- 1Department of Rehabilitation, Shengjing Hospital of China Medical University, Shenyang, Liaoning, China
- 2Department of Physical Medicine and Rehabilitation, The Second Clinical College, China Medical University, Shenyang, Liaoning, China
The escalating prevalence of MetS, driven by global obesity trends, underscores the urgent need for innovative therapeutic strategies. To gain a deeper understanding of the therapeutic potential of vitamin D in addressing MetS, we embarked on a targeted literature review that thoroughly examines the scientific underpinnings and pivotal discoveries derived from pertinent studies, aiming to unravel the intricate mechanisms through which vitamin D exerts its effects on MetS and its components. This article explores the multifunctional role of vitamin D in the management of MetS, focusing on its regulatory effects on insulin sensitivity, lipid metabolism, inflammation, and immune response. Through an extensive review of current research, we unveil the complex mechanisms by which vitamin D influences MetS components, highlighting its potential as a therapeutic agent. Our analysis reveals that vitamin D's efficacy extends beyond bone health to include significant impacts on cellular and molecular pathways critical to MetS. We advocate for further research to optimize vitamin D supplementation as a component of precision medicine for MetS, considering the safety concerns related to dosage and long-term use.
1 Introduction
In the wake of an accelerating modern lifestyle and shifting dietary patterns, obesity has ascended as a paramount challenge within the global public health sphere (1–3). Serving as a pivotal risk factor for MetS, obesity intricately intertwines with an array of metabolic aberrations, notably abnormal glucose metabolism, hypertension, and lipid dysregulation (4). This syndrome, distinguished by its prevalent occurrence and significant health ramifications, has become a critical focus in medical research endeavors. The prevalence of MetS exhibits considerable geographical variance, with rates spanning from 24.3% to 44.2% across specific regions in Asia, Europe, Mexico, and the United States (5). Notably, the prevalence in the United States has escalated from 36.2% to 47.3%, signaling a decline in cardiovascular health intricately linked to obesity, glucose imbalances, and blood pressure complications (6).
Amidst the exploration of metabolic syndrome, the significance of vitamin D has emerged with increasing prominence. This lipophilic vitamin, traditionally recognized for its pivotal roles in bone health and calcium-phosphorus homeostasis, has been unveiled by contemporary research to harbor extensive functionalities in immune modulation, cellular proliferation and differentiation, alongside energy metabolism (7–9). Specifically, within the milieu of obesity and metabolic syndrome, a deficiency in vitamin D is acknowledged as a consequential risk element (10–12).
Despite the widespread attention on vitamin D, its efficacy as a therapeutic agent remains inconsistent. Studies have shown that vitamin D supplementation may not necessarily reduce the risk of fractures or chronic diseases, and there is still debate about its optimal intake and supplementation methods. Therefore, comprehensive consideration of various factors is required to provide precise treatment recommendations for patients.
Given the rising prevalence of metabolic syndrome and its association with obesity, it is imperative to investigate potential therapeutic avenues. One such avenue that has garnered increasing attention is the role of vitamin D. This investigation aims to comprehensively explore the role of vitamin D in the therapeutic management of MetS, elucidating its multifaceted impact on MetS components, potential mechanistic pathways, and practical challenges in clinical implementation, with the ultimate goal of establishing a robust theoretical foundation for precision medicine and tailored therapeutic strategies targeting MetS.
2 Metabolic syndrome
Metabolic syndrome, characterized by multiple metabolic aberrations, has been witnessing a persistent global increase in prevalence, significantly impacting public health (5, 13–15). This intricate condition is driven by a synergy of adverse lifestyle choices, environmental factors, and genetic predispositions, with a notably higher incidence in developed nations (16–18). Concurrent with economic progress, the prevalence in developing countries is also on the rise. For instance, in China, with the rapid economic growth and urbanization over the past decades, there has been a significant increase in the prevalence of metabolic syndrome, particularly in urban areas where lifestyle changes such as sedentary work, westernized diet, and reduced physical activity have become more prevalent (19–21). The syndrome is intimately linked with the global rise in obesity, hypertension, diabetes, and other metabolic disorders, signaling an ongoing escalation in future disease risk (22, 23). Therefore, a thorough investigation into its risk factors and complications is imperative for devising effective prevention and control strategies.
3 Vitamin D
Vitamin D is a fat-soluble vitamin essential for human health (24–26). It serves as a precursor for several metabolites, including 25-hydroxyvitamin D3 [25-(OH)D3] and 1,25-dihydroxyvitamin D3 [1,25-(OH)2D3] (27). The human body cannot synthesize vitamin D on its own and must obtain it through sunlight exposure or dietary intake. Under the influence of ultraviolet radiation on the skin, 7-dehydrocholesterol is converted into a vitamin D precursor, which is then hydroxylated in the liver to form 25-hydroxyvitamin D [25-(OH)D]; this form of vitamin D is transported to the kidneys, where it is converted by the 1α-hydroxylase enzyme into the more active form, 1,25-dihydroxyvitamin D3 [1,25(OH)2D3] (28). 1,25(OH)2D3 exerts its biological effects by binding to the Vitamin D Receptor (VDR) (29, 30). Understanding this metabolic process leads us to further explore the crucial roles that vitamin D plays in the body. Not only is it pivotal in maintaining calcium-phosphorus metabolism balance and promoting bone health, but it also regulates immune functions, highlighting its multifaceted importance in human health (31–33).
4 Vitamin D and metabolic syndrome
Vitamin D plays a pivotal role in the prevention and management of MetS, a cluster of metabolic abnormalities that significantly impact public health. Its multifaceted functions extend beyond bone health, encompassing insulin sensitivity, lipid metabolism, inflammation reduction, and immune regulation, all of which are crucial in MetS prevention. Studies have elucidated a close correlation between vitamin D levels and the risk of metabolic syndrome (34–36). Insufficiency of vitamin D may elevate the incidence of metabolic syndrome, a pathological state encompassing obesity, hypertension, hyperglycemia, and dyslipidemia (37, 38). Vitamin D, through its regulation of calcium-phosphorus metabolism and maintenance of immune function, exhibits a spectrum of biological activities crucial for health (31–33). A deficiency in vitamin D can exacerbate insulin resistance, promote lipogenesis and inflammatory responses, thereby augmenting the risk of metabolic syndrome (39). Moreover, vitamin D influences cell signaling through the regulation of calcium ion balance, closely associating with the development of metabolic syndrome (40). Epidemiological studies support the relationship between low levels of vitamin D and an increased risk of metabolic syndrome, indicating that vitamin D supplementation could mitigate its prevalence (41–43).
Vitamin D plays a significant role in key areas of metabolic syndrome, including insulin sensitivity, lipid metabolism, inflammation reduction, immune response, blood pressure regulation, cardiovascular health, and bone health (Figure 1) (31, 34, 39, 43–46). In terms of insulin sensitivity, vitamin D enhances the binding capacity of insulin to its receptor and improves insulin efficacy by maintaining intracellular calcium ion balance. Regarding lipid metabolism, vitamin D modulates gene expression within adipocytes to reduce lipogenesis, promote lipolysis, and affect lipid transport in the blood, thereby lowering the risk of atherosclerosis. In reducing inflammatory responses, vitamin D inhibits the expression of inflammatory markers through certain signaling pathways, exhibiting anti-inflammatory effects, with its deficiency linked to the onset of various inflammatory diseases. In regulating immune responses, vitamin D, in conjunction with its receptor VDR, regulates the expression of a series of genes and proteins related to immune responses, thereby finely controlling immune reactions, inhibiting excessive activation and inflammation, and maintaining the balance, homeostasis, and health of the immune system, providing effective immune protection for the body. In blood pressure regulation, vitamin D affects the renin-angiotensin system and vascular smooth muscle function, contributing to the maintenance of normal blood pressure. The cumulative effect of these actions helps improve symptoms of metabolic syndrome and reduce the risk of related complications. In regulating cardiovascular health, vitamin D enhances intracellular substance synthesis, promotes vasodilation and anti-inflammation, and reduces the risk of atherosclerosis through cell signaling and gene regulation, ensuring the integrity of vascular structure and function. Additionally, vitamin D is crucial for bone health, enhancing the synthesis of bone matrix proteins and inhibiting bone resorption, while regulating gene transcription to promote calcium absorption and bone mineralization, and also inhibiting inflammation, reducing the risk of osteoporosis and fractures in patients with metabolic syndrome.
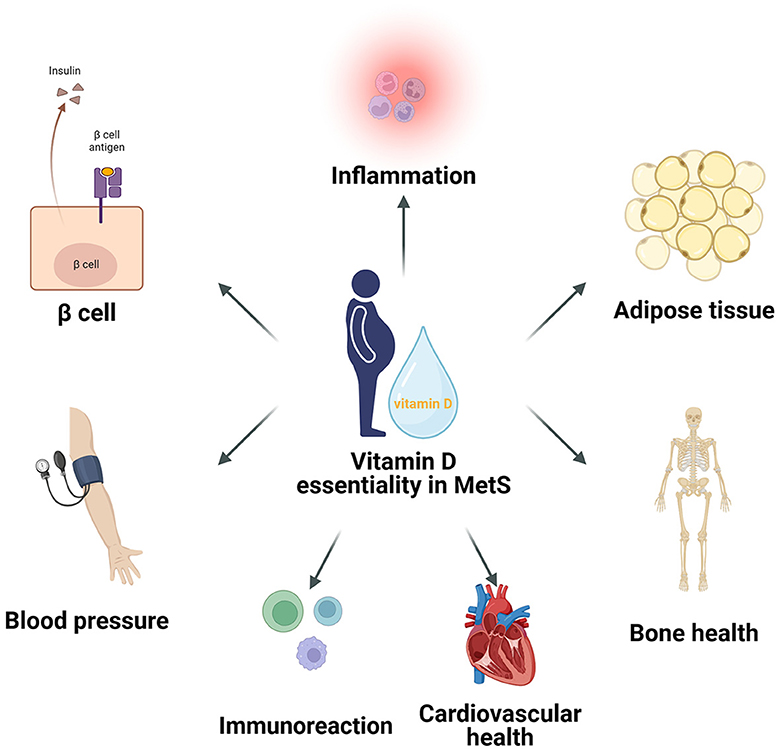
Figure 1. The critical roles of Vitamin D in metabolic syndrome, encompassing its significant impact on insulin sensitivity, lipid metabolism, inflammatory reduction, immune response, blood pressure regulation, cardiovascular health, and bone health.
Although epidemiological studies suggest a link between low vitamin D levels and an elevated risk of metabolic syndrome, limitations such as the observational nature of these studies, population heterogeneity, and the complexity of metabolic syndrome itself hinder the establishment of causal relationships (47, 48). Rigorous clinical trials are needed to further investigate the potential benefits of vitamin D supplementation in mitigating metabolic syndrome symptoms.
5 Mechanisms of vitamin D action
5.1 Effects of vitamin D on metabolic pathways
Table 1 has summarized the molecular pathways of vitamin D's effects on metabolic syndrome-related mechanisms.
5.1.1 Regulation of insulin resistance and glucose metabolism by vitamin D
Vitamin D plays a crucial role in regulating insulin resistance and its associated processes (67, 68). This regulatory function is manifested at multiple levels; it can control gene transcription and cell signaling through various mechanisms, thereby alleviating insulin resistance, especially in adipose tissue (69). Vitamin D can increase the expression of insulin receptors, which is essential for maintaining insulin signaling (70). A deficiency in vitamin D can lead to a decrease in the number of insulin receptors, thereby inducing insulin resistance (71). Additionally, vitamin D deficiency may also cause an increase in intracellular Ca2+ concentration, reducing the activity of glucose transporter 4 (GLUT4), which is another key mechanism of insulin resistance (49). Inflammation in adipose tissue is also a critical factor in insulin resistance, primarily driven by adipose macrophages (72). Cytokines released by these macrophages, such as IL-6 and TNF-α, play significant roles in the development and progression of insulin resistance (50, 51). These cytokines can activate specific signaling pathways, such as Jun N-terminal kinase 1 (JNK1) and IKK-β/NF-κB, whose activation further leads to a reduction in insulin signaling (73). Importantly, vitamin D has anti-inflammatory effects, capable of reducing the inflammatory process by decreasing the release of chemokines and cytokines that drive inflammation and lowering the chemotaxis of monocytes (74). Vitamin D enhances insulin sensitivity by increasing insulin receptor expression and improving intracellular calcium ion balance. This leads to more efficient glucose uptake and utilization, thereby reducing the risk of developing type 2 diabetes, a key component of MetS.
Although vitamin D plays a significant role in regulating insulin resistance and related processes, there are contradictory findings regarding its direct effects on pancreatic β-cells and glucose metabolism (75, 76). Some studies indicate that vitamin D can directly influence β-cell function, enhance insulin secretion, and improve glucose metabolism, while other studies have not observed significant effects or have found inconsistent results. These discrepancies may stem from differences in experimental design and other variables. Therefore, future research needs to further explore the direct mechanisms of vitamin D's action on pancreatic β-cells, as well as how these mechanisms manifest in different populations.
5.1.2 Regulation of fat metabolism by vitamin D
The mechanisms by which vitamin D acts in adipose tissue through the VDR receptor gene are complex (Figure 2). These findings collectively reveal vitamin D's multifaceted role in adipose tissue metabolism, including the regulation of lipolysis, fatty acid oxidation, and adipocyte differentiation (52, 77, 78). In vitro studies suggest that vitamin D can influence the fate of preadipocytes by regulating the expression of FASN (53), indicating its potential role in adipogenesis. Additionally, the vitamin D-VDR complex significantly impacts the differentiation process of adipocytes (79). Animal studies have shown that mice with a knockout of the VDR receptor gene (VDR-/-) exhibit an increased rate of energy metabolism, accompanied by an increase in the expression of the uncoupling protein (UCP) family in the mitochondrial respiratory chain and enhanced capacity for fatty acid β-oxidation, leading to a significant reduction in adipose tissue (80). Conversely, findings in VDR transgenic mice have been conflicting. While some studies show that overexpression of VDR in adipose tissue inhibits lipolysis and fatty acid β-oxidation, thereby inducing obesity (81), other studies may present contrasting results. This highlights the need for further research to clarify the exact role of VDR in adiposity. In vitro experiments further confirm that vitamin D can downregulate the expression of FASN, thereby inhibiting the transformation of pre-adipocytes (3T3-L1) into mature adipocytes (54). This finding reveals that vitamin D, by regulating the expression of key transcription factors, determines the direction of cell differentiation, playing a crucial role in forming different types of adipose tissue, such as white adipose tissue (WAT), brown adipose tissue (BAT), and beige adipose tissue (77). In adipose tissue, vitamin D can inhibit the expression of key transcription factors such as C/EBPα and PPAR-γ, thereby inhibiting fat construction (82). Additionally, vitamin D can influence lipid formation by activating C/EBPβ and ETO, inhibiting the transcription of C/EBPβ (31), and affecting adipocyte differentiation through the WNT/β-catenin signaling pathway (83). Moreover, research by Katayama and others found that VDR is also overexpressed in the sympathetic ganglia, adrenal medulla, and certain neurons in the central nervous system (84). These tissues are potential sites that could affect energy homeostasis, suggesting that vitamin D may influence energy homeostasis not only through its effects on adipose tissue but also through actions within the central nervous system affecting energy metabolism. By modulating gene expression within adipocytes, vitamin D promotes lipolysis and reduces lipogenesis, ultimately lowering plasma triglyceride and cholesterol levels. This reduces the risk of atherosclerosis and other cardiovascular complications associated with MetS.
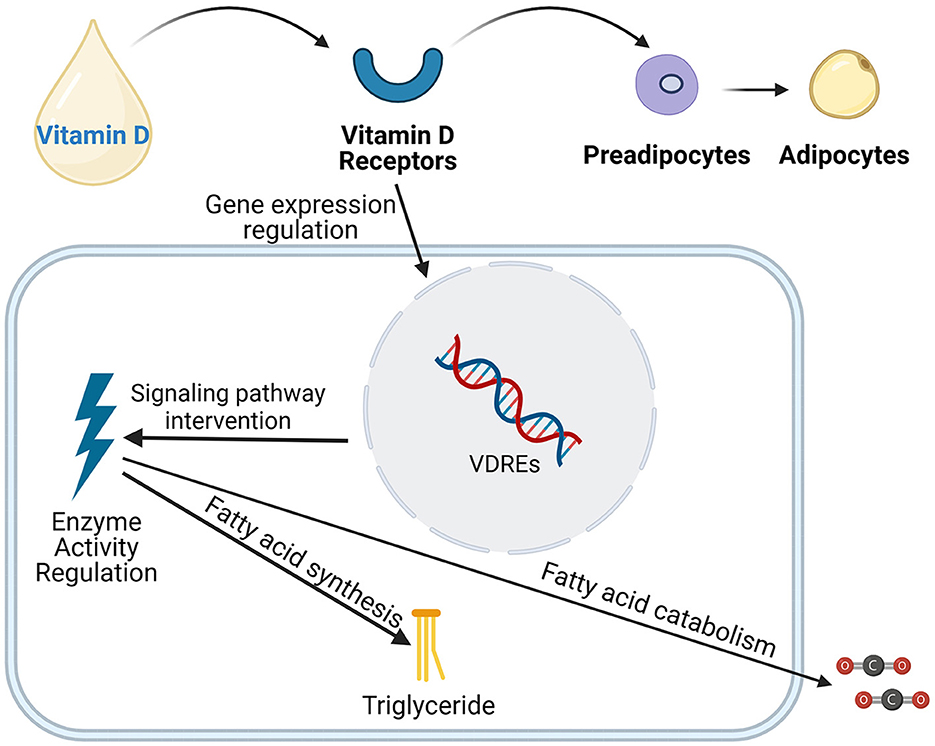
Figure 2. Vitamin D influences the differentiation, development of adipose cells, as well as the synthesis and breakdown of fatty acids through various mechanisms, including the regulation of gene expression, intervention in signaling pathways, and modulation of enzyme activity.
5.1.3 Anti-inflammatory action of vitamin D
Upon binding to the Vitamin D Receptor (VDR), active vitamin D undergoes a series of biochemical reactions, including phosphorylation, to form a heterodimer with the Retinoid X Receptor (RXR) and bind to VDREs to form a complex (85). This complex can influence gene transcription, thereby exerting anti-inflammatory and immunoregulatory biological functions (86). In terms of immunoregulation, vitamin D can affect the activity of immune cells such as antigen-presenting cells, monocytes-macrophages, and T and B lymphocytes (87, 88). However, it's important to note that the outcomes of vitamin D supplementation on inflammation and immune response can vary depending on the dosage, duration of supplementation, and the population studied (89). For instance, while appropriate levels of vitamin D can effectively modulate the immune system, excessive intake may lead to hypercalcemia and other adverse effects, which could potentially exacerbate inflammatory conditions (90). Active vitamin D can specifically activate peripheral CD4+ T cells, inhibiting the proliferation of Th1 cells and their production of pro-inflammatory cytokines, and stimulating Th2 cells to secrete IL-4, IL-5, and IL-10, making the immune tolerance response dominated by Th2 cells prevail over the immune rejection response dominated by Th1 cells (55). Additionally, vitamin D can downregulate the expression of Class II Major Histocompatibility Complex (MHC II), inhibit the maturation of dendritic cells, making it difficult for T cells to be activated and present antigens, thereby inducing immune tolerance (91). Simultaneously, vitamin D can inhibit the activation of B cells, induce the production of regulatory T cells (Treg), and inhibit the activity of Th17 cells. These mechanisms collectively enable vitamin D to play a significant role in anti-inflammation and immunoregulation (92). Moreover, it can inhibit the expression of inflammatory markers through the NF-κB classical inflammation signaling pathway or the p38 MAPK signaling pathway, exerting its anti-inflammatory effects (56). This may explain why vitamin D deficiency is associated with the incidence of inflammatory diseases such as obesity, metabolic syndrome, and type 2 diabetes. Conversely, excessive vitamin D intake, especially in the form of megadoses, has been reported to cause hypercalcemia, which can lead to calcification of soft tissues and potentially exacerbate inflammatory processes (90). Maintaining optimal vitamin D levels within the recommended dietary allowances is crucial to ensure its beneficial effects on inflammation and immune modulation. Vitamin D exerts potent anti-inflammatory effects by inhibiting the production of inflammatory cytokines, by macrophages and other immune cells. This attenuation of inflammation is pivotal in preventing the development of insulin resistance and other inflammatory-mediated metabolic disorders.
5.1.4 Regulation of immune cells and immune responses by vitamin D
Vitamin D plays a pivotal role in immunoregulation, a function closely related to its specific metabolic pathways. Some 25-hydroxyvitamin D3 can also be metabolized into 24,25-dihydroxyvitamin D3 under the action of 24-hydroxylase (93), ensuring vitamin D's effective regulatory role in the immune system. Specifically, vitamin D can finely modulate the Th1/Th2 balance, inhibit the overproliferation of Th1 cells, and limit the secretion of Th1-type cytokines, including IFN-γ, IL-2, and TNF-α, thus preventing excessive activation of autoimmune responses (57). Additionally, vitamin D can block the activation and secretion of the key autocrine growth factor IL-2 in T lymphocytes, further reducing the risk of autoimmune responses (94). Moreover, recent studies have shown that the immune response to vitamin D can vary among different populations due to genetic, environmental, and lifestyle factors. For instance, certain ethnic groups may have different vitamin D receptor polymorphisms, affecting their response to vitamin D and subsequently, their immune reactions (95). Therefore, it is crucial to consider population-specific differences when evaluating the immunomodulatory effects of vitamin D. Vitamin D also induces the polarization of CD4+ T lymphocytes toward a Th2 response, promoting the secretion of Th2-type cytokines (such as IL-4, IL-5, IL-9, IL-10, and IL-13), inhibiting excessive inflammatory responses, and maintaining the balance of the immune system (96). Mariz et al. confirmed that in female groups with higher levels of vitamin D, a lower mean concentration of TNF-α in the serum was observed, providing strong evidence of the significant role of vitamin D in immunoregulation (97). Furthermore, vitamin D deficiency has been linked to an increased risk of autoimmune diseases, particularly those related to MetS. Studies suggest that optimizing vitamin D levels may help reduce the severity and progression of these conditions (98). These findings not only reveal the close link between vitamin D metabolism and immunoregulation but also suggest potential applications of vitamin D in the treatment of immune-related diseases, providing a theoretical basis for such use.
5.1.5 Vitamin D and its role in health issues associated with metabolic syndrome
5.1.5.1 Blood pressure regulation
Vitamin D plays a pivotal role in blood pressure regulation, particularly in the context of metabolic syndrome. Its active form, 1,25-dihydroxyvitamin D3, regulates the expression of genes involved in blood pressure control, including those encoding renin and angiotensinogen (7, 99). By inhibiting renin synthesis, vitamin D reduces the production of Angiotensin II, a vasoconstrictor that increases peripheral vascular resistance. This leads to decreased vasoconstriction and lower peripheral vascular resistance, effectively regulating blood pressure (58). Additionally, vitamin D promotes nitric oxide release from endothelial cells, a potent vasodilator, maintaining homeostasis and normal blood flow (59). Its antioxidant and anti-inflammatory properties reduce oxidative stress and inflammatory responses that damage blood vessels, further contributing to blood pressure reduction (60). Vitamin D not only regulates blood pressure through mechanisms such as inhibiting renin synthesis, promoting nitric oxide release, and reducing oxidative stress and inflammation, but its overall therapeutic effects on these processes also contribute to the prevention and management of metabolic syndrome (100).
5.1.5.2 Cardiovascular health
In the context of metabolic syndrome, vitamin D exerts multifaceted effects on cardiovascular health. It maintains serum calcium levels by regulating calcium balance and bone metabolism, involving interactions with parathyroid hormone and renin (61, 101). Vitamin D directly protects the cardiovascular system by inhibiting inflammatory responses and oxidative stress, improving insulin resistance and glucose metabolism (86, 102). It also regulates endothelial function, lipid metabolism, and reduces arterial calcification (62, 103, 104), critical for maintaining cardiovascular health. By activating intracellular signaling pathways, vitamin D fine-tunes the expression of genes related to cell proliferation, migration, and apoptosis, preventing atherosclerosis development (63, 105). Finally, vitamin D interacts with other nutrients, jointly maintaining normal cardiovascular function (106). In the context of metabolic syndrome, vitamin D exerts multifaceted therapeutic effects on cardiovascular health, including maintaining calcium balance, protecting against inflammatory responses and oxidative stress, regulating endothelial function, reducing arterial calcification, and preventing atherosclerosis development, all of which contribute to the prevention and management of metabolic syndrome.
5.1.5.3 Bone health
Vitamin D is a key regulatory factor for bone health, especially in the context of metabolic syndrome. Its active form forms a complex with the VDR, regulating bone mineralization (64, 65). This regulation is crucial for maintaining bone health in MetS, where bone metabolism may be altered (107, 108). Vitamin D ensures an adequate supply of calcium and phosphate for normal mineralization by regulating their intestinal absorption (66). It also regulates osteoprotegerin, a key factor in bone formation, mineralization, and resorption (109, 110). By stimulating the expression of bone cell-specific genes, vitamin D promotes the synthesis of sclerostin, further contributing to bone health maintenance (111). Additionally, vitamin D modulates the immune system, inhibiting inflammatory cytokine production, reducing bone inflammation, and lowering the risk of osteoporosis and fractures (112–114). Vitamin D exerts therapeutic effects on bone health in the context of metabolic syndrome, regulating bone mineralization, ensuring adequate calcium and phosphate supply, modulating osteoprotegerin levels, promoting sclerostin synthesis, and modulating the immune system to reduce inflammation and lower the risk of osteoporosis and fractures, all contributing to the prevention and management of metabolic syndrome.
Furthermore, future studies should delve deeper into the interactions between vitamin D supplementation and other metabolic syndrome (MetS) treatments. Understanding these interactions is crucial for optimizing therapeutic approaches, enhancing treatment efficacy, and ensuring patient safety. By considering the synergistic or antagonistic effects of vitamin D with other medications, we can provide more personalized and precise treatment strategies for patients.
5.2 Cellular and molecular mechanisms of vitamin D
5.2.1 Vitamin D's multifaceted roles in pancreatic β-cell function and glucose regulation: a deep dive into the mechanisms
Vitamin D holds a pivotal position in pancreatic β-cell function and glucose regulation (115). Its direct interaction with β-cells at the cellular level enhances insulin gene expression, thereby promoting insulin synthesis and release (116). These processes are crucial for maintaining blood glucose homeostasis (117).
5.2.1.1 Vitamin D and the VDR
When vitamin D binds to the VDR, it triggers a cascade of signaling pathways (118, 119). These pathways not only regulate insulin production but also precisely adjust the expression of genes associated with apoptosis. This fine-tuning supports β-cell survival and ensures their optimal function (120, 121).
5.2.1.2 Molecular mechanisms of vitamin D action
Digging deeper, the formation of the vitamin D-VDR complex enables the recognition of Vitamin D Response Elements (VDREs) located within the cell nucleus. This recognition mechanism regulates the transcription of genes closely linked to glucose metabolism and β-cell apoptosis (86). Beyond this, vitamin D also contributes to maintaining cellular homeostasis in β-cells. It achieves this by adjusting calcium ion concentrations, thus preventing calcium overload-induced apoptosis. Additionally, it bolsters the cells' antioxidant defenses against oxidative stress (122, 123).
5.2.1.3 Practical and clinical implications: harnessing vitamin D for improved type 2 diabetes management
Vitamin D plays a pivotal role in pancreatic β-cell function and glucose regulation. It not only promotes insulin synthesis and release, thereby maintaining glucose homeostasis, but also safeguards β-cells against damage by regulating intracellular calcium ion balance and enhancing antioxidant defenses, prolonging their survival and function. This understanding offers novel therapeutic avenues for managing type 2 diabetes, wherein personalized vitamin D supplementation strategies, as adjunctive therapy, may contribute to reducing the need for exogenous insulin and improving glycemic control in patients (124).
5.2.1.4 Challenges, limitations, and future directions
Despite the immense potential of vitamin D in modulating immune function and pancreatic β-cell health, determining the optimal dosage and supplementation approach remains challenging (125). Variations in individual responses to vitamin D necessitate consideration of personal differences in therapeutic strategies to achieve personalized supplementation. Additionally, the safety and potential side effects of long-term vitamin D supplementation warrant further evaluation (126).
Current research predominantly relies on observational studies and animal experiments, lacking large-scale, long-term clinical trials to validate the precise efficacy of vitamin D in diabetes management. Moreover, the incomplete understanding of vitamin D's interactions with other medications and nutrients may impact its application effectiveness in clinical practice.
Future research should prioritize high-quality randomized controlled trials to clarify the effectiveness and safety of vitamin D in diabetes management. Additionally, exploring the synergistic effects of vitamin D with other therapeutic approaches and developing optimized supplementation protocols tailored to different populations will be crucial for advancing its application in diabetes treatment. Furthermore, intensifying studies on the underlying mechanisms of vitamin D's actions can unveil more of its functions in diabetic pathogenesis.
5.2.2 Vitamin D's role in adipocyte differentiation and metabolism: mechanisms and current understanding
Vitamin D plays a crucial role in the differentiation and metabolism of adipocytes, processes that are fundamental to adipose tissue formation and function (80).
5.2.2.1 Adipocyte differentiation and vitamin D
During adipocyte differentiation, pre-adipocytes transform into mature adipocytes in response to specific environmental signals. Vitamin D, through its activation of the VDR, modulates this transition by precisely adjusting the expression of genes intricately involved in adipocyte differentiation (77). This regulatory action notably decelerates the differentiation process, ultimately leading to a reduction in the overall number of fat cells (53, 79).
5.2.2.2 Vitamin D and adipocyte metabolism
Beyond its role in differentiation, vitamin D also regulates the metabolic functions of adipocytes (127). It achieves this by balancing the synthesis and breakdown of fatty acids within these cells. By modulating the activity of enzymes that are involved in fatty acid metabolism, vitamin D effectively decreases fatty acid synthesis and increases its oxidation, thereby reducing intracellular fat storage (128, 129).
5.2.2.3 Molecular mechanisms
At the molecular level, the binding of vitamin D to VDR enables it to specifically recognize and interact with VDREs in the cell nucleus. This interaction regulates the transcription of genes directly implicated in fat formation (130). Through this mechanism, vitamin D selectively inhibits or activates specific genes, offering precise control over the rate and extent of adipogenesis (131). Research by Mutt et al. has shown that vitamin D can downregulate the expression of key genes, such as fatty acid synthase (FASN), which is essential for fatty acid synthesis (132). By suppressing these genes, vitamin D further diminishes fatty acid production, thus inhibiting adipogenesis. Moreover, vitamin D modulates the fat formation process by influencing intracellular signaling pathways, which are crucial for information transfer within cells (133). Harahap et al. suggest that vitamin D can activate or inhibit specific kinases and transcription factors, further regulating adipogenesis (134).
5.2.2.4 Practical and clinical implications: optimizing vitamin D supplementation for weight and lipid control
Given the specific mechanisms by which vitamin D regulates adipocyte differentiation and metabolism, it holds significant practical implications for weight and lipid control in clinical settings. By precisely modulating the dosage of vitamin D supplementation, it can effectively decelerate the differentiation process of adipocytes, leading to a reduction in the total number of fat cells and contributing to weight management (135). Additionally, vitamin D's ability to balance fatty acid synthesis and oxidation, thereby decreasing intracellular fat storage, facilitates improved lipid profiles, specifically reducing triglycerides and low-density lipoprotein (LDL) cholesterol levels while potentially increasing high-density lipoprotein (HDL) cholesterol (136). As such, for patients with obesity and metabolic syndrome, individualized vitamin D supplementation protocols may serve as an adjunct approach to weight control, enhancing the effectiveness of lipid management and mitigating the risk of cardiovascular diseases. Future research and clinical practice should delve deeper into determining optimal vitamin D doses for diverse populations, aiming to maximize its benefits in terms of weight and lipid control.
5.2.2.5 Challenges, limitations, and future directions
In the exploration of vitamin D's therapeutic potential in metabolic syndrome management, several challenges and limitations have emerged. Despite the widely recognized significance of vitamin D in adipocyte growth and metabolism, there remains a lack of consensus on the optimal daily dose of vitamin D supplementation (137). This is primarily due to the inconsistent findings from different studies, which can be attributed to variations in experimental conditions and test populations. Moreover, the potential interaction between vitamin D and other nutrients adds to the complexity of devising effective supplementation strategies (138).
Additionally, individual differences in response to vitamin D underscore the need for personalized supplementation approaches. Given these uncertainties, further research is imperative to determine the optimal individualized dose and method of vitamin D supplementation for patients with metabolic syndrome.
Looking ahead, future research directions should aim to clarify the mechanisms underlying vitamin D's multifaceted effects, explore its interactions with other hormones and nutrients, and assess the long-term safety and efficacy of supplementation. By addressing these challenges and limitations, we can develop more targeted and effective therapeutic strategies for the management of metabolic syndrome.
5.2.3 Vitamin D's role in immune regulation and inflammation: mechanisms and current understanding
Vitamin D profoundly influences the development and functional expression of immune cells (139, 140). It meticulously adjusts the gene expression profiles of key inflammatory regulators, such as macrophages, T cells, and B cells, by activating the VDR within these cells. This, in turn, regulates the inflammatory mediators they release.
5.2.3.1 Vitamin D and immune cell gene expression
Upon binding to VDR, vitamin D forms a complex that can precisely identify and bind to VDREs in the cell nucleus (141). This binding regulates the transcription of genes closely related to inflammation, providing a molecular basis for the immune response modulation (142, 143). Specifically, vitamin D can inhibit the production of inflammatory cytokines, such as TNF-α, IL-1β, and IL-6, by macrophages, effectively mitigating the body's inflammatory response (144).
5.2.3.2 Regulation of inflammatory genes
The vitamin D-VDR complex selectively inhibits or activates specific inflammatory genes, offering a deeper understanding of how inflammation is controlled at the molecular level (145). Studies have confirmed that vitamin D can inhibit the activation of the key inflammatory transcription factor NF-κB, subsequently downregulating the expression of various inflammatory genes and reducing the production of inflammatory mediators (56).
5.2.3.3 Intracellular signaling pathways
Beyond direct gene regulation, vitamin D also influences the inflammatory process by regulating intracellular signaling pathways (146). These pathways, akin to information superhighways within cells, are effectively modulated by vitamin D, adjusting the synthesis and release of inflammatory mediators (147). Specifically, vitamin D can activate signaling molecules such as PKA and PKC, further regulating downstream inflammatory responses (70, 148, 149).
5.2.3.4 Practical and clinical implications of the study: vitamin D as an immunomodulator and anti-inflammatory agent
In clinical practice, vitamin D supplementation demonstrates remarkable immunomodulatory and anti-inflammatory potential (150). By inhibiting the release of pro-inflammatory cytokines and key transcription factors, vitamin D offers a promising therapeutic approach for alleviating systemic inflammation under conditions such as metabolic syndrome and autoimmune diseases (141). This mechanism not only targets the underlying inflammatory pathways but also addresses the broader spectrum of health complications associated with chronic inflammation, thereby enhancing overall patient outcomes and quality of life.
5.2.3.5 Challenges, limitations, and future directions
Despite the immense potential of vitamin D in immune regulation and anti-inflammatory actions, its clinical application faces several challenges. The optimal dosage and duration of vitamin D supplementation remain unclear, necessitating further research (151). Moreover, the incomplete understanding of its interaction mechanisms with other nutrients and medications hinders its use in treating multifactorial diseases. Additionally, long-term excessive intake of vitamin D may pose potential health risks, such as hypercalcemia and soft tissue calcification, limiting its widespread clinical adoption.
Research on vitamin D's immune regulatory and anti-inflammatory effects still has limitations. Most studies focus on animal models and in vitro cell cultures, lacking large-scale clinical trials to validate its efficacy (152). The complex mechanisms of vitamin D involve multiple signaling pathways and cell types, making it difficult to determine its precise role. Furthermore, individual variability in responses to vitamin D may be influenced by genetic polymorphisms and other genetic factors.
Future research should focus on several key areas. Large-scale clinical trials are needed to verify the role of vitamin D in preventing and treating metabolic syndrome and related inflammatory diseases. In-depth exploration of vitamin D's mechanisms, particularly in immune regulation and anti-inflammation, is essential. Moreover, novel strategies for personalizing vitamin D supplementation dosages and durations must be developed to maximize its therapeutic potential while mitigating potential health risks. Through these efforts, we can anticipate vitamin D playing a more significant role in immune modulation and anti-inflammation, offering new insights and approaches for treating metabolic syndrome and related inflammatory conditions.
5.2.4 Roles of vitamin D in regulating other metabolic syndrome-related factors and research status
5.2.4.1 Blood pressure regulation
Vitamin D plays a crucial role in blood pressure regulation by influencing the function of endothelial cells and vascular smooth muscle cells (153, 154). It promotes the release of nitric oxide (NO), a vasodilator, from endothelial cells, contributing to the maintenance of normal blood pressure levels (155). Additionally, vitamin D inhibits the proliferation and migration of vascular smooth muscle cells, enhancing vascular stability and elasticity, further reducing the risk of hypertension (156, 157). Research indicates that vitamin D modulates blood pressure dynamics by regulating the expression of genes associated with blood pressure regulation, such as those involved in the renin-angiotensin system (RAS) (158–160). Furthermore, vitamin D's regulation of intracellular calcium ion balance indirectly affects blood pressure homeostasis (161).
5.2.4.2 Cardiovascular health
Vitamin D contributes significantly to cardiovascular health by enhancing the expression of nitric oxide synthase in cardiovascular cells, thereby promoting nitric oxide synthesis and release (162, 163). This aids in maintaining normal vascular function and elasticity, reducing the risk of cardiovascular diseases (164). Additionally, vitamin D activates intracellular signaling pathways in cardiovascular cells, fine-tuning the expression of genes and proteins related to cell proliferation, migration, and apoptosis (165). This inhibits abnormal proliferation and migration of vascular smooth muscle cells, effectively preventing the development of atherosclerosis. Vitamin D further mitigates oxidative stress and inflammatory responses in the cardiovascular system by regulating the expression of antioxidant and anti-inflammatory genes, thereby maintaining the normal structure and function of blood vessels (157, 166).
5.2.4.3 Bone health
Vitamin D directly affects osteoblasts and osteoclasts, crucial cells for bone formation and remodeling (167). It enhances the synthesis of bone matrix proteins, such as osteocalcin and collagen, essential for bone framework construction (168, 169). Simultaneously, vitamin D inhibits osteoclast activity, reducing bone resorption and degradation, thus maintaining bone strength and stability (170). By regulating the expression of genes related to calcium absorption and transport, vitamin D ensures an adequate calcium supply for bones, promoting normal bone mineralization and growth (171, 172). Furthermore, vitamin D indirectly safeguards bone health by modulating the immune system, inhibiting inflammatory cytokine production, and reducing bone inflammation, thereby lowering the risk of osteoporosis and fractures (173, 174).
5.2.4.4 Practical and clinical implications of the study
For blood pressure control, the crucial role of vitamin D in promoting the release of nitric oxide from endothelial cells and inhibiting the proliferation and migration of vascular smooth muscle cells highlights its potential as an adjuvant therapy in hypertension management (175). Regular supplementation of vitamin D could help maintain normal blood pressure levels and reduce the risk of hypertension, thereby improving cardiovascular outcomes.
In terms of cardiovascular health, the study underscores the importance of vitamin D in promoting nitric oxide synthesis and release, enhancing vascular function and elasticity (176). This suggests that vitamin D supplementation could aid in preventing cardiovascular diseases by improving the overall health of blood vessels. Moreover, vitamin D's ability to regulate intracellular signaling pathways related to cell proliferation, migration, and apoptosis in cardiovascular cells can contribute to the prevention of atherosclerosis.
Regarding bone health, the direct effects of vitamin D on osteoblasts and osteoclasts, as well as its role in regulating calcium metabolism, have important implications for the prevention and treatment of bone-related disorders. By ensuring adequate calcium supply for bones and inhibiting bone resorption, vitamin D supplementation can strengthen bone structure and reduce the risk of osteoporosis and fractures (177). This is particularly relevant for populations at risk of bone loss, such as the elderly or individuals with chronic diseases.
5.2.4.5 Challenges, limitations, and future directions
Vitamin D's clinical use faces challenges. Individual variability in response necessitates tailored dosing. Complex interactions with other nutrients hinder precise supplementation. Factors like lifestyle, environment, and genetics yield inconsistent results (1).
Research limitations include the predominance of observational studies, sample heterogeneity, and limited data on long-term safety and effectiveness, particularly in diverse populations (178).
Randomized trials are needed to establish causality. Explore vitamin D-nutrient interactions for personalized supplementation. Investigate metabolic differences across populations to enhance results' generalizability. Monitor long-term safety and effectiveness for safe clinical application.
5.3 Interactions of vitamin D with other metabolic hormones
The mechanisms of interaction between vitamin D and other metabolic hormones are complex and crucial for maintaining normal physiological functions in the human body (179). Firstly, in the interaction between vitamin D and insulin, the mechanism is primarily manifested by the action of the active form of vitamin D, 1,25-dihydroxyvitamin D3, in the pancreas. It can promote β-cell synthesis and secretion of insulin, thereby regulating blood sugar levels (180). At the same time, vitamin D can inhibit pancreatic α-cells, reducing the secretion of glucagon and stabilizing blood sugar (181). Insulin also affects vitamin D levels by promoting intestinal absorption of vitamin D, increasing the body's vitamin D concentration (182). Their synergistic action is evident as vitamin D enhances the expression of insulin receptor genes, improving cell sensitivity to insulin, which helps in improving insulin resistance (183).
Secondly, the interaction mechanism between vitamin D and parathyroid hormone (PTH) is primarily reflected in maintaining calcium balance (184). When blood calcium levels drop, vitamin D stimulates the activation of the calcium-sensing receptor (CaSR) in the parathyroid gland, leading to an increase in PTH levels, which then promotes the kidney to synthesize 1,25(OH)2D, enhancing the kidney's reabsorption of calcium (185). Simultaneously, 1,25(OH)2D and PTH act together on osteoblasts, regulating bone metabolism processes. This mechanism ensures the body's priority in maintaining calcium balance but may also lead to bone health issues (186).
Furthermore, the interaction mechanism between vitamin D and cortisol is represented by a direct antagonistic action. Vitamin D promotes the absorption and utilization of calcium, while cortisol inhibits these processes (187). This antagonistic action helps maintain the body's calcium homeostasis and related physiological functions.
Lastly, in the interactions between vitamin D and sex hormones like estrogen and testosterone, the mechanism is mainly manifested in their impact on bone health (188). Vitamin D and estrogen jointly regulate the function of osteoblasts and osteoclasts, maintaining bone homeostasis (189). Vitamin D may influence the synthesis and receptor expression of testosterone, regulating the physiological processes of testosterone (190). These interaction mechanisms collectively maintain bone health and the balance of sex hormones (191).
6 Conclusion
Vitamin D plays a crucial role in the treatment of metabolic syndrome. It is not only vital for bone health and calcium-phosphate metabolism but also plays a role in immune regulation and energy metabolism. Epidemiological and clinical studies indicate that vitamin D deficiency is closely associated with obesity, insulin resistance, dyslipidemia, and hypertension, which are components of metabolic syndrome. Its mechanisms of action involve the regulation of key metabolic pathways and interactions with other hormones. Although there is controversy over the dosage and efficacy of vitamin D supplementation, its potential in the treatment of metabolic syndrome warrants further exploration. Considering the safety concerns associated with long-term excessive intake, future research needs to define the appropriate dosage of vitamin D supplementation and its long-term safety. In summary, research on vitamin D provides new perspectives and strategies for the prevention and treatment of metabolic syndrome.
Author contributions
ZL: Writing – original draft, Conceptualization. ZW: Conceptualization, Supervision, Writing – original draft. YH: Conceptualization, Funding acquisition, Supervision, Writing – review & editing. XL: Conceptualization, Supervision, Writing – review & editing.
Funding
The author(s) declare financial support was received for the research, authorship, and/or publication of this article. This paper was funded by the Natural Science Foundation Program of Liaoning, China (No. 2022-YGJC-33).
Acknowledgments
We would like to extend our special thanks to YH, ZW, and Shenglin Xia, our colleagues who provided invaluable suggestions that enriched and refined the content of this manuscript. Their profound knowledge and insightful feedback were a great asset to our research, helping us to capture a more comprehensive understanding of the latest advancements and findings in our field. Moreover, we are deeply appreciative of the journal's editors and reviewers for their meticulous guidance and rigorous approach, which have been instrumental in shaping and polishing our review. Finally, we offer our heartfelt gratitude to all our supportive colleagues and institutions. We remain committed to furthering our contributions to the research and progress of this discipline.
Conflict of interest
The authors declare that the research was conducted in the absence of any commercial or financial relationships that could be construed as a potential conflict of interest.
Publisher's note
All claims expressed in this article are solely those of the authors and do not necessarily represent those of their affiliated organizations, or those of the publisher, the editors and the reviewers. Any product that may be evaluated in this article, or claim that may be made by its manufacturer, is not guaranteed or endorsed by the publisher.
References
1. Grahnemo L, Nethander M, Coward E, Gabrielsen ME, Sree S, Billod J-M, et al. Cross-sectional associations between the gut microbe Ruminococcus gnavus and features of the metabolic syndrome. Lancet Diabetes Endocrinol. (2022) 10:481–3. doi: 10.1016/S2213-8587(22)00113-9
2. Hwang JP, Siu KW, Foreman JT, Razouki Z, Bassaragh A, Boone T, et al. Electronic health records analytics to identify cancer patients with metabolic syndrome. J Clin Oncol. (2022) 40:e18649. doi: 10.1200/JCO.2022.40.16_suppl.e18649
3. Mocanu V, Zhang Z, Deehan EC, Kao DH, Hotte N, Karmali S, et al. Fecal microbial transplantation and fiber supplementation in patients with severe obesity and metabolic syndrome: a randomized double-blind, placebo-controlled phase 2 trial. Nat Med. (2021) 27:1272–9. doi: 10.1038/s41591-021-01399-2
4. Kawano Y, Edwards M, Huang Y, Bilate AM, Araujo LP, Tanoue T, et al. Microbiota imbalance induced by dietary sugar disrupts immune-mediated protection from metabolic syndrome. Cell. (2022) 185:3501–3519.e20. doi: 10.1016/j.cell.2022.08.005
5. Noubiap JJ, Nansseu JR, Lontchi-Yimagou E, Nkeck JR, Nyaga UF, Ngouo AT, et al. Global, regional, and country estimates of metabolic syndrome burden in children and adolescents in 2020: a systematic review and modelling analysis. Lancet Child Adolescent Health. (2022) 6:158–70. doi: 10.1016/S2352-4642(21)00374-6
6. Yang C, Jia X, Wang Y, Fan J, Zhao C, Yang Y, et al. Trends and influence factors in the prevalence, intervention, and control of metabolic syndrome among US adults, 1999–2018. BMC Geriatr. (2022) 22:672. doi: 10.1186/s12877-022-03672-6
7. Su H, Liu N, Zhang Y, Kong J. Vitamin D/VDR regulates peripheral energy homeostasis via central renin-angiotensin system. J Adv Res. (2021) 33:69–80. doi: 10.1016/j.jare.2021.01.011
8. Vernerová L, Vokurková M, Oreska S, Špiritović M, Klein M, Kropackova T, et al. POS0480 vitamin D and its receptor (VDR) gene expression in skeletal muscle associate with disease and muscle function parameters in idiopathic inflammatory myopathies. Ann Rheum Dis. (2022) 81:1–495. doi: 10.1136/annrheumdis-2022-eular.2598
9. Triantos C, Aggeletopoulou I, Mantzaris GJ, Mouzaki A. Molecular basis of vitamin D action in inflammatory bowel disease. Autoimmun Rev. (2022) 21:103136. doi: 10.1016/j.autrev.2022.103136
10. Pott-Junior H, Nascimento CM, Costa-Guarisco LP, Gomes GA, Gramani-Say K, Orlandi FD, et al. Vitamin D deficient older adults are more prone to have metabolic syndrome, but not to a greater number of metabolic syndrome parameters. Nutrients. (2020) 12:748. doi: 10.3390/nu12030748
11. Amirkhizi F, Khademi Z, Shahraki SH, Rahimlou M. Vitamin D insufficiency and its association with adipokines and atherogenic indices in patients with metabolic syndrome: a case-control study. Front Endocrinol. (2023) 14:1080138. doi: 10.3389/fendo.2023.1080138
12. D'Amelio P. Vitamin D deficiency and risk of metabolic syndrome in aging men. World J Mens Health. (2021) 39:291–301. doi: 10.5534/wjmh.200189
13. Peña-Jorquera H, Cid-Jofré V, Landaeta-Díaz L, Petermann-Rocha F, Martorell M, Zbinden-Foncea H, et al. Plant-based nutrition: Exploring health benefits for atherosclerosis, chronic diseases, and metabolic syndrome—A comprehensive review. Nutrients. (2023) 15:3244. doi: 10.3390/nu15143244
14. Liu F, Wang X, Pan M, Zhang K, Zhou F, Tong J, et al. Exposure to air pollution and prevalence of metabolic syndrome: a nationwide study in China from 2011 to 2015. Sci Total Environ. (2023) 855:158596. doi: 10.1016/j.scitotenv.2022.158596
15. Furuta K, Tang X, Islam S, Tapia A, Chen ZB, Ibrahim SH. Endotheliopathy in the metabolic syndrome: Mechanisms and clinical implications. Pharmacol Therapeut. (2023) 244:108372. doi: 10.1016/j.pharmthera.2023.108372
16. Walrabenstein W, Wagenaar C, Van der Leeden M, Gerritsen M, Van der Esch M, Van Schaardenburg D. POS0181 effect of a multidisciplinary lifestyle program on patients with metabolic syndrome-associated osteoarthritis: the plants for joints randomized controlled trial. Ann Rheum Dis. (2022) 81:1–322. doi: 10.1136/annrheumdis-2022-eular.1738
17. Sousa JA, Mendonca MI, Pereira A, Monteiro JP, Temtem AM, Santos M, et al. Shaping the future of metabolic syndrome: genetics, prognosis and individual tailoring. Eur Heart J. (2020) 41:ehaa946–3826. doi: 10.1093/ehjci/ehaa946.3826
18. Thanikachalam M, Lane K, Thanikachalam S. Satellite-based urbanization measures are independent predictors of metabolic syndrome. Circulation. (2021) 144:A12096. doi: 10.1161/circ.144.suppl_1.12096
19. Piuri G, Zocchi M, Della Porta M, Ficara V, Manoni M, Zuccotti GV, et al. Magnesium in obesity, metabolic syndrome, and type 2 diabetes. Nutrients. (2021) 13:320. doi: 10.3390/nu13020320
20. Misra A, Khurana L. Obesity and the metabolic syndrome in developing countries. J Clin Endocr Metab. (2008) 93:1595. doi: 10.1210/jc.2008-1595
21. Huang J, Huang JL, Wang J, Chung VC, Wong MC. Metabolic syndrome and risk of cancer in Chinese populations: a systematic review and meta-analysis in 57 260 individuals. Lancet. (2018) 392:S15. doi: 10.1016/S0140-6736(18)32644-8
22. Chew N, Ng C H, Kong G, Chin Y H, Lim O, Lim W H, et al. Metabolic associated fatty liver disease increases risk of adverse events after acute coronary syndrome. Eur Heart J. (2022) 43:1414. doi: 10.1093/eurheartj/ehac544.1414
23. Teklu M, Zhou W, Patel N, Manyak G, Dey AK, Lateef S, et al. Metabolic syndrome and its factors associate with non-calcified coronary plaque burden in chronic inflammation: results from a prospective observational study. Circulation. (2021) 143:221. doi: 10.1161/circ.143.suppl_1.P221
24. Krist AH, Davidson KW, Mangione CM, Cabana M, Caughey AB, Davis EM, et al. Screening for Vitamin D deficiency in adults: US preventive services task force recommendation statement. JAMA. (2021) 325:1436–42. doi: 10.1001/jama.2021.3069
25. Murai IH, Fernandes AL, Sales LP, Pinto AJ, Goessler KF, Duran CS, et al. Effect of a single high dose of vitamin D3 on hospital length of stay in patients with moderate to severe COVID-19: a randomized clinical trial. JAMA. (2021) 325:1053–60. doi: 10.1001/jama.2020.26848
26. Hahn J, Cook NR, Alexander EK, Friedman S, Walter J, Bubes V, et al. Vitamin D and marine omega 3 fatty acid supplementation and incident autoimmune disease: VITAL randomized controlled trial. BMJ. (2022) 376:452. doi: 10.1136/bmj-2021-066452
27. Bikle D, Christakos S. New aspects of vitamin D metabolism and action—Addressing the skin as source and target. Nat Rev Endocrinol. (2020) 16:234–52. doi: 10.1038/s41574-019-0312-5
28. Christakos S, Dhawan P, Verstuyf A, Verlinden L, Carmeliet G. Vitamin D: metabolism, molecular mechanism of action, and pleiotropic effects. Physiol Rev. (2016) 96:365–408. doi: 10.1152/physrev.00014.2015
29. Chen X, Wan Z, Geng T, Zhu K, Li R, Lu Q, et al. Vitamin D receptor polymorphisms, and risk of microvascular complications among individuals with type 2 diabetes: a prospective study. Diabetes Care. (2023) 46:270–7. doi: 10.2337/dc22-0513
30. Lu C, Zhou W, He X, Zhou X, Yu C. Vitamin D status and vitamin D receptor genotypes in celiac disease: a meta-analysis. Crit Rev Food Sci Nutr. (2020) 61:2098–106. doi: 10.1080/10408398.2020.1772716
31. LeBoff MS, Chou SH, Ratliff KA, Cook NR, Khurana B, Kim E, et al. Supplemental Vitamin D and incident fractures in midlife and older adults. New Engl J Med. (2022) 387:299–309. doi: 10.1056/NEJMoa2202106
32. Lu R, Zhang Y-G, Xia Y, Zhang J, Kaser A, Blumberg R, et al. Paneth cell alertness to pathogens maintained by vitamin D receptors. Gastroenterology. (2020) 160:1269–83. doi: 10.1053/j.gastro.2020.11.015
33. Erdmann J, Wiciński M, Szyperski P, Gajewska S, Ohla J, Słupski M. Vitamin D supplementation and its impact on different types of bone fractures. Nutrients. (2022) 15:103. doi: 10.3390/nu15010103
34. Boughanem H, Ruiz-Limón P, Pilo J, Lisbona-Montañez JM, Tinahones FJ, Indias IM, et al. Linking serum vitamin D levels with gut microbiota after 1-year lifestyle intervention with Mediterranean diet in patients with obesity and metabolic syndrome: a nested cross-sectional and prospective study. Gut Microbes. (2023) 15:2249150. doi: 10.1080/19490976.2023.2249150
35. Hajhashemy Z, Shahdadian F, Moslemi E, Mirenayat FS, Saneei P. Serum vitamin D levels in relation to metabolic syndrome: a systematic review and dose-response meta-analysis of epidemiologic studies. Obesity Reviews. (2021) 22:e13223. doi: 10.1111/obr.13223
36. Rouhani P, Hajhashemy Z, Saneei P. Circulating serum vitamin D levels in relation to metabolic syndrome in children: A systematic review and dose-response meta-analysis of epidemiologic studies. Obes Rev. (2021) 22:e13314. doi: 10.1111/obr.13314
37. Akbarbaglu L, Nozari Mirarkolaei E, Hotelchi M, Khonakdar-Tarsi A, Ghanbari M, Mohamadi P. Evaluation of the relationship between vitamin d deficiency and atherogenic factors in diabetic patients with metabolic syndrome. Tabari Biomed Student Res J. (2021) 3:1–8. doi: 10.18502/tbsrj.v3i4.7794
38. Shulhai A-M, Pavlyshyn H, Oleksandra S, Furdela V. The association between vitamin D deficiency and metabolic syndrome in Ukrainian adolescents with overweight and obesity. Ann Pediatr Endocrinol Metabol. (2021) 27:113–20. doi: 10.6065/apem.2142158.079
39. Brosolo G, Da Porto A, Bulfone L, Scandolin L, Vacca A, Bertin N, et al. Vitamin D deficiency is associated with glycometabolic changes in nondiabetic patients with arterial hypertension. Nutrients. (2022) 14:311. doi: 10.3390/nu14020311
40. Ghaseminejad-Raeini A, Ghaderi A, Sharafi A, Nematollahi-Sani B, Moossavi M, Derakhshani A, et al. Immunomodulatory actions of vitamin D in various immune-related disorders: a comprehensive review. Front Immunol. (2023) 14:950465. doi: 10.3389/fimmu.2023.950465
41. Silveira EA. Cardoso CKd, Moura LdNE, Rodrigues APD, de Oliveira C. Serum and dietary vitamin D in individuals with class II and III obesity: prevalence and association with metabolic syndrome. Nutrients. (2021) 13:2138. doi: 10.3390/nu13072138
42. Melguizo-Rodríguez L, Costela-Ruiz VJ, García-Recio E, De Luna-Bertos E, Ruiz C, Illescas-Montes R. Role of vitamin D in the metabolic syndrome. Nutrients. (2021) 13:830. doi: 10.3390/nu13030830
43. Bima A, Eldakhakhny B, Nuwaylati D, Alnami A, Ajabnoor M, Elsamanoudy A. The interplay of vitamin D deficiency and cellular senescence in the pathogenesis of obesity-related co-morbidities. Nutrients. (2021) 13:4127. doi: 10.3390/nu13114127
44. Contreras-Bolívar V, García-Fontana B, García-Fontana C, Muñoz-Torres M. Mechanisms involved in the relationship between vitamin D and insulin resistance: impact on clinical practice. Nutrients. (2021) 13:3491. doi: 10.3390/nu13103491
45. Argano C, Mirarchi L, Amodeo S, Orlando V, Torres A, Corrao S. The role of vitamin D and its molecular bases in insulin resistance, diabetes, metabolic syndrome, and cardiovascular disease: state of the art. Int J Mol Sci. (2023) 24:15485. doi: 10.3390/ijms242015485
46. Messaritakis I, Koulouridi A, Sfakianaki M, Vogiatzoglou K, Gouvas N, Tsiaousis I, et al. Microbial translocation, toll-like and vitamin D receptor polymorphisms in blood and risk of recurrence in stage III colorectal cancer. J Clin Oncol. (2022) 40:3531–3531. doi: 10.1200/JCO.2022.40.16_suppl.3531
47. Chen YC, Li WC, Ke PH, Chen IC, Yu W, Huang HY, et al. Association between metabolic body composition status and vitamin D deficiency: a cross-sectional study. Front Nutr. (2022) 9:940183. doi: 10.3389/fnut.2022.940183
48. Alzaim M, Al-Daghri NM, Sabico S, Fouda MA, Al-Musharaf S, Khattak MNK, et al. The association between FokI vitamin D receptor polymorphisms with metabolic syndrome among pregnant arab women. Front Endocrinol. (2022) 13:844472. doi: 10.3389/fendo.2022.844472
49. Nakashima M, Mitani, T. 25-Hydroxyvitamin D increases insulin-stimulated glucose uptake by enhancing adipocyte differentiation. J Nutr Sci Vitaminol. (2022) 68:420. doi: 10.3177/jnsv.68.420
50. Lin SY, Wang YY, Chang CY, Wu CC, Chen WY, Liao SL, et al. TNF-α receptor inhibitor alleviates metabolic and inflammatory changes in a rat model of ischemic stroke. Antioxidants. (2021) 10:851. doi: 10.3390/antiox10060851
51. Huang T, Song J, Gao J, Cheng J, Xie H, Zhang L, et al. Adipocyte-derived kynurenine promotes obesity and insulin resistance by activating the AhR/STAT3/IL-6 signaling. Nat Commun. (2022) 13:3489. doi: 10.1038/s41467-022-31126-5
52. Das A, Jawla N, Meena V, Gopinath SD, Arimbasseri GA. Lack of vitamin D signalling shifts skeletal muscles towards oxidative metabolism. J Cachexia Sarcopenia Muscle. (2024) 15:67–80. doi: 10.1002/jcsm.13378
53. Yeh CL, Wu JM, Chen KY, Wu MH, Yang PJ, Lee PC, et al. Effects of different routes and forms of vitamin D administration on CD4+ T cell homeostasis and renin-angiotensin system-associated lung injury in obese mice complicated with polymicrobial sepsis. Biomed Pharmacother. (2022) 156:113961. doi: 10.1016/j.biopha.2022.113961
54. Sun W, Duan X, Chen H, Zhang L, Sun H. Adipogenic activity of 2-ethylhexyl diphenyl phosphate via peroxisome proliferator-activated receptor γ pathway. Sci Total Environ. (2020) 711:134810. doi: 10.1016/j.scitotenv.2019.134810
55. Chauss D, Freiwald T, McGregor R, Yan B, Wang L, Nova-Lamperti E, et al. Autocrine vitamin D signaling switches off pro-inflammatory programs of TH1 cells. Nat Immunol. (2022) 23:62–74. doi: 10.1038/s41590-021-01080-3
56. Lu Y, Guan T, Xu S, Chen Y-E, Shen Q, Zhu S, et al. Asperuloside inhibited epithelial-mesenchymal transition in colitis associated cancer via activation of vitamin D receptor. Phytomedicine. (2022) 101:154070. doi: 10.1016/j.phymed.2022.154070
57. Al-Hassan S, Attia H, Alomar H, Arafa M, Ali RA. The inhibitory mechanisms of losartan and vitamin D on amiodarone-induced lung inflammation in rats: role of mitogen-activated protein kinases/activator protein-1. J Biochem Mol Toxic. (2021) 35:e22923. doi: 10.1002/jbt.22923
58. Suo Z, Liu Y, Li Y, Xu C, Liu Y, Gao M, et al. Calcitriol inhibits COX-1 and COX-2 expressions of renal vasculature in hypertension: reactive oxygen species involved? Clin Exp Hypertens. (2021) 43:1817473. doi: 10.1080/10641963.2020.1817473
59. Xiong Y, Zhou F, Liu Y, Yi Z, Wang X, Wu Y, et al. 1α,25-Dihydroxyvitamin promotes angiogenesis by alleviating AGEs-induced autophagy. Arch Biochem Biophys. (2021) 712:109041. doi: 10.1016/j.abb.2021.109041
60. Moslemi E, Musazadeh V, Kavyani Z, Naghsh N, Shoura SMS, Dehghan, et al. Efficacy of vitamin D supplementation as an adjunct therapy for improving inflammatory and oxidative stress biomarkers: an umbrella meta-analysis. Pharmacol Res. (2022) 186:106484. doi: 10.1016/j.phrs.2022.106484
61. Wang J, Fan J, Yang Y, Moazzen S, Chen D, Sun L, et al. Vitamin D status and risk of all-cause and cause-specific mortality in osteoarthritis patients: results from NHANES III and NHANES 2001–2018. Nutrients. (2022) 14:4629. doi: 10.3390/nu14214629
62. Upadhyay PK, Thakur N, Vishwakarma VK, Srivastav RK, Ansari TM. Role of vitamin D in management of diabetes and unresolved cardiovascular diseases. Curr Diabetes Rev. (2024) 20:128–35. doi: 10.2174/1573399820666230901151019
63. Verdoia M, Daffara V, Pergolini P, Rolla R, Marino P, Bellomo G, et al. Vitamin D Binding Protein rs7041 polymorphism and high-residual platelet reactivity in patients receiving dual antiplatelet therapy with clopidogrel or ticagrelor. Vasc Pharmacol. (2017) 93:42–7. doi: 10.1016/j.vph.2017.04.001
64. Rebelos E, Tentolouris N, Jude E. The role of vitamin D in health and disease: a narrative review on the mechanisms linking vitamin d with disease and the effects of supplementation. Drugs. (2023) 83:665–85. doi: 10.1007/s40265-023-01875-8
65. Jiang H, Chanpaisaeng K, Christakos S, Fleet JC. Intestinal Vitamin D Receptor is dispensable for maintaining adult bone mass in mice with adequate calcium intake. Endocrinology. (2023) 164:51. doi: 10.1210/endocr/bqad051
66. Hasan M, Oster M, Reyer H, Ponsuksili S, Murani E, Wolf P, et al. Tissue-wide expression of genes related to vitamin D metabolism and FGF23 signaling following variable phosphorus intake in pigs. Metabolites. (2022) 12:729. doi: 10.3390/metabo12080729
67. Wan Z, Guo J, Pan A, Chen C, Liu L, Liu G. Association of serum 25-Hydroxyvitamin D concentrations with all-cause and cause-specific mortality among individuals with diabetes. Diabetes Care. (2020) 44:350–7. doi: 10.2337/dc20-1485
68. Qi KJ, Zhao ZT, Zhang W, Yang F. The impacts of vitamin D supplementation in adults with metabolic syndrome: A systematic review and meta-analysis of randomized controlled trials. Front Pharmacol. (2022) 13:1033026. doi: 10.3389/fphar.2022.1033026
69. Marziou A, Aubert B, Couturier C, Astier J, Philouze C, Obert P, et al. Combined beneficial effect of voluntary physical exercise and vitamin D supplementation in diet-induced obese C57BL/6J Mice. Med Sci Sport Exer. (2021) 53:2664. doi: 10.1249/MSS.0000000000002664
70. Mendes AKB, Sulis PM, Cavalari FC, Padilla DPR, Aragón M, Gaspar JM, et al. 1α,25-(OH)2 vitamin D3 prevents insulin resistance and regulates coordinated exocytosis and insulin secretion. J Nutr Biochem. (2021) 99:108864. doi: 10.1016/j.jnutbio.2021.108864
71. Valle M, Mitchell PL, Pilon G, Varin T, Hénault L, Rolin J, et al. Salmon peptides limit obesity-associated metabolic disorders by modulating a gut-liver axis in vitamin D-deficient mice. Obesity. (2021) 29:23244. doi: 10.1002/oby.23244
72. Pellegrinelli V, Rodriguez-Cuenca S, Rouault C, Figueroa-Juarez E, Schilbert H, Virtue S, et al. Dysregulation of macrophage PEPD in obesity determines adipose tissue fibro-inflammation and insulin resistance. Nature Metabolism. (2022) 4:476–94. doi: 10.1038/s42255-022-00561-5
73. Jayaraman S, Devarajan N, Rajagopal P, Babu S, Ganesan SK, Veeraraghavan VP, et al. β-Sitosterol circumvents obesity induced inflammation and insulin resistance by down-regulating IKKβ/NF-κB and JNK signaling pathway in adipocytes of type 2 diabetic rats. Molecules. (2021) 26:2101. doi: 10.3390/molecules26072101
74. Ding C, Wilding JP, Bing C. 1,25-dihydroxyvitamin D3 protects against macrophage-induced activation of NFκB and MAPK signalling and chemokine release in human adipocytes. PLoS ONE. (2013) 8:e61707. doi: 10.1371/journal.pone.0061707
75. Muñoz-Garach A, García-Fontana B, Muñoz-Torres M. Vitamin D status, calcium intake and risk of developing type 2 diabetes: an unresolved issue. Nutrients. (2019) 11:642. doi: 10.3390/nu11030642
76. Zhu L, Li S, Zhong L, Xu S, Zhu H. Optimal vitamin D supplement dosage for improving insulin resistance in children and adolescents with overweight/obesity: a systematic review and network meta-analysis. Eur J Nutr. (2024) 63:763–75. doi: 10.1007/s00394-023-03301-x
77. Zhao Y, Qin R. Vitamin D3 affects browning of white adipocytes by regulating autophagy via PI3K/Akt/mTOR/p53 signaling in vitro and in vivo. Apoptosis. (2022) 27:992–1003. doi: 10.1007/s10495-022-01765-6
78. Crespo-Masip M, Perez-Gomez A, Garcia-Carrasco A, Jover R, Guzmán C, Dolcet X, et al. Elimination of vitamin D signaling causes increased mortality in a model of overactivation of the insulin receptor: role of lipid metabolism. Nutrients. (2022) 14:1516. doi: 10.3390/nu14071516
79. Lu S, Cao Z. Interplay between vitamin D and adipose tissue: implications for adipogenesis and adipose tissue function. Nutrients. (2023) 15:4832. doi: 10.3390/nu15224832
80. Wallbaum P, Rohde S, Ehlers L, Lange F, Hohn A, Bergner C, et al. Antifibrogenic effects of vitamin D derivatives on mouse pancreatic stellate cells. World J Gastroentero. (2018) 24:170–8. doi: 10.3748/wjg.v24.i2.170
81. Wong KE, Kong J, Zhang W, Szeto FL, Ye H, Deb DK, et al. Targeted expression of human vitamin D receptor in adipocytes decreases energy expenditure and induces obesity in mice. J Biol Chem. (2011) 289:257568. doi: 10.1074/jbc.M111.257568
82. Marino M, Venturi S, Del Bo' C, Møller P, Riso P, Porrini M. Vitamin D counteracts lipid accumulation, augments free fatty acid-induced ABCA1 and CPT-1A expression while reducing CD36 and C/EBPβ protein levels in monocyte-derived macrophages. Biomedicines. (2022) 10:775. doi: 10.3390/biomedicines10040775
83. Xu D, Gao HJ, Lu CY, Tian HM, Yu, XJ. Vitamin D inhibits bone loss in mice with thyrotoxicosis by activating the OPG/RANKL and Wnt/β-catenin signaling pathways. Front Endocrinol. (2022) 13:1066089. doi: 10.3389/fendo.2022.1066089
84. Katayama Y. Vitamin D receptor: A critical regulator of inter-organ communication between skeletal and hematopoietic systems. J Steroid Biochem Mol Biol. (2019) 190:281–3. doi: 10.1016/j.jsbmb.2019.02.001
85. Biyong EF, Tremblay C, Leclerc M, Caron V, Alfos S, Helbling JC, et al. Role of Retinoid X Receptors (RXRs) and dietary vitamin A in Alzheimer's disease: evidence from clinicopathological and preclinical studies. Neurobiol Dis. (2021) 161:105542. doi: 10.1016/j.nbd.2021.105542
86. Meza-Meza MR, Ruiz-Ballesteros AI, de la Cruz-Mosso U. Functional effects of vitamin D: from nutrient to immunomodulator. Crit Rev Food Sci. (2022) 62:3042–3062. doi: 10.1080/10408398.2020.1862753
87. Rosenblatt J, Bissonnette A, Wu Z, Vasir B, Zarwan C, Keefe W, et al. Immunomodulatory effects of vitamin d: implications for the prevention and treatment of graft versus host disease. Blood. (2008) 112:1251–1251. doi: 10.1182/blood.V112.11.1251.1251
88. O'Kelly J, Hisatake J, Hisatake Y, Bishop J, Norman A, Koeffler HP, et al. Normal myelopoiesis but abnormal T lymphocyte responses in vitamin D receptor knockout mice. J Clin Invest. (2002) 109:1091–9. doi: 10.1172/JCI0212392
89. Nata N, Siricheepchaiyan W, Supasyndh O, Satirapoj B. Efficacy of high versus conventional dose of ergocalciferol supplementation on serum 25-hydroxyvitamin D and interleukin-6 levels among hemodialysis patients with vitamin D deficiency: a multicenter, randomized, controlled study. Ther Apher Dial. (2022) 26:378–86. doi: 10.1111/1744-9987.13722
90. Mukvich O, Vdovina N, Omelchenko L, Ludvik T, Strizhak S. Personalized choice of dosage regimens and duration of vitamin D use in juvenile idiopathic arthritis. MPU. (2022) 5:69–75. doi: 10.15574/SP.2022.125.69
91. Yang H, Zhang H, Li Y, Xiang L, Liu J. BCG stimulation promotes dendritic cell proliferation and expression of VDR and CYP27B1 in vitamin D-deficient mice. Mol Med Rep. (2019) 20:5265–71. doi: 10.3892/mmr.2019.10780
92. Lopez DV, Al-Jaberi FAH, Woetmann A, Ødum N, Bonefeld CM, Kongsbak-Wismann M, et al. Macrophages control the bioavailability of vitamin D and vitamin D-Regulated T cell responses. Front Immunol. (2021) 12:722806. doi: 10.3389/fimmu.2021.722806
93. Kaufmann M, Schlingmann KP, Berezin L, Molin A, Sheftel J, Vig M, et al. Differential diagnosis of vitamin D-related hypercalcemia using serum vitamin D metabolite profiling. J Bone Miner Res. (2021) 36:4306. doi: 10.1002/jbmr.4306
94. Lopez DV, Al-Jaberi FAH, Damas ND, Weinert BT, Pus U, Torres-Rusillo S, et al. Vitamin D inhibits IL-22 production through a repressive vitamin d response element in the il22 promoter. Front Immunol. (2021) 12:715059. doi: 10.3389/fimmu.2021.715059
95. Arnson Y, Amital H, Shoenfeld, Y. Vitamin D and autoimmunity: new aetiological and therapeutic considerations. Ann Rheum Dis. (2007) 66:69831. doi: 10.1136/ard.2007.069831
96. Ribeiro VR, Romao-Veiga M, Nunes PR, de Oliveira LRC, Romagnoli GG, Peracoli JC, et al. Immunomodulatory effect of vitamin D on the STATs and transcription factors of CD4+ T cell subsets in pregnant women with preeclampsia. Clin Immunol. (2022) 234:108917. doi: 10.1016/j.clim.2021.108917
97. Mariz HA, Sato EI, Cardoso PRG, Gonçalves R, Duarte ALBP, Melo Rego de, et al. Vitamin D Presented In vitro immunomodulatory property on T lymphocyte-related cytokines in systemic lupus erythematosus. Inflammation. (2023) 46:730–8. doi: 10.1007/s10753-022-01768-0
98. Chew C, Reynolds JA, Lertratanakul A, Wu P, Urowitz M, Gladman DD, et al. Lower vitamin D is associated with metabolic syndrome and insulin resistance in systemic lupus: data from an international inception cohort. Rheumatology. (2021) 60:4737–47. doi: 10.1093/rheumatology/keab090
99. Li WX, Qin XH, Poon CC, Wong MS, Feng R, Wang J, et al. Vitamin D/Vitamin D receptor signaling attenuates skeletal muscle atrophy by SD3uppressing renin-angiotensin system. J Bone Miner Res. (2022) 37:121–36. doi: 10.1002/jbmr.4441
100. Wong MS, Delansorne R, Man RY, Svenningsen P, Vanhoutte, PM. Chronic treatment with vitamin D lowers arterial blood pressure and reduces endothelium-dependent contractions in the aorta of the spontaneously hypertensive rat. Am J Physiol-Heart C. (2010) 299:2010. doi: 10.1152/ajpheart.00288.2010
101. Bouillon R, Manousaki D, Rosen C, Trajanoska K, Rivadeneira F, Richards JB. The health effects of vitamin D supplementation: evidence from human studies. Nat Rev Endocrinol. (2022) 18:96–110. doi: 10.1038/s41574-021-00593-z
102. Della Nera G, Sabatino L, Gaggini M, Gorini F, Vassalle, C. Vitamin D determinants, status, and antioxidant/anti-inflammatory-related effects in cardiovascular risk and disease: not the last word in the controversy. Antioxidants (Basel). (2023) 12:948. doi: 10.3390/antiox12040948
103. de la Guía-Galipienso F, Martínez-Ferran M, Vallecillo N, Lavie CJ, Sanchis-Gomar F, Pareja-Galeano H. Vitamin D and cardiovascular health. Clin Nutr. (2021) 40:2946–2957. doi: 10.1016/j.clnu.2020.12.025
104. Moussa S, Khaled H, ElShishtawy H, Elnaggar H. Subclinical atherosclerosis in psoriatic patients: relation with vitamin D. Qjm-Int J Med. (2021) 114:11. doi: 10.1093/qjmed/hcab116.011
105. Janssens W, Lehouck A, Carremans C, Bouillon R, Mathieu C, Decramer M, et al. Vitamin D beyond bones in chronic obstructive pulmonary disease: time to act. Am J Resp Crit Care. (2009) 179:630–6. doi: 10.1164/rccm.200810-1576PP
106. Nicoll R. Cardiovascular calcification and bone: a comparison of the effects of dietary and serum calcium, phosphorous, magnesium and vitamin D. ICFJ. (2015) 1:83. doi: 10.17987/icfj.v2i1.83
107. Chin KY, Wong SK, Ekeuku SO, Pang, KL. Relationship between metabolic syndrome and bone health - an evaluation of epidemiological studies and mechanisms involved. Diab Metab Syndr Obes. (2020) 13:5560. doi: 10.2147/DMSO.S275560
108. Bagherzadeh M, Sajjadi-Jazi SM, Sharifi F, Ebrahimpur M, Amininezhad F, Ostovar A, et al. Effects of metabolic syndrome on bone health in older adults: the Bushehr Elderly Health (BEH) program. Osteopor Int. (2020) 31:1975–84. doi: 10.1007/s00198-020-05455-4
109. Christodoulou M, Aspray TJ, Piec I, Washbourne C, Tang JCY, Fraser WD, et al. Early renal impairment affects hormonal regulators of calcium and bone metabolism and Wnt signalling and the response to vitamin D supplementation in healthy older adults. J Steroid Biochem. (2023) 229:106267. doi: 10.1016/j.jsbmb.2023.106267
110. Buskermolen J, van der Meijden K, Furrer R, Mons DJ, van Essen HW, Heijboer AC, et al. Effects of different training modalities on phosphate homeostasis and local vitamin D metabolism in rat bone. PeerJ. (2019) 7:e6184. doi: 10.7717/peerj.6184
111. Layber M, Goés A, Villarim C, Caldas D, Araújo-Neto I, Silva L, et al. Vitamin D in the prevention and treatment of periodontal diseases: an integrative review. Res Soc Dev. (2021) 10:13. doi: 10.33448/rsd-v10i13.20738
112. Jastrzebska J, Skalska M, Radzimiński Ł, López-Sánchez GF, Weiss K, Hill L, et al. Changes of 25(OH)D concentration, bone resorption markers and physical performance as an effect of sun exposure, supplementation of vitamin D and lockdown among young soccer players during a one-year training season. Nutrients. (2022) 14:521. doi: 10.3390/nu14030521
113. Khalique A, Mohammed AK, Al-Khadran NM, Gharaibeh MA, Abu-Gharbieh E, El-Huneidi W, et al. Reduced retinoic acid receptor beta (Rarβ) affects pancreatic β-Cell physiology. Biology (Basel). (2022) 11:1072. doi: 10.3390/biology11071072
114. Beggs MR, Appel I, Svenningsen P, Skjødt K, Alexander RT, Dimke H, et al. Expression of transcellular and paracellular calcium and magnesium transport proteins in renal and intestinal epithelia during lactation. Am J Physiol-Renal. (2017) 313:F629–40. doi: 10.1152/ajprenal.00680.2016
115. Tkach S, Pankiv V, Pankiv I. Modern views on the metabolism and biological effects of vitamin D. MìŽnarodnij endokrinologìčnij Žurnal. (2022) 18:1156. doi: 10.22141/2224-0721.18.2.2022.1156
116. Zhao Y, Mei G, Zhou F, Kong B, Chen L, Chen H, et al. Vitamin D decreases pancreatic iron overload in type 2 diabetes through the NF-κB-DMT1 pathway. J Nutr Biochem. (2022) 99:108870. doi: 10.1016/j.jnutbio.2021.108870
117. Wu J, Atkins A, Downes M, Wei Z. Vitamin D in diabetes: uncovering the sunshine hormone's role in glucose metabolism and beyond. Nutrients. (2023) 15:1997. doi: 10.3390/nu15081997
118. Wang H, Yu X, Liu D, Qiao Y, Huo J, Pan S, et al. VDR Activation attenuates renal tubular epithelial cell ferroptosis by regulating Nrf2/HO-1 signaling pathway in diabetic nephropathy. Adv Sci (Weinh). (2024) 11:e2305563. doi: 10.1002/advs.202305563
119. Li A, Yi B, Han H, Yang S, Hu Z, Zheng L, et al. Vitamin D-VDR (vitamin D receptor) regulates defective autophagy in renal tubular epithelial cell in streptozotocin-induced diabetic mice via the AMPK pathway. Autophagy. (2022) 18:877–90. doi: 10.1080/15548627.2021.1962681
120. Kim HT, Lee SH, Lee JK, Chung SW. Influence of Vitamin D deficiency on the expression of genes and proteins in patients with medium rotator cuff tears. Am J Sport Med. (2023) 51:2650–8. doi: 10.1177/03635465231184392
121. Zhang QW, Wang Y, Tong ZY, Li CP, He LP. Vitamin D may play a vital role in alleviating type 2 diabetes mellitus by modulating the ferroptosis signaling pathway. Horm Metab Res. (2023) 56:193–6. doi: 10.1055/a-2122-5701
122. Morró M, Vilà L, Franckhauser S, Mallol C, Elias G, Ferré T, et al. Vitamin D receptor overexpression in β-cells ameliorates diabetes in mice. Diabetes. (2020) 69:757. doi: 10.2337/db19-0757
123. Dickerson RN, Turner SC, Holmes WL, Van Matre ET, Swanson JM, Byerly S, et al. Reduction in hypercalcemia following readjustment of target serum 25-Hydroxy vitamin d concentration during cholecalciferol therapy in vitamin d-deficient critically ill patients. Nutrients. (2022) 14:1650. doi: 10.3390/nu14081650
124. Pittas AG, Dawson-Hughes B, Sheehan P, Ware JH, Knowler WC, Aroda VR, et al. Vitamin D supplementation and prevention of type 2 diabetes. New Engl J Med. (2019) 381:906. doi: 10.1056/NEJMoa1900906
125. Chrysostomou S. Vitamin D daily short-term supplementation does not affect glycemic outcomes of patients with type 2 diabetes. Int J Vitam Nutr Res. (2016) 86:169–83. doi: 10.1024/0300-9831/a000239
126. Tatarnikova I, Shpagina L, Gerasimenko, O. Vitamin D plasma concentration and VDR gene polymorphisms in patients with non-dipper hypertension. Eur Heart J. (2020) 41:2793. doi: 10.1093/ehjci/ehaa946.2793
127. Barrea L, Frias-Toral E, Pugliese G, Garcia-Velasquez E, Savastano S, Colao A, et al. Vitamin D in obesity and obesity-related diseases: an overview. Minerva Endocrinol. (2020) 46:177–92. doi: 10.23736/S2724-6507.20.03299-X
128. Salehpour A, Shidfar F, Hedayati M, Farshad AA, Tehrani AN, Mohammadi S. Molecular mechanisms of vitamin D plus Bisphenol A effects on adipogenesis in human adipose-derived mesenchymal stem cells. Diabetol Metabolic Syndr. (2021) 13:41. doi: 10.1186/s13098-021-00661-4
129. Mendoza A, Takemoto Y, Cruzado KT, Masoud SS, Nagata A, Tantipanjaporn A, et al. Controlled lipid β-oxidation and carnitine biosynthesis by a vitamin D metabolite. Cell Chem Biol. (2022) 29:660–669.e12. doi: 10.1016/j.chembiol.2021.08.008
130. Liu J, Wang Y, Lin L. Small molecules for fat combustion: targeting obesity. Acta Pharm Sin B. (2019) 9:220–36. doi: 10.1016/j.apsb.2018.09.007
131. Mutt SJ, Raza GS, Mäkinen MJ, Keinänen-Kiukaanniemi S, Järvelin M-R, Herzig K-H. Vitamin D Deficiency induces insulin resistance and re-supplementation attenuates hepatic glucose output via the PI3K-AKT-FOXO1 mediated pathway. Molec Nutr Food Res. (2020) 64:e1900728. doi: 10.1002/mnfr.201900728
132. Barminko J, Reinholt BM, Emmanuelli A, Lejeune AN, Baron MH. Stimulation of the proliferation of mouse and human definitive erythroid progenitors by activation of the vitamin D receptor transcription factor. Blood. (2018) 132:1029–1029. doi: 10.1182/blood-2018-99-113846
133. Wen J, Hadden MK. Affinity-based protein profiling identifies vitamin as a heat shock protein 70 antagonist that regulates hedgehog transduction in murine basal cell carcinoma. Eur J Med Chem. (2021) 228:114005. doi: 10.1016/j.ejmech.2021.114005
134. Harahap IA, Landrier J, Suliburska J. Interrelationship between vitamin D and calcium in obesity and its comorbid conditions. Nutrients. (2022) 14:3187. doi: 10.3390/nu14153187
135. Guevara J, Velilia A, Tiu S, Ti A, Tinio K, Torres A, et al. Efficacy of vitamin D alone or in combination with weight-reduction programs in weight loss among adults with above-normal BMI: a systematic review and meta-analysis. Eur Heart J. (2021) 42:2603. doi: 10.1093/eurheartj/ehab724.2603
136. Freeburg SH, Shwartz A, Kemény LV, Smith CJ, Weeks O, Miller BM, et al. Hepatocyte vitamin D receptor functions as a nutrient sensor that regulates energy storage and tissue growth in zebrafish. Cell Rep. (2024) 43:114393. doi: 10.1016/j.celrep.2024.114393
137. Kouchaki E, Afarini M, Abolhassani J, Mirhosseini N, Bahmani F, Masoud SA, et al. An expression of concern from the journal of nutrition's editorial office about: high-dose ω-3 fatty acid plus vitamin D3 supplementation affects clinical symptoms and metabolic status of patients with multiple sclerosis: a randomized controlled clinical trial. J Nutr. (2020) 150:3044. doi: 10.1093/jn/nxaa342
138. Abraham ME, Robison CI, Kim WK, Regmi P, Karcher DM. n-3 essential fatty acid and vitamin D supplementation improve skeletal health in laying hens. Poult Sci. (2023) 102:103089. doi: 10.1016/j.psj.2023.103089
139. Castillo-Castellanos F, Ramírez L, Lomelí H. zmiz1a zebrafish mutants have defective erythropoiesis, altered expression of autophagy genes, and a deficient response to vitamin D. Life Sci. (2021) 284:119900. doi: 10.1016/j.lfs.2021.119900
140. Liao X, Lan Y, Shao R, Liu J, Liang S, Yin Z, et al. Vitamin D enhances neutrophil generation and function in zebrafish (Danio rerio). J Innate Immun. (2021) 14:229–42. doi: 10.1159/000519183
141. Moosic K, Olson K, Feith DJ, Loughran Jr TP. Anti-inflammatory response to anti-folate and vitamin D analog combination in large granular lymphocyte leukemia. Blood. (2023) 142:4194. doi: 10.1182/blood-2023-190063
142. Kagoya R, Kondo K, Kishimoto-Urata M, Shimizu Y, Kikuta S, Yamasoba T, et al. murine model of eosinophilic chronic rhinosinusitis using the topical application of a vitamin D3 analog. Allergy. (2020) 76:1432–42. doi: 10.1111/all.14627
143. Kumar S, Nanduri R, Bhagyaraj E, Kalra R, Ahuja N, Chacko AP, et al. Vitamin D3-VDR-PTPN6 axis mediated autophagy contributes to the inhibition of macrophage foam cell formation. Autophagy. (2020) 17:2273–89. doi: 10.1080/15548627.2020.1822088
144. Cui P, Lu W, Wang J, Wang F, Zhang X, Hou X, et al. Microglia/macrophages require vitamin D signaling to restrain neuroinflammation and brain injury in a murine ischemic stroke model. J Neuroinflam. (2023) 20:63. doi: 10.1186/s12974-023-02705-0
145. Gao H, Zhou H, Zhang Z, Gao J, Li J, Li X. Vitamin D3 alleviates inflammation in ulcerative colitis by activating the VDR-NLRP6 signaling pathway. Front Immunol. (2023) 14:1135930. doi: 10.3389/fimmu.2023.1135930
146. Tourkochristou E, Mouzaki A, Triantos C. Gene polymorphisms and biological effects of vitamin D receptor on nonalcoholic fatty liver disease development and progression. Int J Mol Sci. (2023) 24:8288. doi: 10.3390/ijms24098288
147. Jaroslawska J, Carlberg C. In vivo regulation of signal transduction pathways by vitamin d stabilizes homeostasis of human immune cells and counteracts molecular stress. Int J Mol Sci. (2023) 24:14632. doi: 10.3390/ijms241914632
148. Long W, Fatehi M, Soni S, Panigrahi R, Philippaert K, Yu Y, et al. Vitamin D is an endogenous partial agonist of the transient receptor potential vanilloid 1 channel. J Physiol-London. (2020) 598:4321–38. doi: 10.1113/JP279961
149. Haddad Kashani H, Seyed Hosseini E, Nikzad H, Soleimani A, Soleimani M, Tamadon MR, et al. The effects of vitamin D supplementation on signaling pathway of inflammation and oxidative stress in diabetic hemodialysis: a randomized, double-blind, placebo-controlled trial. Front Pharmacol. (2018) 9:50. doi: 10.3389/fphar.2018.00050
150. Krishnan AV, Feldman D. Mechanisms of the anti-cancer and anti-inflammatory actions of vitamin D. Annu Rev Pharmacol Toxicol. (2011) 51:311–36. doi: 10.1146/annurev-pharmtox-010510-100611
151. Chen J, Tang Z, Slominski AT, Li W, Żmijewski MA, Liu Y, et al. Vitamin D and its analogs as anticancer and anti-inflammatory agents. Eur J Med Chem. (2020) 207:112738. doi: 10.1016/j.ejmech.2020.112738
152. Derbyshire EJ, Calder PC. Respiratory tract infections and antibiotic resistance: a protective role for vitamin D? Front Nutr. (2021) 8:652469. doi: 10.3389/fnut.2021.652469
153. Yang S, Wang C, Ruan C, Chen M, Cao R, Sheng L, et al. Novel insights into the cardioprotective effects of calcitriol in myocardial infarction. Cells. (2022) 11:1676. doi: 10.3390/cells11101676
154. Verdoia M, Gioscia R, Viglione F, Rolla R, Viola O, Brancati, et al. Solda', P L, Marcolongo, M, De Luca, G, Vitamin D deficiency predicts the no-reflow phenomenon in STEMI patients undergoing primary percutaneous coronary intervention. Eur Heart J. (2021) 42:2155. doi: 10.1093/eurheartj/ehab724.2155
155. Caus M, Alonso-Montes C, Fernandez-Martin JL, Marti-Antonio M, Bozic M, Valdivielso JM. Vitamin D receptor from VSMCs regulates vascular calcification during CKD: a potential role for miR-145a. Arteriosclerosis Thrombosis Vasc Biol. (2023) 43:1533–48. doi: 10.1161/ATVBAHA.122.318834
156. Rihal V, Khan H, Kaur A, Singh TG. Vitamin D as therapeutic modulator in cerebrovascular diseases: a mechanistic perspectives. Crit Rev Food Sci. (2023) 63:2050349. doi: 10.1080/10408398.2022.2050349
157. Wong MS, Leisegang MS, Kruse C, Vogel J, Schürmann C, Dehne N, et al. Vitamin D promotes vascular regeneration. Circulation. (2014) 130:650. doi: 10.1161/CIRCULATIONAHA.114.010650
158. Cui C, Xu P, Li G, Qiao Y, Han W, Geng C, et al. Vitamin D receptor activation regulates microglia polarization and oxidative stress in spontaneously hypertensive rats and angiotensin II-exposed microglial cells: role of renin-angiotensin system. Redox Biol. (2019) 26:101295. doi: 10.1016/j.redox.2019.101295
159. Shah AK, Dhalla NS, Effectiveness of some vitamins in the prevention of cardiovascular disease: a narrative review. Front Physiol. (2021) 12:729255. doi: 10.3389/fphys.2021.729255
160. Renkema KY, Nijenhuis T, van der Eerden BC, van der Kemp AW, Weinans H, van Leeuwen JP, et al. Hypervitaminosis D mediates compensatory Ca2+ hyperabsorption in TRPV5 knockout mice. J Am Soc Nephrol. (2005) 16:632. doi: 10.1681/ASN.2005060632
161. Туйзарова И, Свеклина Т, Козлов В, Сардинов P. The role of calcium and vitamin D in arterial hypertension. Patol Fiziol Eksp Ter. (2020) 2:123. doi: 10.25557/0031-2991.2020.02.117-123
162. Kashefiolasl S, Leisegang MS, Helfinger V, Schürmann C, Pflüger-Müller B, Randriamboavonjy V, et al. Vitamin D-a new perspective in treatment of cerebral vasospasm. Neurosurgery. (2021) 88:484. doi: 10.1093/neuros/nyaa484
163. Ahmed MA. Impact of vitamin D 3 on cardiovascular responses to glucocorticoid excess. J Physiol Biochem. (2013) 69:267–76. doi: 10.1007/s13105-012-0209-4
164. Wu-Wong JR, Nakane M, Ma J, Ruan X, Kroeger PE. VDR-mediated gene expression patterns in resting human coronary artery smooth muscle cells. J Cell Biochem. (2007) 100:1395–405. doi: 10.1002/jcb.21133
165. Dusso AS. Update on the biologic role of the vitamin D endocrine system. Curr Vasc Pharmacol. (2014) 12:26. doi: 10.2174/15701611113119990026
166. Bell D, Zhao Y, McCoy FP, Devine AB, McDermott, BJ. Differential effects of an anti-oxidant intervention on cardiomyocyte expression of adrenomedullin and intermedin and their receptor components in chronic nitric oxide deficiency. Cell Physiol Biochem. (2007) 20:7513. doi: 10.1159/000107513
167. van Driel M, van Leeuwen JPTM. Vitamin D and bone: a story of endocrine and auto/paracrine action in osteoblasts. Nutrients. (2023) 15:480. doi: 10.3390/nu15030480
168. Latic N, Erben RG. Interaction of vitamin D with peptide hormones with emphasis on parathyroid hormone, FGF23, and the renin-angiotensin-aldosterone system. Nutrients. (2022) 14:5186. doi: 10.3390/nu14235186
169. Botticella G, Pizzonia M, Cossu B, Bruno R, Camellino D, Girasole G, et al. POS1105 effects of cholecalciferol and calcifediol in osteoporotic women with secondary hyperparathyroidism due to severe vitamin d deficiency undergoing zoledronic acid treatment: a randomized-controlled trial. Ann Rheumatic Dis. (2021) 80:1–833. doi: 10.1136/annrheumdis-2021-eular.809
170. Marchenkova L, Vasileva V. AB0627 the effect of biologically active food supplement with calcium and vitamin D3 administration on calcium homeostasis and falls incidence in patients with high fracture risk undergoing medical rehabilitation. Ann Rheumatic Dis. (2021) 80:1–1347. doi: 10.1136/annrheumdis-2021-eular.4254
171. Deng J, Yang Y, He J, Xie Z, Luo F, Xu J, et al. Vitamin D receptor activated by vitamin D administration alleviates Mycobacterium tuberculosis-induced bone destruction by inhibiting NFκB-mediated aberrant osteoclastogenesis. FASEB J. (2021) 35:135. doi: 10.1096/fj.202100135R
172. Nowak JI, Olszewska AM, Wierzbicka JM, Gebert M, Bartoszewski R, Żmijewski, et al. VDR and PDIA3 are essential for activation of calcium signaling and membrane response to 1,25(OH)2D3 in squamous cell carcinoma cells. Cells. (2023) 13:11. doi: 10.3390/cells13010011
173. Crescioli C. Vitamin D, exercise, and immune health in athletes: a narrative review. Front Immunol. (2022) 13:954994. doi: 10.3389/fimmu.2022.954994
174. Dey SK, Kumar S, Rani D, Maurya SK, Banerjee P, Verma M, et al. Implications of vitamin D deficiency in systemic inflammation and cardiovascular health. Crit rev food sci. (2023) 5:2224880. doi: 10.1080/10408398.2023.2224880
175. Tamez H, Kalim S, Thadhani, RI. Does vitamin D modulate blood pressure? Curr Opin Nephrol HY. (2013) 22:e32835. doi: 10.1097/MNH.0b013e32835d919b
176. Levin A, Li YC. Vitamin D and its analogues: do they protect against cardiovascular disease in patients with kidney disease? Kidney Int. (2005) 68:1973–81. doi: 10.1111/j.1523-1755.2005.00651.x
177. LeBoff MS, Chou SH, Murata EM, Donlon CM, Cook NR, Mora S, et al. Effects of supplemental vitamin D on bone health outcomes in women and men in the VITamin D and OmegA-3 TriaL (VITAL). J Bone Miner Res. (2020) 35:3958. doi: 10.1002/jbmr.3958
178. Pilarski A, Penn N, Ratnakumar S, Barker RD, Milburn HJ. Variation in vitamin D deficiency among tuberculosis patients by ethnic group and geographical region of birth: evidence from a diverse south London population. Eur Respir J. (2016) 48:1507–10. doi: 10.1183/13993003.00057-2016
179. Białka-Kosiec A, Orszulak D, Gawlik A, Drosdzol-Cop A. The relationship between the level of vitamin D, leptin and FGF23 in girls and young women with polycystic ovary syndrome. Front Endocrinol. (2022) 13:1000261. doi: 10.3389/fendo.2022.1000261
180. Streicher C, Zeitz U, Andrukhova O, Rupprecht A, Pohl E, Larsson TE, et al. Long-term Fgf23 deficiency does not influence aging, glucose homeostasis, or fat metabolism in mice with a nonfunctioning vitamin D receptor. Endocrinology. (2012) 153:1795–805. doi: 10.1210/en.2011-1878
181. Zugmaier G, Jäger R, Grage B, Gottardis MM, Havemann K, Knabbe C, et al. Growth-inhibitory effects of vitamin D analogues and retinoids on human pancreatic cancer cells. Brit J Cancer. (1996) 73:1341–6. doi: 10.1038/bjc.1996.256
182. Shao R, Liao X, Wang W, Lan Y, Zhang H, Du Q, et al. Vitamin D regulates glucose metabolism in zebrafish (Danio rerio) by maintaining intestinal homeostasis. J Nutr Biochem. (2024) 123:109473. doi: 10.1016/j.jnutbio.2023.109473
183. Guarnotta V, Di Gaudio F, Giordano C. Vitamin D deficiency in Cushing's disease: before and after its supplementation. Nutrients. (2022) 14:973. doi: 10.3390/nu14050973
184. Noh K, Chow EC, Quach HP, Groothuis GM, Tirona RG, Pang KS. Significance of the vitamin D receptor on crosstalk with nuclear receptors and regulation of enzymes and transporters. AAPS J. (2022) 24:71. doi: 10.1208/s12248-022-00719-9
185. Young K, Beggs MR, Grimbly C, Alexander RT. Regulation of 1 and 24 hydroxylation of vitamin D metabolites in the proximal tubule. Exp Biol Med. (2022) 247:1103–11. doi: 10.1177/15353702221091982
186. Nakamichi Y, Liu Z, Mori T, He Z, Yasuda H, Takahashi N, et al. The vitamin D receptor in osteoblastic cells but not secreted parathyroid hormone is crucial for soft tissue calcification induced by the proresorptive activity of 1,25(OH)2D3. J Steroid Biochem. (2023) 232:106351. doi: 10.1016/j.jsbmb.2023.106351
187. Abate M, Salini V. Oxidative stress, testosterone, cortisol, and vitamin d: differences in professional soccer players of African and Caucasian origin. Med Princ Pract. (2022) 31:352–8. doi: 10.1159/000525728
188. de Sire A, Gallelli L, Marotta N, Lippi L, Fusco N, Calafiore D, et al. Vitamin D deficiency in women with breast cancer: a correlation with osteoporosis? A machine learning approach with multiple factor analysis. Nutrients. (2022) 14:1586. doi: 10.3390/nu14081586
189. Kushwaha AC, Mohanbhai SJ, Sardoiwala MN, Jaganathan M, Karmakar S, Roy Choudhury S, et al. Nanoemulsified Genistein and Vitamin D mediated epigenetic regulation to inhibit osteoporosis. ACS Biomater Sci Eng. (2022) 8:3810–8. doi: 10.1021/acsbiomaterials.2c00165
190. Al-Bayyari N, Al-Domi H, Zayed F, Hailat R, Eaton, A. Androgens and hirsutism score of overweight women with polycystic ovary syndrome improved after vitamin D treatment: A randomized placebo controlled clinical trial. Clin Nutr. (2021) 40:24. doi: 10.1016/j.clnu.2020.09.024
Keywords: metabolic syndrome, vitamin D, insulin sensitivity, lipid metabolism, inflammation, immune response, therapeutic strategies
Citation: Liang Z, Wang Z, Liu X and He Y (2024) Confronting the global obesity epidemic: investigating the role and underlying mechanisms of vitamin D in metabolic syndrome management. Front. Nutr. 11:1416344. doi: 10.3389/fnut.2024.1416344
Received: 12 April 2024; Accepted: 22 July 2024;
Published: 09 August 2024.
Edited by:
Catherine Frances Hughes, Ulster University, United KingdomReviewed by:
Maharshi Bhaswant, Tohoku University, JapanAyman Zaky Elsamanoudy, Mansoura University, Egypt
Copyright © 2024 Liang, Wang, Liu and He. This is an open-access article distributed under the terms of the Creative Commons Attribution License (CC BY). The use, distribution or reproduction in other forums is permitted, provided the original author(s) and the copyright owner(s) are credited and that the original publication in this journal is cited, in accordance with accepted academic practice. No use, distribution or reproduction is permitted which does not comply with these terms.
*Correspondence: Xueyong Liu, bGl1eHlzamgmI3gwMDA0MDtzai1ob3NwaXRhbC5vcmc=; Yu He, aGV5dSYjeDAwMDQwO2NtdS5lZHUuY24=
†These authors have contributed equally to this work and share first authorship
‡These authors have contributed equally to this work