- 1Food Processing Institute, Heilongjiang Academy of Agricultural Sciences, Harbin, China
- 2Key Laboratory of Food Processing of Heilongjiang Province, Harbin, China
- 3Soybean Institute, Heilongjiang Academy of Agricultural Sciences, Harbin, China
Acanthopanax senticosus (AS) is a geo-authentic crude medicinal plant that grows in China, Korea, Russia, and Japan. AS contains bioactive compounds such as eleutherosides, polysaccharides, and flavonoids. It is also a key traditional herb in the Red List of Chinese Species. AS is mainly distributed in Northeast China, specifically in Heilongjiang, Jilin, and Liaoning provinces. Its active compounds contribute to significant biological activities, including neuroprotective, antioxidant, anti-fatigue, and antitumor effects. However, the extraction methods of active compounds are complex, the extraction efficiency is poor, and the structure–activity relationship is unclear. This study focused on the nutrients in AS, including protein, carbohydrates, and lipids. Particularly, the active ingredients (eleutherosides, polysaccharides, and flavonoids) in AS and their extraction and purification methods were analyzed and summarized. The biological activities of extracts have been reviewed, and the mechanisms of anti-oxidation, antitumor, anti-inflammation, and other activities are introduced in detail. The applications of AS in various domains, such as health foods, medicines, and animal dietary supplements, are then reported. Compared with other extraction methods, ultrasonic or microwave extraction improves efficiency, yet they can damage structures. Challenges arise in the recovery of solvents and in achieving extraction efficiency when using green solvents, such as deep eutectic solvents. Improvements can be made by combining extraction methods and controlling conditions (power, temperature, and time). Bioactive molecules and related activities are exposited clearly. The applications of AS have not been widely popularized, and the corresponding functions require further development.
1 Introduction
Acanthopanax senticosus (AS), commonly known as “Ci-wu-jia” in China, originates from the dried root and rhizome or stem of AS (Rupr. et Maxim.) and belongs to the Araliaceae family (1). AS is a shrub that grows in forests or shrub areas, typically ranging from 1 to 4 m in height (Figure 1). It is also known as “Siberian ginseng” and is found across China, South Korea, Japan, and Russia (2). In China, it mainly grows in Northeastern provinces, such as Xiaoxing’anling in Heilongjiang, the Changbai Mountains in Jilin, Shenyang in Liaoning, and Hebei and also Shanxi provinces in North China.
The medicinal effect of AS, which has a bitter and astringent taste, is mild. It is considered to nourish the spleen and stomach and stimulate appetite (3). Its active ingredients include glycosides, polysaccharides, flavonoids, lignans, triterpenoids, and organic acids (4). Modern pharmacology has revealed that AS can regulate immunity, treat cardiovascular system diseases, and has anti-inflammatory, antitumor, and anti-oxidation effects (5). Glycosides repel insects, have antibacterial properties, and can improve sleep (6). Polysaccharides and their derivatives considerably affect the treatment of malignant tumors and improve human immunity due to their unique biological activities (7). Moreover, flavonoids have been reported to slow down human aging (3). Previous research involving a systematic study on Araliaceae plants has shown that AS and ginseng have similar pharmacological effects and clinical efficacy (8).
In terms of food supplements, the tender stems and fresh leaves of AS contain carotene, riboflavin, ascorbic acid, and rich vitamins. Its stem flavor is unique, aromatic, and slightly bitter. AS can be used as an ingredient in cooking and soups, and it can also be used to make packaging cans. Its leaves can be processed to produce a light-brown tea with a distinctive aroma and sweet taste.
In this study, the nutritional components, active components, extraction and purification methods, biological activities, and applications of AS are presented and discussed to expand its application range. Figure 2 presents a schematic diagram of the study structure.
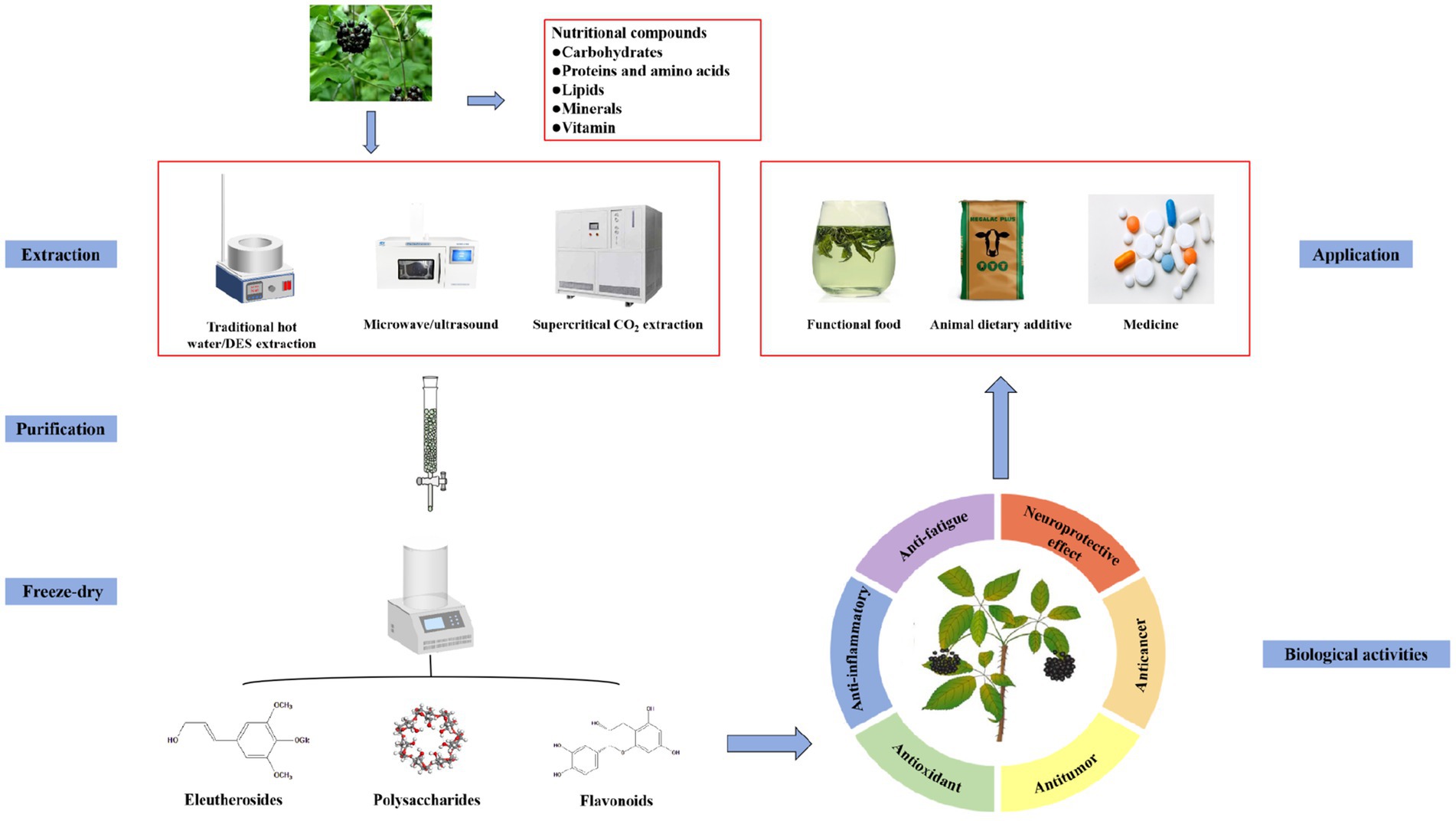
Figure 2. Schematic diagram of extraction, purification, biological activities, and application of Acanthopanax senticosus.
2 Nutritional compounds
AS contains numerous nutrients, such as polysaccharides, essential amino acids, lipids, and vitamins, which are necessary for humans in regulating metabolism (Table 1). Table 2 summarizes the benefits of AS for humans in different countries. The results showed that there was a dose-dependent relationship between carbohydrate accumulation and the nitrogen application rate in AS (19). Furthermore, this content is related to environmental factors such as soil, altitude, moisture, sunshine, and topography (20). Processes such as decoction and fermentation increase the content of soluble solids and polysaccharides (21).
2.1 Carbohydrates
The carbohydrates in AS mainly consist of monosaccharides, disaccharides, polysaccharides, reducing sugar, and crude fiber. Carbohydrates accounted for 72.33% of the total composition in AS (11). The major monosaccharides in AS leaves are glucose, xylose, and rhamnose. Reduced sugar and polysaccharides in AS leaves are more accumulated during the growth and deciduous periods compared to other periods (13). The content of reduced sugar and polysaccharides is approximately 6.24–20.80% (9) and 2.21–5.74% (15), respectively, of the total composition in AS.
2.2 Proteins and amino acids
The protein content in AS ranges from 2.08 to 2.74% (9). The category, content, and ratio of essential amino acids are important indicators for evaluating plant nutrition (10). Su et al. found that the content of amino acids was the highest in seeds. Moreover, amino acids in AS leaves accumulate more during the flourishing and declining stages of growth than in other periods (13). AS consists of amino acid varieties, including alanine, glutamic acid, and aspartic acid. Researchers have identified approximately 13 different amino acids (6 essential amino acids) in AS, with 4 major acids such as glutamic (11.40–15.54% of the total amino acids), aspartic acid (10.08–11.16%), and lysine acids (7.46–12.22%) (10).
2.3 Lipids
Lipids are the main components of plant cell organ membranes and provide energy for metabolism. The fatty acids of leaves accumulate in the growth period to obtain energy (13). Crude fat compromises 2.07% ± 0.09% of fatty acids in AS, including 12.98% saturated fatty acids, 33.13% unsaturated fatty acids, 27.46% unsaturated alcohols, and 15.76% diolefins (9). Zhao et al. isolated and characterized eight fatty acids from the AS root bark extract, including oleic acid methyl acetate, oleic acid ethyl acetate, 10,13-octadecadienoic acid methyl acetate, 10,13-octadecadienoic acid ethyl acetate, myristic acid, palmitoleic acid, 9, 11-octadecadienoic acid, and hexadecatrienoic acid (18).
2.4 Minerals
The mineral content in AS varies with the species, origin, and climate. Previous research has identified the major and trace elements of AS (10), including minerals such as potassium, calcium, sodium, magnesium, iron, copper, zinc, manganese, and selenium.
2.5 Vitamins
AS is rich in vitamins such as VB1, VB2, VB3, VB6, VB12, and VC (9), providing the ability to stimulate cells and enhance immunity.
3 Active compounds
3.1 Polysaccharides
Polysaccharides are polymers linked by multiple glycosidic bonds. The structural analysis of polysaccharides focuses on their primary structure, such as monosaccharide composition, relative molecular mass, and chain conformation analysis. Understanding the structural features of polysaccharides is essential for assessing their functions in biological systems and their macroscopic properties in industrial applications.
The hydrolysis of polysaccharides is typically performed using trifluoroacetic acid (TFA) or specific enzymes to treat the polysaccharide chain and obtain the main chain and fragment structure. These are then combined with techniques such as monosaccharide composition, methylation, and nuclear magnetic resonance for the structure resolution of degraded products (36). Chen et al. extracted crude polysaccharides with antioxidant and immunological activities and subsequently hydrolyzed them with TFA to obtain water-soluble polysaccharides with a relative molecular mass of 14.57 kDa (12). As shown in Table 3, the molecular weights of the polysaccharides of AS range between 5.24 and 169 kDa. The diversities in the molecular weights may be due to the differences in the sources of raw materials, extraction temperatures, and deproteinization methods. The structure and type of polysaccharides in the stem of AS are more complex than those in the leaves, and the fibrous structure of the stem protects the plant cells from damage for better bud differentiation. The high temperature during extraction and the use of dialysis bags during deproteinization will destroy the structure of polysaccharides.
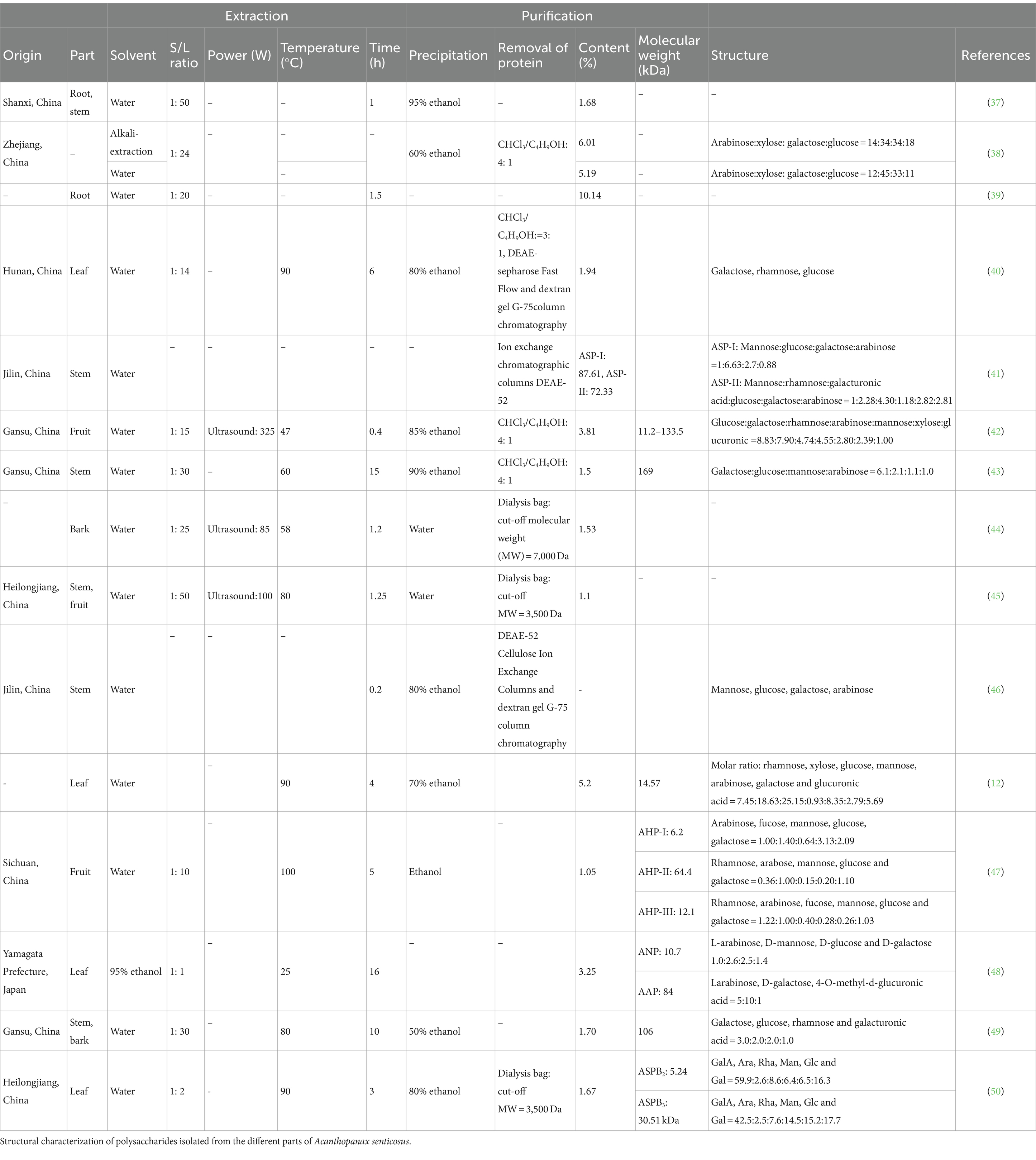
Table 3. Summary of the extraction and purification process of polysaccharides from Acanthopanax senticosus.
3.2 Eleutherosides
Numerous studies have identified eleutherosides as the main components of AS with pharmacological effects. AS contains 16 types of eleutherosides, compromising 18.59% of the total content, in its different components (roots, stems, leaves, etc.). The type and content vary with the plant component. In addition, the eleutheroside content can also vary within the same plant component. Scholars have determined the content of eleutherosides in the roots, stems, and leaves as 1.05, 2.49, and 0.75%, respectively (51).
Eleutherosides are sugar or sugar derivatives that include amino sugar and uronic acid, along with other non-sugar substances, linked through the terminal carbon atoms of the sugar molecules. The non-sugar component is aglycone or ligand, and the linked bond is aglycone. The roots and rhizomes contain a variety of eleutherosides, which can be classified as eleutheroside E, daucosterol, eleutheroside B, eleutheroside D, eleutheroside K, songoroside, copteroside B, and pinoresinol-4,4′-di-β-O-D-glucoside (Figures 3A–H) (52).
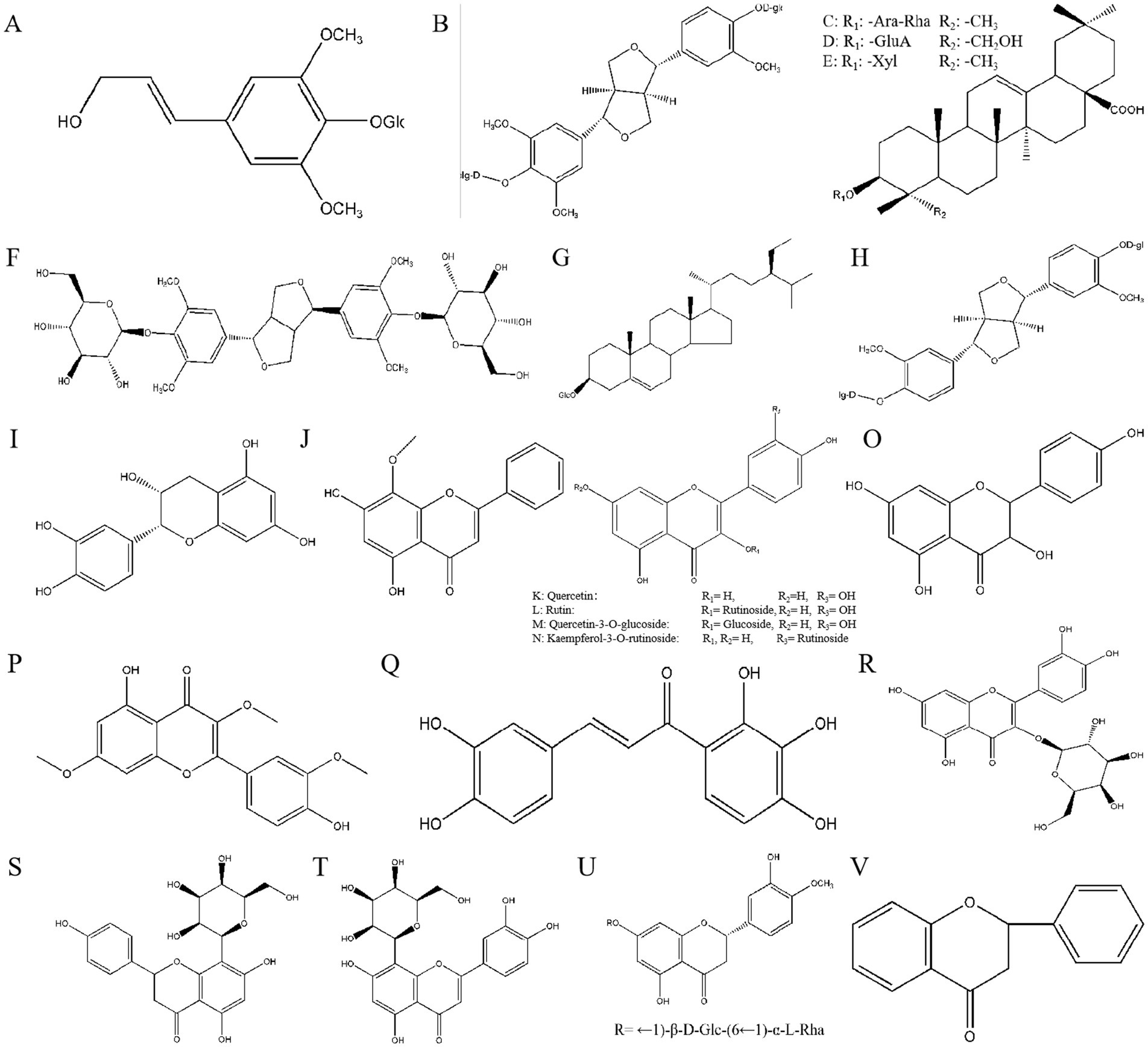
Figure 3. The chemical structures of eleutherosides (A–H). (A): Eleutheroside B, (B): Eleutheroside D, (C): Eleutheroside K, (D): Copteroside B, (E): Songoroside A, (F): Eleutheroside E, (G): Daucosterol, (H): Pinoresinol-4,4′-di-β-O-D-glucoside. The chemical structures of flavonoids and 2-phenylchromogenon (I–V). (I): Epicatechin, (J): Wogonin, (K): Quercetin, (L): Rutin, (M): Quercetin-3-O-glucoside, (N): Kaempferol-3-O-rutinoside, (O): Kaempferol, (P): Pachypodol, (Q): Okanin, (R): Hyperoside, (S): Vitexin, (T): Polygonin, (U): Hesperidin, (V): 2-phenylchromogenone.
3.3 Flavonoids
Flavonoids are present in all AS components, with the leaves containing the highest content. The flavonoid content in leaves is affected by factors including the harvest period, producing area, storage time, and storage conditions and the highest content levels are observed in July. The flavonoid content decreases with the increasing storage time and high humidity (53).
Flavonoids are a class of yellow pigments derived from 2-phenylchromogenone (Figure 3V). Flavonoids have a C6-C3-C6 structure. Figures 3I–U shows that the main flavonoids isolated from AS include epicatechin, wogonin, quercetin, kakaferol, and rutin (54).
4 Extraction and purification of bioactive compounds of AS
4.1 Polysaccharides
With the pursuit of high-quality health, the nutritional and medicinal values of AS polysaccharides have attracted much attention. Various traditional and feasible extraction methods have been established and tested. Table 3 summarizes the extraction and purification conditions of polysaccharides in AS, as well as the yield and structure of polysaccharides from different origins. According to the extreme hydrophilicity and alcohol insolubility of AS, water is often used as a solvent or precipitant. The advantages of hot water extraction (HWE) include practical operation, simple equipment, and easy implementation. Crude polysaccharides can be directly extracted by ethanol precipitation, which is suitable for almost all water-soluble polysaccharides. This method separates the polysaccharide by reducing the dielectric constant of the aqueous solution (1). However, HWE also has significant disadvantages, including a time-consuming process, low efficiency, large solvent consumption, and high temperatures. To improve the extraction efficiency, polysaccharides have been extracted by ultrasound-, microwave-, and enzyme-assisted extraction. Previous research determined the yield of water-soluble polysaccharides from the fruit of AS in Gansu as 3.81% ± 0.18%. Compared with HWE, the yield increased by 154% (42, 43). However, a difference was observed in the molecular weight due to the damaged polysaccharide structure. This damage is attributed to the localized excessive vibration and high temperatures. The extracted crude polysaccharide of AS contains impurities such as proteins, inorganic salts, and pigments, which affect the subsequent analysis. The crude polysaccharide of AS is typically isolated and purified to remove proteins and pigments. The Sevag technique is often applied to remove proteins due to its mild conditions and weak influence on the polysaccharide structures. However, this type of purification requires repeated treatments, which affect the final yield. As a consequence, macroporous resins or dialysis bags are usually necessary to repurify crude polysaccharides (43, 44).
Chain sequencing and structural domains of carbohydrates have been the focus of much in-depth research due to the needs of pharmacology, toxicology, and structural modification. According to the order and mode of main chain glycosylation and the type of monosaccharide, the AS structure can be divided into three types, namely, RG-I, XGA, and AG-II. The RG-I type is mainly high in Rha and GALA, which are linked by 1 → 4 glycosidic bonds. Thus, most polysaccharides belong to the RG-I structure. In addition, polysaccharides with Rha/Gala ratios between 0.25 and 0.73 are easily induced to pectin. The polysaccharide extracted by Hu et al. was composed of glucose, galactose, rhamnose, arabinose, mannose, xylose, and glucuronic, with a molar ratio of 8.83:7.90:4.74:4.55:2.80:2.39:1.00, belonging to the RG-I type. The XGA structural domains represent certain polysaccharides with a high xylose content. For example, the polysaccharides extracted by Li et al. contained xylose (12, 38, 42). In addition, the XGA structural domain coexists with the RG-I structural domain in other samples of AS polysaccharides (55). Lee et al. found that AG-II type Ara and Gal are linked by a 1 → 3 glycosidic bond (48). Moreover, the purified ALP-1 extracted by Hu consists of galactose, glucose, mannose, and arabinose in a molar ratio of 6.1:2.1:1.1:1.0, with the main chain structure of 1,6-α-D-Galp residue, α-D-manp-(1 → 3)-α-L-arf residue at the O-3 position, and α-D-Galp residue at the O-4 position.
4.2 Eleutherosides
Solvents can be used to extract eleutherosides (Table 4). Eleutherosides have strong hydrophilicity, and water, ethanol, or methanol with a high polarity which can be used as the solvent for extraction. To enhance eleutheroside B and E yields, Na-Ri Kim et al. employed the enzyme Novozyme 33095 culture solution to treat AS (78). Enzymes can combine with pectin in the cell walls and decrease the adhesion to the active substance, consequently increasing the dissolution of eleutheroside. Following treatment with Novozyme 33095, eleutheroside B and E yields increased by 25 and 29%, respectively. The optimal extracting conditions have been reported as follows: extraction temperature of 80°C; soaking time of 5 h; ethanol volume fraction of 70%; and material-to-liquid ratio of 1:6 (60).
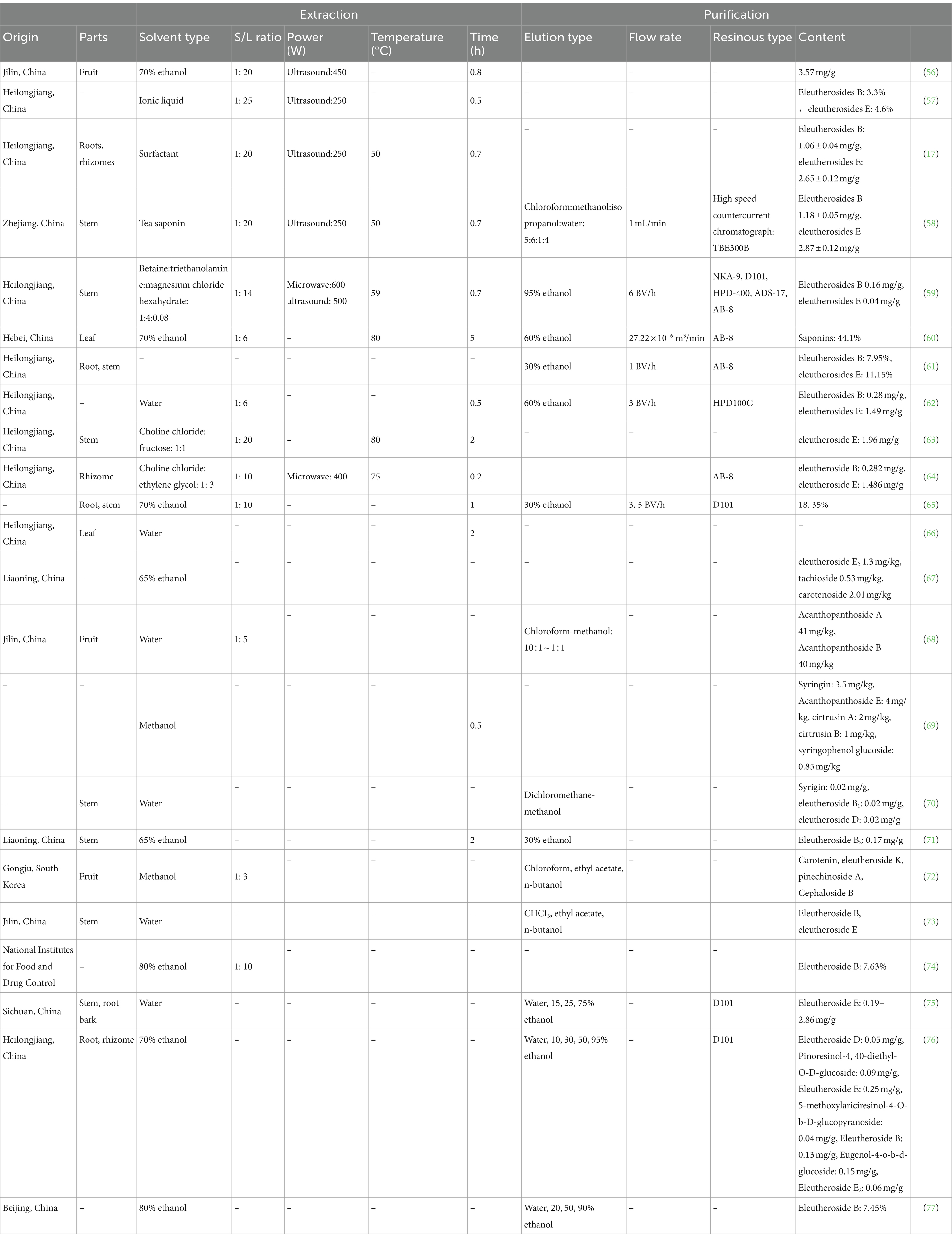
Table 4. Summary of the extraction and purification processes of eleutherosides from Acanthopanax senticosus.
The development and utilization of green solvent systems have recently become a hot spot. In 2003, researchers reported a new type of green solvent denoted as the deep eutectic solvent (DES) (79). DES consists of a hydrogen bond acceptor (HBA) and a hydrogen bond donor (HBD). The HBAs are mostly quaternary ammonium salts and zwitterions, while the HBDs are carboxylic acids, amides, and polyols. The HBD is the main factor affecting the polarity of DES, and the initial polarity of DES is similar to that of 70% of ethanol. DESs are non-toxic and produce minimal environmental pollution. They have high solvency, high extraction efficiency, high selectivity, and a tunable nature compared with traditional organic solvents. The extraction rate of isofraxidin by DES (choline chloride and citric acid) has been reported as 1.56 mg/g, which is 2–3 times that of conventional solvents (80). Ultrasound- and microwave-assisted extraction methods have been employed to improve the movement frequency, macromolecule speed, and extraction efficiency. Du et al. applied alternative ultrasonic- and microwave-assisted methods to extract isofraxidin (81). This method avoids the decomposition and isomerization of natural products due to the long extraction time. Eleutheroside E has also been extracted by ultrasonic extraction mass spectrometry, with DES composed of choline chloride and fructose (1:1) as the extraction solvent (63). The content of glycoside E reached 1.96 mg/g, which was 5–6 times higher than that of 70% of ethanol. Previous research combined microwave ultrasound-assisted extraction with 3-element DES (betaine, triethanolamine, and MgCl2·6H2O) to extract eleutherosides. The yields of isofraxidin, eleutheroside E, eleutheroside B, protocatechuic acid, chlorogenic acid, and ursolic acid reached 18.76, 49.38, 174.82, 2.98, 457.39, and 16.62 μg/g, respectively, representing a 1.04- to 3.19-fold increase compared to those obtained through conventional extraction methods (59).
Eleutherosides in crude extract often coexist with other active ingredients. The separation and purification of eleutherosides are generally carried through ethanol precipitation. High-purity eleutheroside B, eleutheroside E, and isofraxidin can be obtained using a combination of ionic liquid-loaded (1-butyl-3-methyl-imidazolium bisulfate) AB-8 macroporous resin and DESs with molecularly imprinted separation technology (64). Table 4 shows the extraction and purification conditions.
4.3 Flavonoids
The extraction methods of total flavonoids include reflux extraction, ultrasonic/microwave extraction, supercritical fluid extraction, and rapid solvent extraction (Table 5), with ethanol, methanol, enzyme, and DESs typically selected as the solvents. Compared with traditional solvent extraction, the extraction rate of supercritical CO2 extraction is reported to increase by 33.26% (98). This technology is safe, environmentally friendly, has no reagent residue, and consumes little energy. Due to the expensive supercritical extraction equipment and other factors, the ethanol–water reflux extraction of total flavonoids is more suitable for large-scale production. Liu et al. employed enzyme-assisted ultrasonication to extract total flavonoids (85), determining the optimal extraction process as follows: cellulase to pectinase ratio of 3:2; addition of 6,960 U/g of enzymes; enzyme treatment time of 59.80 min; temperature of 53.70°C; and pH of 6.05. Based on these settings, the total flavonoid yield was 36.95 ± 0.05 mg/g.
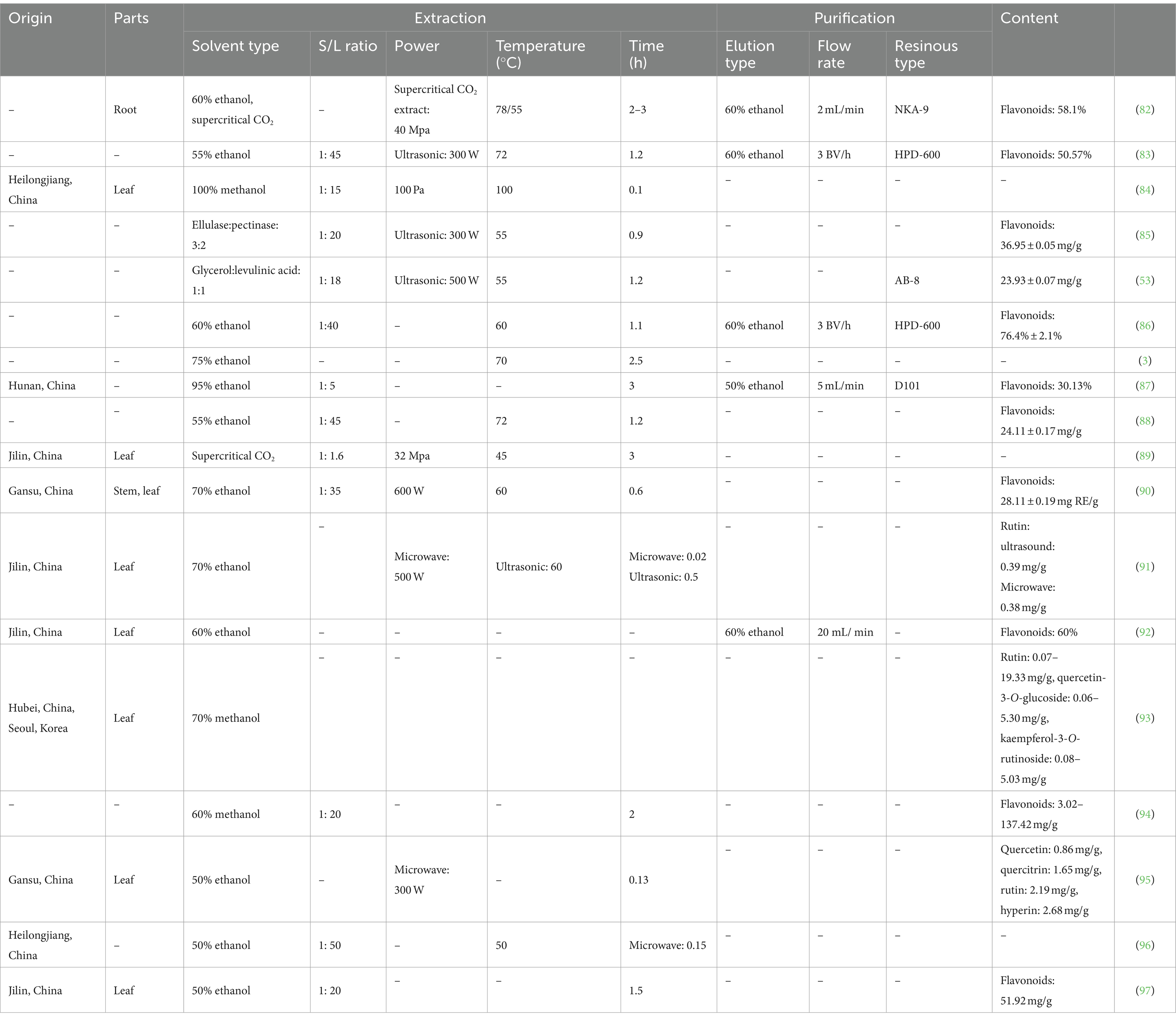
Table 5. Summary of the extraction and purification processes of flavonoids from Acanthopanax senticosus.
Microporous adsorbent resin can be applied to separate the chemical components of herbal medicines. This technique has the advantages of fast adsorption, large adsorption capacity, high elution rate, and simple resin regeneration. Commonly used eluents are water, methanol, ethanol, acetone, and ethyl acetate. Wang et al. employed HPD-600 resin to increase the purity of flavonoids to 50.57% (86). The adsorption process was carried out following quasi-secondary kinetics, in accordance with the Freundlich adsorption model.
5 Biological activities
5.1 Anti-fatigue properties
Fatigue can be divided into sports fatigue and mental fatigue according to its attributes. The former occurs mostly in people who engage in high-intensity physical labor for a long period, while the latter denotes the excessive use of the brain (99). Sports fatigue will lead to mental instability and an accelerated heartbeat with lactic acid accumulation, while central fatigue will produce side effects such as memory loss, drowsiness, and emotional instability (100). Increased exercise endurance is the most potent macroscopic form of fatigue resistance (101).
Studies have reported the anti-fatigue effects of the AS extract (102). Fatigued mice fed with the AS extract showed increased weight-bearing swimming time, tissue glycogen content, and learning memory ability, and decreased blood lactate and urea nitrogen contents. Gao et al. constructed a network diagram of “active ingredients–anti-fatigue targets” to screen the critical components of AS, including eleutherosides B, B1, C, and E (103). The core anti-fatigue targets were predicted to be HSP90AA1, STAT3, VEGFA, and MMP9. Molecular docking predicted that both core components and key targets could spontaneously bind, and the binding ability was similar to that of the clinical drug ATP-2Na. Furthermore, the binding activities of eleutherosides B and B1 were higher than those of the key proteins. The anti-fatigue effect of eleutherosides is mainly executed by regulating HIF-1, PI3K-Akt, insulin signaling pathway, and substance metabolism through biological processes such as drug responses and the positive regulation of cell proliferation (Figure 4A) (52). Cheng et al. established fatigue mouse models to confirm that Erythrina saponins E can reduce blood urea nitrogen (BUN) and enhance the activity of serum lactate dehydrogenase (LDH) to prolong the exhaustion swimming time of mice (104). BUN, the end product of protein metabolism, is an important blood biochemical indicator related to fatigue. Eleutheroside has the ability to reduce the formation of BUN after exercise. Eleutheroside can increase the activity of serum LDH, accelerate the body’s ability to clear lactic acid, and play a role in delaying fatigue. In addition, eleutheroside B regulates the expression of factors associated with the Keap1/Nrf2/ARE signaling pathway to improve learning memory (105).
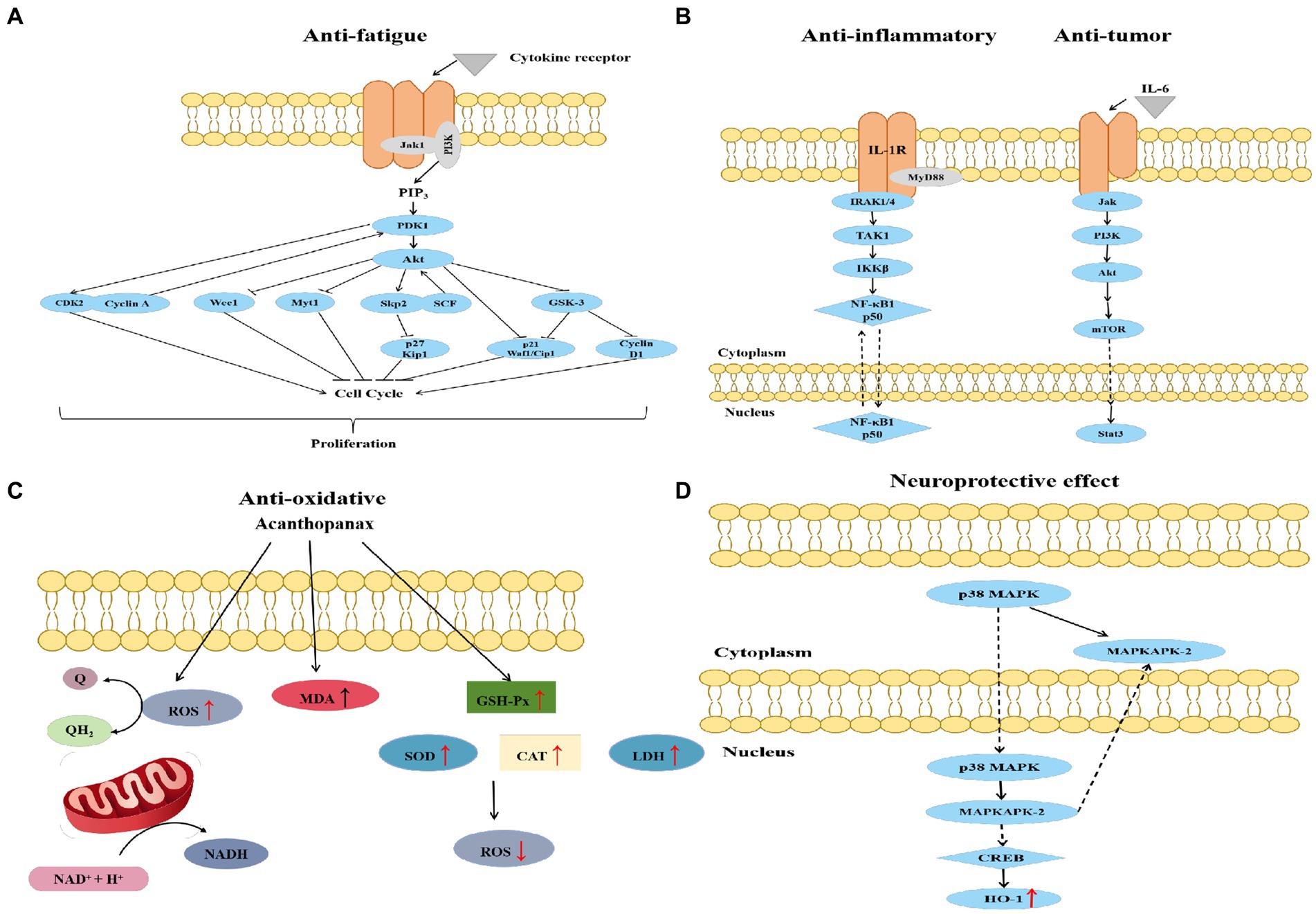
Figure 4. Schematic diagram of anti-fatigue (A), anti-inflammatory, anti-tumor (B), anti-oxidative (C), and neuroprotective (D) mechanisms of Acanthopanax senticosus.
5.2 Anti-inflammatory properties
Fan, Kim, Zhang et al. demonstrated that AS has protective and ameliorative effects on the gastrointestinal tract (106–108). Polysaccharides prevent the lipopolysaccharide-induced amplification of inflammatory mediators via a decrease in ileal mRNA abundance of TNF-α, inducible NO synthase, and IL-1β concentrations. Protein expression of hypoxia-inducible factor-1α, cyclooxygenase-2, and κ-B p65 is also reduced in ileal tissues. Moreover, the height of the ileal villi and the appearance of the epithelial villi of piglets fed with AS polysaccharides have been reported to improve, and polysaccharides can alleviate the reduced average daily feed intake of piglets and decrease the incidence of diarrhea and diarrhea index (106). The extrusion of AS leaves increases the protection of the gastric mucosa and prevents C48/80-induced acute gastric mucosal injury (107). Previous research has shown that serum concentrations of 5-hydroxytryptamine and histamine were reduced in C48/80-modeled rats, while the expression of Bax and Bcl-2 was improved. A similar effect was observed for 200 mg/kg of AS on famotidine, a histamine H2 receptor antagonist, and normalized mucus secretion. These polysaccharides significantly increased the survival of flies and reduced the proliferation and differentiation of intestinal stem cells in response to dextran sodium sulfate through the epidermal growth factor receptor, Jun-N-terminal kinase, and Notch signaling pathways (108). Sodium dodecyl sulfate can induce the melanin phenotype and disrupt epithelial renewal. Therefore, polysaccharides are also effective in reducing sodium dodecyl sulfate-induced epithelial cell death and the accumulation of reactive oxygen species and antimicrobial peptides. AS polysaccharides also have antioxidant effects and improve the level of oxidative stress induced by cerebral ischemia–reperfusion injury (109).
Isofraxidin in AS attenuates the inflammatory response in the lung tissue by decreasing the levels of tumor necrosis factor-α, interleukin-6, neutrophils, and NF-kB (110). Myeloperoxidase activity is also reported to be significantly reduced in the lung tissue. The protective effect on the lungs is associated with the inhibition of cyclooxygenase-2 protein expression. Eleutherosides E can effectively inhibit high-altitude heart injury by regulating NLRP3 inflammatory vesicle activation and cellular death in cardiac tissues (111). Song et al. applied Edwardsiella ictaluri to reveal that AS extract significantly reduced the expression of proinflammatory cytokines including IL-1 (Figure 4B) (112). Edwardsiella ictaluri infection increased IL-1 expression and activated the NF-кB/MyD88 pathway. The results showed that the expression of the NF-кB/MyD88 pathway significantly decreased.
5.3 Antioxidant properties
The excessive production of reactive oxygen species induces the development of cardiovascular diseases such as atherosclerosis and hypertension. It has been shown that AS can reduce lipid peroxidation, with an increase in the activity of superoxide dismutase and catalase in cells (Figure 4C) (113). Oxidative stress also contributes to the development of diabetic complications. AS polysaccharide can significantly reduce the levels of lipid peroxidation markers, such as thiobarbituric acid-responsive substances and lipid hydroperoxides (114). The activities of enzymatic antioxidants, superoxide dismutase, and catalase also significantly increased. Wang et al. showed that AS extracts protected against oxidative stress by increasing the activities of antioxidant enzymes and GSH/GSSG ratios in serum and liver homogenates (115). Both medium and high doses of AS aqueous extracts significantly increased Nrf2 and antioxidant enzymes. The antioxidant properties of AS polysaccharides have been reported as equally effective in zebrafish in vivo. The purified polysaccharide can significantly scavenge hydrogen peroxide and reduce hydrogen peroxide-induced cell death in zebrafish (116).
5.4 Antitumor and anticancer properties
AS has good antitumor and anticancer pharmacological effects. Cytotoxicity experiments revealed that the cells of colon (SW 620), breast (MDA-MB-231, MCF-7), gastric (Kato-III), ductal (BT474), bronchial (Chago K-1), prostate (PC-3), and hepatic hepatoblastoma (Hep-G2) cancers produced certain toxic responses and inhibitory effects under the action of AS (117–121). Syringin from AS can inhibit the proliferation and migration of breast cancer cells and promote apoptosis by regulating the EGFR-RAS–RAF–MEK–ERK and PI3K-AKTCOX-2 signaling pathways (Figure 4B) (117). The ethanol extract of AS induced liver cancer cell apoptosis by inhibiting nuclear factor-κB activity, with an inhibition rate of 57.55% in HepG2 cells (121). The extract can also decrease the protein levels of matrix metalloproteinase-2 (MMP-2), MMP-9, t-protein kinase B, and p-Akt and increase the protein level of E-cadherin. The results demonstrated that the number of cells penetrating the basement membrane was significantly reduced after the treatment with the extract of AS, which suggested that the extract can inhibit the invasion of HepG2 cells. Yamazaki et al. found that isofraxidin, a coumarin component from AS, significantly inhibited hepatocellular carcinoma cell invasion (120). Isofraxidin inhibits terephthalic acid-induced matrix MMP-7 expression in hepatocellular carcinoma cells by preventing ERK1/2 phosphorylation. The IC50 values of the extract for Kato-III and SW 620 cells have been determined as 72.9 and 73.4 μg/mL, respectively (118). Glycoprotein in AS can significantly suppress the metastasis of colon cancer 26-M3.1 cells in the lung (122). In particular, glycoprotein activates macrophages to produce various cytokines and inhibit tumor metastasis or enhance host natural immunity (123).
5.5 Neuroprotective effects
AS can protect neurons and thus has important applications in drug development for Alzheimer’s and Parkinson’s diseases (PD). Mice treated with AS have shown a reduction in climbing time, an increase in the number of voluntary movements, and a reduction in cognitive and spatial memory deficits (28, 124). Synaptic nucleoprotein is the key factor in the pathogenesis of PD (125). In a previous study, after the WT-Syn and A53T-Syn transgenic cells were treated with the extract, the levels of synapsin, caspase-3, parkin, and other related proteins returned to near-normal levels (126). This is consistent with the results reported by Liu et al. (127). The neuroprotective effect of AS can be mediated by the activation of parkin and HO-1 expression, which inhibits caspase-3 activity and concomitantly reduces nitric oxide/ROS production, thereby inhibiting neuronal apoptosis. HO-1, an inducible enzyme present in most cell lines, is protective against glutamate-induced neuronal cell death (128). AS induces HO-1 expression mainly through the p38-CREB pathway (Figure 4D). Previous studies has shown that AS may regulate the structural composition of the gut microbiota, improve metabolic disorders, reduce inflammatory factors, and reverse the PD process (28). Lu et al. found a significant increase in Firmicutes and a significant decrease in Actinobacteria expression in the intestine of ASH-treated Parkinson mice (28). Short-chain fatty acids produced by the fermentation of Firmicutes promoted metabolism by acting on G protein-coupled receptors (129). Actinobacteria cause damage to dopaminergic neurons by producing protease inhibitors (130). These conclusions highlight a novel mechanism underlying the effects of AS on the “brain–gut axis.”
6 Applications
6.1 Functional food
AS is used in wine, beverages, fruit cakes, tea drinks, yogurt, and other products. Zhang et al. employed the rhizome as a raw material to prepare a beverage with the characteristic tea fragrance (131). Zhao et al. also prepared health-preserving tea, and its raw ingredients were AS berries and longan (132). The formula of the health-preserving tea is the ratio of AS to longan was 1:4, with 4% sugar and 0.1% citric acid. Ma et al. produced nutritional yogurt using fresh leaves and milk as the main raw materials. The formulation included 0.2% of xanthan gum, 0.3% of sodium carboxymethyl cellulose, 20% of fresh juice, 7% of sugar, and 3% of milk powder (133). Moreover, Niu et al. developed AS yogurt (134) and He et al. applied AS berries as raw materials to produce a fruit cake. The optimum production formula consisted of sucrose (37.9%), citric acid (2.95%), xanthan gum (2.1%), sodium alginate (2.1%), and agar (1.58%) (135).
6.2 Medicine
AS is a traditional medicinal plant in China. Numerous medicines have been developed with AS raw materials, such as injections, tablets, granules, and capsules. Chinese researchers have developed AS dispersible tablets characterized by a reasonable prescription, a feasible process, a high dissolution degree, and good dispersion uniformity (136). Such medicines can treat weakness, appetite loss, cough, asthma, insomnia, and cardiovascular and cerebrovascular diseases (137). Studies have shown that AS delays physical fatigue and relieves mental fatigue by improving memory retention and increasing spontaneous activity (102). AS is widely used in clinical practice to treat transient ischemic attacks, and its injection is a Chinese patent medicine often used for treating ischemic heart disease.
6.3 Animal dietary additive
Early weaning of piglets leads to decreased growth performance and an increased incidence of diarrhea and disease. Antibiotics are traditionally used to prevent and treat intestinal disorders caused by weaning stress. However, the continued use of antibiotics results in drug and antibiotic residues in the animals. Novel feed additives are thus required to replace antibiotics. AS extract, as a promising alternative to antimicrobial agents, can effectively enhance the digestibility and absorption of amino acids in weaned piglets (138). Yin et al. found that AS promoted the diversity of cecal microbiota, with the number of lactic acid bacteria increasing (p < 0.05) and Escherichia coli decreasing (p < 0.05) as the weaning age progressed.
In summary, the application of AS is mainly centered on functional food and medicine. Despite the great progress made by recent research, the application of AS is still associated with several shortcomings. For example, the development of the product has remained in the laboratory stage and cannot be well industrialized. This indicates that the research on AS lacks depth. Moreover, the product category is simple, which cannot meet the demand of the consumers for healthy lives. The lack of standards for the testing results in the uneven quality of AS products on the market. In view of the aforementioned problems, scholars should focus on the transfer of experiments from the small scale to the industrial scale to achieve industrialization. Additional AS products should also be developed such that consumers can gradually accept them and improve their living standards. Furthermore, scholars and experts should formulate corresponding production and inspection standards to improve the quality of such products and increase consumer satisfaction.
7 Conclusion
AS is rich in active ingredients, has a wide range of pharmacological effects, and shows great potential for clinical applications. However, controversies exist in some areas related to whether AS belongs to the category of medicine-food homology. Moreover, while emerging extraction technologies can improve efficiency, they may decrease the yield, resulting in a greater waste of resources. Although DES extraction is environmentally friendly, the solvent recovery of DES is difficult and reduces extraction efficiency. In terms of application, AS-related health products are limited. There is also a lack of quality control standards for AS products. Furthermore, in recent years, new delivery systems using biological macromolecules as wall materials are developing rapidly, providing humans with new directions and technologies in food and medicine industries. AS and its active components can be applied as a wall core, while biological macromolecules can be adopted as the wall material to construct a drug delivery system. However, the application of AS in this field is relatively new and requires much work. Thus, further research should focus on the following: (1) employing optimized extraction methods to improve the yield of bioactive compounds in AS; (2) establishing a clear definition of AS and formulating relevant quality control indicators; (3) conducting more clinical trials to verify the health benefits of AS for humans; (4) exploring the application of AS in the prevention and treatment of diseases; (5) developing healthcare foods and other products based on AS.
In conclusion, with the development of science and life, health has garnered increasing attention. AS is rich in nutrients and holds economic and developmental value. Moreover, the promotion of AS can maintain, innovate, and develop traditional Chinese medicine culture and products. With the further understanding of AS and the improvement of technology, AS will soon be widely employed in both clinical and functional food sectors.
Author contributions
XZ: Software, Writing – original draft, Writing – review & editing. LG: Software, Writing – original draft, Writing – review & editing. LZ: Software, Writing – review & editing. KW: Software, Writing – review & editing. YG: Writing – review & editing. JL: Writing – review & editing. SY: Writing – review & editing. NJ: Writing – review & editing. YZ: Supervision, Writing – review & editing. XY: Writing – review & editing. BL: Supervision, Writing – review & editing.
Funding
The author(s) declare financial support was received for the research, authorship, and/or publication of this article. This study was supported by the National Key Research and Development Program of China (2021YFD2100902), the Agricultural science and technology innovation project of Heilongjiang Academy of Agricultural Sciences (CX23GG16–04), and the Research Funding for Scientific Research Institutes in Heilongjiang Province of China (CZKYF2022-1-B021).
Conflict of interest
The authors declare that the research was conducted in the absence of any commercial or financial relationships that could be construed as a potential conflict of interest.
Publisher’s note
All claims expressed in this article are solely those of the authors and do not necessarily represent those of their affiliated organizations, or those of the publisher, the editors and the reviewers. Any product that may be evaluated in this article, or claim that may be made by its manufacturer, is not guaranteed or endorsed by the publisher.
References
1. Li, X, Chen, C, Leng, A, and Qu, J. Advances in the extraction, purification, structural characteristics and biological activities of Eleutherococcus senticosus polysaccharides: a promising medicinal and edible resource with development value. Front Pharmacol. (2021) 12:753007. doi: 10.3389/fphar.2021.753007
2. Kim, YH, Cho, ML, Kim, DB, Shin, GH, Lee, JH, Lee, JS, et al. The antioxidant activity and their major antioxidant compounds from Acanthopanax senticosus and A. koreanum. Molecules. (2015) 20:13281–95. doi: 10.3390/molecules200713281
3. Yu, M, Wang, B, Qiao, A, and Ji, Y. Preparation of the particles of Acanthopanax senticosus. Saudi J Biol Sci. (2019) 26:1932–5. doi: 10.1016/j.sjbs.2019.07.008
4. Davydov, M, and Krikorian, AD. Eleutherococcus senticosus (Rupr. & Maxim.) Maxim. (Araliaceae) as an adaptogen: a closer look. J Ethnopharmacol. (2000) 72:345–93. doi: 10.1016/s0378-8741(00)00181-1
5. Saito, T, Nishida, M, Saito, M, Eitsuka, T, and Nishida, H. The fruit of Acanthopanax senticosus (Rupr. Et maxim) harms, or goka, improves insulin resistance in high-fat diet fed obese mice. Free Radical Bio Med. (2016) 100:S177. doi: 10.1016/j.freeradbiomed.2016.10.469
6. Cui, Y, Zhang, Y, and Liu, G. Syringin may exert sleep-potentiating effects through the NOS/NO pathway. Fund Clin Pharmacol. (2015) 29:178–84. doi: 10.1111/fcp.12095
7. Smalinskiene, A, Savickiene, N, Zitkevicius, V, Pangonyte, D, Sadauskiene, I, Kasauskas, A, et al. Effect of Acanthopanax senticosus on the accumulation of cadmium and on the immune response of spleen cells. J Toxicol Env Heal A. (2014) 77:1311–8. doi: 10.1080/15287394.2014.924453
8. Zhang, Y, Liu, BZ, and Pei, YH. Research progress on pharmacological action of Acanthopanax senticosus. J Shenyang Pharma Univ. (2002):143–6. doi: CNKI:SUN:SYYD.0.2002-02-017
9. Ji, Y, Zhou, T, Gong, YX, Sun, CH, Zhang, Y, and Wang, LH. Study of the nutritional components of ultrasonically broken Acanthopanax senticosus leaf tea. Food Res Dev. (2022) 43:177–81. doi: 10.13422/j.cnki.syfjx.20200313
10. Guan, Y, Guo, YD, Chen, G, Wu, ZS, and Yin, JZ. Analysis and quality evaluation of nutritional components in the purple Acanthopanax senticosus. J Food Saf Food Qual. (2018) 9:1021–5.
11. Kim, MK, Jin, YS, Heo, SI, Shim, TH, Sa, JH, and Wang, MH. Studies for component analysis and antioxidant effect, antimicrobial activity in Acanthopanax senticosus harms. Korean J Pharmacogn. (2006) 37:151–6.
12. Chen, RZ, Liu, ZQ, Zhao, JM, Chen, RP, Meng, FL, Zhang, M, et al. Antioxidant and immunobiological activity of water-soluble polysaccharide fractions purified from Acanthopanax senticosu. Food Chem. (2011) 127:434–40. doi: 10.1016/j.foodchem.2010.12.143
13. Song, Y, Wu, KX, Shao, WD, Liu, YL, Liu, J, Liu, Y, et al. Development-specific investigation of leaf primary metabolism of Acanthopanax senticosus and Acanthopanax sessiliflorus. Bull Bot Res. (2022) 42:268–77. doi: 10.7525/j.issn.1673-5102.2022.02.012
14. Li, J, Guo, S, Wang, Q, Fu, S, and Wang, Z. Determination of polysaccharide content, cluster analysis in Acanthopanax senticosus from different producing areas and optimization of its ultrasonic extraction technology. China Pharm. (2019) 30:2541–5. doi: 10.6039/j.issn.1001-0408.2019.18.17
15. Lai, YH, Shen, X, and Yi, GC. Determination and evaluation of crude fiber of common potherbs in Jilin area. J Beihua Univ. (2017) 18:463–465.
16. Wu, KW, Xu, QY, Zhu, LJ, Yang, LN, and Ma, T. Variations of enzymatic activity and main quality components during the fermentation process of Acanthopanax senticosus tea. IOP Conf Ser: Mater Sci Eng. (2019) 592:12021. doi: 10.1088/1757-899x/592/1/012021
17. Yang, X, Liu, T, Qi, S, Gu, H, Li, J, and Yang, L. Tea saponin additive to extract eleutheroside B and E from Eleutherococcus senticosus by ultrasonic mediation and its application in a semi-pilot scale. Ultrason Sonochem. (2022) 86:106039. doi: 10.1016/j.ultsonch.2022.106039
18. Zhao, YQ, Liu, JH, and Zhao, GR. Separation and identification of fatty acids and vinegar from Acanthopanax acanthopanax. ACTA Chinese Med Pharmacol. (1989):55–6.
19. Liu, H, Jin, SY, and Yang, LX. Effects of nitrogen fertilizer on carbon metabolism and growth and development of Acanthopanax senticosus seedlings. Chinese J Exp Tradit Med Formulae. (2021) 26:152–6. doi: 10.13422/j.cnki.syfjx.20200313
20. Chen, H, Tan, S, and Luo, X. Research progress of traditional Chinese medicine for genuine regional drug. Lishizhen Med Mater Med Res. (2018) 9:e12575–230. doi: 10.1016/j.biopha.2021.111310
21. Lee, I-H, Chung, H-J, Shin, J-S, Ha, I-H, Kim, M-R, Koh, W, et al. Influence of boiling duration of GCSB-5 on index compound content and antioxidative and anti-inflammatory activity. Pharmacogn Mag. (2017) 13:418–24. doi: 10.4103/pm.pm_425_16
22. Xue, ZJ, and Zhao, YX. Determination of vitamin C in Acanthopanax senticosus VC oral liquid by HPLC. Heilongjiang Sci Tech Inf. (2009) 175. doi: 10.3969/j.issn.1673-1328.2009.08.177
23. Du, B, Wang, JJ, and Jing, WR. Determination of content of vitamin B1 in compound Acanthopanax senticosus tablet with by HPLC. Chinese J Trauma Disablility Med. (2011) 19:1–2.
24. Kim, C, Bae, HM, and Baik, I. Potential antiaging and hepatoprotective effects of Acanthopanax senticosus extracts in adult rat models. Rejuv Res. (2023) 26:51–6. doi: 10.1089/rej.2022.0051
25. Yang, S, Shan, C, Ma, X, Qin, Y, Ju, A, Duan, A, et al. Immunomodulatory effect of Acanthopanax senticosus polysaccharide on immunosuppressed chickens. Poultry Sci. (2021) 100:623–30. doi: 10.1016/j.psj.2020.11.059
26. Lin, Y, Pan, J, Liu, Y, Yang, H, Wu, G, and Pan, Y. Acanthopanax trifoliatus (L.) Merr polysaccharides ameliorates hyperglycemia by regulating hepatic glycogen metabolism in type 2 diabetic mice. Front Nutr. (2023) 10:1111287. doi: 10.3389/fnut.2023.1111287
27. Song, C, Yin, Y, Qin, Y, Li, T, Zeng, D, Ju, T, et al. Acanthopanax senticosus extract alleviates radiation-induced learning and memory impairment based on neurotransmitter-gut microbiota communication. CNS Neurosci Ther. (2023) 29:129–45. doi: 10.1111/cns.14134
28. Lu, Y, Gao, X, Nan, Y, Mohammed, SAD, Fu, J, Wang, T, et al. Acanthopanax senticosus harms improves Parkinson’s disease by regulating gut microbial structure and metabolic disorders. Heliyon. (2023) 9:e18045. doi: 10.1016/j.heliyon.2023.e18045
29. Li, J, He, Y, Fu, J, Wang, Y, Fan, X, Zhong, T, et al. Dietary supplementation of Acanthopanax senticosus extract alleviates motor deficits in MPTP-induced Parkinson’s disease mice and its underlying mechanism. Front Nutr. (2023) 9:1121789. doi: 10.3389/fnut.2023.1121789
30. Kuang, F, and Xiang, T. Molecular mechanism of Acanthopanax senticosus in the treatment of Alzheimer’s disease based on network pharmacology and molecular docking. Mol Divers. (2022) 27:2849–65. doi: 10.1007/s11030-022-10586-3
31. Su, J, Fu, X, Liu, R, Zhang, X, Xue, J, Li, Y, et al. Antioxidant and antiapoptotic properties of n-butanol fraction the Acanthopanax senticosus extracts in H2O2-RAW264.7 cells and CCl4-induced liver injury in mice. Evid-Based Compl Alt. (2023) 2023:1–17. doi: 10.1155/2023/9190198
32. Gu, X, Zhang, G, Wang, Q, Song, J, Li, Y, Xia, C, et al. Integrated network pharmacology and hepatic metabolomics to reveal the mechanism of Acanthopanax senticosus against major depressive disorder. Front Cell Dev Biol. (2022) 10:900637. doi: 10.3389/fcell.2022.900637
33. Song, C, Li, S, Duan, F, Liu, M, Shan, S, Ju, T, et al. The therapeutic effect of Acanthopanax senticosus components on radiation-induced brain injury based on the pharmacokinetics and peurotransmitters. Molecules. (2022) 27:1106. doi: 10.3390/molecules27031106
34. Jia, A, Shi, Y, Zhang, Y, Diao, Y, Chang, B, Jiang, M, et al. N-butanol extract of Acanthopanax senticosus (Rupr. Et maxim.) harms alleviates atherosclerosis in apolipoprotein E-deficient mice fed a high-fat diet. Chem Biodivers. (2023) 20:e202200949. doi: 10.1002/cbdv.202200949
35. Li, X, Zhang, Z, Guo, Z, Zhao, L, Liu, Y, Ma, X, et al. Macrophage immunomodulatory activity of Acanthopanax senticousus polysaccharide nanoemulsion via activation of P65/JNK/ikkαsignaling pathway and regulation of Th1/Th2 cytokines. Peerj. (2021) 9:e12575. doi: 10.7717/peerj.12575
36. Xie, MY, Yin, YJ, and Nie, SP. Progress in structural analysis of polysaccharides derived from natural products. J Chinese Inst Food Sci Tech. (2017) 17:1–19. doi: 10.16429/j.1009-7848.2010.02.035
37. Duan, X, Ju, H, Jiao, Y, Tang, Z, and Song, Y. Study on ultrasonic extraction technology of polysaccharides from Acanthopanax senticosus based on response surface method. Henan Tradit Chinese Med. (2019) 39:137–41. doi: 10.16367/j.issn.1003-5028.2019.01.0034
38. Li, LH, Li, K, Li, XH, Zhao, K, and Nie, XP. Study on the extraction technology for Acanthopanax senticosus polysaccharide. Feed Res. (2019) 42:93–6. doi: 10.13557/j.cnki.issn1002-2813.2019.06.026
39. Yu, M, Wang, B, Fu, YS, Wang, SW, and Ji, YB. Reaserch on extraction technology of polysaccharides from Acanthopanax senticosus waste residue by response surface methodology. Chinese Tradit Herb Drug. (2019) 50:1610–3. doi: 10.7501/j.issn.0253-2670.2019.07.017
40. Liu, XW, Zheng, LS, Xie, X, Dai, L, and Liu, XQ. Extraction, isolation, and purification of polysaccharides from leaves of Acanthopanax gracilistylus and property of their moisture retention. Chinese Tradit Herbal Drug. (2015) 46:1167–73. doi: 10.7501/j.issn.0253-2670.2015.08.013
41. Bi, X, Xu, K, Zhao, Y, and Zheng, R. Exaction, separation and activity of polysaccharides from Acanthopanax sessiliflorus. Pharmacol Clin Chinese Mater Med. (2022) 38:86–92. doi: 10.13412/j.cnki.zyyl.20220117.002
42. Hu, HB, Liang, HP, Wang, YF, Yuan, RN, Jiao, S, Zhang, LL, et al. Ultrasound-assisted extraction of water-soluble polysaccharides from the fruit of Acanthopanax brachypus: physicochemical, structural and functional properties. Chem Biodivers. (2021) 18:e2000947. doi: 10.1002/cbdv.202000947
43. Hu, HB, Liang, HP, Li, HM, Yuan, RN, Sun, J, Zhang, LL, et al. Isolation, purification, characterization and antioxidant activity of polysaccharides from the stem barks of Acanthopanax leucorrhizus. Carbohyd Polym. (2018) 196:359–67. doi: 10.1016/j.carbpol.2018.05.028
44. Chen, Y, Zhang, J, Li, QQ, Wu, J, Sun, FX, Liu, ZJ, et al. Response surface methodology for optimizing the ultrasound-assisted extraction of polysaccharides from Acanthopanax giraldii. Chem Pharm Bull. (2018) 66:785–93. doi: 10.1248/cpb.c18-00030
45. Zhao, ZY, Xu, XJ, Ye, QW, and Dong, LL. Ultrasound extraction optimization of Acanthopanax senticosus polysaccharides and its antioxidant activity. Int J Biol Macromol. (2013) 59:290–4. doi: 10.1016/j.ijbiomac.2013.04.067
46. Yang, Y, Xiu, Y, and Huang, W. Structural analysis and hypoglycemic activity analysis of polysaccharides from Acanthopanax sessiliflorus. China surfactant Deterg. Cosmetics. (2022) 52:1208–13. doi: 10.16429/j.1009-7848.2021.08.028
47. Chen, Y, Li, Q, Tan, X, Lu, X, Xu, X, Xin, Y, et al. Study on characterizaton of polysaccharides from Acanthopanax giraldll harms. Nat Prod Res Dev. (2004) 16:507–11.
48. Lee, JB, Tanikawa, T, Hayashi, K, Asagi, M, Kasahara, Y, and Hayashi, T. Characterization and biological effects of two polysaccharides isolated from Acanthopanax sciadophylloides. Carbohyd Polym. (2015) 116:159–66. doi: 10.1016/j.carbpol.2014.04.013
49. Hu, HB, Liang, HP, and Wu, Y. Isolation, purification and structural characterization of polysaccharide from Acanthopanax brachypus. Carbohyd Polym. (2015) 127:94–100. doi: 10.1016/j.carbpol.2015.03.066
50. Xia, YG, Huang, YX, Liang, J, and Kuang, HX. Comparable studies of two polysaccharides from leaves of Acanthopanax senticosus: structure and antioxidation. Int J Biol Macromol. (2020) 147:350–62. doi: 10.1016/j.ijbiomac.2019.12.244
51. Zhao, P. Extraction and biotransformation of Acanthopanax senticosus Saponins. Liaoning: Dalian Polytechnic University (2013).
52. Gao, H. Study on the preparation of Acanthopanax senticosus glycosidesmicroemulsion and its anti-fatigue mechanism. Jilin: Changchun University of Chinese Medicine (2021).
53. Zhang, X, Su, J, Chu, X, and Wang, X. A green method of extracting and recovering flavonoids from Acanthopanax senticosus using deep eutectic solvents. Molecules. (2022) 27:923–41. doi: 10.3390/molecules27030923
54. Jian, YF, and Hu, HB. Research progress on chemical constituents and pharmacological activities of Acanthopanax senticosus. J Chinese Med Mater. (2011):1302–6.
55. Yang, SL, Wang, YF, and Chen, N. Study on Acanthopanax senticosus polysaccharide of the present situation and the progress. Heilongjiang Med J. (2006) 66:3447–58. doi: 10.1007/BF02962382
56. Du, K, and Chen, J. Process optimization for ultrasonic extraction of total saponins from Jilin Changbaishan wild Acanthopanax senticosus. Res Dev Nat Prod. (2012) 24:1288–91. doi: 10.16333/j.1001-6880.2012.09.030
57. Yang, L, Ge, HS, Wang, WJ, Zu, YG, Yang, FJ, Zhao, CJ, et al. Development of sample preparation method for eleutheroside B and E analysis in Acanthopanax senticosus by ionic liquids-ultrasound based extraction and high-performance liquid chromatography detection. Food Chem. (2013) 141:2426–33. doi: 10.1016/j.foodchem.2013.05.094
58. Qi, S. Study on the extraction and SeparationTechnology of Eleutheroside B and E. Heilongjiang: Northeast Forestry University (2020).
59. Pan, HY, Wang, ZL, Nie, SM, Yu, L, Chang, YH, Liu, ZG, et al. Novel green three-constituent natural deep eutectic solvent enhances biomass extraction from Acanthopanax senticosus and the extraction mechanism. ACS Sustain Chem Eng. (2021) 9:8835–47. doi: 10.1021/acssuschemeng.1c02116
60. Zhao, P, Tian, J, Fei, X, Xu, LQ, Li, J, and Yu, Y. Extraction of Acanthopanax senticosus saponins. J Dalian Polytech Univ. (2013) 32:316–9.
61. Shao, J, Liu, S, Mou, H, Fan, Z, An, L, and Dong, Y. Separation and purification of syringin and elentheroside E by macroporous adsorbents. Chinese J Exp Tradit Med Formulae. (2011) 17:10–14. doi: 10.13422/j.cnki.syfjx.2011.02.013
62. Yang, F, Yang, L, Wang, W, Liu, Y, Zhao, C, and Zu, Y. Enrichment and purification of syringin, eleutheroside E and isofraxidin from Acanthopanax senticosus by macroporous resin. Int J Mol Sci. (2012) 13:8970–86. doi: 10.3390/ijms13078970
63. Mu, LT, Zhang, QD, Sun, SY, Liu, B, Zhang, Y, Zhang, XR, et al. Study on the technology of efficient extraction of eleutheroside E from Acanthopanax senticosus by green solvent DES. Phytochem Anal PCA. (2022) 33:879–85. doi: 10.1002/pca.3144
64. Li, JH. Study on immobilized ionic liquids and deep eutectic solvents MolecularlyImprinted separation of active constituents from Acanthopanax senticosus efficiently. Heilongjiang: Northeast Forestry University (2020).
65. Tian, Z, Ma, C, Huang, Y, Zhou, Y, Cui, H, and Sun, M. Optimization of extraction and purification processes for anti-fatigue and sedative ingredients from Acanthopanacis senticosi Radix et Rhizoma Seu caulis. Chinese J Exp Tradit Med Formul. (2013) 19:38–42. doi: 10.11653/syfj2013190038
66. Liu, CH, Li, M, Feng, YQ, Hu, YJ, and Yu, BY. Application of ultra-performance liquid chromatography with time-of-flight mass spectrometry for the rapid analysis of constituents and metabolites from the extracts of Acanthopanax senticosus harms leaf. Pediatr Rheumatol Online J. (2016) 12:13–20. doi: 10.4103/0973-1296.176008
67. Wang, Q, and Li, ZF. Chemical constituents from Acanthopanax senticosus (rupr.Et maxim) harms. Liaoning J Tradit Chinese Med. (2010) 37:212–3. doi: 10.13192/j.ljtcm.2010.s1.214.wangq.161
68. Yan, ZW, Liu, JP, Lu, D, and Li, PY. Chemical constituents from pulp of Acanthopanax senticosus (Rupr. Et maxim) harms. Res Dev Nat Prod. (2013) 25:338–41.
69. Wang, Y, Jin, Y, Xu, B, Sun, J, and Li, X. Chemical constituents of stems of Acanthopanax senticosus. Chinese Pharm J. (2014) 49:1701–3. doi: 10.11669/cpj.2014.19.007
70. Zhang, Y, Wu, Z, Gao, H, Huang, J, Sun, B, and Wu, L. Isolation and identification of chemical constituents from stems and leaves of Acanthopanax acanthopanax. J Shenyang Pharm Univ. (2010) 27:110–2. doi: 10.14066/j.cnki.cn21-1349/r.2010.02.007
71. Li, ZF, Wu, ZH, Chen, G, Zhang, QH, and Pei, YH. Two new compounds from Acanthopanax senticosus harms. J Asian Nat Prod Res. (2009) 11:716–9. doi: 10.1080/10286020903020578
72. Lee, JM, Lee, DG, Lee, KH, Cho, SH, Nam, K-W, and Lee, S. Isolation and identification of phytochemical constituents from the fruits of Acanthopanax senticosus. Afr J Pharm Pharmacol. (2013) 7:294–01. doi: 10.5897/ajpp12.898
73. Ryu, J, Son, D, Kang, J, Kim, H-S, Kim, B-K, and Lee, S. A benzenoid from the stem of Acanthopanax senticosus. Arch Pharm Res. (2004) 27:912–4. doi: 10.1007/bf02975842
74. Lu, F, Sun, Q, Bai, Y, Bao, SR, Li, XZ, Yan, GL, et al. Characterization of eleutheroside B metabolites derived from an extract of Acanthopanax senticosus harms by high-resolution liquid chromatography/quadrupole time-of-flight mass spectrometry and automated data analysis. Biomed Chromatogr. (2012) 26:1269–75. doi: 10.1002/bmc.2688
75. Wang, ZW, Zhang, LX, and Sun, YQ. Semipreparative separation and determination of eleutheroside E in Acanthopanax giraldii harms by high-performance liquid chromatography. J Chromatogr Sci. (2005) 43:249–52. doi: 10.1093/chromsci/43.5.249
76. Zhang, XQ, Lai, YC, Wang, L, Xu, FF, Gao, MH, Li, H, et al. Phenylpropanoid constituents from Acanthopanax senticosus. Biochem Syst Ecol. (2011) 39:861–3. doi: 10.1016/j.bse.2011.04.008
77. Li, C, Wang, X, Hu, X, Fan, H, and Qiao, S. Determination of eleutheroside B in antifatigue fraction of Acanthopanax senticosus by HPLC. China J Chinese Mater Med. (2008) 33:2800–2802. doi: CNKI:SUN:ZGZY.0.2008-23-020
78. Kim, NR, Park, JS, Lee, DS, and Shim, JH. Eleutherosides extraction from Acanthopanax sessiliflorus Seeman and Eleutherococcus senticosus maxim using an enzymatic process. J Kor Soc Food Sci Nutr. (2016) 45:1273–8. doi: 10.3746/jkfn.2016.45.9.1273
79. Xu, K, Wang, Y, Huang, Y, Li, N, and Wen, Q. A green deep eutectic solvent-based aqueous two-phase system for protein extracting. Anal Chim Acta. (2015) 864:9–20. doi: 10.1016/j.aca.2015.01.026
80. Sun, CH, Hou, JH, Sun, SY, Zhang, Y, Zhang, XR, and Mu, LT. Efficient extraction and purification of isofraxidin from Acanthopanax senticosus by DES (preprint). (2023).
81. Du, YD, Li, JH, Yu, JT, and Ma, CH. Alternative ultrasonic and microwave assisted extraction of isofraxidin from Acanthopanax senticosus. Bot Res. (2019) 8:25–33. doi: 10.12677/BR.2019.81004
82. Qu, YG. Research of the process for extraction of Total flavonoids from Acanthopanax senticosus. Jilin: Changchun University Of Technology (2012).
83. Wang, X. The isolation and purification of Total flavonoids from Acanthopanax senticosus and anti-inflammation activity. Shandong: Liaocheng University (2022).
84. Wu, T, Xu, H, Zheng, C, and Xu, C. Extraction of flavanoids from Acanthopanax senticosus (Rupr. Et maxim) leaves by pressurized solvent extraction. J Chinese Inst Food Sci Tech. (2013) 13:59–65. doi: CNKI:SUN:ZGSP.0.2013-07-019
85. Liu, RX, Chu, XL, Su, JQ, Fu, X, Kan, QB, Wang, XY, et al. Enzyme-assisted ultrasonic extraction of total flavonoids from Acanthopanax senticosus and their enrichment and antioxidant properties. PRO. (2021) 9:1708. doi: 10.3390/pr9101708
86. Wang, XY, Su, JQ, Chu, XL, Zhang, XY, Kan, QB, Liu, RX, et al. Adsorption and desorption characteristics of total flavonoids from Acanthopanax senticosus on macroporous adsorption resins. Molecules. (2021) 26:4162–80. doi: 10.3390/molecules26144162
87. Luo, J, Li, Z, Zhang, BB, Gao, DL, Zou, QP, and Liu, XQ. Purification of total flavonoids from leaves of Acanthopanax henryi. Chin Tradit Herb Drug. (2017) 48:4661–7. doi: 10.7501/j.issn.0253-2670.2017.22.011
88. Kan, QB, Liu, RX, and Wang, XY. Process optimization and in vitro antioxidant activity of flavonoids extracted from Acanthopanax senticosus. Fujian J Agr Sci. (2021) 36:358–68. doi: 10.19303/j.issn.1008-0384.2021.03.015
89. Liu, CM, Zhao, JM, Li, HM, and Song, FR. Supercritical fluid extraction of total flavonoids from leaves of Acanthopanax senticosus harms. Univ Chem Res (English). (2007) 23:233–6.
90. Wang, YB, Shi, Y, and Yuan, YJ. Ultrasonic assisted extraction of polyphenols and flavonoids from Maijishan wild Acanthopanax and their antioxidant activities in vitro. Res Dev Nat Prod. (2019) 31:2153–62. doi: 10.16333/j.1001-6880.2019.12.020
91. Chen, H, and Kwan, Y. Effects of different extraction methods on the contents of flavonoids in young leaves of Acanthopanax acanthopanax. Health Persp. (2020):99–01.
92. Zhou, H. Purification of Total flavonoids from Acanthopanax senticosus leaves and study on its fingerprints. Jilin: Jilin Agricultural University (2008).
93. Zhang, XD, Zhou, XQ, Liu, XQ, Li, XL, and Whang, W. Development and application of an HPLC-UV procedure to determine multiple flavonoids and phenolics in Acanthopanax leaf extracts. J Chromatogr Sci. (2016) 54:574–82. doi: 10.1093/chromsci/bmv189
94. Liu, C, Zhao, W, Han, Y, Chen, Y, Zhai, X, and Liu, Y. Extraction process and content determination of total flavonoids in Acanthopanax giraldii harms. Med Plants. (2016) 7:37–41. doi: CNKI:SUN:MDPT.0.2016-Z3-012
95. Hu, HB, Zheng, XD, and Hu, HS. Analysis of flavonoids from leaves of Acanthopanax brachypus harms. J Chil Chem Soc. (2013) 58:1549–52. doi: 10.4067/s0717-97072013000100011
96. Wang, Y, and Chu, HT. Analysis of flavonoids in Acanthopanax by UV–vis spectrophotometry with comparative study on different extraction technologies. Adv Mater Res. (2011) 236-238:2630–3. doi: 10.4028/www.scientific.net/AMR.236-238.2630
97. Liu, W. Study on the extraction, isolation and antioxidant activity of the flavonoid compounds from the leaves of Acanthopanax. Jilin: Changchun Normal University (2011).
98. Zhang, MX, Chu, DH, Zhan, RT, Li, JJ, and Hu, B. Study on the extraction process of total flavonoids from Morinda citrifolia L. Biocatal Agr Biotech. (2021) 10:63–5. doi: 10.19759/j.cnki.2164-4993.2021.03.014
99. Huang, LZ. Anti-fatigue constituents and mechanism of Acanthopanacis Senticosi Radix et Rhizoma Seucaulis. Fujian: Fujian University of Traditional Chinese Medicine (2011).
100. Tang, KJ, Nie, RX, Jing, LJ, and Chen, QS. Anti-athletic fatigue activity of saponins (Ginsenosides) from American ginseng (Panax quinquefolium L.). Afr J Pharm Pharmacol. (2009) 3:301–6.
101. Belluardo, N, Westerblad, H, Mudò, G, Casabona, A, Bruton, JD, Caniglia, G, et al. Neuromuscular junction disassembly and muscle fatigue in mice lacking neurotrophin-4. Mol Cell Neurosci. (2001) 18:56–67. doi: 10.1006/mcne.2001.1001
102. Huang, LZ, Huang, BK, Ye, Q, and Qin, LP. Bioactivity-guided fractionation for anti-fatigue property of Acanthopanax senticosus. J Ethnopharmacol. (2011) 133:213–9. doi: 10.1016/j.jep.2010.09.032
103. Gao, H, Xu, W, Zhang, YH, Qiu, ZD, Fu, CM, Qi, XN, et al. Anti-fatigue mechanism of Acanthopanax senticosus glycosides based on network pharmacology. Chinese Tradit Herb Drug. (2021) 2:413–21. doi: 10.7501/j.issn.0253-2670.2021.02.014
104. Cheng, JB. Study on anti-fatigue Spectrum effect relationship and quality evaluation of Acanthopanax senticosus. Heilongjiang: Northeast Forestry University (2022).
105. Zhang, Q, Feng, QX, Zhou, ZY, Li, RQ, and Fang, L. Improvement effect of eleutheroside B on learning and memory abilities of fatigue mice and its mechanism of activating Keap1/Nrf2/ARE signaling pathway. J Jilin Univ. (2020) 46:771–8. doi: 10.13481/j.1671-587x.20200417
106. Fan, C, Han, J, Liu, X, Zhang, F, Long, Y, and Xie, Q. Modulation of hypoxia-inducible factor-1α/cyclo-oxygenase-2 pathway associated with attenuation of intestinal mucosa inflammatory damage by Acanthopanax senticosus polysaccharides in lipopolysaccharide-challenged piglets. Brit J Nutr. (2019) 122:666–75. doi: 10.1017/s0007114519001363
107. Kim, HK, Kim, MG, and Leem, KH. Extrusion process of Acanthopanax senticosus leaves enhances the gastroprotective effect of compound 48/80 on acute gastric mucosal lesion in rats. J Tradit Chin Med. (2016) 36:187–96. doi: 10.1016/s0254-6272(16)30026-7
108. Zhang, H, Wang, SH, and Jin, LH. Acanthopanax senticosus polysaccharide regulates the intestinal homeostasis disruption induced by toxic chemicals in Drosophila. Phytother Res. (2020) 34:193–00. doi: 10.1002/ptr.6522
109. Xie, YL, Zhang, B, and Zhang, YJ. Protective effects of Acanthopanax polysaccharides on cerebral ischemia-reperfusion injury and its mechanisms. Int J Biol Macromol. (2015) 72:946–50. doi: 10.1016/j.ijbiomac.2014.09.055
110. Fei, XJ, Zhu, LL, and Xia, LM. Acanthopanax senticosus attenuates inflammation in lipopolysaccharide-induced acute lung injury by inhibiting the NF-κB pathway. Genet Mol Res. (2014) 13:10537–44. doi: 10.4238/2014.December.12.16
111. Jia, N, Shen, Z, Zhao, S, Wang, Y, Pei, C, Huang, D, et al. Eleutheroside E from pre-treatment of Acanthopanax senticosus (rupr.Et maxim.) harms ameliorates high-altitude-induced heart injury by regulating NLRP3 inflammasome-mediated pyroptosis via NLRP3/caspase-1 pathway. Int Immunopharmacol. (2023) 121:110423. doi: 10.1016/j.intimp.2023.110423
112. Song, Z, Jiao, C, Chen, B, Xu, W, Wang, M, Zou, J, et al. Dietary Acanthopanax senticosus extracts modulated the inflammatory and apoptotic responses of yellow catfish to protect against Edwardsiella ictaluri infection. Aquac Res. (2021) 52:5078–92. doi: 10.1111/are.15379
113. Lyu, SY, Shin, AH, Hahn, DR, and Park, WB. Antioxidant activity of cyanidins isolated from Ogapy (Acanthopanax divaricatus var. albeofructus) fruits in U937 macrophages. Food Sci Biotechnol. (2012) 21:1445–50. doi: 10.1007/s10068-012-0190-2
114. Fu, JF, Yuan, J, Tu, YY, Fu, JF, Zhang, NY, Gao, B, et al. A polysaccharide from Acanthopanax senticosus improves the antioxidant status in alloxan-induced diabetic mice. Carbohyd Polym. (2012) 88:517–21. doi: 10.1016/j.carbpol.2011.12.037
115. Wang, X, Hai, CX, Liang, X, Yu, SX, Zhang, W, and Li, YL. The protective effects of Acanthopanax senticosus harms aqueous extracts against oxidative stress: role of Nrf2 and antioxidant enzymes. J Ethnopharmacol. (2010) 127:424–32. doi: 10.1016/j.jep.2009.10.022
116. Kang, MC, Kim, SY, Kim, EA, Lee, JH, Kim, YS, Yu, SK, et al. Antioxidant activity of polysaccharide purified from Acanthopanax koreanum Nakai stems in vitro and in vivo zebrafish model. Carbohyd Polym. (2015) 127:38–46. doi: 10.1016/j.carbpol.2015.02.043
117. Wang, F, Yuan, C, Liu, B, Yang, YF, and Wu, HZ. Syringin exerts anti-breast cancer effects through PI3K-AKT and EGFR-RAS-RAF pathways. J Transl Med. (2022) 20:310–23. doi: 10.1186/s12967-022-03504-6
118. Ganogpichayagrai, A, and Suksaard, C. Proximate composition, vitamin and mineral composition, antioxidant capacity, and anticancer activity of Acanthopanax trifoliatus. J Adv Pharm Technol. (2020) 11:179–83. doi: 10.4103/japtr.JAPTR_61_20
119. Li, DL, Du, ZY, Li, CY, Liu, Y, Goodin, S, Huang, HR, et al. Potent inhibitory effect of terpenoids from Acanthopanax trifoliatus on growth of PC-3 prostate cancer cells in vitro and in vivo is associated with suppression of NF-κB and STAT3 signalling. J Funct Foods. (2015) 15:274–83. doi: 10.1016/j.jff.2015.03.035
120. Yamazaki, T, and Tokiwa, T. Isofraxidin, a coumarin component from Acanthopanax senticosus, inhibits matrix metalloproteinase-7 expression and cell invasion of human hepatoma cells. Biol Pharm Bull. (2010) 33:1716–22. doi: 10.1248/bpb.33.1716
121. Zhao, B, Zhu, W, Han, X, Li, X, Lu, Y, Cao, X, et al. Ethanol extract of Acanthopanax senticosus (Rupr. & maxim.) harms induces liver cancer cell apoptosis through inhibiting NF-κB. Transl. Cancer Res. (2020) 9:1032–43. doi: 10.21037/tcr.2020.01.02
122. Ha, ES, Hwang, SH, Shin, KS, Yu, KW, Lee, KH, Choi, JS, et al. Anti-metastatic activity of glycoprotein fractionated from Acanthopanax senticosus, involvement of NK-cell and macrophage activation. Arch Pharm Res. (2004) 27:217–24. doi: 10.1007/bf02980109
123. Tanigawa, K, Craig, RA, Stoolman, LM, and Chang, AE. Effects of tumor necrosis factor-&agr; on the in vitro maturation of tumor-reactive effector T cells. J Immunother. (2000) 23:528–35. doi: 10.1097/00002371-200009000-00003
124. Song, C, Duan, F, Ju, T, Qin, Y, Zeng, D, Shan, S, et al. Eleutheroside E supplementation prevents radiation-induced cognitive impairment and activates PKA signaling via gut microbiota. Commun Biol. (2022) 5:680. doi: 10.1038/s42003-022-03602-7
125. Eriksen, JL, Dawson, TM, Dickson, DW, and Petrucelli, L. Caught in the act: α-synuclein is the culprit in Parkinson’s disease (short survey). Neuron. (2003) 40:453–6. doi: 10.1016/s0896-6273(03)00684-6
126. Li, XZ, Zhang, SN, Wang, KX, Liu, HY, Yang, ZM, Liu, SM, et al. Neuroprotective effects of extract of Acanthopanax senticosus harms on SH-SY5Y cells overexpressing wild-type or A53T mutant α-synuclein. Phytomedicine. (2014) 21:704–11. doi: 10.1016/j.phymed.2013.10.012
127. Liu, SM, Li, XZ, Zhang, SN, Yang, Z, Lu, F, Wang, C, et al. Acanthopanax senticosus protects structure andfunction of mesencephalic mitochondria in a mouse model of Parkinson’s disease. Chin J Integr Med. (2018) 24:835–43. doi: 10.1007/s11655-018-2935-5
128. Jin, ML, Park, SY, Kim, YH, Park, G, and Lee, SJ. Acanthopanax senticosus exerts neuroprotective effects through HO-1 signaling in hippocampal and microglial cells. Environ Toxicol Phar. (2013) 35:335–46. doi: 10.1016/j.etap.2013.01.004
129. Karunaratne, TB, Okereke, C, Seamon, M, Purohit, S, Wakade, C, and Sharma, A. Niacin and butyrate: nutraceuticals targeting dysbiosis and intestinal permeability in Parkinson’s disease. Nutrients. (2021) 13:28. doi: 10.3390/nu13010028
130. Caldwell, A, Tucci, ML, Armagost, J, Hodges, TW, Chen, J, Memon, SB, et al. Investigatingbacterial sources of toxicity as an environmental contributor to dopaminergic neurodegeneration. PLoS One. (2009) 4:1–10. doi: 10.1371/journal.pone.0007227
131. Zhang, P, and Yuan, XZ. Production technology of clarified Acanthopanax beverage. Beverage Ind. (2009):33–4. doi: 10.3969/j.issn.1007-7871.2009.04.010
132. Zhao, X, Wang, Y, and Pei, Y. Development of compound beverage of Acanthopanax plus longan. Beverage Ind. (2012) 15:28–30. doi: 10.3969/j.issn.1007-7871.2012.07.008
133. Ma, X. Preparation of health yoghurt from Acanthopanax senticosus. China Brew. (2010):174–6. doi: 10.3969/j.issn.0254-5071.2010.05.053
134. Niu, G, Zhu, D, Zhang, Q, and Meng, X. Processing technology of set Acanthopanax senticosus harms yoghurt. J Shenyang Agr Univ. (2007):194–7. doi: 10.3969/j.issn.1000-1700.2007.02.016
135. He, W, Xu, J, Shao, X, Liu, X, and Li, D. Study on recipe and technology of Changbai mountain Acanthopanax fruit cake. Food Ind Sci Tech. (2012) 343:289–91. doi: CNKI:SUN:SPKJ.0.2012-05-069
136. Gong, F, Liang, T, and Sun, R. Investigation on the formula of Acanthopanax senticosus (Rupr. Et maxim) harms dispersible tablets. Ginseng Res. (2015) 27:37–9. doi: 10.3969/j.issn.1671-1521.2015.01.011
137. Nisibe, S, Kinoshita, H, Takeda, H, and Okano, G. Phenolic compounds from stem bark of Acanthopanax senticosus and their pharmacological effect in chronic swimming stressed rats. Chem Pharm Bull. (1990) 38:1763–5. doi: 10.1248/cpb.38.1763
Keywords: Acanthopanax senticosus, eleutheroside, polysaccharide, flavonoid, biological activity, application
Citation: Zhang X, Guan L, Zhu L, Wang K, Gao Y, Li J, Yan S, Ji N, Zhou Y, Yao X and Li B (2024) A review of the extraction and purification methods, biological activities, and applications of active compounds in Acanthopanax senticosus. Front. Nutr. 11:1391601. doi: 10.3389/fnut.2024.1391601
Edited by:
Emmanouil Apostolidis, Framingham State University, United StatesReviewed by:
Kunming Qin, Jiangsu Ocean University, ChinaSercan Karav, Çanakkale Onsekiz Mart University, Türkiye
Copyright © 2024 Zhang, Guan, Zhu, Wang, Gao, Li, Yan, Ji, Zhou, Yao and Li. This is an open-access article distributed under the terms of the Creative Commons Attribution License (CC BY). The use, distribution or reproduction in other forums is permitted, provided the original author(s) and the copyright owner(s) are credited and that the original publication in this journal is cited, in accordance with accepted academic practice. No use, distribution or reproduction is permitted which does not comply with these terms.
*Correspondence: Lijun Guan, cXFhaXBwaGhAc2luYS5jb20=