- 1College of Life Sciences, Hunan Provincial Key Laboratory of Biological Resources Protection and Utilization in Nanyue Mountain Area, Hengyang Normal University, Hengyang, Hunan, China
- 2Hunan International Joint Laboratory of Animal Intestinal Ecology and Health, Hunan Normal University, Changsha, Hunan, China
- 3College of Bioengineering, Hunan Vocational Technical College of Environment and Biology, Hengyang, Hunan, China
- 4Key Laboratory of Agro-Ecological Processes in Subtropical Region, Institute of Subtropical Agriculture, Chinese Academy of Science, Changsha, Hunan, China
To find out whether dietary amylose/ amylopectin ratio (DAR) could attenuate injury in lipopolysaccharide (LPS)-challenged piglets, sixty male weaned piglets (Duroc × Landrace × Yorkshire, 21 days old, 6.51 ± 0.64 kg) were allotted to 5 dietary treatments with 12 cages per treatment, and fed ad libitum with diets different in DAR (0.00, 0.20, 0.40, 0.60 and 0.80). Feed transformation occurred from D15 to D21. On day 28, 12 h before slaughter, pigs were intraperitoneal injected with 100 μg/kg body weight LPS or sterile saline. Results showed that LPS stress caused an increase in serum urea nitrogen (UREA) and triglyceride (TG), but a decrease in alanine aminotransferase (ALT) activity and glucose (GLU) concentration (p < 0.05). Serum immunoglobulin G (IgG) concentration increased in DAR 0.80 but decreased in other groups after LPS stress (p < 0.05). Compared with the control group, concentrations of Ile, Leu, Phe, Val, Thr, Arg decreased in serum but increased in liver after LPS stress (p < 0.05). Serum Arg, Tyr, Sar, Ans, Orn increased linearly with increasing DAR (p < 0.05). Piglets in diet DAR 0.00 had highest superoxide dismutase (SOD1) and glutathione peroxidase 1 (GPX1) mRNA expression in liver than those in other groups (p < 0.05). There was significant effect of LPS stress * dietary DAR on total SOD activity and SOD1 mRNA gene expression (p < 0.05), LPS stress caused an increase in those two indices for pigs in groups 0.00 and 0.80. Piglets in diet 0.80 had the highest hepatic Cu, Fe, Mn, Zn concentrations than those in other groups (p < 0.05). Cecal indol(e) concentration was higher in diet 0.00 than that in diet 0.80 (p < 0.05). After LPS stress, colonic skatole concentration increased in DAR 0.40, 0.80 but decreased in other groups (p < 0.05). In conclusion, adverse effects of the LPS challenge could be reversed by feeding weaned piglets with low or high DAR diet through regulating amino metabolism and antioxidant function.
1 Introduction
Lipopolysaccharide (LPS) is a major component of the outer membrane of gram-negative bacteria such as Escherichia coli. It triggers a systemic inflammatory process by releasing pro-inflammatory mediators, nitric oxide, and reactive oxygen species (ROS), which are associated with oxidative stress (1). Weaned piglets with weakened mucosal barrier resistance to pathogens are particularly vulnerable to infections by enterotoxigenic E. coli. This strain of E. coli produces and secretes LPS, leading to intestinal dysfunction (2), oxidative stress (3), and hepatic damage, especially at doses like 80 μg/kg body weight of LPS (4). Pigs treated with LPS exhibited hepatocyte nuclear lysis, fibroblast proliferation, and karyopyknosis (5). Furthermore, LPS-induced immune stimulation decreases plasma flux for amino acids such as lysine (Lys), phenylalanine (Phe), and isoleucine (Ile) (6). However, stress biomarkers of inflammation in LPS-challenged piglets can be partially mitigated through supplementation with a 0.3% amino acid mixture containing arginine (Arg), leucine (Leu), valine (Val), and isoleucine (Ile), and cysteine (Cys) (7). Supplementation with tryptophan (Try) has been shown to ameliorate liver damage by reducing activities of alanine aminotransferase (ALT) and aspartate aminotransferase (AST), maintaining the barrier function of the liver and alleviating hepatic oxidative stress in LPS-challenged piglets (5). Additionally, citrulline (Cit) and arginine deiminase (ADI-PEG20) may exert protective effects against inflammation by reducing CD45+ infiltrates in the liver and lowering plasma levels of pro-inflammatory cytokines in the piglets infused with LPS infusion (8).
Starch is composed of amylose and amylopectin. Waxy maize starches exhibit an A-type X-ray diffraction pattern, whereas high-amylose maize shows a B-type pattern (9) and a lower degree of crystallinity than waxy maize starches (10). Compared with waxy maize starch (DAR 0.07) and non-waxy maize starch (DAR 0.19), feeding finishing pigs a pea starch (DAR 0.28) diet downregulated gluconeogenesis, resulting in less fat and more protein deposition in the liver (11). Compared with normal corn starch, supplementing mice with 10% high-amylose maize enhanced the intestinal absorption of calcium, iron, and magnesium (12). Indigestible starch can be converted into short-chain volatile fatty acids (SCFAs) by intestinal microorganisms in the hindgut (13). Increasing carbohydrate availability by cecal corn starch infusion decreases hindgut aromatic amino acid (AAA) metabolism while increasing systemic AAA availability, thereby promoting hypothalamic neurotransmitter synthesis (14). Additionally, the degree of oxidative stress and inflammation was alleviated in chronic kidney disease (CKD) rats after the consumption of 59% high-amylose maize-resistant starch (HAMRS2) (15).
In commercial pig farms, post-weaning diarrhea usually occurs when weaned piglets are exposed to enterotoxigenic E. coli after undergoing the transitional weaning periods, which include changes in feed, environment, and separation from the mother. A dose of 100 μg/kg of LPS secreted by E. coli has been shown to cause both hepatic damage and gut injury (4). However, whether different dietary amylose/amylopectin ratios (DAR), achieved by blending high-amylose maize starch and waxy maize starch, can differently improve piglets’ resilience under such challenges by regulating amino acid metabolism and liver antioxidant function remains unclear. Thus, this study examined the effect of dietary amylose/amylopectin ratio on the amino acid concentration in serum and liver, liver mineral element content, and liver antioxidant function in weaned piglets under LPS stress. We hypothesized that both high and low DAR diets could improve piglets’ health by modulating amino acid metabolism and antioxidant function during stress.
2 Materials and methods
The experimental procedure in this study was reviewed and approved by the Animal Care and Use Committee of Hunan Normal University (ISA-2017-058).
2.1 Animals and diets
A total of 60 castrated male pigs (Landrace × Yorkshire, initial average body weight (BW) 6.51 ± 0.64 kg, 21 days old) were selected, blocked by BW, and assigned to five dietary treatments with 12 replicate cages per treatment and one pig per metabolic cage. “Pre-care period” and “late-care period” diets were formulated as starch-soybean meal-based diets that met the nutrient requirements established by the NRC (16) for pigs weighing 7–11 kg and 11–25 kg, respectively.
High-amylose maize starch (High-Maize 1043, National Starch Industry, Shanghai, China) and waxy maize starch (food market, Hengyang, China) were blended to create diets with differing dietary amylose/amylopectin ratios (DAR) of 0.00, 0.20, 0.40, 0.60, or 0.80, respectively (Supplementary Table 1). According to the manufacturer’s instructions, the amylose and amylopectin contents were determined using their commercial assay kits (l-AMYL, Megazyme International Ireland Ltd., Wicklow, Ireland).
Pigs were fed and provided food ad libitum. Starting on day 15, the feed was gradually transitioned from the “pre-care period” diet to the “late-care period” diet over seven consecutive days, with ratios adjusted as follows: 80:20, 60:40, 50:50, 40:60, 30:70, 20:80, and 0:100. The experiment lasted 28 days.
On day 28, 12 h before slaughter, six pigs from each treatment group received intraperitoneal injections of 100 μg/kg BW lipopolysaccharides (LPS, from Escherichia coli O55:B5, Sigma Chemical Inc., St Louis, MO, USA, L2880), whereas another six pigs were administered an equivalent amount of sterile saline.
2.2 Sample collection
On day 15 before feed transition and on day 29, after LPS stress, blood samples were collected from the jugular vein and centrifuged at 3,000×g at 4°C for 10 min to separate serum. Piglets were euthanized via an intravenous injection of 40 mg/kg BW sodium pentobarbital solution into the jugular vein. The middle right lobe of the liver and chyme from the cecal and colon were collected using sterile scissors and tweezers and immediately stored at −80°C for further study.
2.3 Analysis of serum biochemical variables
Serum samples were thawed, and the concentration of total protein (TP), albumin (ALB), alanine transaminase (ALT), aspartate aminotransferase (AST), blood urea nitrogen (BUN), creatinine (CREA), glucose (GLU), triglyceride (TG), total cholesterol (CHOL), amylase (AMS), hepatic lipase (LIPC) was measured using commercial kits in accordance with the manufacturer’s instructions (Jiancheng Bioengineering Institute, Nanjing, China) at the TBA-120FR Automatic Biochemistry Radiometer (Hitachi Co., Tokyo, Japan). Serum immunoglobulin G and M (IgG, IgM) concentrations were determined using a commercial ELISA kit (Jiancheng Bioengineering Institute, Nanjing, China) according to the manufacturer’s instructions.
2.4 Analysis of AA contents in serum and liver
Samples were prepared for amino acid analysis as follows:
For serum, 600 μL of serum was mixed with an equivalent volume of 8% sulfosalicylic acid and incubated for 1 h at 4°C. Then, the mixture was then centrifuged at 10,000 rpm for 10 min at 4°C to collect supernatants.
For liver samples, approximately 0.1 g of ground, freeze-dried liver tissue was hydrolyzed in 10 mL of 6 mol/L hydrochloric acid solution at 110°C for 24 h. The hydrolyzed solution was diluted with distilled water to a final volume of 100 mL, and 1 mL of the supernatant was used for further analysis.
Amino acid concentrations were measured using an amino acid analyzer (L-8900, Hitachi, Japan) after both the serum supernatant and hepatic diluent solution were filtered through a 0.45-μm membrane.
2.5 Assay of hepatic antioxidant enzyme activities
Hepatic tissue samples were ground in liquid nitrogen, homogenized in saline, and centrifuged at 3,000×g 4°C for 10 min. The concentrations of malonaldehyde (MDA) and the activities of superoxide dismutase (SOD), including coper-zinc SOD (CuZn-SOD) and manganese SOD (Mn-SOD), glutathione peroxidase (GSH-PX/GPx), and total antioxidant capacity (T-AOC), were analyzed using commercial kits (Jiancheng Bioengineering Institute, Nanjing, China) according to the manufacturer’s instructions.
2.6 RNA extraction and real-time quantitative PCR
Total RNA was isolated from the liver using RNAiso Plus (TaKaRa), and then reverse transcription reactions were performed using an RT reagent kit (TaKaRa). All the procedures were carried out as described by the manufacturer’s protocol. The quantity and quality of RNA were determined using the NanoDrop ND-2000 spectrophotometer system (ThermoFisher Scientific). Primers were designed to assay genes related to antioxidant function (Supplementary Table 2). The β-actin was used as a reference gene. Real-time RT-PCR for target genes was performed on the MyIQ instrument (Bio-Rad, Hercules, California) using SYBR Green quantitative PCR mix (TaKaRa).
2.7 Analysis of mineral element contents in the liver
Macro and microelements (Ca, S, Mg, Cu, Fe, Mn, Zn) were analyzed using an analytical method described earlier (17). Briefly, liver samples (5.00 ± 0.20 g) were weighed in triplicate and digested using a mixture of acid (25 mL of HNO3: HClO4 in a volume ratio of 4:1) following heating (80°C for 60 min; 120°C for 30 min; and 180°C for 30 min). Samples were dried at 260°C and redissolved in 5 mL of 1% HNO3. The solution was then transferred to a 25-mL volumetric flask and filled with 1% HNO3. Finally, samples’ target elements (Cu, Fe, Mn, and Zn) were determined on ICP-OES (Varian 720ES Agilent, Santa Clara, CA, USA) for confirmation with standard references.
2.8 Analysis of indole and skatole contents in chyme
Indole and skatole (3-methylindole) of luminal contents in the cecal and colon were analyzed as described previously on high-performance liquid chromatography (HPLC, Agilent 1260, Agilent Technologies Inc., USA) (18). Briefly, luminal contents from the cecum and colon were weighed (1.000 g) and extracted overnight using 8 mL of 0.4 mol/L perchloric acid. Then, the suspension was obtained by centrifugation at 8,000 r/min for 10 min at 4°C. Subsequently, 1 mL of the supernatant was mixed with 1 mL of sodium hydroxide (2 mol/L, pH 10.6) saturated sodium bicarbonate buffer solution. Then, 1 mL of dansulfonyl chloride (10 mg/mL acetone solution) was added to the mixture. The solution was incubated in a water bath at 40°C in a dark environment for 30 min, followed by the addition of 1 mL of 5% ammonia water. Subsequently, 3 mL of anhydrous ether was used to extract the solution. The anhydrous ether layer was dried, dissolved in 1 mL of methanol, filtered through a 0.22-μm syringe filter membrane, and analyzed using the appropriate analytical instrument.
2.9 Statistics analysis
Gene expression data from replicate measurements within the same RNA extraction were averaged and analyzed using the Livak (19) method. One-way ANOVA followed by Duncan’s multiple range test was conducted using SAS 8.0 to evaluate significant differences in serum indices based on DAR on Day 15. Data from D29 were analyzed using two-way ANOVA, with “stress” (saline or LPS), dietary DAR, and their interaction as factors.
Duncan’s multiple range test was used to compare differences among the various DAR groups. SAS’s PROC REG procedure (with stepwise selection at a p-value of <0.15) was used to analyze potential linear or quadratic regressions between dietary DAR and serum or hepatic indices in non-stressed piglets. Data were expressed as least squares mean (Lsmeans) ± SEM. A p-value of <0.05 was considered statistically significant, while 0.05 < p < 0.10 indicated a statistically significant trend.
3 Results
3.1 Serum biochemical variables at D15
DAR did not affect serum ALT, AST, LIPC, TP, ALB, UREA, CREA, or TC on D15 (Table 1). However, piglets in the DAR 0.00 and 0.60 groups exhibited higher serum AMS activity than those in the DAR 0.40 group (p < 0.05). Serum GLU levels were significantly higher in the DAR 0.80 group than in the other groups (p < 0.05). Serum TG levels were higher in the DAR 0.20 group compared to DAR 0.00 and 0.40 (p < 0.05). Furthermore, serum GLU levels increased quadratically with increasing DAR (p < 0.05).
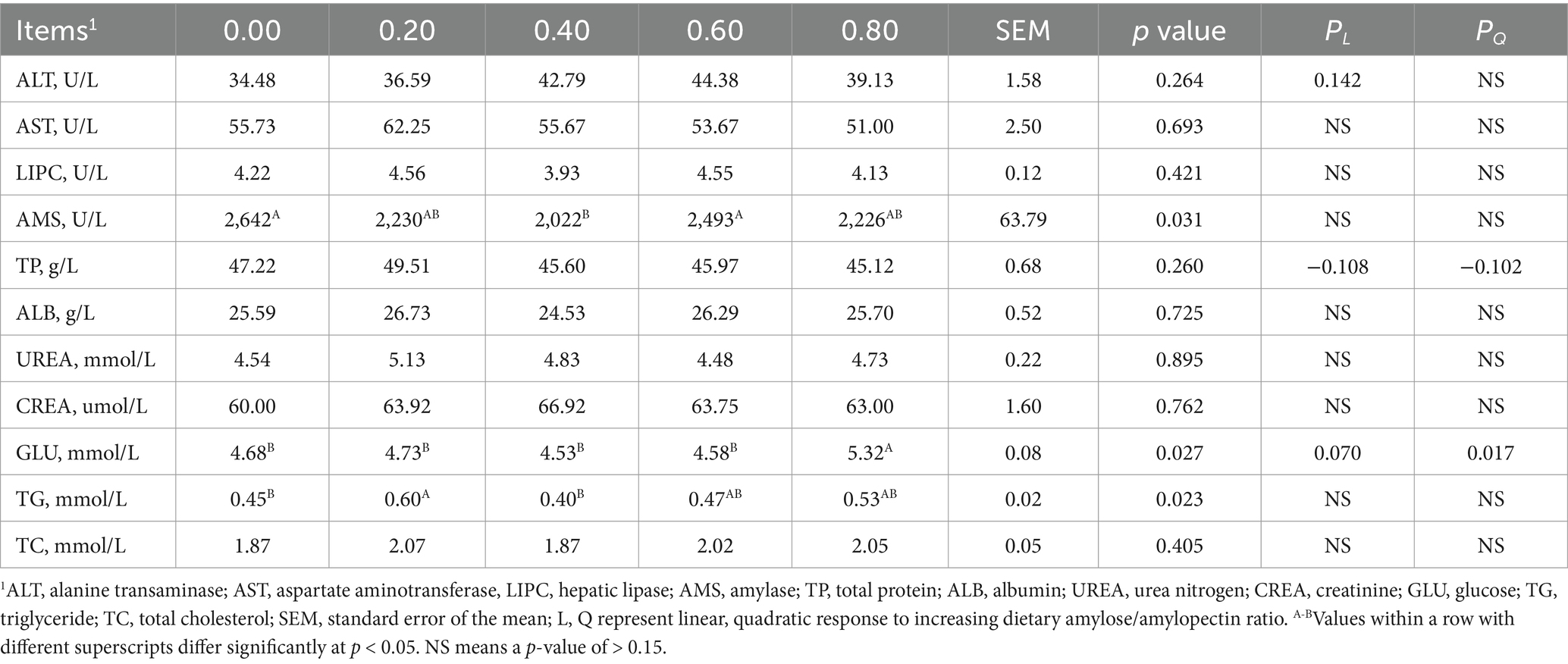
Table 1. Effects of dietary amylose/amylopectin ratio on serum biochemical index on day 15 in weaned piglets.
3.2 Serum-free amino acids at D15
Serum essential amino acids on D15 were not significantly affected by DAR (p > 0.05), except for serum Arg concentrations, which were higher in the DAR 0.40 and 0.60 groups compared to DAR 0.00 (p < 0.05) (Table 2). Serum non-essential amino acids, such as tyrosine (Tyr), glutamate (Glu), glycine (Gly), serine (Ser), cysteine (Cys), taurine (Tau), urea, hydroxylysine (Hylys), ornithine (Orn), β-alanine (β-Ala), β-aminoisobutyric acid (β-AiBA), 1-methylhistidine (1Mehis), 3-methylhistidine (3Mehis), and hydroxy-L-proline (Hypro), were not significantly affected by DAR (p > 0.05).
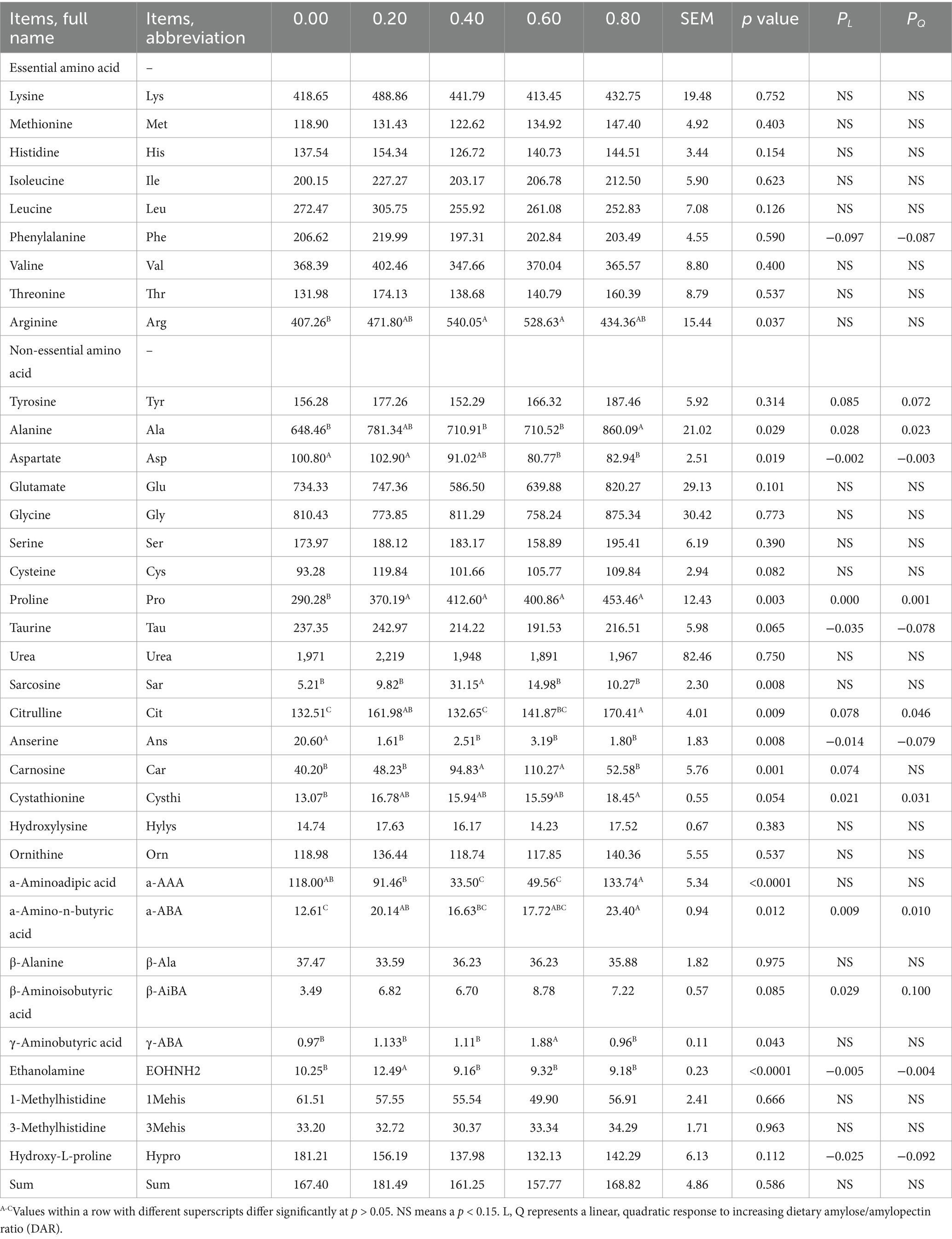
Table 2. Effects of dietary amylose/amylopectin ratio on serum free amino acid concentration on day 15 in weaned piglets, ng/20 uL.
Piglets in the DAR 0.80 group exhibited significantly higher serum alanine (Ala) and citrulline (Cit) concentrations compared to those in the DAR 0.00, 0.40, and 0.60 groups (p < 0.05), but these levels were not significantly different from those in the DAR 0.20 group (p > 0.05). Serum aspartate (Asp) concentrations were significantly lower in the DAR 0.80 and 0.60 groups compared to DAR 0.00 and 0.20 (p < 0.05). Piglets in the DAR 0.40 and 0.60 groups exhibited significantly higher serum carnosine (Car) concentrations than those in other groups (p < 0.05).
Serum α-amino-butyric acid (α-ABA) concentrations were significantly higher in the DAR 0.80 group compared to DAR 0.00 and 0.40 (p < 0.05). Serum proline (Pro) levels were significantly lower in the DAR 0.00 group than in all other groups (p < 0.05). Serum ethanolamine (EOHNH2) levels were significantly higher in the DAR 0.20 group compared to all other groups (p < 0.05). Piglets in the DAR 0.40 group had the highest serum sarcosine (Sar) concentrations among all groups (p < 0.05), while those in the DAR 0.60 group had the highest serum γ-aminobutyric acid (γ-ABA) concentrations (p < 0.05). Serum α-aminoadipic acid (a-AAA) concentrations in the DAR 0.80 group were significantly higher than in DAR 0.20, 0.40, and 0.60 (p < 0.05).
REG analysis showed that serum Phe tended to decrease linearly with increasing DAR (p = 0.097), while serum Tyr (p = 0.085) and Car (p = 0.074) tended to increase linearly with increasing DAR. Serum Ala, Pro, Cysthi, α-ABA, β-AiBA increased linearly with increasing DAR (p < 0.05), whereas serum Asp, Tau, Ans, EOHNH2, and Hypro decreased linearly with increasing DAR (p < 0.05).
3.3 Serum biochemical variables at D29
Different DAR diets showed no significant differences in serum ALT, AST, AMS, TP, ALB, UREA, CREA, GLU, TG, and IgM levels at D29 (Table 3, p > 0.05). Serum LIPC was significantly higher in the DAR 0.60 group compared to the DAR 0.00 and 0.20 groups (p < 0.05). Piglets in the DAR 0.60 and 0.80 groups exhibited higher serum TC levels than those in the DAR 0.40 group (p < 0.05). Serum IgG concentration was significantly higher in the DAR 0.80 group than in the other groups (p < 0.05). Serum AST decreased progressively with increasing DAR (p < 0.05). Serum TP decreased, whereas IgG increased linearly with increasing DAR (p < 0.05). Serum TC tended to increase linearly with increasing DAR (p = 0.066).
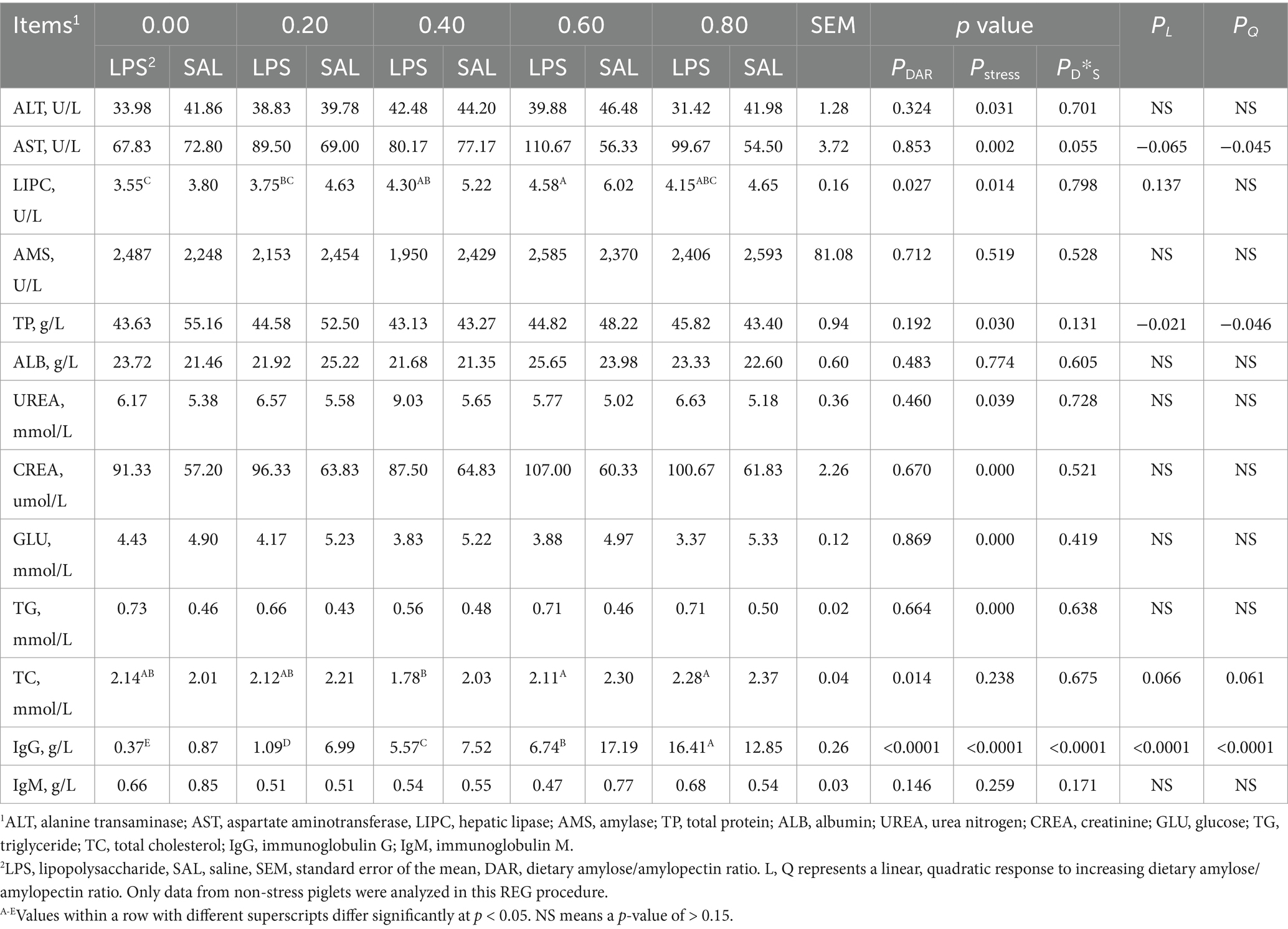
Table 3. Effects of dietary amylose/amylopectin ratio on serum biochemical index on day 29 in weaned piglets under LPS stress.
As shown in Table 3, LPS stimulation significantly increased serum AST, UREA, CREA, and TG levels but decreased serum ALT, LIPC, TP, and GLU concentrations compared to controls (p < 0.05). Serum AMS, ALB, TC, and IgM concentrations were not significantly affected by LPS administration (p > 0.05).
A significant interaction between LPS and DAR was found for serum IgG concentrations (p < 0.01). In LPS-exposed animals, serum IgG concentration significantly increased in the DAR 0.80 group, whereas it decreased in the other groups after LPS administration. Additionally, a two-way interaction between LPS stress and DAR was observed for serum AST concentrations (p = 0.055). Serum AST levels tended to decrease in the DAR 0.00 group after the LPS challenge compared to the control group. In contrast, serum AST levels increased following the LPS challenge in the other DAR groups after the LPS challenge in other groups.
3.4 Serum-free amino acids at D29
Serum essential amino acid concentrations were not significantly affected by DAR (p > 0.05), except for serum Arg, which was higher in the DAR 0.80 group compared to the DAR 0.00, 0.20, and 0.40 groups (Table 4, p < 0.05). Similarly, serum non-essential amino acids were not affected by DAR (p > 0.05), except for Pro, Sar, Cit, Ans, Hylys, β-AiBA, and Hypro (p < 0.05). Serum Pro was significantly higher in the DAR 0.60 group than in the DAR 0.80, 0.40, and 0.00 groups (p < 0.05). Serum concentrations of Sar, Cit, Ans, and Hylys in the DAR 0.80 group were significantly higher than in the DAR 0.00 group (p < 0.05).
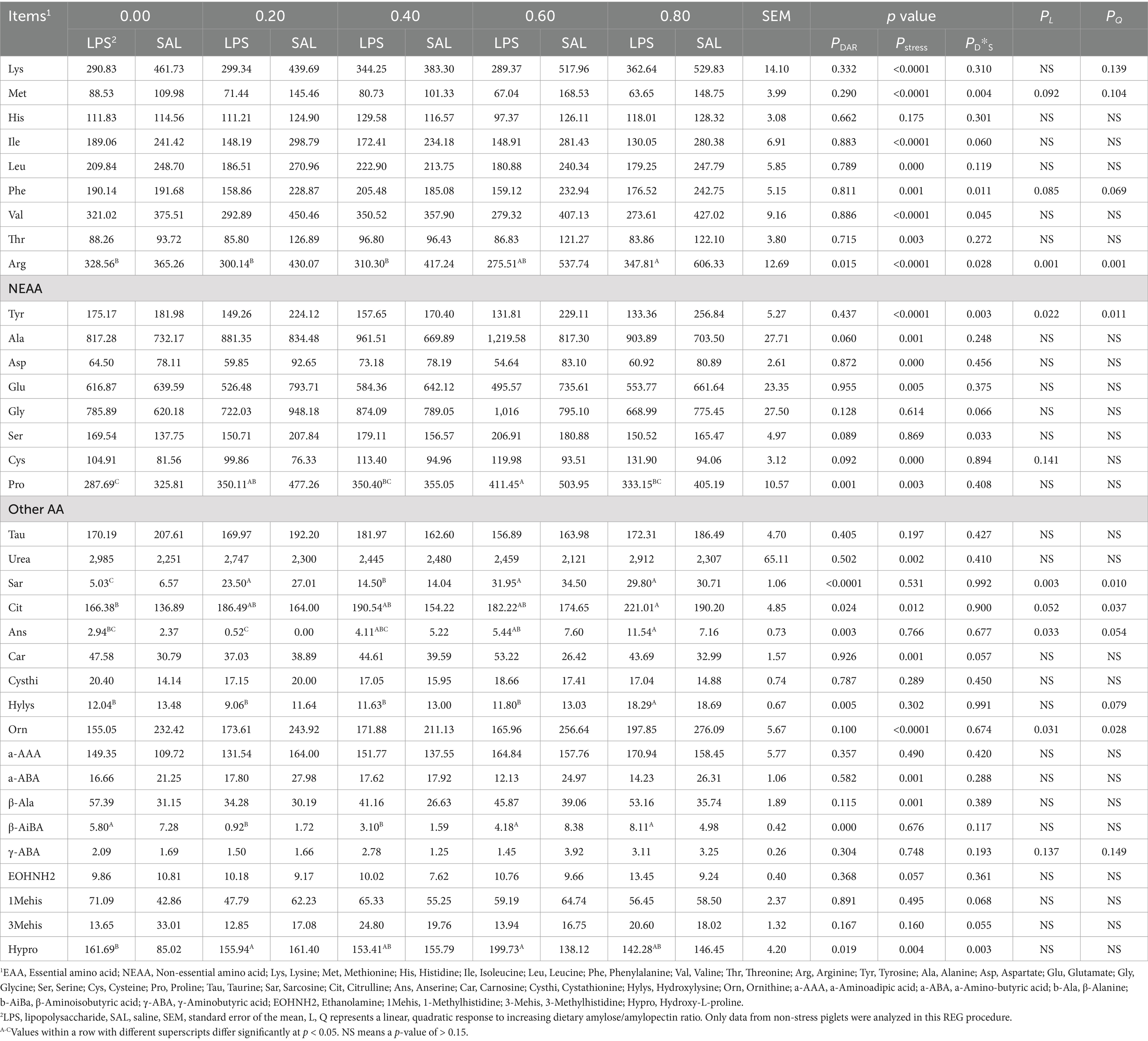
Table 4. Effects of dietary amylose/amylopectin ratio on serum-free AA concentration on day 29 in weaned piglets under LPS stress, ng/20 uL.
Pigs from the DAR 0.00, 0.60, and 0.80 groups exhibited significantly higher serum β-AiBA levels than those from the DAR 0.20 and 0.40 groups (p < 0.05). Serum Hypro concentrations were significantly higher in the DAR 0.20 and 0.60 groups than in the DAR 0.00 group (p < 0.05). Serum essential amino acid concentrations decreased after LPS stress (p < 0.05), except for His, which was not affected by LPS stress (p > 0.05).
Serum concentrations of Tyr, Ala, Asp, Glu, Cys, Pro, urea, Cit, Car, Orn, α-ABA, β-Ala, and Hypro were significantly affected by LPS stress (p < 0.05).
A significant interaction effect between DAR and LPS was observed for serum Met, Phe, Val, Arg, Tyr, Ser, and Hypro (p < 0.05). Serum Phe levels increased after LPS stress in the DAR 0.40 group but decreased in the other groups (p < 0.05). Serum Met and Val concentrations remained stable after LPS stress in the DAR 0.00 and 0.40 groups but decreased in the other groups (p < 0.05). Serum Arg and Tyr levels were stable after LPS stress in the DAR 0.00 group but decreased in the other groups (p < 0.05). Serum Ser concentrations decreased after LPS stress in the DAR 0.20 and 0.80 groups but increased in the other groups (p < 0.05). Serum Hypro levels increased after LPS stress in the DAR 0.00 and 0.60 groups but remained stable in the other groups (p < 0.05).
Serum Arg (p < 0.01), Tyr (p < 0.05), Sar (p < 0.01), Ans (p < 0.05), and Orn (p < 0.05) increased linearly with increasing DAR, while serum Cit exhibited a progressive increase with increasing DAR (p < 0.05).
3.5 Amino acid contents in liver
There was no significant effect of DAR on all tested hepatic amino acid concentrations (p > 0.05), except for hepatic Cys, which was significantly lower in the DAR 0.80 group compared to the DAR 0.00 and 0.20 groups (p < 0.05) (Table 5). The LPS challenge resulted in increased concentrations of all tested amino acid concentrations in the liver compared to the control group (p < 0.05).
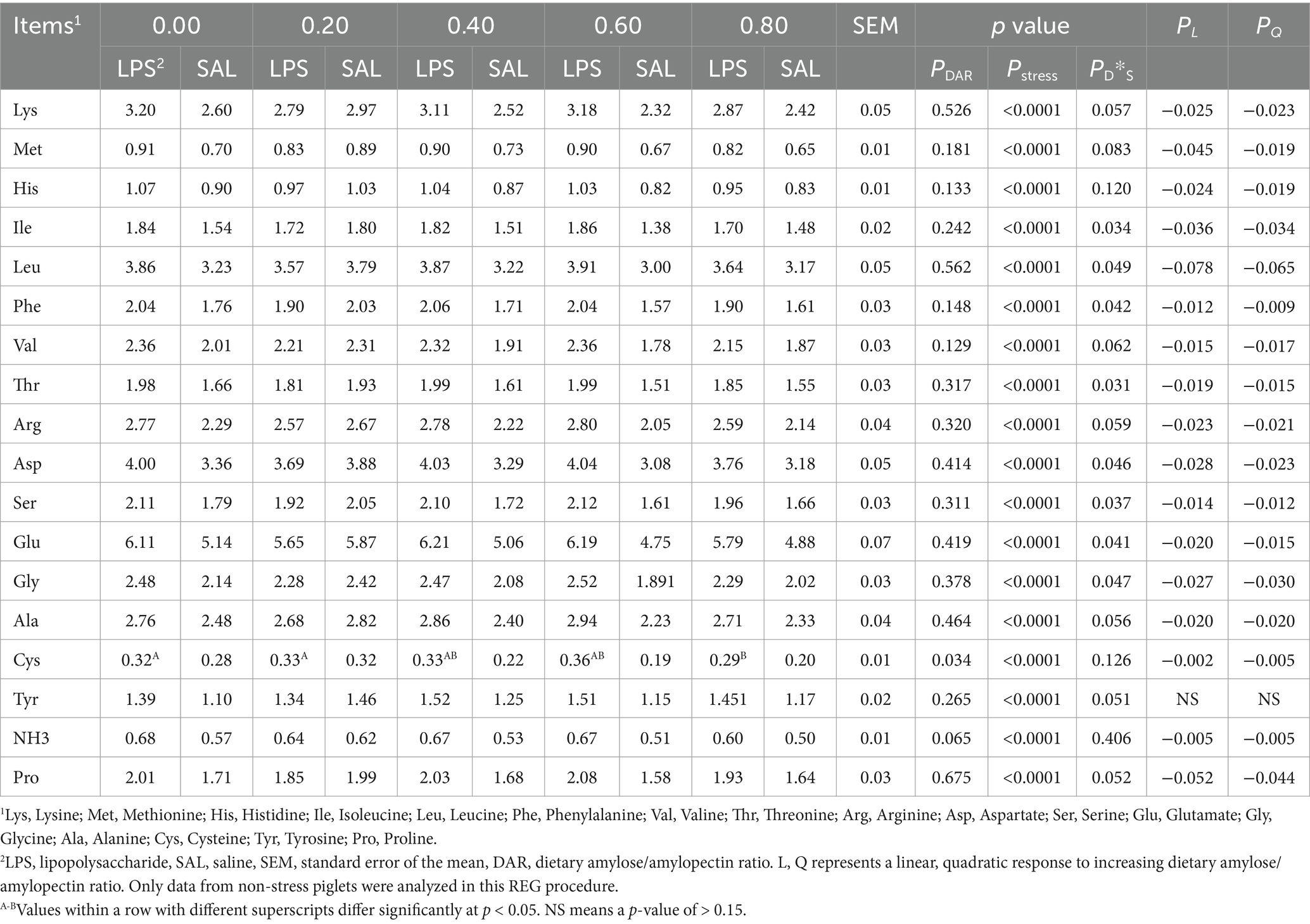
Table 5. Effects of dietary amylose/amylopectin ratio on amino acid composition in the liver of weaned piglets under LPS stress, μg/100 mg.
A significant interaction between LPS and DAR was observed for hepatic Ile, Leu, Phe, Thr, Asp, Ser, Glu, and Gly concentrations (p < 0.05). In animals fed the DAP 0.20, these amino acid concentrations decreased 12 h after LPS exposure. However, their concentrations increased in animals from other dietary groups following LPS stress.
3.6 Antioxidant function of liver
The concentration of hepatic MDA was significantly higher in the DAR 0.20 group compared to the DAR 0.00, 0.40, and 0.80 groups (p < 0.05) (Table 6). The activity of T-AOC was not affected by DAR or LPS stress (p > 0.05). The activity of GSH-PX in the DAR 0.20 group was higher than in the DAR 0.40, 0.60, and 0.80 groups (p < 0.05). It increased after LPS stress (p < 0.05). T-SOD activity in DAR 0.00 was higher than that in DAR 0.20, 0.40, and 0.80 (p < 0.05). After LPS stress, hepatic T-SOD activity decreased in the DAR 0.40 group but increased in the DAR 0.00 and 0.80 groups (p < 0.05). The activity of Cu-SOD was lower in the DAR 0.20 group compared to the other groups (p < 0.05) and increased linearly with rising DAR (p < 0.05).
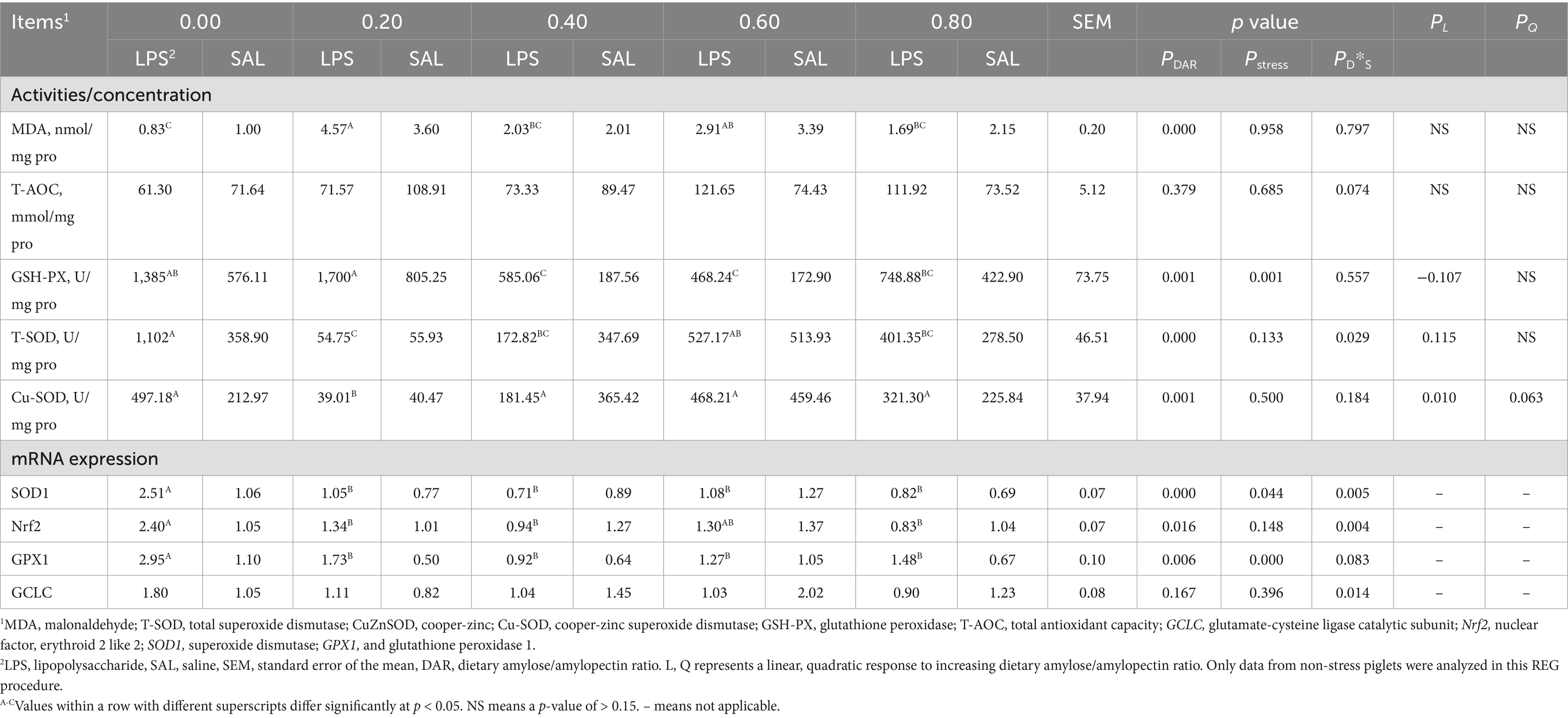
Table 6. Effects of dietary amylose/amylopectin ratio on antioxidant function in the liver of weaned piglets under LPS stress.1
Gene expression related to antioxidant function, such as SOD1, nuclear translocation factors (Nrf2), and GPX1 mRNA expressions, was affected by DAR, which was higher in DAR 0.00 than in other groups (p < 0.05). LPS stress caused an increase in the abundance of gene GPX1 (p < 0.05). mRNA expression of SOD1 decreased in DAR 0.40 and 0.60 after LPS stress but increased in other groups (p < 0.05). Nrf2 mRNA expression decreased in the DAR 0.40, 0.60, and 0.80 groups after LPS stress but increased in the DAR 0.00 and 0.20 groups (p < 0.05). Gene of glutamate-cysteine ligase catalytic subunit (GCLC) expression in the DAR 0.40, 0.60, and 0.80 groups decreased after LPS stress but increased in the DAR 0.00 and 0.20 groups (p < 0.05).
3.7 Mineral element contents in liver
Mineral element concentrations such as Ca, Mg, and S were not affected by DAR (p > 0.05) (Table 7). The DAR 0.80 had higher Cu, Fe, Mn, and Zn concentrations than DAR 0.00, 0.20, and 0.40 (p < 0.05). The LPS stress caused an increase in Mg and S concentrations (p < 0.05). Cu concentration (p < 0.01) increased after LPS stress in DAR 0.00 and 0.80 but decreased in other groups after LPS stress. Hepatic Cu, Fe, Mn, and Zn concentrations increased linearly with increasing DAR (p < 0.01).
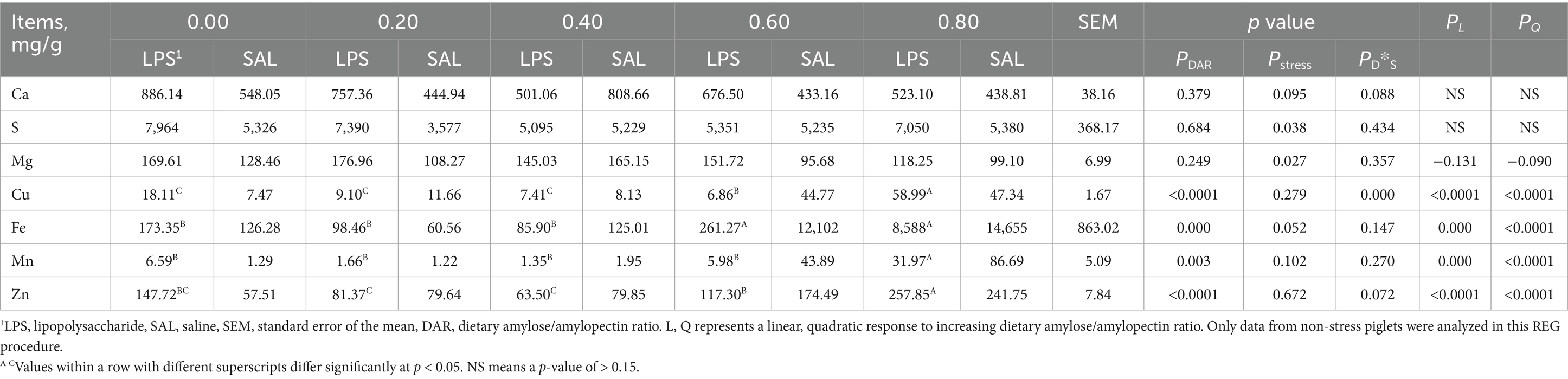
Table 7. Effects of dietary amylose/amylopectin ratio on mineral element concentrations in the liver of weaned piglets under LPS stress.1
3.8 Indole and skatole contents in chyme
Cecal indole concentration in the DAR 0.00 group was higher than that in the DAR 0.80 group (p < 0.05). It was not affected by LPS stress (p > 0.05) (Table 8). Cecal skatole and colonic indole were not affected by DAR or LPS stress (p > 0.05). Piglets that had DAR 0.00 had lower colonic skatole than those from the DAR 0.40, 0.60, and 0.80 groups (p < 0.05). Colonic skatole concentration increased after LPS stress in DAR 0.40 and 0.80 but decreased in other groups (p < 0.05). No variables were selected in the REG procedure at a p-value of <0.15.
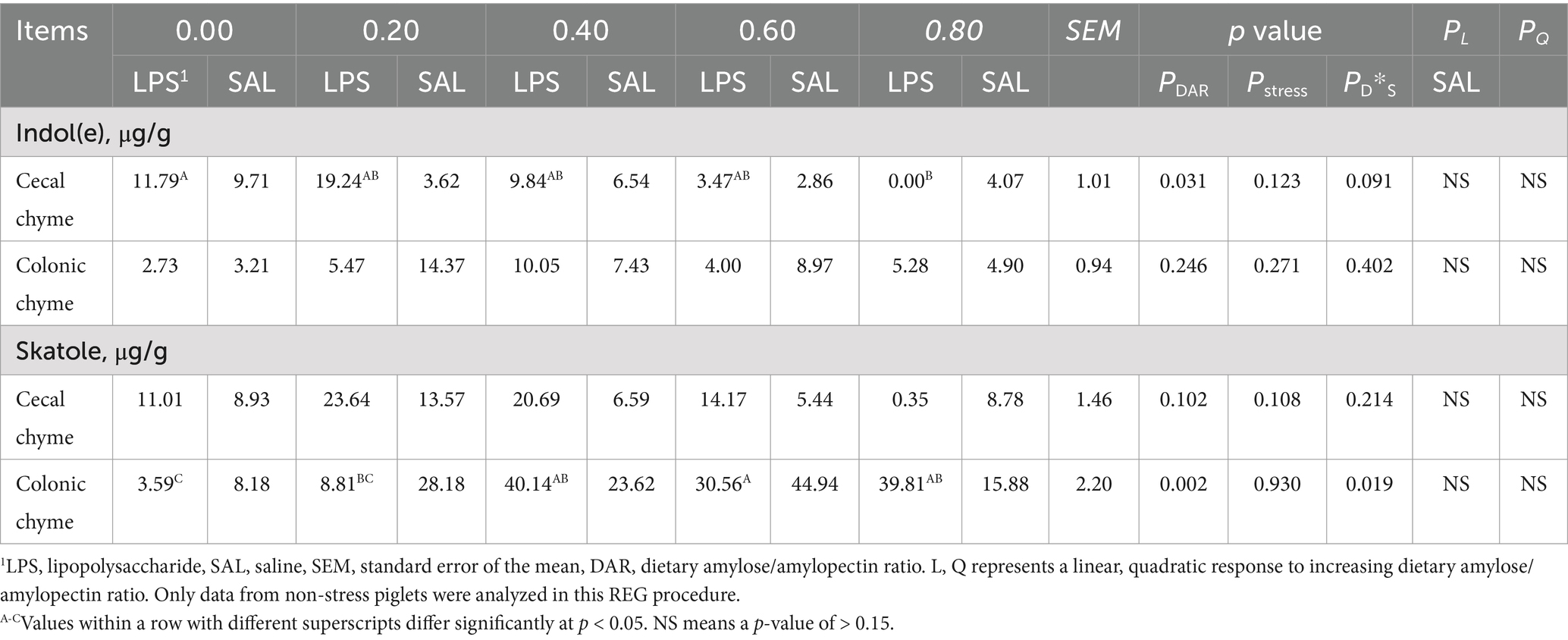
Table 8. Effects of dietary amylose/amylopectin ratio on indole and skatole concentrations in cecal and colonic chyme of weaned piglets under LPS stress.1
4 Discussion
When weaned piglets experienced LPS stress, serum AST activity and urea nitrogen levels increased, while serum glucose and glutamate concentrations decreased. In contrast, hepatic concentrations of Cys, Glu, and Gly increased. Serum AST activity is a key biochemical marker of liver health and function. The observed elevation of serum AST levels following LPS stress (20) indicates hepatic injury occurred under these situations.
In response to LPS stress, piglets may redirect AA from protein retention toward AA utilization in the immune response. The increase in serum urea nitrogen in this study can be associated with elevated AA catabolism, particularly in amino acids in excess. Specific AAs are utilized in the synthesis of immune system metabolites, such as immunoglobulins and glutathione, during LPS stress (6). This was confirmed by the elevated GSH-PX activity, upregulated GPX1 gene expression in the liver, and increased plasma Cys flux in pigs challenged with LPS in this study.
Compared to the diet 0.00, piglets consuming a diet high in amylose (0.80) exhibited higher serum Arg, Cit, and glucose concentrations. Both hepatic T-SOD activity and SOD1 mRNA expression were also elevated in the 0.80 group after LPS stress. Additionally, serum IgG levels increased in piglets on a diet of 0.80 after LPS stress. Copper, zinc-superoxide dismutase (SOD1), a major intracellular antioxidant enzyme in mammals, plays a crucial role in mitigating LPS-induced hepatic protein nitration (21). The fermentation of raw potato starch (RS2) in the cecum enhances the intestinal absorption of minerals such as Ca, Mg, Fe, Zn, and Cu (22). Given the higher amylose content in diet 0.80, piglets from this group had significantly higher hepatic Cu concentrations after LPS stress compared to other groups. While Prates et al. (7) reported that plasma IgG and IgM concentrations in weaned piglets were reduced 3 days after an LPS challenge, the increased IgG observed in the 0.80 group in this study suggests enhanced protection against oxidative stress and inflammation. Indoxyl sulfate and p-cresol sulfate, major microbial-derived pro-inflammatory and pro-oxidant uremic toxins, decrease under lower pH conditions (23). The lower pH associated with the diet 0.80 (24) likely contributed to the observed decrease in cecal indoles in this study. However, colonic skatole concentrations increased in the diet 0.80 group after LPS stress. A diet high in amylose may lead to the reorganization of the intestinal mucosa, thereby increasing gut cell debris availability for microbial skatole production from tryptophan (25). We speculate that piglets consuming the diet 0.80 were better protected against LPS stress due to enhanced antioxidant capacity and immune response.
Although average feed intake was the same across all groups (24), serum-free AAs such as Arg, Ala, Asp, Pro, Cit, Cysthi, a-ABA, and EOHNH2 were either higher or at least not lower in DAR 0.20 compared to other groups at D15. However, by day 29, only serum Pro, Sar, Cit, and Hypro concentrations remained elevated in DAR 0.20 relative to the other groups.
After 24 h of incubation with cecal and colonic digesta, the production of ammonia-nitrogen and branched-chain fatty acids (BCFAs), markers of protein fermentation, decreased as corn-resistant starch levels increased (26). In the DAR 0.20 group, protein fermentation in the large intestine was evident after feed transition, as indicated by significant increases in isobutyrate and isovalerate concentrations (24). Protein fermentation products have been associated with toxic and pro-inflammatory effects on the intestinal epithelium (27), potentially explaining the highest hepatic MDA concentration observed in the diet 0.20. Normally, liver protein synthesis increases when animals undergo immune challenges. However, the higher protein fermentation in DAR 0.20 likely contributed to reductions in hepatic Ile and Leu levels after LPS stress, as BCFAs such as iso-butyrate, 2-methyl-butyrate, and iso-valerate are products of valine, isoleucine, and leucine deamination. Notably, serum Ser concentrations decreased after LPS stress in the DAR 0.20 and 0.80 groups while increasing in the other groups. Serine plays a critical role in metabolic networks interlinking the folate and methionine cycles, supporting cell proliferation (28).
It also serves as a precursor for Gly and Cys, which contribute to the synthesis of the antioxidant glutathione (GSH). Conversely, hepatic amino acids such as Phe, Thr, Asp, Ser, Glu, and Gly were likely mobilized to counteract oxidative stress, as indicated by elevated hepatic MDA levels. Increasing the availability of amino acids entering the portal vein has been suggested to enhance tissue protein synthesis (29). Higher hepatic Cys levels in the DAR 0.20 group suggest active Glu synthesis in this group. Additionally, DAR 0.20 exhibited the highest GSH-PX activity in the liver among all groups, further underscoring its role in combating oxidative stress.
5 Conclusion
In conclusion, feeding weaned piglets a diet high in amylose (DAR 0.80) enhances their ability to cope with LPS stress by mobilizing amino acids for IgG synthesis and improving antioxidant function. This finding provides a new strategy to protect piglets from LPS-induced stress by regulating dietary starch structure.
Data availability statement
The raw data supporting the conclusions of this article will be made available by the authors without undue reservation.
Ethics statement
The animal studies were approved by Animal Care and Use Committee of Hunan Normal University (ISA-2017-058). The studies were conducted in accordance with the local legislation and institutional requirements. Written informed consent was obtained from the owners for the participation of their animals in this study.
Author contributions
CY: Conceptualization, Data curation, Formal analysis, Funding acquisition, Investigation, Writing – original draft, Writing – review & editing. XT: Formal analysis, Investigation, Methodology, Project administration, Writing – review & editing. MW: Investigation, Methodology, Writing – review & editing. HaY: Investigation, Methodology, Project administration, Writing – review & editing. HuY: Conceptualization, Investigation, Writing – review & editing. YW: Investigation, Methodology, Writing – review & editing. YY: Conceptualization, Investigation, Supervision, Writing – review & editing.
Funding
The author(s) declare that financial support was received for the research, authorship, and/or publication of this article. This research was supported by key projects of Hunan provincial department of education (22A0504); Research foundation of the education of Hunan province (21C1122); China postdoctoral science foundation funded project (2016M600630).
Acknowledgments
The authors gratefully thank the pig farm of the Institute of Subtropical Agriculture, Chinese Academy of Science, for using the experimental farm, the provision of experimental animals, and their assistance in animal handling throughout the experimental period.
Conflict of interest
The authors declare that the research was conducted in the absence of any commercial or financial relationships that could be construed as a potential conflict of interest.
The reviewer XL declared a shared affiliation with the author YY to the handling editor at the time of review.
Publisher’s note
All claims expressed in this article are solely those of the authors and do not necessarily represent those of their affiliated organizations, or those of the publisher, the editors and the reviewers. Any product that may be evaluated in this article, or claim that may be made by its manufacturer, is not guaranteed or endorsed by the publisher.
Supplementary material
The Supplementary material for this article can be found online at: https://www.frontiersin.org/articles/10.3389/fnut.2024.1435051/full#supplementary-material
References
1. Qiao, Y, Bai, XF, and Du, YG. Chitosan oligosaccharides protect mice from LPS challenge by attenuation of inflammation and oxidative stress. Int Immunopharmacol. (2011) 11:121–7. doi: 10.1016/j.intimp.2010.10.016
2. Xiao, D, Wang, Y, Liu, G, He, J, Qiu, W, Hu, X, et al. Effects of chitosan on intestinal inflammation in weaned pigs challenged by enterotoxigenic Escherichia coli. PLoS One, 8(2014-8-4). (2014) 9:e104192. doi: 10.1371/journal.pone.0104192
3. Yuan, S, Chen, D, Zhang, K, and Yu, B. Effects of oxidative stress on growth performance, nutrient digestibilities and activities of antioxidative enzymes of weanling pigs. Asian Australas J Anim Sci. (2007) 20:1600–5. doi: 10.5713/ajas.2007.1600
4. Xia, B, Meng, Q, Feng, X, Tang, X, and Jia, Z. Probing the molecular regulation of lipopolysaccharide stress in piglet liver by comparative proteomics analysis. Electrophoresis. (2018) 39:2321–31. doi: 10.1002/elps.201700467
5. Gu, K, Wang, F, Sun, W, Liu, G, Jia, G, Zhao, H, et al. Tryptophan alleviates lipopolysaccharide-induced liver injury and inflammation by modulating piglets' necroptosis and pyroptosis signaling pathways. Anim Biotechnol. (2023) 34:4069–80. doi: 10.1080/10495398.2023.2255064
6. McGilvray, WD, Klein, D, Wooten, H, Dawson, JA, Hewitt, D, Rakhshandeh, AR, et al. Immune system stimulation induced by Escherichia coli lipopolysaccharide alters plasma free amino acid flux and dietary nitrogen utilization in growing pigs. J Anim Sci. (2019) 97:315–26. doi: 10.1093/jas/sky401
7. Prates, JAM, Freire, JPB, de Almeida, AM, Martins, C, Ribeiro, DM, Osório, H, et al. Influence of dietary supplementation with an amino acid mixture on inflammatory markers, immune status and serum proteome in LPS-challenged weaned piglets. Animals. (2021) 11:1143. doi: 10.3390/ani11041143
8. Vonderohe, C, Stoll, B, Didelija, I, Nguyen, T, Mohammad, M, Jones-Hall, Y, et al. Citrulline and ADI-PEG20 reduce inflammation in a juvenile porcine model of acute endotoxemia. Front Immunol. (2024) 15:1400574. doi: 10.3389/fimmu.2024.1400574
9. Luo, ZG, and Shi, YC. Preparation of acetylated waxy, normal, and high-amylose maize starches with intermediate degrees of substitution in aqueous solution and their properties. J Agric Food Chem. (2012) 60:9468–75. doi: 10.1021/jf301178c
10. Lopez-Rubio, A, Flanagan, BM, Gilbert, EP, and Gidley, MJ. A novel approach for calculating starch crystallinity and its correlation with double helix content: a combined XRD and NMR study. Biopolymers. (2008) 89:761–8. doi: 10.1002/bip.21005
11. Xie, C, Li, Y, Li, J, Zhang, L, Zhou, G, and Gao, F. Dietary starch types affect liver nutrient metabolism of finishing pigs. Br J Nutr. (2017) 118:353–9. doi: 10.1017/s0007114517002252
12. Zeng, H, Huang, C, Lin, S, Zheng, M, Chen, C, Zheng, B, et al. Lotus seed-resistant starch regulates gut microbiota and increases short-chain fatty acid production and mineral absorption in mice. J Agric Food Chem. (2017) 65:9217–25. doi: 10.1021/acs.jafc.7b02860
13. Yang, C, He, J, Yu, B, Yu, J, Mao, XB, Chen, DW, et al. The effect of dietary amylose/amylopectin ratio on serum and hepatic lipid content and its molecular mechanisms in growing-finishing pigs. J Anim Physiol Anim Nutr. (2018) 102:1657–65. doi: 10.1111/jpn.12884
14. Gao, K, Pi, Y, Mu, CL, Farzi, A, Liu, Z, and Zhu, WY. Increasing carbohydrate availability in the hindgut promotes hypothalamic neurotransmitter synthesis: aromatic amino acids linking the microbiota-brain axis. J Neurochem. (2019) 149:641–59. doi: 10.1111/jnc.14709
15. Vaziri, ND, Liu, SM, Lau, WL, Khazaeli, M, Nazertehrani, S, Farzaneh, SH, et al. High amylose resistant starch diet ameliorates oxidative stress, inflammation, and progression of chronic kidney disease. PLoS One. (2014) 9:e114881. doi: 10.1371/journal.pone.0114881
17. Zhang, Y, Wan, D, Zhou, X, Long, C, Wu, X, Li, L, et al. Diurnal variations in iron concentrations and expression of genes involved in iron absorption and metabolism in pigs. Biochem Biophys Res Commun. (2017) 490:1210–4. doi: 10.1016/j.bbrc.2017.06.187
18. Xu, L, Geng, M, Zhang, L, Yuan, H, Kong, X, and Wang, J. Determination of biomines in colonic contents of piglets by pre-column column derivatization RP-HPLC. Acta Nutr Sin. (2014) 36:78–83.
19. Livak, KJ, and Schmittgen, TD. Analysis of relative gene expression data using real-time quantitative PCR and the 2(-Delta Delta C (T)) method. Methods (San Diego, Calif). (2001) 25:402–8. doi: 10.1006/meth.2001.1262
20. Jing, M, Munyaka, P, Tactacan, G, Rodriguez-Lecompte, J, O, K, and House, J. Performance, serum biochemical responses, and gene expression of intestinal folate transporters of young and older laying hens in response to dietary folic acid supplementation and challenge with Escherichia coli lipopolysaccharide. Poult Sci. (2014) 93:122–31. doi: 10.3382/ps.2013-03384
21. Zhu, JH, Zhang, X, Roneker, CA, Mcclung, JP, Zhang, S, Thannhauser, TW, et al. Role of copper, zinc-superoxide dismutase in catalyzing nitrotyrosine formation in murine liver. Free Radic Biol Med. (2008) 45:611–8. doi: 10.1016/j.freeradbiomed.2008.05.018
22. Lopez, H, Levrat-Verny, M, Coudray, C, Besson, C, Krespine, V, Messager, V, et al. Class 2 resistant starches lower plasma and liver lipids and improve mineral retention in rats. J Nutr. (2001) 131:1283–9. doi: 10.1093/jn/131.4.1283
23. Smith, EA, and Macfarlane, G. Formation of phenolic and indolic compounds by anaerobic bacteria in the human large intestine. Microb Ecol. (1997) 33:180–8. doi: 10.1007/s002489900020
24. Yang, C, Wang, M, Tang, X, Yang, H, Li, F, Wang, Y, et al. Effect of dietary amylose/amylopectin ratio on intestinal health and cecal microbes' profiles of weaned pigs undergoing feed transition or challenged with Escherichia coli lipopolysaccharide. Front Microbiol. (2021) 12:693839. doi: 10.3389/fmicb.2021.693839
25. Wesoly, R, and Weiler, U. Nutritional influences on skatole formation and skatole metabolism in the pig. Animals. (2012) 2:221–42. doi: 10.3390/ani2020221
26. He, X, Sun, W, Ge, T, Mu, C, and Zhu, W. An increase in corn-resistant starch decreases protein fermentation and modulates gut microbiota during the in vitro cultivation of large intestinal inocula in pigs. Anim Nutr. (2017) 3:219–24. doi: 10.1016/j.aninu.2017.06.004
27. Blaut, M, and Clavel, T. Metabolic diversity of the intestinal microbiota: implications for health and disease. J Nutr. (2007) 137:751S–5S. doi: 10.1093/jn/137.3.751S
28. Ducker, G, and Rabinowitz, J. One-carbon metabolism in health and disease. Cell Metab. (2016) 25:27–42. doi: 10.1016/j.cmet.2016.08.009
Keywords: dietary amylose/amylopectin ratio, LPS stress, amino acid, antioxidant activity, weaned piglet
Citation: Yang C, Tang X, Wang M, Yang H, Yang H, Wang Y and Yin Y (2025) Effects of dietary amylose/amylopectin ratio on antioxidant ability and amino metabolism in the liver of weaned piglets undergoing feed transition and challenged with lipopolysaccharide. Front. Nutr. 11:1435051. doi: 10.3389/fnut.2024.1435051
Edited by:
Hongliang Zeng, Fujian Agriculture and Forestry University, ChinaReviewed by:
Yang Li, Shandong Agricultural University, ChinaLei Wang, Wuhan Polytechnic University, China
Xiaokang Lv, Anhui Science and Technology University, China
Copyright © 2025 Yang, Tang, Wang, Yang, Yang, Wang and Yin. This is an open-access article distributed under the terms of the Creative Commons Attribution License (CC BY). The use, distribution or reproduction in other forums is permitted, provided the original author(s) and the copyright owner(s) are credited and that the original publication in this journal is cited, in accordance with accepted academic practice. No use, distribution or reproduction is permitted which does not comply with these terms.
*Correspondence: Can Yang, eWFuZ2NhbnNreUAxNjMuY29t; Yulong Yin, eWlueXVsb25nQGlzYS5hYy5jbg==
†These authors have contributed equally to this work
‡ORCID: Can Yang, https://orcid.org/0000-0001-8973-6994
Huansheng Yang, https://orcid.org/0000-0003-1164-5771