- 1College of Physical Education and Health Science, Chongqing Normal University, Chongqing, China
- 2College of Physical Education and Health Management, Chongqing University of Education, Chongqing, China
- 3College of Sports and Health, Chengdu University of Traditional Chinese Medicine, Chengdu, China
- 4Sports Coaching College, Beijing Sport University, Beijing, China
- 5China Institute of Sport and Health Science, Beijing Sport University, Beijing, China
- 6Hebrew SeniorLife Hinda and Arthur Marcus Institute for Aging Research, Harvard Medical School, Boston, MA, United States
Background: Physical exertion during exercise often leads to increased oxidative stress and inflammatory responses, significantly affecting physical performance. Current strategies to mitigate these effects are limited by their effectiveness and potential side effects. Molecular hydrogen (H₂) has gained attention for its antioxidant and anti-inflammatory properties. Studies have suggested that H2 supplementation contributes to antioxidant potential and anti-fatigue during exercise, but the variance in the observations and study protocols is presented across those studies.
Objective: This systematic review and meta-analysis aimed to comprehensively characterize the effects of H₂ supplementation on physical performance (i.e., endurance, muscular strength, and explosive power), providing knowledge that can inform strategies using H2 for enhancing physical performance.
Methods: We conducted a literature search of six databases (PubMed, Web of Science, Medline, Sport-Discus, Embase, and PsycINFO) according to the PRISMA guidelines. The data were extracted from the included studies and converted into the standardized mean difference (SMD). After that, we performed random-effects meta-analyses and used the I2 statistic to evaluate heterogeneity. The Grading of Recommendations Assessment, Development, and Evaluation (GRADE) was used to assess the quality of the evidence obtained from this meta-analysis.
Results: In total, 27 publications consisting of 597 participants were included. The search finally included aerobic endurance, anaerobic endurance, muscular strength, lower limb explosive power, rating of perceived exertion (RPE), blood lactate (BLA), and average heart rate (HRavg) in the effect size (ES) synthesis. The ES of H2 on aerobic endurance, including V̇O2max (SMD = 0.09, p = 0.394; I2 = 0%) and aerobic endurance exercise (SMD = 0.04, p = 0.687; I2 = 0%), were not significant and trivial; the ES of H2 on 30 s maximal anaerobic endurance (SMD = 0.19, p = 0.239; I2 = 0%) was not significant and trivial; the ES of H2 on muscular strength (SMD = 0.19, p = 0.265; I2 = 0%) was not significant and trivial; but the ES of H2 on lower limb explosive power (SMD = 0.30, p = 0.018; I2 = 0%) was significant and small. In addition, H2 reduces RPE (SMD = −0.37, p = 0.009; I2 = 58.0%) and BLA (SMD = −0.37, p = 0.001; I2 = 22.0%) during exercise, but not HRavg (SMD = −0.27, p = 0.094; I2 = 0%).
Conclusion: These findings suggest that H2 supplementation is favorable in healthy adults to improve lower limb explosive power, alleviate fatigue, and boost BLA clearance, but may not be effectively improving aerobic and anaerobic endurance and muscular strength. Future studies with more rigorous designs are thus needed to examine and confirm the effects of H2 on these important functionalities in humans.
Systematic review registration: http://www.crd.york.ac.uk/PROSPERO.
1 Introduction
Physical performance, including endurance, muscle strength, and explosive power, is the cornerstone of achievement in sports for non-athletic populations or athletes (1, 2). It not only contributes to improving athletes’ competitive performance on the field but also provides motivation for healthy adults to participate in sports (3–5). Oxidative stress occurs when the oxygen metabolism is produced and accumulated, eventually going beyond oxidation-resist ability (6, 7). Studies have shown that physical activity of various intensities alters the levels of various oxidative biomarkers (8, 9). However, physical exercise, especially with moderate- to high-intensity exertion, could lead to excessive oxidative stress, which may negatively impact redox homeostasis, worsen fatigue, and ultimately reduce physical performance (10–13). Therefore, efforts have been put into exploring potential antioxidant approaches, which can thus help develop appropriate strategies to enhance physical performance (13–15).
Molecular hydrogen (H2) is a promising antioxidant that selectively reduces hydroxyl radicals (·OH) and peroxynitrite (ONOO-) in cells without leading to a reduction of other reactive substances such as superoxide (O2-), hydrogen peroxide (H2O2), and nitric oxide (NO) (16–18). Studies have shown that H2 molecular, which can be delivered via different forms (i.e., H2 gas and water, and intravenous H2-saline), can penetrate cell membranes and diffuse rapidly into organelles (e.g., mitochondria) (19), thus enhancing mitochondria functional performance (e.g., respiration and enzyme activity) and promoting ATP production or lactate oxidation (20, 21). More recently, human studies have emerged to explore the potential benefits of using H2 for physical performance in healthy adults and showed great promise of the H2-based intervention to improve physical performance (22–25). However, the observations and protocol design across these studies on the effects of H2 on physical performance were inconsistent. For example, some studies have observed that H2-rich water (HRW) supplementation before exercise could effectively increase maximal oxygen uptake (V̇O2max), anaerobic endurance, muscle strength, and lower limb explosive power in healthy adults (26–28), but other studies have shown contradictory findings (29–31). These inconsistencies may arise from the variance in participant characteristics, the protocol of H2 administration, and types of exercise across studies. Only one previous review by Kawamura et al. (32) summarized the observations from only six studies and suggested that the validity of the observations from that literature should be examined and confirmed due to the very small number of included studies. Since then, many new studies have been performed to examine the effects of H2 on endurance, muscle strength, and explosive power (24–26, 33, 34). Therefore, it is urgently demanded to more comprehensively characterize and explicitly examine the effects of H2 on physical performance in healthy adults by summarizing the results of the most up-to-date publications.
We have thus conducted a systematic review and meta-analysis based on the available peer-reviewed publications. Only studies with randomized controlled or crossover designs are included, and several subgroup analyses are performed with the goal of providing critical knowledge of the appropriate design of H2-based intervention design for the improvement of physical performance.
2 Methods
This systematic review and meta-analysis were performed according to the Preferred Reporting Items for Systematic Reviews and Meta-Analysis guideline (35) and registered with PROSPERO (ID CRD42022351559).
2.1 Data sources and search strategies
Two authors (K.Z. and Z.S.) independently searched PubMed, Web of Science, Medline, Sport-Discus, Embase, and PsycINFO databases from inception to 10 May 2024. The keywords of the search were as follows: “molecular hydrogen,” “hydrogen rich water,” “hydrogen-rich water,” “hydrogen rich saline,” “hydrogen-rich saline,” “hydrogen gas,” “hydrogen inhalation,” “hydrogen bathing,” “hydrogen-rich calcium powder,” “physical performance,” “athletic performance,” “exercise performance,” “physical exercise,” “aerobic performance,” “aerobic capacity,” “anaerobic performance,” “intermittent exercise,” “sprint,” “strength training,” and “resistance training” (The detailed search strategy is shown in Supplementary Table S1). In addition, a manual search was performed based on the reference lists of selected articles. The search was limited to English only, and no date restrictions were applied.
2.2 Selection criteria
To be included in this systematic review, previous studies must meet the following eligibility criteria in accordance with PICOS.
1. Participants: the participants were healthy adults with a mean age of ≥18 years and were free from any dietary supplements or medications while the experiment lasted;
2. Intervention: the intervention was the supplementation of H2 by the participants. The source of H2 was not limited;
3. Comparator/Control: the control group used placebos that were identical in appearance, texture, and flavor to H2 products (e.g., drinking water, air, and capsules);
4. Outcomes: the outcomes include at least one of the measures related to physical performance (e.g., aerobic and anaerobic endurance, muscular strength, lower limb explosive power, subjective fatigue, blood lactate (BLA), and heart rate);
5. Study design: the design of the study was a randomized crossover or randomized controlled trial.
Articles were excluded if they fulfilled the following criteria: 1) animal trials; 2) written in a language other than English or unable to obtain outcome data; 3) review papers and conference articles; and 4) repeated publications.
2.3 Data extraction and outcomes
According to the Cochrane Collaboration Handbook, the data extraction process was conducted independently by two authors (C.Y. and Z.S.) (36). The extracted information from the publications included the following: the study (authors and year), sample size, participants (age, height, weight, sex, and training status), methods of H2 administration, exercise protocol, and outcome measures. Any outcome measures on which the two authors disagreed were discussed with the other two authors (J.Z. and D.B.) until a consensus was achieved.
The mean and standard deviation of each outcome in post-tests were extracted for each included study. If the post-test values were not available, they were calculated using the following formulas, where the correlation coefficient (Corr) was set at 0.5 (36, 37).
If relevant data were missing, we emailed the corresponding author or other authors to request it (36). We extracted relevant data using WebPlotDigitizer (version 4.6) for studies when the data could not be obtained by contacting the authors (38).
Based on the included studies, aerobic endurance, anaerobic endurance, muscular strength, and lower limb explosive power performance were ultimately incorporated into the data synthesis.
The primary outcome of aerobic endurance performance was maximum oxygen uptake (V̇O2max) during an incremental load exercise test or peak oxygen uptake (V̇O2peak) when V̇O2max was not available (39, 40). The secondary outcome of aerobic endurance performance was aerobic endurance exercise performance, for example, time-to-exhaustion (TTE) or power during incremental load exercise test or fixed-load submaximal test; the time or speed in time trial test (TT).
The primary outcome of anaerobic endurance performance was power output during the 30 s maximal anaerobic test.
The primary outcome of muscle strength was peak torque or force in the maximal voluntary isometric strength test (MVIS) or maximal isokinetic strength test performed pre- or post-high-intensity exercise.
The primary outcome of lower limb explosive power was countermovement jump (CMJ) height, time of short sprint, or peak power output during 10 s maximal effort exercises.
The exploratory outcomes were the rating of perceived exertion (RPE), BLA, and average heart rate (HRavg) during physical performance. The RPE, BLA, and HRavg are widely used and are important metrics to characterize subjective fatigue, intensity, and physiologic stress that are closely associated with physical performance (41–43). By exploring the effects of H2 on them, it will help more comprehensively characterize the effects of H2 supplementation on physical performance.
2.4 Quality assessment
Two authors independently evaluated the risk of bias in included studies using the Cochrane Collaboration’s tool (44), which contains six items: 1) selection bias; 2) performance bias; 3) detection bias; 4) attrition bias; 5) reporting bias; and 6) other bias. Each item is categorized into three levels: low-risk bias (green), unclear risk bias (yellow), and high-risk bias (red). Studies were defined as having a high-risk bias if ≥1 item had a high-risk bias. The risk of bias is low if all items are assessed as low risk of bias. Others were assessed as moderate risk of bias. Additionally, the quality of evidence for outcomes was evaluated using the Grading of Recommendations Assessment, Development, and Evaluation (GRADE) (45, 46). The quality of the GRADE evidence was graded as high, moderate, low, and very low based on the quality of study design, quality of implementation, uncertainty of results, and consistency of results (45).
2.5 Statistical analysis
Standardized mean difference (SMD) with 95% confidence interval (CI) was used to assess the effect size (ES). ES was classified as trivial (< 0.2), small (0.2 ~ 0.49), moderate (0.5 ~ 0.79), or large (> 0.8) (47). Meta-analysis was performed in Stata v15.1 (STATA Corp., College Station, TX) using the inverse-variance method. The I2 statistic was used to evaluate heterogeneity among the trials with the following criteria: trivial (< 25%), low (25 ~ 50%), moderate (50 ~ 75%), and high (> 75%) (48). A random-effects model was used to estimate pooled effects, as heterogeneity was anticipated across studies due to differences in participants and interventions. Subgroup analysis was used to explore potential sources of heterogeneity (49). The Funnel plots and Egger tests were used to evaluate publication bias. If potential publication bias was detected, we used the trim and fill method for the sensitivity analysis of the results (50). All the statistical significance was set at a p-value of <0.05.
3 Results
3.1 Study selection
The screening procedure of the included studies is shown in Figure 1. A total of 401 potentially relevant publications were retrieved (PubMed n = 77, SPORT-Discus n = 65, Medline n = 71, Web of Science n = 89, PsycINFO n = 5, and Embase = 94). Based on the criteria above, 248 publications were discharged after reviewing the titles and abstracts. After evaluating the full texts, 27 publications (29 studies) were included in the systematic review. Finally, 25 publications consisting of 27 studies (23 randomized crossover designs and 4 randomized controlled trials) were included in the quantitative synthesis (Table 1). One study (28) included two randomized controlled trials, and the other study (23) included a randomized crossover trial and a randomized controlled trial.
3.2 Characteristics of included studies
3.2.1 Participant characteristics
A total of 597 participants, with mean ages ranging from 17.5 to 51.5 years, were included. The training status of these participants was classified according to the included studies as untrained (n = 224) and trained (n = 373), with 215 of them being well-trained athletes (e.g., professional soccer players and elite runners).
3.2.2 Methods of H2 administration
A gold standard regimen for H2 application does not appear to exist. The included studies implemented four sources of H2, that is, drinking HRW (n = 18) (22–26, 28–30, 33, 34, 51–53, 55, 56, 58, 63, 65), HRW bathing (n = 2) (54, 59), inhalation of H2-rich gas (HRG) (n = 5) (27, 57, 60, 62, 64), and oral ingestion of H2-rich calcium (HRC) powder (n = 2) (31, 61). H2 concentrations were found to be highly variable (e.g., HRW:0.5 ~ 5.9 ppm; HRG:1 to 68%) among the various products examined. Nine studies did not report the concentration of H2 (22, 24, 27, 31, 53–55, 57, 59). Single (n = 9) or multiple doses (ranging from 3 to 4 doses) of H2 supplementation prior to exercise is a common intervention protocol. In total, 14 studies examined the effects of H2 intake within 24 h before exercise (23, 25, 28, 29, 33, 51, 53, 54, 56, 58, 61–64). Nine studies implemented the protocol of repeated intake of H2 from 2 to 14 days before exercise (22–24, 26, 30, 31, 52, 55, 57). One study (60) used 30 min inhalation of HRG during exercise. Another study (65) examined the effects of multiple doses of H2 supplementation before and during exercise. Two studies examined the effects of a single intake of H2 after exercise (27, 59). One study (34) used 210 mL at 30 min and 1 min before exercise, 210 mL during mid-exercise, another 210 mL immediately after exercise, and 420 mL of HRW 30 min after recovery. The physicochemical properties of HRW, HRW bathing, HRG, and HRC are shown in Table 1. Placebos were identical in appearance, texture, and taste to H2 products, such as drinking water, air, and capsules.
3.2.3 Exercise protocol and outcome measurements
The included studies highlighted the effects of H2 supplementation on aerobic endurance, anaerobic endurance, muscular strength, and lower extremity explosive strength in participants. In these studies, continuous incremental load and fixed-load subliminal exercise were the most commonly used aerobic endurance intervention or testing protocols. V̇O2max, V̇O2peak, TTE, race time, and power were metrics used to measure aerobic endurance performance (24, 26, 28, 29, 33, 55, 57, 61, 63). The 30 s maximal anaerobic power test (i.e., pedaling bicycle or rowing dynamometer) was used to assess the anaerobic endurance (i.e., mean or maximal power) (24, 26, 27). One study (57) used the MVIS to assess the force of knee extension prior to high-intensity aerobic exercise; four studies (27, 30, 51, 62) were conducted to evaluate the magnitude of knee extensor force or peak torque in the MVIC after vigorous exercise. Eight studies (25, 27, 30, 34, 61, 63–65) evaluated alterations in lower limb explosive power (i.e., CMJ height and peak power output during 10 s or 30 m sprint) during or after vigorous exercise in participants. One study (53) used the special fitness test to assess the effects of HRW intake on athletic performance in judo athletes. Additionally, the included studies focused on assessing the effects of H2 administration on various physiological parameters during exercise, such as RPE, BLA, HR, pH, respiratory function, antioxidant levels, muscle oxygenation, and endocrine system. The outcomes of each study are summarized in Table 1.
3.3 Quality assessment
The risk of bias in the 27 publications (29 studies) was assessed, and a consensus was reached after discussion. The overall result is shown in Figure 2. Two studies (23, 28) did not adequately report on participant randomization and concealment methods. Five studies (23, 24, 30, 55, 60) did not adequately describe participant, staff, or evaluator blinding. No studies had incomplete results due to participants’ withdrawal. All studies reported experimental procedures and conducted the experiments as planned. According to the possibility of bias, the study was assessed as being low risk, moderate risk, or high risk. One study (23) was evaluated as having a high-risk bias, five studies (24, 28, 30, 55, 60) had a moderate risk bias, and others were assessed as having a low-risk bias. The quality of evidence for outcomes was evaluated as moderate to high, and details for the evaluation of the GRADE framework are presented in Supplementary Table S2.
3.4 Meta-analysis
A subgroup analysis was performed on aerobic endurance, anaerobic endurance, muscle strength, lower limb explosive power, RPE, and BLA, considering potential sources of heterogeneity, including exercise types and H2 sources. Additionally, we used a subgroup analysis to explore the effects of H2 supplementation on muscle performance before or after vigorous exercise (Table 2).
3.4.1 Effects of H2 on aerobic endurance
3.4.1.1 V̇O2max (V̇O2peak)
Three studies (23, 26, 28) showed that H2 can significantly improve V̇O2max or V̇O2peak as compared to the placebo; while another five publications (six studies) (22, 28, 29, 31, 57) showed opposite results: H2 cannot significantly improve V̇O2max or V̇O2peak (Table 1).
The pooled ES of V̇O2max and V̇O2peak was not significant and trivial (SMD = 0.09, 95% CI −0.11 to 0.28, p = 0.394, Figure 3) and without heterogeneity (I2 = 0%, p = 0.996). The funnel plot (Supplementary Figure S1A) and Egger’s test (t = −0.30, p = 0.776) indicated that there was no publication bias. Subgroup analyses showed non-significant trivial ESs for HRG (SMD = -0.06, 95% CI −0.68 to 0.56, p = 0.861), HRC (SMD = −0.04, 95% CI −0.70 to 0.61, p = 0.895), and HRW (SMD = 0.12, 95% CI −0.10 to 0.34, p = 0.290) on V̇O2max (V̇O2peak).
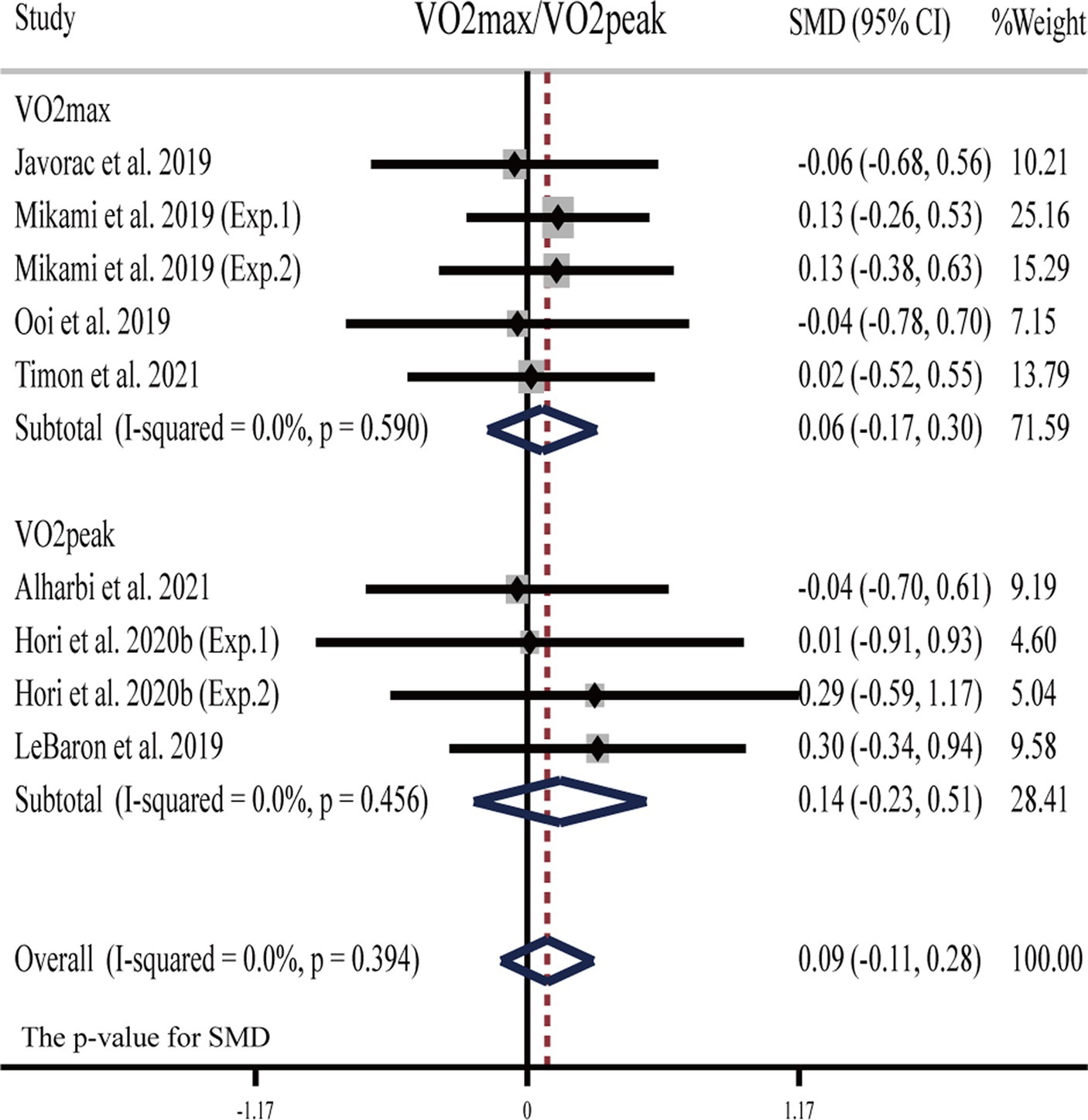
Figure 3. Forest plot of the effects of H2 supplementation on V̇O2max/V̇O2peak. Exp.1, Experiment 1; Exp.2, Experiment 2.
3.4.1.2 Aerobic endurance exercise performance
Two studies (26, 63) showed that H2 can significantly improve aerobic exercise performance as compared to the placebo, while another eight publications (nine studies) (23, 24, 29, 31, 33, 54, 57, 58) showed that H2 cannot (Table 1).
The pooled ES of aerobic exercise performance was not significant and trivial (SMD = 0.04, 95% CI -0.17 to 0.25, p = 0.687, Figure 4) and without heterogeneity (I2 = 0%, p = 0.991). The funnel plot (Supplementary Figure S1B) and Egger’s test (t = 0.75, p = 0.474) indicated that there was no publication bias on these results. Subgroup analyses showed non-significant trivial ESs for HRG (SMD = 0.002, 95% CI −0.618 to −0.622, p = 0.994), HRC (SMD = −0.02, 95% CI −0.68 to 0.63, p = 0.941), and HRW (SMD = 0.06, 95% CI −0.18 to 0.29, p = 0.632) on aerobic exercise performance.
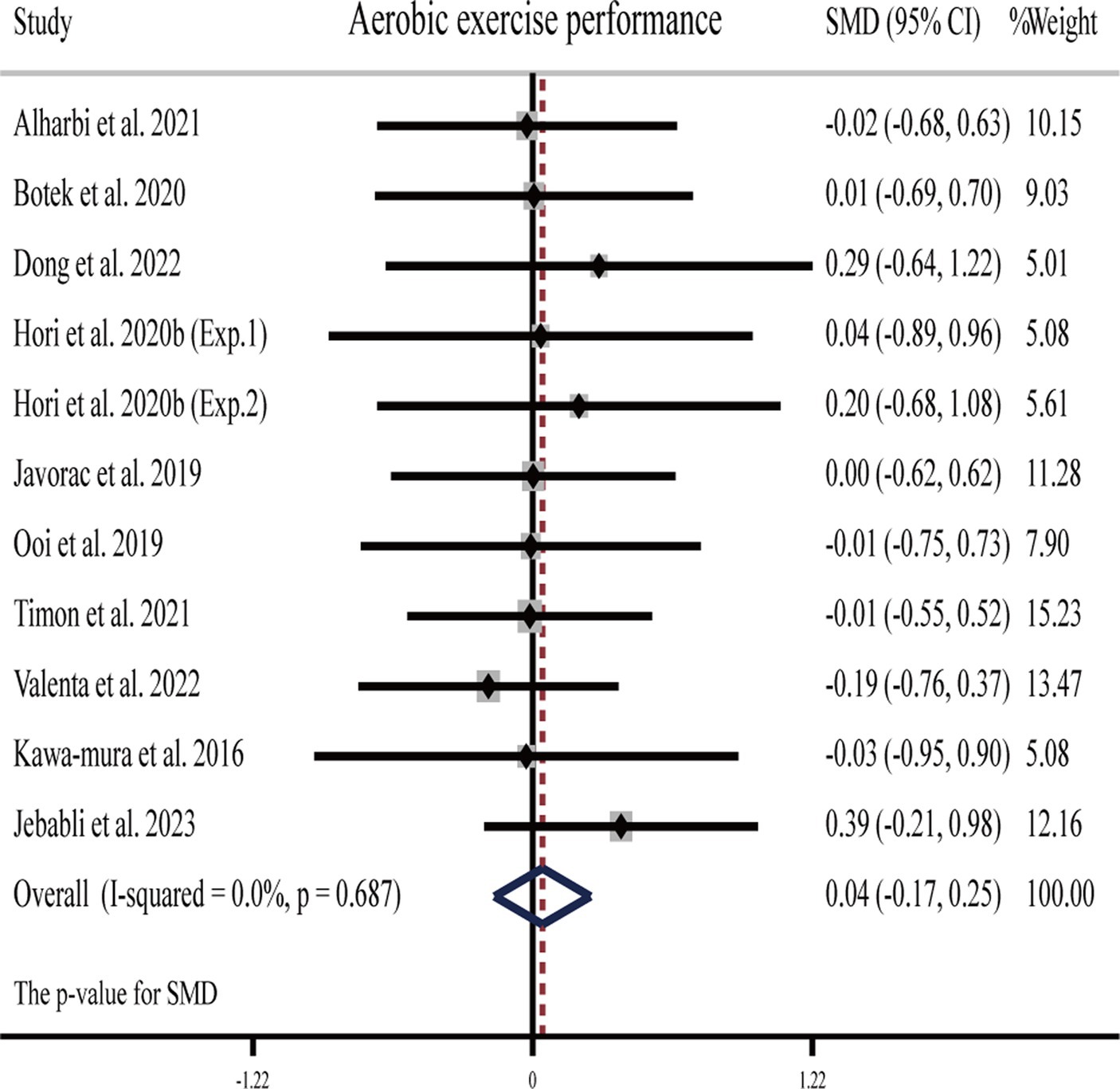
Figure 4. Forest plot of the effects of H2 supplementation on aerobic exercise performance. Exp.1, Experiment 1; Exp.2, Experiment 2.
3.4.2 Effects of H2 on anaerobic endurance
One study (26) showed that H2 can significantly improve mean and peak power output during the 30 s maximal anaerobic test as compared to the placebo. One study (24) showed that H2 can significantly improve peak power output in a 30 s maximal anaerobic test compared to placebo but cannot significantly improve mean power, while another study (27) showed the opposite result that H2 cannot significantly improve mean power output during the 30 s maximal anaerobic test (Table 1).
The pooled ES of anaerobic exercise performance was not significant and close to small (SMD = 0.19, 95% CI −0.12 to 0.50, p = 0.239, Figure 5) with low heterogeneity (I2 = 0%, p = 0.929). The funnel plot (Supplementary Figure S1C) and Egger’s test (t = 0.58, p = 0.586) indicated that there was no publication bias. With regard to the source of H2, the ES was trivial for HRG (SMD = −0.09, 95% CI −1.07 to 0.89, p = 0.853), while it was small (SMD = 0.22, 95% CI −0.11 to 0.55, p = 0.192) for HRW.
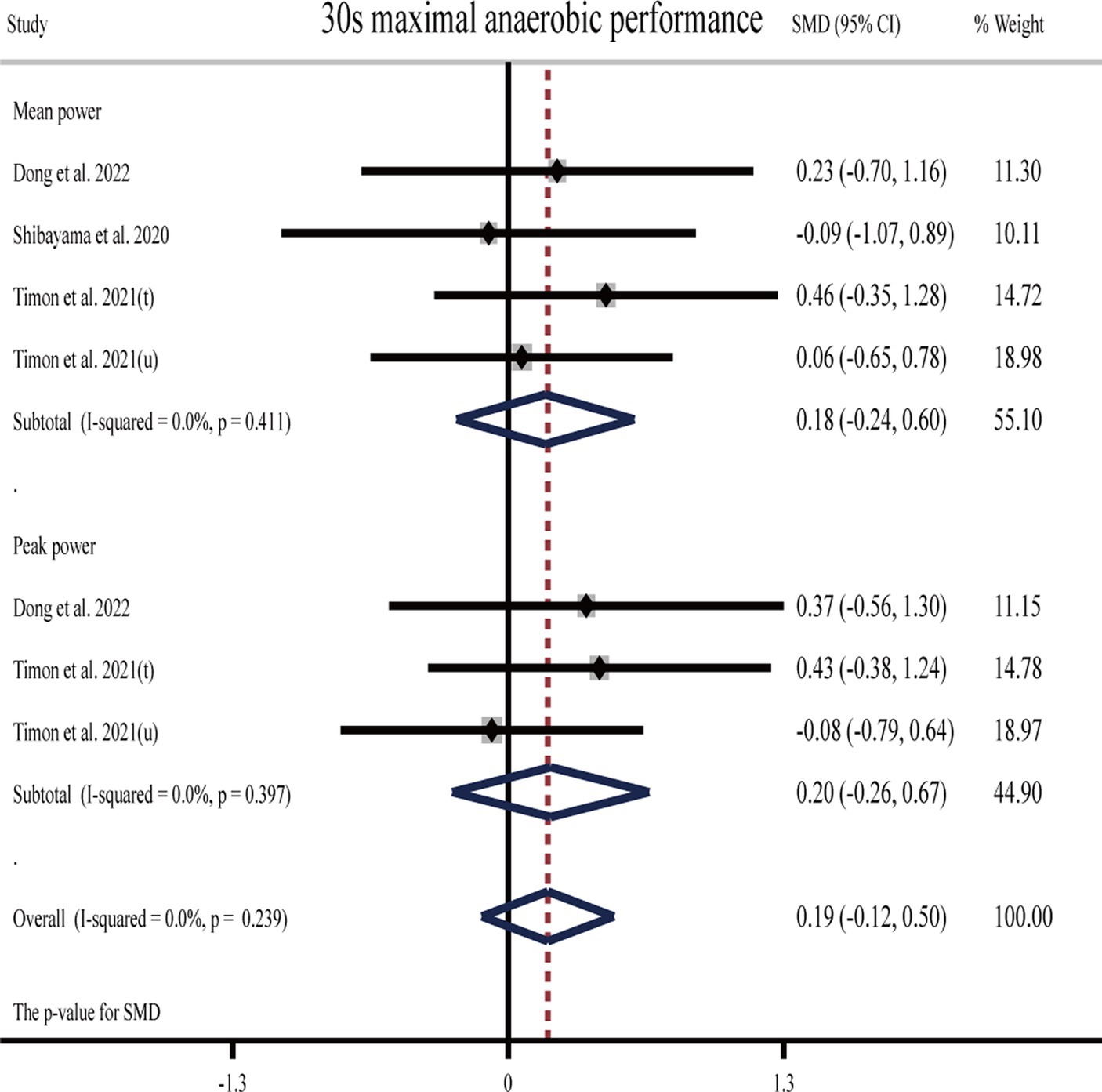
Figure 5. Forest plot of the effects of H2 supplementation on anaerobic exercise performance. t, trained participants; u, untrained participants.
3.4.3 Effects of H2 on muscle strength
Five studies (27, 30, 51, 57, 62) showed that H2 cannot significantly improve maximum strength compared to the placebo (Table 1).
The pooled ES of muscle strength was not significant and close to small (SMD = 0.19, 95% CI -0.14 to 0.52, p = 0.265, Figure 6) and with low heterogeneity (I2 = 0%, p = 0.770). The funnel plot (Supplementary Figure S1D) and Egger’s test (t = 2.67, p = 0.076) indicated no publication bias.
Subgroup analyses showed that the ES was trivial (SMD = 0.10, 95% CI −0.52 to 0.72, p = 0.741) for muscle strength assessed before vigorous exercise, and it was small (SMD = 0.22, 95% CI −0.17 to 0.62, p = 0.266) for H2 on muscle strength assessed after vigorous exercise. With regards to the source of H2, the ES was not significantly trivial for HRG (SMD = 0.13, 95% CI −0.26 to 0.51, p = 0.520), while it was small (SMD = 0.38, 95% CI −0.29 to 1.05, p = 0.265) for HRW.
3.4.4 Effects of H2 on lower limb explosive power
Three studies (25, 61, 65) showed that H2 can significantly improve lower limb explosive power as compared to the placebo, while another five studies (27, 30, 34, 63, 64) showed opposite results that H2 cannot improve lower limb explosive power (Table 1).
The pooled ES of lower limb explosive power was significant and small (SMD = 0.30, 95% CI 0.05 to 0.55, p = 0.018, Figure 7) and without heterogeneity (I2 = 0%, p = 0.949). The funnel plot (Supplementary Figure S1E) and Egger’s test (t = 0.49, p = 0.636) indicated no publication bias. Subgroup analyses showed that the ES of HRG on lower limb explosive power was significant and moderate (SMD = 0.52, 95% CI 0.07 to 0.97, p = 0.023), while HRC was not significant and small (SMD = 0.20, 95% CI −0.68 to 1.08, p = 0.655), and HRW was not significant and small (SMD = 0.20, 95% CI −0.11 to 0.52, p = 0.206).
3.4.5 Effects of H2 on the exploratory outcomes
3.4.5.1 RPE
Four studies (28, 56, 62, 64) showed that H2 can significantly reduce RPE score as compared to the placebo, while another eight publications (nine studies) (23, 25, 26, 29, 34, 55, 58, 63) showed that H2 cannot significantly reduce RPE score (Table 1). The pooled ES of the RPE score was small and significant (SMD = −0.37, 95% CI −0.65 to −0.09, p = 0.009, Supplementary Figure S2), with moderate heterogeneity (I2 = 58.0%, p = 0.005). The funnel plot (Supplementary Figure S1F) and Egger’s test (t = −0.06, p = 0.955) indicated no publication bias.
The results of subgroup analyses revealed that strength training and repeated sprints yielded significant and large ESs (SMD = −1.41, 95% CI −2.32 to −0.50, p = 0.002 and SMD = −0.96, 95% CI −1.70 to −0.22, p = 0.011, respectively), while aerobic endurance exercise produced a significant and small ES (SMD = −0.33, 95% CI −0.59 to −0.07, p = 0.013). Conversely, anaerobic endurance exercise elicited small and non-significant ES (SMD = 0.29, 95% CI −0.25 to 0.83, p = 0.290). With regards to the source of H2, the ES was significantly large for HRG (SMD = −0.91, 95% CI −1.51 to −0.31, p = 0.003), while it was relatively small (SMD = −0.32, 95% CI −0.60 to −0.03, p = 0.029) for HRW.
3.4.5.2 BLA
Five studies (34, 51, 53, 56, 64) showed that H2 can significantly improve BLA as compared to the placebo, while another eight publications (nine studies) (23, 25, 26, 29–31, 33, 55) showed opposite results that H2 cannot significantly improve BLA (Table 1). The pooled ES of BLA was small and significant (SMD = −0.37, 95% CI −0.60 to −0.15, p = 0.001, Supplementary Figure S3), with low heterogeneity (I2 = 22.0%, p = 0.215). The funnel plot (Supplementary Figure S1G) and Egger’s test (t = −3.44, p = 0.005) indicated that there was a potential risk of publication bias on these results, but the trim and fill method for sensitive analysis showed that the pooled ES (fixed: SMD = −0.349, p < 0.001; Random: SMD = −0.375, p = 0.001) was robust after filled meta-analysis.
The results of subgroup analyses revealed that aerobic endurance exercise yielded a significant and small ES (SMD = −0.38, 95% CI −0.67 to −0.08, p = 0.013), while anaerobic endurance exercise produced a non-significant and small ES (SMD = −0.67, 95% CI −1.72 to 0.38, p = 0.213); strength training elicited moderate and non-significant ES (SMD = −0.53, 95% CI −1.34 to 0.29, p = 0.206). Repeated sprints yielded a non-significant and small ES (SMD = −0.20, 95% CI −0.77 to 0.37, p = 0.496). With regards to the source of H2, the ES was significantly small for HRW (SMD = −0.42, 95% CI −0.68 to −0.15, p = 0.002), and the ES of HRG was small and not significant (SMD = −0.47, 95% CI −1.04 to 0.11, p = 0.111), while it was trivial (SMD = 0.00, 95% CI −0.65 to 0.65, p = 0.999) for HRC.
3.4.5.3 HRavg
Two studies (22, 62) showed that H2 can significantly improve HRavg during exercise as compared to the placebo, while another three studies (54, 55, 58) showed the opposite result that H2 cannot significantly improve HRavg (Table 1). The pooled ES of HRavg was not significant and small (SMD = −0.27, 95% CI −0.60 to 0.05, p = 0.094, Supplementary Figure S4) and without heterogeneity (I2 = 0%, p = 0.557). The funnel plot (Supplementary Figure S1H) and Egger’s test (t = 1.26, p = 0.296) indicated that there was no publication bias.
4 Discussion
To our knowledge, this is the first systematic review and meta-analysis exploring the effects of H2 supplementation on physical performance in healthy adults. The results suggest that H2 supplementation is promising for improving lower limb explosive power and reducing RPE and BLA clearance during vigorous exercise. However, it does not enhance endurance performance and muscle strength or decrease HRavg.
This meta-analysis suggests that administering H2 before or after exercise may serve as a potential strategy to effectively enhance lower limb explosive power in healthy adults. One potential mechanism underlying the effects of H2 on explosive power is that H2 can directly react with strong oxidants in vivo [e.g., hydroxyl radicals (•OH)] to modulate Ca2+ or mitochondrial ATP-dependent K+ channels, thus facilitating mitochondrial ATP production (20, 66–69). Additionally, H2 could reduce intracellular reactive oxygen species (ROS) levels and thus enhance muscle contractile function (27, 70). For example, a study conducted on soccer players demonstrated that administering three successive doses of 500 mL of HRW prior to high-intensity aerobic exercise increased the mean power frequency of skeletal muscles during subsequent strength tests (51). However, this finding that H2 promotes lower limb explosive power may be influenced by a small sample size (n = 92) or movement pattern. One example is that H2 significantly improved participants’ sprint performance compared to their vertical jump performance (25, 63). Therefore, more research is still needed to confirm this finding in the future. The result showed that H2 did not significantly improve muscle strength after aerobic endurance exercise. One possible reason is that intense aerobic exercise leads to a consumption of H2 in the body that does not continually provide benefits for subsequent muscle strength performance. One study (34) shows that 1,260 mL of HRW intake can increase the movement velocity of multiple lunges during resistance training. Therefore, more studies are needed in the future to clarify the effects of H2 supplementation on muscular strength performance in isolated resistance training. It has been observed that H2 supplementation cannot significantly improve aerobic and anaerobic endurance performance. Endurance performance depends on the multiple factors of human respiratory function, oxygen transport, and local muscle oxygen utilization during exercise (71, 72). Studies have shown that using H2 failed to significantly improve these critical factors (e.g., V̇O2max and running economy) of endurance performance (23, 29, 31, 56, 57), thus leading to the insignificant benefits of H2 on this important function.
While H2 supplementation does not appear to enhance endurance performance or increase muscle strength, it does demonstrate favorable effects in reducing RPE, BLA levels, and HRavg among individuals engaged in high-intensity exercise. H2 appears to be a neuroprotective agent that facilitates the restoration of neuronal oxidative damage by reducing oxidative stress and neuroinflammation (16, 73–75). H2 intake has also been reported to induce positive effects on exercise acidosis (56), thus modulating intracellular and extracellular buffering capacity during vigorous exercise (76). The decrease in BLA during exercise may be attributed to the fact that molecular H2 accelerates the transport of BLA to the liver for storage and oxidation, as well as increasing the utilization of lactate as a fuel by the muscles (56, 77). Subgroup analysis reveals that H2 supplementation reduces BLA concentration in aerobic endurance exercise, which is superior to other exercise types. The reduction in BLA response during aerobic endurance exercise may indicate that H2 supplementation enhances oxidative energy metabolism (28). Indeed, this finding may be unreliable due to the small number of studies on other exercise types. Therefore, future research should focus more on the effects of H2 supplementation on anaerobic endurance, muscular strength, and repeated sprint performance. Subgroup analyses reveal two important factors that likely contribute to the effects of H2 supplementation on RPE. First, we observed that the effects were greater in strength training and repeated sprints as compared to endurance exercise. The observed variations in RPE could be attributed to the disparities in the energy supply mechanisms across different types of exercises. It is plausible that H2 gas may exhibit a higher affinity toward the phosphagen system when compared to the oxidative and glycolytic systems (66). Second, inhalation of H2 gas (HRG) is superior to the ingestion of HRW in mitigating RPE. The observed discrepancy can be attributed to the fact that the respiratory absorption of molecular H2 is significantly more efficient and comprehensive in comparison to its digestive absorption in HRW. Nonetheless, given the restricted sample size, it is imperative to ensure further validation of the outcomes of the subgroup analysis.
5 Limitations
Five included studies with a small number of participants (n ≤ 10) (23, 27, 30, 52, 53) may lead to potential bias. Most studies to date focus on only younger and middle-aged men, and future studies are highly demanded to examine the benefits of H2 for women and those with older age. The current studies only investigated the effects of H2 supplementation for 1–14 days and future studies need to focus on the effects of longer supplementation periods. Some studies did not report or detect H2 concentrations, and the H2 dosing regimen was highly variable. The dose–response relationship between H2 and physical performance has not been established, which should be explored in the future to determine the most appropriate dosage and intervention protocol for H2 for enhancing physical performance.
6 Conclusion
In summary, this systematic review and meta-analysis suggest that short-term (<14 days) H2 supplementation protocols contribute to improved lower limb explosive power, fatigue relief, and BLA clearance but may not significantly improve aerobic endurance, anaerobic endurance, or muscular strength. Inhaling H2 shows promise as the optimal method for improving physical performance (i.e., lower limb explosive power) in healthy adults. Future studies with rigorous designs are needed to help obtain more definitive conclusions on the effects of H2 on lower limb explosive power and muscle strength in healthy adults.
Data availability statement
The raw data supporting the conclusions of this article will be made available by the authors, without undue reservation.
Author contributions
KZ: Data curation, Formal analysis, Methodology, Project administration, Software, Supervision, Writing – original draft, Writing – review & editing. ZS: Data curation, Formal analysis, Methodology, Software, Writing – original draft, Writing – review & editing. CY: Data curation, Formal analysis, Methodology, Writing – original draft. ZG: Conceptualization, Writing – original draft. YW: Formal analysis, Methodology, Software, Writing – original draft. DB: Conceptualization, Data curation, Formal analysis, Funding acquisition, Methodology, Resources, Supervision, Writing – original draft, Writing – review & editing. JZ: Conceptualization, Formal analysis, Methodology, Supervision, Writing – review & editing.
Funding
The author(s) declare financial support was received for the research, authorship, and/or publication of this article. DB was supported by the Key Research and Development Projects of the Ministry of Science and Technology (2018YFC2000602).
Conflict of interest
The authors declare that the research was conducted in the absence of any commercial or financial relationships that could be construed as a potential conflict of interest.
The author(s) declared that they were an editorial board member of Frontiers, at the time of submission. This had no impact on the peer review process and the final decision.
Publisher’s note
All claims expressed in this article are solely those of the authors and do not necessarily represent those of their affiliated organizations, or those of the publisher, the editors and the reviewers. Any product that may be evaluated in this article, or claim that may be made by its manufacturer, is not guaranteed or endorsed by the publisher.
Supplementary material
The Supplementary material for this article can be found online at: https://www.frontiersin.org/articles/10.3389/fnut.2024.1387657/full#supplementary-material
References
1. Thiele, D, Prieske, O, Chaabene, H, and Granacher, U. Effects of strength training on physical fitness and Sport-specific performance in recreational, sub-elite, and elite rowers: A systematic review with Meta-analysis. J Sports Sci. (2020) 38:1186–95. doi: 10.1080/02640414.2020.1745502
2. Pescatello, LS, Arena, R, Riebe, D, and Thompson, PD. Acsm's guidelines for exercise testing and prescription. Physiotherapy. (2006) 77:311. doi: 10.1016/S0031-9406(10)61772-5
3. Viribay, A, Fernández-Landa, J, Castañeda-Babarro, A, Collado, PS, Fernández-Lázaro, D, and Mielgo-Ayuso, J. Effects of Citrulline supplementation on different aerobic exercise performance outcomes: A systematic review and Meta-analysis. Nutrients. (2022) 14:3479. doi: 10.3390/nu14173479
4. Williams, J, Abt, G, and Kilding, AE. Effects of Creatine monohydrate supplementation on simulated soccer performance. Int J Sports Physiol Perform. (2014) 9:503–10. doi: 10.1123/ijspp.2013-0407
5. Medrano-Ureña, MDR, Ortega-Ruiz, R, and Benítez-Sillero, JD. Physical fitness, exercise self-efficacy, and quality of life in adulthood: A systematic review. Int J Env Res Public Health. (2020) 17:6343. doi: 10.3390/ijerph17176343
6. Lu, Z, Xu, Y, Song, Y, Bíró, I, and Gu, Y. A mixed comparisons of different intensities and types of physical exercise in patients with diseases related to oxidative stress: A systematic review and network Meta-analysis. Front Physiol. (2021) 12:700055. doi: 10.3389/fphys.2021.700055
7. Powers, SK, Deminice, R, Ozdemir, M, Yoshihara, T, Bomkamp, MP, and Hyatt, H. Exercise-induced oxidative stress: friend or foe? J Sport Health Sci. (2020) 9:415–25. doi: 10.1016/j.jshs.2020.04.001
8. El Abed, K, Ammar, A, Boukhris, O, Trabelsi, K, Masmoudi, L, Bailey, SJ, et al. Independent and combined effects of all-out Sprint and low-intensity continuous exercise on plasma oxidative stress biomarkers in trained judokas. Front Physiol. (2019) 10:842. doi: 10.3389/fphys.2019.00842
9. Ammar, A, Trabelsi, K, Boukhris, O, Glenn, JM, Bott, N, Masmoudi, L, et al. Effects of aerobic-, anaerobic- and combined-based exercises on plasma oxidative stress biomarkers in healthy untrained young adults. Int J Env Res Public Health. (2020) 17:2601. doi: 10.3390/ijerph17072601
10. Powers, SK, Nelson, WB, and Hudson, MB. Exercise-induced oxidative stress in humans: cause and consequences. Free Radic Biol Med. (2011) 51:942–50. doi: 10.1016/j.freeradbiomed.2010.12.009
11. Huang, WC, Wei, CC, Huang, CC, Chen, WL, and Huang, HY. The beneficial effects of Lactobacillus Plantarum Ps128 on high-intensity, exercise-induced oxidative stress, inflammation, and performance in triathletes. Nutrients. (2019) 11:353. doi: 10.3390/nu11020353
12. Rubio-Arias, J, Ávila-Gandía, V, López-Román, FJ, Soto-Méndez, F, Alcaraz, PE, and Ramos-Campo, DJ. Muscle damage and inflammation biomarkers after two ultra-Endurance Mountain races of different distances: 54 km vs 111 km. Physiol Behav. (2019) 205:51–7. doi: 10.1016/j.physbeh.2018.10.002
13. Pingitore, A, Lima, GP, Mastorci, F, Quinones, A, Iervasi, G, and Vassalle, C. Exercise and oxidative stress: potential effects of antioxidant dietary strategies in sports. Nutrition. (2015) 31:916–22. doi: 10.1016/j.nut.2015.02.005
14. Fernández-Lázaro, D, Fernandez-Lazaro, CI, Mielgo-Ayuso, J, Navascués, LJ, Córdova Martínez, A, and Seco-Calvo, J. The role of selenium mineral trace element in exercise: antioxidant defense system, muscle performance, hormone response, and athletic performance. A systematic review. Nutrients. (2020) 12:1790. doi: 10.3390/nu12061790
15. Arazi, H, Eghbali, E, and Suzuki, K. Creatine supplementation, physical exercise and oxidative stress markers: A review of the mechanisms and effectiveness. Nutrients. (2021) 13:869. doi: 10.3390/nu13030869
16. Iketani, M, and Ohsawa, I. Molecular hydrogen as a neuroprotective agent. Curr Neuropharmacol. (2017) 15:324–31. doi: 10.2174/1570159x14666160607205417
17. Hong, Y, Chen, S, and Zhang, JM. Hydrogen as a selective antioxidant: A review of clinical and experimental studies. J Int Med Res. (2010) 38:1893–903. doi: 10.1177/147323001003800602
18. Ohsawa, I, Ishikawa, M, Takahashi, K, Watanabe, M, Nishimaki, K, Yamagata, K, et al. Hydrogen acts as a therapeutic antioxidant by selectively reducing cytotoxic oxygen radicals. Nat Med. (2007) 13:688–94. doi: 10.1038/nm1577
19. Ohta, S . Molecular hydrogen as a preventive and therapeutic medical gas: initiation, development and potential of hydrogen medicine. Pharmacol Ther. (2014) 144:1–11. doi: 10.1016/j.pharmthera.2014.04.006
20. Gvozdjáková, A, Kucharská, J, Kura, B, Vančová, O, Rausová, Z, Sumbalová, Z, et al. A new insight into the molecular hydrogen effect on coenzyme Q and mitochondrial function of rats. Can J Physiol Pharmacol. (2020) 98:29–34. doi: 10.1139/cjpp-2019-0281
21. Ostojic, SM . Hydrogen gas as an exotic performance-enhancing agent: challenges and opportunities. Curr Pharm Des. (2021) 27:723–30. doi: 10.2174/1381612826666200922155242
22. LeBaron, TW, Larson, AJ, Ohta, S, Mikami, T, Barlow, J, Bulloch, J, et al. Acute supplementation with molecular hydrogen benefits submaximal exercise indices. Randomized, double-blinded, placebo-controlled crossover pilot study. J Lifestyle Med. (2019) 9:36–43. doi: 10.15280/jlm.2019.9.1.36
23. Hori, A, Sobue, S, Kurokawa, R, Hirano, SI, Ichihara, M, and Hotta, N. Two-week continuous supplementation of Hydrogenrich water increases peak oxygen uptake during an incremental cycling exercise test in healthy humans: A randomized, single-blinded, placebo-controlled study. Med Gas Res. (2020) 10:163–9. doi: 10.4103/2045-9912.304223
24. Dong, G, Fu, J, Bao, D, and Zhou, J. Short-term consumption of hydrogen-rich water enhances power performance and heart rate recovery in dragon boat athletes: evidence from a pilot study. Int J Env Res Public Health. (2022) 19:5413. doi: 10.3390/ijerph19095413
25. Botek, M, Khanna, D, Krejčí, J, Valenta, M, McKune, A, Sládečková, B, et al. Molecular hydrogen mitigates performance decrement during repeated sprints in professional soccer players. Nutrients. (2022) 14:508. doi: 10.3390/nu14030508
26. Timon, R, Olcina, G, Gonzalez-Custodio, A, Camacho-Cardenosa, M, Camacho-Cardenosa, A, and Martinez, GI. Effects of 7-day intake of hydrogen-rich water on physical performance of trained and untrained subjects. Biol Sport. (2021) 38:269–75. doi: 10.5114/biolsport.2020.98625
27. Shibayama, Y, Dobashi, S, Arisawa, T, Fukuoka, T, and Koyama, K. Impact of hydrogen-Rich gas mixture inhalation through nasal cannula during post-exercise recovery period on subsequent oxidative stress, muscle damage, and exercise performances in men. Med Gas Res. (2020) 10:155–62. doi: 10.4103/2045-9912.304222
28. Mikami, T, Tano, K, Lee, H, Lee, H, and Ohta, S. Drinking hydrogen water enhances endurance and relieves psychometric fatigue: randomized, double-blind, placebo-controlled study. Can J Physiol Pharmacol. (2019) 97:857–62. doi: 10.1139/cjpp-2019-0059
29. Ooi, CH, Ng, SK, and Omar, EA. Acute ingestion of hydrogen-rich water does not improve incremental treadmill running performance in endurance-trained athletes. Appl Physiol Nutr Metab. (2019) 45:513–9. doi: 10.1139/apnm-2019-0553
30. Dobashi, S, Takeuchi, K, and Koyama, K. Hydrogen-rich water suppresses the reduction in blood Total antioxidant capacity induced by 3 consecutive days of severe exercise in physically active males. Med Gas Res. (2020) 10:21–6. doi: 10.4103/2045-9912.279979
31. Alharbi, AAD, Ebine, N, Nakae, S, Hojo, T, and Fukuoka, Y. Application of molecular hydrogen as an antioxidant in responses to Ventilatory and ergogenic adjustments during incremental exercise in humans. Nutrients. (2021) 13:459. doi: 10.3390/nu13020459
32. Kawamura, T, Higashida, K, and Muraoka, I. Application of molecular hydrogen as a novel antioxidant in sports science. Oxidative Med Cell Longev. (2020) 2020:1–7. doi: 10.1155/2020/2328768
33. Valenta, M, Botek, M, Krejčí, J, McKune, A, Sládečková, B, Neuls, F, et al. Acute pre-exercise hydrogen rich water intake does not improve running performance at maximal aerobic speed in trained track and field runners: A randomized, double-blind, placebo-controlled crossover study. PLoS One. (2022) 17:e0279307. doi: 10.1371/journal.pone.0279307
34. Botek, M, Krejčí, J, McKune, A, Valenta, M, and Sládečková, B. Hydrogen rich water consumption positively affects muscle performance, lactate response, and alleviates delayed onset of muscle soreness after resistance training. J Strength Cond Res. (2021) 36:2792–9. doi: 10.1519/jsc.0000000000003979
35. Page, MJ, McKenzie, JE, Bossuyt, PM, Boutron, I, Hoffmann, TC, Mulrow, CD, et al. The Prisma 2020 statement: an updated guideline for reporting systematic reviews. BMJ. (2021) 372:n71. doi: 10.1136/bmj.n71
36. Higgins, JP, Thomas, J, Chandler, J, Cumpston, M, Li, T, Page, MJ, et al. Cochrane handbook for systematic reviews of interventions. 2rd ed. Hoboken, NJ: John Wiley & Sons (2019).
37. Xue, Y, Yang, Y, and Huang, T. Effects of chronic exercise interventions on executive function among children and adolescents: A systematic review with Meta-analysis. Br J Sports Med. (2019) 53:1397–404. doi: 10.1136/bjsports-2018-099825
38. Petzold, A, Steeb, T, Wessely, A, Koch, EAT, Vera, J, Berking, C, et al. Is Tebentafusp superior to combined immune checkpoint blockade and other systemic treatments in metastatic uveal melanoma? A comparative efficacy analysis with population adjustment. Cancer Treat Rev. (2023) 115:102543. doi: 10.1016/j.ctrv.2023.102543
39. Baumgart, JK, Brurok, B, and Sandbakk, Ø. Comparison of peak oxygen uptake between upper-body exercise modes: A systematic literature review and Meta-analysis. Front Physiol. (2020) 11:412. doi: 10.3389/fphys.2020.00412
40. Wallace, A, Pietrusz, A, Dewar, E, Dudziec, M, Jones, K, Hennis, P, et al. Community exercise is feasible for neuromuscular diseases and can improve aerobic capacity. Neurology. (2019) 92:e1773–85. doi: 10.1212/wnl.0000000000007265
41. Deshayes, TA, Pancrate, T, and Goulet, EDB. Impact of dehydration on perceived exertion during endurance exercise: A systematic review with Meta-analysis. J Exerc Sci Fit. (2022) 20:224–35. doi: 10.1016/j.jesf.2022.03.006
42. Engel, FA, Ackermann, A, Chtourou, H, and Sperlich, B. High-intensity interval training performed by young athletes: A systematic review and Meta-analysis. Front Physiol. (2018) 9:1012. doi: 10.3389/fphys.2018.01012
43. Delleli, S, Ouergui, I, Messaoudi, H, Trabelsi, K, Ammar, A, Glenn, JM, et al. Acute effects of caffeine supplementation on physical performance, physiological responses, perceived exertion, and technical-tactical skills in combat sports: A systematic review and Meta-analysis. Nutrients. (2022) 14:2996. doi: 10.3390/nu14142996
44. Higgins, JP, Altman, DG, Gøtzsche, PC, Jüni, P, Moher, D, Oxman, AD, et al. The Cochrane Collaboration's tool for assessing risk of Bias in randomised trials. BMJ. (2011) 343:d5928. doi: 10.1136/bmj.d5928
45. Balshem, H, Helfand, M, Schünemann, HJ, Oxman, AD, Kunz, R, Brozek, J, et al. Grade guidelines: 3. Rating the quality of evidence. J Clin Epidemiol. (2011) 64:401–6. doi: 10.1016/j.jclinepi.2010.07.015
46. Meader, N, King, K, Llewellyn, A, Norman, G, Brown, J, Rodgers, M, et al. A checklist designed to aid consistency and reproducibility of grade assessments: development and pilot validation. Syst Rev. (2014) 3:82. doi: 10.1186/2046-4053-3-82
47. Cohen, J . Statistical power analysis for the behavioral sciences. Mahwah, NJ: Lawrence Earlbaum Associates (1988).
48. Higgins, JP, Thompson, SG, Deeks, JJ, and Altman, DG. Measuring inconsistency in Meta-analyses. BMJ. (2003) 327:557–60. doi: 10.1136/bmj.327.7414.557
49. Bandeira-Guimaraes, M, Blanco-Rambo, E, Vieira, AF, Saez de Asteasu, ML, Pinto, RS, Izquierdo, M, et al. Chronic effects of different intensities of power training on neuromuscular parameters in older people: A systematic review with Meta-analysis. Sports Med Open. (2023) 9:98. doi: 10.1186/s40798-023-00646-9
50. Duval, S, and Tweedie, R. Trim and fill: A simple funnel-plot-based method of testing and adjusting for publication Bias in Meta-analysis. Biometrics. (2000) 56:455–63. doi: 10.1111/j.0006-341x.2000.00455.x
51. Aoki, K, Nakao, A, Adachi, T, Matsui, Y, and Miyakawa, S. Pilot study: effects of drinking hydrogen-rich water on muscle fatigue caused by acute exercise in elite athletes. Med Gas Res. (2012) 2:12. doi: 10.1186/2045-9912-2-12
52. Ostojic, SM, and Stojanovic, MD. Hydrogen-rich water affected blood alkalinity in physically active men. Res Sports Med. (2014) 22:49–60. doi: 10.1080/15438627.2013.852092
53. Drid, P, Trivic, T, Casals, C, Trivic, S, Stojanovic, M, and Ostojic, SM. Is molecular hydrogen beneficial to enhance post-exercise recovery in female athletes? Sci Sports. (2016) 31:207–13. doi: 10.1016/j.scispo.2016.04.010
54. Kawamura, T, Gando, Y, Takahashi, M, Hara, R, Suzuki, K, and Muraoka, I. Effects of hydrogen bathing on exercise-induced oxidative stress and delayed-onset muscle soreness. Jpn J Phys Fitness Sports Med. (2016) 65:297–305. doi: 10.7600/jspfsm.65.297
55. Da Ponte, A, Giovanelli, N, Nigris, D, and Lazzer, S. Effects of hydrogen rich water on prolonged intermittent exercise. J Sports Med Phys Fitness. (2018) 58:612–21. doi: 10.23736/s0022-4707.17.06883-9
56. Botek, M, Krejčí, J, McKune, AJ, Sládečková, B, and Naumovski, N. Hydrogen rich water improved Ventilatory, perceptual and lactate responses to exercise. Int J Sports Med. (2019) 40:879–85. doi: 10.1055/a-0991-0268
57. Javorac, D, Stajer, V, Ratgeber, L, Betlehem, J, and Ostojic, S. Short-term H(2) inhalation improves running performance and torso strength in healthy adults. Biol Sport. (2019) 36:333–9. doi: 10.5114/biolsport.2019.88756
58. Botek, M, Krejčí, J, McKune, AJ, and Sládečková, B. Hydrogen-rich water supplementation and up-hill running performance: effect of athlete performance level. Int J Sports Physiol Perform. (2020) 15:1193–6. doi: 10.1123/ijspp.2019-0507
59. Todorovic, N, Javorac, D, Stajer, V, and Ostojic, SM. The effects of supersaturated hydrogen-rich water bathing on biomarkers of muscular damage and soreness perception in young men subjected to high-intensity eccentric exercise. J Sports Med (Hindawi Publ Corp). (2020) 2020:8836070–5. doi: 10.1155/2020/8836070
60. Hori, A, Ichihara, M, Kimura, H, Ogata, H, Kondo, T, and Hotta, N. Inhalation of molecular hydrogen increases breath acetone excretion during submaximal exercise: A randomized, single-blinded, placebo-controlled study. Med Gas Res. (2020) 10:96–102. doi: 10.4103/2045-9912.296038
61. Alharbi, AAD, Iwamoto, N, Ebine, N, Nakae, S, Hojo, T, and Fukuoka, Y. The acute effects of a single dose of molecular hydrogen supplements on responses to ergogenic adjustments during high-intensity intermittent exercise in humans. Nutrients. (2022) 14:3974. doi: 10.3390/nu14193974
62. Hong, Y, Dong, G, Li, Q, Wang, V, Liu, M, Jiang, G, et al. Effects of pre-exercise H(2) inhalation on physical fatigue and related prefrontal cortex activation during and after high-intensity exercise. Front Physiol. (2022) 13:988028. doi: 10.3389/fphys.2022.988028
63. Jebabli, N, Ouerghi, N, Abassi, W, Yagin, FH, Khlifi, M, Boujabli, M, et al. Acute effect of hydrogen-rich water on physical, perceptual and cardiac responses during aerobic and anaerobic exercises: A randomized, placebo-controlled, double-blinded cross-over trial. Front Physiol. (2023) 14:1240871. doi: 10.3389/fphys.2023.1240871
64. Dong, G, Wu, J, Hong, Y, Li, Q, Liu, M, Jiang, G, et al. Inhalation of hydrogen-Rich gas before acute exercise alleviates exercise fatigue. Int J Sports Med. (2024). doi: 10.1055/a-2318-1880
65. Sládečková, B, Botek, M, Krejčí, J, Valenta, M, McKune, A, Neuls, F, et al. Hydrogen-rich water supplementation promotes muscle recovery after two strenuous training sessions performed on the same day in elite fin swimmers: randomized, double-blind, placebo-controlled, crossover trial. Front Physiol. (2024) 15:1321160. doi: 10.3389/fphys.2024.1321160
66. Ohta, S . Direct targets and subsequent pathways for molecular hydrogen to exert multiple functions: focusing on interventions in radical reactions. Curr Pharm Des. (2021) 27:595–609. doi: 10.2174/1381612826666200806101137
67. Zheng, H, and Yu, YS. Chronic hydrogen-rich saline treatment attenuates vascular dysfunction in spontaneous hypertensive rats. Biochem Pharmacol. (2012) 83:1269–77. doi: 10.1016/j.bcp.2012.01.031
68. Ishibashi, T . Therapeutic efficacy of molecular hydrogen: A new mechanistic insight. Curr Pharm Des. (2019) 25:946–55. doi: 10.2174/1381612825666190506123038
69. Iuchi, K, Imoto, A, Kamimura, N, Nishimaki, K, Ichimiya, H, Yokota, T, et al. Molecular hydrogen regulates gene expression by modifying the free radical chain reaction-dependent generation of oxidized phospholipid mediators. Sci Rep. (2016) 6:18971. doi: 10.1038/srep18971
70. Powers, SK, and Jackson, MJ. Exercise-induced oxidative stress: cellular mechanisms and impact on muscle force production. Physiol Rev. (2008) 88:1243–76. doi: 10.1152/physrev.00031.2007
71. Bassett, DR Jr, and Howley, ET. Limiting factors for maximum oxygen uptake and determinants of endurance performance. Med Sci Sports Exerc. (2000) 32:70–84. doi: 10.1097/00005768-200001000-00012
72. Coyle, EF . Integration of the physiological factors determining endurance performance ability. Exerc Sport Sci Rev. (1995) 23:25–63. doi: 10.1249/00003677-199500230-00004
73. Liu, L, Xie, K, Chen, H, Dong, X, Li, Y, Yu, Y, et al. Inhalation of hydrogen gas attenuates brain injury in mice with Cecal ligation and puncture via inhibiting Neuroinflammation, oxidative stress and neuronal apoptosis. Brain Res. (2014) 1589:78–92. doi: 10.1016/j.brainres.2014.09.030
74. Dohi, K, Satoh, K, Miyamoto, K, Momma, S, Fukuda, K, Higuchi, R, et al. Molecular hydrogen in the treatment of acute and chronic neurological conditions: mechanisms of protection and routes of administration. J Clin Biochem Nutr. (2017) 61:1–5. doi: 10.3164/jcbn.16-87
75. Ishii, A, Tanaka, M, and Watanabe, Y. Neural mechanisms of mental fatigue. Rev Neurosci. (2014) 0:469–79. doi: 10.1515/revneuro-2014-0028
76. Lancha Junior, AH, Painelli Vde, S, Saunders, B, and Artioli, GG. Nutritional strategies to modulate intracellular and extracellular buffering capacity during high-intensity exercise. Sports Med. (2015) 45:71–81. doi: 10.1007/s40279-015-0397-5
Keywords: molecular hydrogen, physical performance, aerobic endurance, maximum oxygen uptake, maximal anaerobic test, muscle strength, countermovement jump
Citation: Zhou K, Shang Z, Yuan C, Guo Z, Wang Y, Bao D and Zhou J (2024) Can molecular hydrogen supplementation enhance physical performance in healthy adults? A systematic review and meta-analysis. Front. Nutr. 11:1387657. doi: 10.3389/fnut.2024.1387657
Edited by:
Justin Roberts, Anglia Ruskin University, United KingdomReviewed by:
Lin Cheng, Guangxi Normal University, ChinaAmane Hori, University of Texas Southwestern Medical Center, United States
Yang Song, Óbuda University, Hungary
Copyright © 2024 Zhou, Shang, Yuan, Guo, Wang, Bao and Zhou. This is an open-access article distributed under the terms of the Creative Commons Attribution License (CC BY). The use, distribution or reproduction in other forums is permitted, provided the original author(s) and the copyright owner(s) are credited and that the original publication in this journal is cited, in accordance with accepted academic practice. No use, distribution or reproduction is permitted which does not comply with these terms.
*Correspondence: Dapeng Bao, YmFvZHBAYnN1LmVkdS5jbg==
†These authors have contributed equally to this work and share first authorship