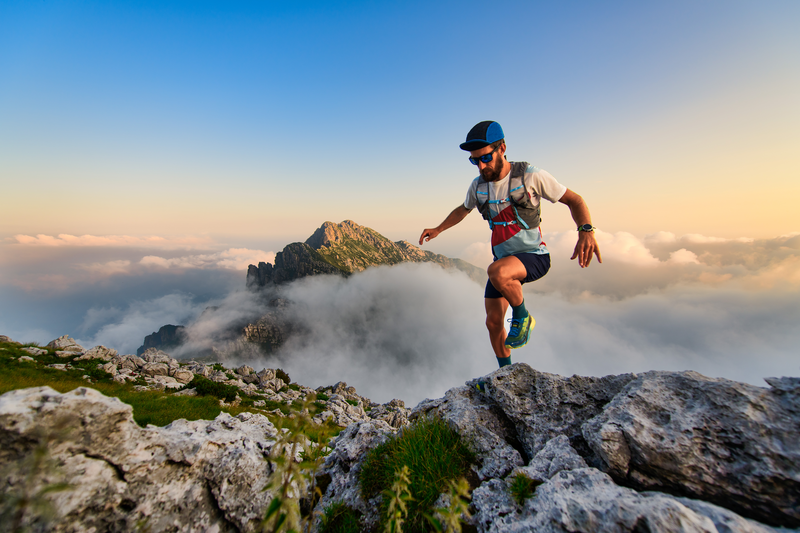
94% of researchers rate our articles as excellent or good
Learn more about the work of our research integrity team to safeguard the quality of each article we publish.
Find out more
ORIGINAL RESEARCH article
Front. Nutr. , 01 August 2024
Sec. Nutrition and Metabolism
Volume 11 - 2024 | https://doi.org/10.3389/fnut.2024.1439217
This article is part of the Research Topic The Intersection of Genes, Nutrition and Disease: A Nutritional Perspective from Mendelian Randomization to Disease Pathogenic Mechanisms View all 5 articles
Background: Multiple clinical studies have observed a close relationship between serum trace elements and nutrients and diabetes and its complications, but it remains unclear whether there is a genetic causal effect between serum trace elements and nutrients and diabetes and its complications.
Objective: This study aims to investigate the causal effects of serum trace elements and nutrients on diabetes and its complications using Mendelian randomization methods.
Methods: The single nucleotide polymorphisms of serum trace elements and vitamins, as exposure factors, were sourced from the published UK Biobank database and public databases of genome-wide association studies. The genome-wide association study data of diabetes and its complications, as outcome events, were sourced from the FinnGen Biobank database. Mendelian randomization methods were employed to explore the causal relationships between 9 trace elements and 6 nutrients and diabetes and its complications. The causal relationships were inferred using inverse variance weighting, MR Egger, weighted median, simple model, and weighted model methods. Sensitivity analyses, including heterogeneity tests, horizontal pleiotropy tests, MR-PRESSO tests, and leave-one-out analysis, were conducted to evaluate the robustness of the study results. Finally, trace elements and nutrients with statistical significance in the IVW method and consistent Beta and OR directions in the five methods were selected as exposure factors with causal relationships with diabetes and its complications. This study also used multivariable Mendelian randomization methods to assess the combined effects of multiple exposure factors on the risk of diabetes and its complications.
Results: Mendelian randomization analysis revealed that selenium was linked to an elevated risk of T2D.Vitamin B6 was correlated with an increased risk of neurological complications in type 2 diabetes. Magnesium exhibited a negative causal relationship with the risk of T1D.Carotene was linked to a higher risk of renal complications in T1D.Vitamin B12 showed a negative causal relationship with renal complications in T1D.Carotene was connected to a higher risk of neurological complications in T1D.Potassium and vitamin B6 exhibited negative causal relationships with neurological complications in T1D.Vitamin E showed a negative causal relationship with peripheral circulation complications in T2D.Multivariable Mendelian randomization analysis suggested that vitamin B6 could independently influence neurological complications in both T1D and T2D, apart from other exposure factors. Vitamin B6 could also independently influence renal complications in T1D.Vitamin E could independently influence peripheral circulation complications in T1D, apart from other exposure factors.
Conclusion: The findings from univariable and multivariable Mendelian randomization studies substantiate the causal relationships between trace elements and nutrients and different subtypes of diabetes and their complications. These findings hold significant clinical implications for developing targeted prevention and treatment strategies for diabetes and its complications.
Diabetes mellitus is a severe metabolic disease with a continually rising incidence rate. It is projected that by 2030, more than 578 million people globally will be affected (1). As the disease progresses, patients may develop various vascular complications, including large vessel diseases (such as cerebrovascular disease, cardiovascular disease, and peripheral vascular disease) and microvascular diseases (such as diabetic nephropathy, retinopathy, and neuropathy) (2). Vascular complications are the leading cause of death among adults aged 20 to 79 with diabetes (2). Diabetic neuropathy (DN) is the most common complication, encompassing distal symmetric polyneuropathy, small-fiber predominant neuropathy, radiculoplexopathy, and mononeuropathy (3). According to the International Diabetes Federation, by 2050, if not effectively managed, half of type 2 diabetes patients will develop some form of neuropathy (4, 5). Research indicates a close association between peripheral arterial disease (PAD) and diabetes, with approximately 20 to 30% of PAD patients having diabetes (6). Diabetic patients are 2 to 4 times more likely to develop PAD than non-diabetic individuals, and the longer the duration of diabetes, the higher the prevalence (7, 8). Moreover, diabetes worsens the severity of PAD, increasing the risk of amputation (9). Globally, 22.27% of diabetes patients have diabetic retinopathy (DR). In 2020, approximately 103 million people had DR, and this number is projected to rise to 165 million by 2045 (10). Almost all type 1 diabetes patients and 60% of type 2 diabetes patients will develop DR within 20 years, resulting in vision impairment and an increased risk of stroke and coronary heart disease (11, 12). Diabetic nephropathy (DN) often leads to end-stage renal disease (ESRD), with approximately 30% of type 1 diabetes patients and 40% of type 2 diabetes patients developing DN (13, 14). DN is a leading cause of ESRD globally and a risk factor for cardiovascular disease, markedly increasing the risk of adverse cardiovascular events, infections, and mortality (15–17). Diabetes and its complications have a profound economic impact on individuals, families, and society, making the exploration of early prevention and intervention methods essential.
The impact of trace elements and vitamins on diabetes and its complications has garnered widespread attention. Studies have shown that the deficiency or excessive intake of certain trace elements is closely related to the occurrence and development of diabetes and its complications. Early studies indicate that the concentrations of iron, chromium, and copper in the adipose tissue of type 2 diabetes mellitus (T2DM) patients are associated with the risk of developing T2DM, while vanadium and zinc may have protective effects (18). Oost et al. (19) found that magnesium deficiency is associated with increased insulin resistance and cardiovascular disease risk in T2DM patients. Cai et al. (20) suggest that low-dose selenium supplementation can regulate hepatic glycolysis and gluconeogenesis, as well as inhibit oxidative stress, thereby beneficially affecting fasting blood glucose (FBG) levels and glucose tolerance, and reducing the risk of diabetes and its complications. Castillo-Otí et al. (21) found that vitamin D deficiency is associated with an increased incidence of diabetic retinopathy and that vitamin D supplementation can improve retinal health in diabetes patients. Xiao et al. (22) found that vitamin D deficiency is significantly associated with a high prevalence of diabetic foot ulcers in T2DM patients, and that vitamin D screening or supplementation may be beneficial for preventing diabetic foot ulcers and improving outcomes in T2DM patients. Nevertheless, the specific causal relationships between trace elements and nutrients and diabetes and its complications still require further research to be confirmed.
Rigorously designed randomized controlled trials are deemed the gold standard for determining causality and effectively managing potential confounding factors. However, due to ethical constraints, limitations in external validity, challenges in double-blind design, interference from internal and external factors (such as personal behavior, lifestyle, environmental factors), insufficient statistical power, and the need for substantial cost and time, the implementation of randomized controlled trials is often restricted. With the increasing number of large-scale genome-wide association studies (GWAS), Mendelian randomization (MR) has emerged as an effective tool for causal inference between different phenotypes (23). MR is a method that integrates epidemiology and genetics, utilizing naturally occurring genetic variations to simulate randomized experiments for causal inference, thereby more accurately estimating the influence of specific factors on outcomes (24, 25). In MR, single nucleotide polymorphisms (SNPs) related to exposure events are used as instrumental variables. Since instrumental variables are independent of other confounding factors, MR can evaluate the causal relationship between previously observed exposures and outcomes, effectively avoiding confounding bias in traditional epidemiological studies (26). Multivariate Mendelian randomization (MVMR) expands upon MR by considering the causal relationships between multiple exposure factors and diseases. This approach allows MVMR studies to evaluate the joint impact of multiple exposure factors on disease risk, as well as the interactions among these exposure factors (27, 28). Against this backdrop, this study utilizes both two-sample MR and MVMR methods to evaluate the influence of nine trace elements and six nutrients on diabetes and its complications. The goal is to gain deeper insights into the effects of dietary nutrients on diabetes and to offer new perspectives for the future application of micronutrients in the prevention and treatment of diabetes and its complications.
This study utilizes a two-sample MR study for causal inference, with trace elements (copper, calcium, carotene, folate, iron, magnesium, zinc, potassium, selenium) and nutrients (vitamins A, B12, B6, C, D, and E) as exposure factors, and diabetes and its complications as outcome factors. The three main MR assumptions are illustrated in Figure 1. Assumption 1: The selected SNPs are significantly associated with the exposure factors (trace elements and nutrients). Assumption 2: The SNPs must be independent of potential confounders between the exposure and the outcome. Assumption 3: The SNPs are not directly related to diabetes and its complications, but can only be causally linked through trace elements and nutrients.
Figure 1. Flow chart of the Mendelian randomization study of trace elements and nutrients on diabetes and its complications.
The Genome-Wide Association Studies (GWAS) data for trace elements and nutrients in this study were obtained from IEU OpenGWAS,1 and the corresponding GWAS information for trace elements and nutrients are detailed in Table 1. GWAS information for diabetes and its complications was obtained from the Finnish database.2 The FinnGen R9 database includes publicly available GWAS data on various diabetes complications. Our study conducted different subtype analyses for type 1 and type 2 diabetes mellitus, covering a range of diabetes complications, including diabetes with renal complications, diabetes with ophthalmic complications, diabetes with neurological complications, and diabetes with peripheral circulatory complications. Diabetes endpoints were defined according to the recommendations of the World Health Organization, and the inclusion and exclusion criteria were based on the International Classification of Diseases,3 particularly the 9th or 10th edition (29). Detailed information on the disease data is provided in Table 2.
According to the STROBE-MR research guidelines (26), each SNP of trace elements and nutrients undergoes the following screening steps: (i) A genome-wide significance threshold of p < 5 × 10^-8 is used, and if the number of significant SNPs under this criterion is small, a threshold of p < 5 × 10^-6 is adopted. (ii) Linkage disequilibrium (LD) testing is performed using the Clump function with criteria set to r^2 < 0.001 and kb = 10,000. (iii) The PhenoScanner database4 is used to exclude SNPs associated with outcomes, eliminating confounders. (iv) The F-statistic for each SNP is calculated, and SNPs with F < 10 are excluded to avoid bias from weak instrumental variables. Meanwhile, the proportion of exposure explained by the instrumental variable (R^2) is calculated to quantify the strength of the genetic instrument, using the following formula: R^2 = [2 × Beta^2 × (1-EAF) × EAF] / [2 × Beta^2 × (1-EAF) × EAF + 2 × SE^2 × N×(1-EAF) × EAF], where Beta represents the genetic effect of each SNP, EAF is the effect allele frequency, SE is the standard error, and N is the sample size. To evaluate the strength of the selected SNPs, the F-statistic for each SNP is calculated using the following formula: F = R^2(N-k-1) / k(1-R^2), where R^2 represents the proportion of exposure explained by the selected SNPs, N is the sample size, and k is the number of included instrumental variables. SNPs with F < 10 are excluded as weak instrumental variables. The remaining independent instrumental variables are used for the subsequent MR analysis (5). MR-PRESSO is used to detect outliers and adjust for horizontal pleiotropy. If horizontal pleiotropy is detected among the instrumental variables, the outliers are removed.
Each trace element and nutrient was assessed for its causal relationship with diabetes and its complications. The potential causal effects were evaluated using the inverse variance-weighted method, weighted median method, weighted mode method, simple mode method, and MR-Egger method. The causal effect reflects the impact of a one standard deviation (SD) increase in each input trace element and nutrient on the risk of outcome characteristics, presented as odds ratio (OR) values and their 95% confidence intervals (CI). Sensitivity analyses included heterogeneity tests, gene pleiotropy tests, and the leave-one-out method. Heterogeneity was tested using Cochran’s Q, where p > 0.05 indicates no heterogeneity and p < 0.05 suggests possible inter-gene heterogeneity. The ideal outcome of the leave-one-out method is that the results does not change significantly after removing each SNP one by one. Horizontal pleiotropy was detected using the MR-Egger method (commonly expressed by the intercept of MR-Egger) and the MR-PRESSO global test. The above statistical analyses were mainly performed using the TwoSampleMR package (version 0.5.5) of R software (version 4.0.2). All analyses were conducted using the R packages ‘TwoSampleMR (version 0.5.6)’ and ‘MR-PRESSO (version 1.0)’ in R software (version 4.3.1). If the results show no pleiotropy and heterogeneity, IVW is significant, other methods are significant, and the results are stable.
Finally, this study utilized multivariate Mendelian randomization research to analyze the causal relationships between multiple exposure factors and disease, evaluating the joint impact of multiple exposure factors on disease risk and their interactions. Through these analyses, this study aims to deepen the understanding of the causal relationships between trace elements and vitamins and diabetes and its complications, and to provide scientific evidence for the prevention and treatment of the disease.
SNPs meeting the three main assumptions were selected based on the set criteria, and variables that might affect the outcomes were excluded using the PhenoScanner database. The F-values of the remaining instrumental variables were all greater than 10. When using the genome-wide significance threshold of p < 5 × 10^-8, the number of available SNPs was too small to analyze. Therefore, according to the STROBE-MR guidelines and literature review, the p-value was set to p < 5 × 10^-6. Finally, 188 SNPs significantly associated with all trace elements were identified as instrumental variables. Detailed information on the instrumental variables can be found in the Supplementary material.
Using the IVW method as the primary result, we found that the selenium is associated with an increased risk of type 2 diabetes (OR = 1.040, 95% CI = 1.009–1.072, p = 0.010) (Figure 2). Vitamin B6 is associated with an increased risk of type 2 diabetes with neurological complications (OR = 2.029, 95% CI = 1.101–3.741, p = 0.023). The study found no effect of trace elements and nutrients on type 2 diabetes with ophthalmic complications, peripheral circulatory complications, and renal complications. Magnesium is negatively causally related to the risk of type 1 diabetes (OR = 0.529, 95% CI = 0.344–0.814, p = 0.004), suggesting that magnesium may be a protective factor against type 1 diabetes. Carotene is associated with an increased risk of type 1 diabetes with renal complications (OR = 2.049, 95% CI = 1.049–4.005, p = 0.036). Vitamin B12 is negatively causally related to type 1 diabetes with renal complications (OR = 0.307, 95% CI = 0.133–0.707, p = 0.006). Carotene is associated with an increased risk of type 1 diabetes with neurological complications (OR = 2.309, 95% CI = 1.028–5.187, p = 0.043). Potassium (OR = 0.358, 95% CI = 0.136–0.945, p = 0.038) and vitamin B6 (OR = 0.291, 95% CI = 0.130–0.651, p = 0.291) are negatively causally related to type 1 diabetes with neurological complications. Vitamin E is negatively causally related to type 2 diabetes with peripheral circulatory complications (OR = 0.284, 95% CI = 0.102–0.790, p = 0.016). No effect of trace elements and vitamins on type 1 diabetes with ophthalmic complications was found. To avoid excessive bias, we conducted a series of sensitivity analyses to test the reliability of MR analyses and to detect potential horizontal pleiotropy. The intercept of the MR-Egger showed no horizontal pleiotropy for all causal effects (p > 0.05). Cochran’s Q and leave-one-out tests indicated no significant heterogeneity. This suggests that the MR analysis results are robust.
Through multivariable Mendelian randomization studies, we analyzed the causal relationships between multiple exposures and diseases, assessed the combined impact of multiple exposures on disease risk, and evaluated the interactions among these exposures.
In the study of type 2 diabetes with neurological complications, we used vitamin B6, vitamin C, vitamin E, and vitamin A as the exposure factors to assess the combined impact of these exposure factors on disease risk and their interactions. The results showed that under the combined effect of multiple vitamins, vitamin B6 still had a direct impact on type 2 diabetes with neurological complications (OR = 4.230; 95% CI =1.200–14.905; p = 0.025) (Figure 3). Sensitivity analysis showed no horizontal pleiotropy or heterogeneity for all causal effects, indicating robust MR analysis results. This suggests that vitamin B6 can independently affect type 2 diabetes with neurological complications, separate from vitamins C, E, and A.
Figure 3. Results of a multivariate Mendelian study of type 2 diabetes with neurological complications.
In the study of type 1 diabetes with neurological complications, we used carotene, potassium, and vitamin B6 as exposure factors to assess the combined impact of these exposure factors on disease risk and their interactions. The results showed that under the combined effect of multiple exposure factors, carotene still had a direct impact on type 1 diabetes with neurological complications (OR = 9.131; 95% CI =2.900–28.749; p < 0.001) (Figure 4). Sensitivity analysis showed no horizontal pleiotropy or heterogeneity for all causal effects, indicating robust MR analysis results. This suggests that carotene can independently affect type 1 diabetes with neurological complications, separate from potassium and carotene.
Figure 4. Results of a multivariate Mendelian study of type 1 diabetes with neurological complications.
In the study of type 1 diabetes with renal complications, we used carotene and vitamin B12 as exposure factors to assess the combined impact of these exposure factors on disease risk and their interactions. The results showed that under the combined effect of multiple exposure factors, vitamin B12 still had a direct impact on type 1 diabetes with renal complications (OR = 0.339; 95% CI =0.195–0.591; p < 0.001) (Figure 5). Sensitivity analysis showed no horizontal pleiotropy or heterogeneity for all causal effects, indicating robust MR analysis results. This suggests that vitamin B12 can independently affect type 1 diabetes with renal complications, separate from carotene.
Figure 5. Results of a multivariate Mendelian study of type 1 diabetes with peripheral circulatory complications.
In the study of type 1 diabetes with peripheral circulatory complications, we used vitamin D, vitamin C, vitamin B12, vitamin E, and vitamin B6 as exposure factors to assess the combined impact of these exposure factors on disease risk and their interactions. The results showed that under the combined effect of multiple vitamins, vitamin E still had a direct impact on type 1 diabetes with peripheral circulatory complications (OR = 0.126; 95% CI =0.023–0.703; p = 0.018) (Figure 6). Sensitivity analysis showed no horizontal pleiotropy or heterogeneity for all causal effects, indicating robust MR analysis results. This suggests that vitamin E can independently affect type 1 diabetes with peripheral circulatory complications, separate from carotene.
Figure 6. Results of a multivariate Mendelian study of type 1 diabetes with peripheral circulatory complications.
To our knowledge, this study is the first to evaluate the effects of trace elements and vitamins on different subtypes of diabetes and its complications using Mendelian randomization (MR) methods. The study used published GWAS data to infer the causal relationship between serum trace elements and vitamins and different subtypes of diabetes and its complications. The analysis results revealed that selenium is associated with an increased risk of developing type 2 diabetes. Vitamin B6 is associated with an increased risk of neurological complications in type 2 diabetes. Magnesium is negatively related to the risk of developing type 1 diabetes. Carotene is associated with an increased risk of kidney complications in type 1 diabetes. Vitamin B12 is negatively related to the risk of kidney complications in type 1 diabetes. Carotene is associated with an increased risk of neurological complications in type 1 diabetes. Potassium and vitamin B6 are negatively related to the risk of neurological complications in type 1 diabetes. Vitamin E is negatively associated with the risk of peripheral circulatory complications in type 2 diabetes. The multivariable Mendelian randomization analysis results showed that Vitamin B6 can affect type 1 diabetes with neurological complications and type 2 diabetes with neurological complications independently of other exposure factors. Vitamin B6 may affect type 1 diabetes with renal complications independently of other exposure factors. Vitamin E may affect type 1 diabetes with peripheral circulatory complications independently of other exposure factors.
Selenium is an essential trace element in the human body, garnering significant attention due to its dual role in health and disease. However, the relationship between selenium and diabetes is complex and appears to be dose-dependent. Research has shown that elevated serum selenium levels are related to an increased risk of type 2 diabetes (T2D), aligning with our results. A randomized controlled trial discovered that supplementing 200 μg of selenium daily might increase the risk of type 2 diabetes (30). Another secondary analysis of a nasopharyngeal cancer trial suggested that during 7.7 years of follow-up, individuals (mostly men) who achieved relatively high serum selenium levels (around 190 μg/L) by daily supplementation with 200 μg of selenium yeast showed an increased incidence of type 2 diabetes (31). Multiple cross-sectional and cohort studies jointly found that serum selenium levels between 124 and 147 μg/L are positively associated with hyperglycemia and self-reported prevalence of T2D (32–35). Research suggests that selenoprotein P (SELENOP) produced by the liver can transport selenium to peripheral tissues, and that elevated serum SELENOP levels may result in decreased insulin sensitivity and impaired glucose tolerance (36, 37). Excessive selenium may lead to oxidative stress, harming pancreatic β-cells and insulin-sensitive tissues, eventually increasing the risk of type 2 diabetes (38, 39). Furthermore, glutathione peroxidase 1 (GPX1), a selenium-containing antioxidant enzyme, has been discovered to inhibit insulin signaling by lowering reactive oxygen species levels, resulting in insulin resistance and possibly affecting pancreatic β-cell function (38, 39). Huang et al. (40) noted that an increase in selenium primarily affects oxidative stress, insulin signalling, selenium metabolism, and the expression of specific selenoproteins, thereby increasing the risk of type 2 diabetes. Selenoprotein P (SELENOP) is a selenium-containing protein that has been found to impact insulin signalling and glucose metabolism negatively. Elevated levels of SELENOP are associated with decreased insulin sensitivity and an increased risk of type 2 diabetes. Experimental studies have demonstrated that SELENOP affects the phosphorylation of key molecules in the insulin signaling pathway, thereby impairing insulin signaling and leading to insulin resistance in hepatocytes and myocytes (41). Over time, this disruption can result in reduced insulin sensitivity and elevated blood glucose levels, heightening the risk of developing diabetes. Furthermore, meta-analyses have confirmed the association between high circulating levels of SELENOP and various glucose and lipid metabolism disorders. These conditions include gestational diabetes, metabolic syndrome, non-alcoholic fatty liver disease, obesity, and type 2 diabetes (42). Given its close relationship with markers of metabolic dysfunction, SELENOP can serve as a biomarker for these diseases. Nevertheless, these mechanisms need further investigation to be fully elucidated.
Research indicates that elevated levels of vitamin B6 are linked to an increased risk of neurological complications in type 2 diabetes, consistent with our findings (43). Although vitamin B6 is beneficial at regular doses, high-dose supplementation may induce neurotoxicity, resulting in sensory axonal neuropathy. This neuropathy usually alleviates after stopping high-dose vitamin B6 intake (44). Vitamin B6 is inversely correlated with homocysteine levels, and diabetic patients with peripheral neuropathy often exhibit reduced vitamin B6 levels. Vitamin B6 is a crucial coenzyme in the metabolism of homocysteine, participating in the transsulfuration pathway. In type 1 diabetes patients, due to insufficient or absent insulin secretion, blood glucose levels are chronically elevated. This chronic hyperglycemic condition may influence the metabolism of homocysteine. If vitamin B6 levels are inadequate, it may result in homocysteine metabolism disorders, thereby impacting nervous system health. Additionally, vitamin B6 deficiency may affect the nitric oxide neurotransmitter system, potentially reducing NMDA receptor function, thereby impacting nitric oxide synthase activity, leading to decreased nitric oxide synthesis and further promoting the development of diabetic peripheral neuropathy (45–47). Research indicates that both vitamin B6 deficiency and elevated levels may be related to peripheral neuropathy, with high levels potentially worsening diabetes-related neurological issues (45). In summary, although vitamin B6 is vital for human health, its intake should be cautious, particularly for type 2 diabetes patients, as excessive supplementation may cause adverse neurological effects. For type 1 diabetes patients, vitamin B6 supplementation might have a protective effect; however, its specific impact and mechanisms require further study. Additionally, for diabetic patients requiring vitamin B6 supplementation, physicians should balance the benefits and risks and choose the appropriate dose and form to minimize the risk of neurotoxicity.
Numerous studies (48, 49) indicate that type 1 diabetes patients commonly exhibit decreased serum magnesium levels, particularly among children and adolescents. Low serum magnesium levels are closely related to poor glycemic control, increased risk of complications, and early development of cardiovascular complications. Oost et al. (50) found that in type 1 diabetes patients receiving high doses of insulin therapy, hypomagnesemia may exacerbate insulin resistance, thereby affecting glycemic control and inflammation levels. Additionally, research suggests (51) that magnesium supplementation can improve glycemic control and lipid profiles in children with type 1 diabetes, indicating that magnesium supplementation may have a positive effect on metabolic control in type 1 diabetes patients. A systematic review and meta-analysis (52) also support the association between decreased magnesium levels and poor glycemic control and/or complications in type 1 diabetes patients. Moreover, decreased magnesium levels are associated with elevated levels of triglycerides, total cholesterol, and low-density lipoprotein cholesterol, as well as reduced high-density lipoprotein cholesterol levels. These findings further underscore the importance of magnesium in metabolic regulation in type 1 diabetes patients. Additionally, magnesium deficiency may interfere with enzymatic reactions in the insulin signaling pathway or induce inflammation or oxidative stress, thereby affecting glucose metabolism (53). Although this condition is usually observed in type 2 diabetes, specific studies on type 1 diabetes still require further exploration and validation. In summary, abnormalities in magnesium metabolism play a crucial role in type 1 diabetes, affecting disease progression and patients’ metabolic status. Therefore, intervention strategies targeting magnesium metabolism may offer new directions for the treatment of type 1 diabetes. Future research should delve into the mechanisms of magnesium in type 1 diabetes and the potential clinical applications of magnesium supplementation.
Du et al. evaluated the effects of carotenoids from Sporidiobolus pararoseus on STZ-induced diabetic nephropathy (DN) in mice. The results showed (54) that these carotenoids significantly alleviated DN symptoms, including reduced fasting blood glucose, decreased urine output, urinary albumin, serum creatinine, and blood urea nitrogen, and improved renal histological morphology. Additionally, they improved oxidative stress status in DN mice by activating the expression of Nrf2, NQO-1, HO-1, GST, and CAT in the kidneys. Shi et al. found (55) that higher carotenoid intake in women was significantly negatively correlated with the prevalence of chronic kidney disease (CKD). However, our findings showed that carotenoids were associated with an increased risk of type 1 diabetes with renal complications, which is inconsistent with previous conclusions. One possible explanation is the difference in study populations and disease types. Furthermore, we focused on the total intake of carotenoids rather than specific sources of carotenoids. Therefore, further in-depth studies may be needed to explore the effects of carotenoids on diabetic nephropathy.
Wu et al. found (56) that supplementation with folic acid and vitamin B12 can reduce serum homocysteine and malondialdehyde levels in patients with diabetes and diabetic nephropathy, decrease the excretion of 24-h urinary microalbumin, and increase levels of nitric oxide and superoxide dismutase. Additionally, with the progression of kidney disease, vitamin B12 levels in T2DM patients decrease while homocysteine levels increase (57). Studies have shown that vitamin B12 has neuroprotective effects in streptozotocin-induced type 1 diabetic rats (58). Specifically, vitamin B12 can reduce neuron apoptosis induced by type 1 diabetes, improve neurotrophic support and synaptic plasticity, and mitigate astrocyte proliferation and endoplasmic reticulum stress (58). In summary, vitamin B12 supplementation not only helps improve the symptoms and progression of diabetic nephropathy but also shows potential significance in neuroprotection. However, there is currently insufficient evidence to support the positive effects of vitamin B12 on kidney complications in type 1 diabetes, although its neuroprotective role is more prominent.
Potassium ions are crucial for maintaining normal nerve cell function, especially in nerve impulse conduction and muscle contraction. The balance of potassium ions is vital for the health of the nervous system. Studies have shown (59) that potassium ion channels play a key role in neuronal excitability and conduction. Imbalances in potassium ion levels can affect nerve function, leading to nerve conduction disorders and neuropathy (59). Additionally, potassium deficiency may exacerbate symptoms of diabetic neuropathy, such as pain and numbness (59). Research indicates (59, 60) that low potassium levels may worsen symptoms of diabetic neuropathy because the lack of potassium’s key role in nerve conduction can lead to neuronal dysfunction, thereby worsening neuropathy. In conclusion, there is a negative causal relationship between potassium and neurological complications in type 1 diabetes, and maintaining appropriate potassium levels may help alleviate these symptoms.
Research indicates that vitamin E significantly contributes to reducing oxidative stress and enhancing vascular health, which is vital for managing diabetes-related peripheral vascular complications. A study highlighted the protective role of antioxidant nutrients, including vitamin E, stating that vitamin E improves endothelial function and reduces oxidative stress (61). The findings of Bi′s study confirm that vitamin E can substantially decrease the occurrence and severity of peripheral circulatory complications in diabetic patients by mitigating oxidative stress and enhancing vascular health (62). Thus, appropriate vitamin E supplementation could be a viable approach to preventing and treating peripheral vascular complications in diabetes.
Oxidative stress plays a pivotal role in the onset and progression of diabetes and its associated complications. Under hyperglycemic conditions, oxidative stress induces cellular damage and inflammatory responses, subsequently leading to complications such as cardiovascular diseases, retinopathy, and nephropathy (63, 64). Previous studies have reported the impact of trace elements and nutrients on oxidative stress. Selenium, as an essential component of glutathione peroxidase (GPx) and thioredoxin reductase (TrxR), is crucial in eliminating peroxides and protecting cells from oxidative damage (65). However, an excess of selenium can disrupt redox homeostasis, resulting in heightened oxidative stress and insulin resistance, thereby exacerbating the complications of diabetes (38–40). Therefore, strict control of selenium supplementation within a safe range is imperative to harness its beneficial effects while avoiding potential harm. Vitamin C, a water-soluble antioxidant, can directly neutralize free radicals, protecting both intracellular and extracellular molecules from oxidative damage. Additionally, it can regenerate other antioxidants such as vitamin E (64, 66). Given the high oxidative stress levels typically present in diabetic patients, vitamin C supplementation can alleviate oxidative stress, improve insulin sensitivity, and reduce the incidence of cardiovascular complications associated with diabetes (67). Vitamin E, predominantly located in cell membranes, protects membrane integrity by inhibiting lipid peroxidation. It neutralizes lipid radicals, thereby preventing oxidative damage to cell membranes (68). Research indicates that vitamin E supplementation can delay the onset and progression of diabetic complications, enhancing vascular endothelial function, retinal blood flow, and renal function in diabetic patients (69). Moreover, copper and manganese are integral components of superoxide dismutase (SOD). SOD catalyzes the dismutation of superoxide anions into oxygen and hydrogen peroxide, which is subsequently decomposed by other antioxidant enzymes such as catalase (70). Thus, adequate levels of copper and manganese are deemed beneficial for maintaining the normal function of the antioxidant defense system, mitigating oxidative stress-related damage in diabetes, and reducing the risk of complications. In summary, the relationship between trace elements, nutrients, and oxidative stress is complex and interrelated. Further research is warranted to comprehensively understand the role of trace elements and nutrients in the pathogenesis and progression of diabetes and its complications through oxidative stress mechanisms.
Diet is the primary source of trace elements and nutrients, and different dietary patterns have a significant impact on the nutritional status of the body. High-carbohydrate diets are typically energy-dense but may be deficient in trace elements and vitamins, particularly those crucial for glucose metabolism and antioxidant defense, such as chromium, magnesium, and the B vitamins. Conversely, diets high in meat products may provide higher intakes of iron and zinc (71). Diets with high intakes of fruits and vegetables are usually associated with higher intakes of vitamin C, vitamin E, β-carotene, and selenium, which are essential for protecting cells from oxidative damage (72, 73). Fruits and vegetables are also rich in fiber, which is vital for maintaining gut health and stabilizing blood glucose levels. Diets low in fruit and vegetable intake may lead to deficiencies in these key nutrients, increasing the risk of diabetes and other chronic diseases. Epidemiological studies have shown an association between dietary patterns and the risk of developing diabetes. High-carbohydrate diets, especially those rich in refined sugars and refined grains, are linked to an increased risk of type 2 diabetes (74, 75). This may be due to the rapid fluctuations in blood glucose and insulin levels caused by high-carbohydrate diets, as well as the decreased antioxidant defense capacity related to inadequate intake of trace elements and vitamins. In contrast, the relationship between high meat consumption and diabetes risk is more complex. Studies have indicated that high red meat intake is associated with an increased risk of diabetes, while high intake of white meat and fish is associated with a lower risk (76). This could reflect differences in the fat and trace element content of different types of meat. Diets high in fruit and vegetable intake are generally associated with a lower risk of diabetes (77). This may be attributed to the high levels of antioxidants and fiber in these foods, which help improve blood glucose control and reduce oxidative stress. In summary, dietary patterns significantly influence the intake of trace elements and nutrients, thereby affecting the risk of developing diabetes. A balanced diet rich in whole grains, fruits, and vegetables, along with moderate consumption of meat products, may be an effective strategy for preventing and managing diabetes.
Cardiovascular disease is one of the primary complications of diabetes, with trace elements playing a crucial role in maintaining cardiovascular health. The antioxidant properties of selenium and zinc protect vascular endothelial cells from oxidative damage (78). Endothelial dysfunction is a key factor in atherosclerosis, the primary pathological basis of cardiovascular disease (79). Thus, the antioxidant defense capabilities of selenium and zinc are significant for preventing atherosclerosis and its complications. Elastin and collagen are major components of the vascular wall, with their structure and function directly affecting the elasticity and tensile strength of blood vessels. Copper is essential in the synthesis of these proteins (80). Defects in copper metabolism can lead to cardiac and muscle dysfunction, thereby increasing the risk of heart disease (81). Additionally, copper plays a role in collagen synthesis and antioxidant defense; supplementation can improve endothelial function and reduce oxidative stress (82). Low selenium levels are associated with an increased risk of cardiovascular disease, and selenium supplements can reduce oxidative stress and inflammation (78). Magnesium is vital for protein synthesis, muscle and nerve function, and blood pressure regulation. Low magnesium levels are linked to an increased risk of cardiovascular disease, and magnesium supplementation has been shown to lower blood pressure and improve endothelial function (78). Withaferin A (WA), an active component of Withania somnifera, exhibits a diverse range of biological activities including anti-inflammatory, immunomodulatory, anti-stress, antioxidant, and anti-angiogenic properties. WA inhibits cancer cell growth through multiple mechanisms, such as inducing apoptosis, suppressing cell migration and invasion, and regulating the cell cycle, demonstrating significant anti-cancer activity across various cancer types (83). Diabetic patients typically experience elevated levels of oxidative stress, which can lead to cellular damage and inflammatory responses, thereby increasing the risk of cardiovascular disease. The antioxidant and anti-inflammatory properties of WA may help reduce the occurrence of cardiovascular complications. Future research should explore the synergistic effects of micronutrients and WA in the prevention and management of cardiovascular issues in diabetic patients. Furthermore, studies have found that there are differences between women and men in terms of cardiovascular disease risk and pathogenesis (84). Future research should focus more on the relationship between trace elements and cardiovascular health in women. This includes studies on gender differences, intervention measures, and clinical trials, to understand the role of trace elements in women’s cardiovascular health. Such research could provide new insights and methods for the prevention and treatment of cardiovascular diseases in women. In summary, the impact of trace elements on cardiovascular function is multifaceted, encompassing antioxidant properties and the maintenance of vascular structure and function. Considering the role of trace elements in the study of diabetic complications is essential for a comprehensive understanding of disease mechanisms and the development of effective treatment strategies. Therefore, the influence of trace elements on cardiovascular function, including their antioxidant effects and roles in maintaining vascular structure and function, is paramount in understanding and managing diabetic complications.
In 2022, the European Society of Clinical Nutrition and Metabolism (ESPEN) published micronutrient guidelines (85). We have summarized the common micronutrients covered in them for adequate intake, dietary reference intake, enteral nutrition, tolerable upper intake level, parenteral nutrition, recommended dietary allowances, and risk of deficiency and excess, which are summarized in the Table 3. By referring to ESPEN’s micronutrient guidelines, clinicians can be helped to more accurately assess the nutritional needs of patients with diabetes, better formulate nutritional support programmes to ensure that they receive adequate and not excessive amounts of micronutrients, optimize health outcomes, and improve patients’ quality of life.
The strengths of this study are as follows: First, the analysis results indicate that magnesium, vitamin B12, potassium, vitamin B6, and vitamin E have protective effects against diabetes and its complications, whereas selenium, vitamin B6, and carotene are risk factors for diabetes and its complications. Therefore, for high-risk clinical populations, controlling the intake of selenium and carotene and appropriately supplementing magnesium, potassium, vitamin B12, and vitamin E in daily life may be a potential measure for preventing diabetes and its complications. Additionally, this study provides possible experimental directions for researching the molecular mechanisms through which trace nutrients influence diabetes, laying a research foundation for the future prevention and treatment of diabetes and its complications from the perspective of biological trace nutrients. Second, the study used publicly available GWAS data for causal inference, resulting in a larger sample size and higher statistical power.
However, this study has several limitations: First, the study is limited to European populations, and the results may not be generalizable to populations with different genetic backgrounds. There could be significant differences in various racial backgrounds. Second, this study is primarily a foundational theoretical research and requires more animal experiments and cohort studies to confirm the conclusions before they can be better applied in clinical settings. Additionally, the different impacts of trace elements and nutrients on type 1 and type 2 diabetes and their complications can be attributed to the distinct pathogenic mechanisms of the two types. Type 1 diabetes is caused by the extensive destruction of pancreatic β-cells, ultimately leading to absolute insulin deficiency. Type 2 diabetes primarily stems from insulin resistance and progressive deterioration of insulin secretion. Due to their different pathogenic mechanisms, their effects on different subtypes of diabetes and its complications vary. Furthermore, type 2 diabetes mainly occurs in middle-aged and older adults (typically over the age of 40), whereas type 1 diabetes usually occurs in children and adolescents, who generally have different intakes of trace elements and nutrients. This factor may also play a role in elucidating their differential impacts between the subtypes.
We have found that the intake of micronutrients may have a nonlinear relationship with health outcomes, using selenium as an example. Research has shown that when selenium intake exceeds the nutritionally required levels, the risk of T2D increases with higher selenium intake. However, it is currently uncertain whether low selenium status promotes T2D risk in humans. Although case–control studies have suggested a U-shaped response of T2D risk to selenium status, with approximately 100 μg/L of serum selenium being the point of lowest risk, this has not been supported by cohort or cross-sectional studies. In animal studies, researchers compared different dietary selenium levels and specific selenoproteins in a homogeneous background, finding that low selenium status is associated with increased T2D risk. These studies collectively suggest that increased oxidative stress in both high and low selenium status may be a mechanism leading to higher T2D risk. Overall, current evidence indicates that T2D risk is minimized at the bottom of the U-shaped dose–response curve for selenium intake, meaning that both selenium deficiency and excessive supplementation can increase the risk.
We propose several potential reasons for the nonlinear relationship between micronutrient intake and health outcomes: (i) Optimal Range: Each micronutrient has an optimal intake range within the body, where it can most effectively support health. Intake below this range can lead to deficiency diseases, affecting normal physiological functions, while intake above this range can cause toxicity and health problems (refer to Table 3). (ii) U-shaped or J-shaped Curve: Many micronutrients exhibit U-shaped or J-shaped dose–response relationships, indicating that both insufficient and excessive intake increase the risk of diseases, with moderate intake being the safest (refer to Table 3). (iii) Regulatory Mechanism Limitations: The body has complex mechanisms to regulate micronutrient levels. For example, when the intake of a specific nutrient is low, the body increases its absorption and reduces its excretion. However, when intake is too high, these regulatory mechanisms may fail, leading to increased risk from overconsumption.(iv) Nutrient Interactions: There are interactions between different micronutrients that affect their bioavailability. For instance, high doses of zinc can lead to copper deficiency (refer to Table 3). Therefore, excessive intake of one nutrient can cause deficiency in another, resulting in health problems. (v) Individual Differences: Factors such as genetics, age, sex, and health status can affect the optimal intake levels of micronutrients. For example, the nutritional needs of pregnant and lactating women differ from those of the general adult population, leading to varying risks and impacts of under- or overconsumption.
In summary, the nonlinear relationship between micronutrient intake and health outcomes is primarily due to the body’s complex regulatory mechanisms, bioavailability, individual differences, and nutrient interactions. Therefore, we recommend conducting further large-scale, long-term cohort studies to confirm and refine the nonlinear relationship between micronutrient intake and health outcomes. Additionally, personalized nutritional assessment and supplementation plans should be developed based on individual health status, lifestyle, and genetic background. Regular monitoring of patients’ micronutrient levels is essential, with dynamic adjustments to supplementation doses based on test results to prevent health issues arising from long-term high or low intake.
In conclusion, our study elucidates the causal relationship between trace elements, nutrients, and diabetes along with its complications through Mendelian randomization analysis. This insight offers a new perspective for subsequent mechanistic studies and provides new scientific evidence and strategies for the clinical application of micronutrients in the prevention and treatment of diabetes and its complications.
The datasets presented in this study can be found in online repositories. The names of the repository/repositories and accession number(s) can be found in the article/Supplementary material.
Ethical approval was not required for the studies involving humans because Ethical approval was not required for the study involving humans in accordance with the local legislation and institutional requirements. Written informed consent to participate in this study was not required from the participants or the participants’ legal guardians/next of kin in accordance with the national legislation and the institutional requirements. All data in this study were generated from studies approved by the respective ethics committees, and informed consent was granted to all subjects. Therefore, no additional approval from the institutional review board was required. The studies were conducted in accordance with the local legislation and institutional requirements. The participants provided their written informed consent to participate in this study. Written informed consent was obtained from the individual(s) for the publication of any potentially identifiable images or data included in this article.
M-JJ: Writing – original draft, Writing – review & editing. LC: Writing – original draft, Writing – review & editing.
The author(s) declare that financial support was received for the research, authorship, and/or publication of this article. This research was funded by National Natural Science Foundation of China (grant number: 82274419) and Natural Science Foundation of Guangdong (grant number: 2020A1515010775).
The authors declare that the research was conducted in the absence of any commercial or financial relationships that could be construed as a potential conflict of interest.
All claims expressed in this article are solely those of the authors and do not necessarily represent those of their affiliated organizations, or those of the publisher, the editors and the reviewers. Any product that may be evaluated in this article, or claim that may be made by its manufacturer, is not guaranteed or endorsed by the publisher.
The Supplementary material for this article can be found online at: https://www.frontiersin.org/articles/10.3389/fnut.2024.1439217/full#supplementary-material
1. ^https://gwas.mrcieu.ac.uk/
2. ^https://www.finngen.fi/en/access_results
1. Duh, EJ, Sun, JK, and Stitt, AW. Diabetic retinopathy: current understanding, mechanisms, and treatment strategies. JCI Insight. (2017) 2:e93751. doi: 10.1172/jci.insight.93751
2. Dal Canto, E, Ceriello, A, Rydén, L, Ferrini, M, Hansen, TB, Schnell, O, et al. Diabetes as a cardiovascular risk factor: an overview of global trends of macro and micro vascular complications. Eur J Prev Cardiol. (2019) 26:25–32. doi: 10.1177/2047487319878371
3. Peltier, A, Goutman, SA, and Callaghan, BC. Painful diabetic neuropathy. BMJ. (2014) 348:g1799. doi: 10.1136/bmj.g1799
4. Bommer, C, Heesemann, E, Sagalova, V, Manne-Goehler, J, Atun, R, Bärnighausen, T, et al. The global economic burden of diabetes in adults aged 20-79 years: a cost-of-illness study. Lancet Diabetes Endocrinol. (2017) 5:423–30. doi: 10.1016/S2213-8587(17)30097-9
5. Boyle, JP, Thompson, TJ, Gregg, EW, Barker, LE, and Williamson, DF. Projection of the year 2050 burden of diabetes in the US adult population: dynamic modeling of incidence, mortality, and prediabetes prevalence. Popul Health Metrics. (2010) 8:29. doi: 10.1186/1478-7954-8-29
6. Marso, SP, and Hiatt, WR. Peripheral arterial disease in patients with diabetes. J Am Coll Cardiol. (2006) 47:921–9. doi: 10.1016/j.jacc.2005.09.065
7. Criqui, MH, and Aboyans, V. Epidemiology of peripheral artery disease. Circ Res. (2015) 116:1509–26. doi: 10.1161/CIRCRESAHA.116.303849
8. Adler, AI, Stevens, RJ, Neil, A, Stratton, IM, Boulton, AJM, Holman, RR, et al. UKPDS 59: hyperglycemia and other potentially modifiable risk factors for peripheral vascular disease in type 2 diabetes. Diabetes Care. (2002) 25:894–9. doi: 10.2337/diacare.25.5.894
9. Barnes, JA, Eid, MA, Creager, MA, and Goodney, PP. Epidemiology and risk of amputation in patients with diabetes mellitus and peripheral artery disease. Arterioscler Thromb Vasc Biol. (2020) 40:1808–17. doi: 10.1161/ATVBAHA.120.314595
10. Teo, ZL, Tham, YC, Yu, M, Chee, ML, Rim, TH, Cheung, N, et al. Global prevalence of diabetic retinopathy and projection of burden through 2045: systematic review and Meta-analysis. Ophthalmology. (2021) 128:1580–91. doi: 10.1016/j.ophtha.2021.04.027
11. Fong, DS, Aiello, L, Gardner, TW, King, GL, Blankenship, G, Cavallerano, JD, et al. Retinopathy in diabetes. Diabetes Care. (2004) 27:S84–7. doi: 10.2337/diacare.27.2007.s84
12. Cheung, N, Mitchell, P, and Wong, TY. Diabetic retinopathy. Lancet. (2010) 376:124–36. doi: 10.1016/S0140-6736(09)62124-3
13. Saran, R, Li, Y, Robinson, B, Abbott, KC, Agodoa, LYC, Ayanian, J, et al. US renal data system 2015 annual data report: epidemiology of kidney disease in the United States. Am J Kidney Dis. (2016) 67:A7. doi: 10.1053/j.ajkd.2015.12.014
14. Reutens, AT . Epidemiology of diabetic kidney disease. Med Clin North Am. (2013) 97:1–18. doi: 10.1016/j.mcna.2012.10.001
15. Selby, NM, and Taal, MW. An updated overview of diabetic nephropathy: diagnosis, prognosis, treatment goals and latest guidelines. Diabetes Obes Metab. (2020) 22:3–15. doi: 10.1111/dom.14007
16. Tuttle, KR, Brosius, FC 3rd, Cavender, MA, Fioretto, P, Fowler, KJ, Heerspink, HJL, et al. SGLT2 inhibition for CKD and cardiovascular disease in type 2 diabetes: report of a scientific workshop sponsored by the National Kidney Foundation. Diabetes. (2021) 70:1–16. doi: 10.2337/dbi20-0040
17. GBD Chronic Kidney Disease Collaboration . Global, regional, and national burden of chronic kidney disease, 1990-2017: a systematic analysis for the global burden of disease study 2017. Lancet. (2020) 395:709–33. doi: 10.1016/S0140-6736(20)30045-3
18. Rodríguez-Pérez, C, Gómez-Peña, C, Pérez-Carrascosa, FM, Vrhovnik, P, Echeverría, R, Salcedo-Bellido, I, et al. Trace elements concentration in adipose tissue and the risk of incident type 2 diabetes in a prospective adult cohort. Environ Pollut. (2021) 286:117496. doi: 10.1016/j.envpol.2021.117496
19. Oost, LJ, Tack, CJ, and de Baaij, JHF. Hypomagnesemia and cardiovascular risk in type 2 diabetes. Endocr Rev. (2023) 44:357–78. doi: 10.1210/endrev/bnac028
20. Cai, X, Hu, Z, Zhang, M, Dang, Q, Yang, Q, Zhao, X, et al. Dosage-effect of selenium supplementation on blood glucose and oxidative stress in type 2 diabetes mellitus and normal mice. J Trace Elements Med Biol. (2024) 83:127410. doi: 10.1016/j.jtemb.2024.127410
21. Castillo-Otí, JM, Galván-Manso, AI, Callejas-Herrero, MR, Vara-González, LA, Salas-Herrera, F, and Muñoz-Cacho, P. Vitamin D deficiency is significantly associated with retinopathy in type 2 diabetes mellitus: a case-control study. Nutrients. (2021) 14:84. doi: 10.3390/nu14010084
22. Xiao, Y, Wei, L, Xiong, X, Yang, M, and Sun, L. Association between vitamin D status and diabetic complications in patients with type 2 diabetes mellitus: a cross-sectional study in Hunan China. Front Endocrinol. (2020) 11:564738. doi: 10.3389/fendo.2020.564738
23. Zhu, RC, Li, FF, Wu, YQ, Yi, QY, and Huang, XF. Minimal effect of sleep on the risk of age-related macular degeneration: a Mendelian randomization study. Front Aging Neurosci. (2023) 15:1159711. doi: 10.3389/fnagi.2023.1159711
24. Di Resta, C, Pipitone, GB, Carrera, P, and Ferrari, M. Current scenario of the genetic testing for rare neurological disorders exploiting next generation sequencing. Neural Regen Res. (2021) 16:475–81. doi: 10.4103/1673-5374.293135
25. Zhong, X, Wang, L, Xu, L, Lian, J, Chen, J, Gong, X, et al. Disturbance of skin sensation and autism spectrum disorder: a bidirectional Mendelian randomization study. Brain Behav. (2023) 13:e3238. doi: 10.1002/brb3.3238
26. Skrivankova, VW, Richmond, RC, Woolf, BAR, Yarmolinsky, J, Davies, NM, Swanson, SA, et al. Strengthening the reporting of observational studies in epidemiology using Mendelian randomization: the STROBE-MR statement. JAMA. (2021) 326:1614–21. doi: 10.1001/jama.2021.18236
27. Sanderson, E . Multivariable Mendelian randomization and mediation. Cold Spring Harb Perspect Med. (2021) 11:a038984. doi: 10.1101/cshperspect.a038984
28. Sanderson, E, Davey Smith, G, Windmeijer, F, and Bowden, J. An examination of multivariable Mendelian randomization in the single-sample and two-sample summary data settings. Int J Epidemiol. (2019) 48:713–27. doi: 10.1093/ije/dyy262
29. Hemani, G, Zheng, J, Elsworth, B, Wade, KH, Haberland, V, Baird, D, et al. The MR-base platform supports systematic causal inference across the human phenome. eLife. (2018) 7:e34408. doi: 10.7554/eLife.34408
30. Stranges, S, Marshall, JR, Natarajan, R, Donahue, RP, Trevisan, M, Combs, GF, et al. Effects of long-term selenium supplementation on the incidence of type 2 diabetes: a randomized trial. Ann Intern Med. (2007) 147:217–23. doi: 10.7326/0003-4819-147-4-200708210-00175
31. Laclaustra, M, Navas-Acien, A, Stranges, S, Ordovas, JM, and Guallar, E. Serum selenium concentrations and diabetes in U.S. adults: National Health and nutrition examination survey (NHANES) 2003-2004. Environ Health Perspect. (2009) 117:1409–13. doi: 10.1289/ehp.0900704
32. Wei, J, Zeng, C, Gong, QY, Yang, HB, Li, XX, Lei, GH, et al. The association between dietary selenium intake and diabetes: a cross-sectional study among middle-aged and older adults. Nutr J. (2015) 14:18. doi: 10.1186/s12937-015-0007-2
33. Akbaraly, TN, Arnaud, J, Rayman, MP, Hininger-Favier, I, Roussel, AM, Berr, C, et al. Plasma selenium and risk of dysglycemia in an elderly French population: results from the prospective epidemiology of vascular ageing study. Nutr Metab. (2010) 7:21. doi: 10.1186/1743-7075-7-21
34. Yang, KC, Lee, LT, Lee, YS, Huang, HY, Chen, CY, and Huang, KC. Serum selenium concentration is associated with metabolic factors in the elderly: a cross-sectional study. Nutr Metabolism. (2010) 7:38. doi: 10.1186/1743-7075-7-38
35. Stranges, S, Sieri, S, Vinceti, M, Grioni, S, Guallar, E, Laclaustra, M, et al. A prospective study of dietary selenium intake and risk of type 2 diabetes. BMC Public Health. (2010) 10:564. doi: 10.1186/1471-2458-10-564
36. Misu, H, Takamura, T, Takayama, H, Hayashi, H, Matsuzawa-Nagata, N, Kurita, S, et al. A liver-derived secretory protein, selenoprotein P, causes insulin resistance. Cell Metab. (2010) 12:483–95. doi: 10.1016/j.cmet.2010.09.015
37. Mita, Y, Nakayama, K, Inari, S, Nishito, Y, Yoshioka, Y, Sakai, N, et al. Selenoprotein P-neutralizing antibodies improve insulin secretion and glucose sensitivity in type 2 diabetes mouse models. Nat Commun. (2017) 8:1658. doi: 10.1038/s41467-017-01863-z
38. McClung, JP, Roneker, CA, Mu, W, Lisk, DJ, Langlais, P, Liu, F, et al. Development of insulin resistance and obesity in mice overexpressing cellular glutathione peroxidase. Proc Natl Acad Sci USA. (2004) 101:8852–7. doi: 10.1073/pnas.0308096101
39. Loh, K, Deng, H, Fukushima, A, Cai, X, Boivin, B, Galic, S, et al. Reactive oxygen species enhance insulin sensitivity. Cell Metab. (2009) 10:260–72. doi: 10.1016/j.cmet.2009.08.009
40. Huang, YC, Combs, GF Jr, Wu, TL, Zeng, H, and Cheng, WH. Selenium status and type 2 diabetes risk. Arch Biochem Biophys. (2022) 730:109400. doi: 10.1016/j.abb.2022.109400
41. Mao, J, and Teng, W. The relationship between selenoprotein P and glucose metabolism in experimental studies. Nutrients. (2013) 5:1937–48. doi: 10.3390/nu5061937
42. Yu, R, Wang, Z, Ma, M, Xu, P, Liu, L, Tinkov, AA, et al. Associations between circulating SELENOP level and disorders of glucose and lipid metabolism: a meta-analysis. Antioxidants. (2022) 11:1263. doi: 10.3390/antiox11071263
43. Muhamad, R, Akrivaki, A, Papagiannopoulou, G, Zavridis, P, and Zis, P. The role of vitamin B6 in peripheral neuropathy: a systematic review. Nutrients. (2023) 15:2823. doi: 10.3390/nu15132823
44. Reddy, P . Preventing vitamin B6-related neurotoxicity. Am J Ther. (2022) 29:e637–43. doi: 10.1097/MJT.0000000000001460
45. Mascolo, E, and Vernì, F. Vitamin B6 and diabetes: relationship and molecular mechanisms. Int J Mol Sci. (2020) 21:3669. doi: 10.3390/ijms21103669
46. Khobrani, M, Kandasamy, G, Vasudevan, R, Alhossan, A, Puvvada, RC, Devanandan, P, et al. Impact of vitamin B6 deficiency on the severity of diabetic peripheral neuropathy - a cross sectional study. Saudi Pharm J. (2023) 31:655–8. doi: 10.1016/j.jsps.2023.03.005
47. Stach, K, Stach, W, and Augoff, K. Vitamin B6 in health and disease. Nutrients. (2021) 13:3229. doi: 10.3390/nu13093229
48. Lin, CC, Tsweng, GJ, Lee, CF, Chen, BH, and Huang, YL. Magnesium, zinc, and chromium levels in children, adolescents, and young adults with type 1 diabetes. Clin Nutr. (2016) 35:880–4. doi: 10.1016/j.clnu.2015.05.022
49. Galli-Tsinopoulou, A, Maggana, I, Kyrgios, I, Mouzaki, K, Grammatikopoulou, MG, Stylianou, C, et al. Association between magnesium concentration and HbA1c in children and adolescents with type 1 diabetes mellitus. J Diabetes. (2014) 6:369–77. doi: 10.1111/1753-0407.12118
50. Oost, LJ, van Heck, JIP, Tack, CJ, and de Baaij, JHF. The association between hypomagnesemia and poor glycaemic control in type 1 diabetes is limited to insulin resistant individuals. Sci Rep. (2022) 12:6433. doi: 10.1038/s41598-022-10436-0
51. Shahbah, D, Hassan, T, Morsy, S, Saadany, HE, Fathy, M, al-Ghobashy, A, et al. Oral magnesium supplementation improves glycemic control and lipid profile in children with type 1 diabetes and hypomagnesaemia. Medicine. (2017) 96:e6352. doi: 10.1097/MD.0000000000006352
52. Rodrigues, AK, Melo, AE, and Domingueti, CP. Association between reduced serum levels of magnesium and the presence of poor glycemic control and complications in type 1 diabetes mellitus: a systematic review and meta-analysis. Diabetes Metab Syndr. (2020) 14:127–34. doi: 10.1016/j.dsx.2020.01.015
53. Dubey, P, Thakur, V, and Chattopadhyay, M. Role of minerals and trace elements in diabetes and insulin resistance. Nutrients. (2020) 12:1864. doi: 10.3390/nu12061864
54. Du, C, Lv, T, and Liu, Q. Carotenoids in Sporidiobolus pararoseus ameliorate diabetic nephropathy in mice through attenuating oxidative stress. Biol Chem. (2021) 402:785–94. doi: 10.1515/hsz-2021-0127
55. Shi, Y, Xu, Y, and Zhou, W. Dietary carotenoids intake and sex differences in relation to chronic kidney disease a cross-sectional assessment in the NHANES study. BMC Public Health. (2024) 24:293. doi: 10.1186/s12889-024-17771-z
56. Wu, HHL, and Wang, AY. Vitamin B12 and chronic kidney disease. Vitam Horm. (2022) 119:325–53. doi: 10.1016/bs.vh.2022.01.011
57. Kibirige, D, and Mwebaze, R. Vitamin B12 deficiency among patients with diabetes mellitus: is routine screening and supplementation justified? J Diabetes Metab Disord. (2013) 12:17. doi: 10.1186/2251-6581-12-17
58. Suryavanshi, U, Angadi, KK, Reddy, VS, and Reddy, GB. Neuroprotective role of vitamin B12 in streptozotocin-induced type 1 diabetic rats. Chem Biol Interact. (2024) 387:110823. doi: 10.1016/j.cbi.2023.110823
59. Pop-Busui, R, Ang, L, and Boulton, AJM. Diagnosis and treatment of painful diabetic peripheral neuropathy American Diabetes Association (2022).
60. Feldman, EL, Callaghan, BC, Pop-Busui, R, Zochodne, DW, Wright, DE, Bennett, DL, et al. Diabetic neuropathy. Dis Primers. (2019) 5:42. doi: 10.1038/s41572-019-0097-9
61. Chan, YH, Schooling, CM, Zhao, JV, Yeung, SLA, Hai, JJ, Thomas, GN, et al. Mendelian randomization analysis of vitamin D in the secondary prevention of hypertensive-diabetic subjects: role of facilitating blood pressure control. Genes Nutr. (2022) 17:1. doi: 10.1186/s12263-022-00704-z
62. Bi, Y, Gao, Y, Xie, Y, Zhou, M, Liu, Z, Tian, S, et al. White blood cells and type 2 diabetes: a Mendelian randomization study. PLoS One. (2024) 19:e0296701. doi: 10.1371/journal.pone.0296701
63. Singh, H, Singh, R, Singh, A, Singh, H, Singh, G, Kaur, S, et al. Role of oxidative stress in diabetes-induced complications and their management with antioxidants. Arch Physiol Biochem. (2023):1–26. doi: 10.1080/13813455.2023.2243651
64. Abdel-Daim, MM, Abo-el-Sooud, K, Aleya, L, Bungǎu, SG, Najda, A, and Saluja, R. Alleviation of drugs and chemicals toxicity: biomedical value of antioxidants. Oxidat Med Cell Longev. (2018) 2018:1–2. doi: 10.1155/2018/6276438
65. Steinbrenner, H, Speckmann, B, and Klotz, LO. Selenoproteins: antioxidant selenoenzymes and beyond. Arch Biochem Biophys. (2016) 595:113–9. doi: 10.1016/j.abb.2015.06.024
66. Mason, SA, Parker, L, van der Pligt, P, and Wadley, GD. Vitamin C supplementation for diabetes management: a comprehensive narrative review. Free Radic Biol Med. (2023) 194:255–83. doi: 10.1016/j.freeradbiomed.2022.12.003
67. Mason, SA, Keske, MA, and Wadley, GD. Effects of vitamin C supplementation on glycemic control and cardiovascular risk factors in people with type 2 diabetes: a GRADE-assessed systematic review and Meta-analysis of randomized controlled trials. Diabetes Care. (2021) 44:618–30. doi: 10.2337/dc20-1893
68. Pazdro, R, and Burgess, JR. The role of vitamin E and oxidative stress in diabetes complications. Mech Ageing Dev. (2010) 131:276–86. doi: 10.1016/j.mad.2010.03.005
69. Baburao Jain, A, and Anand Jain, V. Vitamin E, its beneficial role in diabetes mellitus (DM) and its complications. J Clin Diagn Res. (2012) 6:1624–8. doi: 10.7860/JCDR/2012/4791.2625
70. Wang, Y, Branicky, R, Noë, A, and Hekimi, S. Superoxide dismutases: dual roles in controlling ROS damage and regulating ROS signaling. J Cell Biol. (2018) 217:1915–28. doi: 10.1083/jcb.201708007
71. Agarwal, S, and Fulgoni, VL. Contribution of beef to key nutrient intakes in American adults: an updated analysis with NHANES 2011-2018. Nutr Res. (2022) 105:105–12. doi: 10.1016/j.nutres.2022.06.009
72. Nishi, SK, Khoury, N, Valle Hita, C, Zurbau, A, Salas-Salvadó, J, and Babio, N. Vegetable and fruit intake variety and cardiovascular health and mortality: a systematic review and Meta-analysis of observational studies. Nutrients. (2023) 15:4913. doi: 10.3390/nu15234913
73. Institute of Medicine (US) Panel on Dietary Antioxidants and Related Compounds . Dietary reference intakes for vitamin C In: Vitamin E, selenium, and carotenoids. National: Academies Press (US) (2000)
74. Gaesser, GA . Perspective: refined grains and health: genuine risk, or guilt by association? Adv Nutr. (2019) 10:361–71. doi: 10.1093/advances/nmy104
75. Gross, LS, Li, L, Ford, ES, and Liu, S. Increased consumption of refined carbohydrates and the epidemic of type 2 diabetes in the United States: an ecologic assessment. Am J Clin Nutr. (2004) 79:774–9. doi: 10.1093/ajcn/79.5.774
76. Du, H, Guo, Y, Bennett, DA, Bragg, F, Bian, Z, Chadni, M, et al. Red meat, poultry and fish consumption and risk of diabetes: a 9 year prospective cohort study of the China Kadoorie biobank. Diabetologia. (2020) 63:767–79. doi: 10.1007/s00125-020-05091-x
77. Schwingshackl, L, Hoffmann, G, Lampousi, AM, Knüppel, S, Iqbal, K, Schwedhelm, C, et al. Food groups and risk of type 2 diabetes mellitus: a systematic review and meta-analysis of prospective studies. Eur J Epidemiol. (2017) 32:363–75. doi: 10.1007/s10654-017-0246-y
78. Wechselberger, C, Messner, B, and Bernhard, D. The role of trace elements in cardiovascular diseases. Toxics. (2023) 11:956. doi: 10.3390/toxics11120956
79. Xu, S, Ilyas, I, Little, PJ, Li, H, Kamato, D, Zheng, X, et al. Endothelial dysfunction in atherosclerotic cardiovascular diseases and beyond: from mechanism to pharmacotherapies. Pharmacol Rev. (2021) 73:924–67. doi: 10.1124/pharmrev.120.000096
80. Wang, K, Meng, X, and Guo, Z. Elastin structure, synthesis, regulatory mechanism and relationship with cardiovascular diseases. Front Cell Dev Biol. (2021) 9:596702. doi: 10.3389/fcell.2021.596702
81. Liu, Y, and Miao, J. An emerging role of defective copper metabolism in heart disease. Nutrients. (2022) 14:700. doi: 10.3390/nu14030700
82. das, A, Sudhahar, V, Chen, GF, Kim, HW, Youn, SW, Finney, L, et al. Endothelial Antioxidant-1: a Key mediator of copper-dependent wound healing in vivo. Sci Rep. (2016) 6:33783. doi: 10.1038/srep33783
83. Sivasankarapillai, VS, Madhu Kumar Nair, R, Rahdar, A, Bungau, S, Zaha, DC, Aleya, L, et al. Overview of the anticancer activity of withaferin a, an active constituent of the Indian ginseng Withania somnifera. Environ Sci Pollut Res Int. (2020) 27:26025–35. doi: 10.1007/s11356-020-09028-0
84. Gheorghe, G, Toth, PP, Bungau, S, Behl, T, Ilie, M, Pantea Stoian, A, et al. Cardiovascular risk and statin therapy considerations in women. Diagnostics. (2020) 10:483. doi: 10.3390/diagnostics10070483
Keywords: Mendelian randomization, type 1 diabetes mellitus, type 2 diabetes mellitus, diabetes and its complications, trace elements
Citation: Jia M-J and Chen L (2024) Effect of trace elements and nutrients on diabetes and its complications: a Mendelian randomization study. Front. Nutr. 11:1439217. doi: 10.3389/fnut.2024.1439217
Received: 27 May 2024; Accepted: 23 July 2024;
Published: 01 August 2024.
Edited by:
Chaoyan Yue, Fudan University, ChinaReviewed by:
Mostafa Waly, Sultan Qaboos University, OmanCopyright © 2024 Jia and Chen. This is an open-access article distributed under the terms of the Creative Commons Attribution License (CC BY). The use, distribution or reproduction in other forums is permitted, provided the original author(s) and the copyright owner(s) are credited and that the original publication in this journal is cited, in accordance with accepted academic practice. No use, distribution or reproduction is permitted which does not comply with these terms.
*Correspondence: Ming-Jie Jia, eXExMzMyMjY0NjAxQDE2My5jb20=
†These authors have contributed equally to this work
Disclaimer: All claims expressed in this article are solely those of the authors and do not necessarily represent those of their affiliated organizations, or those of the publisher, the editors and the reviewers. Any product that may be evaluated in this article or claim that may be made by its manufacturer is not guaranteed or endorsed by the publisher.
Research integrity at Frontiers
Learn more about the work of our research integrity team to safeguard the quality of each article we publish.