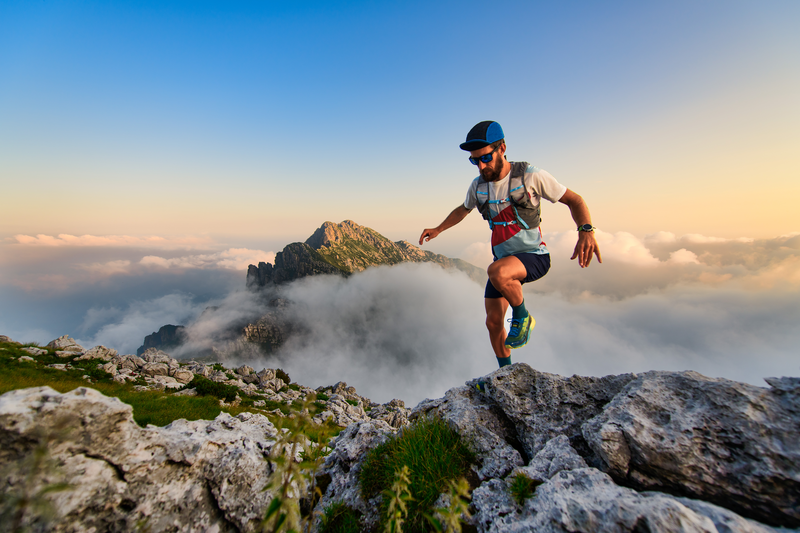
94% of researchers rate our articles as excellent or good
Learn more about the work of our research integrity team to safeguard the quality of each article we publish.
Find out more
CLINICAL TRIAL article
Front. Nutr. , 01 July 2024
Sec. Clinical Nutrition
Volume 11 - 2024 | https://doi.org/10.3389/fnut.2024.1407736
Background: The eradication regimen for Helicobacter pylori (H. pylori) infection can induce gut dysbiosis. In this open-label, prospective, and randomized clinical trial, we aimed to assess the effects of fucoidan supplementation on the eradication rate and gut microbial homeostasis in the context of quadruple therapy, as well as to investigate the combined effects of fucoidan and synbiotics supplementations.
Methods: Eighty patients with H. pylori infection were enrolled and randomly assigned to one of four treatment groups: the QT (a 2-week quadruple therapy alone), QF (quadruple therapy plus a 6-week fucoidan supplementation), QS (quadruple therapy plus a 6-week synbiotics supplementation), and QFS (quadruple therapy with a 6-week fucoidan and synbiotics supplementation), with 20 patients in each group. The QT regimen included rabeprazole, minocycline, amoxicillin, and bismuth potassium citrate. The synbiotics supplementation contained three strains of Bifidobacterium, three strains of Lactobacillus, along with three types of dietary fiber. All of the patients underwent 13C-urea breath test (13C-UBT) at baseline and at the end of the 6th week after the initiation of the interventions. Fresh fecal samples were collected at baseline and at the end of the 6th week for gut microbiota analysis via 16S rRNA gene sequencing.
Results: The eradication rates among the four groups showed no significant difference. In the QT group, a significant reduction in α-diversity of gut microbiota diversity and a substantial shift in microbial composition were observed, particularly an increase in Escherichia-Shigella and a decrease in the abundance of genera from the Lachnospiraceae and Ruminococcaceae families. The Simpson index was significantly higher in the QF group than in the QT group. Neither the QS nor QFS groups exhibited significant changes in α-diversity or β-diversity. The QFS group was the only one that did not show a significant increase in the relative abundance of Escherichia-Shigella, and the relative abundance of Klebsiella significantly decreased in this group.
Conclusion: The current study provided supporting evidence for the positive role of fucoidan and synbiotics supplementation in the gut microbiota. The combined use of fucoidan and synbioticss might be a promising adjuvant regimen to mitigate gut dysbiosis during H. pylori eradication therapy.
Helicobacter pylori (H. pylori) infection is a global health problem, resulting in chronic inflammation and the increased risk of peptic ulcer disease, atrophic gastritis, gastric carcinoma, and mucosa-associated lymphoid tissue (MALT) lymphoma (1). The global pooled prevalence of H. pylori infection has been reported to be 48.5%, ranging from 70.1% in Africa to 24.4% in Oceania (2). A recent meta-analysis reported that the prevalence of H. pylori infection in mainland China was 40.0% during the period 2015–2019, highlighting China as one of the countries with a substantial burden of H. pylori infection, despite a declining trend from 2000 to 2019 (3). Besides, considering the high resistance rates of clarithromycin and metronidazole (4), bismuth quadruple therapy is recommended as the first-line treatment in China (5), including 2 antibiotics (with five different regimens), a standard dose of a proton pump inhibitor (PPI), and bismuth (6). Both antibiotics and PPIs are known to disrupt the gut bacterial equilibrium, and the bacterial shifts caused by eradication therapy may contribute to the disturbance of carbohydrate and lipid metabolism (7). Consequently, it is necessary to develop a therapeutic regimen for H. pylori infection that not only achieves a satisfactory eradication rate but also minimizes the impact on the gut microbiota.
Fucoidan is a complex sulfated polysaccharide extracted from various species of brown seaweed (8). There is a growing body of evidence suggesting that fucoidan possesses various physiological properties, including high antioxidant activity (9), anti-tumor effect (10), and anti-allergic property (11). Besides, fucoidan can exert anti-inflammatory effects by modulating neutrophil migration and the expression of genes related to COX-2 and the NF-κB signaling pathway (12). Therefore, fucoidan is regarded as a promising component in functional food or adjuvant therapy. Fucoidan has two primary structural backbones: Type I, such as fucoidan from Laminaria japonica, is composed of repeated α (1 → 3)-linked α-L-fucopyranose, with fucose-linked sulfate groups in C2- and C4- positions; Type II, such as fucoidan from Ascophyllum nodosum, is composed of alternate α (1 → 3)- and α (1 → 4)-linked α-L-fucopyranose, with fucose-linked sulfate groups in C2-, C3-, and C4-positions (13). The sulfate groups are important determinants for the bioactivities of fucoidan, depending on the sulfate content and position of the groups (14).
Research in a rat model of breast cancer has indicated that fucoidan intervention can enhance the diversity of the intestinal microbiota and change the microbiota composition, including a decrease in the abundance of Collinsella, Coprococcus, Oscillospira, and Sporosarcina as well as an increase in the abundance of Parabacteroides and Blautia (15). In an ulcerative colitis mice model, fucoidan was also found to significantly increase the abundance of Ruminococcaceae and Clostridium-IV (16). Moreover, fucoidan has been demonstrated to increase several beneficial bacterial taxa and decrease several harmful taxa in mice intervened with ciprofloxacin-metronidazole (17), indicating a potential capacity to mitigate dysbiosis caused by antibiotics, which is common during the eradication therapy of H. pylori infection. Nevertheless, data on the effects of fucoidan on the human gut microbiota are limited. In patients with chronic superficial gastritis, a combination of wheat peptides and fucoidan was reported to relieve symptoms, reduce gastric mucosal damage, and regulate gut microbiome, promoting the growth of Bifidobacterium pseudocatenulatum, Eubacterium siraeum, and Bacteroides intestinali (18), but the isolated effects of fucoidan were not evaluated.
Both in vitro and in vivo studies have shown that fucoidan can prohibit H. pylori adhesion to host cells, reduce cytotoxic effects in H. pylori, and decrease the infection rate of H. pylori in a dose-dependent manner (19). However, clinical data regarding the effects of fucoidan on H. pylori infection remains limited. Thus, we conducted a prospective, randomized, open-label trial in patients with H. pylori infection. The aim was to assess the effects of fucoidan supplementation, either alone or in combination with synbiotics supplementation, on the eradication rate and the maintenance of gut microbiota homeostasis in these patients.
This clinical trial was conducted at China-Japan Friendship Hospital from January 2021 to August 2021. H. pylori-infected patients aged above 18 years were enrolled. The infection of H. pylori was diagnosed for the first time in these patients based on a positive 13C-urea breath test (13C-UBT) and/or detection of H. pylori on gastric specimens collected during gastroscopy. All H. pylori-infected patients enrolled in this study were treatment-naïve. Patients would be excluded from this study if they fulfilled any of the following criteria: prior eradication therapy for H. pylori infection; peptic ulcer or other upper gastrointestinal lesions; upper gastrointestinal malignant tumor; acute or chronic lesions of major organs (e.g., cardiac, pulmonary, hepatic, or renal diseases); endocrine and metabolic disorders (e.g., diabetes mellitus, thyroid diseases); autoimmune diseases; use of antibiotics, antacids, bismuth, probiotics, prebiotics, or synbioticss within the previous 4 weeks; and pregnancy or lactation. This study was approved by the Ethics Committee of China-Japan Friendship Hospital (2020-64-K35) and was registered on the Chinese Clinical Trial Registry, number CTR2400080402. All participants provided their informed consent before participating in the study.
All patients underwent 13C-UBT at baseline, and then a computer-generated list of random numbers using SAS 9.0 (SPSS Institute, Cary, NC, United States) was used to randomly assign the patients to four treatment groups, and the corresponding intervention began immediately: (1) the QT group, receiving bismuth quadruple therapy, including rabeprazole 10 mg, minocycline 100 mg, amoxicillin 1,000 mg, and bismuth potassium citrate 220 mg, all administered twice daily for 2 weeks, namely the RMAB regimen; (2) the QF group, receiving RMAB therapy along with fucoidan 1 g once daily for 6 weeks, with fucoidan supplementation extending 4 weeks beyond the completion of quadruple therapy; (3) the QS group, receiving RMAB therapy along with a packet of synbiotics once daily for 6 weeks; and (4) the QFS group, receiving RMAB therapy along with 1 g fucoidan and a packet of synbiotics daily for 6 weeks. To assess the eradication of H. pylori, all of the patients underwent 13C-UBT 4 weeks after the completion of quadruple therapy. To evaluate possible side effects of interventions, new-onset symptoms during the 6 weeks were recorded.
The fucoidan used in this study was extracted from Laminaria japonica (Qingdao Brightmoon Seaweed Group Co., Ltd., Qingdao, China), with an average molecular weight of 276,038 Da and a purity of 90%. The monosaccharide fucose and the sulfuric acid group contents were 22.66% (w/w) and 29.65% (w/w), respectively. The prebiotics in the synbiotics used in this study were Bifidobacterium (accounted for 30%, including B. animalis BB12, B. lactis Bi07, and B. bifidum Bb06) and Lactobacillus (accounted for 70%, including L. helveticus R52, L. rhamnosus R11, and L. acidophilus NCFM), with a total colony forming unit (CFU) reaching 18 × 109 in each packet; The prebiotics in the synbiotics packet included resistant dextrin 0.5 g, fructooligosaccharides 0.3 g, and inulin 1.0 g (Yu Jun Gong Wu®, lyophilized powder, Baoding Xiongnan Biotechnology Co., Ltd., Baoding, China).
Fresh stool samples were collected with sterile plastic tubes from enrolled patients at baseline and at the end of 6th week in China-Japan Friendship Hospital. All of the samples were immediately transferred to the laboratory and stored at −80°C until analysis. Microbial DNA was extracted from the fecal samples using a QIAamp PowerFecal DNA Kit (QIAGEN, Germany). The amplification of the hypervariable V3-V4 region was conducted using primers 341F (5′-CCTACGGGNBGCASCAG-3′) and 805R (5′-GACTACNVGGGTATCTAATCC-3′). PCR reactions were performed in 25 μL mixture containing 5 μL of 5 × GC Buffer, 0.5 μL of KAPA dNTP Mix, 0.5 μL of KAPA HiFi HotStart DNA Polymerase, 0.5 μL of each primer (10 pM) and 50 ~ 100 ng of template DNA. After purifying the 16S V3 and V4 amplicon away from free primers and primer dimer species, PCR reactions were performed in 25 μL mixture containing 5 μL of 5 × GC Buffer, 0.75 μL of KAPA dNTP Mix, 0.5 μL of KAPA HiFi HotStart DNA Polymerase, 1.5 μL of each primer (10 pM) and 5 μL of purified product. The amplicons were subsequently purified by AMPure XP beads to construct the final library. Then sequencing was performed using the Illumina Hiseq platform (Illumina, CA, United States).
Fast Length Adjustment of SHort reads (FLASH) was used to merge paired-end reads from next-generation sequencing. The sequences were grouped into operational taxonomic units (OTUs) with a 97% threshold of similarity. Alpha diversity was analyzed with QIIME (V1.9.1), including the Shannon index and Simpson index to depict microbial diversity as well as the Chao1 index and observed species to depict microbial richness. Beta diversity was assessed based on weighted Unifrac distance matric, and principal coordinate analysis (PCoA) was conducted to visualize the distance between data. Analysis of similarities (Anosim) was used to evaluate the differences in microbial composition before and after interventions or between groups. The comparison of relative abundance bacterial taxa between groups was performed by Wilcoxon–Mann–Whitney test. The distinguishing features of the fecal microbiota were analyzed using linear discriminant analysis (LDA) effect size (LEfSe), and differences with log 10 LDA scores (absolute values) > 2.0 and p value < 0.05 were considered statistically significant. Meanwhile, metastats was used to identify the taxa with significantly different abundances between groups.
Statistical analyses were performed using SPSS version 22.0 (SPSS Inc., Chicago, IL, United States). Comparisons of continuous parameters were performed using independent samples t-test between two groups or one-way ANOVA among multiple groups, and comparisons of non-normal distribution data were performed using Wilcoxon–Mann–Whitney test or Kruskal-Wallis test. Categorical data were described as percentages and were compared using the chi-squared test or Fisher’s exact test. A two-sided p < 0.05 was considered statistically significant.
A total of 80 patients were enrolled in this study and randomly assigned to receive either QT, QS, QF, or QFS therapy, with 20 patients in each group. The sex distribution and age of the patients in the four groups showed no significant difference. All of the participants fully completed the therapies and underwent 13C-UBT at week 0 and week 6. The QT, QS, QF, and QFS eradication rates were 95.0, 90.0, 100.0, and 95.0%, respectively, showing no significant difference. Detailed demographic characteristics and eradication rates are presented in Table 1. Several new-onset gastrointestinal symptoms were reported by patients, without significant differences among groups, indicating that the use of fucoidan or synbiotics neither improved nor exacerbated new-onset gastrointestinal symptoms during eradication therapy of H. pylori infection (Table 2).
All of the patients in the four groups provided fecal samples before the interventions. Four patients in the QT group, 3 patients in the QF group, 3 patients in the QS group, and 1 patient in the QFS group did not provide fecal samples at week 6. There were no significant differences in α-diversity among the four groups at week 0 (Figure 1A). In the QT group, observed species (p < 0.001), Chao 1 index (p = 0.001), and Shannon index (p = 0.006) were all significantly lower at week 6 compared with those at week 0, indicating that the decrease of α-diversity did not restore at 4 weeks after the quadruple therapy. Similarly, significant decreases in observed species (p < 0.001), Chao 1 index (p < 0.001), and Shannon index (p = 0.009) were observed in the QF group. However, these three indices and the Simpson index showed no significant differences between week 0 and week 6 in both the QS and QFS groups. Compared to the QT group, the Simpson index at week 6 was significantly higher (p = 0.038) and the Shannon index tended to be higher (p = 0.055) in the QF group (Figure 1B).
Figure 1. Alpha diversity was depicted with observed species, Chao1, Shannon, and Simpson indices. QT group, bismuth quadruple therapy for 2 weeks; QF group, bismuth quadruple therapy for 2 weeks combined with fucoidan for 6 weeks; QS group, bismuth quadruple therapy combined with synbiotics for 6 weeks; QFS group, bismuth quadruple therapy combined with both fucoidan and synbiotics for 6 weeks. QT1, QF1, QS1, and QFS1 represent the results at baseline; QT2, QF2, QS2, and QFS2 represent the results at the end of week 6. (A) No significant differences in the α-diversity were observed among the four groups at baseline. (B) The α-diversity significantly decreased in the QT group; Observed species, Chao 1 and Shannon index also significantly decreased in the QF group. No significant differences in the α-diversity were observed in the QS and QFS groups. Compared with the QT group, the Simpson index at week 6 was significantly higher and the Shannon index tended to be higher in the QF group.
In β-diversity analysis, weighted UniFrac PCoA and Anosim showed that the global microbiota structure in QT group between week 0 and week 6 differed significantly (Figure 2A). However, no significant differences in β-diversity were observed before and after interventions in the other three groups. By week 6, the β-diversity of both the QS and QFS groups tended to be different from that of the QT group, although statistical significance was not reached (Figures 2B,C).
Figure 2. In β-diversity analysis, (A) showed that the global microbiota structure in QT group between week 0 and week 6 differed significantly. QT1 represented the results at baseline and QT2 represented the results at the end of week 6. (B) showed that the global microbiota structure tended to be different between QT2 and QS2,although statistical significance was not reached. (C) showed that the global microbiota structure tended to be different between QT2 and QFS2, although statistical significance was not reached. QT2, QS2 and QFS2 all represented the results at the end of week 6.
The relative abundance of the four dominant phyla, Firmicutes, Bacteroidetes, Proteobacteria, and Actinobacteria, displayed no significant differences between week 0 and week 6 in the four groups. After 4 weeks of the completion of quadruple therapy, the gut microbiota of the QT group was characterized by the decreased relative abundance of the Alphaproteobacteria class and the Coriobacteriaceae family, as well as the increased relative abundance of the Oxyphotobacteria class, as identified by LEfSe. At the genus level, 28 taxa showed significantly different levels between week 0 and week 6, including 8 increased genera and 20 decreased genera. Most of the decreased genera belong to the Lachnospiraceae family (10 genera, including Coprococcus 1, Coprococcus 2, Lachnospiraceae FCS020 group, Lachnospiraceae ND3007 group, Lachnospiraceae NC2004 group, Fusicatenibacter, Dorea, Tyzzerella 3, gauvreauii group, and CAG-56) or Ruminococcaceae (6 genera, including Butyricicoccus, Ruminococcaceae UCG-005, Ruminococcaceae UCG-013, Ruminococcaceae UCG14, Ruminococcus 1, and Subdoligranulum), the others included Collinsella, Megamonas, Ochrobactrum, and Prevotella 9. Among the increased genera, Escherichia-Shigella contributed most to the differences in the microbiome configuration between week 0 and week 6, with the LDA score (log10) close to 4 (Figure 3A).
Figure 3. Differences in the gut microbiota composition between week 0 and week 6 in the QT group. (A–D) showed differences in the gut microbiota composition between week 0 and week 6 in the QT, QF, QS and QFS group, respectively.
In the QF group, the intervention also resulted in significant variations in gut microbiota composition at the genus level. Four genera belonging to Ruminococcaceae (Subdoligranulum, Ruminococcus 1, Ruminococcaceae UCG-005, Ruminococcaceae UCG-014) and 3 genera belonging to Lachnospiraceae (Fusicatenibacter, Coprococcus 2, Lachnospiraceae ND3007 group) were significantly decreased in both QT and QF groups at week 6. Escherichia-Shigella also increased significantly after the intervention in the QF group. Unlike the QT group, Parabacteroides, Akkermansia, Blautia, and Erysipelatoclostridium significantly increased in the QF group at week 6 (Figure 3B).
The results of LEfSe analysis demonstrated that patients in the QS and QFS groups experienced relatively slight microbiota perturbation during the interventions. Escherichia-Shigella significantly increased in the QS group but not in the QFS group. The Pseudomonadaceae family and Pseudomonas significantly increased in the QFS group while significantly decreased in the QS group. Eubacteriaceae family and Eubacterium were significantly more abundant in the QS group after the intervention. Additionally, in the QFS group, Blautia and Erysipelatoclostridium became significantly more abundant while the Christensenellaceae family became significantly less abundant at week 6 (Figures 3C,D).
To further detect the effects of fucoidan and symbiotic supplementation on the variation of microbiota in the context of quadruple therapy, we used LEfSe and Metastats to compare the microbiota taxa between the QT group and the QF, QS, or QFS groups, respectively.
Between the QT and QF groups, LEfSe analysis revealed that Butyricicoccus in Ruminococcaceae family and Ochrobactrum in Rhizobiaceae family were significantly more abundant in the QF group at week 6, while their relative abundances were not significantly different between the two groups at week 0 (Figure 4A). Metastats also confirmed that the level of Butyricicoccus was significantly higher in the QF group at week 6.
Figure 4. (A) showed differences in the gut microbiota composition between QT group and QF group after the intervention. (B) showed differences in the gut microbiota composition between QT group and QS group after the intervention. (C) showed differences in the gut microbiota composition between QT group and QFS group after the intervention.
Between the QT and QS groups, LEfSe showed that 4 genera belonging to Ruminococcaceae (Butyricicoccus, Negativibacillus, RuminococcaceaeUCG-014, coprostanoligenes group), 4 genera belonging to Lachnospiraceae (LachnospiraceaeNC2004group, Coprococcus 1, Coprococcus 3, xylanophilum group), Prevotella 2 in Prevotellaceae, Megamonas in Veillonellaceae, and Barnesiella in Barnesiellaceae had significantly higher levels in QS group at week 6, while the relative abundance of Negativibacillus, Prevotella 2, LachnospiraceaeNC2004group, xylanophilum group did not reach 0.01% (Figure 4B). Metastats also supported that Butyricicoccus, RuminococcaceaeUCG-014, and Megamonas were significantly more abundant in the QS group at week 6.
Between the QT and QFS groups, LEfSe found that the relative abundance of Ochrobactrum and Megamonas significantly increased in the QFS group. Among the genera with a relative abundance of over 0.01%, Tyzzerella 3 and Ruminoclostridium 6 were significantly more abundant while Klebsiella was significantly less abundant in the QFS group (Figure 4C). In parallel, Metastats confirmed the higher abundance of Ochrobactrum in the QFS group and detected that Butyricicoccus was significantly more abundant in the QFS group. The comparison of Butyricicoccus in the two groups at week 6 showed a trend of a higher relative abundance of Butyricicoccus in the QFS group, but statistical significance was not reached. Table 3 shows the relative abundance of the genera mentioned above before and after different interventions, displaying the variations in gut microbiota across different groups.
Table 3. Comparison of the relative abundance of genera between the quadruple therapy group and the groups with fucoidan and/or symbiotic.
To our knowledge, this study is the first to report the effects of fucoidan combined with synbioticss on the gut microbiota of H. pylori-infected patients treated with bismuth quadruple therapy. We comprehensively compared the gut microbiota before and after 4 regimens of therapy in patients with H. pylori infection. As anticipated, quadruple therapy led to significant variations in the microbiome configuration. Although the α-diversity was also decreased in the QF group, a significantly higher level of Simpson index was observed in the QF group compared with the QT group, and β-diversity did not change significantly in the QF group. In contrast, analyses of α-diversity and β-diversity demonstrated that the microbiota composition after the 6 weeks of interventions had no significant alteration compared with baseline in the QS group and the QFS group, with only a few bacterial taxa significantly changed in relative abundance. The supplementation of fucoidan or synbioticss might promote the growth of Butyricicoccus, evidenced by the significantly higher level of Butyricicoccus in all of the QF, QS, and QFS groups after the interventions. Moreover, the combination of fucoidan and synbioticss might inhibit the growth of Escherichia-Shigella, supported by enriched Escherichia-Shigella in the QT, QF, and QS groups except for QFS group.
The perturbation of microbiota caused by eradication therapy has attracted attention in H. pylori infection-related research. It has been demonstrated that the significant reduction of α-diversity of gut microbiota after bismuth quadruple therapy may not be restored even a year after the therapy. This phenomenon was also observed in β-diversity (20). Our results also supported striking variations in α-diversity and β-diversity following quadruple therapy. Chen L et al. found that a 14-day bismuth quadruple therapy induced a significant decrease in the relative abundance of Lachnospiraceae and Ruminococcaceae, in which the stool samples were collected at the end of the therapy (21). Different from Chen’s study, the fecal samples were collected 4 weeks after completion of the quadruple therapy in our study, yet we still observed that most of the decreased genera belonged to Lachnospiraceae or Ruminococcaceae, and the relative abundance of the Coriobacteriaceae family was also significantly decreased. Evidence is accumulating that Lachnospiraceae and Ruminococcaceae contribute to the production of SCFAs from dietary fibers in the gut (22, 23). SCFAs can modulate both innate and adaptive immunity by inhibiting cytokines and regulating migration and differentiation of immune cells (24). Moreover, SCFAs can enhance the expression of tight junction proteins in the intestinal epithelium and help maintain the integrity of the colonic mucus layer, playing an important part in gut barrier function (25, 26). Among the three major SCFAs, namely acetate, propionate, and butyrate, butyrate serves as the main energy source for colonocytes and can inhibit the growth of pathogens by modulating luminal pH (27). It has been reported that genera such as Butyricicoccus (28), Coprococcus (29), and Subdoligranulum (30) can all exert butyrate-producing function. Besides, Dorea, Collinsella, and Fusicatenibacter were found to increase in patients with Crohn’s disease or functional constipation after fecal microbiota transplantation, in parallel with an increase of fecal butyrate (31, 32), suggesting the correlation between these genera and butyrate production. Notably, all of the 6 genera mentioned above significantly decreased in the QT group, indicating the inhibition of growth of these beneficial genera and potential disturbance of SCFAs production caused by bismuth quadruple therapy. In agreement with previous studies (33, 34), we also observed that the opportunistic pathogen in Enterobacteriaceae, Escherichia-Shigella, significantly increased after the bismuth quadruple therapy (35). Escherichia-Shigella may exert a pro-inflammatory effect on the host by increasing serum Lipopolysaccharide (36), potentially promoting low-grade systematic inflammation.
In the present study, fucoidan exhibited the potential in preserving the α-diversity of the gut microbiota. As a soluble dietary fiber, fucoidan from Laminaria japonica can be fermented by the gut microbiota of human and increase the level of SCFAs and several probiotics, such as Bifidobacterium, Lactobacillus, and Enterobacter (37), indicating its prebiotic properties. Animal experiments have shown that fucoidan can significantly increase the α-diversity of microbiota, ameliorate dyslipidemia, protect gut barrier function, and decrease systematic inflammation (38, 39). Our results indicated that the reduction in intestinal microbiota diversity caused by quadruple therapy can be reversed partially by fucoidan. Patients in the QF group also experienced perturbations in gut microbiota, primarily at the genus level. However, different from the QT group, several beneficial genera became significantly more abundant in the QF group after the intervention, including Butyricicoccus, Akkermansia, Blautia, and Parabacteroides. Shi et al. reported that fucoidan supplementation could increase the levels of Butyricicoccus as well as fecal SCFAs and SCFA receptors in the small intestine (40). Additionally, there is also evidence showing that fucoidan can increase the levels of Blautia, Akkermansia, and Parabacteroides (41, 42). Blautia and Parabacteroides can exert the function of SCFAs-production (43, 44), while Akkermansia has been reported to protect the gut barrier function by modulating the number of goblet cells (45). Moreover, Erysipelatoclostridium significantly increased in the QF group compared with baseline level, and Ochrobactrum significantly increased in the QF group at week 6 compared to the QT group. Interestingly, swimming can induce the increase of SCFAs and Erysipelatoclostridium in feces of colitis-associated cancer mice (46), and fecal Ochrobactrum significantly increased after the butyrate supplement in mice (47), indicating that a gut environment with more SCFAs might promote the growth of Erysipelatoclostridium and Ochrobactrum. Collectively, the detrimental effects of bismuth quadruple therapy on gut microbiota, especially on some SCFAs-producing taxa, may be partly mitigated by fucoidan.
Synbioticss, composed of both probiotics and prebiotics, may have superior effects compared to probiotics or prebiotics alone, owing to enhanced probiotic survival in the gastrointestinal tract and stimulation of native beneficial bacterial proliferation by prebiotics (48). Strains of Lactobacillus and Bifidobacterium are mainstream probiotics and have been proven safe and effective, capable of not only producing lactic acid and acetic acid but also promoting the growth of some butyrate-producing bacteria (49). Our findings indicate that synbioticss could protect patients from the harmful effects of bismuth quadruple therapy on the richness and stability of gut microbiota. Moreover, compared to the QT group, the levels of the beneficial genera mentioned above, such as Butyricicoccus and Coprococcus, were significantly higher after the daily use of the synbiotics for 6 weeks. Additionally, Megamonas and Barnesiella also significantly increased in the synbiotics group. Megamonas is reported as SCFAs-producer (50, 51), and there is evidence supporting that the supplement of dietary fiber may facilitate the growth of Megamonas (52). Barnesiella may help to treat infections caused by antibiotic-resistant bacteria (53) and ameliorate oxidative stress levels (54). Moreover, when comparing to the level before intervention, the relative abundance of Eubacterium significantly increased in the synbiotics group, which is a major butyrate producer and participates in the modulation of gut inflammation and maintenance of gut barrier integrity (55). It is worth noting that the addition of fucoidan or synbiotics to bismuth quadruple therapy did not prevent the increase of Escherichia-Shigella, whereas the combined use of these two supplementations showed a promising effect on inhibiting the growth of Escherichia-Shigella. Another interesting finding is that the relative abundance of Klebsiella significantly decreased in the group using both fucoidan and synbioticss. Same as Escherichia-Shigella, Klebsiella spp. are also categorized within Enterobacteriaceae family and are recognized as opportunistic pathogens, with Klebsiella pneumoniae being the most notable one (56). Therefore, in terms of the inhibition of potential pathogens, the combined administration of synbiotics and fucoidan might offer a significant advantage over synbiotics supplementation alone.
In this study, supplementation of fucoidan, synbioticss, or their combination showed no improvement in the eradication rate of bismuth quadruple therapy. Fucoidan has been reported to inhibit the growth of H. pylori and disrupt the adherence of H. pylori to gastric epithelial cells (57). Besides, fucoidan has been demonstrated to dampen inflammation in the stomach of H. pylori-infected mice (58). However, the application of fucoidan in patients with H. pylori infection is limited, and there has been no convincing evidence supporting the efficacy of fucoidan in increasing the eradication rate of H. pylori infection. In terms of synbioticss, a meta-analysis showed that in per-protocol analysis, the eradication rate had no significant improvement when adding synbioticss to H. pylori eradication therapy, despite that pooled effect size from intention-to-treat analysis supported the role of synbioticss in the eradication of H. pylori (59). It should be noted that the eradication rate in the group only used RMAB therapy in our study reached 95.0%, consistent with a previous study showing that the eradication rate of RMAB therapy was 92.6% in per-protocol analysis (60). Therefore, the satisfactory eradication rate of RMAB therapy may leave little space for us to observe the effects of the supplementations on H. pylori eradication.
There were certain limitations in this study. Firstly, the variations in gut microbiota induced by fucoidan may vary with the dosage of fucoidan (16), thus it is necessary to compare the effects of different doses of fucoidan in H. pylori-infected patients treated with bismuth quadruple therapy in a future study, and more beneficial functions of fucoidan may be found besides those on the gut microbiota. Secondly, since the most significant perturbation of gut microbiome may occur at the end of bismuth quadruple therapy (i.e., week 2), collecting stool samples at this time point would provide further insight into the protective role of fucoidan and synbioticss in maintaining intestinal microecology homeostasis. Lastly, multi-center studies with larger sample sizes are required to validate the findings of this study.
This study showed that the gut microbiota of patients with H. pylori infection had remarkable changes after bismuth quadruple therapy, especially the increase in the relative abundance of Escherichia-Shigella and decrease in certain genera of Lachnospiraceae and Ruminococcaceae families. Fucoidan supplementation might ameliorate the adverse effects of quadruple therapy on the diversity of intestinal microbiota. The synbiotics containing Bifidobacterium. spp., Lactobacillus. spp. and dietary fibers could help to maintain the stability of microbiota composition. Fucoidan or synbiotics supplementation might help to increase the relative abundance of several beneficial taxa. Notably, combined supplementation of fucoidan and the synbiotics packet showed the potency to inhibit the growth of potential pathogens including Escherichia-Shigella and Klebsiella, which might be a promising adjuvant regimen to alleviate gut dysbiosis during H. pylori eradication therapy.
The raw data supporting the conclusions of this article will be made available by the authors, without undue reservation.
The studies involving humans were approved by the Ethics Committee of China-Japan Friendship Hospital. The studies were conducted in accordance with the local legislation and institutional requirements. The participants provided their written informed consent to participate in this study.
HW: Formal analysis, Investigation, Methodology, Writing – review & editing. WW: Formal analysis, Investigation, Writing – original draft. FL: Data curation, Validation, Writing – original draft. MW: Investigation, Writing – original draft. YZ: Conceptualization, Supervision, Validation, Writing – review & editing. SD: Conceptualization, Funding acquisition, Project administration, Resources, Writing – review & editing.
The author(s) declare that financial support was received for the research, authorship, and/or publication of this article. This work was supported by grants from Chinese Nutrition Society Nutrition Science Foundation (CNS-BMSG2020-77), Chinese Nutrition Society Nutrition Science Foundation (CNS-ZD2022-127), the Fundamental Research Funds for the Central Universities (3332022001), and China Postdoctoral Science Foundation (2022M710453).
The authors declare that the research was conducted in the absence of any commercial or financial relationships that could be construed as a potential conflict of interest.
All claims expressed in this article are solely those of the authors and do not necessarily represent those of their affiliated organizations, or those of the publisher, the editors and the reviewers. Any product that may be evaluated in this article, or claim that may be made by its manufacturer, is not guaranteed or endorsed by the publisher.
1. FitzGerald, R, and Smith, SM. An overview of Helicobacter pylori infection. Methods Mol Biol. (2021) 2283:1–14. doi: 10.1007/978-1-0716-1302-3_1
2. Hooi, JKY, Lai, WY, Ng, WK, Suen, MMY, Underwood, FE, Tanyingoh, D, et al. Global prevalence of Helicobacter pylori infection: systematic review and Meta-analysis. Gastroenterology. (2017) 153:420–9. doi: 10.1053/j.gastro.2017.04.022
3. Ren, S, Cai, P, Liu, Y, Wang, T, Zhang, Y, Li, Q, et al. Prevalence of Helicobacter pylori infection in China: a systematic review and meta-analysis. J Gastroenterol Hepatol. (2022) 37:464–70. doi: 10.1111/jgh.15751
4. Sun, QJ, Liang, X, Zheng, Q, Gu, WQ, Liu, WZ, Xiao, SD, et al. Resistance of Helicobacter pylori to antibiotics from 2000 to 2009 in Shanghai. World J Gastroenterol. (2010) 16:5118–21. doi: 10.3748/wjg.v16.i40.5118
5. Malfertheiner, P, Megraud, F, O'Morain, CA, Gisbert, JP, Kuipers, EJ, Axon, AT, et al. Management of Helicobacter pylori infection—the Maastricht V/Florence consensus report. Gut. (2017) 66:6–30. doi: 10.1136/gutjnl-2016-312288
6. Lee, SY . Current progress toward eradicating helicobacter pylori in east Asian countries: differences in the 2013 revised guidelines between China, Japan, and South Korea. World J Gastroenterol. (2014) 20:1493–502. doi: 10.3748/wjg.v20.i6.1493
7. Dang, Y, Reinhardt, JD, Zhou, X, and Zhang, G. The effect of probiotics supplementation on Helicobacter pylori eradication rates and side effects during eradication therapy: a meta-analysis. PLoS One. (2014) 9:e111030. doi: 10.1371/journal.pone.0111030
8. Vo, T-S, and Kim, S-K. Fucoidans as a natural bioactive ingredient for functional foods. J Funct Foods. (2013) 5:16–27. doi: 10.1016/j.jff.2012.08.007
9. Zhao, D, Xu, J, and Xu, X. Bioactivity of fucoidan extracted from Laminaria japonica using a novel procedure with high yield. Food Chem. (2018) 245:911–8. doi: 10.1016/j.foodchem.2017.11.083
10. Lin, Y, Qi, X, Liu, H, Xue, K, Xu, S, and Tian, Z. The anti-cancer effects of fucoidan: a review of both in vivo and in vitro investigations. Cancer Cell Int. (2020) 20:154. doi: 10.1186/s12935-020-01233-8
11. Iwamoto, K, Hiragun, T, Takahagi, S, Yanase, Y, Morioke, S, Mihara, S, et al. Fucoidan suppresses IgE production in peripheral blood mononuclear cells from patients with atopic dermatitis. Arch Dermatol Res. (2011) 303:425–31. doi: 10.1007/s00403-010-1115-7
12. Apostolova, E, Lukova, P, Baldzhieva, A, Katsarov, P, Nikolova, M, Iliev, I, et al. Immunomodulatory and anti-inflammatory effects of Fucoidan: a review. Polymers (Basel). (2020) 12:12. doi: 10.3390/polym12102338
13. Gotteland, M, Riveros, K, Gasaly, N, Carcamo, C, Magne, F, Liabeuf, G, et al. The pros and cons of using algal polysaccharides as prebiotics. Front Nutr. (2020) 7:163. doi: 10.3389/fnut.2020.00163
14. van Weelden, G, Bobinski, M, Okla, K, van Weelden, WJ, Romano, A, and Pijnenborg, JMA. Fucoidan structure and activity in relation to anti-cancer mechanisms. Mar Drugs. (2019) 17:17. doi: 10.3390/md17010032
15. Xue, M, Ji, X, Liang, H, Liu, Y, Wang, B, Sun, L, et al. The effect of fucoidan on intestinal flora and intestinal barrier function in rats with breast cancer. Food Funct. (2018) 9:1214–23. doi: 10.1039/c7fo01677h
16. Wei, Q, Xing, M, Wang, K, Yang, Q, Zhao, J, Wang, Y, et al. Fucoidan is not completely dependent on degradation to Fucose to relieve ulcerative colitis. Pharmaceuticals (Basel). (2022) 15:15. doi: 10.3390/ph15040430
17. Wang, L, Ai, C, Wen, C, Qin, Y, Liu, Z, Wang, L, et al. Fucoidan isolated from Ascophyllum nodosum alleviates gut microbiota dysbiosis and colonic inflammation in antibiotic-treated mice. Food Funct. (2020) 11:5595–606. doi: 10.1039/d0fo00668h
18. Kan, J, Cheng, J, Xu, L, Hood, M, Zhong, D, Cheng, M, et al. The combination of wheat peptides and fucoidan protects against chronic superficial gastritis and alters gut microbiota: a double-blinded, placebo-controlled study. Eur J Nutr. (2020) 59:1655–66. doi: 10.1007/s00394-019-02020-6
19. Besednova, NN, Zaporozhets, TS, Somova, LM, and Kuznetsova, TA. Review: prospects for the use of extracts and polysaccharides from marine algae to prevent and treat the diseases caused by Helicobacter pylori. Helicobacter. (2015) 20:89–97. doi: 10.1111/hel.12177
20. Liou, JM, Chen, CC, Chang, CM, Fang, YJ, Bair, MJ, Chen, PY, et al. Long-term changes of gut microbiota, antibiotic resistance, and metabolic parameters after Helicobacter pylori eradication: a multicentre, open-label, randomised trial. Lancet Infect Dis. (2019) 19:1109–20. doi: 10.1016/S1473-3099(19)30272-5
21. Chen, L, Xu, W, Lee, A, He, J, Huang, B, Zheng, W, et al. The impact of Helicobacter pylori infection, eradication therapy and probiotic supplementation on gut microenvironment homeostasis: an open-label, randomized clinical trial. EBioMedicine. (2018) 35:87–96. doi: 10.1016/j.ebiom.2018.08.028
22. Xie, J, Li, LF, Dai, TY, Qi, X, Wang, Y, Zheng, TZ, et al. Short-chain fatty acids produced by Ruminococcaceae mediate alpha-linolenic acid promote intestinal stem cells proliferation. Mol Nutr Food Res. (2022) 66:e2100408. doi: 10.1002/mnfr.202100408
23. Vacca, M, Celano, G, Calabrese, FM, Portincasa, P, Gobbetti, M, and De Angelis, M. The controversial role of human gut Lachnospiraceae. Microorganisms. (2020) 8:8. doi: 10.3390/microorganisms8040573
24. Steinmeyer, S, Lee, K, Jayaraman, A, and Alaniz, RC. Microbiota metabolite regulation of host immune homeostasis: a mechanistic missing link. Curr Allergy Asthma Rep. (2015) 15:24. doi: 10.1007/s11882-015-0524-2
25. Wang, HB, Wang, PY, Wang, X, Wan, YL, and Liu, YC. Butyrate enhances intestinal epithelial barrier function via up-regulation of tight junction protein Claudin-1 transcription. Dig Dis Sci. (2012) 57:3126–35. doi: 10.1007/s10620-012-2259-4
26. Schroeder, BO, Birchenough, GMH, Stahlman, M, Arike, L, Johansson, MEV, Hansson, GC, et al. Bifidobacteria or Fiber protects against diet-induced microbiota-mediated colonic mucus deterioration. Cell Host Microbe. (2018) 23:e7:27–40.e7. doi: 10.1016/j.chom.2017.11.004
27. Fu, X, Liu, Z, Zhu, C, Mou, H, and Kong, Q. Nondigestible carbohydrates, butyrate, and butyrate-producing bacteria. Crit Rev Food Sci Nutr. (2019) 59:S130–52. doi: 10.1080/10408398.2018.1542587
28. Zeng, Q, Li, D, He, Y, Li, Y, Yang, Z, Zhao, X, et al. Discrepant gut microbiota markers for the classification of obesity-related metabolic abnormalities. Sci Rep. (2019) 9:13424. doi: 10.1038/s41598-019-49462-w
29. Altemani, F, Barrett, HL, Gomez-Arango, L, Josh, P, David McIntyre, H, Callaway, LK, et al. Pregnant women who develop preeclampsia have lower abundance of the butyrate-producer Coprococcus in their gut microbiota. Pregnancy Hypertens. (2021) 23:211–9. doi: 10.1016/j.preghy.2021.01.002
30. Gerasimidis, K, Bryden, K, Chen, X, Papachristou, E, Verney, A, Roig, M, et al. The impact of food additives, artificial sweeteners and domestic hygiene products on the human gut microbiome and its fibre fermentation capacity. Eur J Nutr. (2020) 59:3213–30. doi: 10.1007/s00394-019-02161-8
31. Osaki, H, Jodai, Y, Koyama, K, Omori, T, Horiguchi, N, Kamano, T, et al. Clinical response and changes in the fecal microbiota and metabolite levels after fecal microbiota transplantation in patients with inflammatory bowel disease and recurrent Clostridioides difficile infection. Fujita Med J. (2021) 7:87–98. doi: 10.20407/fmj.2020-021
32. Zhang, X, Li, N, Chen, Q, and Qin, H. Fecal microbiota transplantation modulates the gut Flora favoring patients with functional constipation. Front Microbiol. (2021) 12:700718. doi: 10.3389/fmicb.2021.700718
33. Zhou, Y, Ye, Z, Wang, Y, Huang, Z, Zheng, C, Shi, J, et al. Long-term changes in the gut microbiota after triple therapy, sequential therapy, bismuth quadruple therapy and concomitant therapy for Helicobacter pylori eradication in Chinese children. Helicobacter. (2021) 26:e12809. doi: 10.1111/hel.12809
34. Guillemard, E, Poirel, M, Schafer, F, Quinquis, L, Rossoni, C, Keicher, C, et al. A randomised, controlled trial: effect of a multi-strain fermented Milk on the gut microbiota recovery after Helicobacter pylori therapy. Nutrients. (2021) 13:3171. doi: 10.3390/nu13093171
35. Xu, X, Wang, H, Guo, D, Man, X, Liu, J, Li, J, et al. Curcumin modulates gut microbiota and improves renal function in rats with uric acid nephropathy. Ren Fail. (2021) 43:1063–75. doi: 10.1080/0886022X.2021.1944875
36. Peng, L, Gao, X, Nie, L, Xie, J, Dai, T, Shi, C, et al. Astragalin attenuates dextran sulfate sodium (DSS)-induced acute experimental colitis by alleviating gut microbiota Dysbiosis and inhibiting NF-kappaB activation in mice. Front Immunol. (2020) 11:2058. doi: 10.3389/fimmu.2020.02058
37. Kong, Q, Dong, S, Gao, J, and Jiang, C. In vitro fermentation of sulfated polysaccharides from E. prolifera and L. japonica by human fecal microbiota. Int J Biol Macromol. (2016) 91:867–71. doi: 10.1016/j.ijbiomac.2016.06.036
38. Huang, J, Huang, J, Li, Y, Lv, H, Yin, T, Fan, S, et al. Fucoidan protects against high-fat diet-induced obesity and modulates gut microbiota in Institute of Cancer Research mice. J Med Food. (2021) 24:1058–67. doi: 10.1089/jmf.2021.K.0030
39. Zhang, Y, Zuo, J, Yan, L, Cheng, Y, Li, Q, Wu, S, et al. Sargassum fusiforme Fucoidan alleviates high-fat diet-induced obesity and insulin resistance associated with the improvement of hepatic oxidative stress and gut microbiota profile. J Agric Food Chem. (2020) 68:10626–38. doi: 10.1021/acs.jafc.0c02555
40. Shi, H, Chang, Y, Gao, Y, Wang, X, Chen, X, Wang, Y, et al. Dietary fucoidan of Acaudina molpadioides alters gut microbiota and mitigates intestinal mucosal injury induced by cyclophosphamide. Food Funct. (2017) 8:3383–93. doi: 10.1039/c7fo00932a
41. Wu, Q, Wu, S, Cheng, Y, Zhang, Z, Mao, G, Li, S, et al. Sargassum fusiforme fucoidan modifies gut microbiota and intestinal metabolites during alleviation of hyperglycemia in type 2 diabetic mice. Food Funct. (2021) 12:3572–85. doi: 10.1039/d0fo03329d
42. Jia, J, Zheng, W, Zhang, C, Zhang, P, Guo, X, Song, S, et al. Fucoidan from Scytosiphon lomentaria protects against destruction of intestinal barrier, inflammation and lipid abnormality by modulating the gut microbiota in dietary fibers-deficient mice. Int J Biol Macromol. (2023) 224:556–67. doi: 10.1016/j.ijbiomac.2022.10.144
43. Liu, X, Mao, B, Gu, J, Wu, J, Cui, S, Wang, G, et al. Blautia-a new functional genus with potential probiotic properties? Gut Microbes. (2021) 13:1–21. doi: 10.1080/19490976.2021.1875796
44. Li, W, Zhang, K, and Yang, H. Pectin alleviates high fat (lard) diet-induced nonalcoholic fatty liver disease in mice: possible role of short-chain fatty acids and gut microbiota regulated by pectin. J Agric Food Chem. (2018) 66:8015–25. doi: 10.1021/acs.jafc.8b02979
45. Shin, NR, Lee, JC, Lee, HY, Kim, MS, Whon, TW, Lee, MS, et al. An increase in the Akkermansia spp. population induced by metformin treatment improves glucose homeostasis in diet-induced obese mice. Gut. (2014) 63:727–35. doi: 10.1136/gutjnl-2012-303839
46. Wang, W, Xu, Y, Wang, X, Chu, Y, Zhang, H, Zhou, L, et al. Swimming impedes intestinal microbiota and lipid metabolites of tumorigenesis in colitis-associated cancer. Front Oncol. (2022) 12:929092. doi: 10.3389/fonc.2022.929092
47. Li, X, Lei, S, Liu, L, Zhang, Y, Zheng, B, and Zeng, H. Synergistic effect of lotus seed resistant starch and short-chain fatty acids on mice fecal microbiota in vitro. Int J Biol Macromol. (2021) 183:2272–81. doi: 10.1016/j.ijbiomac.2021.06.016
48. Markowiak, P, and Slizewska, K. Effects of probiotics, prebiotics, and Synbioticss on human health. Nutrients. (2017) 9:1021. doi: 10.3390/nu9091021
49. Sanders, ME, Merenstein, DJ, Reid, G, Gibson, GR, and Rastall, RA. Probiotics and prebiotics in intestinal health and disease: from biology to the clinic. Nat Rev Gastroenterol Hepatol. (2019) 16:605–16. doi: 10.1038/s41575-019-0173-3
50. Xu, Y, Wang, Y, Li, H, Dai, Y, Chen, D, Wang, M, et al. Altered fecal microbiota composition in older adults with frailty. Front Cell Infect Microbiol. (2021) 11:696186. doi: 10.3389/fcimb.2021.696186
51. Qin, R, Wang, J, Chao, C, Yu, J, Copeland, L, Wang, S, et al. RS5 produced more butyric acid through regulating the microbial Community of Human gut Microbiota. J Agric Food Chem. (2021) 69:3209–18. doi: 10.1021/acs.jafc.0c08187
52. Zhao, J, Nian, L, Kwok, LY, Sun, T, and Zhao, J. Reduction in fecal microbiota diversity and short-chain fatty acid producers in methicillin-resistant Staphylococcus aureus infected individuals as revealed by PacBio single molecule, real-time sequencing technology. Eur J Clin Microbiol Infect Dis. (2017) 36:1463–72. doi: 10.1007/s10096-017-2955-2
53. Bilinski, J, Grzesiowski, P, Sorensen, N, Madry, K, Muszynski, J, Robak, K, et al. Fecal microbiota transplantation in patients with blood disorders inhibits gut colonization with antibiotic-resistant Bacteria: results of a prospective, single-center study. Clin Infect Dis. (2017) 65:364–70. doi: 10.1093/cid/cix252
54. Xu, D, Pan, D, Liu, H, Yang, C, Yang, X, Wang, X, et al. Improvement in cardiometabolic risk markers following an oatmeal diet is associated with gut microbiota in mildly hypercholesterolemic individuals. Food Res Int. (2022) 160:111701. doi: 10.1016/j.foodres.2022.111701
55. Mukherjee, A, Lordan, C, Ross, RP, and Cotter, PD. Gut microbes from the phylogenetically diverse genus Eubacterium and their various contributions to gut health. Gut Microbes. (2020) 12:1802866. doi: 10.1080/19490976.2020.1802866
56. Dong, N, Yang, X, Chan, EW, Zhang, R, and Chen, S. Klebsiella species: taxonomy, hypervirulence and multidrug resistance. EBioMedicine. (2022) 79:103998. doi: 10.1016/j.ebiom.2022.103998
57. Chua, EG, Verbrugghe, P, Perkins, TT, and Tay, CY. Fucoidans disrupt adherence of Helicobacter pylori to AGS cells in vitro. Evid Based Complement Alternat Med. (2015) 2015:120981:1–6. doi: 10.1155/2015/120981
58. Chen, BR, Li, WM, Li, TL, Chan, YL, and Wu, CJ. Fucoidan from Sargassum hemiphyllum inhibits infection and inflammation of Helicobacter pylori. Sci Rep. (2022) 12:429. doi: 10.1038/s41598-021-04151-5
59. Pourmasoumi, M, Najafgholizadeh, A, Hadi, A, Mansour-Ghanaei, F, and Joukar, F. The effect of synbioticss in improving Helicobacter pylori eradication: a systematic review and meta-analysis. Complement Ther Med. (2019) 43:36–43. doi: 10.1016/j.ctim.2019.01.005
Keywords: Helicobacter pylori infection, quadruple therapy, fucoidan, synbiotics, gut microbiota
Citation: Wang H, Wei W, Liu F, Wang M, Zhang Y and Du S (2024) Effects of fucoidan and synbiotics supplementation during bismuth quadruple therapy of Helicobacter pylori infection on gut microbial homeostasis: an open-label, randomized clinical trial. Front. Nutr. 11:1407736. doi: 10.3389/fnut.2024.1407736
Received: 27 March 2024; Accepted: 18 June 2024;
Published: 01 July 2024.
Edited by:
Palash Mandal, Charotar University of Science and Technology, IndiaReviewed by:
Bowen Yan, Nanjing Forestry University, ChinaCopyright © 2024 Wang, Wei, Liu, Wang, Zhang and Du. This is an open-access article distributed under the terms of the Creative Commons Attribution License (CC BY). The use, distribution or reproduction in other forums is permitted, provided the original author(s) and the copyright owner(s) are credited and that the original publication in this journal is cited, in accordance with accepted academic practice. No use, distribution or reproduction is permitted which does not comply with these terms.
*Correspondence: Yanli Zhang, MTM1MjEyMzQwNjdAMTYzLmNvbQ==; Shiyu Du, ZHVzaGl5dTE5NzVAMTI2LmNvbQ==
†These authors have contributed equally to this work
Disclaimer: All claims expressed in this article are solely those of the authors and do not necessarily represent those of their affiliated organizations, or those of the publisher, the editors and the reviewers. Any product that may be evaluated in this article or claim that may be made by its manufacturer is not guaranteed or endorsed by the publisher.
Research integrity at Frontiers
Learn more about the work of our research integrity team to safeguard the quality of each article we publish.