- 1Department of Physiology, Faculty of Basic Medical Sciences, Federal University of Health Sciences, Ila Orangun, Nigeria
- 2Reproductive Biology and Toxicology Research Laboratory, Oasis of Grace Hospital, Osogbo, Nigeria
- 3Department of Physiology, Ladoke Akintola University of Technology, Ogbomoso, Nigeria
- 4Department of Medical Laboratory Science, Afe Babalola University, Ado Ekiti, Nigeria
- 5The Brainwill Laboratories and Biomedical Services, Osogbo, Nigeria
- 6Department of Physiology, Crescent University, Abeokuta, Nigeria
- 7Department of Physiology, University of Ilorin, Ilorin, Nigeria
Background: Tamoxifen (TAM) is a widely used drug in patients with gynecomastia and breast cancer. TAM exerts its anticancer effects via its antiestrogenic activities. Unfortunately, TAM has been reported to exert gonadotoxic effects on male testes. Therefore, this study was designed to explore the possible associated mechanisms involved in TAM-induced testicular dysfunction and the possible ameliorative effects of omega-3 fatty acids (O3FA).
Methodology: Animals were randomly divided into control, O3FA, TAM, and TAM + O3FA. All treatment lasted for 28 days.
Results: TAM exposure impaired sperm qualities (count, motility, and normal morphology) and decreased testicular 3β-HSD and 17β-HSD. It was accompanied by a decline in serum testosterone and an increase in estradiol, luteinizing and follicle-stimulating hormones. These observed alterations were associated with an increase in testicular injury markers, oxido-inflammatory response, and mitochondria-mediated apoptosis. These observed alterations were ameliorated by O3FA treatments.
Conclusions: O3FA ameliorated TAM-induced testicular dysfunction in male Wistar rats by modulating XO/UA and Nrf2/NF-kb signaling and cytochrome c-mediated apoptosis in TAM-treated rats.
Introduction
Tamoxifen (TAM; Z-1-[4-(2-dimethylaminoethoxy)-phenyl]-1,2-diphenyl-1-butene) is a synthetic nonsteroidal estrogen agonist-antagonist antineoplastic agent (1, 2). TAM is the major anti-estrogen therapy for the management of hormone receptor-positive breast cancer in pre-menopausal women (3). TAM has also been recommended for the management of gynecomastia in males (4). In fact, TAM is recommended for pubertal gynecomastia once it is accompanied by significant pain, irrespective of the disc size (5). TAM is believed to majorly act through its inhibitory effect on estradiol binding at the ligand-binding domain of the estrogen receptor (ER) alpha and blockage of estrogen receptor interaction with co-activator proteins (6, 7). However, TAM has also been shown to act as an estrogen agonist. These dual actions on estrogen could depend on the type of species, cell types, tissue, and organs (8, 9). In humans and rats, TAM primarily exhibits antiestrogenic activities with residual estrogenic effects (10). Apart from these estrogenic effects, TAM also acts via different signaling proteins such as protein kinase C, mitogen-activated protein kinases, and c-jun N-terminal kinase (JNK) and also distorts bcl-2-like protein 4 (BAX)/B-cell lymphoma 2 (BCL-2) ratio. Furthermore, TAM stimulates the mitochondrial permeability transition and cytochrome C release, which eventually results in increased apoptosis (11). With the increasing usage of TAM for the management of gynecomastia (4) and possibly benign prostatic hyperplasia (12), attention has been drawn to its possible testicular toxic effects.
TAM has been shown to impair spermatogenesis and steroidogenesis (13). TAM administration has also been shown to disrupt the hypothalamic-pituitary-gonadal (HPG)-axis (14) responsible for maintaining testicular functions. TAM-induced testicular toxicities could be associated with reactive oxygen species (ROS) generation (15), which are capable of reacting with the cellular DNA, proteins, and lipids to form DNA-adducts, protein crosslink, and lipid peroxidation products (16) in the testis. As a result, these activities can create oxidative stress (redox imbalance), inflammatory response, mitochondrial dysfunction, uncontrolled cell death, and impair testicular cells integrity and functionality. Hence, this study sought to establish a supplement for managing TAM-induced gonadotoxicity in patients who require TAM treatment.
Nutritional supplements can be recommended for the prevention and management of toxicants-induced health disorders. Omega-3 fatty acids (O3FA) is one of these natural supplements that has been shown to possess various pharmacological and biological activities (17, 18). O3FA are essential fatty acids commonly found in plants and marine life. They are referred to as essential fatty acids because they cannot be synthesized in the body; they can only be obtained from diets. O3FA are required for different functions such as growth, brain development, vision, and fertility enhancement (19). O3FA might be performing these functions via its anti-inflammatory (20), anti-oxidant (21, 22), and anti-apoptotic (23) activities.
Despite O3FA's established protective activities, no study has explored its possible ameliorative role on TAM-induced testicular injury. Hence, we hypothesize that O3FA might attenuate TAM-induced testicular toxicity in male Wistar rats. The findings from this study will establish O3FA as a supplement that can be introduced as an adjunct therapy together with TAM.
Materials and methods
Chemicals/reagents
TAM 20 mg was purchased from Milpharm, Ltd, UK, while O3FA was procured from Gujarat Liqui Pharmacaps Pvt. Ltd. Vadodara, Gujarat, India. Each of the O3FA capsules consists of eicosapentaenoic acid (EPA) and docosahexaenoic acid (DHA) in 3:2. All other chemicals used in this study except otherwise stated were of analytical grades and were procured from Sigma (MS, USA).
Ethical consideration
The animals were humanly handled in accordance with the Guidelines for Laboratory Animal Care published by the National Institute of Health (NIH). The experimental protocol complied with the US NAS guidelines, and ethical approval was obtained from the Institutional Ethical Review Committee (UERC/ASN/2022/2396).
Animals
Twenty-four (24) male Wistar rats (aged 10–12 weeks and weighing 180–200 g) were purchased from the Biochemistry Department, University of Ilorin, and housed in standard ventilated cages. The rats were allowed free access to feed and water under a normal 12-h light and darkness cycle.
Experimental procedure
The animals were allowed to acclimatize for 2 weeks before they were randomly divided into 4 groups (n = 6 groups): Group 1: Control (Cntrl), vehicle-treated animals with 0.5 ml of corn oil, Group 2: animals treated with 300 mg/kg of O3FA, Group 4: animals exposed to 0.4 mg/kg of TAM, Group 5: animals co-treated with 0.4 mg/kg of TAM and 300 mg/kg of O3FA. All treatments were via oral gavage and lasted for 28 days. The dose of 0.4 mg/kg used in this study has been earlier reported as the most effective dosage of TAM for antifertility studies (7) and is similar to that of Motrich et al. (1) and Lee et al. (15), while the 300 mg/kg of O3FA was the most effective dosage based on the reports from our previous findings (19, 22).
The study was terminated 24 h after the last treatment, and animals were sacrificed via an intraperitoneal administration of ketamine (40 mg/kg) and xylazine (4 mg/kg) (24). Blood samples were obtained via cardiac puncture and put into plain bottles. The obtained blood samples were centrifuged at 3,000 rpm for 10 min, and the obtained serum was used for hormonal assay. Both testes were removed, and the surrounding tissues were separated. The left testes were homogenized in phosphate buffer for biochemical assays, while the right testes were preserved with bouin solution for histology.
Sperm analysis
Caudal epididymis was meticulously cut into a clean petri dish, and sperm count, motility, and abnormal sperm morphology were estimated based on previous methods (25, 26). Briefly, for sperm motility, cauda epididymis was cut with surgical blade; the spermatozoa released onto a sterile glass slide and then diluted with pre-warmed 2.9% sodium citrate dehydrate solution. The glass slide was covered with a coverslip, and sperm motility was evaluated under microscope by examining at least ten microscopic fields at × 40 magnification. For sperm count, the cauda epididymis was gently crushed in normal saline and filtered with a nylon mesh to obtain the sperm suspension. Five μL of the sperm suspension was mixed with 95 μl of 0.35% formalin containing 0.25% trypan blue and 5% NaHCO3. A fraction (10 μl) of the diluted spermatozoa was placed on the haemocytometer, allowed to sediment for 5 min, and then counted using the Improved Neubauer chamber and a light microscope at × 40. For sperm morphology, The abnormalities in the head, middle-piece and tail (tailless head, bent mid piece, curved mid-piece, headless tail, bent tail, curved tail, looped tail) were counted and classified as documented by Bloom (27) and Parkinson (28).
Steroidogenic enzymes
Testicular 3 beta-hydroxysteroid (3β-HSD) (29, 30) and 17 beta-hydroxysteroid (17 β-HSD) dehydrogenase (30, 31) were estimated as previously established respectively. “For 3β-HSD, testicular tissue was homogenized, and the supernatant was carefully separated. 1 ml of the supernatant was mixed with 1 ml of 100 μmol sodium pyrophosphate buffer (pH 8.9), 30 μg of dehydroepiandrosterone in 40 μl of ethanol, and 960 μl of 25% BSA. The mixture was then incubated and 0.5 μmol of NAD was added. The absorbance was read spectrophotometrically at a wavelength of 340 nm using a blank as reference. For testicular 17β-HSD, 1 ml of the supernatant obtained from the testicular sample was mixed with 1 ml of 440 μmol sodium pyrophosphate buffer (pH 10.2), 40 μl of ethanol containing 0.3 μmol of testosterone, and 960 μl of 25% BSA. The mixture was incubated and 1.1 μmol of NAD was added in a U 2,000 spectrophotometer cuvette at 340 nm against a blank.”
Reproductive hormones
The serum levels of luteinizing hormone (LH), follicle-stimulating hormone (FSH), testosterone, and estradiol (Bio-Inteco, UK) were determined using an ELISA method according to the manufacturer's description.
Testicular histology
Histology was performed according to the established method (32, 33). The preserved testis in bouin solution was dehydrated using ethanol series and cleared with toluene. The cleared testes were embedded and blocked in paraffin wax. After that, 5 μm thick paraffin sections were stained with hematoxylin and eosin (H&E). Testicular biopsy/Johnsen score was estimated as previously described (30, 34).
Testicular injury markers
Testicular lactate dehydrogenase (LDH) and Gamma-glutamyl transferase (GGT) activities were determined as described by the manufacturer (Agape Diagnostics Ltd.). Additionally, testicular lactate concentration was evaluated based on the manufacturer's guideline (EnzyChrom, ELAC-100).
Oxidative stress markers
Testicular malondialdehyde (MDA) level was assayed as previously reported (35, 36). In addition, testicular glutathione (GSH), glutathione peroxidase (GPx), Glutathione-S-transferase (GST), superoxide dismutase (SOD), and catalase (CAT) activities were assayed based on established methods (30, 37, 38).
“Malondialdehyde (MDA), a marker of oxidative stress, was determined as previously documented based on the generated amount of thiobarbituric acid reactive substance (TBARS) during lipid peroxidation. This method involves the reaction between 2- thiobarbituric acid (TBA) and malondialdehyde, a byproduct of lipid peroxidation, by analyzing the pink chromogen complex [(TBA) 2-malondialdehyde adduct] formed upon heating at acidic pH. The sample (200 μl) was first treated with 500 μl of Trichloroacetic acid (TCA) to remove proteins and centrifuged at 3,000 rpm for 10 min. Next, 1 ml of 0.75% TBA was added to 0.1 ml of the supernatant and heated in a water bath at 100°C for 20 min, then cooled with ice water. The absorbance of the sample/standard was then read at 532 nm using a spectrophotometer and compared to a blank. The concentration of TBARS was determined by extrapolating from a standard curve.
For GSH, an aliquot of the sample was deproteinized by adding an equal volume of 4% sulfosalicylic acid, and was centrifuged at 4,000 rpm for 5 min. 0.5 ml of the supernatant was then added to 4.5 ml of Ellman's reagent. A blank was prepared by mixing 0.5 ml of the diluted precipitating agent with 4.5 ml of Ellman's reagent. The level of GSH was calculated by measuring the absorbance at 412 nm.
For catalase, 1:29 dilution of the sample was made by mixing 1 ml of the supernatant of the testicular homogenate with 19 ml of diluted water. 4 ml of H2O2 solution (800 μmoles) and 5 ml of phosphate buffer were added to a 10 ml flat bottom flask. 1 ml of the diluted enzyme preparation was mixed into the reaction mixture by gentle swirling at 37°C. Samples of the reaction mixture were withdrawn at 60 s intervals, and the H2O2 content was determined by blowing 1 ml of the sample into 2 ml dichromate/acetic acid reagent. Catalase levels in the sample were determined by comparing the absorbance at 653 nm to that of a certified catalase standard.
For GPx, the sample was incubated at 37°C for 3 min, then 0.5 ml of 10% trichloroacetic acid (TCA) was added and the mixture was centrifuged at 3,000 rpm for 5 min. The supernatant was then mixed with 2 ml of phosphate buffer and 1 ml of 5′- 5′- dithiobis-2-dinitrobenzoic acid (DTNB) solution, and the absorbance was measured at 412 nm using a blank as reference. The GPx activity was determined by plotting a standard curve and determining the concentration of remaining GSH from the curve.
The activity of glutathione-S-transferase in testicles was also measured. This method utilizes the enzyme's high activity with 1-chloro-2,4-dinitrobenzene as a substrate. The assay was performed at 37°C for 60 s and the absorbance was read at 340 nm after comparing it with a blank sample.
For SOD, a 1:10 dilution of the sample was made using 1 ml of sample and 9 ml of distilled water. 0.2 ml of the diluted sample was added to 2.5 ml of 0.05 M carbonate buffer (pH 10.2) and the reaction was initiated by adding 0.3 ml of freshly prepared 0.3 mM adrenaline. The mixture was mixed and the increase in absorbance was monitored at 480 nm every 30 s for 150 s using a spectrophotometer. A reference cuvette containing 2.5 ml buffer, 0.3 ml substrate (adrenaline), and 0.2 ml water was also used.”
Inflammatory markers
Testicular tumor necrotic factor-alpha (TNF-α) and interleukin 6 (IL-6) were assayed using ELISA kits (Solarbio, China). Also, testicular myeloperoxidase (MPO) was estimated according to previously reported methods (30, 39), while testicular nitric oxide (NO) concentration was determined based on the Griess reaction (40).
Briefly, “the method for measuring MPO is based on the ability of myeloperoxidase to catalyze the oxidation of guaiacol to oxidized guaiacol in the presence of hydrogen peroxide. The oxidized form of guaiacol has a brown color, which is measured spectrophotometrically at a wavelength of 470 nm. The intensity of the color produced is proportional to the concentration of oxidized guaiacol produced in the reaction, thus providing a measure of myeloperoxidase activity.
For NO, a mixture of 100 μl of Griess reagent, 300 μl of a nitrate-containing testicular homogenate, and 2.6 ml of deionized water were incubated for 30 min at room temperature in a spectrophotometer cuvette. A blank was prepared by mixing 100 μl of Griess reagent and 2.9 ml of deionized water. The absorbance of the nitrate-containing sample was measured at 548 nm in relation to the reference sample.”
Xanthine oxidase/uric acid
The activities of testicular xanthine oxidase (XO) were determined based on the method of Zahide and Bahad (41), while a colorimetric method was used for uric acid (UA) concentration (Precision, UK).
Transcriptional factors
Testicular nuclear factor kappa B (NFkB) and nuclear factor erythroid 2-related factor 2 (Nrf2) levels were determined using the ELISA method (Elabscience Biotechnology Inc., USA).
Apoptotic markers
Testicular BCl-2, cytochrome C, and caspase 3 activities were determined by an ELISA method as described by the manufacturer (Elabscience Biotechnology Inc., USA). At the same time, the testicular DNA Fragmentation Index (DFI) was estimated according to the method of Perandones et al. (42). Five ml each of testicular homogenate supernatant and pellet were treated with 3 ml of freshly prepared diphenylamine (DPA) reagent for color development. The solution was incubated at 37°C for 16 to 24h. The absorbance of light green/yellowish-green supernatant was read spectrophotometrically at 620 nm. The percentage of fragmented DNA was calculated by dividing the absorbance of the homogenate supernatant by the sum of the absorbance of the pellet and the absorbance of the supernatant.
Statistical analysis
Data were analyzed using a one-way analysis of variance (ANOVA) followed by Tukey's post hoc test using GraphPad PRISM 5 software, and they were reported as mean ± standard deviation. Also, all P values below 0.05 were classified as statistically significant.
Results
As shown in Figure 1, TAM exposure led to a significant decrease in sperm count (p < 0.0001), motility (p < 0.0001), and an increase in abnormal morphology (p < 0.0001) compared with the control and O3FA groups. These impaired sperm qualities were ameliorated by O3FA treatment.
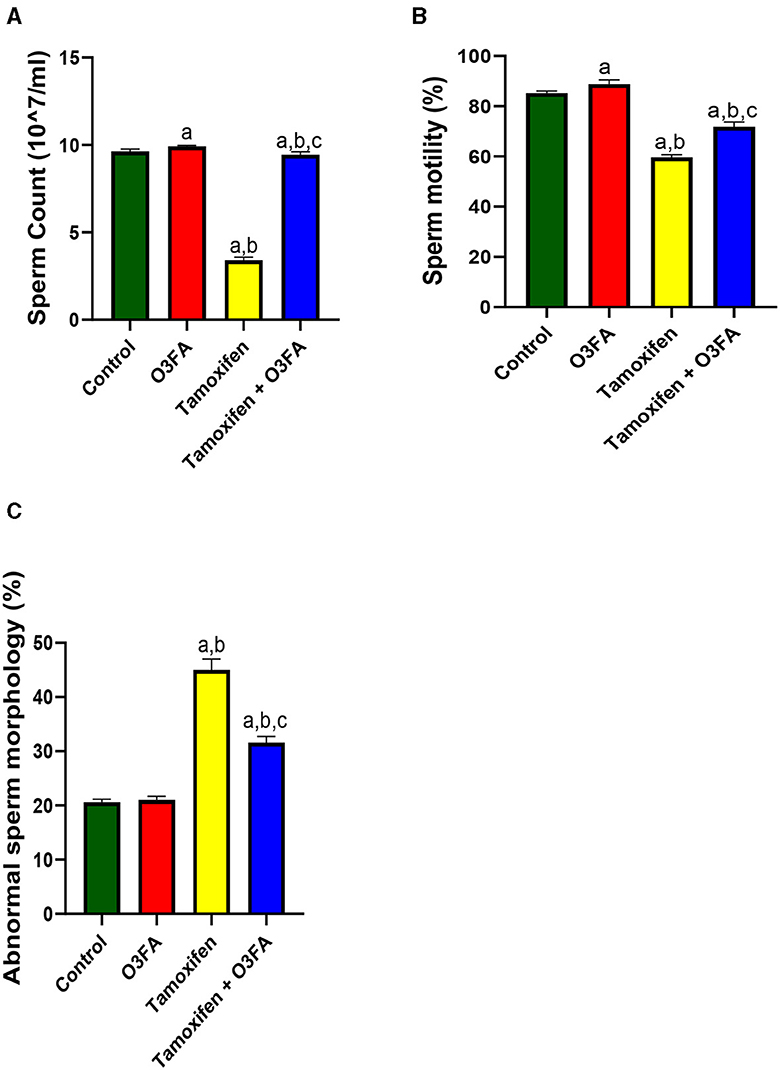
Figure 1. Effect of O3FA on sperm (A) count (B) motility (C) abnormal morphology in TAM exposed rats. aP < 0.05 vs. control, bP < 0.05 vs. O3FA; cP < 0.05 vs. TAM. Data were analyzed by one way ANOVA and Tukey's post-hoc test. O3FA, Omega-3 fatty acids; TAM, Tamoxifen.
In the same vein, TAM administration impaired steroidogenic enzymatic activities, evidenced by a significant decrease in 3β-HSD (p < 0.0001) and 17β-HSD (p < 0.0001) compared with the control and O3FA groups (Figure 2). These observed decreases were abrogated in animals in the TAM+ O3FA group.
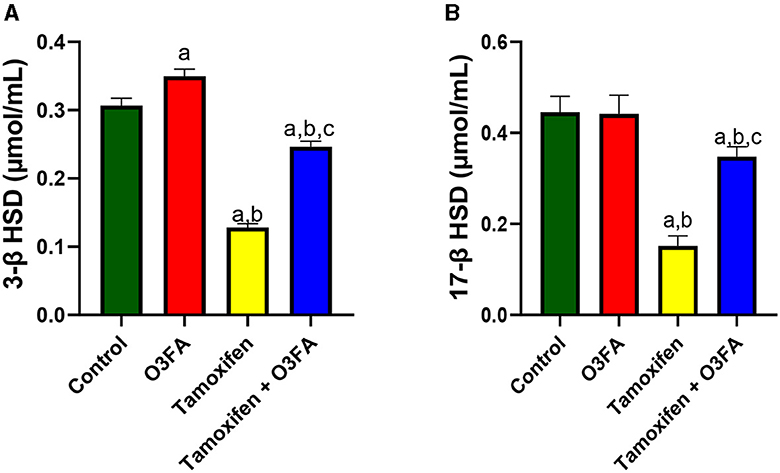
Figure 2. Effect of O3FA on testicular (A) 3β-HSD (B) 17β-HSD in TAM exposed rats. aP < 0.05 vs. control, bP < 0.05 vs. O3FA; cP < 0.05 vs. TAM. Data were analyzed by one way ANOVA and Tukey's post-hoc test. O3FA, Omega-3 fatty acids; TAM Tamoxifen; 3β-HSD, 3 beta-hydroxysteroid dehydrogenase; 17β-HSD, 17 beta-hydroxysteroid dehydrogenase.
Furthermore, compared with the control groups, animals administered with TAM had a significant increase in serum LH (p < 0.0001), FSH (p < 0.0001), and estradiol and a decrease in testosterone (Figure 3). TAM and O3FA co-administration blunted these observed hormonal imbalances.
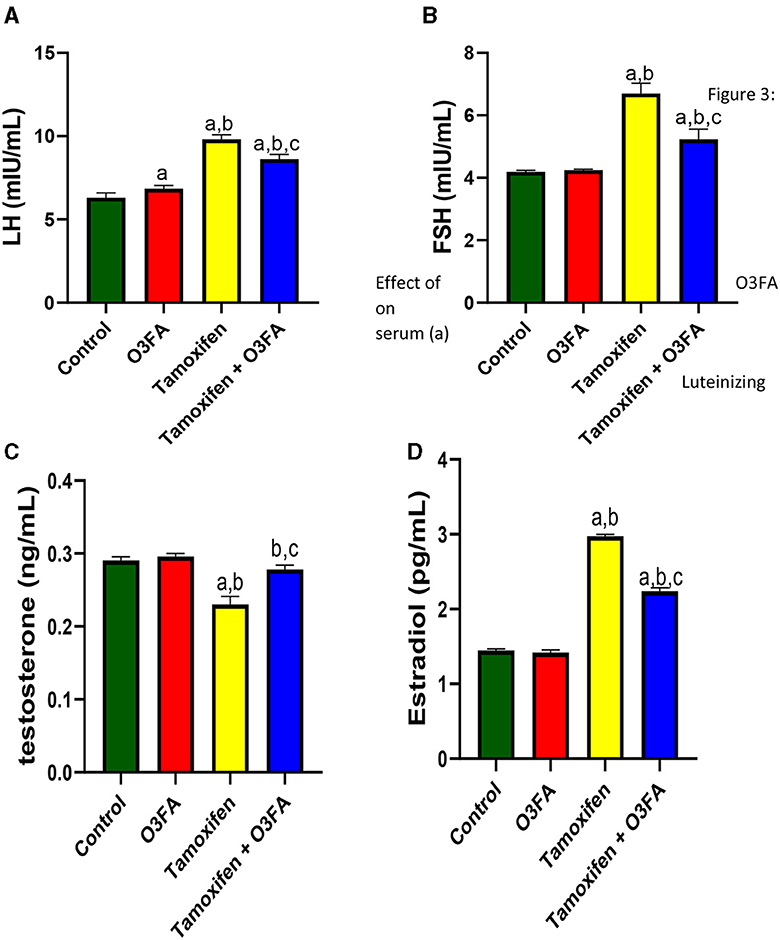
Figure 3. Effect of O3FA on serum (A) Luteinizing hormone (LH) (B) Follicle stimulating hormone (FSH) (C) testosterone (D) estradiol in TAM exposed rats. aP < 0.05 vs. control, bP < 0.05 vs. O3FA; cP < 0.05 vs. TAM. Data were analyzed by one way ANOVA and Tukey's post-hoc test. O3FA, Omega-3 fatty acids; TAM, Tamoxifen.
Histopathological findings revealed features consistent with normal testicular tissue of animals in the control, O3FA, and TAM + O3FA groups, while their counterparts in the TAM group exhibited histological features that suggest cellular reaction to injury and inflammatory response (Figure 4). Also, a decrease in Johnsen score was observed in TAM-exposed animals compared with the controls (Figure 5). This alteration was ameliorated in animals that received TAM and O3FA co-treatment.
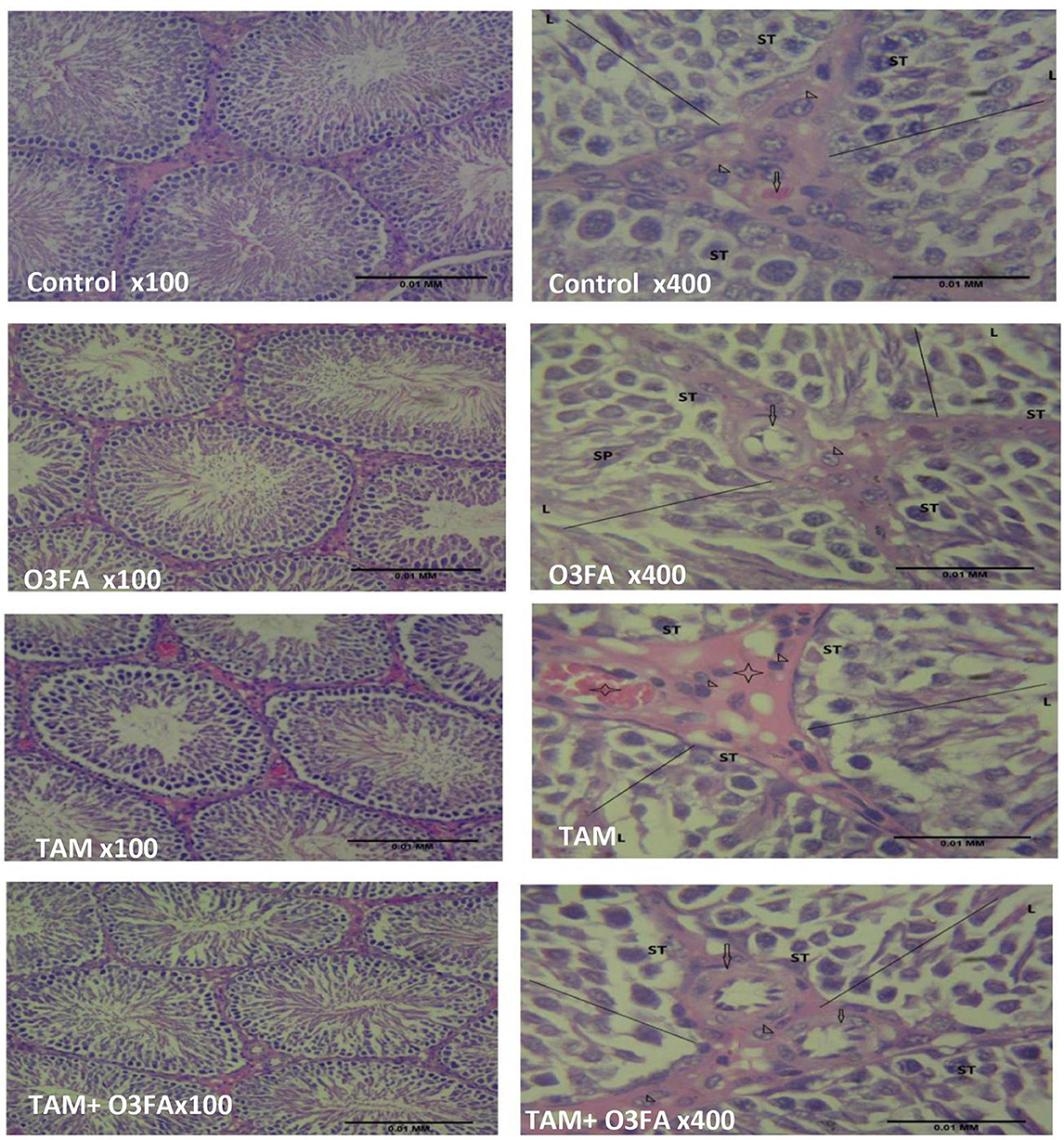
Figure 4. Testicular histology. Control, O3FA, and TAM + O3FA: section shows the testicular tissue composed of coils of seminiferous tubules (ST) with a defined lumen (L) containing sperm cells (SP), the seminiferous tubules contained germinal epithelium with germ cells at varying degree of maturation (line). The interstitium, contained blood vessel (arrow) which is free of collection and contained interstitial cells of Leydig (arrow head) appearing unremarkable. TAM, The blood vessels (black star) within the interstitium (plain star) appeared congested, and the interstitial cells of Leydig (arrow head) appears unremarkable.
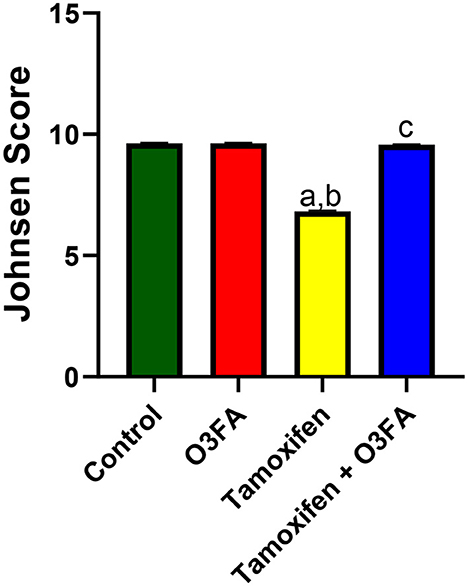
Figure 5. Effect of O3FA on Johnsen Score in TAM exposed rats. aP < 0.05 vs. control, bP < 0.05 vs. O3FA; cP < 0.05 vs. TAM. Data were analyzed by one way ANOVA and Tukey's post-hoc test. O3FA, Omega 3 fatty acids; TAM, Tamoxifen.
Additionally, TAM administration led to a significant increase in testicular lactate, LDH, and GGT and a decrease in testicular SDH (Figure 6) compared with the controls. These observed increases in testicular injury markers were ameliorated in animals treated with TAM and O3FA.
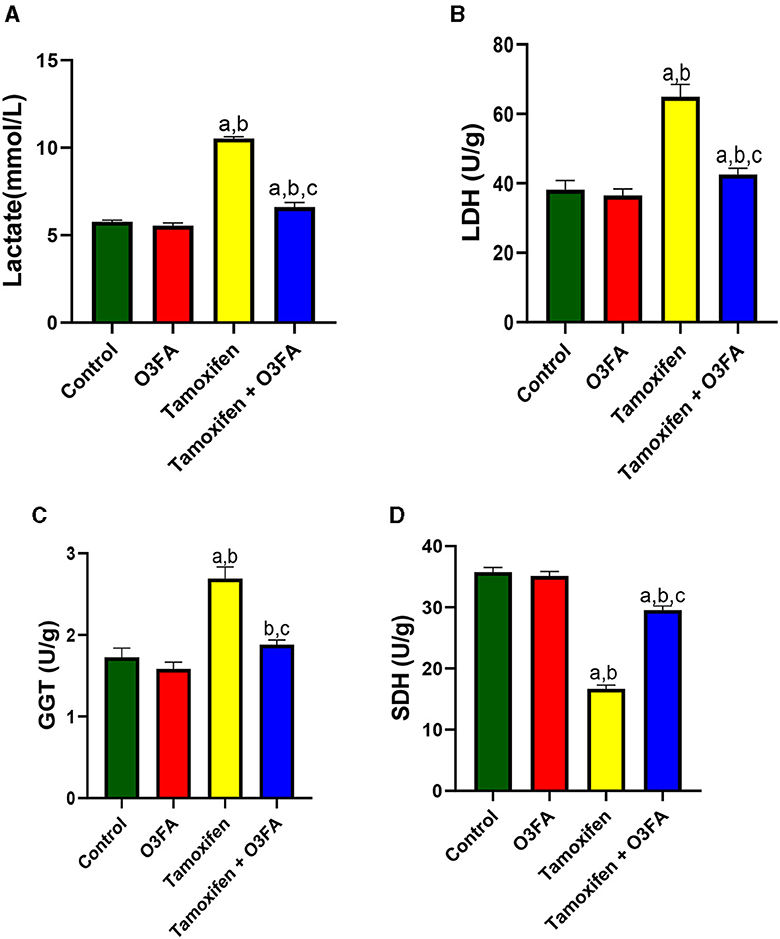
Figure 6. Effect of O3FA on testicular (A) lactate (B) lactate dehydrogenase (LDH) (C) Gamma-glutamyl transferase (GGT) (D) sorbitol dehydrogenase (SDH) in TAM exposed rats. aP < 0.05 vs. control, bP < 0.05 vs. O3FA; cP < 0.05 vs. TAM. Data were analyzed by one way ANOVA and Tukey's post-hoc test. O3FA, Omega 3 fatty acids; TAM, Tamoxifen.
Also, Figure 7 showed that TAM administration led to a significant increase in testicular MDA and a decrease in testicular SOD, CAT, GSH, GPX, and GST. This observed redox imbalance was abrogated in animals that received O3FA and TAM co-treatment.
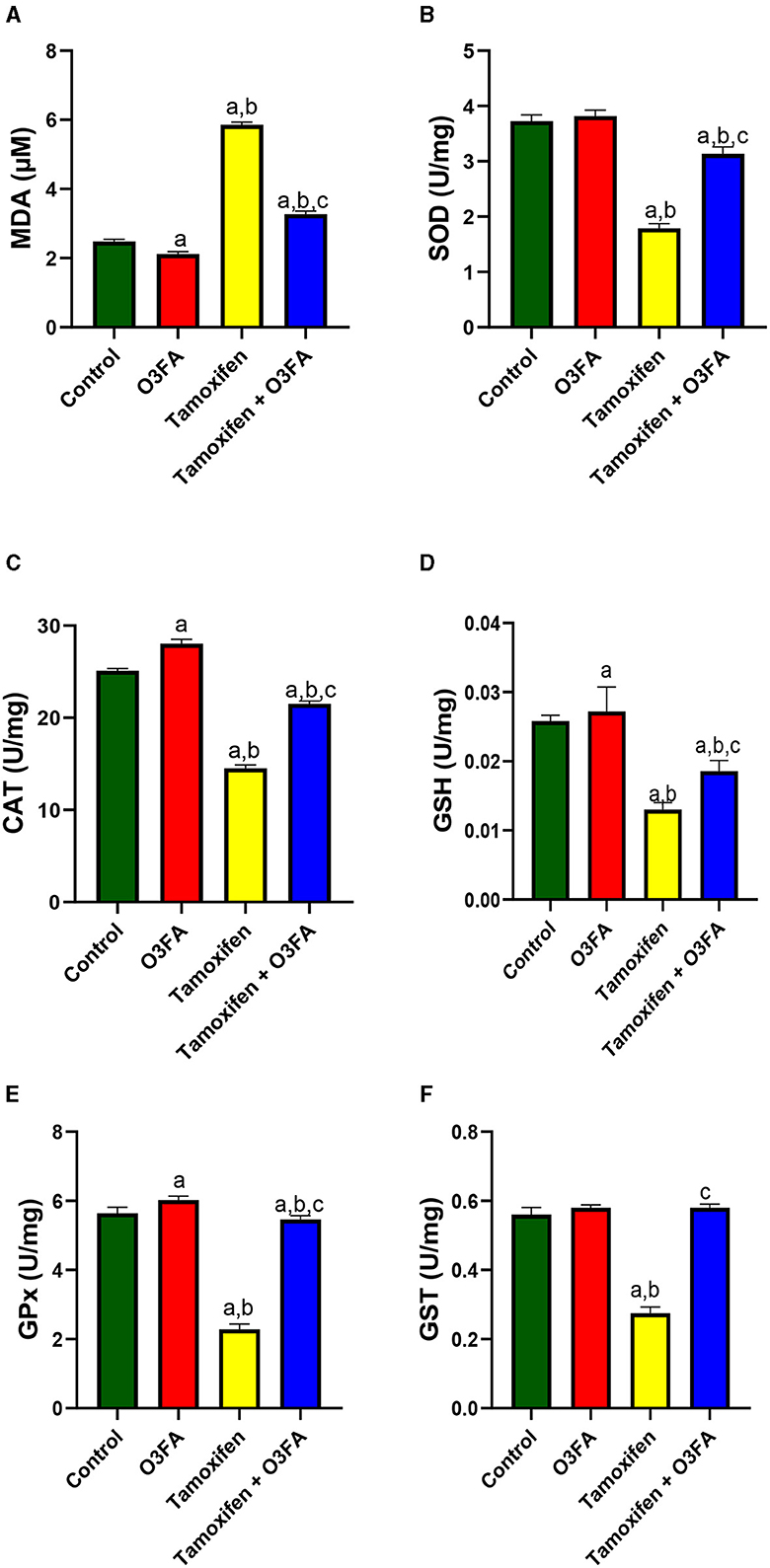
Figure 7. Effect of O3FA on testicular (A) malondialdehyde (MDA) (B) superoxide dismutase (SOD) (C) catalase (CAT) (D) glutathione (GSH) (E) glutathione peroxidase (GPx) (F) Glutathione-S-transferase (GST) in TAM exposed rats. aP < 0.05 vs. control, bP < 0.05 vs. O3FA; cP < 0.05 vs. TAM. Data were analyzed by one way ANOVA and Tukey's post-hoc test. O3FA, Omega 3 fatty acids; TAM, Tamoxifen.
Similarly, TAM treatment significantly led to an increase in testicular TNF-α, IL-6, MPO, and NO compared with the control groups (Figure 8). These observed TAM-induced inflammatory responses were ameliorated in animals treated with TAM and O3FA.
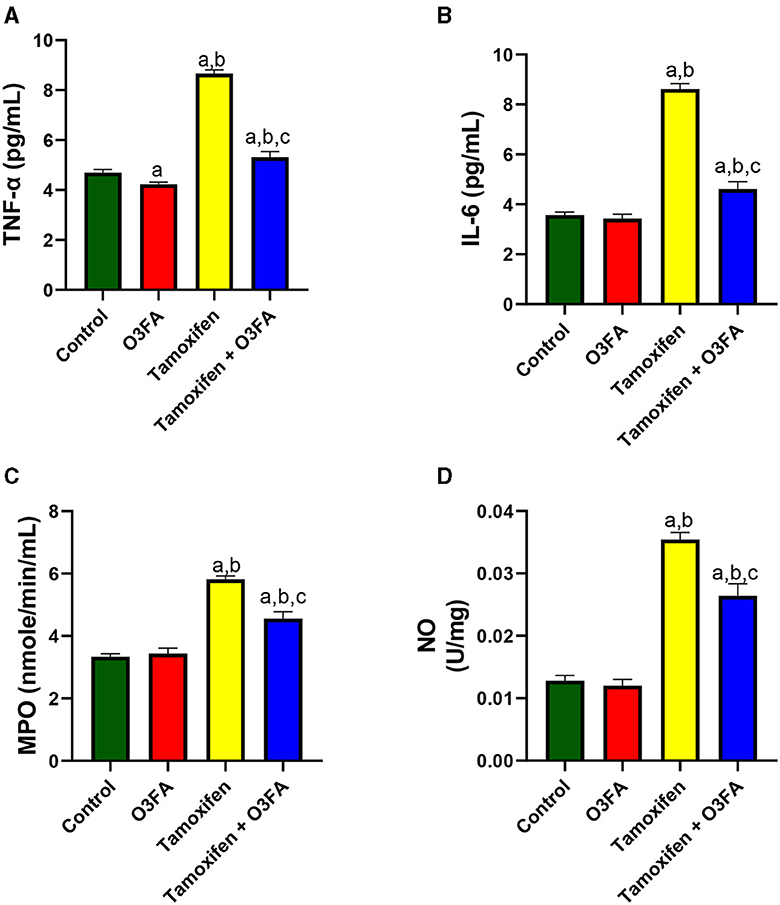
Figure 8. Effect of O3FA on testicular (A) tumor necrotic factor-alpha (TNF-α) (B) interleukin 6 (IL-6) (C) myeloperoxidase (MPO) (D) nitric oxide (NO) in TAM exposed rats. aP < 0.05 vs. control, bP < 0.05 vs. O3FA; cP < 0.05 vs. TAM. Data were analyzed by one way ANOVA and Tukey's post-hoc test. O3FA, Omega 3 fatty acids; TAM, Tamoxifen.
Furthermore, testicular XO and UA were significantly elevated in animals treated with TAM compared with the control (Figure 9). These observed TAM-induced XO/UA signaling distortions were blunted in animals treated with TAM and O3FA.
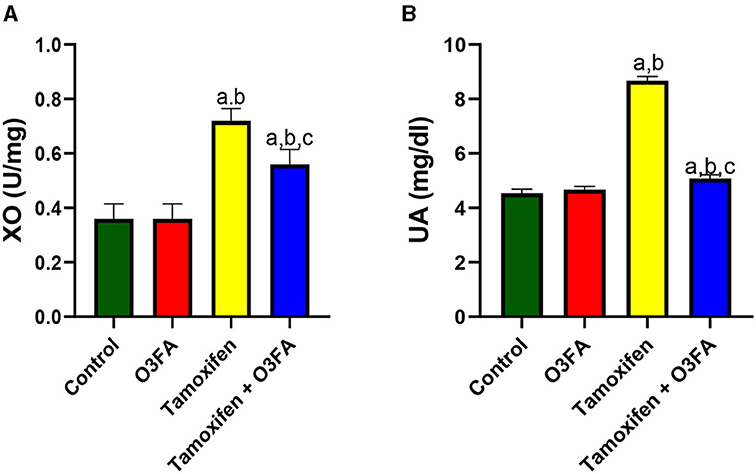
Figure 9. Effect of O3FA on testicular (A) Xanthine oxidase (XO) (B) uric acid (UA) in TAM exposed rats. aP < 0.05 vs. control, bP < 0.05 vs. O3FA; cP < 0.05 vs. TAM. Data were analyzed by one way ANOVA and Tukey's post-hoc test. O3FA, Omega 3 fatty acids; TAM, Tamoxifen.
Additionally, TAM administration led to a significant decrease in testicular Nrf2 and an increase in testicular Nf-κB compared with the control groups (Figure 10). This observed TAM-induced Nrf2/Nf-κB signaling distortion in TAM-treated animals was ameliorated in their counterparts treated with TAM and O3FA.
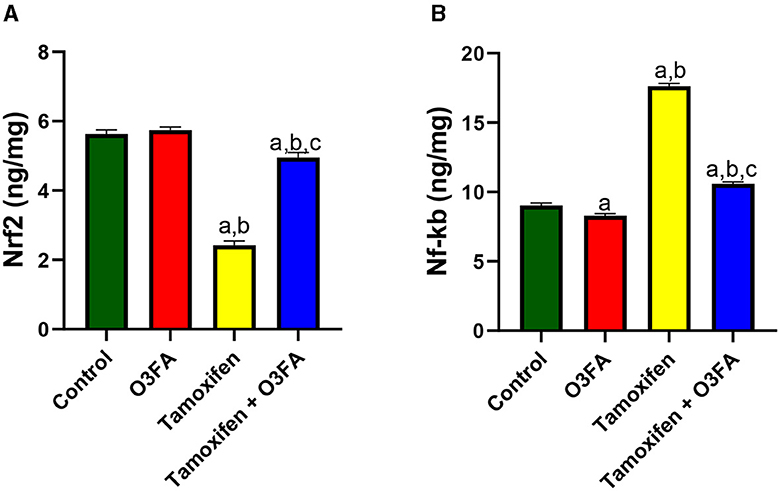
Figure 10. Effect of O3FA on testicular (A) Nrf2 (B) Nf-kb in TAM exposed rats. aP < 0.05 vs. control, bP < 0.05 vs. O3FA; cP < 0.05 vs. TAM. Data were analyzed by one way ANOVA and Tukey's post-hoc test. O3FA, Omega 3 fatty acids; TAM, Tamoxifen.
Finally, TAM exposure led to a significant increase in testicular cytochrome C, BCl-2, caspase 3, and DFI compared with the animals in the control group (Figure 11). TAM and O3FA co-treatment blunted this observed increase in apoptotic markers.
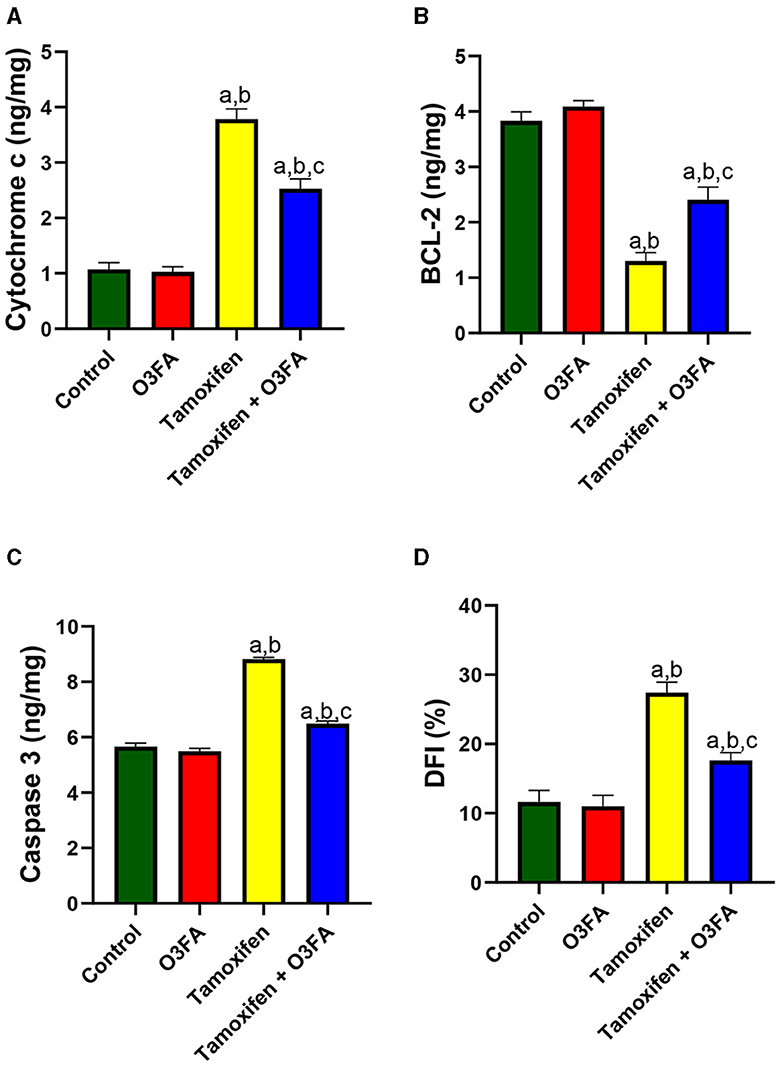
Figure 11. Effect of O3FA on testicular (A) cytochrome c (B) B-cell lymphoma 2 (BCl-2) (C) caspase 3 (D) DNA fragmentation index (DFI) in TAM exposed rats. aP < 0.05 vs. control, bP < 0.05 vs. O3FA; cP < 0.05 vs. TAM. Data were analyzed by one way ANOVA and Tukey's post-hoc test. O3FA, Omega 3 fatty acids; TAM, Tamoxifen.
Discussion
It has been sufficiently established that estrogen plays a major role in male reproductive system development and maintenance. In fact, the presence of ER α and β in the testicular (43, 44) and sperm cells (45, 46) indicates the cognate role of estrogen in testicular functions. Supportively, Korach (47) reported impaired testicular functions in ER α and β knockout mice. TAM is a potent nonsteroidal antiestrogen that has been recommended for managing breast cancer and gynecomastia. In fact, it has been recommended for treating idiopathic oligospermia despite insufficient data on its effectiveness (48, 49). However, these authors did not compare their findings with a placebo control, which is a fundamental aspect to consider when testing the real therapeutic effect of a drug. In fact, Rolf et al. (50) concluded that the beneficial role of TAM may not justify its side effects in healthy males after reviewing 29 clinical trials involving 1,586 patients. TAM has been reported to negatively impact male fertility status in different strains, including rats, monkeys, and dogs (1). Although different studies have studied the antiestrogenic and estrogenic effects of TAM, information on its effect on oxido-inflammatory response and apoptosis on testicular tissue is still lacking. Hence, we investigated the putative gonadotoxic effects of TAM and the possible role of redox imbalance, inflammation, and apoptotic response in TAM-induced testicular dysfunction. Also, we explored the possible ameliorative effect of O3FA on TAM-induced gonadotoxicity.
Our findings revealed that O3FA treatment inhibited the impaired sperm quality, steroidogenesis, HPG-axis, and surge in testicular injury markers following TAM exposure. These histological and biochemical events were accompanied by O3FA-induced amelioration of TAM-mediated distortion of Nrf2/Nf-κb signaling and the consequent redox balance, the suppression of inflammatory response, and cytochrome C-mediated apoptosis.
In this study, TAM administration led to a significant decrease in sperm motility, count, and normal morphology. This impaired sperm quality was accompanied by a significant decline in serum testosterone and steroidogenic enzymatic activities, which are in tandem with previous reports (13). Different mechanisms might be responsible for the observed spermatogenesis and steroidogenesis impairment. TAM might impair testicular function by disrupting the HPG axis activities or via direct testicular damage. In male reproduction, the hypothalamus is responsible for secreting GnRH, which stimulates LH and FSH secretion from the pituitary gland, which also stimulates the testis. The secreted LH stimulates steroidogenesis (testosterone and estrogen secretion), while FSH stimulates spermatogenesis. Additionally, testosterone and estrogen also play a dominant role in spermatogenesis. Testosterone and estrogens, in turn, inhibit the synthesis of the gonadotropins at the level of the pituitary or directly inhibit GnRH secretion from the hypothalamus (51). Thus, the disruption of the HPG axis activities at any level will directly impair testicular functions (spermatogenesis and steroidogenesis). The fact that TAM exposure led to a significant increase in gonadotropins (LH and FSH) might suggest that TAM-induced testicular dysfunction could be independent of the HPG axis activities rather than via direct testicular damage since circulatory LH was unable to stimulate the gonad (testis) to synthesize testosterone.
The fact that TAM exposure disrupted the normal testicular cytoarchitecture supports our claim that TAM might impair testicular function via direct testicular damage. Also, the increase in testicular injury markers (Lactate, LDH, GGT, and SDH) following TAM exposure further substantiates our claim. Additionally, spermatogenesis is a complex process that requires energy balance (52). Unfortunately, the observed increase in lactate is a marker of energy imbalance (53), which is an indication of impaired spermatogenesis and testicular degeneration (22). These findings corroborated previous findings of Marek et al. (54), who reported that TAM activities are associated with energy imbalance and an increase in lactate.
This direct testicular damage could result from oxidative stress or redox imbalance, which plays a key role in testicular functions (55, 56). Oxidative stress occurs when there is an imbalance between pro-oxidant generation and antioxidant activities. Oxidative stress, on the other hand, can stimulate different transcription factors to activate inflammatory pathways (57, 58). TAM treatment could impair testicular function via its oxido-inflammatory activities evidenced by an increase in testicular MDA, IL-6, TnF-a, MPO, and NO and a decrease in CAT, SOD, GSH, GST, and GPx. These observed TAM-induced oxido-inflammatory responses could be mediated by the increase in XO/UA signaling. An increase in XO and the consequent increase in UA has been implicated in lipid peroxidation (59). Although UA is an antioxidant, it becomes a pro-oxidant once produced in excess (60), thereby generating excessive ROS. Excessive ROS can overwhelm Nrf2 activities, the endogenous transcription factor responsible for maintaining redox balance (35). The consequent redox imbalance might activate Nf-κb, responsible for increasing pro-inflammatory gene induction, leading to an inflammatory response (61). The increase in Nf-κb will further inhibit Nrf2 activities, thereby leading to a further decline in the endogenous antioxidant activities. This observed XO/UA and Nrf2/Nf-κb-mediated oxido-inflammatory response following tamoxifen exposure agreed with the study of Ahmed et al. (62) and Schieber and Chandel (63), who reported that TAM can impair cellular functions via oxidative stress.
Additionally, excessive oxidative stress and inflammatory response can collaborate to stimulate apoptosis (64), which is another key factor that can be responsible for TAM-induced testicular dysfunction. The observed increase in testicular cytochrome c following TAM exposure could account for the observed TAM-induced apoptotic response. In mammals, the cytochrome c-initiated pathway is a key caspase activation pathway (65). Various apoptotic stimuli can stimulate the release of cytochrome c from the mitochondria, leading to a series of biochemical reactions that activate caspase and the consequent cell death. Mitochondria plays a major role in the redistribution of cytochrome c (66). Also, the anti-apoptotic protein (BCl-2) located predominantly at the outer mitochondria membrane assists in blocking Δψm reduction and cytochrome c release (67). Hence, during mitochondrial dysfunction, there is a leakage of cytochrome c from the mitochondria and a decrease in BCl-2 (66), thereby leading to caspase 3- 3-mediated apoptosis (68). Hence, it is plausible that the observed increase in testicular caspase 3 and DFI and decrease in testicular BCl-2 might be associated with the leakage of cytochrome c from the mitochondria of TAM-treated rats. Our guess that TAM disrupts testicular function via mitochondria dysfunction-mediated apoptosis corroborates the findings of Unten et al. (69) and Nazarewicz et al. (70).
Another key finding from this study is the therapeutic potential of O3FA against TAM-induced testicular dysfunction. This study revealed that O3FA ameliorated TAM-induced testicular dysfunction by decreasing testicular injury markers and oxido-inflammatory and apoptotic response, thus improving spermatogenesis, sperm quality, hormone synthesis, and testicular histoarchitecture. These findings agreed with previous studies that established the antioxidant (71), anti-inflammatory (22), and anti-apoptotic (72) effects of O3FA. Hence, it is safe to infer that the increase in testicular SOD, CAT, GSH, GPX, and GST and decrease in TNF-a, IL-6, MPO, and NO of TAM exposed rats showed that O3FA –driven repression UA release via XO activities downregulation probably modulated the Nrf2/Nf-κb signaling, thereby inhibiting the transcription of genes responsible for encoding pro-inflammatory cytokines and oxidative response. Furthermore, the observed increase in BCl-2 and decrease in caspase 3 and DFI in O3FA and TAM co-treated rats could also be associated with O3FA-associated decline in cytochrome c release from the mitochondria.
Conclusion
The findings from this study revealed that O3FA ameliorated impaired sperm quality, hormonal imbalance, oxido-inflammatory response, and apoptosis by modulating XO/UA and Nrf2/NF-kb signaling and cytochrome c-mediated apoptosis in TAM-treated rats. These findings suggests the combination therapy with TAM and O3FA in the management of gynecomastia and breast cancer, since O3FA can help alleviate the side effects associated with TAM with respect to male fertility.
Limitations
This study was conducted in healthy animals and we suggest a replica of it in gynecomastia subjects receiving TAM treatment. Additionally, downstream target genes responsible for maintain oxido-inflammatory response and apoptosis were not estimated using real-time PCR, western blot, immunohistochemistry, or TUNEL analysis (for apoptosis). However, the observed modulation of XO/UA and Nrf2/NF-kb and cytochrome c-mediated apoptosis accompanied by oxido-inflammatory response suggests the involvement of these pathways in TAM-induced testicular dysfunction.
Data availability statement
The raw data supporting the conclusions of this article will be made available by the authors, without undue reservation.
Ethics statement
The animal study was approved by the University of Ilorin Ethical Review Committee. The study was conducted in accordance with the local legislation and institutional requirements.
Author contributions
AO: Conceptualization, Data curation, Formal analysis, Funding acquisition, Investigation, Methodology, Project administration, Resources, Software, Supervision, Validation, Visualization, Writing – original draft, Writing – review & editing. RA: Formal analysis, Investigation, Methodology, Project administration, Supervision, Validation, Visualization, Writing – review & editing. MH: Formal analysis, Funding acquisition, Investigation, Methodology, Project administration, Resources, Supervision, Validation, Visualization, Writing – review & editing. MB: Methodology, Project administration, Supervision, Validation, Visualization, Writing – review & editing. DO: Methodology, Project administration, Supervision, Visualization, Writing – review & editing. LO: Methodology, Project administration, Resources, Supervision, Validation, Visualization, Writing – review & editing.
Funding
The author(s) declare that no financial support was received for the research, authorship, and/or publication of this article.
Conflict of interest
The authors declare that the research was conducted in the absence of any commercial or financial relationships that could be construed as a potential conflict of interest.
Publisher's note
All claims expressed in this article are solely those of the authors and do not necessarily represent those of their affiliated organizations, or those of the publisher, the editors and the reviewers. Any product that may be evaluated in this article, or claim that may be made by its manufacturer, is not guaranteed or endorsed by the publisher.
References
1. Motrich RD, Ponce AA, Rivero VE. Effect of tamoxifen treatment on the semen quality and fertility of the male rat. Fertil Steril. (2007) 88:452–61. doi: 10.1016/j.fertnstert.2006.11.196
2. Kavishahi NN, Rezaee A, Jalalian S. The impact of miRNAs on the efficacy of tamoxifen in breast cancer treatment: a systematic review. Clin Breast Cancer. (2024) 24:341–50. doi: 10.1016/j.clbc.2024.01.015
3. De Oliveira Andrade F, Yu W, Zhang X, Carney E, Hu R, Clarke R, et al. Effects of Jaeumkanghwa-tang on tamoxifen responsiveness in preclinical ER+ breast cancer model. Endocr Relat Cancer. (2019) 26:339–53. doi: 10.1530/ERC-18-0393
4. Senkoro E, Varadarajan M, Candela C, Gebreselassie A, Antoniadi C, Boffito M. Anastrozole as a therapeutic option for gynecomastia in a person receiving antiretroviral therapy: Case report. Br J Clin Pharmacol. (2024) 90:350–3. doi: 10.1111/bcp.15951
5. Sabanci E, Pehlivantürk-Kizilkan M, Akgül S, Derman O, Kanbur N. Tamoxifen treatment for pubertal gynecomastia: when to start and how long to continue. Breast Care. (2023) 18:249–55. doi: 10.1159/000530408
6. Singh B, Bhat NK, Bhat HK. Partial inhibition of estrogen-induced mammary carcinogenesis in rats by tamoxifen: balance between oxidant stress and estrogen responsiveness. PLoS ONE. (2011) 6:e25125. doi: 10.1371/journal.pone.0025125
7. Gill-Sharma M, Balasinor N, Parte P, Aleem M, Juneja H. Effects of tamoxifen metabolites on fertility of male rat. Contraception. (2001) 63:103–9. doi: 10.1016/S0010-7824(01)00178-0
8. Hoffmann B, Schuler G. Receptor blockers - general aspects with respect to their use in domestic animal reproduction. Animal Reproduction Sci. (2000) 60:295–312. doi: 10.1016/S0378-4320(00)00129-9
9. Corrada Y, Arias D, Rodríguez R, Spaini E, Fava F, Gobello C. Effect of tamoxifen citrate on reproductive parameters of male dogs. Theriogenology. (2004) 61:1327–41. doi: 10.1016/j.theriogenology.2003.07.020
10. Furr BJA, Jordan VC. The pharmacology and clinical uses of tamoxifen. Pharmacol Ther. (1984) 25:127–205. doi: 10.1016/0163-7258(84)90043-3
11. Owumi SE, Anaikor RA, Arunsi UO, Adaramoye OA, Oyelere AK. Chlorogenic acid co-administration abates tamoxifen-mediated reproductive toxicities in male rats: An experimental approach. J Food Biochem. (2021) 45:e13615. doi: 10.1111/jfbc.13615
12. Chen B, Bai JL, Zhang SS, Fu W. An experimental study of the inhibition of tamoxifen on rat model of benign prostatic hyperplasia. National J Androl. (2002) 8:98–102.
13. Verma R, Krishna A. Effect of tamoxifen on spermatogenesis and testicular steroidogenesis. Biochem Biophys Res Commun. (2017) 486:36–42. doi: 10.1016/j.bbrc.2017.02.092
14. Mitwally M, Casper R, Diamond M. Oestrogen-selective modulation of FSH and LH secretion by pituitary gland. Br J Cancer. (2005) 92:416–7. doi: 10.1038/sj.bjc.6602292
15. Lee S, Lee MS, Park J, Zhang JY, Jin DI. Oxidative stress in the testis induced by tamoxifen and its effects on early embryo development in isogenic mice. J Toxicol Sci. (2012) 37:675–9. doi: 10.2131/jts.37.675
17. Oktar S, Karadeniz M, Acar M, Zararsiz I. The effects of omega-3 fatty acids on antioxidant enzyme activities and nitric oxide levels in the cerebral cortex of rats treated ethanol. Biomed Khim. (2024) 70:83–8. doi: 10.18097/pbmc20247002083
18. Xia Y, Zhang Y, Li Y, Li X, Wu Y, Yao Q. Omega-3 polyunsaturated fatty acids play a protective role in a mouse model of Parkinson's disease by increasing intestinal inducible Treg cells. Cell Mol Biol. (2024) 70:107–12. doi: 10.14715/cmb/2024.70.4.17
19. Odetayo AF, Olayaki LA. Omega-3 fatty acid improves sexual and erectile function in BPF-treated rats by upregulating NO/cGMP signaling and steroidogenic enzymes activities. Sci Rep. (2023) 13:18060. doi: 10.1038/s41598-023-45344-4
20. Akhigbe RE, Hamed MA, Odetayo AF, Akhigbe TM, Ajayi AF, Ajibogun F. Omega-3 fatty acid rescues ischaemia/perfusion-induced testicular and sperm damage via modulation of lactate transport and xanthine oxidase/uric acid signaling. Biomed Pharmacother. (2021) 142:111975. doi: 10.1016/j.biopha.2021.111975
21. Tian A, Zheng Y, Li H, Zhang Z, Du L, Huang X, et al. Eicosapentaenoic acid activates the P62/KEAP1/NRF2 pathway for the prevention of diabetes-associated cognitive dysfunction. Food Funct. (2024) 15:5251–71. doi: 10.1039/D4FO00774C
22. Odetayo AF, Adeyemi WJ, Olayaki LA. Omega-3 fatty acid ameliorates bisphenol F-induced testicular toxicity by modulating Nrf2/NFkB pathway and apoptotic signaling. Front Endocrinol. (2023) 14:1256154. doi: 10.3389/fendo.2023.1256154
23. Elsafty M, Abdeen A, Aboubakr M. Allicin and Omega-3 fatty acids attenuates acetaminophen mediated renal toxicity and modulates oxidative stress, and cell apoptosis in rats. Naunyn-Schmiedeberg's Arch Pharmacol. (2024) 397:317–28. doi: 10.1007/s00210-023-02609-z
24. Okesina KB, Odetayo AF, Adeyemi WJ, Ajibare AJ, Okesina AA, Olayaki LA. Naringin from sweet orange peel improves testicular function in high fat diet-induced diabetic rats by modulating xanthine oxidase/uric acid signaling and maintaining redox balance. Lab Anim Res. (2024) 40:5. doi: 10.1186/s42826-024-00188-5
25. Akhigbe RE, Hamed MA, Odetayo AF. HAART and anti-Koch's impair sexual competence, sperm quality and offspring quality when used singly and in combination in male Wistar rats. Andrologia. (2021) 53:e13951. doi: 10.1111/and.13951
26. Fatai OA, Aribidesi OL. Effect of bisphenol F on sexual performance and quality of offspring in Male Wistar rats. Ecotoxicol Environ Saf. (2022) 244:114079. doi: 10.1016/j.ecoenv.2022.114079
27. Bloom E. The ultrastructure of some characteristic sperm defects. Nord Vet Med. (1973) 12:125–39.
28. Parkinson T. Fertility and infertility in male animals. In:Noakes DE, Parkinson TJ, England GCW., , editors Arthur's Veterinary Reproduction andObstetrics, 8th ed. Saunders Publishers (2001). p. 695–750. doi: 10.1016/B978-070202556-3.50034-7
29. Talalay P. Enzymatic analysis of steroid hormone methods. Biochem Anal. (1960) 8:119. doi: 10.1002/9780470110249.ch3
30. Odetayo AF, Adeyemi WJ, Olayaki LA. In vivo exposure to bisphenol F induces oxidative testicular toxicity: role of Erβ and p53/Bcl-2 signaling pathway. Front Reprod Health. (2023) 5:1204728. doi: 10.3389/frph.2023.1204728
31. Jarabak J, Adams JA, Williams-Ashman HG, Talalay PJ. Purification of a 17betahydroxysteroid dehydrogenase of human placenta and studies on its transhydrogenase function. Biol Chem. (1962) 237:345–335. doi: 10.1016/S0021-9258(18)93926-8
32. Oluwasola A, Ayoola OE, Odetayo AF, Saa'du G, Olayaki LA. Ameliorative effect of melatonin on reproductive hormones in ethanol extracts of cannabis sativa-treated female wistar Rats. Soc Exp Biol Nigeria. (2023) 22:53–8.
33. Ulusoy E, Cayan S, Yilmaz N, Aktaş S, Acar D, Doruk E. Interferon alpha-2b may impair testicular histology including spermatogenesis in a rat model. Arch Androl. (2004) 50:379–85. doi: 10.1080/01485010490474823
34. Johnsen SG. Testicular biopsy score count–a method for registration of spermatogenesis in human testes: normal values and results in 335 hypogonadal males. Hormones. (1970) 1:2–25. doi: 10.1159/000178170
35. Ajibare AJ, Odetayo AF, Akintoye OO, Olayaki LA. Zinc ameliorates acrylamide-induced oxidative stress and apoptosis in testicular cells via Nrf2/HO-1/NfkB and Bax/Bcl2 signaling pathway. Redox Rep. (2024) 29:2341537. doi: 10.1080/13510002.2024.2341537
36. Afolabi OA, Anyogu DC, Hamed MA, Odetayo AF, Adeyemi DH, Akhigbe RE. Glutamine prevents upregulation of NF-kB signaling and caspase 3 activation in ischaemia/reperfusion-induced testicular damage: an animal model. Biomed Pharmacother. (2022) 150:113056. doi: 10.1016/j.biopha.2022.113056
37. Hamed MA, Akhigbe RE, Aremu AO, Odetayo AF. Zinc normalizes hepatic lipid handling via modulation of ADA/XO/UA pathway and caspase 3 signaling in highly active antiretroviral therapy-treated Wistar rats. Chem Biol Interact. (2022) 368:110233. doi: 10.1016/j.cbi.2022.110233
38. Olayaki LA, Okesina KB, Jesubowale JD, Ajibare AJ, Odetayo AF. Orange peel extract and physical exercise synergistically ameliorate type 2 diabetes mellitus-induced dysmetabolism by upregulating GLUT4 concentration in male wistar rats. J Med Food. (2023) 26:470–9. doi: 10.1089/jmf.2023.0061
39. Desser RK, Himmelhoch SR, Evans WH, Januska M, Mage M, Shelton E. Guinea pig heterophil and eosinophil peroxidase. Arch Biochem Biophys. (1972) 148:452–65. doi: 10.1016/0003-9861(72)90164-6
40. Ridnour LA, Sim JE, Hayward MA, Wink DA, Martin SM, Buettner GR, et al. A spectrophotometric method for the direct detection and quantitation of nitric oxide, nitrite, and nitrate in cell culture media. Anal Biochem. (2000) 281:223–9. doi: 10.1006/abio.2000.4583
41. Zahide ED, Bahad OA. Modified xanthine oxidase activity method based on uric acid absorption. ChemXpress. (2014) 6:09–13.
42. Perandones CE, Illera VA, Peckham D, Stunz LL, Ashman RF. Regulation of apoptosis in vitro in mature murine spleen T cells. J Immunol. (1993) 151:3521–9. doi: 10.4049/jimmunol.151.7.3521
43. Liguori G, Tafuri S, Pelagalli A, Ali S, Russo M, Mirabella N, et al. G protein-coupled estrogen receptor (GPER) and ERs are modulated in the testis-epididymal complex in the normal and cryptorchid dog. Veter Sci. (2024) 11:21. doi: 10.3390/vetsci11010021
44. Pelletier G, El-Alfy M. Immunocytochemical localization of estrogen receptors alpha and beta in the human reproductive organs. J Clin Endocrinol Metab. (2000) 85:4835–40. doi: 10.1210/jcem.85.12.7029
45. Dewaele A, Dujardin E, André M, Albina A, Jammes H, Giton F, et al. Absence of testicular estrogen leads to defects in spermatogenesis and increased semen abnormalities in male rabbits. Genes. (2022) 13:2070. doi: 10.3390/genes13112070
46. Solakidi S, Psarra AG, Nikolaropoulos S, Sekeris CE. Estrogen receptors α and β (ERα and ERβ) and androgen receptor (AR) in human sperm: localization of ERβ and AR in mitochondria of the midpiece. Hum Reprod. (2005) 20:3481–7. doi: 10.1093/humrep/dei267
47. Korach KS. Estrogen receptor knock-out mice: molecular and endocrine phenotypes. J Soc Gynecol. Investig. (2000) 7:S16–S17. doi: 10.1177/1071557600007001S06
48. Cavallini G. Male idiopathic oligoasthenoteratozoospermia. Asian J Androl. (2006) 8:143–57. doi: 10.1111/j.1745-7262.2006.00123.x
49. Vandekerckhove P, Lilford R, Vail A, Hughes E. Clomiphene or tamoxifen for idiopathic oligo/asthenospermia. Cochr Datab System Rev. (2000) 1996:CD000151. doi: 10.1002/14651858.CD000151
50. Rolf C, Behre HM, Nieschlag E. Tamoxifen bei männlicher Infertilität. Analyse einer fragwürdigen Therapie [Tamoxifen in male infertility Analysis of a questionable therapy]. Deutsche medizinische Wochenschrift. (1996) 121:33–9. doi: 10.1055/s-2008-1042969
51. Odetayo AF, Akhigbe RE, Bassey GE, Hamed MA, Olayaki LA. Impact of stress on male fertility: role of gonadotropin inhibitory hormone. Front Endocrinol. (2024) 14:1329564. doi: 10.3389/fendo.2023.1329564
52. Oliveira PF, Sousa M, Silva BM, Monteiro MP, Alves MG. Obesity, energy balance and spermatogenesis. Reproduction. (2017) 153:R173–85. doi: 10.1530/REP-17-0018
53. Allen MO, Salman TM, Alada A, Odetayo AF, Patrick EB, Salami SA. Effect of the beta-adrenergic blockade on intestinal lactate production and glycogen concentration in dogs infused with hexoses. J Complem Integr Med. (2021) 19:287–96. doi: 10.1515/jcim-2021-0062
54. Marek CB, Peralta RM, Itinose AM, Bracht A. Influence of tamoxifen on gluconeogenesis and glycolysis in the perfused rat liver. Chem Biol Interact. (2011) 193:22–33. doi: 10.1016/j.cbi.2011.04.010
55. Hussain T, Kandeel M, Metwally E, Murtaza G, Kalhoro DH, Yin Y, et al. Unraveling the harmful effect of oxidative stress on male fertility: a mechanistic insight. Front Endocrinol. (2023) 14:1070692. doi: 10.3389/fendo.2023.1070692
56. Odetayo AF, Abdulrahim HA, Fabiyi OT, Adewole TA, Ajiboye BE, Omeiza AN, et al. Synergistic effects of vitamin D and exercise on diabetes-induced gonadotoxicity in male wistar rats: role of xanthine oxidase/uric acid and Nrf2/NfkB signaling. Cell Biochem Biophy. (2024) 2024:1–13. doi: 10.1007/s12013-024-01313-w
57. Scarian E, Viola C, Dragoni F, Di Gerlando R, Rizzo B, Diamanti L, et al. New insights into oxidative stress and inflammatory response in neurodegenerative diseases. Int J Mol Sci. (2024) 25:2698. doi: 10.3390/ijms25052698
58. Hussain T, Tan B, Yin Y, Blachier F, Tossou MC, Rahu N. Oxidative stress and inflammation: what polyphenols can do for us? Oxid Med Cell Longev. (2016) 2016:7432797. doi: 10.1155/2016/7432797
59. Chen C, Lü JM, Yao Q. Hyperuricemia-related diseases and xanthine oxidoreductase (XOR) inhibitors: an overview. Med Sci Monitor. (2016) 22:2501–12. doi: 10.12659/MSM.899852
60. Liu N, Xu H, Sun Q, Yu X, Chen W, Wei H, et al. The role of oxidative stress in hyperuricemia and xanthine oxidoreductase (XOR) inhibitors. Oxid Med Cell Longev. (2021) 2021:1470380. doi: 10.1155/2021/1470380
61. Guo Q, Jin Y, Chen X, Ye X, Shen X, Lin M, et al. NF-κB in biology and targeted therapy: new insights and translational implications. Signal Transd Targ Ther. (2024) 9:53. doi: 10.1038/s41392-024-01757-9
62. Ahmed NS, Samec M, Liskova A, Kubatka P, Saso L. Tamoxifen and oxidative stress: an overlooked connection. Disc Oncol. (2021) 12:17. doi: 10.1007/s12672-021-00411-y
63. Schieber M, Chandel NS, ROS. function in redox signaling and oxidative stress. Current Biol. (2014) 24:R453–62. doi: 10.1016/j.cub.2014.03.034
64. Kumar S, Saxena J, Srivastava VK, Kaushik S, Singh H, Abo-El-Sooud K, et al. The interplay of oxidative stress and ROS scavenging: antioxidants as a therapeutic potential in sepsis. Vaccines. (2022) 10:1575. doi: 10.3390/vaccines10101575
65. Jiang X, Wang X. Cytochrome C-mediated apoptosis. Annu Rev Biochem. (2004) 73:87–106. doi: 10.1146/annurev.biochem.73.011303.073706
66. Chen Q, Gong B, Almasan A. Distinct stages of cytochrome c release from mitochondria: evidence for a feedback amplification loop linking caspase activation to mitochondrial dysfunction in genotoxic stress induced apoptosis. Cell Death Differ. (2000) 7:227–33. doi: 10.1038/sj.cdd.4400629
67. Yang J, Liu X, Bhalla K, Kim C, Ibrado A-M, Cai J, et al. Prevention of apoptosis by Bcl-2: release of cytochrome c from mitochondria blocked. Science. (1997) 275:1129–32. doi: 10.1126/science.275.5303.1129
68. Yuan J, Murrell GA, Trickett A, Wang MX. Involvement of cytochrome c release and caspase-3 activation in the oxidative stress-induced apoptosis in human tendon fibroblasts. Biochim Biophys Acta. (2003) 1641:35–41. doi: 10.1016/S0167-4889(03)00047-8
69. Unten Y, Murai M, Koshitaka T, Kitao K, Shirai O, Masuya T, et al. Comprehensive understanding of multiple actions of anticancer drug tamoxifen in isolated mitochondria. Biochim Biophys Acta Bioener. (2022) 1863:148520. doi: 10.1016/j.bbabio.2021.148520
70. Nazarewicz RR, Zenebe WJ, Parihar A, Larson SK, Alidema E, Choi J, et al. Tamoxifen induces oxidative stress and mitochondrial apoptosis via stimulating mitochondrial nitric oxide synthase. Cancer Res. (2007) 67:1282–90. doi: 10.1158/0008-5472.CAN-06-3099
71. Meital LT, Windsor MT, Perissiou M, Schulze K, Magee R, Kuballa A, et al. Omega-3 fatty acids decrease oxidative stress and inflammation in macrophages from patients with small abdominal aortic aneurysm. Sci Rep. (2019) 9:12978. doi: 10.1038/s41598-019-49362-z
Keywords: anticancer drugs, nuclear factor erythroid 2-related factor 2 (Nrf2) and nuclear factor-kappa B (NF-κB) signaling, selective estrogen receptor modulators, testicular function, cytochrome c
Citation: Odetayo AF, Akhigbe RE, Hamed MA, Balogun ME, Oluwole DT and Olayaki LA (2024) Omega-3 fatty acids abrogates oxido-inflammatory and mitochondrial dysfunction-associated apoptotic responses in testis of tamoxifen-treated rats. Front. Nutr. 11:1443895. doi: 10.3389/fnut.2024.1443895
Received: 05 June 2024; Accepted: 19 July 2024;
Published: 01 August 2024.
Edited by:
Julius Liobikas, Lithuanian University of Health Sciences, LithuaniaReviewed by:
Irmak Ferah Okkay, Atatürk University, TürkiyeÇevik Gürel, Harran University, Türkiye
Uche Okuu Arunsi, Georgia Institute of Technology, United States
Copyright © 2024 Odetayo, Akhigbe, Hamed, Balogun, Oluwole and Olayaki. This is an open-access article distributed under the terms of the Creative Commons Attribution License (CC BY). The use, distribution or reproduction in other forums is permitted, provided the original author(s) and the copyright owner(s) are credited and that the original publication in this journal is cited, in accordance with accepted academic practice. No use, distribution or reproduction is permitted which does not comply with these terms.
*Correspondence: Adeyemi Fatai Odetayo, YWRleWVtaW9kZXRheW9AZ21haWwuY29t; YWRleWVtaS5vZGV0YXlvQGZ1aHNpLmVkdS5uZw==