementation on maltodextrin encapsulation enhance the potential probiotic yeast survival by spray drying. LWT. (2019) 107:243–8. doi: 10.1016/j.lwt.2019.03.002
20. Das, M, Mayookha, VP, Geetha, V, Chetana, R, and Suresh Kumar, G. Influence of different drying techniques on quality parameters of mushroom and its utilization in development of ready to cook food formulation. J Food Sci Technol. (2023) 60:1342–54. doi: 10.1007/s13197-023-05680-9
21. Singh, V, Kushwaha, R, and Kaur, D. Optimization study of sweeteners for preparation of syrup for sugar free traditional sweet: Gulab jamun. Int J Curr Res Rev. (2017) 9:45–53. doi: 10.7324/ijcrr.2017.9217
22. Leni, G, Soetemans, L, Caligiani, A, Sforza, S, and Bastiaens, L. Degree of hydrolysis affects the techno-functional properties of lesser mealworm protein hydrolysates. Food Secur. (2020) 9:381. doi: 10.3390/foods9040381
23. Sanjukta, S, Rai, AK, Muhammed, A, Jeyaram, K, and Talukdar, NC. Enhancement of antioxidant properties of two soybean varieties of Sikkim Himalayan region by proteolytic Bacillus subtilis fermentation. Elsevier. (2015) 14:650–8. doi: 10.1016/j.jff.2015.02.033
24. Saisavoey, T, Sangtanoo, P, Reamtong, O, and Karnchanatat, A. Antioxidant and anti-inflammatory effects of defatted Rice bran (Oryza Sativa L.) protein hydrolysates on raw 264.7 macrophage cells. J Food Biochem. (2016) 40:731–40. doi: 10.1111/jfbc.12266
25. CPCSEA . CPCSEA guidelines for laboratory animal facility. Indian J. Pharmacol. (2003) 35:257–74.
26. Hussein, FA, Chay, SY, Ghanisma, SBM, Zarei, M, Auwal, SM, Hamid, AA, et al. Toxicity study and blood pressure–lowering efficacy of whey protein concentrate hydrolysate in rat models, plus peptide characterization. J Dairy Sci. (2020) 103:2053–64. doi: 10.3168/jds.2019-17462
27. Nasri, M. (2017) Protein hydrolysates and biopeptides: production, biological activities, and applications in foods and health benefits. 1stst edn, ed. F. Toldrá (Department of Food Science, Instituto de Agroquímica y Tecnología de Alimentos (CSIC)). 81. Advances in food and nutrition research. San diego, CA: United States: Elsevier Inc
28. Bhandari, D, Rafiq, S, Gat, Y, Gat, P, Waghmare, R, and Kumar, V. A review on bioactive peptides: physiological functions, bioavailability and safety. Int J Pept Res Ther. (2020) 26:139–50. doi: 10.1007/s10989-019-09823-5
29. Embiriekah, S, Bulatović, M, Borić, M, Zarić, D, and Rakin, M. Antioxidant activity, functional properties and bioaccessibility of whey protein hydrolysates. Int J Dairy Technol. (2018) 71:243–52. doi: 10.1111/1471-0307.12428
30. Kim, SY, Park, PSW, and Rhee, KC. Functional properties of proteolytic enzyme modified soy protein isolate. J Agric Food Chem. (2002) 38:651–6. doi: 10.1021/JF00093A014
31. Chalamaiah, M, Jyothirmayi, T, Diwan, PV, and Dinesh Kumar, B. Antioxidant activity and functional properties of enzymatic protein hydrolysates from common carp (Cyprinus carpio) roe (egg). J Food Sci Technol. (2015) 52:5817–25. doi: 10.1007/s13197-015-1714-6
32. Zamora-sillero, J, Gharsallaoui, A, and Prentice, C. Peptides from fish by-product protein hydrolysates and its functional properties: an overview. New York, NY: Marine Biotechnology. (2018) 20:118–130. doi: 10.1007/s10126-018-9799-3
33. Balti, R, Bougatef, A, el-Hadj Ali, N, Zekri, D, Barkia, A, and Nasri, M. Influence of degree of hydrolysis on functional properties and angiotensin I-converting enzyme-inhibitory activity of protein hydrolysates from cuttlefish (Sepia officinalis) by-products. J Sci Food Agric. (2010) 90:2006–14. doi: 10.1002/jsfa.4045
34. Karimi, A, Azizi, MH, and Ahmadi Gavlighi, H. Frationation of hydrolysate from corn germ protein by ultrafiltration: in vitro antidiabetic and antioxidant activity. Food Sci Nutr. (2020) 8:2395–405. doi: 10.1002/fsn3.1529
35. Rawdkuen, S, Rodzi, N, and Pinijsuwan, S. Characterization of sacha inchi protein hydrolysates produced by crude papain and Calotropis proteases. LWT. (2018) 98:18–24. doi: 10.1016/j.lwt.2018.08.008
36. Suresh, PV . Protein hydrolysate from duck egg white by Flavourzyme® digestion: process optimisation by model design approach and evaluation of antioxidant capacity and characteristic properties. LWT. (2022) 156:113018. doi: 10.1016/j.lwt.2021.113018
37. Yathisha, UG, Bhat, I, Karunasagar, I, and Mamatha, BS. Antihypertensive activity of fish protein hydrolysates and its peptides. Crit Rev Food Sci Nutr. (2019) 59:2363–74.
38. Fan, W, Tan, X, Xu, X, Li, G, Wang, Z, and du, M. Relationship between enzyme, peptides, amino acids, ion composition, and bitterness of the hydrolysates of Alaska Pollock frame. J Food Biochem. (2019) 43:e12801–9. doi: 10.1111/jfbc.12801
39. Newman, J, Harbourne, N, O’Riordan, D, Jacquier, JC, and O’Sullivan, M. Comparison of a trained sensory panel and an electronic tongue in the assessment of bitter dairy protein hydrolysates. J Food Eng. (2014) 128:127–31. doi: 10.1016/j.jfoodeng.2013.12.019
40. Horax, R, Vallecios, MS, Hettiarachchy, N, Osorio, LF, and Chen, P. Solubility, functional properties, ACE-I inhibitory and DPPH scavenging activities of Alcalase hydrolysed soy protein hydrolysates. Int J Food Sci Technol. (2017) 52:196–204. doi: 10.1111/ijfs.13267
41. Chatterjee, R, Dey, TK, Ghosh, M, and Dhar, P. Enzymatic modification of sesame seed protein, sourced from waste resource for nutraceutical application. Food Bioprod Process. (2015) 94:70–81. doi: 10.1016/j.fbp.2015.01.007
42. Moghadam, M, Salami, M, Mohammadian, M, Emam-Djomeh, Z, Jahanbani, R, and Moosavi-Movahedi, AA. Physicochemical and bio-functional properties of walnut proteins as affected by trypsin-mediated hydrolysis. Food Biosci. (2020) 36:100611. doi: 10.1016/j.fbio.2020.100611
43. Mokni Ghribi, A, Maklouf Gafsi, I, Sila, A, Blecker, C, Danthine, S, Attia, H, et al. Effects of enzymatic hydrolysis on conformational and functional properties of chickpea protein isolate. Food Chem. (2015) 187:322–30. doi: 10.1016/j.foodchem.2015.04.109
44. Cingöz, A, and Yildirim, M. Effects of hydrolysis degree on the functional properties of hydrolysates from sour cherry kernel protein concentrate. Foods Raw Materials. (2023) 11:197–205. doi: 10.21603/2308-4057-2023-2-566
45. Guan, X, Yao, H, Chen, Z, Shan, L, and Zhang, M. Some functional properties of oat bran protein concentrate modified by trypsin. Food Chem. (2007) 101:163–70. doi: 10.1016/j.foodchem.2006.01.011
46. Aryee, ANA, and Boye, JI. Improving the digestibility of lentil flours and protein isolate and characterization of their enzymatically prepared hydrolysates. Int J Food Prop. (2016) 19:2649–65. doi: 10.1080/10942912.2015.1123269
47. Popović, L, Peričin, D, Vaštag, Ž, Popović, S, Krimer, V, and Torbica, A. Antioxidative and functional properties of pumpkin oil cake globulin hydrolysates. J Am Oil Chemists Soc. (2013) 90:1157–65. doi: 10.1007/s11746-013-2257-5
48. Jogi, N, Mathew, A, and Mamatha, BS. Modification of bioactive properties in food protein hydrolysates by Alcalase and trypsin. J Health Allied Sci. (2024) 2024. doi: 10.1055/s-0044-1782643
49. Liu, R, Wang, L, Liu, Y, Wu, T, and Zhang, M. Fabricating soy protein hydrolysate/xanthan gum as fat replacer in ice cream by combined enzymatic and heat-shearing treatment. Food Hydrocoll. (2018) 81:39–47. doi: 10.1016/j.foodhyd.2018.01.031
Keywords: soybean, duel hydrolysis, modification, functional food, bitternes
Citation: Jogi N, Adusumilli S, Nagesh M, Yannam SK and Mamatha BS (2024) The role of duel hydrolysis of soybean on functional properties and protein digestibility: a sustainable approach. Front. Nutr. 11:1444329. doi: 10.3389/fnut.2024.1444329
Edited by:
Sajad Shokri, University of Bath, United KingdomReviewed by:
Jie Tu, Jiangsu University of Science and Technology, ChinaYakindra Timilsena, RMIT University, Australia
Kingsley George Masamba, Lilongwe University of Agriculture and Natural Resources, Malawi
Copyright © 2024 Jogi, Adusumilli, Nagesh, Yannam and Mamatha. This is an open-access article distributed under the terms of the Creative Commons Attribution License (CC BY). The use, distribution or reproduction in other forums is permitted, provided the original author(s) and the copyright owner(s) are credited and that the original publication in this journal is cited, in accordance with accepted academic practice. No use, distribution or reproduction is permitted which does not comply with these terms.
*Correspondence: Bangera Sheshappa Mamatha, bWFtYXRoYS5ic0BuaXR0ZS5lZHUuaW4=