- Department of Urology II, The First Hospital of Jilin University, Changchun, China
Background: The impact of micronutrients, including vitamins and minerals, on different kidney diseases has been reported in some observational studies; however, their causal relationship remains uncertain. We aimed to ascertain the causal genetic relationships between micronutrients and different kidney diseases using the Mendelian randomization (MR) method.
Methods: Instrumental variables (IVs) for genetically predicting calcium (Ca), iron (Ir), Zinc (Zn), selenium (Se), copper (Cu), vitamin D (Vit D), and vitamin C (Vit C) levels in humans were obtained, and a bidirectional two-sample MR was used to examine potential associations between the levels of these seven micronutrients and the risk of seven different kidney diseases including hypertensive renal disease, diabetic nephropathy, IgA nephropathy, membranous nephropathy, cystic nephropathy, chronic kidney disease (CKD), and chronic tubulo-interstitial nephritis. Five different MR analyses were conducted, with the main method being the inverse variance-weighted (IVW) method. Moreover, sensitivity analyses were performed to assess heterogeneity and potential pleiotropy.
Results: The IVW method revealed that Ca levels were associated with a decreased risk of hypertensive renal disease (OR = 0.61, 95% CI: 0.40–0.93, p-value = 0.022), and Se levels were associated with a decreased risk of hypertensive renal disease (OR = 0.72, 95% CI: 0.53–0.99, p-value = 0.040), diabetic nephropathy (OR = 0.83, 95% CI: 0.73–0.93, p-value = 0.002), and CKD (OR = 0.87, 95% CI: 0.77–0.99, p-value = 0.028). Conversely, Vit D levels were associated with an increased risk of polycystic kidney disease (OR = 1.76, 95% CI: 1.15–2.69, p-value = 0.0095). In addition, no potential causal relationship was found between vitamin C levels, iron levels, zinc levels, and copper levels and different kidney diseases. Meanwhile, inverse Mendelian randomization showed no potential causal relationship between different chronic kidney diseases and micronutrients. The Cochrane’s Q test, MR-Egger regression, and MR-PRESSO did not suggest heterogeneity and pleiotropy, providing evidence of the validity of the MR estimates.
Conclusion: Our results indicate a cause-and-effect connection between micronutrients and certain kidney diseases, but additional study is required to provide more conclusive evidence. This research has the potential to assist clinicians in managing the consumption of specific micronutrients among individuals with chronic kidney diseases, as well as in promoting disease prevention among both healthy populations and those who are susceptible to chronic underlying conditions.
Introduction
Kidney diseases are a general term that encompasses a variety of disease types with numerous classification criteria, including primary glomerular diseases (such as membranous nephropathy, IgA nephropathy, glomerulonephritis), acute kidney failure, chronic tubulointerstitial nephritis, diabetic nephropathy, hypertensive renal disease, polycystic kidney disease, and chronic kidney disease (CKD), among others (1). According to the International Society of Nephrology, at least 850 million people worldwide suffer from kidney diseases. In addition, The global prevalence of CKD varies between 8 and 16%, as reported by the International Society of Nephrology. In 2010, CKD ranked 18th among all causes of death worldwide, with an annual mortality rate of 16.3 deaths per 100,000 individuals. This rate is rising, presenting a significant challenge to the global public health system (2, 3). The pathogenic mechanisms of different kidney diseases are complex and not entirely understood due to their numerous origins and consequences. In the absence of appropriate intervention and therapy, the condition has the potential to rapidly advance towards end-stage renal failure.
Micronutrients, which encompass minerals and vitamins, typically comprise non-metallic or metallic components, including calcium (Ca), iron (Ir), Zinc (Zn), selenium (Se), copper (Cu), as well as both fat-soluble and water-soluble vitamins. These nutrients are necessary for normal growth, development, and physiological activities, even if they are required in smaller amounts (4). However, the available data indicates that almost 30% of the global population experiences at least one kind of trace nutrient deficiency, which primarily affects children and pregnant women (5). Furthermore, observational studies have revealed the correlation between micronutrients and different kidney diseases. A prospective study conducted by Chen CY et al. found a positive correlation between serum Se and Zn levels and renal function, as well as improved kidney outcomes. Specifically, higher Zn concentrations were found to independently predict better kidney survival (6). In addition, cohort research conducted over a period of 12 years demonstrated correlations between inadequate consumption of phosphorus, vitamin B2, and folate in one’s diet and elevated consumption of vitamin B6 and vitamin C in one’s diet, with an elevated susceptibility to CKD stage 3B and higher (7). Additionally, vitamin D and its metabolites play an important role in regulating calcium-phosphorus balance as well as bone formation and calcification. They are associated with the risk of various diseases and complications. A meta-analysis of observational studies showed that CKD patients treated with vitamin D had a lower mortality rate compared to those who did not receive treatment (8).
Mendelian randomization (MR) analysis is an effective epidemiological method that uses genetic variation as instrumental variables (IVs) to analyze causal relationships between exposure factors and outcomes (9). Mendel’s law of inheritance posits that genetic differences are predetermined and independent of postnatal conditions, significantly mitigating the impact of common confounding variables and the potential for reverse causation (10, 11). In this study, MR analyses were conducted to investigate the causal relationships between trace nutrients and different kidney diseases.
Materials and methods
Study design
The STROBE-MR (Strengthening Reporting of Observational Studies in Epidemiology Using Mendelian Randomization) statement was followed in the conduct of our study (12). In this study, serum levels of Cu, Ir, Zn, Ca, Se, Vitamin C (Vit C), and Vitamin D (Vit D) were considered as exposures to seven different kidney diseases, including hypertensive renal disease, diabetic kidney disease, IgA nephropathy, membranous nephropathy, polycystic kidney disease, different kidney disease (CKD), and chronic tubulointerstitial nephritis as outcomes. The screening of MR is contingent upon the rigorous adherence to three fundamental assumptions (1): a robust correlation exists between the chosen IVs and the factors of exposure (2); The IVs do not exhibit any associations with confounding factors that could potentially influence the outcome (3); The IVs can solely impact outcomes via the exposure factor route, and there is no direct association between IVs and outcomes (13). The data analysis in this study relied solely on publicly accessible data, obviating the need for ethical approval from an ethics commission (Figure 1).
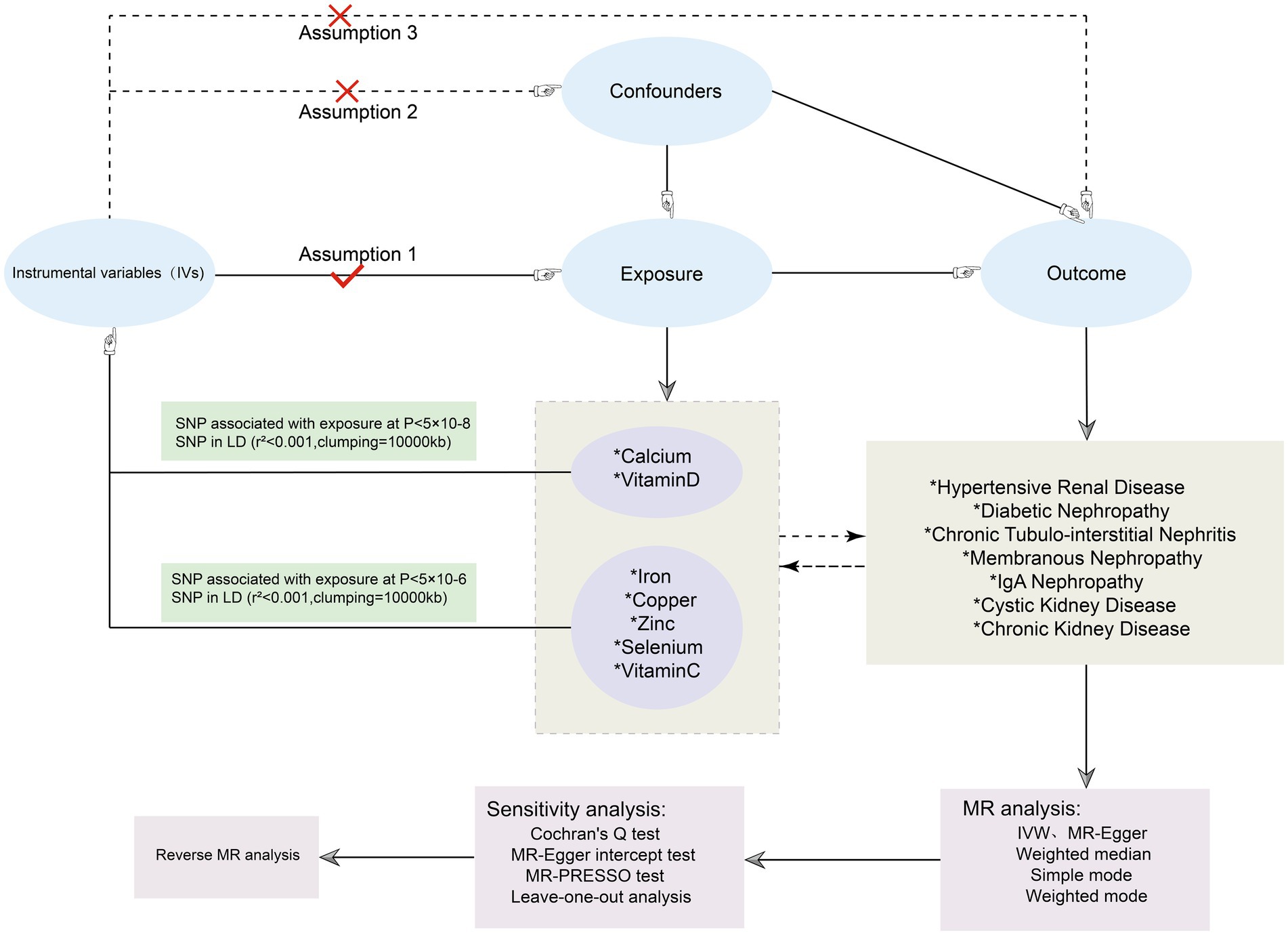
Figure 1. Flowchart of the MR investigating the causal relationship between serum micronutrients and different kidney diseases. SNPs, Single nucleotide polymorphisms; LD, linkage disequilibrium; MR, Mendelian randomization.
GWAS statistics source
The micronutrient data utilized in this work were obtained from the IEU OpenGWAS program and are accessible at the following website: https://gwas.mrcieu.ac.uk/. The FinnGen Consortium (website: https://www.finngen.fi/en) provides Genome-wide association studies (GWAS) for seven different kidney diseases. The study included exclusively European populations as participants, and there was no overlap in the samples between exposures and outcomes. This approach significantly minimized mistakes caused by confounding factors. In cases where multiple independent GWAS were accessible, the study with a greater number of participants was chosen. Pooled GWAS data were chosen for the analysis of five important minerals and two vitamins (14, 15). The detailed information of GWAS on exposures and outcomes used in this MR is shown in Table 1.
Selection of the IVs
Typically, we set the threshold at p < 5 × 10−8 in order to identify single Nucleotide Polymorphisms (SNPs) that are substantially associated with exposures. Nevertheless, certain summarized GWAS datasets exhibited a restricted number of SNPs that achieved genome-wide significance. Consequently, we choose to lower the criterion for certain datasets. The association thresholds for Ca and Vit D were established at p < 5 × 10−8, whereas for Cu, Ir, Zn, Se, and VitC, the association thresholds were set at p < 5 × 10−6. The implementation of various thresholds was employed in earlier MR research with the objective of achieving a higher number of compatible SNPs (16, 17). In order to minimize bias, we then eliminated linkage disequilibrium (LD) and established a threshold of r2 < 0.001, with kb = 10,000. In addition, in order to minimize bias, we eliminated palindromic variants of incompatible alleles and evaluated the potency of the chosen SNPs by computing the F statistic with the following formula:
where N denotes the sample size, and R2 indicates the extent to which IVs explain the exposure. And we would eliminate the SNPs with F < 10 (18, 19). Then, we conducted the Steiger test before each MR analysis to avoid reverse causality and included SNPs with TRUE results. Furthermore, we manually screened these SNPs using PhenoScanner1 and excluded any SNPs associated with confounding factors, such as smoking and alcohol, which could potentially affect the study results. Additionally, this study conducted a reverse Mendelian randomization analysis to reduce the potential impact of chronic kidney diseases on micronutrient levels. Since a threshold of p < 5 × 10−8 did not yield enough SNPs, we also set a threshold of p < 5 × 10−6 for selecting instrumental variables and proceeded with subsequent analyses.
MR analyses and sensitivity analyses
Five different methods of MR, including MR Egger, weighted median, inverse variance weighted (IVW), simple mode, and weighted mode, were utilized to investigate the causal effects between micronutrients and chronic kidney diseases. The basic statistical model employed in this study was IVW estimation, while the remaining four methods were utilized to enhance their robustness. A significance level of less than 0.05 was deemed to be statistically significant. The Cochran’s Q test was employed to evaluate the heterogeneity of results, and a p-value of less than 0.05 was utilized to indicate the presence of heterogeneity (20). The assessment and correction of horizontal pleiotropy were conducted using MR-Egger regression and MR-PRESSO, and p-value of less than 0.05 was obtained, confirming the presence of horizontal pleiotropy (21). Furthermore, a leave-one-out analysis was conducted to evaluate the potential impact of aberrant SNPs on the obtained results. Funnel plots were employed to evaluate potential directional pleiotropy. The statistical analyses were conducted using R software version 4.3.2, specifically utilizing the TwoSampleMR R package (v0.5.7) and MR-PRESSO (v1.0; Figure 2).
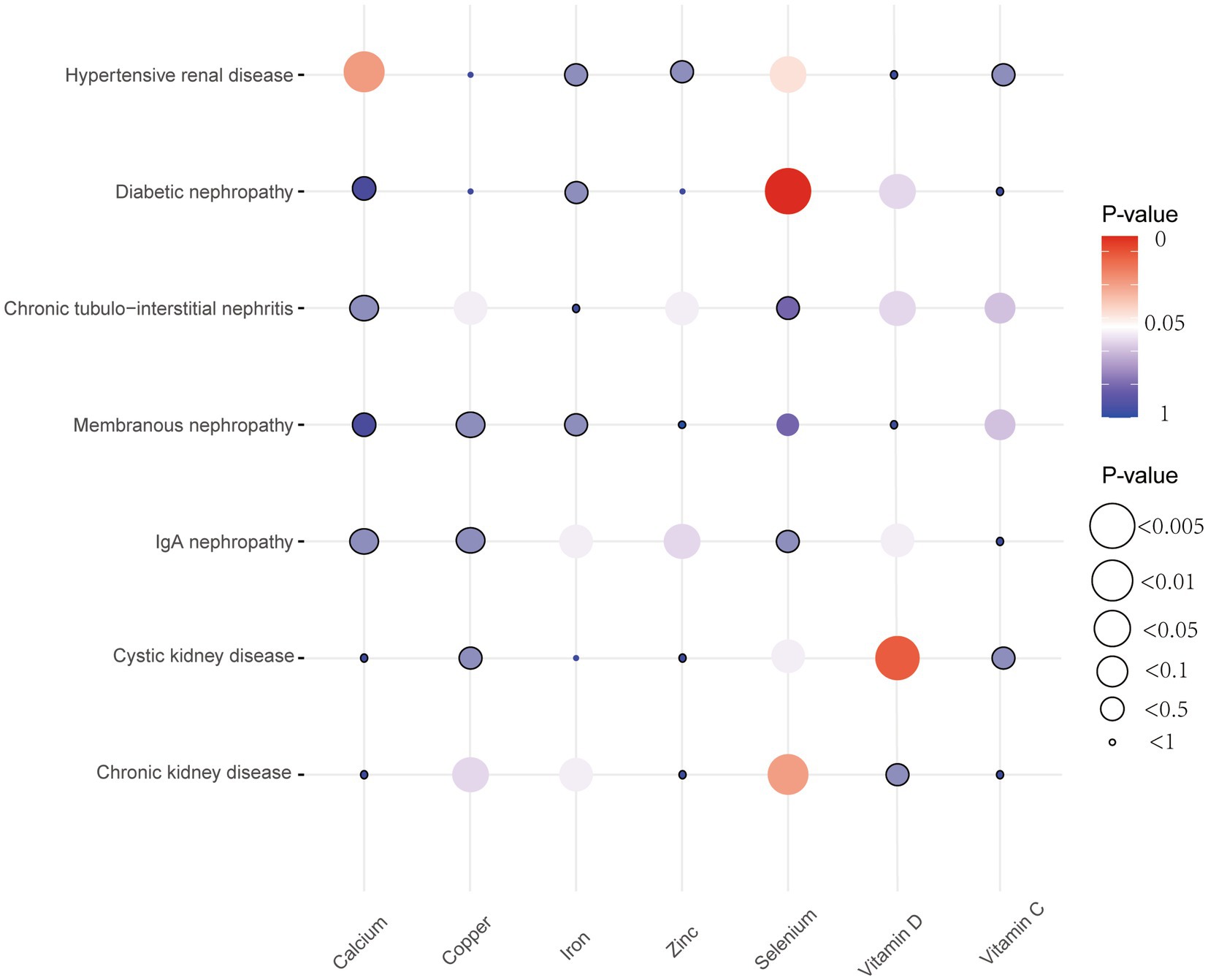
Figure 2. Bubble plots of MR results between micronutrients and seven chronic kidney diseases derived using the IVW method. MR, Mendelian randomization; IVW, inverse variance weighted.
Results
After strictly following the IVs screening process, we finally obtained SNPs for this MR Study (Supplementary Table 1). Figures 3, 4 show the positive results of this two-sample MR analysis of micronutrients and seven chronic kidney diseases using the IVW approach. The IVW analysis revealed that Ca was associated with a decreased risk of hypertensive renal disease (OR = 0.61, 95% CI: 0.40–0.93, p-value = 0.022), and Se was associated with a decreased risk of hypertensive kidney disease (OR = 0.72, 95% CI: 0.53–0.99, p-value = 0.040), diabetic nephropathy (OR = 0.83, 95% CI: 0.73–0.93, p-value = 0.002), and chronic kidney disease (OR = 0.87, 95% CI: 0.77–0.99, p-value = 0.028). Conversely, vitamin D levels were associated with an increased risk of polycystic kidney disease (OR = 1.76, 95% CI: 1.15–2.69, p-value = 0.0095). In addition, no potential causal relationship was found between vitamin C levels, iron levels, zinc levels, and copper levels and different kidney diseases (Supplementary Tables 4–7). Meanwhile, the results of reverse Mendelian randomization showed no potential causal association between different chronic kidney diseases and micronutrients (p > 0.05; Supplementary Table 9). The scatterplot in Figure 4 illustrates the positive outcomes obtained through the IVW method, with a p-value < 0.05. The results of the MR analysis for the other exposures and outcomes are presented in Supplementary Tables 2–8. The Cochran’s Q test, which incorporates the IVW and MR-Egger methods, did not indicate any significant heterogeneity, as shown by a p-value > 0.05. Additionally, the MR-PRESSO and MR-Egger regressions did not reveal any horizontal pleiotropy with p-value > 0.05 (Table 2). Furthermore, the Leave-One-Out analysis yielded no SNPs exhibiting significant bias effects, and the funnel plots demonstrated minimal directional pleiotropy, as depicted in Supplementary Figures 1–5. All these sensitivity analysis results provided evidence of the validity of the MR estimates.
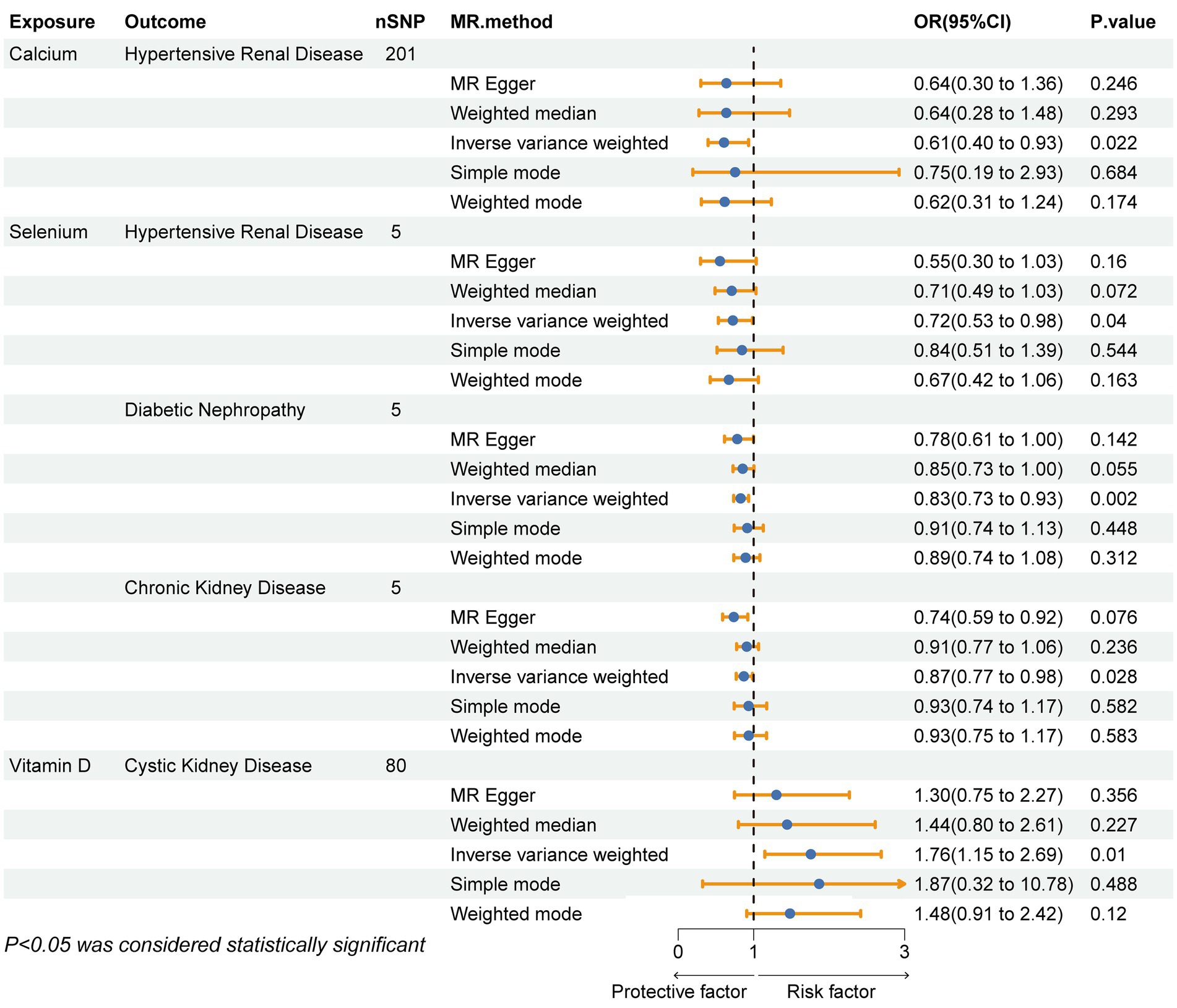
Figure 3. Forest plot showing the causal relationship between micronutrients and chronic kidney disease with a P- value <0.05 calculated using the IVW method. IVW, inverse variance weighting method; OR, odds ratio; CI, confidence interval.
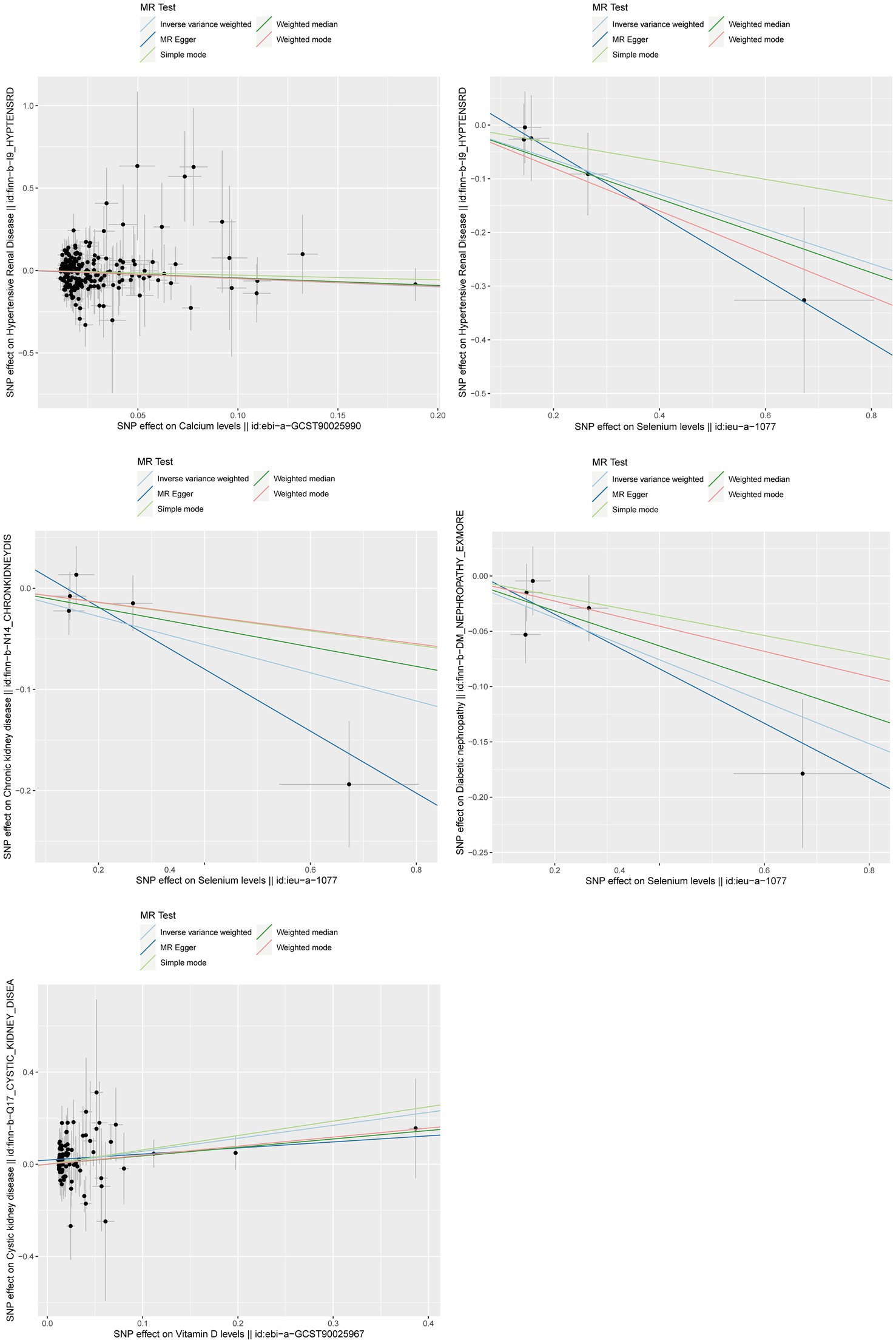
Figure 4. Scatterplot of positive results from MR analysis between exposure and outcome using IVW method. IVW, inverse variance weighted; MR, Mendelian randomization.
Discussion
This study employed MR to examine the potential causal associations between serum levels of micronutrients and the occurrence of different kidney diseases. The study revealed that increased Vit D levels were associated with an elevated risk of cystic nephropathy. Additionally, elevated levels of calcium and selenium were identified as protective factors against Hypertensive renal disease, while selenium levels were found to decrease the risk of diabetic nephropathy and CKD. Nevertheless, our study is subject to several limitations. Firstly, the levels of Ir, Cu, Zn, Se, and Vit C did not produce a sufficient number of single nucleotide polymorphisms (SNPs) for the analysis of MR at a significance level of 5 × 10−8. As a result, we changed the threshold criterion to 5 × 10−6. However, this adjustment would have reduced the overall significance of our study. It is worth noting that this approach has been commonly used in numerous studies and does not inherently impact the outcomes. Secondly, it is important to note that our study was limited to participants of European descent, potentially impacting the applicability of the findings to other populations. Thirdly, the levels of micronutrients may exert varying degrees of influence on different kidney diseases patients of different genders or ages. However, due to the lack of individual-level information in GWAS data, stratified studies cannot be conducted. Finally, in addition to exploring causal relationships, the quantitative relationship between exposure and outcome is also crucial, as it can provide better clinical guidance. Nevertheless, due to the lack of quantitative data on micronutrients and kidney diseases in the GWAS Catalog, this study cannot further investigate the dose–response relationship between exposure and outcome at this time. It is hoped that specific datasets in this area will be available for further study in the future.
Potential causal relationship between Ca and hypertensive renal disease
Hypertensive renal disease (Hypertensive Nephropathy, HTN) is a chronic disease characterized by elevated blood pressure and chronic kidney disease, including the presence of protein in the urine and reduced glomerular filtration rate. HTN is believed to arise from prolonged and unregulated hypertension, and it ranks as the second most prevalent factor contributing to the advancement of end-stage renal disease following diabetic nephropathy (22). The primary pathogenic processes of Hypertensive nephropathy involve changes in renal hemodynamics and remodeling of the renal vasculature. Furthermore, previous studies have demonstrated the significance of podocyte damage, epithelial-mesenchymal transition (EMT), and tubulointerstitial fibrosis as pathogenic mechanisms in HTN (23, 24). The present amount of research on the association between calcium levels and hypertensive nephropathy is currently minimal. A meta-analysis of cohort studies revealed a significant association between calcium consumption and a decreased likelihood of HTN. Specifically, for every incremental increase of 500 mg/day in dietary calcium intake, there was an approximate 7% reduction in the risk of HTN (25). Several studies have indicated that a deficiency in calcium levels can induce the secretion of parathyroid hormone (PTH). The type-1 parathyroid hormone receptor (PTHR1)/ Galpha(s)/3′,5′-cyclic adenosine monophosphate (cAMP)/protein kinase A (PKA) pathway is activated by parathyroid hormone to raise the concentration of calcium in vascular smooth muscle cells. This, in turn, leads to an increase in vascular reactivity and an elevation in blood pressure. Furthermore, parathyroid hormone (PTH) stimulates the production of cyclic adenosine monophosphate (cAMP) and the release of renin by PTHR-1, thereby facilitating the synthesis of angiotensin II (Ang II) and aldosterone (26, 27). Increased synthesis of Ang II and aldosterone affects blood pressure regulation. Prolonged elevation of these hormones leads to changes in renal hemodynamics, causing renal arteriole narrowing, increased vascular resistance, and reduced blood circulation. This, in turn, induces vascular remodeling in the kidneys, manifested as thickening of the renal arteriole intima, reduced local renal blood flow, and compensatory hypertrophy of adjacent renal units. Meanwhile, Ang II causes podocyte damage and, as a potent vasoconstrictor in the RAS, it plays a crucial role by inducing various cytokines involved in inflammation, cell proliferation, and tubulointerstitial fibrosis (23, 28). In summary, the sequence of events described leads to the development of microalbuminuria, a reduction in glomerular filtration rate, and ultimately promotes the development of HTN.
Relationship between Se and hypertensive renal disease and chronic kidney disease
Se is a non-metal element that plays a crucial role in the human body due to its antioxidant properties. It and its amino acid derivatives are involved in various physiological and pathological processes, including oxidative stress and immune-inflammatory responses. The role of selenium in certain kidney diseases has been mentioned in several observational studies (29). A research examining the correlation between selenium consumption and CKD among middle-aged and elderly individuals in China, utilizing data from the Chinese National Health Service (CHNS), indicates that sufficient se intake could potentially exert a beneficial influence on CKD (30). Lifu Lei et al. constructed a rat model with a lack of Se and observed that Se deficiency leads to an increase in the production of hydrogen peroxide by reducing the expression of renal glutathione peroxidase 1. Additionally, it enhances the activity of nuclear factor kappa-B(NF-κB), which in turn increases the expression of renal Angiotensin II type-1 receptor (AT1R), resulting in sodium retention and elevated blood pressure (31). In addition, a study conducted by Liu et al. has revealed that a deficiency in selenium may contribute to the inflammation of renal fibrosis. This deficiency has been observed to facilitate the process of epithelial-mesenchymal transition (EMT) in renal fibrosis, hence promoting the deposition of extracellular matrix (ECM) in this particular condition. The mechanism may involve selenium deficiency activating the PI3K/Akt signaling pathway, leading to the promotion of fibroblast proliferation and collagen synthesis. This activation subsequently regulates the proliferation and survival of epithelial cells, impacts protein synthesis and the cell cycle in renal interstitial cells, and ultimately contributes to the development and advancement of renal fibrosis. In summary, Se plays a crucial role in oxidative stress, EMT, and the renal fibrosis process within the body. It is noteworthy that renal oxidative stress contributes to the development and progression of CKD, and EMT and renal fibrosis are significant pathological processes in the progression of HTN (32, 33). Therefore, consistent with the findings of most observational studies, Se levels are associated with a reduced risk of HTN and CKD.
Effect of Se levels on diabetic nephropathy
Diabetic kidney disease (DKD) is the primary cause of end-stage renal disease (ESRD), with about 40% of diabetic patients having varying degrees of impaired renal function, and DKD is mainly due to microvascular damage caused by prolonged uncontrolled glucose (34). DKD is characterized by several key pathological symptoms, including the thickening of the basement membranes of the glomeruli and tubules, the loss of podocytes, the dilation of the thylakoid membranes, and the localized degeneration of thylakoid cells and thylakoid stroma, among others (35). Furthermore, the pathophysiological processes responsible for the development of DKD predominantly encompass the production of reactive oxygen species (ROS), nutritional detection, recruitment of inflammatory cells, mitochondrial impairment, tubulointerstitial fibrosis, and various other mechanisms (34, 36, 37). Meanwhile, certain metabolic factors (oxidative stress) and hemodynamic factors (activation of the RAS system) may enhance the effects of common pathogenic mechanisms in the context of hyperglycemia, contributing to the development of DKD (38). Studies have demonstrated that selenium can decrease elevated levels of glucose in the blood and enhance the ability of rats to tolerate glucose. An RCT study conducted on humans demonstrated that administering Se supplements for a duration of 12 weeks had a positive impact on serum insulin levels in patients with DKD (39). A study conducted on rats revealed that a lack of selenium caused oxidative stress through the activation of TGF-β1 in both normal and diabetic rats. In both normal and diabetic rats, the presence of selenium deficiency resulted in an increase in albuminuria. Additionally, normal rats exhibited higher plasma glucose levels, whereas diabetic rats experienced worsened plasma glucose levels. These findings indicate that a lack of selenium can cause an increase in the expression of TGF-β1, impact the levels and management of glucose in the blood, enhance the body’s reaction to oxidative stress, and result in the presence of protein in the urine. Ultimately, these factors contribute to the onset and progression of DKD (40). Although the existing body of information on the association between selenium and kidney illness primarily relies on animal models, it offers valuable insights into the underlying mechanisms in humans. However, additional study and exploration are necessary to understand this relationship fully.
Association between vitamin D and cystic kidney disease
Cystic kidney disease is categorized into congenital and acquired kidney diseases, including autosomal dominant polycystic kidney disease, autosomal recessive polycystic kidney disease, Meckel syndrome, and simple renal cysts. The latter is characterized by the presence of renal cysts of varying degrees (41). Currently, there are very few studies on the relationship between vitamin D levels and cystic kidney disease, and the connection between them remains unclear. Some scholars believe that fibroblast growth factor 23 (FGF23) plays a significant role in the development of cystic kidney disease. This hormone, primarily synthesized by osteoblasts and osteocytes in the bone, is crucial for maintaining normal kidney function. Phosphate, PTH, calcitriol, and calcium are the primary regulatory variables that influence its secretion (42). A study revealed that individuals with vitamin D deficiency rickets exhibited reduced serum FGF23 levels, which subsequently rose following vitamin D therapy (43). The comprehensive review and meta-analysis conducted by Charoenngam et al. yielded findings indicating that the administration of vitamin D3 supplementation resulted in a considerable elevation of intact FGF23 levels in individuals diagnosed with vitamin D deficiency (44). The study conducted by Spichtig et al. provided evidence of the production of FGF23 in the kidneys of rodents with polycystic kidneys. This production resulted in an elevation of FGF23 levels, which subsequently led to a deterioration in kidney function (45, 46). So, FGF23 might be a key point in the relationship between vitamin D levels and cystic kidney disease. However, further research is needed to elucidate the mechanisms of vitamin D’s effects on cystic kidney disease.
Conclusion
Our research provides initial findings on the genetic link between micronutrients and specific chronic kidney disorders. The results of this study have the potential to assist clinicians in managing the consumption of specific micronutrients among individuals with chronic kidney diseases, as well as in promoting disease prevention among both healthy populations and those who are susceptible to chronic underlying conditions. Nevertheless, further research is required in order to establish more definitive evidence.
Data availability statement
Publicly available datasets were analyzed in this study. This data can be found at: https://www.finngen.fi/en; https://gwas.mrcieu.ac.uk.
Author contributions
CS: Conceptualization, Methodology, Software, Writing – original draft. HC: Methodology, Software, Writing – original draft. GZ: Conceptualization, Methodology, Writing – review & editing. HW: Methodology, Software, Writing – original draft. YW: Conceptualization, Writing – review & editing.
Funding
The author(s) declare that no financial support was received for the research, authorship, and/or publication of this article.
Conflict of interest
The authors declare that the research was conducted in the absence of any commercial or financial relationships that could be construed as a potential conflict of interest.
Publisher’s note
All claims expressed in this article are solely those of the authors and do not necessarily represent those of their affiliated organizations, or those of the publisher, the editors and the reviewers. Any product that may be evaluated in this article, or claim that may be made by its manufacturer, is not guaranteed or endorsed by the publisher.
Supplementary material
The Supplementary material for this article can be found online at: https://www.frontiersin.org/articles/10.3389/fnut.2024.1440800/full#supplementary-material
Footnotes
References
1. Jha, V, Garcia-Garcia, G, Iseki, K, Li, Z, Naicker, S, Plattner, B, et al. Chronic kidney disease: global dimension and perspectives. Lancet. (2013) 382:260–72. doi: 10.1016/S0140-6736(13)60687-X
2. Dwyer, JP, Parving, HH, Hunsicker, LG, Ravid, M, Remuzzi, G, and Lewis, JB. Renal dysfunction in the presence of Normoalbuminuria in type 2 diabetes: results from the DEMAND study. Cardiorenal Med. (2012) 2:1–10. doi: 10.1159/000333249
3. Cockwell, P, and Fisher, LA. The global burden of chronic kidney disease. Lancet. (2020) 395:662–4. doi: 10.1016/S0140-6736(19)32977-0
4. Bailey, RL, West, KP Jr, and Black, RE. The epidemiology of global micronutrient deficiencies. Ann Nutr Metab. (2015) 66:22–33. doi: 10.1159/000371618
5. Han, X, Ding, S, Lu, J, and Li, Y. Global, regional, and national burdens of common micronutrient deficiencies from 1990 to 2019: a secondary trend analysis based on the global burden of disease 2019 study. EClinicalMedicine. (2022) 44:101299. doi: 10.1016/j.eclinm.2022.101299
6. Chen, CY, Chiu, CH, Wu, IW, Hsu, HJ, Chen, YT, Hsu, CK, et al. Micronutrients and renal outcomes: a prospective cohort study. Nutrients. (2022) 14:3063. doi: 10.3390/nu14153063
7. Lee, J, Oh, KH, and Park, SK. Dietary micronutrients and risk of chronic kidney disease: a cohort study with 12 year follow-up. Nutrients. (2021) 13:1517. doi: 10.3390/nu13051517
8. Zheng, Z, Shi, H, Jia, J, Li, D, and Lin, S. Vitamin D supplementation and mortality risk in chronic kidney disease: a meta-analysis of 20 observational studies. BMC Nephrol. (2013) 14:199. doi: 10.1186/1471-2369-14-199
9. Smith, GD, and Ebrahim, S. 'Mendelian randomization': can genetic epidemiology contribute to understanding environmental determinants of disease? Int J Epidemiol. (2003) 32:1–22. doi: 10.1093/ije/dyg070
10. Palmer, TM, Lawlor, DA, Harbord, RM, Sheehan, NA, Tobias, JH, Timpson, NJ, et al. Using multiple genetic variants as instrumental variables for modifiable risk factors. Stat Methods Med Res. (2012) 21:223–42. doi: 10.1177/0962280210394459
11. Lawlor, DA, Harbord, RM, Sterne, JA, Timpson, N, and Davey, SG. Mendelian randomization: using genes as instruments for making causal inferences in epidemiology. Stat Med. (2008) 27:1133–63. doi: 10.1002/sim.3034
12. Skrivankova, VW, Richmond, RC, Woolf, BAR, Yarmolinsky, J, Davies, NM, Swanson, SA, et al. Strengthening the reporting of observational studies in epidemiology using Mendelian randomization: the STROBE-MR statement. JAMA. (2021) 326:1614–21. doi: 10.1001/jama.2021.18236
13. Emdin, CA, Khera, AV, and Kathiresan, S. Mendelian randomization. JAMA. (2017) 318:1925–6. doi: 10.1001/jama.2017.17219
14. Cornelis, MC, Fornage, M, Foy, M, Xun, P, Gladyshev, VN, Morris, S, et al. Genome-wide association study of selenium concentrations. Hum Mol Genet. (2015) 24:1469–77. doi: 10.1093/hmg/ddu546
15. O'Seaghdha, CM, Wu, H, Yang, Q, Kapur, K, Guessous, I, Zuber, AM, et al. Meta-analysis of genome-wide association studies identifies six new loci for serum calcium concentrations. PLoS Genet. (2013) 9:e1003796. doi: 10.1371/journal.pgen.1003796
16. Choi, KW, Chen, CY, Stein, MB, Klimentidis, YC, Wang, MJ, Koenen, KC, et al. Assessment of bidirectional relationships between physical activity and depression among adults: a 2-sample Mendelian randomization study. JAMA Psychiatry. (2019) 76:399–408. doi: 10.1001/jamapsychiatry.2018.4175
17. Cai, J, Li, X, Wu, S, Tian, Y, Zhang, Y, Wei, Z, et al. Assessing the causal association between human blood metabolites and the risk of epilepsy. J Transl Med. (2022) 20:437. doi: 10.1186/s12967-022-03648-5
18. Burgess, S, and Thompson, SGCollaboration CCG. Avoiding bias from weak instruments in Mendelian randomization studies. Int J Epidemiol. (2011) 40:755–64. doi: 10.1093/ije/dyr036
19. Burgess, S, Small, DS, and Thompson, SG. A review of instrumental variable estimators for Mendelian randomization. Stat Methods Med Res. (2017) 26:2333–55. doi: 10.1177/0962280215597579
20. Burgess, S, Bowden, J, Fall, T, Ingelsson, E, and Thompson, SG. Sensitivity analyses for robust causal inference from Mendelian randomization analyses with multiple genetic variants. Epidemiology. (2017) 28:30–42. doi: 10.1097/EDE.0000000000000559
21. Bowden, J, Davey Smith, G, and Burgess, S. Mendelian randomization with invalid instruments: effect estimation and bias detection through Egger regression. Int J Epidemiol. (2015) 44:512–25. doi: 10.1093/ije/dyv080
22. Udani, S, Lazich, I, and Bakris, GL. Epidemiology of hypertensive kidney disease. Nat Rev Nephrol. (2011) 7:11–21. doi: 10.1038/nrneph.2010.154
23. Wang, L, Wang, J, Zhang, Y, and Zhang, H. Current perspectives and trends of the research on hypertensive nephropathy: a bibliometric analysis from 2000 to 2023. Ren Fail. (2024) 46:2310122. doi: 10.1080/0886022X.2024.2310122
24. Seccia, TM, Caroccia, B, and Calo, LA. Hypertensive nephropathy. Moving from classic to emerging pathogenetic mechanisms. J Hypertens. (2017) 35:205–12. doi: 10.1097/HJH.0000000000001170
25. Mirmiran, P, Bahadoran, Z, and Azizi, F. Dietary oxalate-calcium balance and the incidence of hypertension and chronic kidney disease: a prospective study among an Asian population. Nutr Metab (Lond). (2022) 19:74. doi: 10.1186/s12986-022-00709-w
26. Villa-Etchegoyen, C, Lombarte, M, Matamoros, N, Belizan, JM, and Cormick, G. Mechanisms involved in the relationship between low calcium intake and high blood pressure. Nutrients. (2019) 11:1112. doi: 10.3390/nu11051112
27. Vilardaga, JP, Romero, G, Friedman, PA, and Gardella, TJ. Molecular basis of parathyroid hormone receptor signaling and trafficking: a family B GPCR paradigm. Cell Mol Life Sci. (2011) 68:1–13. doi: 10.1007/s00018-010-0465-9
28. Hill, GS, Heudes, D, and Bariety, J. Morphometric study of arterioles and glomeruli in the aging kidney suggests focal loss of autoregulation. Kidney Int. (2003) 63:1027–36. doi: 10.1046/j.1523-1755.2003.00831.x
29. Weekley, CM, and Harris, HH. Which form is that? The importance of selenium speciation and metabolism in the prevention and treatment of disease. Chem Soc Rev. (2013) 42:8870–94. doi: 10.1039/c3cs60272a
30. Xie, C, Zeng, M, Shi, Z, Li, S, Jiang, K, and Zhao, Y. Association between selenium status and chronic kidney disease in middle-aged and older Chinese based on CHNS data. Nutrients. (2022) 14:2695. doi: 10.3390/nu14132695
31. Lei, L, Zhang, F, Huang, J, Yang, X, Zhou, X, Yan, H, et al. Selenium deficiency causes hypertension by increasing renal AT(1) receptor expression via GPx1/H(2)O(2)/NF-kappaB pathway. Free Radic Biol Med. (2023) 200:59–72. doi: 10.1016/j.freeradbiomed.2023.02.021
32. Liu, H, Lin, X, Chilufya, MM, Qiao, L, Bao, M, Wen, X, et al. Synergistic effects of T-2 toxin and selenium deficiency exacerbate renal fibrosis through modulation of the ERalpha/PI3K/Akt signaling pathway. Ecotoxicol Environ Saf. (2024) 269:115748. doi: 10.1016/j.ecoenv.2023.115748
33. Hu, H, Hu, S, Xu, S, Gao, Y, Zeng, F, and Shui, H. miR-29b regulates Ang II-induced EMT of rat renal tubular epithelial cells via targeting PI3K/AKT signaling pathway. Int J Mol Med. (2018) 42:453–60. doi: 10.3892/ijmm.2018.3579
34. Thomas, MC, Brownlee, M, Susztak, K, Sharma, K, Jandeleit-Dahm, KA, Zoungas, S, et al. Diabetic kidney disease. Nat Rev Dis Primers. (2015) 1:15018. doi: 10.1038/nrdp.2015.18
35. Lane, PH, Steffes, MW, and Mauer, SM. Renal histologic changes in diabetes mellitus. Semin Nephrol. (1990) 10:254–9.
36. Mao, J, Li, C, Wu, F, Wang, Y, Zhu, J, and Wen, C. The relationship between kidney disease and mitochondria: a bibliometric study. Ren Fail. (2024) 46:2302963. doi: 10.1080/0886022X.2024.2302963
37. Qian, Y, Feldman, E, Pennathur, S, Kretzler, M, and Brosius, FC 3rd. From fibrosis to sclerosis: mechanisms of glomerulosclerosis in diabetic nephropathy. Diabetes. (2008) 57:1439–45. doi: 10.2337/db08-0061
38. Cooper, ME . Pathogenesis, prevention, and treatment of diabetic nephropathy. Lancet. (1998) 352:213–9. doi: 10.1016/S0140-6736(98)01346-4
39. Bahmani, F, Kia, M, Soleimani, A, Asemi, Z, and Esmaillzadeh, A. Effect of selenium supplementation on glycemic control and lipid profiles in patients with diabetic nephropathy. Biol Trace Elem Res. (2016) 172:282–9. doi: 10.1007/s12011-015-0600-4
40. Reddi, AS, and Bollineni, JS. Selenium-deficient diet induces renal oxidative stress and injury via TGF-beta1 in normal and diabetic rats. Kidney Int. (2001) 59:1342–53. doi: 10.1046/j.1523-1755.2001.0590041342.x
41. Hanna, C, Iliuta, I-A, Besse, W, Mekahli, D, and Chebib, FT. Cystic kidney diseases in children and adults: differences and gaps in clinical management. Semin Nephrol. (2023) 43:151434. doi: 10.1016/j.semnephrol.2023.151434
42. Pons-Belda, OD, Alonso-Álvarez, MA, González-Rodríguez, JD, Mantecón-Fernández, L, and Santos-Rodríguez, F. Mineral metabolism in children: interrelation between vitamin D and FGF23. Int J Mol Sci. (2023) 24:6661. doi: 10.3390/ijms24076661
43. Kubota, T, Kitaoka, T, Miura, K, Fujiwara, M, Ohata, Y, Miyoshi, Y, et al. Serum fibroblast growth factor 23 is a useful marker to distinguish vitamin D-deficient rickets from hypophosphatemic rickets. Horm Res Paediatr. (2014) 81:251–7. doi: 10.1159/000357142
44. Charoenngam, N, Rujirachun, P, Holick, MF, and Ungprasert, P. Oral vitamin D3 supplementation increases serum fibroblast growth factor 23 concentration in vitamin D-deficient patients: a systematic review and meta-analysis. Osteop Int. (2019) 30:2183–93. doi: 10.1007/s00198-019-05102-7
45. Lang, F, and Föller, M. Enigmatic Cassandra: renal FGF23 formation in polycystic kidney disease. Kidney Int. (2014) 85:1260–2. doi: 10.1038/ki.2013.534
Keywords: kidney diseases, micronutrients, Mendelian randomization, causal effect, genome-wide association studies
Citation: Shi C, Cao H, Zeng G, Wu H and Wang Y (2024) Mendelian randomization analyses explore the effects of micronutrients on different kidney diseases. Front. Nutr. 11:1440800. doi: 10.3389/fnut.2024.1440800
Edited by:
Sladjana Sobajic, University of Belgrade, SerbiaReviewed by:
Ken-Ichi Kobayashi, Notre Dame Seishin University, JapanRicardo Adrian Nugraha, Airlangga University, Indonesia
Guangyuan Zhang, Southeast University, China
Copyright © 2024 Shi, Cao, Zeng, Wu and Wang. This is an open-access article distributed under the terms of the Creative Commons Attribution License (CC BY). The use, distribution or reproduction in other forums is permitted, provided the original author(s) and the copyright owner(s) are credited and that the original publication in this journal is cited, in accordance with accepted academic practice. No use, distribution or reproduction is permitted which does not comply with these terms.
*Correspondence: Yuantao Wang, wangyuantaobs@jlu.edu.cn
†These authors have contributed equally to this work