- 1Food Processing Research Institute, Heilongjiang Academy of Agricultural Sciences, Harbin, China
- 2Heilongjiang Province Key Laboratory of Food Processing, Harbin, China
- 3Heilongjiang Province Engineering Research Center of Whole Grain Nutritious Food, Harbin, China
Introduction: Germinated brown rice is a functional food with a promising potential for alleviating metabolic diseases. This study aimed to explore the hypolipidemic effects of autoclaving-treated germinated brown rice (AGBR) and the underlying mechanisms involving gut microbiota.
Methods: Dietary intervention with AGBR or polished rice (PR) was implemented in patients with hyperlipidemia for 3 months, and blood lipids were analyzed. Nutritional characteristics of AGBR and PR were measured and compared. Additionally, 16S rDNA sequencing was performed to reveal the differences in gut microbiota between the AGBR and PR groups.
Results: AGBR relieves hyperlipidemia in patients, as evidenced by reduced levels of triglycerides, total cholesterol, low-density lipoprotein cholesterol, and apolipoprotein-B, and elevated levels of high-density lipoprotein cholesterol and apolipoprotein-A1. In terms of nutrition, AGBR had significantly higher concentrations of free amino acids (10/16 species), γ-aminobutyric acid, resistant starch, soluble dietary fiber, and flavonoids (11/13 species) than PR. In addition, higher microbial abundance, diversity, and uniformity were observed in the AGBR group than in the PR group. At the phylum level, AGBR reduced Firmicutes, Proteobacteria, Desulfobacterota, and Synergistota, and elevated Bacteroidota and Verrucomicrobiota. At the genus level, AGBR elevated Bacteroides, Faecalibacterium, Dialister, Prevotella, and Bifidobacterium, and reduced Escherichia-Shigella, Blautia, Romboutsia, and Turicibacter.
Discussion: AGBR contributes to the remission of hyperlipidemia by modulating the gut microbiota.
1 Introduction
Hyperlipidemia is a common pathological condition in humans and is characterized by an abnormal elevation of blood lipids (1). High-fat diet, physical inactivity, and smoking are the main modifiable risk factors for hyperlipidemia that directly contribute to an increase in its prevalence worldwide (2). As lipid disorders are the leading causes of cardiovascular diseases, active treatment and prevention of hyperlipidemia are necessary in clinical practice (3).
Dietary habits are closely associated with the occurrence and development of hyperlipidemia. With the advancement of functional foods, a variety of crops have been developed that exhibit therapeutic potential for hyperlipidemia, such as oats (4), tartary buckwheat (5), black rice (6), and giant embryo rice (7). Brown rice is a whole grain with a bran layer and germ, and its sensory and nutritional qualities are improved by germination (8). Germinated brown rice (GBR) is not only rich in minerals, vitamins, essential amino acids, and dietary fibers, but also in various bioactive components, such as ferulic acid, γ-oryzanol, and γ-aminobutyric acid (GABA) (9). As a promising functional food, GBR is beneficial for the treatment of various human disorders, including hyperlipidemia, hypertension, diabetes, cardiovascular diseases, Alzheimer’s disease, and cancers (9). GBR has also been shown to contribute to the remission of hyperlipidemia by improving lipid metabolism (10–12). However, previous studies have mainly been conducted in animal models, and our knowledge of the hypolipidemic effects and mechanisms of GBR in the human body remains limited.
The gut microbiota is a complex system composed of large and diverse microbial communities (13). The gut microbiota plays a crucial role in maintaining human health, and its dysbiosis is associated with the progression of various human diseases, such as metabolic, gastrointestinal, cardiovascular, and neurodegenerative diseases (14). Notably, lipid metabolism in vivo can be affected by the gut microbiota, and hyperlipidemia is associated with obvious alterations in microbial composition (15). Previous studies have reported that many cereal foods are beneficial to the remission of hyperlipidemia via mediating gut microbiota homeostasis, such as oats (4), tartary buckwheat (5), black rice (6), red yeast rice (16), probiotics-fermented rice buckwheat (17), and highland barley (18). Zhao et al. reported that GBR relieves hyperglycemia, insulin resistance, and inflammation in high-fat diet-treated mice by increasing the abundance of probiotic bacteria such as Lactobacillus gasseri (19). However, how the gut microbiota participates in the action of GBR on hyperlipidemia in the human body remains unclear.
Autoclaving-treated GBR (AGBR) is a type of processed rice with high-quality taste, aroma, and nutrition (20). Our previous study showed that autoclaving improved the nutrition and storage of GBR by influencing the metabolites (21). In this study, AGBR and polished rice (PR) diets were implemented in patients with hyperlipidemia. After dietary intervention for 3 months, blood lipids and gut microbiota were analyzed and compared. We aimed to reveal the therapeutic potential of AGBR in hyperlipidemia and its mechanism of action involving the gut microbiota.
2 Materials and methods
2.1 Participants
A total of 56 patients with hyperlipidemia (25–65 years old; 32 men and 24 women) were included in the study. All enrolled patients had total cholesterol (TC) > 5.18 mmol/L and/or triglyceride (TG) > 1.70 mmol/L. The exclusion criteria were as follows: (1) pregnant or lactating women; (2) individuals with severe diseases involving the heart, liver, kidneys, hematopoietic system, or gastrointestinal system; (3) individuals with an allergic constitution or allergy to rice; (4) individuals with psychiatric disorders; and (5) excessive smoking or alcohol abuse.
2.2 Materials
Suijing 18, a Japonica cultivar (Suihua Branch of Heilongjiang Academy of Agricultural Sciences, Harbin, China), was used as the experimental material. Brown rice without husks and PR without a bran layer or germs were processed. To obtain AGBR, brown rice was germinated at 30°C and 95% humidity for 40 h, and then treated at 115°C for 20 min in a pressure-sterilizing pot. The PR and AGBR used for the intervention were semi-finished products in a vacuum package.
2.3 Dietary interventions
Before consumption, the product was steamed for 15 min. The enrolled patients were randomly divided into the PR and AGBR groups (N = 28 in each group). The PR or AGBR products were consumed for lunch (200 g) and dinner (120 g) as alternatives to the principal food. The dietary intervention lasted for 3 months. This study was approved by the Ethics Committee of Lianshui People’s Hospital Affiliated to Kanda College of Nanjing Medical University, and informed consent was obtained from all participants.
2.4 Measurement of biochemical parameters
Whole blood samples were collected from patients at baseline (0 month) and 3 months after dietary intervention. After centrifugation at 2500 rpm for 10 min at 4°C, the supernatants (serum samples) were collected and immediately used for measurements. Blood lipid parameters, including TG; TC, high-density lipoprotein cholesterol (HDL-c), low-density lipoprotein cholesterol (LDL-c), apolipoprotein-A1, and apolipoprotein-B, as well as liver function parameters, including alanine aminotransferase (ALT), aspartate aminotransferase (AST), and total bile acid (TBA) in the serum were measured by an automatic biochemical analyzer (GRT-3006, Glitter, Jinan, China) using specific kits (blood lipid parameters: Mlbio, Shanghai, China; liver function parameters: Jiancheng, Nanjing, China). Measurements were performed in triplicate for each patient.
2.5 Measurement of nutritional parameters
Nutritional characteristics of AGBR and PR were analyzed based on amino acids, dietary fiber, resistant starch, GABA, and flavonoids. Free amino acids (16 types) were measured using an amino acid analyzer. Soluble dietary fiber was measured using the enzyme weight method and resistant starch was measured by spectrophotometry (absorbance at 510 nm). GABA and flavonoids (13 types) were measured using a high-performance liquid chromatography-O-Orbitrap-mass spectrometry system (Vanquish, Thermo Fisher Scientific, Waltham, MA, United States), and the data were acquired using Xcalibur 4.1 and processed using TraceFinder 4.1 (Thermo Fisher Scientific). Measurements were performed in triplicate for each patient.
2.6 16S rDNA sequencing
Fecal samples were collected at baseline (0 month) and 3 months after dietary intervention, and were stored in sterile tubes at −80°C until further use (PR, N = 19; AGBR, N = 18). Genomic DNA was extracted from fecal samples of patients with hyperlipidemia using the CTAB method. The V3–V4 regions of 16S rDNAs were amplified by PCR, and the products were purified. Subsequently, a library was constructed using the NEBNext Ultra II DNA Library Prep Kit (New England Biolabs, Ipswich, MA, United States). The established library was quantified using a Qubit 2.0 fluorometer (Life Technologies, Carlsbad, CA, United States), assessed using an Agilent 2100 Bioanalyzer system (Agilent, Santa Clara, CA, United States), and finally sequenced using NovaSeq 6000 (Illumina, San Diego, CA, United States).
2.7 Microbial analyses
Reads were assigned to samples and merged into raw tags using FLASH after removing barcodes and primer sequences. Effective tags were obtained by screening high-quality clean tags using fastp, and filtering chimeras using Usearch. Effective tags were then denoised using DADA2 to obtain amplicon sequence variants (ASVs). The ASV species information was annotated using QIIME2 based on the Silva138.1 database. Alpha (goods_coverage, chao1, observed_utos, Shannon, Simpson, and pielou_e) and beta diversities (unweighted and weighted PCoA) were analyzed using QIIME2.
2.8 Statistical analyses
Quantitative data were expressed as mean ± standard deviation (SD) and were statistically analyzed using SPSS (version 17.0, SPSS Inc., Chicago, IL, United States). The differences between the PR and AGBR groups were determined using independent t test. Differences were considered statistically significant for p < 0.05.
3 Results
3.1 Dietary intervention of AGBR reduces blood lipids in patients with hyperlipidemia
The potential of AGBR in lowering blood lipid levels was evaluated in patients with hyperlipidemia. As shown in Table 1, dietary intervention with AGBR for 3 months significantly reduced TG, TC, and LDL-c levels in patients with hyperlipidemia (p < 0.05). Patients with hyperlipidemia who consumed AGBR for 3 months also exhibited significantly higher HDL-c levels than those who consumed PR (p < 0.01). The levels of apolipoproteins (A1 and B) were measured to determine the effects of AGBR on hyperlipidemia. As expected, 3 months of AGBR diet significantly elevated apolipoprotein-A1 levels; however, it reduced apolipoprotein-B levels in patients with hyperlipidemia (p < 0.05) (Table 1).
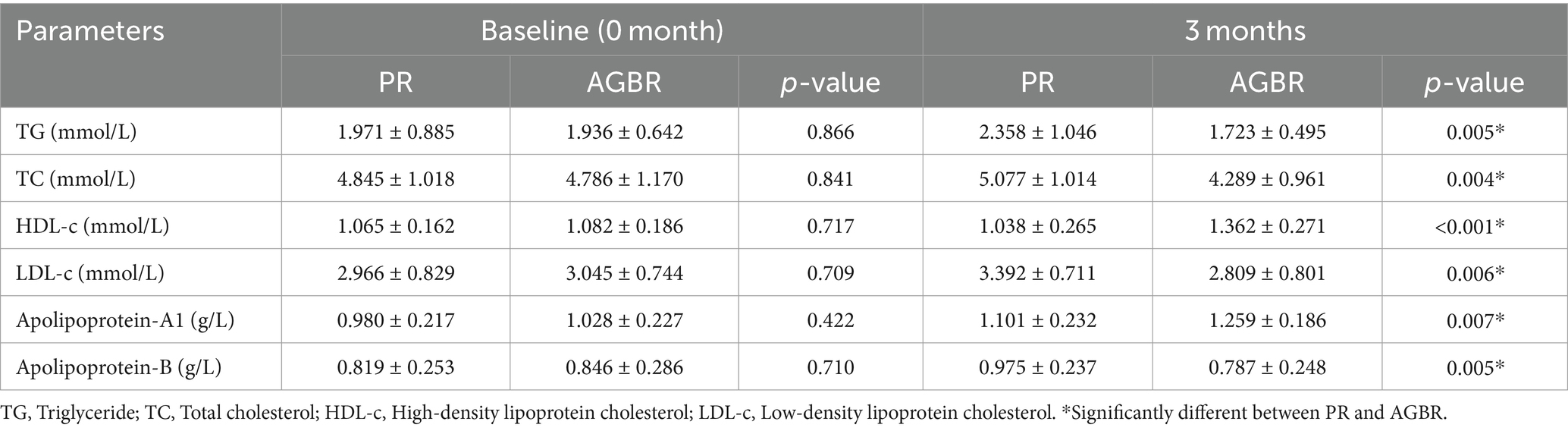
Table 1. Blood lipids in patients with hyperlipidemia who consumed polished rice (PR) or autoclaving-treated germinated brown rice (AGBR).
3.2 Dietary intervention of AGBR does not affect liver function in patients with hyperlipidemia
As liver function is closely associated with lipid metabolism, some representative parameters were measured in patients with hyperlipidemia who consumed PR or AGBR. At baseline (0 month), no significant differences in liver function parameters were observed between the PR and AGBR groups. After 3 months of dietary intervention, ALT, AST, and TBA levels were slightly decreased in patients with hyperlipidemia who consumed AGBR compared to those who consumed PR; however, the differences were not significant (Table 2).

Table 2. Liver function in patients with hyperlipidemia who consumed polished rice (PR) or autoclaving-treated germinated brown rice (AGBR).
3.3 Nutritional characteristics of AGBR
The nutritional characteristics of AGBR and PR were compared to reveal the underlying mechanisms by which AGBR alleviates hyperlipidemia. As shown in Table 3, the levels of most free amino acids (10/16) in AGBR were higher than those in PR (p < 0.05). Compared with PR, AGBR also exhibited significantly higher concentrations of GABA, resistant starch, and soluble dietary fiber (p < 0.05). Additionally, the concentrations of 11/13 flavonoids were significantly higher in AGBR than in PR (p < 0.05).
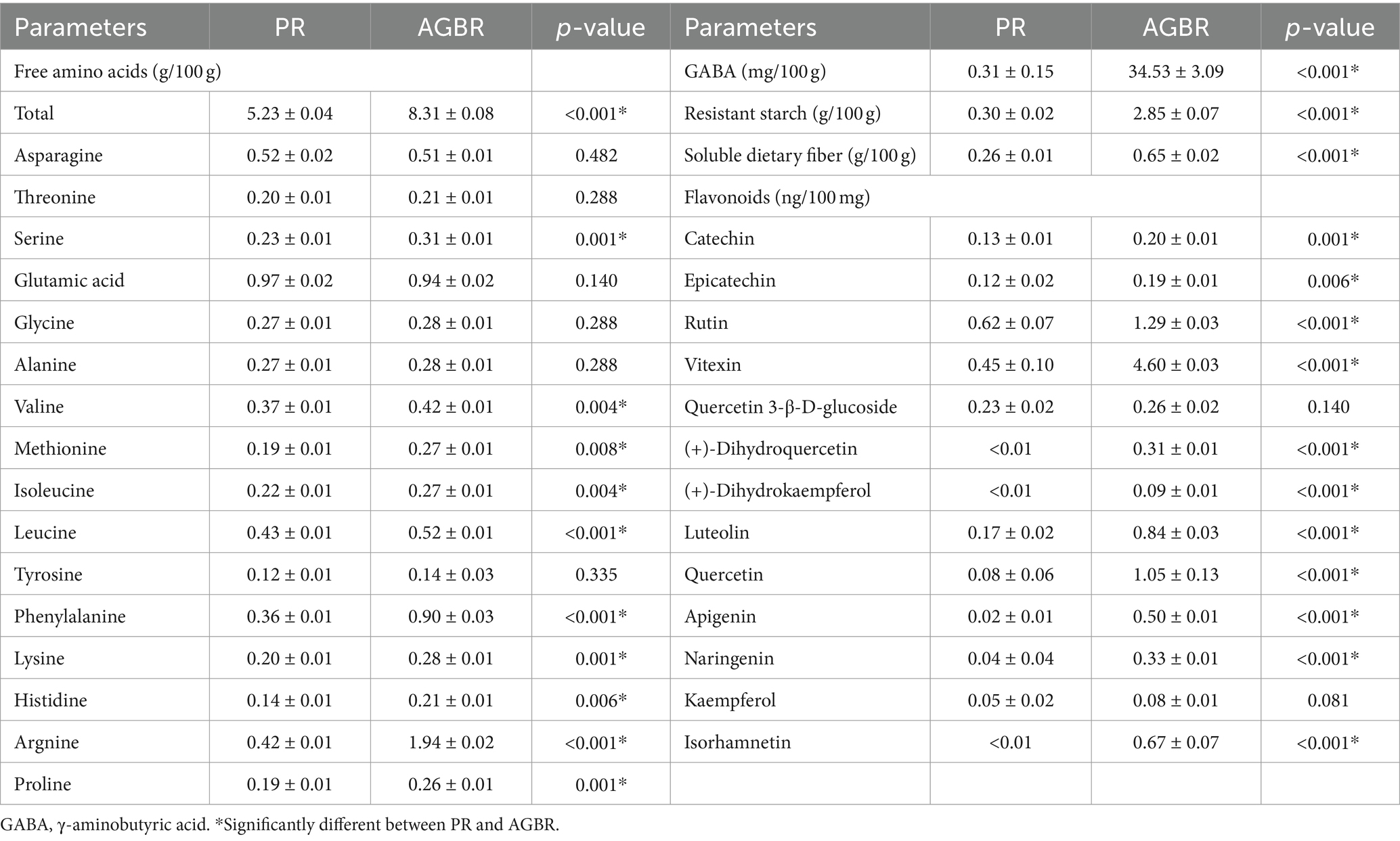
Table 3. Comparisons of nutritional parameters between polished rice (PR) and autoclaving-treated germinated brown rice (AGBR).
3.4 Dietary intervention with AGBR changes gut microbiota in patients with hyperlipidemia
To understand the mechanisms of action of AGBR in the gut microenvironment, the gut microbiota of patients with hyperlipidemia was analyzed using 16S rDNA sequencing. As shown in Figure 1A, 1,237 and 1,259 ASVs were detected in the AGBR and PR groups, respectively. A total of 638 ASVs overlapped between the two groups.
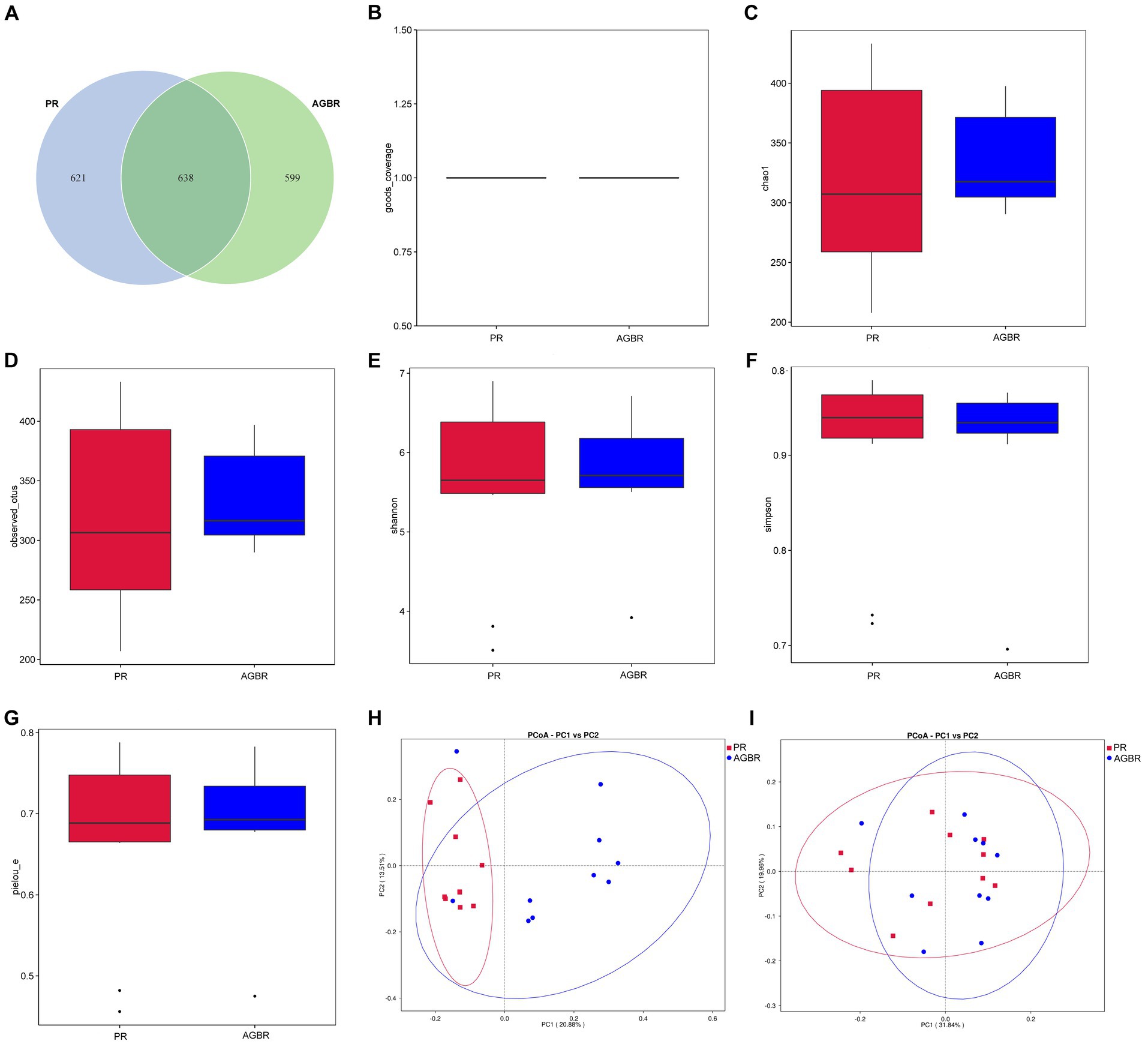
Figure 1. The distribution of gut microbiota in patients with hyperlipidemia receiving AGBR or PR diets. (A) Venn diagram. (B) Goods_coverage. (C) Chao1. (D) Observed_utos. (E) Shannon. (F) Simpson. (G) Pielou_e. (H) Unweighted PCoA. (I) Weighted PCoA.
Subsequently, an alpha diversity analysis was performed. The goods_coverage was close to one in both groups, indicating sufficient sequencing depth (Figure 1B). The chao1 and observed_utos, which represent the microbial abundance, were both slightly higher in the AGBR group than in the PR group (Figures 1C,D). However, microbial diversity was slightly higher in the PR group than that in the AGBR group, with relatively higher Shannon and lower Simpson indices (Figures 1E,F). Additionally, the pielou_e, which represents microbial uniformity, was slightly higher in the AGBR group (Figure 1G). Microbial differences between the two groups were further analyzed using beta diversity. As shown in Figures 1H,I, a certain overlap and difference in microbial composition was observed between the AGBR and PR groups, either by weighted or unweighted PCoA.
3.5 Differential microbiota in patients with hyperlipidemia receiving AGBR or PR diets
Differences in the gut microbiota between the AGBR and PR groups were further analyzed. As shown in Figure 2A, Firmicutes, Bacteroidota, and Proteobacteria were the dominant phyla in the PR group, accounting for 56.47, 17.25, and 20.09%, respectively. Dietary intervention with AGBR decreased Firmicutes (53.51%) and Proteobacteria (8.41%); however, it elevated Bacteroidota (23.67%) in patients with hyperlipidemia. Some microbial phyla with relatively low abundance were also changed by the intervention of AGBR, such as Verrucomicrobiota (0.25% vs. 5.98%), Desulfobacterota (0.65% vs. 0.45%), and Synergistota (0.08% vs. 0.06%). Additionally, the ratio of Firmicutes/Bacteroidetes (F/B), an important indicator for the homeostasis of gut microbiota was lower in the AGBR group than in the PR group (Figure 2B).
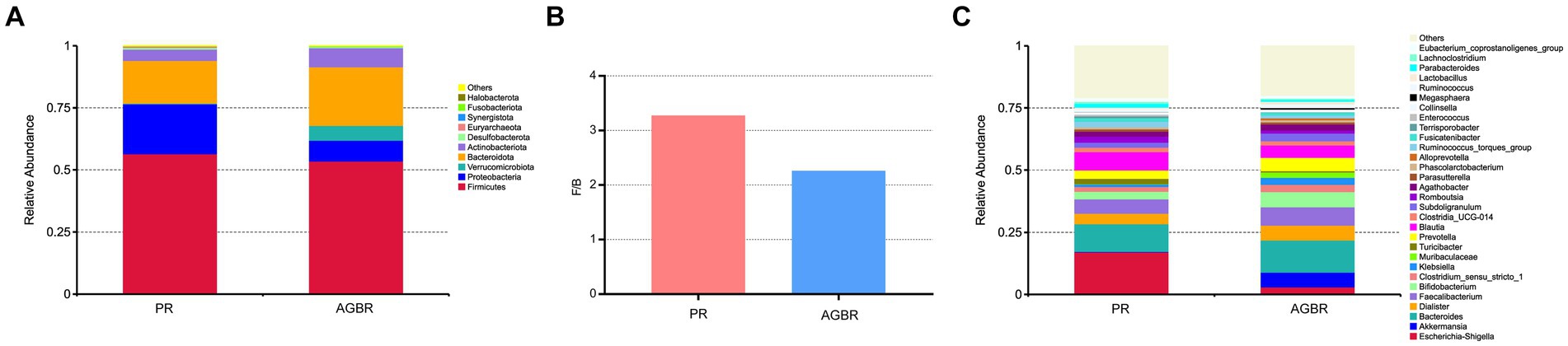
Figure 2. The differences of gut microbiota in patients with hyperlipidemia receiving AGBR or PR diets. (A) Relative abundances of top 10 microbial phyla. (B) F/B ratio. (C) Relative abundances of top 30 microbial genera.
At the genus level, Escherichia-Shigella was the most abundant in patients with hyperlipidemia; however, its abundance was reduced by AGBR intervention (17.05% vs. 2.98%). Bacteroides, another abundant microbial genus, was higher in the AGBR group than in the PR group (11.12% vs. 12.92%). In addition, AGBR elevated the abundances of Faecalibacterium (5.80% vs. 7.22%), Dialister (4.18% vs. 6.05%), Prevotella (3.33% vs. 5.34%), and Bifidobacterium (3.05% vs. 6.15%) in patients with hyperlipidemia. In contrast, AGBR reduced the abundances of Blautia (7.38% vs. 5.00%), Romboutsia (2.46% vs. 1.18%), and Turicibacter (2.17% vs. 0.51%) in vivo (Figure 2C). The top 100 microbial genera in the top 10 microbial phyla are shown in Figure 3.
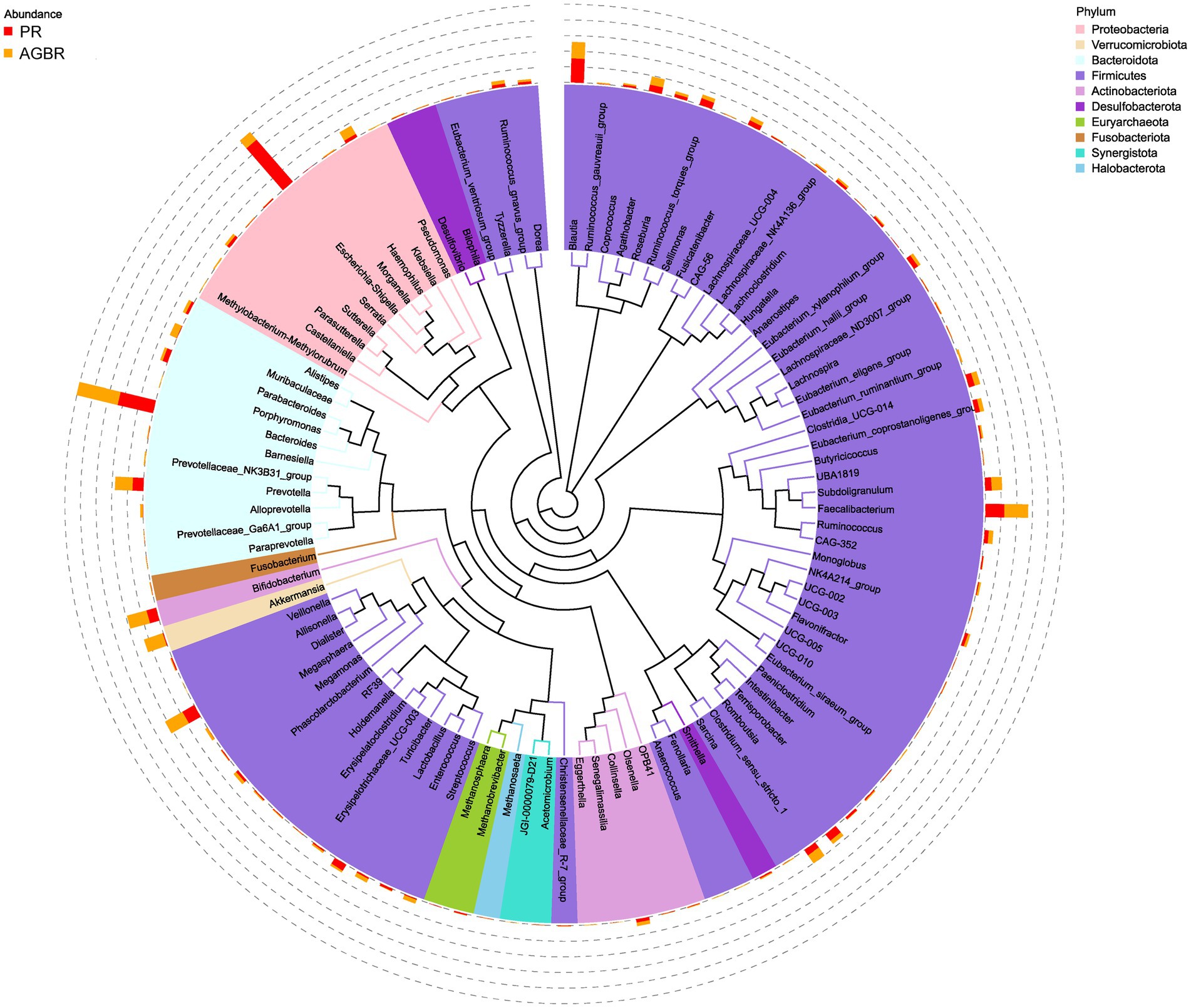
Figure 3. A tree representing the top 100 microbial genera in the top 10 microbial phyla in patients with hyperlipidemia receiving AGBR or PR diets.
4 Discussion
Hyperlipidemia is a prevalent metabolic disorder accompanied by the elevation of lipids in the blood (1). Hyperlipidemia seriously threatens human health because of the high risk of cardiovascular diseases (3). As diet is an important factor influencing hyperlipidemia, various functional foods have been developed to manage hyperlipidemia (22). GBR is a functional food rich in healthy components that are beneficial for the remission of human disorders, including hyperlipidemia (9). GBR inhibited high-fat diet-induced hyperlipidemia by improving lipid synthesis and metabolism in mice (10). In rat and rabbit models of hypercholesterolemia, GBR decreased TC and LDL-c levels and increased HDL-c levels (11, 12). However, research on the human body has been limited. In this study, the therapeutic potential of AGBR, a processed GBR, on hyperlipidemia was explored. Notably, dietary intervention with AGBR reduced TG, TC, LDL-c, and apolipoprotein-B levels and elevated HDL-c and apolipoprotein-A1 levels in patients with hyperlipidemia. These results are similar to those of previous studies on GBR in animal models, and illustrate that AGBR contributes to the remission of hyperlipidemia by affecting blood lipids.
Germination is an effective method for improving the sensory and nutritional qualities of brown rice. GBR is enriched in various nutritional components, including minerals, vitamins, dietary fibers, ferulic acid, γ-oryzanol, and γ-aminobutyric acid, which contribute to the health-promoting functions of GBR (9). Our previous study revealed that autoclaving further improves the taste of GBR and elevates the levels of GABA and ferulic acid (20). In this study, the nutritional characteristics of AGBR were analyzed. Free amino acids (10/16 species), resistant starch, soluble dietary fiber, and GABA were significantly higher in AGBR than in PR. The nutritional components in AGBR are similar to those in GBR and may be beneficial for the remission of hyperlipidemia through specific mechanisms. For example, resistant starch upregulates Acox1 (a lipid oxidation gene) and downregulates SREBP-1 (a fatty acid and triglyceride synthesis and metabolism-related gene) and Fads1 (a fatty acid synthesis gene) (23). Dietary fiber affects HMG-CoA reductase, LDL receptors, CYP7A1, MAPK signaling pathways, and other lipid metabolism-related target genes (24). GABA is involved in cholesterol metabolism in macrophages by blocking the p38MAPK and NF-κB signaling pathways (25). Notably, this study also found that AGBR was enriched in a considerable proportion of flavonoids (11/13 species). Flavonoids are a group of phytoestrogens with physiological activities in humans (26). Flavonoids have great potential to prevent and treat diverse metabolic disorders in humans, such as obesity, diabetes, hyperlipidemia, and osteoporosis (27). Some flavonoids that were detected to be elevated by AGBR in this study have also been demonstrated to be associated with the remission of hyperlipidemia, such as epicatechin (28), rutin (29), luteolin (30), quercetin (31), apigenin (32), and isorhamnetin (33). Therefore, flavonoids may be effective components of AGBR in the treatment of hyperlipidemia. However, the mechanisms underlying the effects of various flavonoids on hyperlipidemia are different and diverse and require systematic research and analysis.
The gut microbiota is a large and diverse group of microorganisms that play a crucial role in host metabolism and immune responses (34, 35). Evidence has shown that the gut microbiota is involved in the mechanisms of action of diverse crops, such as oats (4), tartary buckwheat (5), black rice (6), and red yeast rice (16), in alleviating hyperlipidemia. In this study, the therapeutic mechanisms of AGBR on the gut microbiota were explored. Compared with the PR group, patients with hyperlipidemia in the AGBR group exhibited relatively higher microbial abundance, diversity, and uniformity. These results indicate that AGBR may relieve hyperlipidemia by improving the gut microbiota.
At the phylum level, Firmicutes and Bacteroidetes are the main components of the human gut microbiota (36). The F/B ratio is regarded as an indicator of gut microbiota homeostasis and its elevation is associated with various metabolic syndromes, including obesity (37), hypertension (38), diabetes (39), and hyperlipidemia (40). Previous studies have revealed that many active substances with therapeutic potential against hyperlipidemia can reduce the F/B ratio, such as glycosaminoglycan (Ostrea rivularis) (41), polysaccharides (Tremella fuciformis) (42), edible kynurenic acid (Tachypleus tridentatus) (43), genistein (44), tomato seed oil (45), and highland barley whole grain (18). In this study, AGBR intervention decreased Firmicutes and elevated Bacteroidota in patients with hyperlipidemia, along with a decrease in the F/B ratio. These results are similar to previous findings and illustrate that the hypolipidemic effects of AGBR are associated with an increase in the F/B ratio. Additionally, AGBR intervention decreased Proteobacteria, Desulfobacterota, and Synergistota, and elevated Verrucomicrobiota in patients with hyperlipidemia. These microbial phyla may be involved in lipid homeostasis. As reported, Proteobacteria are more enriched in women with high total cholesterol levels than in those with normal serum lipid levels (46). Konjac glucomannan-dihydromyricetin reduces serum TG, total glycerol, and Desulfobacterota in mice fed a high-fat diet (47). Synergistetes are positively associated with cholesterol (48). Lactobacillus sakei MJM60958 inhibits lipid accumulation and increases Verrucomicrobia in mice with fatty liver (49). These microbial phyla may participate in the mechanism of action of AGBR in the treatment of hyperlipidemia.
The specific differences in the microbial composition between the PR and AGBR groups were further analyzed at the genus level. The results showed that Escherichia-Shigella and Bacteroides were the dominant genera, among which the former was reduced and the latter was elevated by AGBR intervention. Escherichia-Shigella is known as a harmful bacteria that accumulates in metabolic diseases (50). Li et al. reported that Spirulina platensis polysaccharides reduces Escherichia-Shigella and improves lipid and carbohydrate metabolism in rats fed a high-fat diet (51). Bacteroides, belonging to Bacteroidetes, is a beneficial bacteria associated with metabolic diseases (52). Previous studies have revealed that an increase in Bacteroides is associated with remission of hyperlipidemia in rats (53, 54). Changes in these two genera may have contributed to the hypolipidemic effects of AGBR in vivo. In addition, this study also found that AGBR elevated Faecalibacterium, Dialister, Prevotella, and Bifidobacterium; however, it reduced Blautia, Romboutsia, and Turicibacter in patients with hyperlipidemia. These microbial genera may also participate in the therapeutic mechanisms of GBR on hyperlipidemia through regulating lipid homeostasis (4, 52, 55–60).
However, this study has some limitations. For example, the sample size was relatively small and the intervention time was relatively short. The relationship between the nutritional components of AGBR, especially flavonoids, and blood lipids, has not been thoroughly studied. Additionally, the specific effects and mechanisms of action of different bacteria on blood lipid levels remain unclear. Further studies on these fields are warranted in the future.
5 Conclusion
In conclusion, dietary intervention with AGBR reduced blood lipid levels in patients with hyperlipidemia. AGBR is rich in free amino acids, resistant starch, soluble dietary fibers, GABA, and flavonoids. Additionally, AGBR improved the gut microbiota in patients with hyperlipidemia, evidenced by reduced Firmicutes, Proteobacteria, Desulfobacterota, and Synergistota, and elevated Bacteroidota and Verrucomicrobiota at the phylum level, as well as reduced Escherichia-Shigella, Blautia, Romboutsia, and Turicibacter, and elevated Bacteroides, Faecalibacterium, Dialister, Prevotella, and Bifidobacterium at the genus level. AGBR may be an effective functional food to relieve hyperlipidemia by modulating the gut microbiota.
Data availability statement
The data presented in this study are deposited in the SRA repository, accession number: PRJNA1107690.
Ethics statement
The studies involving humans were approved by Lianshui People’s Hospital affiliated to Kangda College, Nanjing Medical University. The studies were conducted in accordance with the local legislation and institutional requirements. The participants provided their written informed consent to participate in this study.
Author contributions
CR: Conceptualization, Data curation, Formal analysis, Funding acquisition, Visualization, Writing – original draft. BH: Data curation, Formal analysis, Writing – original draft. SZ: Data curation, Formal analysis, Writing – original draft. DY: Data curation, Investigation, Writing – original draft. JF: Data curation, Investigation, Writing – original draft. SS: Data curation, Investigation, Writing – original draft. JZ: Data curation, Investigation, Writing – original draft. LG: Data curation, Investigation, Writing – original draft. LZ: Data curation, Investigation, Writing – original draft. SL: Conceptualization, Funding acquisition, Supervision, Writing – review & editing.
Funding
The author(s) declare that financial support was received for the research, authorship, and/or publication of this article. This study was funded by the National Key Research and Development Program Project (2021YFD2100902), Heilongjiang Province Research Business Project (CZKYF2023-1-C015), National Rice Industry Technology System (CARS-01-51), and Outstanding Youth Project of Heilongjiang Province Innovation and Leap Forward Project (CX22JQ05).
Conflict of interest
The authors declare that the research was conducted in the absence of any commercial or financial relationships that could be construed as a potential conflict of interest.
The reviewer WL declared a past co-authorship with the author(s) CR, LG, BH, and SL to the handling editor.
Publisher’s note
All claims expressed in this article are solely those of the authors and do not necessarily represent those of their affiliated organizations, or those of the publisher, the editors and the reviewers. Any product that may be evaluated in this article, or claim that may be made by its manufacturer, is not guaranteed or endorsed by the publisher.
References
1. He, N, and Ye, H. Exercise and hyperlipidemia. Adv Exp Med Biol. (2020) 1228:79–90. doi: 10.1007/978-981-15-1792-1_5
3. Alloubani, A, Nimer, R, and Samara, R. Relationship between hyperlipidemia, cardiovascular disease and stroke: a systematic review. Curr Cardiol Rev. (2021) 17:e051121189015. doi: 10.2174/1573403X16999201210200342
4. Xu, D, Feng, M, Chu, Y, Wang, S, Shete, V, Tuohy, KM, et al. The prebiotic effects of oats on blood lipids, gut microbiota, and short-chain fatty acids in mildly Hypercholesterolemic subjects compared with Rice: a randomized, controlled trial. Front Immunol. (2021) 12:787797. doi: 10.3389/fimmu.2021.787797
5. Wang, Y, Qi, W, Guo, X, Song, G, Pang, S, Fang, W, et al. Effects of oats, Tartary buckwheat, and foxtail millet supplementation on lipid metabolism, Oxido-inflammatory responses, gut microbiota, and colonic SCFA composition in high-fat diet fed rats. Nutrients. (2022) 14:2760. doi: 10.3390/nu14132760
6. Li, X, Du, Y, Zhang, C, and Wang, L. Black rice regulates lipid metabolism, liver injury, oxidative stress and adipose accumulation in high-fat/cholesterol diet mice based on gut microbiota and untargeted metabonomics. J Nutr Biochem. (2023) 117:109320. doi: 10.1016/j.jnutbio.2023.109320
7. Hu, M, Chen, G, Peng, L, Li, C, He, X, Zhang, Q, et al. Response of yield and quality of giant embryo rice to nitrogen application and analysis of lipid-lowering effect. Front Plant Sci. (2022) 13:1023677. doi: 10.3389/fpls.2022.1023677
8. Cho, DH, and Lim, ST. Germinated brown rice and its bio-functional compounds. Food Chem. (2016) 196:259–71. doi: 10.1016/j.foodchem.2015.09.025
9. Wu, F, Yang, N, Toure, A, Jin, Z, and Xu, X. Germinated brown rice and its role in human health. Crit Rev Food Sci Nutr. (2013) 53:451–63. doi: 10.1080/10408398.2010.542259
10. Shen, KP, Hao, CL, Yen, HW, Chen, CY, Chen, JH, Chen, FC, et al. Pre-germinated brown rice prevented high fat diet induced hyperlipidemia through ameliorating lipid synthesis and metabolism in C57BL/6J mice. J Clin Biochem Nutr. (2016) 59:39–44. doi: 10.3164/jcbn.15-117
11. Roohinejad, S, Omidizadeh, A, Mirhosseini, H, Saari, N, Mustafa, S, Yusof, RM, et al. Effect of pre-germination time of brown rice on serum cholesterol levels of hypercholesterolaemic rats. J Sci Food Agric. (2010) 90:245–51. doi: 10.1002/jsfa.3803
12. Mohd Esa, N, Abdul Kadir, KK, Amom, Z, and Azlan, A. Improving the lipid profile in hypercholesterolemia-induced rabbit by supplementation of germinated brown rice. J Agric Food Chem. (2011) 59:7985–91. doi: 10.1021/jf201323x
13. Chen, D, Wu, J, Jin, D, Wang, B, and Cao, H. Fecal microbiota transplantation in cancer management: current status and perspectives. Int J Cancer. (2019) 145:2021–31. doi: 10.1002/ijc.32003
14. Chen, Y, Zhou, J, and Wang, L. Role and mechanism of gut microbiota in human disease. Front Cell Infect Microbiol. (2021) 11:625913. doi: 10.3389/fcimb.2021.625913
15. Schoeler, M, and Caesar, R. Dietary lipids, gut microbiota and lipid metabolism. Rev Endocr Metab Disord. (2019) 20:461–72. doi: 10.1007/s11154-019-09512-0
16. Yang, H, Pan, R, Wang, J, Zheng, L, Li, Z, Guo, Q, et al. Modulation of the gut microbiota and liver transcriptome by red yeast Rice and Monascus pigment fermented by purple Monascus SHM1105 in rats fed with a high-fat diet. Front Pharmacol. (2020) 11:599760. doi: 10.3389/fphar.2020.599760
17. Yan, J, Xue, Q, Chen, W, Wang, K, Peng, D, Jiang, J, et al. Probiotic-fermented rice buckwheat alleviates high-fat diet-induced hyperlipidemia in mice by suppressing lipid accumulation and modulating gut microbiota. Food Res Int. (2022) 155:111125. doi: 10.1016/j.foodres.2022.111125
18. Deng, N, He, Z, Guo, R, Zheng, B, Li, T, and Liu, RH. Highland barley whole grain (Hordeum vulgare L.) ameliorates hyperlipidemia by modulating Cecal microbiota, miRNAs, and AMPK pathways in leptin receptor-deficient db/db mice. J Agric Food Chem. (2020) 68:11735–46. doi: 10.1021/acs.jafc.0c04780
19. Zhao, R, Huang, F, Liu, C, Asija, V, Cao, L, Zhou, M, et al. Impact of germinated Brown Rice and Brown Rice on metabolism, inflammation, and gut microbiome in high fat diet-induced insulin resistant mice. J Agric Food Chem. (2022) 70:14235–46. doi: 10.1021/acs.jafc.2c06662
20. Ren, C, Hong, B, Zheng, X, Wang, L, Zhang, Y, Guan, L, et al. Improvement of germinated brown rice quality with autoclaving treatment. Food Sci Nutr. (2020) 8:1709–17. doi: 10.1002/fsn3.1459
21. C-y, REN, S-w, LU, L-j, GUAN, Bin, H, Y-l, ZHANG, W-g, HUANG, et al. The metabolomics variations among rice, brown rice, wet germinated brown rice, and processed wet germinated brown rice. J Integr Agric. (2022) 21:2767–76. doi: 10.1016/j.jia.2022.07.025
22. Chen, G, Wang, H, Zhang, X, and Yang, ST. Nutraceuticals and functional foods in the management of hyperlipidemia. Crit Rev Food Sci Nutr. (2014) 54:1180–201. doi: 10.1080/10408398.2011.629354
23. Zhou, Z, Wang, F, Ren, X, Wang, Y, and Blanchard, C. Resistant starch manipulated hyperglycemia/hyperlipidemia and related genes expression in diabetic rats. Int J Biol Macromol. (2015) 75:316–21. doi: 10.1016/j.ijbiomac.2015.01.052
24. Nie, Y, and Luo, F. Dietary Fiber: an opportunity for a global control of hyperlipidemia. Oxidative Med Cell Longev. (2021) 2021:1–20. doi: 10.1155/2021/5542342
25. Yang, Y, Lian, YT, Huang, SY, Cheng, LX, and Liu, K. GABA and topiramate inhibit the formation of human macrophage-derived foam cells by modulating cholesterol-metabolism-associated molecules. Cell Physiol Biochem. (2014) 33:1117–29. doi: 10.1159/000358681
26. Kiyama, R. Estrogenic flavonoids and their molecular mechanisms of action. J Nutr Biochem. (2023) 114:109250. doi: 10.1016/j.jnutbio.2022.109250
27. Chen, L, Cao, H, Huang, Q, Xiao, J, and Teng, H. Absorption, metabolism and bioavailability of flavonoids: a review. Crit Rev Food Sci Nutr. (2022) 62:7730–42. doi: 10.1080/10408398.2021.1917508
28. Cheng, H, Xu, N, Zhao, W, Su, J, Liang, M, Xie, Z, et al. (-)-Epicatechin regulates blood lipids and attenuates hepatic steatosis in rats fed high-fat diet. Mol Nutr Food Res. (2017) 61:1700303. doi: 10.1002/mnfr.201700303
29. Manzoni, AG, Passos, DF, da Silva, JLG, Bernardes, VM, Bremm, JM, Jantsch, MH, et al. Rutin and curcumin reduce inflammation, triglyceride levels and ADA activity in serum and immune cells in a model of hyperlipidemia. Blood Cells Mol Dis. (2019) 76:13–21. doi: 10.1016/j.bcmd.2018.12.005
30. Sun, J, Wang, Z, Chen, L, and Sun, G. Hypolipidemic effects and preliminary mechanism of chrysanthemum flavonoids, its main components luteolin and luteoloside in hyperlipidemia rats. Antioxidants (Basel). (2021) 10:1309. doi: 10.3390/antiox10081309
31. Juzwiak, S, Wojcicki, J, Mokrzycki, K, Marchlewicz, M, Bialecka, M, Wenda-Rozewicka, L, et al. Effect of quercetin on experimental hyperlipidemia and atherosclerosis in rabbits. Pharmacol Rep. (2005) 57:604–9.
32. Li, S, Wang, Z, Zhou, Z, Gao, Z, Liu, Y, Li, J, et al. Molecular mechanism of the role of Apigenin in the treatment of hyperlipidemia: a network pharmacology approach. Chem Biodivers. (2023) 20:e202200308. doi: 10.1002/cbdv.202200308
33. Ressaissi, A, Attia, N, Fale, PL, Pacheco, R, Victor, BL, Machuqueiro, M, et al. Isorhamnetin derivatives and piscidic acid for hypercholesterolemia: cholesterol permeability, HMG-CoA reductase inhibition, and docking studies. Arch Pharm Res. (2017) 40:1278–86. doi: 10.1007/s12272-017-0959-1
34. Gomaa, EZ. Human gut microbiota/microbiome in health and diseases: a review. Antonie Van Leeuwenhoek. (2020) 113:2019–40. doi: 10.1007/s10482-020-01474-7
35. Zhao, J, Zhang, X, Liu, H, Brown, MA, and Qiao, S. Dietary protein and gut microbiota composition and function. Curr Protein Pept Sci. (2019) 20:145–54. doi: 10.2174/1389203719666180514145437
36. Jandhyala, SM, Talukdar, R, Subramanyam, C, Vuyyuru, H, Sasikala, M, and Nageshwar, RD. Role of the normal gut microbiota. World J Gastroenterol. (2015) 21:8787–803. doi: 10.3748/wjg.v21.i29.8787
37. Magne, F, Gotteland, M, Gauthier, L, Zazueta, A, Pesoa, S, Navarrete, P, et al. The firmicutes/bacteroidetes ratio: a relevant marker of gut Dysbiosis in obese patients? Nutrients. (2020) 12:1474. doi: 10.3390/nu12051474
38. Yang, T, Santisteban, MM, Rodriguez, V, Li, E, Ahmari, N, Carvajal, JM, et al. Gut dysbiosis is linked to hypertension. Hypertension. (2015) 65:1331–40. doi: 10.1161/HYPERTENSIONAHA.115.05315
39. Xia, F, Wen, LP, Ge, BC, Li, YX, Li, FP, and Zhou, BJ. Gut microbiota as a target for prevention and treatment of type 2 diabetes: mechanisms and dietary natural products. World J Diabetes. (2021) 12:1146–63. doi: 10.4239/wjd.v12.i8.1146
40. Kappel, BA, De Angelis, L, Puetz, A, Ballanti, M, Menghini, R, Marx, N, et al. Antibiotic-induced gut microbiota depletion exacerbates host hypercholesterolemia. Pharmacol Res. (2023) 187:106570. doi: 10.1016/j.phrs.2022.106570
41. Kong, Y, Li, Y, Dai, ZR, Qin, M, Fan, HL, Hao, JG, et al. Glycosaminoglycan from Ostrea rivularis attenuates hyperlipidemia and regulates gut microbiota in high-cholesterol diet-fed zebrafish. Food Sci Nutr. (2021) 9:5198–210. doi: 10.1002/fsn3.2492
42. He, G, Chen, T, Huang, L, Zhang, Y, Feng, Y, Qu, S, et al. Tremella fuciformis polysaccharide reduces obesity in high-fat diet-fed mice by modulation of gut microbiota. Front Microbiol. (2022) 13:1073350. doi: 10.3389/fmicb.2022.1073350
43. Li, J, Zhang, Y, Yang, S, Lu, Z, Li, G, Wu, S, et al. The beneficial effects of edible Kynurenic acid from marine horseshoe crab (Tachypleus tridentatus) on obesity, hyperlipidemia, and gut microbiota in high-fat diet-fed mice. Oxidative Med Cell Longev. (2021) 2021:1–13. doi: 10.1155/2021/8874503
44. Yang, R, Jia, Q, Mehmood, S, Ma, S, and Liu, X. Genistein ameliorates inflammation and insulin resistance through mediation of gut microbiota composition in type 2 diabetic mice. Eur J Nutr. (2021) 60:2155–68. doi: 10.1007/s00394-020-02403-0
45. He, WS, Li, L, Rui, J, Li, J, Sun, Y, Cui, D, et al. Tomato seed oil attenuates hyperlipidemia and modulates gut microbiota in C57BL/6J mice. Food Funct. (2020) 11:4275–90. doi: 10.1039/D0FO00133C
46. Guo, L, Wang, YY, Wang, JH, Zhao, HP, Yu, Y, Wang, GD, et al. Associations of gut microbiota with dyslipidemia based on sex differences in subjects from northwestern China. World J Gastroenterol. (2022) 28:3455–75. doi: 10.3748/wjg.v28.i27.3455
47. Ruan, S, Gao, X, Li, B, and Tian, J. The synergic effects and mechanism of KGM-DMY complex in the prevention of obesity and enhancement of fatigue resistance in mice. Food Funct. (2023) 14:2607–20. doi: 10.1039/D2FO03677K
48. Nuli, R, Cai, J, Kadeer, A, Zhang, Y, and Mohemaiti, P. Integrative analysis toward different glucose tolerance-related gut microbiota and diet. Front Endocrinol (Lausanne). (2019) 10:295. doi: 10.3389/fendo.2019.00295
49. Nguyen, HT, Gu, M, Werlinger, P, Cho, JH, Cheng, J, and Suh, JW. Lactobacillus sakei MJM60958 as a potential probiotic alleviated non-alcoholic fatty liver disease in mice fed a high-fat diet by modulating lipid metabolism, inflammation, and gut microbiota. Int J Mol Sci. (2022) 23:13436. doi: 10.3390/ijms232113436
50. Wang, L, Gou, X, Ding, Y, Liu, J, Wang, Y, Zhang, J, et al. The interplay between herbal medicines and gut microbiota in metabolic diseases. Front Pharmacol. (2023) 14:1105405. doi: 10.3389/fphar.2023.1105405
51. Li, TT, Huang, ZR, Jia, RB, Lv, XC, Zhao, C, and Liu, B. Spirulina platensis polysaccharides attenuate lipid and carbohydrate metabolism disorder in high-sucrose and high-fat diet-fed rats in association with intestinal microbiota. Food Res Int. (2021) 147:110530. doi: 10.1016/j.foodres.2021.110530
52. Michels, N, Zouiouich, S, Vanderbauwhede, B, Vanacker, J, Indave Ruiz, BI, and Huybrechts, I. Human microbiome and metabolic health: an overview of systematic reviews. Obes Rev. (2022) 23:e13409. doi: 10.1111/obr.13409
53. Wen, JJ, Li, MZ, Chen, CH, Hong, T, Yang, JR, Huang, XJ, et al. Tea polyphenol and epigallocatechin gallate ameliorate hyperlipidemia via regulating liver metabolism and remodeling gut microbiota. Food Chem. (2023) 404:134591. doi: 10.1016/j.foodchem.2022.134591
54. Xu, M, Lan, R, Qiao, L, Lin, X, Hu, D, Zhang, S, et al. Bacteroides vulgatus ameliorates lipid metabolic disorders and modulates gut microbial composition in Hyperlipidemic rats. Microbiol Spectr. (2023) 11:e0251722. doi: 10.1128/spectrum.02517-22
55. Li, TT, Tong, AJ, Liu, YY, Huang, ZR, Wan, XZ, Pan, YY, et al. Polyunsaturated fatty acids from microalgae Spirulina platensis modulates lipid metabolism disorders and gut microbiota in high-fat diet rats. Food Chem Toxicol. (2019) 131:110558. doi: 10.1016/j.fct.2019.06.005
56. Ren, Y, Wu, S, Xia, Y, Huang, J, Ye, J, Xuan, Z, et al. Probiotic-fermented black tartary buckwheat alleviates hyperlipidemia and gut microbiota dysbiosis in rats fed with a high-fat diet. Food Funct. (2021) 12:6045–57. doi: 10.1039/D1FO00892G
57. Duan, R, Guan, X, Huang, K, Zhang, Y, Li, S, Xia, J, et al. Flavonoids from whole-grain oat alleviated high-fat diet-induced hyperlipidemia via regulating bile acid metabolism and gut microbiota in mice. J Agric Food Chem. (2021) 69:7629–40. doi: 10.1021/acs.jafc.1c01813
58. Wang, Y, Han, C, Cheng, J, Wang, Z, Liu, L, Huang, H, et al. Fermented Cerasus humilis fruits protect against high-fat diet induced hyperlipidemia which is associated with alteration of gut microbiota. J Sci Food Agric. (2023) 103:2554–63. doi: 10.1002/jsfa.12377
59. Tong, X, Xu, J, Lian, F, Yu, X, Zhao, Y, Xu, L, et al. Structural alteration of gut microbiota during the amelioration of human type 2 diabetes with hyperlipidemia by metformin and a traditional Chinese herbal formula: a multicenter, randomized, open label clinical trial. MBio. (2018) 9:e02392-17. doi: 10.1128/mBio.02392-17
Keywords: germinated brown rice, hyperlipidemia, gut microbiota, nutrition, functional food
Citation: Ren C, Hong B, Zhang S, Yuan D, Feng J, Shan S, Zhang J, Guan L, Zhu L and Lu S (2024) Autoclaving-treated germinated brown rice relieves hyperlipidemia by modulating gut microbiota in humans. Front. Nutr. 11:1403200. doi: 10.3389/fnut.2024.1403200
Edited by:
Aimin Shi, Chinese Academy of Agricultural Sciences, ChinaReviewed by:
Yucun Niu, Harbin Medical University, ChinaWeihong Lu, Harbin Institute of Technology, China
Copyright © 2024 Ren, Hong, Zhang, Yuan, Feng, Shan, Zhang, Guan, Zhu and Lu. This is an open-access article distributed under the terms of the Creative Commons Attribution License (CC BY). The use, distribution or reproduction in other forums is permitted, provided the original author(s) and the copyright owner(s) are credited and that the original publication in this journal is cited, in accordance with accepted academic practice. No use, distribution or reproduction is permitted which does not comply with these terms.
*Correspondence: Shuwen Lu, chuanying1023@163.com
†ORCID: Shuwen Lu, http://orcid.org/0000-0002-9462-8215