- 1Neat Science, Chatel-Saint-Denis, Switzerland
- 2School of Molecular Biosciences, College of Medical, Veterinary and Life Sciences, University of Glasgow, Glasgow, United Kingdom
- 3Amway Innovation and Science, Ada, MI, United States
Considering a growing, aging population, the need for interventions to improve the healthspan in aging are tantamount. Diet and nutrition are important determinants of the aging trajectory. Plant-based diets that provide bioactive phytonutrients may contribute to offsetting hallmarks of aging and reducing the risk of chronic disease. Researchers now advocate moving toward a positive model of aging which focuses on the preservation of functional abilities, rather than an emphasis on the absence of disease. This narrative review discusses the modulatory effect of nutrition on aging, with an emphasis on promising phytonutrients, and their potential to influence cellular, organ and functional parameters in aging. The literature is discussed against the backdrop of a recent conceptual framework which describes vitality, intrinsic capacity and expressed capacities in aging. This aims to better elucidate the role of phytonutrients on vitality and intrinsic capacity in aging adults. Such a review contributes to this new scientific perspective—namely—how nutrition might help to preserve functional abilities in aging, rather than purely offsetting the risk of chronic disease.
1 Introduction
By the year 2100, it is projected that a quarter of the world’s population will be aged over 64 (1). Living longer has not necessarily been accompanied by continued good health, therefore while lifespan may have increased, the healthspan has been improving at a much slower pace (2). Healthspan, or healthy aging, is defined as the number of years lived in good physical, cognitive, and emotional health (3).
Diet and lifestyle stand out as pivotal factors in this quest, with nutrition playing a crucial role in modulating multiple cellular aging processes (4–10). Good quality plant-based diets which provide essential nutrients and bioactive phytonutrients, have the potential to impact multiple aging mechanisms, such as, inflammation, metabolism, and cellular repair (10–15). Phytonutrients have been defined as, “Compounds present in and/or derived from plants that confer a health benefit (including metabolites post consumption)” (16). These compounds are found in edible plants and have a variety of important functions including protecting plants from various environmental stressors (17). Phytonutrients should ideally be provided via healthy dietary patterns which emphasize plant foods, or they may also be provided in safe, concentrated forms via plant nutraceuticals. Nutraceuticals can be defined as “A compound or mixture of compounds present in food or food supplements intended to exert a therapeutic effect” (11, 16).
Recently, the preservation of vitality and intrinsic capacity, as opposed to specifically focusing on the presence and absence of disease, has come to the fore in healthy aging research (7). In this narrative review, we present and discuss the scientific evidence around certain dietary phytonutrients and their modulatory effects on aging at the cellular, organ and functional levels. With more than 10,000 potential phytonutrients, this review has selected phytonutrients with varying levels of research ranging from the emerging to the well-studied. We will discuss this broad range of phytonutrients against the backdrop of the conceptual framework on vitality and intrinsic capacity in aging originally proposed by Beard et al. (18) and recently elaborated by a WHO working group (7). In the context of nutrition, the consideration of this framework in a narrative review helps to contextualize and elucidate how certain phytonutrients might influence biological aging across the aforementioned levels. Furthermore, we identify research gaps and future opportunities for phytonutrients to help influence the vitality and intrinsic capacity of aging adults.
1.1 Aging is a multifactorial and multiorgan loss of functional ability
Aging is a multifactorial process, driven by a complex interplay of numerous molecular mechanisms that collectively contribute to the gradual decline in physiological function and increased susceptibility to age-related diseases (19). Understanding the intricacies of aging involves examining both organ or system specific changes and the broader network of interactions among organ systems. For instance, the aging immune system is characterized by immunosenescence, inflammaging, and age-related dysbiosis (20). This progressively leads to a diminishing ability of the body to protect itself, making older adults more susceptible to infections, and less responsive to vaccination (21). In the cardiovascular system, vascular aging results in endothelial dysfunction and impaired vasodilation, placing older adults at a higher risk of cardiovascular disease (CVD). In addition, metabolic dysfunction arising from disrupted nutrient sensing, coupled with low-grade inflammation and oxidative stress, contribute to worsening atherosclerotic plaque, arterial thickness and stiffness further increasing that risk (22). Similar declines are observed in the nervous and musculoskeletal systems with cognitive impairment and sarcopenia as known consequences (23–25).
Aging organs are interconnected, with the biological aging rate in one organ system affecting the aging trajectory of others (26, 27). Consequently, accelerated biological aging in the kidney or the brain, can exert systemic effects on other organ systems through shared pathways, including inflammation, oxidative stress, and hormonal dysregulation (28). This interorgan communication forms a complex network of interactions, leading to the concept of multiorgan aging and therefore comorbidity (29).
1.2 Nutrition as a contributor to healthy aging
The ideal scenario of healthy aging is that lifespan extension is accompanied by a proportional increase in healthspan, resulting in an older population that lives longer, in better health, with a condensed period of morbidity (Figure 1).
Building on this foundation, Figure 2 provides a comprehensive overview of healthspan, detailing its definition, key components, influencing factors, and methods for optimization. Importantly, Figure 2 aims to highlight that the notion of healthspan should recognize that aging is not solely defined by the absence of disease or compression of morbidity, but also encompasses the preservation of intrinsic capacity and vitality (30).
In addition, Figure 2 highlights the notion that diet is central to influencing healthspan (14, 22, 31), and interlinked with other lifestyle and environmental factors, such as physical activity, sleep quality, access to medical care and preventive measures. Adequate nutrition is associated with maintaining intrinsic capacity by supporting bodily functions and cognitive health, and it may also play a vital role in boosting vitality (6, 7, 9). Diet and nutrition are also at the heart of current research areas, including studies on longevity genes, the biological impacts of nutrition and exercise synergies, the effects of senolytics, and the potential benefits of caloric restriction and its mimetics (32, 33). Such research underscores the importance of nutritional intervention strategies aimed at promoting healthy aging and enhancing quality of life.
The concept of intrinsic capacity, introduced by Beard et al. (34) refers to individuals’ physical and mental abilities that enable them to perform daily activities effectively and maintain independence as they age (6, 35). This notion is pivotal in understanding aging, as it shifts the focus from the mere absence of disease to the positive aspects of aging, emphasizing the potential for well-being and functionality into older age. Intrinsic capacity encompasses five domains, including cognitive function, sensory capacity, locomotor function, psychological well-being, and vitality (6). Vitality is described by physical and mental vigor, reflecting an individual’s overall sense of well-being, engagement in life and resilience to challenges associated with aging (7). The longitudinal analysis and validation of intrinsic capacity suggest that it is a robust outcome and applicable across culturally diverse population groups (18, 34). Understanding the complex interplay between nutrition and aging requires a nuanced appreciation of intrinsic capacity and vitality. By nurturing vitality and supporting the various domains of intrinsic capacity, nutrition emerges as a key modulator of aging, offering promising pathways for enhancing quality of life and functional independence among older adults.
Beard’s framework (Figure 3) is useful for this narrative review since it encapsulates the relevant domains of the cells, organs and potential functional outcomes on which nutrients and phytonutrients might act. Hence, the framework will help to contextualize the literature and elucidate findings. Furthermore, narrative reviews have been criticized for not using existing theoretical or conceptual thinking in their framing, discussion, and consideration of the literature (36).
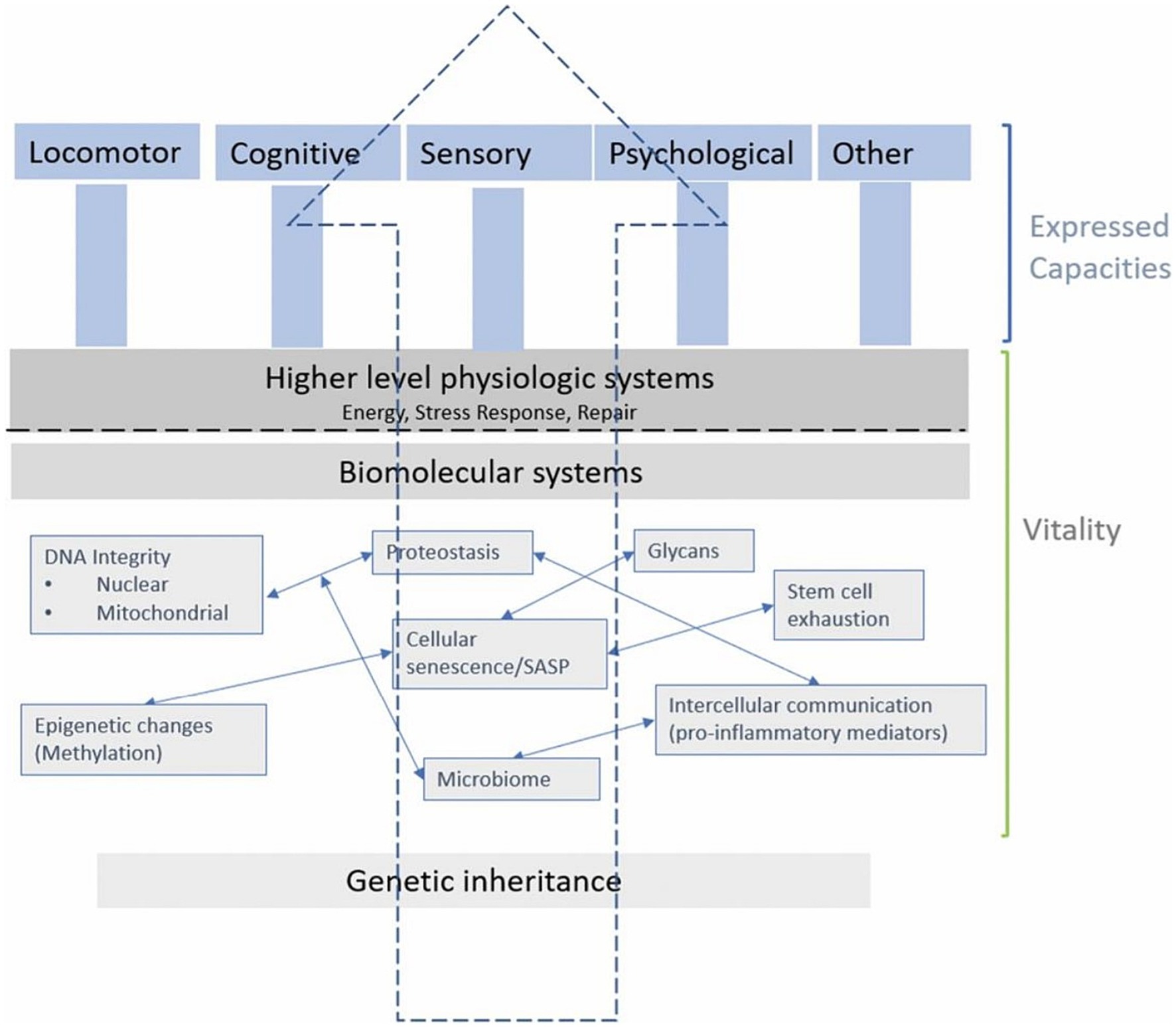
Figure 3. Reproduced with permission from Beard et al. (18)—a conceptual framework explaining vitality and intrinsic capacity.
1.3 A framework to conceptualize vitality, intrinsic capacity, and expressed capacities in aging
Enhancing the intrinsic capacity of individuals early in life is likely to have a substantial impact on maintaining independence in older age, thereby reducing the impending strain on healthcare and social support systems globally. Implementing policies and healthcare strategies focused on healthy aging is becoming increasingly crucial as we face an unprecedented global aging population.
Targeting cellular mechanisms involved in the hallmarks of aging can induce shifts in biomolecular systems and organs, affecting vitality (determined by genetic inheritance and other environmental factors) (19) which in turn can modulate intrinsic capacity and expressed capacities (measured as, e.g., locomotor, psychological, cardiorespiratory abilities and more). A recent consensus by a WHO working group underscores the role of vitality as a foundational element of intrinsic capacity (7). While frailty might be considered as an “accumulation of deficits,” the notion of vitality advances thinking in this area toward considering the trajectories of aging.
The overall architecture of this conceptual framework demonstrates the complex interplay between biological factors at distinct levels that cumulatively affect vitality, illustrating how biomolecular and physiological systems interconnect to shape the health and functionality of an organism.
1.4 Phytonutrients in the context of aging, vitality, and intrinsic capacity
The relevance of plant-based diets for aging may be attributed, in part, to their high content of nutrients and phytonutrients. Several studies suggest that phytonutrients are an important dietary constituent for healthy aging as they modulate oxidative stress, inflammation, and various signaling pathways involved in the hallmarks of aging, potentially playing a key role in preventing age-related diseases, maintaining normal metabolism, and supporting overall quality of life (37–46).
The “hallmarks of aging” were initially described by López-Otín et al. (20) and further revised a decade later (47). The 12 hallmarks have been extensively described in the literature as being genomic instability; telomere attrition; epigenetic alterations; loss of proteostasis; deregulated nutrient sensing; cellular senescence; mitochondrial dysfunction; stem cell exhaustion; altered intercellular communication; disabled macroautophagy; chronic inflammation and age-related dysbiosis. Although these hallmarks are independent drivers of aging, they are highly interconnected as they share common pathways, namely the sirtuins, mammalian target of rapamycin (mTOR), the nuclear factor erythroid 2–related factor 2 (Nrf2), and the nuclear factor kappa (NF-kb) signaling pathways (20, 47).
Plant-based solutions that attenuate cellular hallmarks of aging may confer benefits to subsequent tissues, organs, and systems (48), and potentially contribute to the intrinsic capacity of aging adults and therefore have the theoretical potential to influence the healthspan of this population (18). For instance, consumption of flavonoids, commonly found in fruits, vegetables, cocoa, wine, and tea, have demonstrated significant efficacy in reducing cellular damage through regulating the phosphoinositide 3-kinases (PI3K)/Akt/mTOR pathway, activation of sirtuin 1 (SIRT1), forkhead box, subgroup O (FOXO) and suppressing pro-inflammatory pathways in aged animal models (49). Inflammation being an important hallmark of aging, the observed anti-aging effect of flavonoids is likely to be mediated by their modulation of molecular pathways driving this hallmark.
However, the scientific literature on this topic presents a varied range of findings. A comprehensive analysis of the current evidence is necessary to discern the effects of different phytonutrients on the key aspects of aging and the development of age-associated conditions. This would help in identifying plant-derived compounds that have potential benefits for human aging. Moreover, existing reviews have not yet aligned their findings with a theoretical model of healthy aging that emphasizes vitality and intrinsic capacity.
1.5 The modulatory effect of nutrition on the hallmarks of aging
In the exploration of the molecular mechanisms of how nutrition regulates the aging process, recent advancements in “nutrigerontology” have highlighted the potential of dietary patterns and interventions to modulate the hallmarks of aging, aiming to extend healthspan and combat age-related diseases (33). López-Otín et al. emphasize the crucial role of dietary modulation in addressing the hallmarks of aging, suggesting that it is a viable approach to slow down the degenerative processes (47).
Key findings reveal that caloric restriction, specific nutrient supplementation, and adherence to diets rich in defined nutrients and bioactive compounds, such as NAD+ precursors, omega-3 fatty acids, and polyphenols can influence critical cellular pathways—ranging from mitochondrial function and telomere maintenance to intercellular communication and chronic inflammation (19). Among these bioactive compounds, polyphenols like resveratrol, quercetin, and epigallocatechin gallate have garnered attention for their ability to modulate nutrient sensing pathways including AMPK, SIRTs, and mTOR, which are pivotal in enhancing cellular energy efficiency, orchestrating appropriate metabolic responses, and promoting longevity while reducing disease risk (50, 51). Their impact extends to improving metabolic health and stress resistance through the activation of AMPK and SIRT1, and potentially decreasing cancer risk by inhibiting the mTOR pathway, thus supporting vital cellular processes like autophagy and mitophagy. Additionally, by modulating nutrient sensing pathways, polyphenols exhibit senolytic properties, allowing them to selectively induce the death of senescent cells—cells that have stopped dividing and thus contribute to aging and various chronic diseases (52, 53). Polyphenols, therefore, may have the potential to help improve tissue function and reduce inflammation associated with aging.
Furthermore, polyphenols are recognized for their role in mitigating immunosenescence—a gradual decline in the immune system’s functionality with age—by reducing senescent cell accumulation, decreasing cellular oxidative stress, and modulating immune function through epigenetic mechanisms (54, 55). Their ability to alter the gut microbiome composition, promoting the growth of beneficial bacteria, further supports immune function via the gut-immune system axis (56). These dietary strategies, including the enhancement of microbial diversity that affects systemic health by modulating neuroendocrine and inflammatory pathways, have been shown to regulate cellular mechanisms involved in aging, such as influencing metabolic health and potentially altering the course of aging and disease onset (57). The impact of polyphenols on immunometabolism highlights their potential in integrating metabolic and immune responses to maintain health and delay age-associated diseases (58).
Collectively, these findings underscore the intricate link between diet, cellular health, and healthy aging, offering promising avenues for dietary interventions to improve aging outcomes. While healthy, balanced diets may provide important quantities of phytonutrients from dietary sources (such as anthocyanins from berries, flavanones from citrus fruits and chlorogenic acid from coffee) some dietary patterns contain very low amounts of plant foods (59) and would benefit from dietary modification or supplementation. In the next sections, therefore, we will specifically focus on the potential role of phytonutrients to influence aging processes and relevant outcomes, against the backdrop of Beard et al. framework highlighted in Figure 3.
The relationship between diet and chronic disease is well established. High intakes of fruits and vegetables have been associated with reduced risk of chronic disease and mortality (60–62). The investigation of plant compounds involved in the mitigation of disease in aging has attracted widespread research. Some phytonutrients have been extensively investigated, whereas others that have recently emerged show promise, but well-designed randomized controlled trials (RCTs) are lacking. The next section discuss a range of phytonutrients, from the well-studied to the emerging and innovative, with the potential to support healthy aging. Furthermore, in addition to the dietary sources of phytonutrients, this review considers studies in which the concentrated form of the compound (or “plant nutraceutical) has been studied.
2 Amino acids and amines
2.1 Spermidine
Spermidine is a naturally occurring polyamine (63) with highest amounts reported in soybeans, peas, and pears (64), as well as in wheat germ, mushrooms, some nuts, and spinach (65). Spermidine is well-absorbed and rapidly distributed to the body’s tissues (66).
In aging, cellular levels of spermidine and ornithine decarboxylase (ODC), the enzyme involved in the synthesis of spermidine, decline with age (67, 68). Spermidine administration significantly extended the lifespan of yeast, flies and worms, and mice (69). In-vitro and in-vivo data demonstrates the ability of spermidine to impact the cellular hallmarks of aging via enhancing mitochondrial function, inducing cell growth and proliferation along with exerting anti-inflammatory, antioxidant and anti-senescent effects (63). Spermidine has also been shown to enhance autophagy in cellular and animal models of aging (63).
Epidemiological studies have correlated higher dietary spermidine intake with reduced all-cause mortality (70), including reductions in CVD and cancer-specific mortality (71). The first randomized controlled trial (RCT) to investigate the effect of spermidine supplementation on memory performance in older adults was a pilot study published in 2018 which reported a positive effect in adults aged 60–80 years (72). A further RCT found a positive effect of 3 months of spermidine supplementation on cognitive performance, using bread rolls containing either 3.3 mg or 1.9 mg of spermidine, in adults aged 60–96 years. In particular, those receiving the higher dose of spermidine showed improvements in cognitive performance among those with mild and moderate dementia (65). In one double-blind, randomized, placebo-controlled trial, 100 older adults (age 60–90 years) with subjective cognitive decline were supplemented with 0.9 mg/day of spermidine or placebo for 12 months (73). Results indicated no difference in memory performance and biomarkers in the spermidine supplemented group versus the placebo, though exploratory analysis showed possible beneficial effect of the intervention on inflammation and verbal memory. Overall, human RCTs show mixed results from spermidine supplementation.
The pre-clinical data and emerging studies indicate a potential for spermidine to impact cellular hallmarks of aging, and functional parameters such as cognitive function. Further clinical trials are required to confirm the preliminary data effect in humans, in particular for cellular health, neuro-protective function, and the potential to contribute to vitality and intrinsic capacity in aging.
2.2 Ergothioneine
Ergothioneine (EGT) is a naturally occurring amino acid, with good free-radical scavenging activity (74). The antioxidant activity of EGT makes it a potential candidate to offset the cycle of damage to cells via its anti-inflammatory, anti-neurodegenerative and anti-senescence properties demonstrated in cellular and animal models (75–78). EGT can only be obtained from a limited number of foods such as mushrooms, liver, black beans, garlic and oat bran (79, 80).
Absorption studies support the ability of EGT to be absorbed via the intestine, passed into circulation, and transferred into tissues throughout the body (81, 82). EGT levels decline after 60 years of age and more so in individuals with cognitive impairments (83, 84). On the contrary, a relationship between healthier dietary patterns, lower CVD risk, and higher circulating EGT levels has been observed (85, 86).
Human clinical studies have been conducted to investigate the effect on EGT on postprandial triglyceride response (87), oxidative stress and inflammation (82), and effects on lipid peroxidation, DNA damage, urate oxidation, protein carbonylation and C-reactive protein with limited to no effects observed (88). A single study examined the functional effect of EGT supplementation (combined with other anti-inflammatory nutrients and analgesics) on joint pain and range of motion (ROM) in adults with significant improvements over 6 weeks (88).
Despite a lack of data from human RCTs related to EGT, its role in cellular signaling pathways associated with aging, the protection of mitochondria and anti-inflammatory, anti-senescent effects make it a promising candidate for improving vitality and intrinsic capacity (34, 89).
3 Polyphenols
Polyphenols are natural compounds synthesized by plants and present in a variety of plant foods. They can be classified as phenolic acids, flavonoids (the largest subclass of polyphenols), polyphenolic amide, and other non-flavonoids (90). Epidemiological data highlights the association between polyphenol and flavonoid intake and a reduction of mortality from all causes and from CVD (91), cancer, diabetes, neurodegenerative disease, and osteoporosis (92). Polyphenols have shown multifaceted effects contributing to healthy aging by attenuating the development and accumulation of senescent cells, cellular oxidative stress, and immune dysfunctions (57); promoting cardiovascular health (93), reducing oxidative stress and inflammation; preventing neurodegenerative diseases, modulating autophagy and gut microbiota (94), and potentially extending healthspan by targeting various cellular mechanisms and signaling pathways.
This section will consider some well-known polyphenols and flavonoids, along with lesser-known emerging candidates, and discuss their potential to influence cellular exposure to oxidative stress, inflammation and a reduced activation of protective stress response pathways related to aging (95).
3.1 Flavonoids
3.1.1 Cocoa flavanols
Research into the flavanols from cocoa beans has shown that they possess properties which may offset the risk of chronic disease in aging, in particular CVD and type 2 diabetes (T2D), via their antioxidant and anti-inflammatory potential (96, 97). The main flavanols found in cocoa beans are epicatechin, catechin, and procyanidins, which are associated with various health benefits (98). The absorption and bioavailability of the flavanol compounds have been extensively described elsewhere and are well-understood, in particular for epicatechin (99). However, there is less information in relation to the complex metabolism of flavonoids by gut microbial pathways (100). It is thought that the active component of polyphenols might not be the polyphenols themselves, but their active metabolites (101).
The antioxidant potential of cocoa flavanols were first described by Waterhouse et al. who reported that cocoa polyphenols were superior to red wine polyphenols in their ability to inhibit LDL oxidation (102). Cell models have since elucidated that cocoa polyphenols are likely to prevent oxidation by acting on tumor necrosis factor alpha (TNFα), enzymes cyclo-oxygenase-2 (COX-2), C-reactive protein and soluble adhesion molecules (101) to down-regulate inflammation. However, some authors do not agree with the notion that flavonoids act as antioxidant molecules, but rather modulate the antioxidant response by targeting cellular pathways (103).
Several human intervention studies have demonstrated that cocoa and cocoa-containing products are associated with reducing CVD risk (104) via increasing HDL-cholesterol (105), improving endothelial function (106, 107), reducing blood pressure (108), decreasing blood triglycerides (104, 105), and reducing markers of oxidative stress (109). A large clinical trial in the US (COSMOS) reported that while cocoa extract supplementation did not reduce CVD events, it reduced deaths from CVD by 27% (110). Cocoa has also been found to improve insulin sensitivity in human RCTs (107, 111, 112).
Several studies have examined the effect of cocoa flavanols on cognitive performance in both old and younger populations (113) and have shown to have a potential effect on cognitive performance in aged populations since cocoa flavanols enhance cerebral blood flow in older subjects (114). In older adults (115) and adults with mild cognitive impairment (116), cocoa flavanols have been shown to improve cognitive performance and flexibility, processing speed (117), and working memory (118). However, one study in older adults showed no benefit of flavanols from chocolate, or a cocoa beverage, on cognitive performance (118). Inconsistencies in clinical studies on cocoa flavanols may be due to the variability of cocoa flavanol-containing food matrices along with inter and intra-individual variation in the response to cocoa polyphenols (39, 101). Moreover, cocoa flavanol supplementation was shown to positively affects facial wrinkles and elasticity in moderately photo-aged women (119). Nevertheless, future research should elucidate how the timing, form, and dosage of cocoa flavanols could elicit beneficial neuromodulatory effects in aging populations along with genetic factors that may impact an individual’s response to coco flavanols.
Overall, these studies suggest that flavanols in cocoa beans have multiple beneficial effects on health in aging, including improvements in oxidative stress, inflammation, cardiometabolic health, physical performance, skin health, cognitive function, neuroprotection, vascular function, and may reduce the risk of chronic diseases. While research on the specific effects of flavanols in cocoa beans on aging is ongoing, accumulating evidence suggests that incorporating cocoa or cocoa-derived products rich in flavanols into the diet may have beneficial effects on overall vitality and intrinsic capacity in aging.
3.1.2 Luteolin
Luteolin, a flavone metabolite, and luteolin-7-O-glucoside (LUT-7G) is a dietary derived compound found in carrots, peppers, celery, olive oil, rosemary, artichoke, pomegranate and plant extracts such as chrysanthemum (120, 121). Luteolin and LUT-7G have been shown to have potential anti-inflammatory, antioxidant, neuroprotective, DNA-protective, anti-cancer, anti-diabetic properties along with an ability to modulate cellular signaling pathways in in-vitro and in-vivo studies (122–130). Luteolin has also been shown to protect heart tissue in a diabetic mouse model via modulating Nrf2-mediated resistance to oxidative stress and NF-κB-induced inflammatory responses (131). Such data places luteolin as an emerging candidate to attenuate cellular hallmarks of aging. In particular luteolin has been cited as having widespread neuroprotective effects in both in-vitro and in-vivo models of Alzheimer’s disease (AD), Parkinsons disease (PD) and cognitive decline, and is able to suppress inflammation in brain tissue (127, 132).
Epidemiological evidence suggests that luteolin possesses anti-inflammatory and cardioprotective effects. Studies have shown a significant reduction in CVD mortality and decreased incidence of epithelial ovarian cancer associated with higher luteolin intake (133, 134).
Human clinical trials investigating luteolin supplementation are limited, with mixed results. One study examining luteolin’s role in Gulf War Illness, characterized by high levels of inflammatory markers, showed no significant improvement in symptom severity (135, 136). However, in a placebo-controlled trial targeting pre-obese individuals, a phytonutrient blend containing luteolin demonstrated promising cardiometabolic outcomes, including weight reduction and improvements in glycemic and lipid parameters. Authors postulated that the findings may, in part, be due to the ability of luteolin to attenuate adipose tissue inflammation and insulin resistance, as demonstrated in animal models (137).
Overall, more research is required to understand effective human doses, metabolism and absorption of luteolin in order to perform well-designed human clinical trials involving luteolin and LUT-7G (138). Nonetheless, in-vitro and in-vivo data indicate the potential for luteolin to impact vitality via its neuroprotective, cardioprotective, anti-inflammatory and anti-obesity properties. Further research would help to elucidate any eventual impact of luteolin on vitality and intrinsic capacity in aging.
3.1.3 Fisetin
Fisetin is a flavonoid present in fruits such as strawberries, apples, kiwi and mangoes (139). Fisetin demonstrated pre-clinical potential to offset inflammatory pathways that lead to chronic diseases in aging (140). Chronic inflammation, if left uninhibited, may trigger cellular pathways that lead to CVD, osteoporosis, cancer and neurodegenerative disease, via its inter-connectedness with many of the cellular hallmarks of aging (141). Furthermore, fisetin has been widely studied for its antioxidant effects, along with its ability to inhibit cellular senescence (142). Notably, in a pre-clinical trial, out of 10 flavonoids studied, fisetin was found to be the most potent senolytic, reducing senescence markers in multiple tissues (53). Neurodegenerative diseases linked to mitochondrial dysfunction and ROS may benefit from intervention with fisetin since it has been shown to interact with diverse REDOX signaling pathways, restore mitochondrial function, and contribute to prevention of neuronal cell death (143). Despite encouraging in-vitro and in-vivo data on the multiple beneficial effects of fisetin in relation to the hallmarks of aging, its translation to human RCTs has been limited for the time being. Small scale human clinical trials focus on fisitin supplementation post cardiovascular events and inflammatory conditions (such as Gulf War Illness) with mixed effects (144–148). Its poor absorption in humans has limited its therapeutic potential. To address this issue, an innovative study proposed encapsulating fisetin into a fisetin-loaded dietary fiber hydrogel scaffolds to improve its delivery and bioavailability in human subjects (149).
While further research is needed to fully elucidate the therapeutic potential of fisetin in humans, both pre-clinical and small-scale clinical studies suggest that fisetin holds promise as a natural compound for promoting vitality in aging. Its multifaceted mechanisms of action make it a promising candidate to impact multiple hallmarks of aging, including cellular senescence, mitochondrial dysfunction, and chronic inflammation.
3.1.4 Quercetin
Quercetin is one of the most common and studied flavonoids, present in many different fruits and vegetables such as onions, apples, berries, kale, leeks, asparagus and capers (150). However, dietary intake of quercetin varies depending on the fruit and vegetables intake (151).
It has been shown to have anti-inflammatory, antioxidant, anti-cancer, anti-hypertensive, anti-diabetic, anti-neurodegenerative and cardio-protective properties in pre-clinical models and in human RCTs (144, 152). Several studies have found beneficial effects of quercetin with respect to antioxidant biomarkers and the inhibition of LDL oxidation (145, 153–155). However, a systematic review of RCTs concluded that quercetin supplementation did not lead to a clinically significant effect on plasma lipids, but a dose of >50 mg/day may have a beneficial effect on reduction of triglycerides (156). One study found that quercetin supplementation inhibited platelet aggregation (157) improved some biomarkers of endothelial dysfunction (158) reduced systolic blood pressure (155) and reduced blood pressure in hypertensive patients (159, 160). Furthermore, a systematic review of RCTs concluded that quercetin supplementation of >500 mg/day could significantly reduce blood pressure (161). Together, these studies suggest that quercetin may confer some cardioprotective effects. However, more studies of longer duration are required, in different populations, to confirm these findings and to gain more insight into potential mechanisms of action. A systematic review of in-vitro and in-vivo data support the potential anti-diabetic effect of quercetin (162). It has been shown to act on signaling pathways such as TNFα, NFkB, AMPK, Akt, and Nrf2 which are implicated in the pathogenesis of T2D and insulin resistance (163). The oral administration of 250 mg/day for 8 weeks improved the antioxidant status among subjects with T2D (164).
Furthermore, quercetin interacts with SIRT1, a key enzyme in cellular processes linked to aging (165, 166). Animal studies suggest increased SIRT1 expression may protect against AD (167), while decreased expression is observed in aging mice (168). Quercetin regulates SIRT1 pathways, potentially initiating protective mechanisms against AD. A randomized trial in older adults showed quercetin supplementation improved reaction time and preserved cerebral blood flow over 40 weeks (169). Further research is needed, but quercetin shows promise for mitigating age-related cognitive decline and supporting neurocognitive health.
Further investigation is warranted to fully comprehend the potential cardioprotective and cognitive benefits of quercetin, a naturally occurring senolytic compound. Its interaction with SIRT1 makes it a promising candidate for targeting cellular senescence and enhancing vitality. However, a deeper understanding of its mechanism of action within specific disease phenotypes is essential for guiding future research and potential applications of quercetin in alleviating chronic conditions associated with aging. Nonetheless, encouraging diets that contain food sources of quercetin, or the inclusion of bioavailable sources of quercetin as supplements may contribute to vitality and intrinsic capacity in aging.
3.2 Stilbenes
3.2.1 Resveratrol
Resveratrol is a polyphenol present in plant foods, with top sources being grape skin, red wine, blueberries, and peanuts (44). The polyphenolic nature of resveratrol makes it a powerful antioxidant shown to upregulate the expression of antioxidant enzymes and to reduce mitochondrial superoxide generation by stimulating mitochondrial biogenesis in pre-clinical studies (170). Several studies suggest that resveratrol has the potential to extend lifespan and improve healthspan in various species, particularly through mechanisms involving metabolic regulation, stress resistance, and activation of longevity genes, although its effects may vary across different organisms (171–173). Resveratrol also has demonstrated significant potential to activate autophagy, increase mitochondrial biogenesis, support free radical quenching, and induce anti-inflammatory effects (44). This may occur through stimulating SIRT1 and NRF2 pathways, and by downregulating NF-kB and Akt/mTOR pathways. Consequently, by regulating multiple longevity-related signaling pathways, resveratrol demonstrates considerable potential in addressing various hallmarks of aging, such as low-grade inflammation, compromised autophagy, and deregulated nutrient sensing (44).
Multiple rodent studies have provided evidence supporting the neuroprotective effects of resveratrol. These studies have demonstrated that resveratrol supplementation improves antioxidant status in the brain, reduces concentrations of inflammatory markers, and enhances memory performance in animal models of memory impairment, including working memory, spatial memory, and learning memory (146–148). Specifically, resveratrol has been found to stimulate brain SIRT1 activity while suppressing NF-kb and enhancing AMPK. This activation of AMPK is crucial in protecting against the accumulation of Amyloid-β through the regulation of neuro-inflammation and oxidative stress (174–176).
One clinical trial has demonstrated that resveratrol supplementation for 30 days induces metabolic changes in obese humans, mimicking the positive effects of calorie restriction (177). Resveratrol supplementation significantly improved glucose control and insulin sensitivity in persons with T2D but did not affect glycemic measures in nondiabetic persons (178). Overall, resveratrol is regarded as a caloric restriction mimetic (CRM) based on evidence from clinical trials demonstrating its ability to replicate some of the metabolic benefits observed with caloric restriction.
Resveratrol has popularly been investigated for its cardioprotective benefits and has been studied in models of CVD (148). In humans, clinical trials have shown contradicting results in terms of the cardioprotective effect of resveratrol. In fact, while consistent reductions in inflammatory markers and improvements in endothelial function are observed, the hypolipidemic, hypoglycemic, and hypotensive effects of resveratrol remain inconclusive (179). Regarding neuroprotection, administering resveratrol orally led to dose-dependent increases in cerebral blood flow during task performance. However, cognitive function remained unaffected in one RCT (179).
Resveratrol is rapidly metabolized after absorption, leading to low bioavailability in its unmetabolized form (180). This may be one of the reasons behind the variability seen in clinical trials. For instance, in a study investigating the effect of resveratrol on blood pressure, only doses higher than 300 mg/day were shown to reduce systolic and diastolic blood pressure, possibly compensating for the low bioavailability of resveratrol (181). Indeed, its bioavailability was shown to depend on the dose administered, and other bioactives contained in the meal (182). Co-ingestion of piperine and quercetin with resveratrol increased its bioavailability in animal studies (182) however further human studies are required to confirm this outcome.
In summary, resveratrol has demonstrated significant benefits as an antioxidant and anti-inflammatory bioactive in model organisms and humans, making it a potential positive contributor to vitality. While resveratrol shows promise as a metabolic enhancer and caloric restriction mimetic, clinical trials have yielded mixed results, particularly concerning its cardioprotective effects. Further research is necessary to clarify its therapeutic potential and optimize its efficacy in promoting vitality and health in aging.
3.2.2 Pterostilbene
Pterostilbene is a naturally occurring polyphenol most abundantly found in blueberries (183), with lower concentrations in other plant sources such as grapes, cranberries, almonds, and peanuts (184). Yet, the quantity of pterostilbene in blueberries may be too low to induce health benefits which means that delivery of pterostilbene via dietary supplements would enable provision of nutritionally relevant amounts (185).
Pterostilbene is a 3,5-dimethoxyl-resveratrol, which has been reported to have superior bioavailability according to data in animal models (186). Many in-vitro and in-vivo studies have demonstrated the potential vitality enhancing effects of pterostilbene via its ability to exert anti-inflammatory, antioxidant, neuroprotective (187, 188) and cardioprotective effects (189–191). It is understood to elicit these beneficial effects via its action on mitochondrial oxidative stress, mitochondrial biogenesis and mitochondrial apoptosis (192). In addition, there is evidence pterostilbene may have the potential to decrease glucose and increase plasma insulin levels in animal models of T2D (193, 194).
Unfortunately, human RCTs using pterostilbene are scarce. One such study found a beneficial effect of pterostilbene supplementation on systolic and diastolic blood pressure (195). However, an interesting observation from the study was that while pterostilbene supplementation improved blood pressure, there was an increase in LDL cholesterol levels. Remarkably, this rise in LDL cholesterol was not observed in participants who received a combination of grape extract and pterostilbene. Grape extract is known to contain various bioactive compounds with potential cardiovascular benefits and combining it with pterostilbene seemed to mitigate the adverse effect on LDL cholesterol levels.
Further research is warranted in order to design human RCTs which can shed light on the required dosage, delivery method, disease-specific formulations, and efficacy in the longer term (196). Nonetheless, the pre-clinical data support pterostilbene as a potential phytonutrient which may promote vitality in aging via effects on cellular pathways related to hallmarks of aging.
3.3 Beta-diketones
3.3.1 Curcumin
Curcumin (diferuloylmethane) is a polyphenolic compound extracted from the turmeric (Curcuma longa L.) root that is widely used in Asia, as a spice, food additive, and herbal remedy (197). In fact, in Ayurveda, turmeric is prescribed to support immunity and treat respiratory disorders such as asthma (197). In the scientific literature, curcumin is mostly known for its anti-inflammatory and antioxidant properties and has demonstrated efficacy on reducing the severity of arthritis symptoms (198, 199) and glucose impairment (200, 201) as reported in meta-analyses of clinical trials. Curcumin can target multiple pathways involved in inflammation, autophagy, and other hallmarks of aging. However, its clinical application is limited by poor bioavailability, attributed to low water solubility, rapid metabolism, and fast systemic elimination. To address these challenges, various strategies have been employed, including nanotechnology-based approaches (such as liposomes, nanoparticles, and solid lipid nanoparticles), the use of adjuvants (notably piperine, which inhibits metabolic degradation), and formulation modifications (like water-soluble derivatives and polymeric micelles) (202).
Curcumin induces cellular stress responses in normal human skin fibroblasts, potentially offering a useful anti-aging approach by enhancing cellular antioxidant defenses (203). Curcumin and its metabolite, tetrahydrocurcumin, increase lifespan in model organisms by regulating oxidative stress responses and age-related genes (204). Animal studies have facilitated the understanding of molecular mechanisms underlying curcumin benefits. Curcumin triggers the Nrf2 pathway which activates antioxidative enzymes, thereby mitigating oxidative stress and facilitating ROS removal from the cells, ultimately leading to lower lipid peroxidation, lower ROS concentration in tissues, and increased antioxidant capacity (197). In humans, supplementation with 600 mg of curcumin per day reduces circulating malondialdehyde, a marker of oxidative stress, and increases superoxide dismutase, an antioxidant enzyme, in red blood cells, as reported in a meta-analysis of 8 clinical trials (205). These findings highlight curcumin as a promising candidate for targeting oxidative stress associated with aging and supporting healthy aging. This is particularly true for managing age-related diseases like atherosclerosis and T2D, along with their complications such as retinopathy and nephropathy.
The clinical trial literature on curcumin shows promising efficacy in the prevention of atherosclerosis. Accordingly, two meta-analyses of five (206) and two (207) randomized controlled trials, respectively, reported a significant overall increase in flow-mediated dilation, generally associated with better vascular health, in both healthy and metabolically unhealthy individuals following daily curcumin supplementation. In addition, there is good evidence supporting the role of curcumin in reducing LDL-cholesterol, triglycerides, and increasing HDL-C levels in healthy subjects as well as those at risk of CVD (205, 208, 209).
Although the anti-inflammatory capacity of curcumin may originate from its antioxidant effects, this bioactive has also shown to directly modulate the inflammatory cascade through its inhibitory effect on NF-kB, a known regulator of pro-inflammatory gene expression and promoter of inflammatory cytokines and chemokines (210). Research on curcumin supports its role in attenuating systemic inflammation and reducing symptoms of age-related inflammatory conditions such as osteoarthritis (199, 211), improving CVD risk factors, and reducing severity of non-alcoholic fatty liver disease (212, 213).
Curcumin affects multiple nutrient sensing pathways, including the insulin/insulin growth factor, AKT, and mTOR pathways, crucial for aging hallmarks. In vitro studies demonstrate curcumin’s suppression of mTOR and FOXO signaling while enhancing AKT signaling. These effects correlate with lifespan extension in multiple model organisms (197). Curcumin’s signaling effects extend to systemic protection in vital organs like the brain. Animal models of cognitive deficit or neuroinflammation reveal curcumin’s ability to reduce brain tissue inflammation, neuronal loss, and cognitive impairment. However, human studies on curcumin and cognition are limited and yield inconsistent results (214).
In summary, curcumin emerges as a widely utilized phytonutrient with demonstrated antioxidant properties across in vitro, animal studies, and human clinical trials. Its potential as an anti-aging bioactive is underscored by its mechanism of action, which involves suppressing mTOR and FOXO pathways while upregulating the P13K–AKT cascade. Notably, curcumin influences several hallmarks of aging, including nutrient sensing, oxidative phosphorylation, and chronic inflammation. Although further research is necessary to validate its role in aging, numerous clinical trials substantiate its efficacy in mitigating inflammatory conditions, endothelial impairment, metabolic deregulation, and CVD—each of which may significantly impact expressed capacities in older adults. As a low-cost intervention, curcumin holds promise for enhancing vitality and intrinsic capacity in aging, thereby warranting continued investigation.
3.4 Benzo-coumarins
3.4.1 Urolithin A
Urolithin A (UroA) emerges as a pivotal metabolite derived from the gut microbiota’s conversion of ellagitannins and ellagic acid, abundantly found in red fruits, pomegranates, and nuts. This conversion exhibits considerable variability, influenced by individual age and health status. In a series of in vitro, animal, and human studies, a pure form of urolithin A was shown to upregulate genes and activate pathways involved in mitophagy (215), activate antioxidant pathways (216) and reduce oxidative stress markers (217, 218), improve age-related functional health (219, 220), and even increase the lifespan in an aging model (221). In human cell lines, particularly dermal fibroblasts damaged by UV light, UroA has been shown to activate the NRF2 pathway, thereby boosting ROS scavenging and the release of antioxidant enzymes (222). This effect is partly mediated by the SIRT3-FOXO3-PINK1-PARKIN network, which plays a crucial role in mitophagy. Remarkably, treatment with UroA has led to significant reductions in inflammatory cytokines such as TNF-α and IL-6 in studies involving microglia and neural cells (215). Inducing mitophagy has a beneficial effect on mitochondrial health, an effect that has been observed in healthy volunteers after UroA interventions. Significant decreases in acylcarnitines, C-reactive protein, and ceramides—markers of mitochondrial dysfunction and age-related diseases—were observed in middle-aged and older volunteers post-UroA intervention (219, 220).
The functional effects of UroA have been further investigated in the context of age-related diseases. Significant improvements in neuroinflammation and memory impairment have been noted in mice (223), while human studies have reported enhanced muscle strength and exercise performance in middle-aged and older adults (219, 220). Clinical trials focusing on urolithin-producing foods like raspberries, strawberries, and pomegranate have also highlighted potential health benefits. For instance, the consumption of raspberry juice was associated with improved endothelial function, correlating positively with UroA plasma concentration (224). Two clinical trials (225, 226) investigated the consumption of strawberries (226) and pomegranate extract in relation to microbiota compositional changes, reporting an increased abundance of strains associated with longevity (226) and a healthy metabolic profile (225, 226). Although these results are very promising, further studies isolating the effect of UroA itself are warranted to support the emerging evidence on UroA and functional benefits linked to the hallmarks of aging and potential contribution to vitality.
In summary, the mechanistic effects of UroA on mitophagy, mitochondrial health, oxidative balance, and low-grade inflammation could potentially translate into clinically significant advantages for a range of age-related conditions, including sarcopenia, osteoarthritis, Alzheimer’s disease, and more. Clinical evidence supports UroA’s enhancement of muscle strength and metabolic health, with pre-clinical studies suggesting broader anti-aging effects on organs and systems such as the brain and skin.
4 Glucosinolates
4.1 Glucoraphanin
Glucoraphanin (GPh) is a glucosinolate found in cruciferous vegetables such as broccoli, particularly concentrated in broccoli sprouts, cauliflower, kale, cabbage and Brussel sprouts, and has garnered attention for its antioxidant and anti-inflammatory properties (227–230).
GPh is converted into sulforaphane (SPh) through a process that involves an enzyme known as myrosinase (230). This conversion can occur within the plant itself, as well as through the action of the oral and gut microbiota after ingestion (231). SPh is capable of interacting with many different molecular pathways associated with aging (229). In particular, SPh is a potent inducer of phase 2 enzymes which may help cells to better detoxify and remove potentially harmful compounds (232). There is also a genetic influence which may mediate the benefit of GPh consumption. About 50% of the population possess the glutathione S-transferases 1 genotype (GSTM-1) and may gain additional benefit from GPh ingestion, versus those without the genotype who excrete more SPh in 24 h (233).
Pre-clinical evidence, largely from cell culture and animal studies, has indicated that sulforaphane may have a role in the prevention and amelioration of various conditions such as certain cancers, T2D, and asthma due to its activation of Nrf2 signaling and resulting induction of cytoprotective enzymes (234).
Clinical trials involving broccoli sprout preparations, which contain high levels of GPh, began in the late 1990s (235). These trials have evaluated the safety, acceptability, bioavailability, and pharmacokinetics of these preparations. However, it should be noted that most clinical studies to date have used broccoli sprout preparations rather than pure GPh or SPh, and it remains to be determined whether there is bioequivalence in these interventions. A systematic review cited 8 human RCTs which have examined the effect of broccoli sprouts on various outcomes (236). In a Chinese study, a broccoli sprout containing beverage was consumed for 12 weeks, providing 600 μmol GPh and 40 μmol SPh. The authors reported that SPh was capable of binding and excreting airborne pollutants (237).
Two human clinical trials demonstrated that diets containing 400 g of high-GPh broccoli per week, for 12 weeks, can significantly lower LDL-C levels, versus diets containing 400 g of standard broccoli per week (238). Moreover, among healthy, overweight subjects consuming 30 g of broccoli sprouts per day led to a significant decrease in inflammatory markers as well as in body fat mass (239). A further study demonstrated that a SPh-rich broccoli sprout extract improved liver function by reducing oxidative stress in Japanese men with fatty liver (240). Another small trial randomized 14 patients to a high-glucosinolate diet for 14 days prior to knee replacement surgery. They found that glucosinolates were able to penetrate the knee joint and synovial fluid (241). Such trials pave the way for the exploration of GPh on osteoarthritis of the knee. This builds on prior in-vitro and in-vivo data demonstrating SPh can protect cartilage destruction (242).
Helicobacter pylori (H. pylori) infected patients who consumed 70 g/day of broccoli sprouts for 8 weeks displayed decreased markers of H. pylori colonization and reduced markers of gastric inflammation (243). A further study found that an aqueous broccoli seed extract reduced levels of pro-inflammatory cytokines and led to greater H. pylori eradication versus the placebo (11.1% vs. 3.7% at 60 days) (244). Eradication of H. pylori infection is associated with a reduced incidence of stomach cancer (245). These findings from clinical trials are supported by similar results from a study in a female mouse model infected with H. pylori (243).
In conclusion, emerging studies highlight the potential health benefits of broccoli, broccoli sprouts, broccoli seeds and sources of myrosinase in the diet, beyond the usual consideration of the nutrient contributions that vegetables make to the diet. Nonetheless, studies are somewhat sparse and heterogeneous. Future research on the benefits of GPh will open up possibilities for a low-cost dietary intervention to enhance antioxidant and anti-inflammatory pathways which may influence vitality capacity in aging.
5 Carotenoids
5.1 Astaxanthin
Astaxanthin, a xanthophyll carotenoid, has been acclaimed for its anti-aging properties, eclipsing beta-carotene and vastly outperforming vitamins E and C in neutralizing ROS (246, 247). Originating from aquatic sources like crustaceans and salmon, where it imparts a distinct orange-pink-red hue, astaxanthin is integral to their diet for both coloration and health (248). Its bioactivity surpasses that of lutein and zeaxanthin, with promising improvements in inflammation and oxidative markers in preclinical studies (249). Given its foundational role in chronic disease mechanisms, astaxanthin’s research trajectory is directed toward its efficacy as an anti-aging agent (250).
Termed a “mitochondrial antioxidant,” astaxanthin has demonstrated defensive capabilities against ROS in cellular studies, safeguarding cell and mitochondrial membranes (251, 252). Human randomized controlled trials (RCTs) have indicated that astaxanthin supplementation can significantly reduce oxidative stress markers, such as malondialdehyde, and inflammatory markers like IL-6, particularly in T2D patients (253). Furthermore, astaxanthin has been shown to improve skin health, evidenced by enhancements in skin appearance, texture, and UV damage protection (44, 254). Clinical studies have also touched on astaxanthin’s potential neuroprotective effects, with certain studies reporting improved cognitive functions, such as verbal episodic memory, in middle-aged adults following astaxanthin supplementation (255).
Consequently, there is emerging potential for astaxanthin to support healthy aging, as highlighted in studies that demonstrate anti-inflammatory and antioxidant properties, skin- aging benefits and potential protection in the context of neurodegenerative diseases. Astaxanthin warrants further investigation as a potential geroprotector with the potential to contribute to vitality and intrinsic capacity in aging.
6 Plant extracts
6.1 Rosemary
Rosemary (Rosmarinus officinalis L.) contains biologically active phenolic compounds, the principal components being rosmarinic acid (RA), carnosic acid (CA) and carnosol.
RA has been described as having a myriad of healthspan-promoting biological activities in-vivo and in-vitro (256), such as protecting cell membranes from oxidation (257), promoting antioxidant activity against ROS production and IL-6 release in human keratinocytes (258), along with anti-viral, anti-mutagenic, anti-bacterial, analgesic, and anti-inflammatory activities (259–262). RA has also shown promise in helping to avoid the build-up of amyloid-β aggregation by inhibition of aggregation pathways in-vivo (263) and may help to improve motor control and extend lifespan of ALS mouse models (264). How RA exerts these beneficial effects at the level of the cell is not fully understood but experts suggest it may be linked to its ability to act as a regulator of TNF-α-induced NF-κB signaling, which is crucial to the balance between cell life and death (265). Two further active compounds CA and carnosol have been found to have anti-inflammatory, anti-tumor and antioxidant activity in vitro and in vivo (266–268). In particular, the anti-inflammatory effect of CA on brain microglia (269) and anti-depressant effect in mouse models (270) has led to interest in the use of CA for therapeutic treatment in neurodegenerative diseases.
In human clinical trials, rosemary has frequently been studied for its ability to enhance memory and cognitive function. One clinical trial testing 68 university students found that 500 mg of dried rosemary supplementation (aerial parts) for 1 month significantly improved memory performance and sleep quality, while decreasing anxiety and symptoms of depression (271). One RCT provided RA capsules to patients for 8 weeks as an adjunct therapy for depression. The scores on measures of anxiety and depression reduced significantly in the RA treated group versus the placebo therefore RA may be of use in the reduction of anxiety and depression in depressed patients, alongside anti-depressant medication (272). Another RCT found that short-term low-dose rosemary supplementation (750 mg) induced a statistically significant beneficial effect on speed of memory in older adults (mean age, 75 years old) compared to placebo (273). This study also indicates that exceedingly high RA doses (6,000 mg) lead to impairment in cognitive performance, emphasizing the significance of appropriate dosage in optimizing cognitive benefits. Furthermore, an extract of Melissa officinalis (M. officinalis), which supplied 500 mg of RA daily, was found to be effective in helping to prevent the worsening of neuropsychiatric symptoms related to AD (274). The same extract was found to be beneficial in preventing cognitive decline in non-hypertensive adults without dementia following supplementation with 500 mg of RA for 96 weeks (275).
Moreover, rosemary has often been studied in combination with other botanical ingredients and therapies. For example, an extract of Lemon balm (Melissa officinalis), which supplied 500 mg of RA daily, was found to be effective in helping to prevent the worsening of neuropsychiatric symptoms related to AD (274). The same extract was found to be beneficial in preventing cognitive decline in non-hypertensive adults without dementia following supplementation with 500 mg of RA for 96 weeks (275). One RCT found no effect of a proprietary spearmint extract, high in RA, on executive function in adults (276) whereas a trial in older adults with memory impairment found that supplementation with the spearmint extract (900 mg/day) improved working memory and spatial working memory (277). Improvements in cognitive function were also found in young, healthy adults who consumed the high RA spearmint extract (278). A further trial using the same extract reported an improvement in reactive agility among healthy adults who were supplemented with 900 mg of the extract versus placebo (279). More evidence is provided by one study where a combination of sage, rosemary and lemon balm extract was more effective than a placebo in supporting verbal episodic memory in healthy subjects under 63 years of age (280).
Overall, these studies support the notion that rosemary and its constituents may offer a new avenue in combating age-related cognitive decline. The urgent need for effective treatments in the face of devastating neurodegenerative conditions underscores the critical importance of further research into rosemary extracts. Its potential ability to mitigate cognitive decline and oxidative stress marks a significant breakthrough in the pursuit of safe, cost-effective interventions. Rosemary extracts hold promise in revitalizing cognitive vitality and influencing expressed capacities in aging individuals, paving the way for a future where cognitive problems may find formidable adversaries in nature’s own remedies.
6.2 Ginger
Although originating in Southeast Asia, ginger (Zingiber officinale) has become a globally consumed spice deeply ingrained in various cultures, cherished for its perceived health benefits and medicinal attributes (281). The gingerols, shogaols, paradols, and zingiberene are the principal bioactive compounds (282, 283). Ginger and its constituents may improve vitality and intrinsic capacity via their ability to modulate cellular pathways related to hallmarks of aging, eliciting anti-inflammatory and antioxidant benefits (284–286).
Preclinical research demonstrates that ginger and its bioactive compounds scavenge free radicals and mitigate oxidative stress (286, 287). Studies in animal models have shown that ginger supplementation enhances antioxidant enzyme activity and reduces oxidative damage to cellular macromolecules, thereby promoting cellular longevity (285, 288). Anti-inflammatory properties are also demonstrated both in vitro and in vivo indicating that gingerols and related compounds inhibit pro-inflammatory mediators such as NF-κB and cytokines (289–291). By modulating inflammatory pathways, ginger may attenuate age-related inflammation and mitigate associated health risks (289). Moreover, ginger and its bioactive constituents exert neuroprotective effects by enhancing antioxidant defenses, reducing neuroinflammation, and modulating neurotransmitter activity (292, 293). Animal models of AD have demonstrated improvements in cognitive function and decreased neuroinflammation following ginger supplementation, implicating its potential in promoting brain health and longevity (294). Fermented ginger was found to reduce synaptic disorder and neuron cell death (295).
Human trials corroborate these findings (286, 296). A meta-analysis of clinical trials examining the effect of ginger on oxidative stress biomarkers concluded that ginger supplementation decreased biomarkers of oxidative stress such as malondialdehyde, and increased antioxidant enzymes such as GPx (297). Moreover, a comprehensive systematic review of RCTs on ginger and human health concluded that ginger was effective in studies related to digestive function and anti-inflammatory action (298). Commonly recognized for relieving nausea and vomiting, this benefit of ginger is also validated by animal and human trials (299). A single dose of ginger (1.2 g) has been shown to stimulate gastric emptying and stomach contractions (300). One gram of ginger root was able to prevent hyperglycemia-evoked slow-wave gastric dysrhythmias potentially via blunting the production of prostaglandins (301). There are several proposed mechanisms via which ginger may alleviate nausea and vomiting via its anti-inflammatory properties, redox signaling abilities, effect on motility and gastric emptying, and vasopressin release (299). Ginger has been found to promote gastric motility, a beneficial function in healthy individuals (302) and those with dyspepsia (303) and to enhance swallowing in older populations (304).
Ginger’s impact extends to various aging-related health issues, including metabolic disorders, CVD, neurodegenerative conditions, and arthritis. Systematic reviews and meta-analyses of controlled trials show that ginger can significantly modulate metabolic health markers—reducing body weight, waist-to-hip ratio, fasting glucose, insulin resistance index, and elevating HDL-cholesterol (305–309). Cardiovascular health, integral to healthspan and longevity, may also be favorably influenced by ginger. Meta-analyses of RCTs suggest that ginger can lower blood pressure, improve serum lipid profiles, and enhance endothelial function, particularly in individuals with hypertension and dyslipidemia (310). Cognitive health, especially in neurodegenerative diseases, benefits from ginger as well. In fact, findings from an RCT demonstrate that ginger extracts, at doses of 400 or 800 mg/day, could improve cognitive performance in middle-aged women (311).
In the context of arthritis, the anti-inflammatory properties of ginger have shown promise in reducing pain associated with osteoarthritis (OA) and decreasing reliance on pain medication (312, 313). A human trial indicated that 500 mg/day of ginger could lower proinflammatory cytokines after 3 months of supplementation (314). While ginger combined with pain medication was more effective than either alone for OA symptom relief, a separate study found no benefit of ginger powder over placebo for knee OA pain (315, 316). In rheumatoid arthritis (RA), ginger supplementation was observed to potentially influence inflammation and immunity genes, as indicated by changes in disease activity scores and gene expression (317, 318).
Overall, both preclinical and clinical studies provide compelling evidence for the potential health-promoting effects of ginger and its bioactive components, particularly in relation to inflammation, oxidative stress, cardiovascular health, neuroprotection, and metabolic regulation. The evidence points to ginger as a promising natural extract to contribute to vitality, intrinsic capacity and potentially expressed capacities in aging. By targeting underlying mechanisms of aging and age-related diseases, ginger holds promise as a natural intervention for promoting healthspan and longevity in human populations. Further studies which elucidate the effective dosage for different age-related diseases, along with an improved understanding of the pharmacodynamics and pharmacokinetics will accelerate our understanding of gingers’ potential to influence healthspan in aging.
7 Discussion
Our review introduces a novel conceptual thinking in relation to phytonutrients and their ability to impact cellular processes in aging, which may improve vitality and intrinsic capacity (35). Additionally, plants which provide phytonutrients like ginger, curcumin, and rosemary, have been the subject of significant research, could potentially impact the expressed capacities associated with aging. Plant-based diets or supplements that provide phytonutrients could be valuable alongside wider diet and lifestyle interventions in healthy aging (7). The theoretical framework shown in Figure 3 introduces a novel perspective for assessing the efficacy of nutritional components or interventions, proposing a structured framework to support the empirical substantiation of nutrition’s role in preserving and augmenting intrinsic capacity. By scrutinizing the functions of select plant-based nutrients through this lens, we contribute to a refined comprehension of how dietary practices which emphasize the nutrient contribution of plant-foods might be instrumental in advancing healthspan (Figure 4). Indeed, it should be noted that the evidence supporting the efficacy of these phytonutrients spans a spectrum from emerging to multiple human randomized controlled trials, necessitating a balanced and ongoing assessment of nutritional research findings in the context of healthy aging.
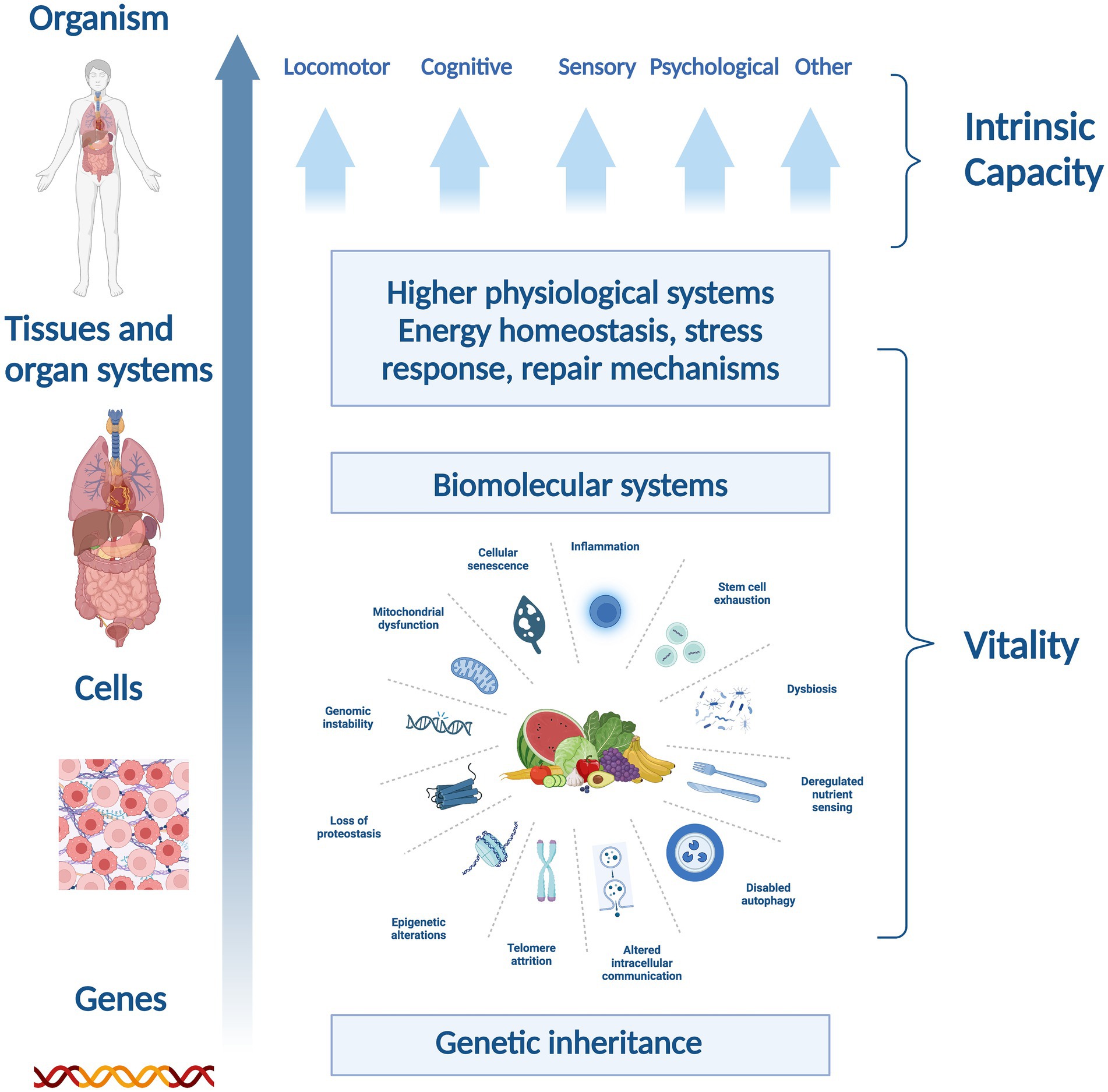
Figure 4. Schematic representation of the relationship between intrinsic capacity and vitality across biological hierarchies.
Cesari et al. highlights the relationship between nutrition and intrinsic capacity (9). Their research further supports the hypothesis that nutrition significantly influences the core components of intrinsic capacity, thereby affecting vitality and the process of aging. Cesari et al. argue that nutrition is not merely about the prevention of deficiencies but is integral to optimizing health, functionality, and well-being in older adults. A nutritious diet supports all dimensions of an individual’s intrinsic capacity, including cognitive function, physical strength, immune response, and emotional health (6, 9, 25). This conceptual shift underscores the importance of dietary factors in supporting the expressed capacities of aging individuals, and consequently, their functional health (9) (Table 1).
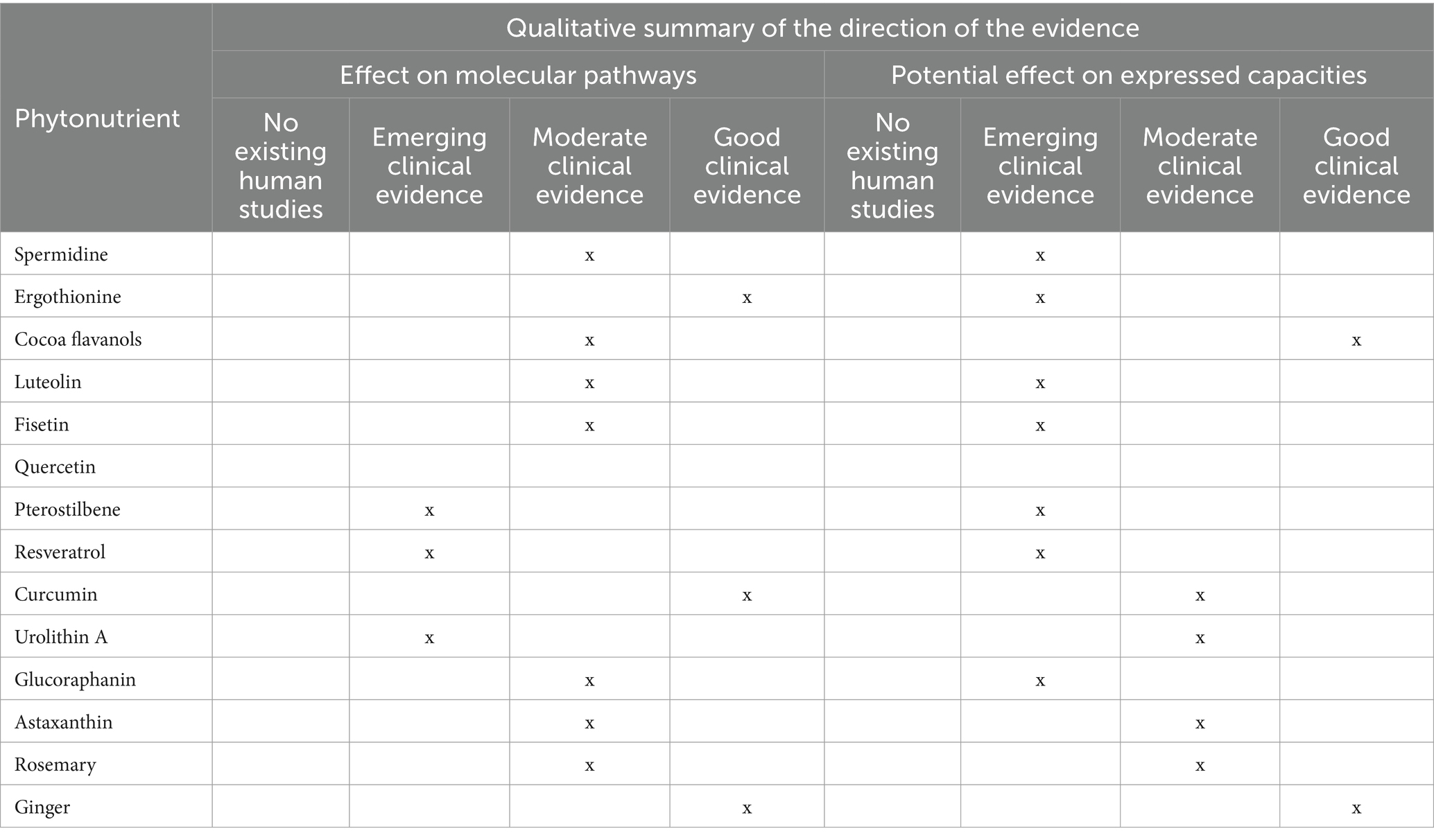
Table 1. A qualitative summary of the direction of the evidence in relation to molecular pathways in aging and the potential to influence expressed capacities in aging.
Emphasizing a diet rich in plant foods to provide bioactive compounds could become a cornerstone of public health strategies aimed at the aging population (10, 319). Adopting nutritional guidelines that promote such diets has the potential to enhance functional health, decrease comorbidity, and improve overall well-being. Although further clinical research is needed, the use of carefully designed supplements, with adequate safety evaluations, containing standardized, bioavailable extracts may prove helpful in ensuring efficacious doses are consumed. Meanwhile, improving diets by emphasizing plant foods, or Mediterranean-style dietary patterns could lead to reduced healthcare costs and improved quality of life for seniors, aligning healthcare systems and practitioners more closely with the preventive and holistic perspective that the Beard et al. (18, 34) and Bautman et al. (7) advocate.
7.1 Crosstalk in molecular mechanisms of action
Integrating the effects of these phytonutrients within the intrinsic capacity framework, it is evident that their roles in healthy aging are interdependent and multifaceted. The cross-over in their mechanisms of action reflects a complex interplay that could potentially support overall vitality. Amino acids such as spermidine and amines like ergothioneine engage in enhancing autophagy and reducing oxidative stress, respectively, sharing common pathways such as the upregulation of sirtuin activity. Polyphenols, including resveratrol, cocoa flavanols, luteolin, fisetin, quercetin, curcumin, and pterostilbene, exhibit overlapping mechanisms like the modulation of the NF-kB pathway, indicating a collective impact on inflammation, cellular senescence, and metabolic regulation (40, 42, 48, 90, 93, 99, 320). The plant extracts from rosemary and glucoraphanin, as well as other nutrients like astaxanthin and urolithin A, contribute to these shared pathways, reinforcing antioxidant defenses, and improving mitochondrial function. The convergence of their effects on molecular targets like mTOR, AMPK, and Nrf2 underscores the potential of a diet rich in these compounds to combat the complex process of aging.
This complex interplay of phytonutrient effects enhances the theoretical framework’s focus on maintaining intrinsic capacity and functional ability, offering a holistic approach to the multifactorial nature of aging. Nutritional interventions that incorporate a range of bioactive compounds could potentially address the interconnected cellular pathways implicated in physiological decline. By doing so, such interventions may serve as a preventive strategy against the cascade of age-related changes. The potential of these compounds to collectively target longevity-regulating pathways suggests that a diet rich in phytonutrients could be a cornerstone for preventive health strategies, aiming to reduce the rate of physiological decline and maintain quality of life in aging populations. Continued research is essential to fully understand the dosage, combinations, and long-term impact of these nutritional interventions.
7.2 Reflection on phytonutrients and higher physiological systems
Reflecting on the potential biomarkers of vitality capacity across various physiological systems, such as energy and metabolism, neuromuscular function and immune and stress response, aids in elucidating the potential impact of various bioactive compounds on aging (Figure 4) (7).
Spermidine’s ability to enhance autophagy could theoretically improve metabolic functions and reduce fatigue by clearing damaged proteins and organelles (63). Ergothioneine is recognized for its strong antioxidant properties (76, 89). Thus, higher dietary ergothioneine intake is associated with lower CVD risk in epidemiological studies (86). On the other hand, polyphenols have been largely studied for their antioxidant properties and their effects on vasodilation, energy metabolism and adiposity. (40, 92, 93). GPh reduces LDL cholesterol and improves liver function, indicative of its potential impact on metabolism and antioxidant capacity (229). Astaxanthin can reduce oxidative stress markers and improve lipid profiles, influencing energy metabolism positively (249). UroA may improve mitochondrial health and reduce markers of mitochondrial dysfunction, with associated potential benefits for energy metabolism (218, 219, 223).
In terms of neuromuscular function, polyphenols, especially resveratrol, have been linked to improved muscle strength and function, potentially through their effects on mitochondrial biogenesis and inflammation (92, 101, 144). Moreover, potential benefits for neuroprotection and cognitive health are indicated for resveratrol, cocoa flavanols, curcumin and rosemary extracts, but require further validation in human studies (115, 147, 175, 272). Rosemary’s active compounds as well as curcumin, have demonstrated strong anti-inflammatory effects in some human trials, potentially impacting immune status and stress response (199, 211, 214, 257, 272). There is also moderate evidence for glucoraphanin, astaxanthin and urolithin A to modulate the immune system.
The gaps in understanding the impact of phytonutrients on vitality capacity largely revolve around the complexity of human metabolism and the multifaceted nature of aging. While certain phytonutrients have been linked to improvements in metabolism and inflammation biomarkers the translation to clinically significant outcomes require further investigation. The potential for phytonutrients to impact the attributes of vitality capacity is promising, with several bioactive compounds showing synergistic effects on various physiological systems and some expressed capacities. They may offer non-pharmacological strategies to support healthspan through diet or supplementation. However, realizing this potential requires addressing the aforementioned gaps through well-designed clinical trials, longer study durations, larger and more diverse populations, and standardized outcome measures.
7.3 Future directions
Transitioning from the traditional medical paradigm, which primarily focuses on reactive treatment of diseases after symptoms manifest, to a preventive model of care is imperative (15). In this paradigm shift, the emphasis lies on preventive interventions and lifestyle changes to avert future diseases and mitigate physiological decline. Long-term studies are essential to track the aging process accurately; however, they are costly, require sustained funding, and may encounter participant attrition, potentially affecting the consistency of the data. The use of biomarkers could provide more accessible means of monitoring aging, enabling the evaluation of interventions without the need for extensive longitudinal studies (321, 322). Investigating how dietary components like phytonutrients interact with identified biomarkers could advance our understanding of their role in promoting intrinsic capacity.
To effectively preserve intrinsic capacity and vitality in aging, targeting specific biomarkers within panels categorized into physical, cognitive, and metabolic health is crucial. For physical capacity, biomarkers like creatinine and serum cystatin C could be potential candidates as they indicate muscle mass and function (323). Cognitive health might be assessed through brain-derived neurotrophic factor (BDNF) and homocysteine, which are key indicators of neural health and cognitive function (324). Metabolic health is evaluated via insulin sensitivity and lipid profiles, including HDL and LDL cholesterol (325, 326).
Among these, certain biomarkers are particularly likely to be modulated by nutrition: antioxidative markers such as glutathione peroxidase and superoxide dismutase in the physical panel, influenced by dietary antioxidants (327); anti-inflammatory markers like C-reactive protein (CRP) and interleukin-6 (IL-6) within the cognitive realm, modulated by omega-3 fatty acids and polyphenols (328); and Vitamin D levels, vital for both physical and metabolic health, enhanced by dietary intake or supplementation (329). These nutrition-sensitive biomarkers may facilitate a targeted approach to dietary interventions aimed at enhancing overall health and longevity.
Adding a holistic perspective, the systematic review by Leitão et al. (330) addressed how different dietary patterns, particularly those low in carbohydrates and rich in vegetables, fruits, nuts, cereals, fish, and unsaturated fats, not only influence these biomarkers but also play a crucial role in decreasing CVD and obesity risks and protecting brain health (330). This integration underscores the necessity of considering the broad impact of diet when evaluating biomarkers for aging, to formulate nutritional strategies that effectively support preservation of intrinsic capacity and vitality in aging populations. This may pave the way for dietary guidelines and interventions targeting longevity and vitality.
Future studies may also shed light on how these compounds interact at the cellular and molecular levels and improve our understanding of potential combined effects on aging processes. Future research should also explore the dose–response relationships of phytonutrients in clinical settings to optimize dietary recommendations. Exploring the synergy and interaction between different phytonutrients could lead to more effective dietary strategies for aging populations. Identifying which phytonutrients work best together might further enhance their beneficial effects on health.
Therapeutic algorithms may provide a systematic framework for integrating and monitoring the use of phytonutrients alongside established pharmaceutical treatments in managing age-related diseases. Given the complexity of interactions between various compounds and medications, these algorithms can guide healthcare providers in customizing treatment plans that capitalize on the benefits of both natural compounds and conventional medicine while mitigating potential risks. For example, in mice supplementation of 0.05% trans-resveratrol did not interact with warfarin, whereas 0.5% trans-resveratrol enhanced the anticoagulant activity of warfarin (331). Moreover, there is theoretical concern that astaxanthin could interfere with the metabolism of statins prescribed for treatment of cardiovascular diseases. However, other studies have found that astaxanthin could be used as an alternative therapy in statin intolerant patients (332). Through continuous evaluation of potential interactions and effectiveness, therapeutic algorithms could enable healthcare professionals to optimize treatment plans—balancing benefits and risks—thereby enhancing patient outcomes in age-related conditions.
Lastly, personalized nutrition, focusing on tailoring dietary recommendations or nutrient supply based on individual genetic, metabolic, and microbiome profiles, offers a promising avenue for optimizing the efficacy of phytonutrient interventions in healthy aging. This approach could help identify which individuals are most likely to benefit from specific phytonutrients or combinations thereof, based on their unique biological characteristics. By examining genetic markers related to nutrient metabolism and the gut microbiota’s role in phytonutrient bioavailability and effects, personalized nutrition could enhance health outcomes and prevent age-related diseases more effectively.
8 Conclusion
In conclusion, our narrative review reinforces the notion that diets, rich in plant foods providing phytonutrients, have the potential to positively influence the aging trajectory. Furthermore, emerging data highlights the potential of phytonutrients as a future intervention to enhance vitality and intrinsic capacity in aging, due to their ability to modulate cellular hallmarks of aging. This highlights the need for a paradigm shift toward preventive nutrition, integrating nutrigerontology into mainstream healthcare practices to support the health and well-being of the aging global population.
Author contributions
EJ: Conceptualization, Data curation, Formal analysis, Investigation, Methodology, Software, Visualization, Writing – original draft, Writing – review & editing. AK: Conceptualization, Data curation, Methodology, Writing – original draft, Writing – review & editing, Formal analysis. DM: Data curation, Validation, Visualization, Writing – review & editing, Formal analysis. NC: Writing – review & editing. JH: Conceptualization, Writing – review & editing. CH: Conceptualization, Writing – review & editing. MK: Conceptualization, Methodology, Writing – original draft, Writing – review & editing. CL: Writing – review & editing. AR: Conceptualization, Writing – original draft, Writing – review & editing.
Funding
The author(s) declare that financial support was received for the research, authorship, and/or publication of this article. This work was funded by Amway Innovation and Science.
Conflict of interest
EJ is a paid consultant to Amway Innovation and Science. AK is a consulting partner to EJ. NC, CH, JH, MK, CL, and AR are employees of Amway Innovation and Science. The funder had the following involvement in this review article: review of the design, preparation and review of the manuscript and decision to publish.
The remaining author declares that the research was conducted in the absence of any commercial or financial relationships that could be construed as a potential conflict of interest.
The author(s) declared that they were an editorial board member of Frontiers, at the time of submission. This had no impact on the peer review process and the final decision.
Publisher’s note
All claims expressed in this article are solely those of the authors and do not necessarily represent those of their affiliated organizations, or those of the publisher, the editors and the reviewers. Any product that may be evaluated in this article, or claim that may be made by its manufacturer, is not guaranteed or endorsed by the publisher.
References
1. Ritchie, H. The world population is changing: for the first time there are more people over 64 than children younger than 5. UN, World Population Prospects (2022).
2. Murray, CJ, Barber, RM, Foreman, KJ, Ozgoren, AA, Abd-Allah, F, Abera, SF, et al. Global, regional, and National Disability-Adjusted Life Years (Dalys) for 306 diseases and injuries and healthy life expectancy (Hale) for 188 countries, 1990–2013: quantifying the epidemiological transition. Lancet. (2015) 386:2145–91. doi: 10.1016/S0140-6736(15)61340-X
3. Kaeberlein, M . How healthy is the Healthspan concept? GeroScience. (2018) 40:361–4. doi: 10.1007/s11357-018-0036-9
4. Dominguez, LJ, Veronese, N, Baiamonte, E, Guarrera, M, Parisi, A, Ruffolo, C, et al. Healthy aging and dietary patterns. Nutrients. (2022) 14:889. doi: 10.3390/nu14040889
5. Black, M, and Bowman, M. Nutrition and healthy aging. Clin Geriatr Med. (2020) 36:655–69. doi: 10.1016/j.cger.2020.06.008
6. Cesari, M, Araujo de Carvalho, I, Amuthavalli Thiyagarajan, J, Cooper, C, Martin, FC, Reginster, J-Y, et al. Evidence for the domains supporting the construct of intrinsic capacity. J Gerontol A. (2018) 73:1653–60. doi: 10.1093/gerona/gly011
7. Bautmans, I, Knoop, V, Amuthavalli Thiyagarajan, J, Maier, AB, Beard, JR, Freiberger, E, et al. Who working definition of vitality capacity for healthy longevity monitoring. Lancet Healthy Longev. (2022) 3:e789–96. doi: 10.1016/s2666-7568(22)00200-8
8. Robinson, SM, Reginster, JY, Rizzoli, R, Shaw, SC, Kanis, JA, Bautmans, I, et al. Does nutrition play a role in the prevention and Management of Sarcopenia? Clin Nutr. (2018) 37:1121–32. doi: 10.1016/j.clnu.2017.08.016
9. Cesari, M, Sadana, R, Sumi, Y, Amuthavalli Thiyagarajan, J, and Banerjee, A. What is intrinsic capacity and why should nutrition be included in the vitality domain? J Gerontol A. (2022) 77:91–3. doi: 10.1093/gerona/glab318
10. Yeung, SSY, Kwan, M, and Woo, J. Healthy diet for healthy aging. Nutrients. (2021) 13:4310. doi: 10.3390/nu13124310
11. Beecher, GR . Phytonutrients' role in metabolism: effects on resistance to degenerative processes. Nutr Rev. (2009) 57:3–6. doi: 10.1111/j.1753-4887.1999.tb01800.x
12. Gupta, C, and Prakash, D. Phytonutrients as therapeutic agents. J Complemen Integr Med. (2014) 11:151–69. doi: 10.1515/jcim-2013-0021
13. Fekete, M, Szarvas, Z, Fazekas-Pongor, V, Feher, A, Csipo, T, Forrai, J, et al. Nutrition strategies promoting healthy aging: from improvement of cardiovascular and brain health to prevention of age-associated diseases. Nutrients. (2022) 15:47. doi: 10.3390/nu15010047
14. Roberts, SB, Silver, RE, Das, SK, Fielding, RA, Gilhooly, CH, Jacques, PF, et al. Healthy aging—nutrition matters: start early and screen often. Adv Nutr. (2021) 12:1438–48. doi: 10.1093/advances/nmab032
15. Moskalev, A, Guvatova, Z, Lopes, IDA, Beckett, CW, Kennedy, BK, De Magalhaes, JP, et al. Targeting aging mechanisms: pharmacological perspectives. Trends Endocrinol Metab. (2022) 33:266–80. doi: 10.1016/j.tem.2022.01.007
16. Frank, J, Fukagawa, NK, Bilia, AR, Johnson, EJ, Kwon, O, Prakash, V, et al. Terms and nomenclature used for plant-derived components in nutrition and related research: efforts toward harmonization. Nutr Rev. (2020) 78:451–8. doi: 10.1093/nutrit/nuz081
17. Monjotin, N, Amiot, MJ, Fleurentin, J, Morel, JM, and Raynal, S. Clinical evidence of the benefits of phytonutrients in human healthcare. Nutrients. (2022) 14:1712. doi: 10.3390/nu14091712
18. Beard, JR, Si, Y, Liu, Z, Chenoweth, L, and Hanewald, K. Intrinsic capacity: validation of a new who concept for healthy aging in a longitudinal Chinese study. J Gerontol A. (2021) 77:94–100. doi: 10.1093/gerona/glab226
19. Nielsen, JL, Bakula, D, and Scheibye-Knudsen, M. Clinical trials targeting aging. Front Aging. (2022) 3:820215. doi: 10.3389/fragi.2022.820215
20. López-Otín, C, Blasco, MA, Partridge, L, Serrano, M, and Kroemer, G. The hallmarks of aging. Cell. (2013) 153:1194–217. doi: 10.1016/j.cell.2013.05.039
21. Bosco, N, and Noti, M. The aging gut microbiome and its impact on host immunity. Genes Immun. (2021) 22:289–303. doi: 10.1038/s41435-021-00126-8
22. Kassis, A, Fichot, MC, Horcajada, MN, Horstman, AMH, Duncan, P, Bergonzelli, G, et al. Nutritional and lifestyle management of the aging journey: a narrative review. Front Nutr. (2022) 9:1087505. doi: 10.3389/fnut.2022.1087505
23. Jishi, A, and Qi, X. Altered mitochondrial protein homeostasis and proteinopathies. Front Mol Neurosci. (2022) 15:867935. doi: 10.3389/fnmol.2022.867935
24. Reuter-Lorenz, PA, and Lustig, C. Brain aging: reorganizing discoveries about the aging mind. Curr Opin Neurobiol. (2005) 15:245–51. doi: 10.1016/j.conb.2005.03.016
25. Azzolino, D, Spolidoro, GCI, Saporiti, E, Luchetti, C, Agostoni, C, and Cesari, M. Musculoskeletal changes across the lifespan: nutrition and the life-course approach to prevention. Front Med. (2021) 8:697954. doi: 10.3389/fmed.2021.697954
26. Divo, MJ, Martinez, CH, and Mannino, DM. Ageing and the epidemiology of multimorbidity. Eur Respir J. (2014) 44:1055–68. doi: 10.1183/09031936.00059814
27. Tian, YE, Cropley, V, Maier, AB, Lautenschlager, NT, Breakspear, M, and Zalesky, A. Heterogeneous aging across multiple organ systems and prediction of chronic disease and mortality. Nat Med. (2023) 29:1221–31. doi: 10.1038/s41591-023-02296-6
28. Benzing, T, and Schumacher, B. Chronic kidney disease promotes ageing in a multiorgan disease network. Nat Rev Nephrol. (2023) 19:542–3. doi: 10.1038/s41581-023-00729-6
29. Kennedy, BK, Berger, SL, Brunet, A, Campisi, J, Cuervo, AM, Epel, ES, et al. Geroscience: linking aging to chronic disease. Cell. (2014) 159:709–13. doi: 10.1016/j.cell.2014.10.039
30. World Health Organization . World report on ageing and health. Geneva: World Health Organization (2015).
31. Bojang, KP, and Manchana, V. Nutrition and healthy aging: a review. Curr Nutr Rep. (2023) 12:369–75. doi: 10.1007/s13668-023-00473-0
32. Seals, DR, Justice, JN, and Larocca, TJ. Physiological geroscience: targeting function to increase healthspan and achieve optimal longevity. J Physiol. (2016) 594:2001–24. doi: 10.1113/jphysiol.2014.282665
33. Verburgh, K . Nutrigerontology: why we need a new scientific discipline to develop diets and guidelines to reduce the risk of aging-related diseases. Aging Cell. (2015) 14:17–24. doi: 10.1111/acel.12284
34. Beard, JR, Jotheeswaran, AT, Cesari, M, and Carvalho, IA. The structure and predictive value of intrinsic capacity in a longitudinal study of ageing. BMJ Open. (2019) 9:e026119. doi: 10.1136/bmjopen-2018-026119
35. Beard, JR, Officer, A, De Carvalho, IA, Sadana, R, Pot, AM, Michel, J-P, et al. The world report on ageing and health: a policy framework for healthy ageing. Lancet. (2016) 387:2145–54. doi: 10.1016/s0140-6736(15)00516-4
36. Lo, SM, Gardner, GE, Reid, J, Napoleon-Fanis, V, Carroll, P, Smith, E, et al. Prevailing questions and methodologies in biology education research: a longitudinal analysis of research in Cbe-life sciences education and at the Society for the Advancement of biology education research. CBE Life Sci Educ. (2019) 18:ar9. doi: 10.1187/cbe.18-08-0164
37. Medawar, E, Huhn, S, Villringer, A, and Veronica, WA. The effects of plant-based diets on the body and the brain: a systematic review. Transl Psychiatry. (2019) 9:226. doi: 10.1038/s41398-019-0552-0
38. Crous-Bou, M, Molinuevo, JL, and Sala-Vila, A. Plant-rich dietary patterns, plant foods and nutrients, and telomere length. Adv Nutr. (2019) 10:S296–303. doi: 10.1093/advances/nmz026
39. Manach, C, Milenkovic, D, Van de Wiele, T, Rodriguez-Mateos, A, de Roos, B, Garcia-Conesa, MT, et al. Addressing the inter-individual variation in response to consumption of plant food bioactives: towards a better understanding of their role in healthy aging and cardiometabolic risk reduction. Mol Nutr Food Res. (2017) 61:1600557. doi: 10.1002/mnfr.201600557
40. Leri, M, Scuto, M, Ontario, ML, Calabrese, V, Calabrese, EJ, Bucciantini, M, et al. Healthy effects of plant polyphenols: molecular mechanisms. Int J Mol Sci. (2020) 21:1250. doi: 10.3390/ijms21041250
41. Ames, BN . Prolonging healthy aging: longevity vitamins and proteins. Proc Natl Acad Sci USA. (2018) 115:10836–44. doi: 10.1073/pnas.1809045115
42. Peng, C, Wang, X, Chen, J, Jiao, R, Wang, L, Li, YM, et al. Biology of ageing and role of dietary antioxidants. Biomed Res Int. (2014) 2014:831841:1–13. doi: 10.1155/2014/831841
43. Luo, M, Mai, M, Song, W, Yuan, Q, Feng, X, Xia, E, et al. The antiaging activities of phytochemicals in dark-colored plant foods: involvement of the autophagy- and apoptosis-associated pathways. Int J Mol Sci. (2022) 23:11038. doi: 10.3390/ijms231911038
44. Zhou, DD, Luo, M, Huang, SY, Saimaiti, A, Shang, A, Gan, RY, et al. Effects and mechanisms of resveratrol on aging and age-related diseases. Oxidative Med Cell Longev. (2021) 2021:9932218–5. doi: 10.1155/2021/9932218
45. Alegre, GFS, and Pastore, GM. NAD+ precursors nicotinamide mononucleotide (NMN) and nicotinamide riboside (NR): potential dietary contribution to health. Curr Nutr Rep. (2023) 12:445–64. doi: 10.1007/s13668-023-00475-y
46. Poljsak, B, Kovač, V, and Milisav, I. Healthy lifestyle recommendations: do the beneficial effects originate from NAD(+) amount at the cellular level? Oxidative Med Cell Longev. (2020) 2020:8819627. doi: 10.1155/2020/8819627
47. López-Otín, C, Blasco, MA, Partridge, L, Serrano, M, and Kroemer, G. Hallmarks of aging: an expanding universe. Cell. (2023) 186:243–78. doi: 10.1016/j.cell.2022.11.001
48. Pereira, QC, Dos Santos, TW, Fortunato, IM, and Ribeiro, ML. The molecular mechanism of polyphenols in the regulation of ageing hallmarks. Int J Mol Sci. (2023) 24:5508. doi: 10.3390/ijms24065508
49. Fan, X, Fan, Z, Yang, Z, Huang, T, Tong, Y, Yang, D, et al. Flavonoids-natural gifts to promote health and longevity. Int J Mol Sci. (2022) 23:2176. doi: 10.3390/ijms23042176
50. Park, SJ, Ahmad, F, Philp, A, Baar, K, Williams, T, Luo, H, et al. Resveratrol ameliorates aging-related metabolic phenotypes by inhibiting camp phosphodiesterases. Cell. (2012) 148:421–33. doi: 10.1016/j.cell.2012.01.017
51. Howitz, KT, Bitterman, KJ, Cohen, HY, Lamming, DW, Lavu, S, Wood, JG, et al. Small molecule activators of Sirtuins extend Saccharomyces cerevisiae lifespan. Nature. (2003) 425:191–6. doi: 10.1038/nature01960
52. Zhu, Y, Tchkonia, T, Pirtskhalava, T, Gower, AC, Ding, H, Giorgadze, N, et al. The Achilles’ heel of senescent cells: from transcriptome to Senolytic drugs. Aging Cell. (2015) 14:644–58. doi: 10.1111/acel.12344
53. Yousefzadeh, MJ, Zhu, Y, McGowan, SJ, Angelini, L, Fuhrmann-Stroissnigg, H, Xu, M, et al. Fisetin is a senotherapeutic that extends health and lifespan. EBioMedicine. (2018) 36:18–28. doi: 10.1016/j.ebiom.2018.09.015
54. Fulop, T, Larbi, A, Dupuis, G, Le Page, A, Frost, EH, Cohen, AA, et al. Immunosenescence and inflamm-aging as two sides of the same coin: friends or foes? Front Immunol. (2018) 8:8. doi: 10.3389/fimmu.2017.01960
55. Cuevas, A, Saavedra, N, Salazar, LA, and Abdalla, DS. Modulation of immune function by polyphenols: possible contribution of epigenetic factors. Nutrients. (2013) 5:2314–32. doi: 10.3390/nu5072314
56. Vaiserman, AM, Koliada, AK, and Marotta, F. Gut microbiota: a player in aging and a target for anti-aging intervention. Ageing Res Rev. (2017) 35:36–45. doi: 10.1016/j.arr.2017.01.001
57. Sharma, R, and Padwad, Y. Perspectives of the potential implications of polyphenols in influencing the interrelationship between oxiinflammatory stress, cellular senescence and immunosenescence during aging. Trends Food Sci Technol. (2020) 98:41–52. doi: 10.1016/j.tifs.2020.02.004
58. Man, AWC, Zhou, Y, Xia, N, and Li, H. Involvement of gut microbiota, microbial metabolites and interaction with polyphenol in host immunometabolism. Nutrients. (2020) 12:3054. doi: 10.3390/nu12103054
59. Frank, SM, Webster, J, McKenzie, B, Geldsetzer, P, Manne-Goehler, J, Andall-Brereton, G, et al. Consumption of fruits and vegetables among individuals 15 years and older in 28 low- and middle-income countries. J Nutr. (2019) 149:1252–9. doi: 10.1093/jn/nxz040
60. Aune, D, Giovannucci, E, Boffetta, P, Fadnes, LT, Keum, N, Norat, T, et al. Fruit and vegetable intake and the risk of cardiovascular disease, total cancer and all-cause mortality-a systematic review and dose-response meta-analysis of prospective studies. Int J Epidemiol. (2017) 46:1029–56. doi: 10.1093/ije/dyw319
61. Chan, HHL, Ribeiro, RV, Haden, S, and Hirani, V. Plant-based dietary patterns, body composition, muscle strength and function in middle and older age: a systematic review. J Nutr Health Aging. (2021) 25:1012–22. doi: 10.1007/s12603-021-1666-7
62. Shlisky, J, Bloom, DE, Beaudreault, AR, Tucker, KL, Keller, HH, Freund-Levi, Y, et al. Nutritional considerations for healthy aging and reduction in age-related chronic disease. Adv Nutr. (2017) 8:17–26. doi: 10.3945/an.116.013474
63. Madeo, F, Eisenberg, T, Pietrocola, F, and Kroemer, G. Spermidine in health and disease. Science. (2018) 359:6374. doi: 10.1126/science.aan2788
64. Atiya Ali, M, Poortvliet, E, Strömberg, R, and Yngve, A. PolyAmines in foods: development of a food database. Food Nutr Res (2011):55–55. doi: 10.3402/fnr.v55i0.5572
65. Pekar, T, Bruckner, K, Pauschenwein-Frantsich, S, Gschaider, A, Oppliger, M, Willesberger, J, et al. The positive effect of spermidine in older adults suffering from dementia: first results of a 3-month trial. Wien Klin Wochenschr. (2021) 133:484–91. doi: 10.1007/s00508-020-01758-y
66. Milovic, V . Polyamines in the gut lumen: bioavailability and biodistribution. Eur J Gastroenterol Hepatol. (2001) 13:1021–5. doi: 10.1097/00042737-200109000-00004
67. Minois, N, Carmona-Gutierrez, D, and Madeo, F. Polyamines in aging and disease. Aging. (2011) 3:716–32. doi: 10.18632/aging.100361
68. Pucciarelli, S, Moreschini, B, Micozzi, D, De Fronzo, GS, Carpi, FM, Polzonetti, V, et al. Spermidine and Spermine are enriched in whole blood of Nona/centenarians. Rejuvenation Res. (2012) 15:590–5. doi: 10.1089/rej.2012.1349
69. Eisenberg, T, Abdellatif, M, Schroeder, S, Primessnig, U, Stekovic, S, Pendl, T, et al. Cardioprotection and lifespan extension by the natural polyamine spermidine. Nat Med. (2016) 22:1428–38. doi: 10.1038/nm.4222
70. Kiechl, S, Pechlaner, R, Willeit, P, Notdurfter, M, Paulweber, B, Willeit, K, et al. Higher spermidine intake is linked to lower mortality: a prospective population-based study. Am J Clin Nutr. (2018) 108:371–80. doi: 10.1093/ajcn/nqy102
71. Wu, H, Wang, J, Jiang, H, Liu, X, Sun, X, Chen, Y, et al. The Association of Dietary Spermidine with all-cause mortality and CVD mortality: the U.S. National Health and nutrition examination survey, 2003 to 2014. Front Public Health. (2022) 10:949170. doi: 10.3389/fpubh.2022.949170
72. Wirth, M, Benson, G, Schwarz, C, Köbe, T, Grittner, U, Schmitz, D, et al. The effect of spermidine on memory performance in older adults at risk for dementia: a randomized controlled trial. Cortex. (2018) 109:181–8. doi: 10.1016/j.cortex.2018.09.014
73. Schwarz, C, Benson, GS, Horn, N, Wurdack, K, Grittner, U, Schilling, R, et al. Effects of spermidine supplementation on cognition and biomarkers in older adults with subjective cognitive decline: a randomized clinical trial. JAMA Netw Open. (2022) 5:e2213875. doi: 10.1001/jamanetworkopen.2022.13875
74. Franzoni, F, Colognato, R, Galetta, F, Laurenza, I, Barsotti, M, Di Stefano, R, et al. An in vitro study on the free radical scavenging capacity of Ergothioneine: comparison with reduced glutathione, uric acid and Trolox. Biomed Pharmacother. (2006) 60:453–7. doi: 10.1016/j.biopha.2006.07.015
75. Paul, BD . Ergothioneine: a stress vitamin with antiaging, vascular, and neuroprotective roles? Antioxid Redox Signal. (2022) 36:1306–17. doi: 10.1089/ars.2021.0043
76. Halliwell, B, Cheah, IK, and Tang, RM. Ergothioneine–a diet-derived antioxidant with therapeutic potential. FEBS Lett. (2018) 592:3357–66. doi: 10.1002/1873-3468.13123
77. Laurenza, I, Colognato, R, Migliore, L, Del Prato, S, and Benzi, L. Modulation of palmitic acid-induced cell death by Ergothioneine: evidence of an anti-inflammatory action. Biofactors. (2008) 33:237–47. doi: 10.1002/biof.5520330401
78. Moncaster, JA, Walsh, DT, Gentleman, SM, Jen, L-S, and Aruoma, OI. Ergothioneine treatment protects neurons against N-methyl-D-aspartate excitotoxicity in an in Vivo rat retinal model. Neurosci Lett. (2002) 328:55–9. doi: 10.1016/S0304-3940(02)00427-5
79. Ey, J, Schömig, E, and Taubert, D. Dietary sources and antioxidant effects of Ergothioneine. J Agric Food Chem. (2007) 55:6466–74. doi: 10.1021/jf071328f
80. Li, X, Feng, X, Sun, X, Hou, N, Han, F, and Liu, Y. Global, regional, and National Burden of Alzheimer's disease and other dementias, 1990-2019. Front Aging Neurosci. (2022) 14:937486. doi: 10.3389/fnagi.2022.937486
81. Tschirka, J, Kreisor, M, Betz, J, and Gründemann, D. Substrate selectivity check of the Ergothioneine transporter. Drug Metab Dispos. (2018) 46:779–85. doi: 10.1124/dmd.118.080440
82. Cheah, IK, Tang, RM, Yew, TS, Lim, KH, and Halliwell, B. Administration of Pure Ergothioneine to healthy human subjects: uptake, metabolism, and effects on biomarkers of oxidative damage and inflammation. Antioxid Redox Signal. (2017) 26:193–206. doi: 10.1089/ars.2016.6778
83. Cheah, IK, Feng, L, Tang, RM, Lim, KH, and Halliwell, B. Ergothioneine levels in an elderly population decrease with age and incidence of cognitive decline; a risk factor for neurodegeneration? Biochem Biophys Res Commun. (2016) 478:162–7. doi: 10.1016/j.bbrc.2016.07.074
84. Kondoh, H, Teruya, T, Kameda, M, and Yanagida, M. Decline of Ergothioneine in frailty and cognition impairment. FEBS Lett. (2022) 596:1270–8. doi: 10.1002/1873-3468.14299
85. Ericson, U, Brunkwall, L, Alves Dias, J, Drake, I, Hellstrand, S, Gullberg, B, et al. Food patterns in relation to weight change and incidence of type 2 diabetes, coronary events and stroke in the Malmö diet and Cancer cohort. Eur J Nutr. (2019) 58:1801–14. doi: 10.1007/s00394-018-1727-9
86. Smith, E, Ottosson, F, Hellstrand, S, Ericson, U, Orho-Melander, M, Fernandez, C, et al. Ergothioneine is associated with reduced mortality and decreased risk of cardiovascular disease. Heart. (2020) 106:691–7. doi: 10.1136/heartjnl-2019-315485
87. Weigand-Heller, AJ, Kris-Etherton, PM, and Beelman, RB. The bioavailability of Ergothioneine from mushrooms (Agaricus Bisporus) and the acute effects on antioxidant capacity and biomarkers of inflammation. Prev Med. (2012) 54:S75–8. doi: 10.1016/j.ypmed.2011.12.028
88. Benson, KF, Ager, DM, Landes, B, Aruoma, OI, and Jensen, GS. Improvement of joint range of motion (rom) and reduction of chronic pain after consumption of an Ergothioneine-containing nutritional supplement. Prev Med. (2012) 54:S83–9. doi: 10.1016/j.ypmed.2012.02.001
89. Apparoo, Y, Phan, CW, Kuppusamy, UR, and Sabaratnam, V. Ergothioneine and its prospects as an anti-ageing compound. Exp Gerontol. (2022) 170:111982. doi: 10.1016/j.exger.2022.111982
90. Papuc, C, Goran, GV, Predescu, CN, Nicorescu, V, and Stefan, G. Plant polyphenols as antioxidant and antibacterial agents for shelf-life extension of meat and meat products: classification, structures, sources, and action mechanisms. Compr Rev Food Sci Food Saf. (2017) 16:1243–68. doi: 10.1111/1541-4337.12298
91. Liu, XM, Liu, YJ, Huang, Y, Yu, HJ, Yuan, S, Tang, BW, et al. Dietary Total flavonoids intake and risk of mortality from all causes and cardiovascular disease in the general population: a systematic review and Meta-analysis of cohort studies. Mol Nutr Food Res. (2017) 61. doi: 10.1002/mnfr.201601003
92. Hollman, P, and Arts, I. Polyphenols and disease risk in epidemiological studies. Am J Clin Nutr. (2005) 81:317S–25S. doi: 10.1093/ajcn/81.1.317S
93. Khurana, S, Venkataraman, K, Hollingsworth, A, Piche, M, and Tai, T. Polyphenols: benefits to the cardiovascular system in health and in aging. Nutrients. (2013) 5:3779–827. doi: 10.3390/nu5103779
94. Wu, M, Luo, Q, Nie, R, Yang, X, Tang, Z, and Chen, H. Potential implications of polyphenols on aging considering oxidative stress, inflammation, autophagy, and gut microbiota. Crit Rev Food Sci Nutr. (2021) 61:2175–93. doi: 10.1080/10408398.2020.1773390
95. Dai, D-F, Chiao, YA, Marcinek, DJ, Szeto, HH, and Rabinovitch, PS. Mitochondrial oxidative stress in aging and Healthspan. Long Healthspan. (2014) 3:1–22. doi: 10.1186/2046-2395-3-6
96. Katz, DL, Doughty, K, and Ali, A. Cocoa and chocolate in human health and disease. Antioxid Redox Signal. (2011) 15:2779–811. doi: 10.1089/ars.2010.3697
97. Goya, L, Martín, MÁ, Sarriá, B, Ramos, S, Mateos, R, and Bravo, L. Effect of cocoa and its flavonoids on biomarkers of inflammation: studies of cell culture, animals and humans. Nutrients. (2016) 8:212. doi: 10.3390/nu8040212
98. Wollgast, J, and Anklam, E. Review on polyphenols in Theobroma cacao: changes in composition during the manufacture of chocolate and methodology for identification and quantification. Food Res Int. (2000) 33:423–47. doi: 10.1016/S0963-9969(00)00068-5
99. Williamson, G . The role of polyphenols in modern nutrition. Nutr Bull. (2017) 42:226–35. doi: 10.1111/nbu.12278
100. Kay, CD, Kroon, PA, and Cassidy, A. The bioactivity of dietary anthocyanins is likely to be mediated by their degradation products. Mol Nutr Food Res. (2009) 53 Suppl 1:S92–S101. doi: 10.1002/mnfr.200800461
101. Barrera-Reyes, PK, Hernández-Ramírez, N, Cortés, J, Poquet, L, Redeuil, K, Rangel-Escareño, C, et al. Gene expression changes by high-polyphenols cocoa powder intake: a randomized crossover clinical study. Eur J Nutr. (2019) 58:1887–98. doi: 10.1007/s00394-018-1736-8
102. Waterhouse, AL, Shirley, JR, and Donovan, JL. Antioxidants in chocolate. Lancet. (1996) 348:834. doi: 10.1016/s0140-6736(05)65262-2
103. Lotito, SB, and Frei, B. Consumption of flavonoid-rich foods and increased plasma antioxidant capacity in humans: cause, consequence, or epiphenomenon? Free Radic Biol Med. (2006) 41:1727–46. doi: 10.1016/j.freeradbiomed.2006.04.033
104. Hooper, L, Kay, C, Abdelhamid, A, Kroon, PA, Cohn, JS, Rimm, EB, et al. Effects of chocolate, cocoa, and Flavan-3-Ols on cardiovascular health: a systematic review and Meta-analysis of randomized trials. Am J Clin Nutr. (2012) 95:740–51. doi: 10.3945/ajcn.111.023457
105. Lin, X, Zhang, I, Li, A, Manson, JE, Sesso, HD, Wang, L, et al. Cocoa Flavanol intake and biomarkers for cardiometabolic health: a systematic review and meta-analysis of randomized controlled trials. J Nutr. (2016) 146:2325–33. doi: 10.3945/jn.116.237644
106. Dicks, L, Haddad, Z, Deisling, S, and Ellinger, S. Effect of an (−)-Epicatechin intake on cardiometabolic parameters-a systematic review of randomized controlled trials. Nutrients. (2022) 14:4500. doi: 10.3390/nu14214500
107. Sun, Y, Zimmermann, D, De Castro, CA, and Actis-Goretta, L. Dose–response relationship between cocoa Flavanols and human endothelial function: a systematic review and meta-analysis of randomized trials. Food Funct. (2019) 10:6322–30. doi: 10.1039/C9FO01747J
108. Ried, K, Sullivan, TR, Fakler, P, Frank, OR, and Stocks, NP. Effect of cocoa on blood pressure. Cochrane Database Syst Rev. (2012) 2012:Cd008893. doi: 10.1002/14651858.CD008893.pub2
109. Mehrabani, S, Arab, A, Mohammadi, H, and Amani, R. The effect of cocoa consumption on markers of oxidative stress: a systematic review and meta-analysis of interventional studies. Complement Ther Med. (2020) 48:102240. doi: 10.1016/j.ctim.2019.102240
110. Sesso, HD, Manson, JE, Aragaki, AK, Rist, PM, Johnson, LG, Friedenberg, G, et al. Effect of cocoa Flavanol supplementation for the prevention of cardiovascular disease events: the cocoa supplement and multivitamin outcomes study (Cosmos) randomized clinical trial. Am J Clin Nutr. (2022) 115:1490–500. doi: 10.1093/ajcn/nqac055
111. Grassi, D, Desideri, G, Necozione, S, Lippi, C, Casale, R, Properzi, G, et al. Blood pressure is reduced and insulin sensitivity increased in glucose-intolerant, hypertensive subjects after 15 days of consuming high-polyphenol dark chocolate. J Nutr. (2008) 138:1671–6. doi: 10.1093/jn/138.9.1671
112. Davison, K, Coates, AM, Buckley, JD, and Howe, PR. Effect of cocoa Flavanols and exercise on cardiometabolic risk factors in overweight and obese subjects. Int J Obes. (2008) 32:1289–96. doi: 10.1038/ijo.2008.66
113. Socci, V, Tempesta, D, Desideri, G, De Gennaro, L, and Ferrara, M. Enhancing human cognition with cocoa flavonoids. Front Nutr. (2017) 4:19. doi: 10.3389/fnut.2017.00019
114. Sorond, FA, Lipsitz, LA, Hollenberg, NK, and Fisher, ND. Cerebral blood flow response to Flavanol-rich cocoa in healthy elderly humans. Neuropsychiatr Dis Treat. (2008) 4:433–40. doi: 10.2147/NDT.S2310
115. Mastroiacovo, D, Kwik-Uribe, C, Grassi, D, Necozione, S, Raffaele, A, Pistacchio, L, et al. Cocoa Flavanol consumption improves cognitive function, blood pressure control, and metabolic profile in elderly subjects: the cocoa, cognition, and aging (cocoa) study--a randomized controlled trial. Am J Clin Nutr. (2015) 101:538–48. doi: 10.3945/ajcn.114.092189
116. Desideri, G, Kwik-Uribe, C, Grassi, D, Necozione, S, Ghiadoni, L, Mastroiacovo, D, et al. Benefits in cognitive function, blood pressure, and insulin resistance through cocoa Flavanol consumption in elderly subjects with mild cognitive impairment: the cocoa, cognition, and aging (cocoa) study. Hypertension. (2012) 60:794–801. doi: 10.1161/hypertensionaha.112.193060
117. Garcia-Yu, IA, Garcia-Ortiz, L, Gomez-Marcos, MA, Rodriguez-Sanchez, E, Mora-Simon, S, Maderuelo-Fernandez, JA, et al. Effects of cocoa-rich chocolate on cognitive performance in postmenopausal women. A randomised clinical trial. Nutr Neurosci. (2022) 25:1147–58. doi: 10.1080/1028415x.2020.1840119
118. Crews, WD Jr, Harrison, DW, and Wright, JW. A double-blind, placebo-controlled, randomized trial of the effects of dark chocolate and cocoa on variables associated with neuropsychological functioning and cardiovascular health: clinical findings from a sample of healthy, cognitively intact older adults. Am J Clin Nutr. (2008) 87:872–80. doi: 10.1093/ajcn/87.4.872
119. Yoon, H-S, Kim, JR, Park, GY, Kim, J-E, Lee, DH, Lee, KW, et al. Cocoa Flavanol supplementation influences skin conditions of photo-aged women: a 24-week double-blind, randomized, controlled trial. J Nutr. (2016) 146:46–50. doi: 10.3945/jn.115.217711
120. López-Lázaro, M . Distribution and biological activities of the flavonoid Luteolin. Mini Rev Med Chem. (2009) 9:31–59. doi: 10.2174/138955709787001712
121. Gu, R, Wang, Y, Long, B, Kennelly, E, Wu, S, Liu, B, et al. Prospecting for bioactive constituents from traditional medicinal plants through ethnobotanical approaches. Biol Pharm Bull. (2014) 37:903–15. doi: 10.1248/bpb.b14-00084
122. Gendrisch, F, Esser, PR, Schempp, CM, and Wölfle, U. Luteolin as a modulator of skin aging and inflammation. Biofactors. (2021) 47:170–80. doi: 10.1002/biof.1699
123. Imran, M, Rauf, A, Abu-Izneid, T, Nadeem, M, Shariati, MA, Khan, IA, et al. Luteolin, a flavonoid, as an anticancer agent: a review. Biomed Pharmacother. (2019) 112:108612. doi: 10.1016/j.biopha.2019.108612
124. Aziz, N, Kim, M-Y, and Cho, JY. Anti-inflammatory effects of Luteolin: a review of in vitro, in vivo, and in silico studies. J Ethnopharmacol. (2018) 225:342–58. doi: 10.1016/j.jep.2018.05.019
125. Prasher, P, Sharma, M, Singh, SK, Gulati, M, Chellappan, DK, Zacconi, F, et al. Luteolin: a flavonoid with a multifaceted anticancer potential. Cancer Cell Int. (2022) 22:1–11. doi: 10.1186/s12935-022-02808-3
126. Wang, Z, Zeng, M, Wang, Z, Qin, F, Chen, J, and He, Z. Dietary Luteolin: a narrative review focusing on its pharmacokinetic properties and effects on glycolipid metabolism. J Agric Food Chem. (2021) 69:1441–54. doi: 10.1021/acs.jafc.0c08085
127. Nabavi, SF, Braidy, N, Gortzi, O, Sobarzo-Sanchez, E, Daglia, M, Skalicka-Woźniak, K, et al. Luteolin as an anti-inflammatory and neuroprotective agent: a brief review. Brain Res Bull. (2015) 119:1–11. doi: 10.1016/j.brainresbull.2015.09.002
128. Caporali, S, De Stefano, A, Calabrese, C, Giovannelli, A, Pieri, M, Savini, I, et al. Anti-inflammatory and active biological properties of the plant-derived bioactive compounds Luteolin and Luteolin 7-glucoside. Nutrients. (2022) 14:1155. doi: 10.3390/nu14061155
129. Romanova, D, Vachalkova, A, Cipak, L, Ovesna, Z, and Rauko, P. Study of antioxidant effect of Apigenin, Luteolin and quercetin by DNA protective method. Neoplasma. (2001) 48:104–7.
130. Kwon, Y . Luteolin as a potential preventive and therapeutic candidate for Alzheimer's disease. Exp Gerontol. (2017) 95:39–43. doi: 10.1016/j.exger.2017.05.014
131. Li, L, Luo, W, Qian, Y, Zhu, W, Qian, J, Li, J, et al. Luteolin protects against diabetic cardiomyopathy by inhibiting Nf-Κb-mediated inflammation and activating the Nrf2-mediated antioxidant responses. Phytomedicine. (2019) 59:152774. doi: 10.1016/j.phymed.2018.11.034
132. Daily, JW, Kang, S, and Park, S. Protection against Alzheimer's disease by Luteolin: role of brain glucose regulation, anti-inflammatory activity, and the gut microbiota-liver-brain Axis. Biofactors. (2021) 47:218–31. doi: 10.1002/biof.1703
133. Zhang, W, Li, D, Shan, Y, Tao, Y, Chen, Q, Hu, T, et al. Luteolin intake is negatively associated with all-cause and cardiac mortality among patients with type 2 diabetes mellitus. Diabetol Metab Syndr. (2023) 15:59. doi: 10.1186/s13098-023-01026-9
134. Gates, MA, Tworoger, SS, Hecht, JL, De Vivo, I, Rosner, B, and Hankinson, SE. A prospective study of dietary flavonoid intake and incidence of epithelial ovarian Cancer. Int J Cancer. (2007) 121:2225–32. doi: 10.1002/ijc.22790
135. Hodgin, KS, Donovan, EK, Kekes-Szabo, S, Lin, JC, Feick, J, Massey, RL, et al. A placebo-controlled, Pseudo-randomized, crossover trial of botanical agents for gulf war illness: resveratrol (Polygonum cuspidatum), Luteolin, and Fisetin (Rhus succedanea). Int J Environ Res Public Health. (2021) 18:2483. doi: 10.3390/ijerph18052483
136. Johnson, GJ, Slater, BC, Leis, LA, Rector, TS, and Bach, RR. Blood biomarkers of chronic inflammation in gulf war illness. PLoS One. (2016) 11:e0157855. doi: 10.1371/journal.pone.0157855
137. Baek, Y, Lee, MN, Wu, D, and Pae, M. Luteolin reduces adipose tissue macrophage inflammation and insulin resistance in postmenopausal obese mice. J Nutr Biochem. (2019) 71:72–81. doi: 10.1016/j.jnutbio.2019.06.002
138. Hayasaka, N, Shimizu, N, Komoda, T, Mohri, S, Tsushida, T, Eitsuka, T, et al. Absorption and metabolism of Luteolin in rats and humans in relation to in vitro anti-inflammatory effects. J Agric Food Chem. (2018) 66:11320–9. doi: 10.1021/acs.jafc.8b03273
139. Lall, RK, Syed, DN, Adhami, VM, Khan, MI, and Mukhtar, H. Dietary polyphenols in prevention and treatment of prostate cancer. Int J Mol Sci. (2015) 16:3350–76. doi: 10.3390/ijms16023350
140. Pal, HC, Pearlman, RL, and Afaq, F. Fisetin and its role in chronic diseases. Adv Exp Med Biol. (2016) 928:213–44. doi: 10.1007/978-3-319-41334-1_10
141. Baechle, JJ, Chen, N, Makhijani, P, Winer, S, Furman, D, and Winer, DA. Chronic inflammation and the hallmarks of aging. Mol Metab. (2023) 74:101755. doi: 10.1016/j.molmet.2023.101755
142. Grynkiewicz, G, and Demchuk, OM. New perspectives for Fisetin. Front Chem. (2019) 7:697. doi: 10.3389/fchem.2019.00697
143. Fazel Nabavi, S, Braidy, N, Habtemariam, S, Sureda, A, Manayi, A, and Mohammad, NS. Neuroprotective effects of Fisetin in Alzheimer’s and Parkinson’s diseases: from chemistry to medicine. Curr Top Med Chem. (2016) 16:1910–5. doi: 10.2174/1568026616666160204121725
144. Deepika, MPK . Health benefits of quercetin in age-related diseases. Molecules. (2022) 27:27(8). doi: 10.3390/molecules27082498
145. Chopra, M, Fitzsimons, PE, Strain, JJ, Thurnham, DI, and Howard, AN. Nonalcoholic red wine extract and quercetin inhibit LDL oxidation without affecting plasma antioxidant vitamin and carotenoid concentrations. Clin Chem. (2000) 46:1162–70. doi: 10.1093/clinchem/46.8.1162
146. Sarubbo, F, Ramis, MR, Aparicio, S, Ruiz, L, Esteban, S, Miralles, A, et al. Improving effect of chronic resveratrol treatment on central monoamine synthesis and cognition in aged rats. Age. (2015) 37:9777. doi: 10.1007/s11357-015-9777-x
147. Gocmez, SS, Gacar, N, Utkan, T, Gacar, G, Scarpace, PJ, and Tumer, N. Protective effects of resveratrol on aging-induced cognitive impairment in rats. Neurobiol Learn Mem. (2016) 131:131–6. doi: 10.1016/j.nlm.2016.03.022
148. Wiciński, M, Domanowska, A, Wódkiewicz, E, and Malinowski, B. Neuroprotective properties of resveratrol and its derivatives-influence on potential mechanisms leading to the development of Alzheimer's disease. Int J Mol Sci. (2020) 21:2749. doi: 10.3390/ijms21082749
149. Krishnakumar, IM, Jaja-Chimedza, A, Joseph, A, Balakrishnan, A, Maliakel, B, and Swick, A. Enhanced bioavailability and pharmacokinetics of a novel hybrid-hydrogel formulation of Fisetin orally administered in healthy individuals: a randomised double-blinded comparative crossover study. J Nutr Sci. (2022) 11:11. doi: 10.1017/jns.2022.72
150. Costa, LG, Garrick, JM, Roquè, PJ, and Pellacani, C. Mechanisms of neuroprotection by quercetin: counteracting oxidative stress and more. Oxidative Med Cell Longev. (2016) 2016:2986796–10. doi: 10.1155/2016/2986796
151. Murphy, MM, Barraj, LM, Spungen, JH, Herman, DR, and Randolph, RK. Global assessment of select phytonutrient intakes by level of fruit and vegetable consumption. Br J Nutr. (2014) 112:1004–18. doi: 10.1017/s0007114514001937
152. Boots, AW, Haenen, GR, and Bast, A. Health effects of quercetin: from antioxidant to nutraceutical. Eur J Pharmacol. (2008) 585:325–37. doi: 10.1016/j.ejphar.2008.03.008
153. Boyle, S, Dobson, V, Duthie, SJ, Kyle, J, and Collins, A. Absorption and DNA protective effects of flavonoid glycosides from an onion meal. Eur J Nutr. (2000) 39:213–23. doi: 10.1007/s003940070014
154. McAnlis, G, McEneny, J, Pearce, J, and Young, I. Absorption and antioxidant effects of quercetin from onions, in Man. Eur J Clin Nutr. (1999) 53:92–6. doi: 10.1038/sj.ejcn.1600682
155. Egert, S, Bosy-Westphal, A, Seiberl, J, Kürbitz, C, Settler, U, Plachta-Danielzik, S, et al. Quercetin reduces systolic blood pressure and plasma oxidised low-density lipoprotein concentrations in overweight subjects with a high-cardiovascular disease risk phenotype: a double-blinded, placebo-controlled cross-over study. Br J Nutr. (2009) 102:1065–74. doi: 10.1017/S0007114509359127
156. Sahebkar, A . Effects of quercetin supplementation on lipid profile: a systematic review and Meta-analysis of randomized controlled trials. Crit Rev Food Sci Nutr. (2017) 57:666–76. doi: 10.1080/10408398.2014.948609
157. Hubbard, G, Wolffram, S, Lovegrove, J, and Gibbins, J. Ingestion of quercetin inhibits platelet aggregation and essential components of the collagen-stimulated platelet activation pathway in humans. J Thromb Haemost. (2004) 2:2138–45. doi: 10.1111/j.1538-7836.2004.01067.x
158. Dower, JI, Geleijnse, JM, Gijsbers, L, Schalkwijk, C, Kromhout, D, and Hollman, PC. Supplementation of the pure flavonoids Epicatechin and quercetin affects some biomarkers of endothelial dysfunction and inflammation in (pre) hypertensive adults: a randomized double-blind, placebo-controlled, crossover trial. J Nutr. (2015) 145:1459–63. doi: 10.3945/jn.115.211888
159. Edwards, RL, Lyon, T, Litwin, SE, Rabovsky, A, Symons, JD, and Jalili, T. Quercetin reduces blood pressure in hypertensive subjects. J Nutr. (2007) 137:2405–11. doi: 10.1093/jn/137.11.2405
160. Larson, A, Witman, MA, Guo, Y, Ives, S, Richardson, RS, Bruno, RS, et al. Acute, quercetin-induced reductions in blood pressure in hypertensive individuals are not secondary to lower plasma angiotensin-converting enzyme activity or Endothelin-1: nitric oxide. Nutr Res. (2012) 32:557–64. doi: 10.1016/j.nutres.2012.06.018
161. Serban, MC, Sahebkar, A, Zanchetti, A, Mikhailidis, DP, Howard, G, Antal, D, et al. Effects of quercetin on blood pressure: a systematic review and meta-analysis of randomized controlled trials. J Am Heart Assoc. (2016) 5:e002713. doi: 10.1161/jaha.115.002713
162. Shi, G-J, Li, Y, Cao, Q-H, Wu, H-X, Tang, X-Y, Gao, X-H, et al. In vitro and in vivo evidence that quercetin protects against diabetes and its complications: a systematic review of the literature. Biomed Pharmacother. (2019) 109:1085–99. doi: 10.1016/j.biopha.2018.10.130
163. Yan, L, Vaghari-Tabari, M, Malakoti, F, Moein, S, Qujeq, D, Yousefi, B, et al. Quercetin: an effective polyphenol in alleviating diabetes and diabetic complications. Crit Rev Food Sci Nutr. (2022) 63:9163–86. doi: 10.1080/10408398.2022.2067825
164. Mazloom, Z, Abdollah, ZSM, Dabbaghmanesh, MH, and Rezaianzadeh, A. The effect of quercetin supplementation on oxidative stress, glycemic control, lipid profile and insulin resistance in type 2 diabetes: a randomized clinical trial. (2014).
165. Yu, X, Li, Y, and Mu, X. Effect of quercetin on Pc12 Alzheimer’s disease cell model induced by Aβ 25-35 and its mechanism based on Sirtuin1/Nrf2/Ho-1 pathway. Biomed Res Int. (2020) 2020:1–10. doi: 10.1155/2020/8210578
166. Hwang, J-w, Yao, H, Caito, S, Sundar, IK, and Rahman, I. Redox regulation of Sirt1 in inflammation and cellular senescence. Free Radic Biol Med. (2013) 61:95–110. doi: 10.1016/j.freeradbiomed.2013.03.015
167. Donmez, G, Wang, D, Cohen, DE, and Guarente, L. Retracted: Sirt1 suppresses Β-amyloid production by activating the Α-secretase gene Adam10. Cell. (2010) 142:320–32. doi: 10.1016/j.cell.2010.06.020
168. Alcendor, RR, Gao, S, Zhai, P, Zablocki, D, Holle, E, Yu, X, et al. Sirt1 regulates aging and resistance to oxidative stress in the heart. Circ Res. (2007) 100:1512–21. doi: 10.1161/01.RES.0000267723.65696.4a
169. Nakamura, Y, Watanabe, H, Tanaka, A, Nishihira, J, and Murayama, N. Effect of quercetin glycosides on cognitive functions and cerebral blood flow: a randomized, double-blind, and placebo-controlled study. Eur Rev Med Pharmacol Sci. (2022) 26:8700–12. doi: 10.26355/eurrev_202212_30541
170. Xia, N, Daiber, A, Förstermann, U, and Li, H. Antioxidant effects of resveratrol in the cardiovascular system. Br J Pharmacol. (2017) 174:1633–46. doi: 10.1111/bph.13492
171. Hector, KL, Lagisz, M, and Nakagawa, S. The effect of resveratrol on longevity across species: a meta-analysis. Biol Lett. (2012) 8:790–3. doi: 10.1098/rsbl.2012.0316
172. Baur, JA, and Sinclair, DA. Therapeutic potential of resveratrol: the in Vivo evidence. Nat Rev Drug Discov. (2006) 5:493–506. doi: 10.1038/nrd2060
173. Valenzano, DR, Terzibasi, E, Genade, T, Cattaneo, A, Domenici, L, and Cellerino, A. Resveratrol prolongs lifespan and retards the onset of age-related markers in a short-lived vertebrate. Curr Biol. (2006) 16:296–300. doi: 10.1016/j.cub.2005.12.038
174. Koronowski, KB, Dave, KR, Saul, I, Camarena, V, Thompson, JW, Neumann, JT, et al. Resveratrol preconditioning induces a novel extended window of ischemic tolerance in the mouse brain. Stroke. (2015) 46:2293–8. doi: 10.1161/strokeaha.115.009876
175. Wan, D, Zhou, Y, Wang, K, Hou, Y, Hou, R, and Ye, X. Resveratrol provides neuroprotection by inhibiting Phosphodiesterases and regulating the camp/Ampk/Sirt1 pathway after stroke in rats. Brain Res Bull. (2016) 121:255–62. doi: 10.1016/j.brainresbull.2016.02.011
176. Jiao, F, and Gong, Z. The beneficial roles of Sirt1 in neuroinflammation-related diseases. Oxidative Med Cell Longev. (2020) 2020:1–19. doi: 10.1155/2020/6782872
177. Timmers, S, Konings, E, Bilet, L, Houtkooper, RH, van de Weijer, T, Goossens, GH, et al. Calorie restriction-like effects of 30 days of resveratrol supplementation on energy metabolism and metabolic profile in obese humans. Cell Metab. (2011) 14:612–22. doi: 10.1016/j.cmet.2011.10.002
178. Liu, K, Zhou, R, Wang, B, and Mi, M-T. Effect of resveratrol on glucose control and insulin sensitivity: a meta-analysis of 11 randomized controlled trials. Am J Clin Nutr. (2014) 99:1510–9. doi: 10.3945/ajcn.113.082024
179. Banez, MJ, Geluz, MI, Chandra, A, Hamdan, T, Biswas, OS, Bryan, NS, et al. A systemic review on the antioxidant and anti-inflammatory effects of resveratrol, curcumin, and dietary nitric oxide supplementation on human cardiovascular health. Nutr Res. (2020) 78:11–26. doi: 10.1016/j.nutres.2020.03.002
180. Zheng, X, Liu, Z, Zhang, Y, Lin, Y, Song, J, Zheng, L, et al. Relationship between Myo-inositol supplementary and gestational diabetes mellitus: a meta-analysis. Medicine. (2015) 94:e1604. doi: 10.1097/md.0000000000001604
181. Fogacci, F, Tocci, G, Presta, V, Fratter, A, Borghi, C, and Cicero, AFG. Effect of resveratrol on blood pressure: a systematic review and meta-analysis of randomized, controlled, clinical trials. Crit Rev Food Sci Nutr. (2019) 59:1605–18. doi: 10.1080/10408398.2017.1422480
182. Vesely, O, Baldovska, S, and Kolesarova, A. Enhancing bioavailability of nutraceutically used resveratrol and other Stilbenoids. Nutrients. (2021) 13:3095. doi: 10.3390/nu13093095
183. Rodríguez-Bonilla, P, López-Nicolás, JM, Méndez-Cazorla, L, and García-Carmona, F. Development of a reversed phase high performance liquid chromatography method based on the use of Cyclodextrins as Mobile phase additives to determine Pterostilbene in blueberries. J Chromatogr B Analyt Technol Biomed Life Sci. (2011) 879:1091–7. doi: 10.1016/j.jchromb.2011.03.025
184. Estrela, JM, Ortega, A, Mena, S, Rodriguez, ML, and Asensi, M. Pterostilbene: biomedical applications. Crit Rev Clin Lab Sci. (2013) 50:65–78. doi: 10.3109/10408363.2013.805182
185. Remsberg, CM, Yáñez, JA, Ohgami, Y, Vega-Villa, KR, Rimando, AM, and Davies, NM. Pharmacometrics of Pterostilbene: preclinical pharmacokinetics and metabolism, anticancer, antiinflammatory, antioxidant and analgesic activity. Phytother Res. (2008) 22:169–79. doi: 10.1002/ptr.2277
186. Kapetanovic, IM, Muzzio, M, Huang, Z, Thompson, TN, and McCormick, DL. Pharmacokinetics, oral bioavailability, and metabolic profile of resveratrol and its Dimethylether analog, Pterostilbene, in rats. Cancer Chemother Pharmacol. (2011) 68:593–601. doi: 10.1007/s00280-010-1525-4
187. Bickford, PC, Gould, T, Briederick, L, Chadman, K, Pollock, A, Young, D, et al. Antioxidant-rich diets improve cerebellar physiology and motor learning in aged rats. Brain Res. (2000) 866:211–7. doi: 10.1016/S0006-8993(00)02280-0
188. Andres-Lacueva, C, Shukitt-Hale, B, Galli, RL, Jauregui, O, Lamuela-Raventos, RM, and Joseph, JA. Anthocyanins in aged blueberry-fed rats are found centrally and may enhance memory. Nutr Neurosci. (2005) 8:111–20. doi: 10.1080/10284150500078117
189. Ahmet, I, Spangler, E, Shukitt-Hale, B, Juhaszova, M, Sollott, SJ, Joseph, JA, et al. Blueberry-enriched diet protects rat heart from ischemic damage. PLoS One. (2009) 4:e5954. doi: 10.1371/journal.pone.0005954
190. Youdim, KA, McDonald, J, Kalt, W, and Joseph, JA. Potential role of dietary flavonoids in reducing microvascular endothelium vulnerability to oxidative and inflammatory insults. J Nutr Biochem. (2002) 13:282–8. doi: 10.1016/S0955-2863(01)00221-2
191. Park, E-S, Lim, Y, Hong, J-T, Yoo, H-S, Lee, C-K, Pyo, M-Y, et al. Pterostilbene, a natural Dimethylated analog of resveratrol, inhibits rat aortic vascular smooth muscle cell proliferation by blocking AKT-dependent pathway. Vasc Pharmacol. (2010) 53:61–7. doi: 10.1016/j.vph.2010.04.001
192. Kosuru, R, Vijayakumar, MR, and Singh, S. Preclinical evidence of the therapeutic role of Pterostilbene: focus on the mitochondria. Mitochond Physiol Veg Mol. (2021) 2021:301–15. doi: 10.1016/B978-0-12-821562-3.00033-2
193. McCormack, D, and McFadden, D. A review of Pterostilbene antioxidant activity and disease modification. Oxidative Med Cell Longev. (2013) 2013:575482:1–15. doi: 10.1155/2013/575482
194. Pari, L, and Satheesh, MA. Effect of Pterostilbene on hepatic key enzymes of glucose metabolism in Streptozotocin-and nicotinamide-induced diabetic rats. Life Sci. (2006) 79:641–5. doi: 10.1016/j.lfs.2006.02.036
195. Riche, DM, Riche, KD, Blackshear, CT, McEwen, CL, Sherman, JJ, Wofford, MR, et al. Pterostilbene on metabolic parameters: a randomized, double-blind, and placebo-controlled trial. Evid Based Complement Alternat Med. (2014) 2014:1–8. doi: 10.1155/2014/459165
196. Nagarajan, S, Mohandas, S, Ganesan, K, Xu, B, and Ramkumar, KM. New insights into dietary Pterostilbene: sources, metabolism, and health promotion effects. Molecules. (2022) 27:27(19). doi: 10.3390/molecules27196316
197. Zia, A, Farkhondeh, T, Pourbagher-Shahri, AM, and Samarghandian, S. The role of curcumin in aging and senescence: molecular mechanisms. Biomed Pharmacother. (2021) 134:111119. doi: 10.1016/j.biopha.2020.111119
198. Kou, H, Huang, L, Jin, M, He, Q, Zhang, R, and Ma, J. Effect of curcumin on rheumatoid arthritis: a systematic review and meta-analysis. Front Immunol. (2023) 14:1121655. doi: 10.3389/fimmu.2023.1121655
199. Zeng, L, Yu, G, Hao, W, Yang, K, and Chen, H. The efficacy and safety of Curcuma longa extract and curcumin supplements on osteoarthritis: a systematic review and Meta-analysis. Biosci Rep. (2021) 41:BSR20210817. doi: 10.1042/bsr20210817
200. Altobelli, E, Angeletti, PM, Marziliano, C, Mastrodomenico, M, Giuliani, AR, and Petrocelli, R. Potential therapeutic effects of curcumin on glycemic and lipid profile in uncomplicated type 2 diabetes-a Meta-analysis of randomized controlled trial. Nutrients. (2021) 13:404. doi: 10.3390/nu13020404
201. Kumar, S, Sharma, SK, Mudgal, SK, Gaur, R, Agarwal, R, Singh, H, et al. Comparative effectiveness of six herbs in the management of glycemic status of type 2 diabetes mellitus patients: a systematic review and network meta-analysis of randomized controlled trials. Diabetes Metab Syndr. (2023) 17:102826. doi: 10.1016/j.dsx.2023.102826
202. Liu, Y, Ma, M, and Yuan, Y. The potential of curcumin-based co-delivery systems for applications in the food industry: food preservation, freshness monitoring, and functional food. Food Res Int. (2023) 171:113070. doi: 10.1016/j.foodres.2023.113070
203. Lima, CF, Pereira-Wilson, C, and Rattan, SIS. Curcumin induces Heme Oxygenase-1 in Normal human skin fibroblasts through redox signaling: relevance for anti-aging intervention. Mol Nutr Food Res. (2011) 55:430–42. doi: 10.1002/mnfr.201000221
204. Shen, LR, Parnell, LD, Ordovas, JM, and Lai, CQ. Curcumin and aging. Biofactors. (2013) 39:133–40. doi: 10.1002/biof.1086
205. Qin, S, Huang, L, Gong, J, Shen, S, Huang, J, Tang, Y, et al. Meta-analysis of randomized controlled trials of 4 weeks or longer suggest that curcumin May afford some protection against oxidative stress. Nutr Res. (2018) 60:1–12. doi: 10.1016/j.nutres.2018.08.003
206. Changal, KH, Khan, MS, Bashir, R, and Sheikh, MA. Curcumin preparations can improve flow-mediated dilation and endothelial function: a meta-analysis. Complement Med Res. (2020) 27:272–81. doi: 10.1159/000506180
207. Hallajzadeh, J, Milajerdi, A, Kolahdooz, F, Amirani, E, Mirzaei, H, and Asemi, Z. The effects of curcumin supplementation on endothelial function: a systematic review and meta-analysis of randomized controlled trials. Phytother Res. (2019) 33:2989–95. doi: 10.1002/ptr.6477
208. Saeedi, F, Farkhondeh, T, Roshanravan, B, Amirabadizadeh, A, Ashrafizadeh, M, and Samarghandian, S. Curcumin and blood lipid levels: an updated systematic review and meta-analysis of randomised clinical trials. Arch Physiol Biochem. (2022) 128:1493–502. doi: 10.1080/13813455.2020.1779309
209. Musazadeh, V, Roshanravan, N, Mohammadizadeh, M, Kavyani, Z, Dehghan, P, and Mosharkesh, E. Curcumin as a novel approach in improving lipid profile: an umbrella meta-analysis. Nutr Metab Cardiovasc Dis. (2022) 32:2493–504. doi: 10.1016/j.numecd.2022.07.021
210. Tak, PP, and Firestein, GS. Nf-Kappab: a key role in inflammatory diseases. J Clin Invest. (2001) 107:7–11. doi: 10.1172/jci11830
211. Zeng, L, Yang, T, Yang, K, Yu, G, Li, J, Xiang, W, et al. Efficacy and safety of curcumin and Curcuma longa extract in the treatment of arthritis: a systematic review and Meta-analysis of randomized controlled trial. Front Immunol. (2022) 13:891822. doi: 10.3389/fimmu.2022.891822
212. Panahi, Y, Valizadegan, G, Ahamdi, N, Ganjali, S, Majeed, M, and Sahebkar, A. Curcuminoids plus Piperine improve nonalcoholic fatty liver disease: a clinical trial. J Cell Biochem. (2019) 120:15989–96. doi: 10.1002/jcb.28877
213. Safari, Z, Bagherniya, M, Khoram, Z, Ebrahimi Varzaneh, A, Heidari, Z, Sahebkar, A, et al. The effect of curcumin on anthropometric indices, blood pressure, lipid profiles, fasting blood glucose, liver enzymes, fibrosis, and steatosis in non-alcoholic fatty livers. Front Nutr. (2023) 10:10. doi: 10.3389/fnut.2023.1163950
214. Voulgaropoulou, SD, van Amelsvoort, T, Prickaerts, J, and Vingerhoets, C. The effect of curcumin on cognition in Alzheimer's disease and healthy aging: a systematic review of pre-clinical and clinical studies. Brain Res. (2019) 1725:146476. doi: 10.1016/j.brainres.2019.146476
215. Velagapudi, R, Lepiarz, I, El-Bakoush, A, Katola, FO, Bhatia, H, Fiebich, BL, et al. Induction of autophagy and activation of Sirt-1 deacetylation mechanisms mediate neuroprotection by the pomegranate metabolite Urolithin a in Bv2 microglia and differentiated 3d human neural progenitor cells. Mol Nutr Food Res. (2019) 63:e1801237. doi: 10.1002/mnfr.201801237
216. Liu, CF, Li, XL, Zhang, ZL, Qiu, L, Ding, SX, Xue, JX, et al. Antiaging effects of Urolithin a on replicative senescent human skin fibroblasts. Rejuvenation Res. (2019) 22:191–200. Epub 2018/09/15. doi: 10.1089/rej.2018.2066
217. Lee, HJ, Jung, YH, Choi, GE, Kim, JS, Chae, CW, Lim, JR, et al. Urolithin a suppresses high glucose-induced neuronal Amyloidogenesis by modulating Tgm2-dependent Er-mitochondria contacts and calcium homeostasis. Cell Death Differ. (2021) 28:184–202. doi: 10.1038/s41418-020-0593-1
218. Esselun, C, Theyssen, E, and Eckert, GP. Effects of Urolithin a on mitochondrial parameters in a cellular model of early Alzheimer disease. Int J Mol Sci. (2021) 22:8333. doi: 10.3390/ijms22158333
219. Liu, S, D'Amico, D, Shankland, E, Bhayana, S, Garcia, JM, Aebischer, P, et al. Effect of Urolithin a supplementation on muscle endurance and mitochondrial health in older adults: a randomized clinical trial. JAMA Netw Open. (2022) 5:e2144279. doi: 10.1001/jamanetworkopen.2021.44279
220. Singh, A, D'Amico, D, Andreux, PA, Fouassier, AM, Blanco-Bose, W, Evans, M, et al. Urolithin a improves muscle strength, exercise performance, and biomarkers of mitochondrial health in a randomized trial in middle-aged adults. Cell Rep Med. (2022) 3:100633. doi: 10.1016/j.xcrm.2022.100633
221. Ryu, D, Mouchiroud, L, Andreux, PA, Katsyuba, E, Moullan, N, Nicolet-dit-Félix, AA, et al. Urolithin a induces mitophagy and prolongs lifespan in C. elegans and increases muscle function in rodents. Nat Med. (2016) 22:879–88. doi: 10.1038/nm.4132
222. Liu, W, Yan, F, Xu, Z, Chen, Q, Ren, J, Wang, Q, et al. Urolithin a protects human dermal fibroblasts from Uva-induced Photoaging through Nrf2 activation and Mitophagy. J Photochem Photobiol B. (2022) 232:112462. doi: 10.1016/j.jphotobiol.2022.112462
223. Gong, Z, Huang, J, Xu, B, Ou, Z, Zhang, L, Lin, X, et al. Urolithin a attenuates memory impairment and neuroinflammation in app/Ps1 mice. J Neuroinflammation. (2019) 16:1–13. doi: 10.1186/s12974-019-1450-3
224. Istas, G, Feliciano, RP, Weber, T, Garcia-Villalba, R, Tomas-Barberan, F, Heiss, C, et al. Plasma Urolithin metabolites correlate with improvements in endothelial function after red raspberry consumption: a double-blind randomized controlled trial. Arch Biochem Biophys. (2018) 651:43–51. doi: 10.1016/j.abb.2018.05.016
225. Li, Z, Henning, SM, Lee, RP, Lu, QY, Summanen, PH, Thames, G, et al. Pomegranate extract induces Ellagitannin metabolite formation and changes stool microbiota in healthy volunteers. Food Funct. (2015) 6:2487–95. doi: 10.1039/c5fo00669d
226. Ezzat-Zadeh, Z, Henning, SM, Yang, J, Woo, SL, Lee, R-P, Huang, J, et al. California strawberry consumption increased the abundance of gut microorganisms related to lean body weight, health and longevity in healthy subjects. Nutr Res. (2021) 85:60–70. doi: 10.1016/j.nutres.2020.12.006
227. Favela-González, KM, Hernández-Almanza, AY, and De la Fuente-Salcido, NM. The value of bioactive compounds of cruciferous vegetables (Brassica) as antimicrobials and antioxidants: a review. J Food Biochem. (2020) 44:e13414. doi: 10.1111/jfbc.13414
228. Fahey, JW, Zhang, Y, and Talalay, P. Broccoli sprouts: an exceptionally rich source of inducers of enzymes that protect against chemical carcinogens. Proc Natl Acad Sci USA. (1997) 94:10367–72. doi: 10.1073/pnas.94.19.10367
229. Conzatti, A, Fróes, FC, Schweigert Perry, ID, and Souza, CG. Clinical and molecular evidence of the consumption of broccoli, glucoraphanin and Sulforaphane in humans. Nutr Hosp. (2014) 31:559–69. doi: 10.3305/nh.2015.31.2.7685
230. Rochfort, SJ, and Jones, R. Glucosinolate phytochemicals from broccoli (Brassica oleracea L. Var. Botrytis L.) seeds and their potential health effects. Nuts Seeds Health Dis Prevent. (2011) 2011:253–61. doi: 10.1016/B978-0-12-375688-6.10030-1
231. Sivapalan, T, Melchini, A, Saha, S, Needs, PW, Traka, MH, Tapp, H, et al. Bioavailability of glucoraphanin and Sulforaphane from high-glucoraphanin broccoli. Mol Nutr Food Res. (2018) 62:e1700911. doi: 10.1002/mnfr.201700911
232. James, D, Devaraj, S, Bellur, P, Lakkanna, S, Vicini, J, and Boddupalli, S. Novel concepts of broccoli Sulforaphanes and disease: induction of phase ii antioxidant and detoxification enzymes by enhanced-glucoraphanin broccoli. Nutr Rev. (2012) 70:654–65. doi: 10.1111/j.1753-4887.2012.00532.x
233. Gasper, AV, Al-Janobi, A, Smith, JA, Bacon, JR, Fortun, P, Atherton, C, et al. Glutathione S-transferase M1 polymorphism and metabolism of Sulforaphane from standard and high-Glucosinolate broccoli. Am J Clin Nutr. (2005) 82:1283–91. doi: 10.1093/ajcn/82.6.1283
234. Vanduchova, A, Anzenbacher, P, and Anzenbacherova, E. Isothiocyanate from broccoli, Sulforaphane, and its properties. J Med Food. (2019) 22:121–6. doi: 10.1089/jmf.2018.0024
235. Fahey, JW, and Kensler, TW. The challenges of designing and implementing clinical trials with broccoli sprouts… and turning evidence into public health action. Front Nutr. (2021) 8:648788. doi: 10.3389/fnut.2021.648788
236. Costa-Pérez, A, Núñez-Gómez, V, Baenas, N, Di Pede, G, Achour, M, Manach, C, et al. Systematic review on the metabolic interest of Glucosinolates and their bioactive derivatives for human health. Nutrients. (2023) 15:1424. doi: 10.3390/nu15061424
237. Egner, PA, Chen, JG, Zarth, AT, Ng, DK, Wang, JB, Kensler, KH, et al. Rapid and sustainable Detoxication of airborne pollutants by broccoli sprout beverage: results of a randomized clinical trial in China. Cancer Prev Res. (2014) 7:813–23. doi: 10.1158/1940-6207.Capr-14-0103
238. Armah, CN, Derdemezis, C, Traka, MH, Dainty, JR, Doleman, JF, Saha, S, et al. Diet rich in high glucoraphanin broccoli reduces plasma Ldl cholesterol: evidence from randomised controlled trials. Mol Nutr Food Res. (2015) 59:918–26. doi: 10.1002/mnfr.201400863
239. López-Chillón, MT, Carazo-Díaz, C, Prieto-Merino, D, Zafrilla, P, Moreno, DA, and Villaño, D. Effects of Long-term consumption of broccoli sprouts on inflammatory markers in overweight subjects. Clin Nutr. (2019) 38:745–52. doi: 10.1016/j.clnu.2018.03.006
240. Kikuchi, M, Ushida, Y, Shiozawa, H, Umeda, R, Tsuruya, K, Aoki, Y, et al. Sulforaphane-rich broccoli sprout extract improves hepatic abnormalities in male subjects. World J Gastroenterol. (2015) 21:12457–67. doi: 10.3748/wjg.v21.i43.12457
241. Davidson, R, Gardner, S, Jupp, O, Bullough, A, Butters, S, Watts, L, et al. Isothiocyanates are detected in human synovial fluid following broccoli consumption and can affect the tissues of the knee joint. Sci Rep. (2017) 7:3398. doi: 10.1038/s41598-017-03629-5
242. Kim, H-A, Yeo, Y, Jung, HA, Jung, YO, Park, SJ, and Kim, SJ. Phase 2 enzyme inducer Sulphoraphane blocks prostaglandin and nitric oxide synthesis in human articular chondrocytes and inhibits cartilage matrix degradation. Rheumatology. (2012) 51:1006–16. doi: 10.1093/rheumatology/ker525
243. Yanaka, A, Fahey, JW, Fukumoto, A, Nakayama, M, Inoue, S, Zhang, S, et al. Dietary Sulforaphane-rich broccoli sprouts reduce colonization and attenuate gastritis in Helicobacter pylori-infected mice and humans. Cancer Prev Res (Phila). (2009) 2:353–60. doi: 10.1158/1940-6207.Capr-08-0192
244. Guo, K, Wang, L, Mahe, J, Li, L, Jiao, S, Wang, H, et al. Effect of aqueous extract of seed of broccoli on inflammatory cytokines and Helicobacter pylori infection: a randomized, double-blind, controlled trial in patients without atrophic gastritis. Inflammopharmacology. (2022) 30:1659–68. doi: 10.1007/s10787-022-01030-x
245. Lee, Y-C, Chiang, T-H, Chou, C-K, Tu, Y-K, Liao, W-C, Wu, M-S, et al. Association between Helicobacter pylori eradication and gastric Cancer incidence: a systematic review and Meta-analysis. Gastroenterology. (2016) 150:1113–1124.e5. e5. doi: 10.1053/j.gastro.2016.01.028
246. Naguib, YM . Antioxidant activities of Astaxanthin and related carotenoids. J Agric Food Chem. (2000) 48:1150–4. doi: 10.1021/jf991106k
247. Kumar, S, Kumar, R, Diksha, KA, and Panwar, A. Astaxanthin: a super antioxidant from microalgae and its therapeutic potential. J Basic Microbiol. (2022) 62:1064–82. doi: 10.1002/jobm.202100391
248. Bjørklund, G, Gasmi, A, Lenchyk, L, Shanaida, M, Zafar, S, Mujawdiya, PK, et al. The role of Astaxanthin as a nutraceutical in health and age-related conditions. Molecules. (2022) 27:27(21). doi: 10.3390/molecules27217167
249. Chang, MX, and Xiong, F. Astaxanthin and its effects in inflammatory responses and inflammation-associated diseases: recent advances and future directions. Molecules. (2020) 25:5342. doi: 10.3390/molecules25225342
250. Yuan, JP, Peng, J, Yin, K, and Wang, JH. Potential health-promoting effects of Astaxanthin: a high-value carotenoid mostly from microalgae. Mol Nutr Food Res. (2011) 55:150–65. doi: 10.1002/mnfr.201000414
251. Nishida, Y, Nawaz, A, Hecht, K, and Tobe, K. Astaxanthin as a novel mitochondrial regulator: a new aspect of carotenoids, beyond antioxidants. Nutrients. (2021) 14:107. doi: 10.3390/nu14010107
252. Wolf, AM, Asoh, S, Hiranuma, H, Ohsawa, I, Iio, K, Satou, A, et al. Astaxanthin protects mitochondrial redox state and functional integrity against oxidative stress. J Nutr Biochem. (2010) 21:381–9. doi: 10.1016/j.jnutbio.2009.01.011
253. Ma, B, Lu, J, Kang, T, Zhu, M, Xiong, K, and Wang, J. Astaxanthin supplementation mildly reduced oxidative stress and inflammation biomarkers: a systematic review and Meta-analysis of randomized controlled trials. Nutr Res. (2022) 99:40–50. doi: 10.1016/j.nutres.2021.09.005
254. Ng, QX, De Deyn, MLZQ, Loke, W, Foo, NX, Chan, HW, and Yeo, WS. Effects of Astaxanthin supplementation on skin health: a systematic review of clinical studies. J Diet Suppl. (2021) 18:169–82. doi: 10.1080/19390211.2020.1739187
255. Nouchi, R, Suiko, T, Kimura, E, Takenaka, H, Murakoshi, M, Uchiyama, A, et al. Effects of lutein and Astaxanthin intake on the improvement of cognitive functions among healthy adults: a systematic review of randomized controlled trials. Nutrients. (2020) 12:627. doi: 10.3390/nu12030617
256. Bulgakov, VP, Inyushkina, YV, and Fedoreyev, SA. Rosmarinic acid and its derivatives: biotechnology and applications. Crit Rev Biotechnol. (2012) 32:203–17. doi: 10.3109/07388551.2011.596804
257. Pérez-Fons, L, MaT, GÓ, and Micol, V. Relationship between the antioxidant capacity and effect of rosemary (Rosmarinus officinalis L.) polyphenols on membrane phospholipid order. J Agric Food Chem. (2010) 58:161–71. doi: 10.1021/jf9026487
258. Panya, A, Laguerre, M, Lecomte, J, Villeneuve, P, Weiss, J, McClements, DJ, et al. Effects of chitosan and Rosmarinate esters on the physical and oxidative stability of liposomes. J Agric Food Chem. (2010) 58:5679–84. doi: 10.1021/jf100133b
259. Furtado, RA, De Araujo, FRR, Resende, FA, Cunha, WR, and Tavares, DC. Protective effect of Rosmarinic acid on V79 cells evaluated by the micronucleus and comet assays. J Appl Toxicol. (2010) 30:254–9. doi: 10.1002/jat.1491
260. Koşar, M, Göger, F, and Can Başer, KH. In vitro antioxidant properties and phenolic composition of Salvia virgata Jacq. From Turkey. J Agric Food Chem. (2008) 56:2369–74. doi: 10.1021/jf073516b
261. Osakabe, N, Yasuda, A, Natsume, M, and Yoshikawa, T. Rosmarinic acid inhibits epidermal inflammatory responses: anticarcinogenic effect of Perilla frutescens extract in the murine two-stage skin model. Carcinogenesis. (2004) 25:549–57. doi: 10.1093/carcin/bgh034
262. Lucarini, R, Bernardes, WA, Ferreira, DS, Tozatti, MG, Furtado, R, Bastos, JK, et al. In Vivo analgesic and anti-inflammatory activities of Rosmarinus officinalis aqueous extracts, Rosmarinic acid and its acetyl Ester derivative. Pharm Biol. (2013) 51:1087–90. doi: 10.3109/13880209.2013.776613
263. Hase, T, Shishido, S, Yamamoto, S, Yamashita, R, Nukima, H, Taira, S, et al. Rosmarinic acid suppresses Alzheimer's disease development by reducing amyloid Β aggregation by increasing monoamine secretion. Sci Rep. (2019) 9:8711. doi: 10.1038/s41598-019-45168-1
264. Shimojo, Y, Kosaka, K, Noda, Y, Shimizu, T, and Shirasawa, T. Effect of Rosmarinic acid in motor dysfunction and life span in a mouse model of familial amyotrophic lateral sclerosis. J Neurosci Res. (2010) 88:896–904. doi: 10.1002/jnr.22242
265. Moon, D-O, Kim, M-O, Lee, J-D, Choi, YH, and Kim, G-Y. Rosmarinic acid sensitizes cell death through suppression of Tnf-Α-induced Nf-Κb activation and Ros generation in human leukemia U937 cells. Cancer Lett. (2010) 288:183–91. doi: 10.1016/j.canlet.2009.06.033
266. Kuo, C-F, Su, J-D, Chiu, C-H, Peng, C-C, Chang, C-H, Sung, T-Y, et al. Anti-inflammatory effects of supercritical carbon dioxide extract and its isolated Carnosic acid from Rosmarinus officinalis leaves. J Agric Food Chem. (2011) 59:3674–85. doi: 10.1021/jf104837w
267. Foresti, R, Bains, SK, Pitchumony, TS, de Castro Brás, LE, Drago, F, Dubois-Randé, J-L, et al. Small molecule activators of the Nrf2-Ho-1 antioxidant Axis modulate Heme metabolism and inflammation in Bv2 microglia cells. Pharmacol Res. (2013) 76:132–48. doi: 10.1016/j.phrs.2013.07.010
268. Guo, Q, Shen, Z, Yu, H, Lu, G, Yu, Y, Liu, X, et al. Carnosic acid protects against acetaminophen-induced hepatotoxicity by potentiating Nrf2-mediated antioxidant capacity in mice. Korean J Physiol Pharmacol. (2016) 20:15–23. doi: 10.4196/kjpp.2016.20.1.15
269. Yanagitai, M, Itoh, S, Kitagawa, T, Takenouchi, T, Kitani, H, and Satoh, T. Carnosic acid, a pro-electrophilic compound, inhibits LPS-induced activation of microglia. Biochem Biophys Res Commun. (2012) 418:22–6. doi: 10.1016/j.bbrc.2011.12.087
270. Machado, DG, Bettio, LE, Cunha, MP, Capra, JC, Dalmarco, JB, Pizzolatti, MG, et al. Antidepressant-like effect of the extract of Rosmarinus officinalis in mice: involvement of the monoaminergic system. Prog Neuro-Psychopharmacol Biol Psychiatry. (2009) 33:642–50. doi: 10.1016/j.pnpbp.2009.03.004
271. Nematolahi, P, Mehrabani, M, Karami-Mohajeri, S, and Dabaghzadeh, F. Effects of Rosmarinus officinalis L. on memory performance, anxiety, depression, and sleep quality in university students: a randomized clinical trial. Complement Ther Clin Pract. (2018) 30:24–8. doi: 10.1016/j.ctcp.2017.11.004
272. Azizi, S, Mohamadi, N, Sharififar, F, Dehghannoudeh, G, Jahanbakhsh, F, and Dabaghzadeh, F. Rosemary as an adjunctive treatment in patients with major depressive disorder: a randomized, double-blind, placebo-controlled trial. Complement Ther Clin Pract. (2022) 49:101685. doi: 10.1016/j.ctcp.2022.101685
273. Pengelly, A, Snow, J, Mills, SY, Scholey, A, Wesnes, K, and Butler, LR. Short-term study on the effects of rosemary on cognitive function in an elderly population. J Med Food. (2012) 15:10–7. doi: 10.1089/jmf.2011.0005
274. Noguchi-Shinohara, M, Ono, K, Hamaguchi, T, Nagai, T, Kobayashi, S, Komatsu, J, et al. Safety and efficacy of Melissa officinalis extract containing Rosmarinic acid in the prevention of Alzheimer’s disease progression. Sci Rep. (2020) 10:18627. doi: 10.1038/s41598-020-73729-2
275. Noguchi-Shinohara, M, Hamaguchi, T, Sakai, K, Komatsu, J, Iwasa, K, Horimoto, M, et al. Effects of Melissa officinalis extract containing Rosmarinic acid on cognition in older adults without dementia: a randomized controlled trial. J Alzheimers Dis. (2023) (Preprint):1-10) 91:805–14. doi: 10.3233/JAD-220953
276. Ostfeld, I, Ben-Moshe, Y, Hoffman, MW, Shalev, H, and Hoffman, JR. Effect of spearmint extract containing Rosmarinic acid on physical and executive functioning after a tactical operation. J Spec Operat Med. (2018) 18:92–6. doi: 10.55460/HVYN-6PAG
277. Herrlinger, KA, Nieman, KM, Sanoshy, KD, Fonseca, BA, Lasrado, JA, Schild, AL, et al. Spearmint extract improves working memory in men and women with age-associated memory impairment. J Altern Complement Med. (2018) 24:37–47. doi: 10.1089/acm.2016.0379
278. Falcone, PH, Nieman, KM, Tribby, AC, Vogel, RM, Joy, JM, Moon, JR, et al. The attention-enhancing effects of spearmint extract supplementation in healthy men and women: a randomized, double-blind, placebo-controlled, parallel trial. Nutr Res. (2019) 64:24–38. doi: 10.1016/j.nutres.2018.11.012
279. Falcone, PH, Tribby, AC, Vogel, RM, Joy, JM, Moon, JR, Slayton, CA, et al. Efficacy of a nootropic spearmint extract on reactive agility: a randomized, double-blind, placebo-controlled, parallel trial. J Int Soc Sports Nutr. (2018) 15:58. doi: 10.1186/s12970-018-0264-5
280. Perry, NSL, Menzies, R, Hodgson, F, Wedgewood, P, Howes, MJR, Brooker, HJ, et al. A randomised double-blind placebo-controlled pilot trial of a combined extract of sage, rosemary and Melissa, traditional herbal medicines, on the enhancement of memory in Normal healthy subjects, including influence of age. Phytomedicine. (2018) 39:42–8. doi: 10.1016/j.phymed.2017.08.015
281. Moghaddasi, MS, and Kashani, HH. Ginger (Zingiber officinale): a review. J Med Plants Re. (2012) 6:4255–8. doi: 10.5897/JMPR011.787
282. Liu, Y, Liu, J, and Zhang, Y. Research Progress on chemical constituents of Zingiber officinale roscoe. Biomed Res Int. (2019) 2019:1–21. doi: 10.1155/2019/5370823
283. Imo, C, and Za’aku, JS. Medicinal properties of ginger and garlic: a review. Curr Trends Biomed Eng Biosci. (2019) 18:47–52. doi: 10.19080/CTBEB.2019.18.555985
284. Semwal, RB, Semwal, DK, Combrinck, S, and Viljoen, AM. Gingerols and Shogaols: important nutraceutical principles from ginger. Phytochemistry. (2015) 117:554–68. doi: 10.1016/j.phytochem.2015.07.012
285. Butt, MS, and Sultan, MT. Ginger and its health claims: molecular aspects. Crit Rev Food Sci Nutr. (2011) 51:383–93. doi: 10.1080/10408391003624848
286. Jalali, M, Mahmoodi, M, Moosavian, SP, Jalali, R, Ferns, G, Mosallanezhad, A, et al. The effects of ginger supplementation on markers of inflammatory and oxidative stress: a systematic review and Meta-analysis of clinical trials. Phytother Res. (2020) 34:1723–33. doi: 10.1002/ptr.6638
287. Mao, Q-Q, Xu, X-Y, Cao, S-Y, Gan, R-Y, Corke, H, Beta, T, et al. Bioactive compounds and bioactivities of ginger (Zingiber officinale roscoe). Food Secur. (2019) 8:185. doi: 10.3390/foods8060185
288. Srinivasan, K . Ginger rhizomes (Zingiber officinale): a spice with multiple health beneficial potentials. PharmaNutrition. (2017) 5:18–28. doi: 10.1016/j.phanu.2017.01.001
289. Ezzat, SM, Ezzat, MI, Okba, MM, Menze, ET, and Abdel-Naim, AB. The hidden mechanism beyond ginger (Zingiber officinale Rosc.) potent in Vivo and in vitro anti-inflammatory activity. J Ethnopharmacol. (2018) 214:113–23. doi: 10.1016/j.jep.2017.12.019
290. Ho, S-C, Chang, K-S, and Lin, C-C. Anti-neuroinflammatory capacity of fresh ginger is attributed mainly to 10-Gingerol. Food Chem. (2013) 141:3183–91. doi: 10.1016/j.foodchem.2013.06.010
291. Park, K-K, Chun, K-S, Lee, J-M, Lee, SS, and Surh, Y-J. Inhibitory effects of [6]-Gingerol, a major pungent principle of ginger, on Phorbol Ester-induced inflammation, epidermal ornithine decarboxylase activity and skin tumor promotion in Icr mice. Cancer Lett. (1998) 129:139–44. doi: 10.1016/s0304-3835(98)00081-0
292. Adetuyi, BO, and Farombi, EO. 6-Gingerol, an active constituent of ginger, attenuates lipopolysaccharide-induced oxidation, inflammation, cognitive deficits, neuroplasticity, and Amyloidogenesis in rat. J Food Biochem. (2021) 45:e13660. doi: 10.1111/jfbc.13660
293. Lim, S, Moon, M, Oh, H, Kim, HG, Kim, SY, and Oh, MS. Ginger improves cognitive function via Ngf-induced Erk/Creb activation in the Hippocampus of the mouse. J Nutr Biochem. (2014) 25:1058–65. doi: 10.1016/j.jnutbio.2014.05.009
294. Zeng, G-f . Protective effects of ginger root extract on Alzheimer disease-induced behavioral dysfunction in rats. Rejuvenation Res. (2013) 16:124–33. doi: 10.1089/rej.2012.1389
295. Huh, E, Choi, JG, Choi, Y, Ju, IG, Noh, D, Shin, DY, et al. 6-Shogaol, an active ingredient of ginger, improves intestinal and brain abnormalities in Proteus mirabilis-induced Parkinson's disease mouse model. Biomol Ther. (2023) 31:417–24. doi: 10.4062/biomolther.2023.098
296. Ozkur, M, Benlier, N, Takan, I, Vasileiou, C, Georgakilas, AG, Pavlopoulou, A, et al. Ginger for healthy ageing: a systematic review on current evidence of its antioxidant, anti-inflammatory, and anticancer properties. Oxidative Med Cell Longev. (2022) 2022:4748447–16. doi: 10.1155/2022/4748447
297. Sheikhhossein, F, Borazjani, M, Jafari, A, Askari, M, Vataniyan, E, Gholami, F, et al. Effects of ginger supplementation on biomarkers of oxidative stress: a systematic review and Meta-analysis of randomized controlled trials. Clin Nutr ESPEN. (2021) 45:111–9. doi: 10.1016/j.clnesp.2021.07.010
298. Anh, NH, Kim, SJ, Long, NP, Min, JE, Yoon, YC, Lee, EG, et al. Ginger on human health: a comprehensive systematic review of 109 randomized controlled trials. Nutrients. (2020) 12:157. doi: 10.3390/nu12010157
299. Marx, W, Ried, K, McCarthy, AL, Vitetta, L, Sali, A, McKavanagh, D, et al. Ginger—mechanism of action in chemotherapy-induced nausea and vomiting: a review. Crit Rev Food Sci Nutr. (2017) 57:141–6. doi: 10.1080/10408398.2013.865590
300. Wu, KL, Rayner, CK, Chuah, SK, Changchien, CS, Lu, SN, Chiu, YC, et al. Effects of ginger on gastric emptying and motility in healthy humans. Eur J Gastroenterol Hepatol. (2008) 20:436–40. doi: 10.1097/MEG.0b013e3282f4b224
301. Gonlachanvit, S, Chen, YH, Hasler, WL, Sun, WM, and Owyang, C. Ginger reduces hyperglycemia-evoked gastric dysrhythmias in healthy humans: possible role of endogenous prostaglandins. J Pharmacol Exp Ther. (2003) 307:1098–103. Epub 20031008. doi: 10.1124/jpet.103.053421
302. Micklefield, GH, Redeker, Y, Meister, V, Jung, O, Greving, I, and May, B. Effects of ginger on gastroduodenal motility. Int J Clin Pharmacol Ther. (1999) 37:341–6.
303. Hu, ML, Rayner, CK, Wu, KL, Chuah, SK, Tai, WC, Chou, YP, et al. Effect of ginger on gastric motility and symptoms of functional dyspepsia. World J Gastroenterol. (2011) 17:105–10. doi: 10.3748/wjg.v17.i1.105
304. Hirata, A, Funato, H, Nakai, M, Iizuka, M, Abe, N, Yagi, Y, et al. Ginger orally disintegrating tablets to improve swallowing in older people. Biol Pharm Bull. (2016) 39:1107–11. doi: 10.1248/bpb.b15-01045
305. Maharlouei, N, Tabrizi, R, Lankarani, KB, Rezaianzadeh, A, Akbari, M, Kolahdooz, F, et al. The effects of ginger intake on weight loss and metabolic profiles among overweight and obese subjects: a systematic review and meta-analysis of randomized controlled trials. Crit Rev Food Sci Nutr. (2019) 59:1753–66. doi: 10.1080/10408398.2018.1427044
306. Macit, MS, Sözlü, S, Kocaadam, B, and Acar-Tek, N. Evaluation of ginger (Zingiber officinale roscoe) on energy metabolism and obesity: systematic review and Meta-analysis. Food Rev Intl. (2019) 35:685–706. doi: 10.1080/87559129.2019.1608556
307. Pourmasoumi, M, Hadi, A, Rafie, N, Najafgholizadeh, A, Mohammadi, H, and Rouhani, MH. The effect of ginger supplementation on lipid profile: a systematic review and Meta-analysis of clinical trials. Phytomedicine. (2018) 43:28–36. doi: 10.1016/j.phymed.2018.03.043
308. Mazidi, M, Gao, HK, Rezaie, P, and Ferns, GA. The effect of ginger supplementation on serum C-reactive protein, lipid profile and glycaemia: a systematic review and Meta-analysis. Food Nutr Res. (2016) 60:32613. doi: 10.3402/fnr.v60.32613
309. Jafarnejad, S, Keshavarz, SA, Mahbubi, S, Saremi, S, Arab, A, Abbasi, S, et al. Effect of ginger (Zingiber officinale) on blood glucose and lipid concentrations in diabetic and Hyperlipidemic subjects: a Meta-analysis of randomized controlled trials. J Funct Foods. (2017) 29:127–34. doi: 10.1016/j.jff.2016.12.006
310. Hasani, H, Arab, A, Hadi, A, Pourmasoumi, M, Ghavami, A, and Miraghajani, M. Does ginger supplementation lower blood pressure? A systematic review and Meta-analysis of clinical trials. Phytother Res. (2019) 33:1639–47. doi: 10.1002/ptr.6362
311. Saenghong, N, Wattanathorn, J, Muchimapura, S, Tongun, T, Piyavhatkul, N, Banchonglikitkul, C, et al. Zingiber officinale improves cognitive function of the middle-aged healthy women. Evid Based Complement Alternat Med. (2012) 2012:383062. doi: 10.1155/2012/383062
312. Altman, RD, and Marcussen, KC. Effects of a ginger extract on knee pain in patients with osteoarthritis. Arthritis Rheum. (2001) 44:2531–8. doi: 10.1002/1529-0131(200111)44:11<2531::aid-art433>3.0.co;2-j
313. Wigler, I, Grotto, I, Caspi, D, and Yaron, M. The effects of Zintona Ec (a ginger extract) on symptomatic Gonarthritis. Osteoarthr Cartil. (2003) 11:783–9. doi: 10.1016/s1063-4584(03)00169-9
314. Mozaffari-Khosravi, H, Naderi, Z, Dehghan, A, Nadjarzadeh, A, and Fallah, HH. Effect of ginger supplementation on Proinflammatory cytokines in older patients with osteoarthritis: outcomes of a randomized controlled clinical trial. J Nutr Gerontol Geriatr. (2016) 35:209–18. doi: 10.1080/21551197.2016.1206762
315. Paramdeep, G . Efficacy and tolerability of ginger (Zingiber officinale) in patients of osteoarthritis of knee. Indian J Physiol Pharmacol. (2013) 57:177–83.
316. Niempoog, S, Pawa, KK, and Amatyakul, C. The efficacy of powdered ginger in osteoarthritis of the knee. J Med Assoc Thail. (2012) 95:S59–64.
317. Mueller, AL, Payandeh, Z, Mohammadkhani, N, Mubarak, SMH, Zakeri, A, Alagheband Bahrami, A, et al. Recent advances in understanding the pathogenesis of rheumatoid arthritis: new treatment strategies. Cells. (2021) 10:3017. doi: 10.3390/cells10113017
318. Aryaeian, N, Shahram, F, Mahmoudi, M, Tavakoli, H, Yousefi, B, Arablou, T, et al. The effect of ginger supplementation on some immunity and inflammation intermediate genes expression in patients with active rheumatoid arthritis. Gene. (2019) 698:179–85. doi: 10.1016/j.gene.2019.01.048
319. Fontana, L, and Partridge, L. Promoting health and longevity through diet: from model organisms to humans. Cell. (2015) 161:106–18. doi: 10.1016/j.cell.2015.02.020
320. Luo, J, Si, H, Jia, Z, and Liu, D. Dietary anti-aging polyphenols and potential mechanisms. Antioxidants. (2021) 10:283. doi: 10.3390/antiox10020283
321. Lu, W-H, González-Bautista, E, Guyonnet, S, Martinez, LO, Lucas, A, Parini, A, et al. Investigating three ways of measuring the intrinsic capacity domain of vitality: nutritional status, handgrip strength and ageing biomarkers. Age Ageing. (2023) 52:afad133. doi: 10.1093/ageing/afad133
322. Lu, W-H, Guyonnet, S, Martinez, LO, Lucas, A, Parini, A, Vellas, B, et al. Association between aging-related biomarkers and longitudinal trajectories of intrinsic capacity in older adults. GeroScience. (2023) 45:3409–18. doi: 10.1007/s11357-023-00906-2
323. Ladang, A, Beaudart, C, Reginster, J-Y, Al-Daghri, N, Bruyère, O, Burlet, N, et al. Biochemical markers of musculoskeletal health and aging to be assessed in clinical trials of drugs aiming at the treatment of sarcopenia: consensus paper from an expert group meeting organized by the European Society for Clinical and Economic Aspects of osteoporosis, osteoarthritis and musculoskeletal diseases (Esceo) and the Centre Académique De Recherche et D'expérimentation En Santé (cares Sprl), under the auspices of the World Health Organization collaborating Center for the Epidemiology of musculoskeletal conditions and aging. Calcif Tissue Int. (2023) 112:197–217. doi: 10.1007/s00223-022-01054-z
324. Schulz, RJ . Homocysteine as a biomarker for cognitive dysfunction in the elderly. Curr Opin Clin Nutr Metab Care. (2007) 10:718–23. doi: 10.1097/MCO.0b013e3282f0cfe3
325. Krentz, AJ, Viljoen, A, and Sinclair, A. Insulin resistance: a risk marker for disease and disability in the older person. Diabet Med. (2013) 30:535–48. doi: 10.1111/dme.12063
326. Lalia, AZ, Dasari, S, Johnson, ML, Robinson, MM, Konopka, AR, Distelmaier, K, et al. Predictors of whole-body insulin sensitivity across ages and adiposity in adult humans. J Clin Endocrinol Metab. (2016) 101:626–34. doi: 10.1210/jc.2015-2892
327. Park, SK, Kim, K, Page, GP, Allison, DB, Weindruch, R, and Prolla, TA. Gene expression profiling of aging in multiple mouse strains: identification of aging biomarkers and impact of dietary antioxidants. Aging Cell. (2009) 8:484–95. doi: 10.1111/j.1474-9726.2009.00496.x
328. Medina-Remón, A, Casas, R, Tressserra-Rimbau, A, Ros, E, Martínez-González, MA, Fitó, M, et al. Polyphenol intake from a Mediterranean diet decreases inflammatory biomarkers related to atherosclerosis: a substudy of the Predimed trial. Br J Clin Pharmacol. (2017) 83:114–28. doi: 10.1111/bcp.12986
329. LeBoff, MS, and Bischoff-Ferrari, HA. The effects of vitamin D supplementation on musculoskeletal health: the vital and do-health trials. J Gerontol A Biol Sci Med Sci. (2023) 78:73–8. doi: 10.1093/gerona/glad073
330. Leitão, C, Mignano, A, Estrela, M, Fardilha, M, Figueiras, A, Roque, F, et al. The effect of nutrition on aging-a systematic review focusing on aging-related biomarkers. Nutrients. (2022) 14:554. doi: 10.3390/nu14030554
331. Chiba, T, Kimura, Y, Suzuki, S, Tatefuji, T, and Umegaki, K. Trans-resveratrol enhances the anticoagulant activity of warfarin in a mouse model. J Atheroscler Thromb. (2016) 23:1099–110. doi: 10.5551/jat.31765
Keywords: aging, nutrition, healthspan, phytonutrients, vitality, intrinsic capacity
Citation: Jacquier EF, Kassis A, Marcu D, Contractor N, Hong J, Hu C, Kuehn M, Lenderink C and Rajgopal A (2024) Phytonutrients in the promotion of healthspan: a new perspective. Front. Nutr. 11:1409339. doi: 10.3389/fnut.2024.1409339
Edited by:
Eric Gumpricht, Isagenix International, LLC, United StatesReviewed by:
Sofia Viana, University of Coimbra, PortugalChristine Morand, Institut National de recherche pour l’agriculture, l’alimentation et l’environnement (INRAE), France
Fahrul Nurkolis, State Islamic University of Sunan Kalijaga (UIN Sunan Kalijaga Yogyakarta), Indonesia
Copyright © 2024 Jacquier, Kassis, Marcu, Contractor, Hong, Hu, Kuehn, Lenderink and Rajgopal. This is an open-access article distributed under the terms of the Creative Commons Attribution License (CC BY). The use, distribution or reproduction in other forums is permitted, provided the original author(s) and the copyright owner(s) are credited and that the original publication in this journal is cited, in accordance with accepted academic practice. No use, distribution or reproduction is permitted which does not comply with these terms.
*Correspondence: Emma F. Jacquier, emma@theneatscience.com