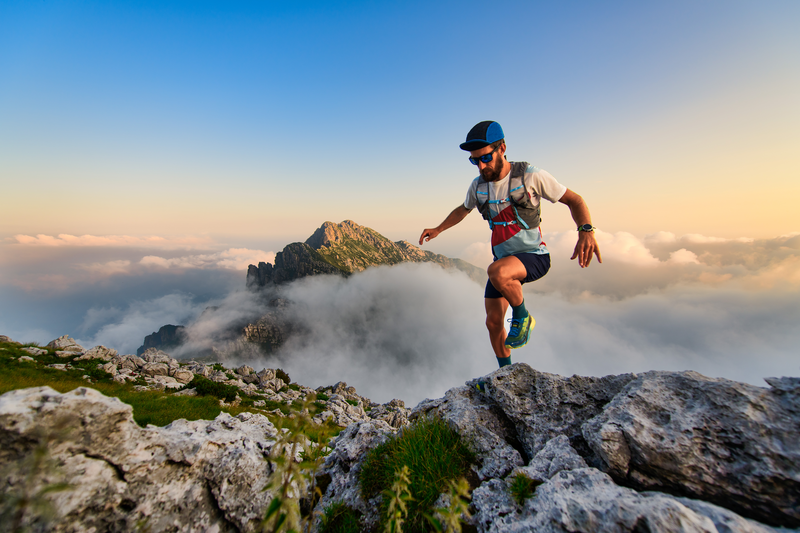
94% of researchers rate our articles as excellent or good
Learn more about the work of our research integrity team to safeguard the quality of each article we publish.
Find out more
REVIEW article
Front. Nutr. , 25 April 2024
Sec. Food Chemistry
Volume 11 - 2024 | https://doi.org/10.3389/fnut.2024.1378884
This article is part of the Research Topic Novel Analytical Technologies and Chemometrics: Quality and Structural Biological Analysis of Agri-food Components View all 6 articles
Myofibrillar proteins are an important component of proteins. Flavor characteristics are the key attributes of food quality. The ability of proteins to bind flavor is one of their most fundamental functional properties. The dynamic balance of release and retention of volatile flavor compounds in protein-containing systems largely affects the sensory quality and consumer acceptability of foods. At present, research on flavor mainly focuses on the formation mechanism of flavor components, while there are few reports on the release and perception of flavor components. This review introduces the composition and structure of myofibrillar proteins, the classification of flavor substances, the physical binding and chemical adsorption of myofibrillar proteins and volatile flavor substances, as well as clarifies the regulation law of flavor substances from the viewpoint of endogenous flavor characteristics and exogenous environment factors, to provide a theoretical reference for the flavor regulation of meat products.
Myofibrillar protein (MP) is a group of salt-soluble structural proteins with biological functions, and it is also an important component of contracting muscle fibers in muscle (1). However, MP itself does not have odor, but it can interact with flavor compounds through specific molecular bonds to enhance and regulate the overall flavor of meat products, thus affecting the flavor intensity of food (2). MP can interact with flavor compounds through special molecular bonds, which can affect the flavor intensity of the food (3). Through physical or chemical interaction with flavor compounds, MP can adsorb volatile flavor substances, affect the flavor intensity of food to different degrees, change the overall balance of flavor, and thus affect the release of flavor (4).
Because sensory organs are very sensitive to changes in flavor levels, interactions between flavor compounds and other components in food can have a profound effect on flavor perception by altering the rate at which volatile flavors are released. Protein in meat products provides a complex scientific site for interaction with flavor compounds, protein molecules can bind and separate lipophilic flavor molecules, so that adverse flavor in food is transmitted to food, seriously affecting the sensory and edible quality of food (5). Graf et al. (6) first observed the transmission of this odor property in some products containing whey protein and soy protein. The flavor adsorption properties of proteins are largely affected by the conformational state of proteins and the factors that change the conformational state (7). In addition, it is also affected by exogenous factors such as temperature and pH, which ultimately lead to changes in food flavor and quality.
Flavor is one of the important factors for consumers to choose meat products (8). The adsorption and release of protein and flavor substances will affect the sensory experience of meat products to some extent, so that adverse flavor in food is transmitted to food, which seriously affects the sensory and edible quality of food. There are reversible non-covalent bonds and irreversible covalent bonds in the binding process of protein-aroma compounds. Covalent bond cooperation mainly includes covalent cross-linking of aldehyde groups (–CHO), carbonyl groups (C = O) and amino acid residues (–NH2 and –SH) (9). Studies have shown that with the increase of trypsin content, the adsorption capacity of myosin to aldehydes and ketones is enhanced, mainly due to the increase of amino activity and sulfhydryl group content, which leads to the development of the secondary structure of proteins, thus enhancing the binding behavior of proteins with volatile components (10). In addition, the ε-amino group of the lysine residue side chain also binds with aldehydes through the formation of Schiff bases. Anantharamkrishnan et al. (11) found that milk proteins can be covalently bonded with aldehydes, mercaptans and furans through Schiff bases, the formation of disulfide bonds and the Michael addition reaction. In addition, some amine volatiles form amide bonds with the terminal carboxyl groups of aspartate and glutamate, which also belong to covalent bonding (12). The formation rate of these combinations is slow or fast, which can lead to changes in food flavor characteristics.
There are many definitions of food flavor, which was first proposed by Hall R. L. in 1986. He believes that food flavor is the sum of sensory impressions produced by people’s sensory organs after ingesting oral food, including smell, taste, touch, temperature and pain (13). There are thousands of known flavor substances, which can be divided into hydrocarbons, aldehydes, ketones, alcohols, esters, sulfur compounds, furans, pyrazines and so on (14). Protein degradation can promote the formation of flavor substances, and it can change the headspace concentration through adsorption of volatile components by molecular bonds (15), it also affects the gel and texture of food. By increasing the mass transfer resistance of volatile components, the overall flavor balance can be changed to different degrees, which has a great impact on the flavor release of food.
This review mainly introduces the composition and structure of MP, the classification of flavor substances, the physical binding and chemisorption of MP and volatile flavor substances, and the influencing factors (see Figure 1).
MP is divided into three categories according to their composition structure: (1) filament in, which is mainly composed of myosin and actin (2) regulatory proteins, including the tropomyosin-troponin complex, α-/β-actinin, M-protein and C-protein (3) scaffold protein, which consists of titin, associated actin (nebulin), desmin, vimentin and synemin (16), myosin and actin participate in the contraction process of muscles and combine to form actomyosin. The adsorption capacity of G-actin and myosin in MP to volatile flavor substances is different. Pérez-Juan et al. (15) find that G-actin and myosin in dry-cured ham were mainly bound to flavor components with small partition coefficients. Cao et al. (17) found that different concentrations of H2O2 affected the changes of α dynamic helix, carbonyl group, hydrogen bond, sulfhydryl group and hydrophobic stage point in G-actin, thus causing differences in its adsorption capacity for alcohols and aldehydes (Figure 2).
Flavor not only includes aroma, but also taste. At present, a variety of flavor substances produced in meat products can be roughly divided into two categories: one is volatile flavor substances, whose production and existence of flavor substances can be released from food into the environment, causing changes in food flavor profile. The other is non-volatile flavor substances, such as inorganic salts, free amino acids, small peptides and nucleic acid metabolites such as inosine acid, ribose and so on. Flavor precursors themselves do not produce flavor, and the products after the reaction of flavor precursors are usually summarized as odor and taste (18). The research on odor mainly focuses on volatile components, while the research on taste mostly focuses on non-volatile components (see Figure 3).
Figure 3. The interaction between MP and flavor substance and its influencing factors and detection methods.
The most important contribution to the characteristic flavor of meat products is the volatile flavor substance (19). Volatile flavor substances can be divided into esters, aldehydes, ketones, alkanes, aromatics, alkenes and halohydrocarbons (20). Muscle protein itself has no fragrance, and processed meat products will produce specific flavor compounds. In different processing methods, due to different heat transfer methods, meat products will produce and release a large number of flavor compounds through fat oxidation and degradation. Maillard reaction and degradation of flavor precursor substances, such as: Alcohol, aldehydes, carboxylic acid, ester, furan, pyridine, pyrazine, thiazole, thiophene, nitrogen, sulfur, etc., and then produce meat flavor (21). Song et al. (22) reported that one-hundred-and-nine volatile compounds were identified by GC-MS using SPME and SDE methods in two braised porks. From the SDE-AEDA-GC/O analysis, it was found that pentanal (almond, pungent), nonanal (fat, green) (E, E)-2,4-decadienal (fat, roast), phenyl acetaldehyde (hawthorne, honey, sweet), dodecanal (lily, fat, citrus) and linalool showed the highest OAV values (>200), indicating a contribution to the aroma of braised pork.
Non-volatile flavor substances, namely non-volatile flavor substances, determine the flavor characteristics of meat products. Flavor compounds refer to non-volatile or water-soluble substances that have taste or sense of touch, and are often the precursor substances of volatile flavor substances. The taste substances in meat products mainly include amino acids, small peptides, nucleic acid metabolites and inorganic salts, etc. (18). Chiang et al. (23) studied the non-volatile flavor substances in chicken soup, pork soup, mushroom soup and seafood soup, and the analysis showed that guanylic acid, inosine acid and xanthylate played the main role in flavor in the broth.
The original and processed flavor substances of meat and meat products mainly include sulfur compounds, oxygen-containing heterocyclic compounds, nitrogen-containing heterocyclic compounds, aldehydes, ketones, alcohols, acids and lactones (24). The interaction of proteins with flavor substances mainly consists of physical binding and chemical interactions. In meat and meat products, hydrophobic interaction is the main force to maintain the interaction between protein and flavor substances (7). The use of reversible combinations can be used to reduce flavor loss during processing and re-release of flavor components during consumption, while irreversible combinations are important for the removal of off-flavors from foods. Anantharamkrishnan and Reineccius (25) used Fourier infrared spectroscopy, nuclear magnetic resonance, equilibrium dialysis and other analytical methods to study the flavor combination sites of external lactoglobulin, and confirmed that the flavor binding sites of globulin-lactoglobulin were mostly distributed in the protein hydrophobic region, and there were multiple different combination sites and secondary combination sites with weak effects. Various flavor components either bind to the hydrophobic region on the outer surface of the protein, or act on the central hole of the protein. However, which flavor components each of these action sites tends to bind to, and the specific effects on the conformation of the protein, are unclear. The binding mechanism of protein and volatile flavor components still needs to be further studied.
The diversity of amino acid side chain structure enables proteins to interact with volatile flavor components in a variety of forces, most of which are reversible, including ionic bonds, hydrogen bonds and hydrophobic interactions (26). The covalent bond between the two is irreversible, mainly including the covalent cross-linking of aldehydes and the amino group of the side chain of lysine residues through the condensation of aldehyde group and imino to Schiff base and the binding of sulfur compounds to proteins (4) (Table 1). In addition, proteins can physically adsorb volatile components through van der Waals force and capillary adsorption.
Table 1. Interaction mechanism and main influencing factors between myofibrillar protein and flavor substances.
The physical combination between proteins and flavor substances is mainly achieved by van der Waals forces and electrostatic forces, including van der Waals forces and capillary adsorption, which are reversible. In general, flavor compounds are physically trapped in the capillaries and fissures of proteins to influence their flavor properties, and non-polar compounds such as hydrocarbons, lipids, and proteins have reversible hydrophobic interactions. Polar compounds such as alcohols interact with proteins to form hydrogen bonds, and fatty acids generally interact with proteins in an electrostatic manner (38).
Chemical interactions include reversible weak hydrophobic interactions, strong ionic effects, and irreversible strong covalent bonds, namely electrostatic adsorption, hydrogen bonding, and covalent bonding (39). Some protein groups can have strong binding with some flavor substances, such as aldehydes and ketones volatiles can form a Schiff base with the terminal amino group of lysine, while some amine volatiles can form an amide bond with the terminal carboxyl group of aspartate and glutamate, and aldehyde group compounds can form a Schiff base with amino acids to covalently bind proteins (40).
Some flavor molecules can interact with the side chains of proteins via covalent bonds, including aldehyde-lysine and amine-carbonyl. These interactions are usually irreversible. The binding of sulfur compounds to proteins can also be classified as covalent interactions (9, 41). Anantharamkrishnan et al. (11) showed that covalent bonds were formed between β-lactoglobulin and aldehydes, mercaptans and furans containing functional groups, resulting in Schiff base, Michael addition and disulfide bonds. The formation of covalent bonds between proteins and flavor compounds is responsible for the loss of flavor and shortened shelf life of foods (42). Only the aroma that interacts with the protein through non-covalent forces is conducive to the flavor characteristics of protein-containing foods. The interaction of flavor compounds with proteins is usually completely reversible, but in some cases the volatile flavor substances are covalently bound to proteins, which is usually irreversible. For example, the amino group of aldehydes and lysine residues, and the amino group and carboxyl group are irreversible. In meat and meat products, hydrophobic interaction is the main force to maintain the interaction between proteins and flavor substances, which is also a manifestation of the special folding of polypeptide chains and the tendency of protein structure to stabilize (43). In liquid and high-moisture foods, the mechanism of binding flavor substances to proteins mainly involves the interaction of non-polar flavor compounds with hydrophobic regions or cavities on the protein surface. In general, proteins with strong surface hydrophobicity will also directly adsorb flavor compounds on the molecular surface (44). For those protein molecules with weak surface hydrophobicity, flavor substances enter the protein molecules and bind to the hydrophobic point of the cavity (44). In addition, flavor compounds containing polar groups, such as hydroxyl and carboxylic groups, can combine with proteins through hydrogen bonding and ionic effects. In general, most interactions between proteins and flavor compounds are reversible, such as ionic bonding, hydrogen bonding, and hydrophobic interactions. Guichard (4) found that the hydrophobicity of proteins and flavor compounds showed a positive correlation during flavor-protein interactions (see Table 2).
Table 2. Method for analysis and detection of binding sites and binding constants of protein and flavor substances.
The spatial conformation changes of proteins in meat products affect the binding degree of volatile flavor substances, and these changes can be detected by chromatography, mass spectrometry and spectroscopy. At present, many relevant studies focus on fluorescence spectroscopy, UV–VIS absorption spectroscopy, circular dichroism spectroscopy and molecular docking to analyze the mechanism of protein adsorption of volatile flavor substances. The following reviews the mechanism of protein adsorption of volatile flavor substances from three aspects: the degree of flavor adsorption, the change of protein structure, the force and the action site.
The degree of protein adsorption of volatile flavor substances is mainly resolved by liquid chromatography with gas chromatography-mass spectrometry (GC-MS) (27). The structural changes of proteins are mainly analyzed by thermodynamic methods and circular dichroism spectroscopy, with thermodynamic methods having the advantages of small sample size and high method sensitivity. The force and site of volatile flavor adsorption by proteins are mainly analyzed by spectroscopy, nuclear magnetic resonance (NMR) and molecular docking techniques. Currently, fluorescence spectroscopy, ultraviolet-visible absorption spectroscopy, circular dichroism spectroscopy and molecular docking are more frequently applied to analyze the mechanism of protein adsorption of volatile flavor substances.
The flavor substances such as aldehydes, alcohols, esters, sulfur-containing and nitrogen-containing have the characteristics of fat solubility and hydrophobicity (66). At present, omics methods are mainly used for analysis, including GC-MS, thin layer chromatography (TLC), matrix-assisted laser desorption ionization time-of-flight MS (MALDI-TOF-MS), electrospray ionization MS (ESI-MS) Q-Exactive high-resolution MS and NMR. Single technology cannot fully reflect the interaction between molecules, has certain limitations, can be used in combination with MS, multi-spectrum, molecular simulation and other technologies (67).
Commonly used instrumental analysis methods to study the interaction between protein and flavor components include: gas chromatography-mass spectrometry, electronic nose (68); high performance liquid chromatography-mass spectrometry; gas chromatography-olfactory measurement (69, 70). Common detection methods: fluorescence spectrometry, differential scanning calorimetry, infrared spectroscopy, sedimentation velocity analysis, light scattering method, circular dichroic chromatography.
When the flavor diffuses into the interior of the protein molecule, it destroys the hydrophobic interaction between the protein chain segments and destabilizes the protein structure, thus changing the protein conformation. For instance, flavor substances containing active groups, such as aldehydes, covalently binds lysine residues to promote the exposure of protein hydrophobic groups. As the protein molecule increases in size, it has a corresponding increase in the ability to absorb flavor substances, and the ability of proteins with porous properties to absorb flavor substances is significantly enhanced (71). After protein denaturation, the ability of proteins to absorb flavor substances increases after denaturation because more hydrophobic groups are exposed when the hydrophobic bonds that maintain the spatial helical structure of proteins are broken, enhancing the uptake of nonpolar flavor compounds. Any factor that can change the conformation of a protein can affect its binding to flavor substances, except for factors in the protein itself, such as moisture, temperature, pH, and the presence of salts and lipids. The interaction between muscle proteins and volatile compounds is related to protein properties, flavor types, ionic strength, pH value, protein oxidation and heating temperature, but there is no unified mechanism. Protein concentration, heating temperature, pH value and protein oxidation can induce the change of protein conformation, and then affect the interaction between protein and flavor substances (72). It is a complicated and arduous systematic project to study the adsorption properties of proteins for flavor. Therefore, it is of great significance to explore the factors that induce the change of muscle protein structure and how the structural changes affect the interaction between muscle protein and flavor substances.
MP exhibits varying adsorption capacities for different flavor compounds, which is closely associated with the nature of flavor compounds (73). The adsorption binding of MP to flavor substances is altered by changes in protein concentration and properties. In food systems with high water content, the mechanism of protein interaction with flavor substances depends not only on water content, but also on the number and structure of protein side chains (74). As the concentration of volatile compounds increases, the more volatile compounds added to the water phase, the more volatile compounds F-actin binds (34). Compared with G-actin and actomyosin, F-actin binds to higher amounts of 3-methyl-butyraldehyde, 2-methyl-butyraldehyde, methoxy, caproaldehyde, and 2-pentanone. On the other hand, in the case of 3-methyl-butyral, caproaldehyde, methoxy, and octylaldehyde, the number of moles of G-actin binding is reduced and these volatile compounds are released into headspace. Under conditions of higher actomyosin and G-actin concentrations, octylaldehyde, 3-methylbutyral, hexal, methioaldehyde, and octylaldehyde are released into headspace, probably due to the presence of proteins that weaken their interactions (75). With the increase of protein concentration, the release of flavor components may be due to the enhanced interaction between proteins to weaken the adsorption between proteins and flavor components. Gu et al. (76) studied the interaction between carbonyl compounds and bovine serum protein, and also found that with the increase of bovine serum protein concentration, the interaction between proteins was strengthened, while the interaction between proteins and flavor distribution sites was weakened. In addition, it is also possible that the increase in protein concentration leads to a decrease in surface tension and thus an increase in the release of flavor components. O’Neill (5) also pointed out that MP is an effective surface tension inhibitor. Changes in protein conformation can also affect its effect on flavor substances, possibly due to changes in available protein binding sites that affect the interaction between volatile compounds and proteins (77).
Potential of hydrogen is one of the important factors that affect the binding ability of food protein and flavor compounds. Potential of hydrogen can change the secondary structure of proteins by changing the microenvironment of amino acid residues, surface hydrophobicity, protein aggregation degree, protein solubility, amount of charge carried by protein molecules, and inducing protein denaturation (78), thus changing the non-covalent interaction between protein molecules and flavor substances. Shimizu et al. (79) studied the structural characteristics of β-lactoglobulin at different potential of hydrogen, and the effect of β-lactoglobulin on flavor components was stronger at high potential of hydrogen. Gianelli et al. (80) reported the effect of curing agents treated with different potential of hydrogen on the binding ability of soluble protein and flavor compounds in skeletal muscle in the model system. It was confirmed by calculating the thermodynamic binding parameters (binding sites n and binding constant K) that potential of hydrogen treatment affects the ability of soluble proteins to bind flavor compounds in the model system. When Shen et al. (78) studied the mechanism of structural changes induced by different potential of hydrogen, they found that the adsorption capacity of MP to pyrazine compounds was jointly affected by pH value and pyrazine compounds, and the protein–protein interaction was enhanced at low potential of hydrogen (4.9–5.5), resulting in the formation of large-particle aggregates of MP. In addition, the binding fluorescence quenching and thermodynamic parameters were used to study the effect of pH-induced conformation changes on the interaction mechanism of MP with pyrazines. It was found that with the increase of potential of hydrogen (pH 5.0–8.0), the adsorption capacity of MP to aldehydes and esters increased, while the adsorption capacity of ketones decreased. The results showed that electrostatic and hydrophobic action were the main binding forces of MP and 2,5-dimethylpyrazine. The interaction is greatly influenced by potential of hydrogen, and at lower potential of hydrogen conditions (pH 4.9), protein–protein interactions are enhanced, MPs aggregate into larger particles, and surface hydrophobicity increases, thus exposing more hydrophobic binding sites (81). However, with the increase of surface hydrophobicity, the interaction between proteins (aggregation and sedimentation) is enhanced, and the steric hindrance generated prevents the binding of proteins with flavor substances, and the flavor release behavior of proteins is enhanced. In addition, establishing a correlation between pH-induced myofibrillar conformational changes and flavor substance interactions is critical for controlling meat-flavored myofibrillar-containing products.
Temperature mainly affects the flavor adsorption capacity and the number of action sites of proteins, in addition, it also affects the flavor release by affecting the rheological properties of food and the distribution coefficient of flavor components (82). The influence of temperature on adsorption is based on the change of protein structure, especially the thermal denaturation of protein, but different sites of action are affected differently by temperature (82, 83).
Heat treatment is one of the most important steps in food processing and is commonly used in meat processing (84). The effect of heat treatment on protein flavor adsorption capacity has also been studied for many times. During the process of protein structure development and polymerization, the flavor of meat products can be changed by modification of specific binding sites or exposure of more hydrophobic flavor binding sites. Kang et al. (85) found that the structure of beef myofibrillar protein would change to different degrees with the increase of temperature and the extension of heating time. Lv et al. (10) studied the effects of different temperatures (45, 55, 65, 75, and 85°C) on myosin structure and flavor adsorption capacity, they pointed out that the interaction of myosin with aldehydes at different heating temperatures mainly depended on hydrophobicity, and that sulfhydryl and hydrogen bonding interactions determined the unfolding and polymerization of myosin structure, providing or modifying more flavor-binding sites, which in turn affected the adsorption of aldehydes. Zhou et al. (86) found that the secondary structure and surface hydrophobicity of myofibrillar gel changed within 0–5 min of heat treatment, which was consistent with the change time of the adsorption capacity of myofibrillar gel for different volatile flavor substances affected by heat treatment. The results showed that the changes of protein structure were related to the adsorption of volatile flavor compounds. Xu et al. (87) studied the effects of two-step heat treatment on the structure of myofibrillar protein of grass carp and its binding ability with hexal, heptyl, caprylic and nonaldehyde. The results showed that the surface hydrophobicity and total sulfhydryl content of myofibrillar protein increased within 30 min of the first heating at 40°C and 5–10 min of the second heating at 90°C, respectively. It may be due to the exposure of hydrophobic amino acids and sulfhydryl groups that lead to the unfolding of the secondary structure of myofibrillar protein, thereby improving the flavor binding capacity of myofibrillar protein. Prolonged heating at 90°C accelerates the aggregation of unfolded myofibrillar protein, reduces the hydrophobic binding site, and thus reduces the binding capacity of myofibrillar protein to aldehydes.
In addition to protein, animal meat also contains reducing substances such as ribose and glucose, which underwent maillard reactions during heat treatment, not only giving meat products their characteristic flavor and color, but also affecting the digestion and utilization of protein. Setyabrata et al. (88) compared the volatile flavor components of yak meat myofibrillar protein with those of fructose and glucose in the melad reaction. At a reaction temperature of 130°C, fructose and glucose were involved in the melad reaction to produce the most abundant and the highest content of volatile flavor components, and the types and contents of flavor components detected in the melad reaction involving glucose were significantly lower than those of fructose.
In addition to heat treatment, low temperatures also affect the interaction of myofibrillar proteins with flavor substances. The presence of fresh myosin resulted in a significant decrease (p < 0.05) in the percentage of free hexanal, methylthioal, and octanal, which indicated the ability of fresh myosin to bind these volatile compounds. However, the effect of frozen storage on actomyosin binding ability was different. The percentage of free caproaldehyde and methioaldehyde increased significantly when actomyosin was frozen with or without glycerin. This means that less volatile compounds are bound compared to fresh actomyosin. When actomyosin was frozen with glycerin, the percentage of free octaldehyde decreased significantly, while the percentage of free octaldehyde increased without glycerin. Fresh actomyosin is not bound to 3-methylbutyraldehyde, 2-methylbutyraldehyde and 2-pentanone, and the dissociation rate of these compounds is not affected by freezing when glycerol is used, but the dissociation rate of these compounds is significantly reduced when glycerol is not used (89).
The binding of MP to flavor substances is also affected by the types and properties of flavor substances. Hexal, heptyl, octyl, nonylal and 1-octene-3-ol are the main volatile substances that provide meat flavor (90) (E)-2-decenal (E, Z)-2,4-decenal, and (E, E)-2,4-nonadienal have a strong meat flavor, and dimethyl trisulfide and phenylacetaldehyde contribute to the formation of meat flavor (84). These 10 volatile substances have important effects on the formation of meat flavor.
In terms of flavor substance types, it was found that MPs adsorb several typical flavor compounds such as alcohols, aldehydes, ketones, and esters with different strengths and effects. The adsorption of aldehydes, aldehydes, ketones and esters by the same concentration of protein was in the order of aldehydes > esters > ketones > alcohols from strong to weak. Myofibrillar protein has little adsorption effect on lower alcohols. This is similar to the findings of Kühn et al. (91), possibly because there is no significant interaction between the hydroxyl group of alcohols and the amino group of proteins. However, with the increase of carbon chain length, the hydrophobicity of alcohols increases gradually, and myofibrillar protein adsorbs them to some extent through hydrophobic interaction. In addition, among various flavor substances, ketones are the main compounds in the flavor of meat products, contributing significantly (92).
The difference of molecular structure of flavor compounds can also lead to the difference of adsorption capacity of MP to flavor compounds. For similar flavor compounds, the larger the molecular weight, the longer the carbon chain, the stronger the molecular hydrophobicity, the stronger the hydrophobic binding ability of proteins, and the higher the adsorption efficiency (93). On the contrary, the larger the molecular volume of the compound, the more obvious the steric hindrance effect, the more difficult it is to enter the binding site inside the protein molecule, resulting in low adsorption efficiency (94). Hansen et al. (95) found that the adsorption capacity of proteins to ketones increased with the increase of the number of carbon atoms. Han et al. (96) found that the interaction between nonylaldehyde and MP had no significant effect on its conformation, and the binding constant and number of binding sites increased with the increase of temperature.
Another important factor that plays a key role in food flavor binding is the stability and reactivity of flavor substances. Weerawatanakorn et al. (97) conducted a comprehensive review of the chemical reactivity of 10 food flavor compounds frequently detected in food. In general, active functional groups, such as hydroxyl, sulfhydryl and carbonyl groups, can affect the chemical reactivity of these compounds, or can affect the physical or chemical interactions between flavor agents and various MPs. During food processing, including heat treatment, flavor compounds not only interact with proteins, but also easily undergo structural changes through various chemical reactions. Such as the degradation of free amino acids, the oxidation of aldehydes to acids, and the rearrangement and isomerization of terpenes under acidic conditions (98). The “kinetic” reactions of flavor substances can affect the overall flavor characteristics of foods, making the study of protein-flavor interactions more complicated (99). Due to the complexity of the food system, flavor substances can simultaneously interact with other components in the food matrix such as water, lipids, carbohydrates, vitamins, and minerals. Nevertheless, for protein-rich foods, such as dairy and meat products, protein is still considered to be an important component that causes flavor loss or release (8).
The interaction between proteins and flavors is not only affected by intrinsic factors such as the properties of proteins and flavors, as well as pH, temperature, protein hydration capacity, but also by other external factors such as ionic strength and oxidation conditions (100). In addition to the internal mechanism of binding, factors such as enzymatic reactions, high pressure, microwaves, or pulsed electric fields can also be affected, and they are the basic methods for altering protein-flavor interactions by changing the external or internal environmental conditions of the food system (101). These factors can influence the binding behavior of proteins and flavors by changing the structure of proteins,and they are reflected by binding parameters.
The structure of MP and the sequence of adsorption of flavor substances. Due to the differences in the chemical structure and functional groups of proteins and flavor compounds, there is currently no general theory to explain protein-flavor substance interactions (102). In general, studies tend to consider the binding strength of flavor components to proteins as follows: aldehydes are greater than ketones and alcohols are greater than alcohols (91, 102). When the flavor molecules have the same chemical identity, high flavor retention effects can be observed as the length of the fat chain increases (103). Ma et al. (104) studied the interaction of pyrazine compounds 2-methylpyrazine (MP), 2,5-dimethylpyrazine (DP), 2,3,5-trimethylpyrazine (TRP) and 2,3,5,6-tetramethylpyrazine (TEP) with proteins. To elucidate the effect of alkyl distribution in pyrazine ring on flavor release in bovine serum albumin (BSA) solution (pH 7.0). The results of SPME-GC-MS showed that the distribution of methyl group in pyrazine ring significantly affected its release from BSA solution. The release sequence of pyrazine compounds in BSA solution was MP > DP > TRP > TEP. Yin et al. (105) studied the interaction between furan derivatives and pork MPs and observed that the binding capacity of MPs decreased in the order of 5-methyl furfural > furfural >2-acetyl furan > furanol. The results indicate that hydrogen bonding, van der Waals forces, and hydrophobic interactions are the main ways that MPs interact with the four furan derivatives. Another study conducted by Menezes et al. (106), who confirmed that carboxyl derivatives of furfural have a higher affinity for BSA and human transferrin than ketone derivatives. The additional H-bond between the carboxyl derivatives of furfural and the protein contributes to the most stable complex. These results suggest that both the structure of flavor homologues and the properties of proteins in the food substrate can influence the release or retention of aroma compounds. The adsorption of proteins on certain and similar flavor substances also needs to be studied according to specific conditions to determine the influence of differences in physical and chemical properties of flavor substances on protein adsorption (15, 74, 93, 103, 107). In general, the adsorption efficiency of protein for aldehydes is higher than that for esters, ketones and alcohols, indicating that functional group activity and location play an important role in the protein-flavor compound interaction. In addition, hydrophobicity and steric hindrance of flavor compounds also play an important role in protein adsorption of flavor molecules. For similar flavor compounds, the larger the molecular weight and the longer the carbon chain, the stronger the molecular hydrophobicity, the stronger the protein’s hydrophobic binding ability, and the higher the adsorption efficiency. At the same time, the larger the molecular volume of the compound, the more obvious the steric hindrance effect, the more difficult it is to enter the binding site inside the protein molecule, resulting in low adsorption efficiency. Since the binding of flavor compounds to proteins is mainly attributed to the active groups of flavor molecules, steric hindrance effects are also mainly reflected in the effects on functional groups. Therefore, the adsorption effect of protein on flavor compounds should be considered by various factors, including not only the change of protein structure, but also the physicochemical properties of flavor compounds.
Scatchard (or Klotz) Since most protein-flavorant interactions can be attributed to reversible non-covalent binding, the classical theoretical models used to describe flavorant compounds in equilibrium with proteins follow the Scatchard (or Klotz) equation (108) Eq. 1 or Hill or the Hill model (109) Eq. 2:
where v is the number of moles of flavor ligand bound by protein per mole; n is the number of binding sites on the protein; K and [L] are the binding constant (mol/L)−1 and the concentration of the free flavoring ligand in solution at equilibrium (mol/L). The binding constant K can be thought of as the gas–liquid partition coefficient, which defines the concentration ratio of the flavor agent in the air and liquid phase at equilibrium. In general, the binding of flavor and protein is a spontaneous process, and the binding mechanism can be divided into two groups from the perspective of thermodynamic parameters: driven by enthalpy or entropy (110, 111). The enthalpy-driven process shows that the binding of flavors and proteins can occur at low temperatures, and that the forces of interaction between them include van der Waals forces and hydrogen bonds. In addition, the binding of flavor to protein is entropy-driven, which means that high temperatures favor the interaction. In other words, heat treatment or other processes that can induce protein unfolding are more conducive to promoting flavor-protein interactions (112–114). Previous studies have evidenced that modification of protein conformation exposes more hydrophobic interaction sites, which may contribute to the binding of flavor proteins (115).
In the post-2018 study, additional mathematical models were developed to predict flavor distribution in protein solutions. Tan et al. (116) constructed the equation model by partial least squares regression (PLSR) as a function of molecular descriptors describing the binding behavior of BSA and four types of flavor ligands (ketones, esters, aldehydes and alcohols) in the aqueous state. Viry et al. (43) attempted to evaluate the mechanism of protein-flavor binding using a practical dairy system containing caseinate and whey protein, and proposed a mathematical model for flavor distribution in protein solutions. It is assumed that the interaction between the flavor agent (F, 0.05–0.7 mg/kg) and the protein (P, 3–9%, w/v) is limited to bimolecular interactions and that no other interactions are involved in the reaction system. The result of appeal showed that the hydrophobicity of the flavor played a leading role in the retention of esters, alcohols and other flavor substances. Recent studies have confirmed that MP can bind to flavor substances such as ketones, alcohols, esters, aldehydes and some sulfur-containing compounds, which affects the final quality of meat products by changing the functional properties of proteins (15, 117). Lou et al. (103) found that the binding ability of ketones (2-heptanone, 2-pentanone, 2-nononone and 2-octanone) was positively correlated with the hydrophobicity and sulfhydryl content of G-actin in carp. The unfolding of G-actin secondary structure may expose new flavor compound binding sites, while the refolding and aggregation of proteins may be responsible for the decreased binding ability (87). To further elucidate the influence of ketone molecular structure, a study conducted by Shen et al. (118) systematically evaluated the binding behavior of MP to ketone flavor. By comparing the binding capacity of MP with 5-methyl-2-hexanone, 2-nonone, 2-pentanone, 2-heptanone, 2-octanone, 3-pentanone, 2,3-pentenedione and 2-nonanone, the results showed that the number and location of keto groups, molecular polarity and size played an important role in the interaction mechanism. The properties of MP have an insignificant effect on flavor binding behavior. In addition, Pérez-Juan et al. (15) found that MPs do not seem to be primarily responsible for volatile compound interactions, while sarcoplasmic proteins are important. The conformation and concentration of myofibrillar protein determines the binding capacity of the protein, and while F-actin can bind higher amounts of flavor compounds, G-actin cannot bind any selected flavor compounds.
In addition, computational methods for studying proteins have become increasingly powerful and popular in recent decades. Molecular docking and MD simulation are powerful tools for studying protein-ligand interactions, which can be used to aid research. Molecular docking is often used to find potential high-affinity ligands from a large number of different chemical libraries and to predict possible binding patterns. Molecular docking programs are commonly used in drug development and are favored because of their low computational cost. MD requires more computational resources than molecular docking, but allows research to study the dynamic properties of proteins. MD simulation has contributed to the study of protein folding, drug design and protein engineering. While molecular docking is commonly used for drug discovery, it has many other applications, such as protein engineering. Protein engineering is used to modify existing proteins to increase stability or change function, or to design entirely new protein sequences. Typically, the goal of protein engineering is to interact with modifications of small ligands. In this case, the binding of molecular docking with MD is an ideal way to characterize this interaction. Molecular docking can predict the binding posture and binding affinity of ligands, while MD adds flexibility, solvation effects, and dynamical information about bonds. Although both molecular docking methods and MD methods are useful individually, they complement each other and together facilitate many studies (119). Ren et al. (120) developed an enhanced sampling scheme for multi-domain proteins (generalized replica exchange with solute backfire selected surface-charged residues: gREST_SSCR) and applied it to ligand-mediated conformational changes in ribo-binding protein (RBPG134R) G134R mutants in solution.
In this paper, we reviewed the research progress of the interaction between MP protein and flavor substances in recent years, as well as the factors affecting the interaction and the methods of analysis and detection of binding sites and binding constants, which are helpful for the design and formulation of specific flavors in meat products, and also for qualitative and quantitative prediction of flavor binding behavior. In addition, we discuss in detail the influence of other components of the food system such as protein, moisture, temperature (thermal denaturation), pH, etc. on protein-flavor interactions.
At present, the adsorption of protein on flavor compounds is basically studied by constructing protein solution model and studying the interaction mechanism of the two in solution system. However, it is not the state of existence of meat proteins that is liquid in actual production. Many foods are solid or semi-solid phase, and are composed of several food components and flavor mixtures, which are much more complex than protein solution system. How to more truly analyze the mechanism of protein adsorption flavor substances in meat product matrix will be an important research content. These complex systems are important for predicting and controlling flavor release in real food systems. This paper presents a preliminary evaluation of the effect of temperature on protein flavor. In recent years, the application of non-thermal technology in the food industry has become increasingly extensive, and it has outstanding advantages in improving energy efficiency, improving product quality, and developing new products. Most studies show that compared with traditional treatment, some new non-thermal technologies such as supercritical fluid extraction, pulsed electromagnetic field, high-power ultrasonic wave, etc. are effective methods to improve the interaction between proteins and flavor substances. These techniques have broad application prospects in the modification of protein flavor in the future. In addition, the method of analysis and detection of binding sites and binding constants is discussed in this paper. It is found that the verification of binding sites for protein adsorption of flavor substances and the calculation of force-determined flavor retention coefficient still need theoretical breakthroughs. How the modification of flavor substances on meat substrates affects the adsorption of flavor substances needs to be systematically analyzed. Future research directions may also be highlighted. Food matrix is a complex system, based on the study of a single protein, the study of complex protein system and multi-component food matrix and flavor components is also a future development trend.
RQ: Writing – original draft, Writing – review & editing. CS: Writing – original draft, Writing – review & editing. TB: Formal analysis, Project administration, Writing – review & editing. JY: Funding acquisition, Writing – review & editing. JC: Formal analysis, Writing – review & editing. JZ: Funding acquisition, Project administration, Writing – review & editing.
The author(s) declare that financial support was received for the research, authorship, and/or publication of this article. This work was supported by the Key Research and Development Program of Sichuan Province (2022ZHCG0065), the earmarked fund for CAR-43, the National Modern Agricultural Industrial Technology System, Sichuan Innovation Team Construction Project (SCSZTD-2022-08-07) and the Natural Science Foundation of Sichuan Province (2022NSFSC1644), and the Open Funding Project of Meat Processing Key Laboratory of Sichuan Province (23-R-08 and 23-R-09).
JY was employed by Sichuan Laochuan East Food Co., Ltd.
The remaining authors declare that the research was conducted in the absence of any commercial or financial relationships that could be construed as a potential conflict of interest.
All claims expressed in this article are solely those of the authors and do not necessarily represent those of their affiliated organizations, or those of the publisher, the editors and the reviewers. Any product that may be evaluated in this article, or claim that may be made by its manufacturer, is not guaranteed or endorsed by the publisher.
1. Zhou, F, Zhao, M, Zhao, H, Sun, W, and Cui, C. Effects of oxidative modification on gel properties of isolated porcine myofibrillar protein by peroxyl radicals. Meat Sci. (2013) 96:1432–9. doi: 10.1016/j.meatsci.2013.12.001
2. Wu, Z., Xu, J., Ruan, J., Chen, J., Li, X., Yu, Y., et al. Probing the mechanism of interaction between capsaicin and myofibrillar proteins through multispectral, molecular docking, and molecular dynamics simulation methods. Food Chem X (2023), 18,:100734, doi: 10.1016/j.fochx.2023.100734
3. María, P-J, Mónica, F, and Fidel, T. Model studies on the efficacy of protein homogenates from raw pork muscle and dry-cured ham in binding selected flavor compounds. J Agric Food Chem. (2006) 54:4802–8. doi: 10.1021/jf060374x
4. Guichard, E . Interactions between flavor compounds and food ingredients and their influence on flavor perception. Food Rev Intl. (2002) 18:49–70. doi: 10.1081/fri-120003417
6. Graf, E, and de Roos, KB. Performance of vanilla flavor in low-fat ice cream. Agr food Sci (1996). 24–35.
7. Guo, J, He, Z, Wu, S, Zeng, M, and Chen, J. Effects of concentration of flavor compounds on interaction between soy protein isolate and flavor compounds. Food Hydrocoll. (2020) 100:105388. doi: 10.1016/j.foodhyd.2019.105388
8. Xu, J, Zhang, M, Wang, Y, and Bhandari, B. Novel technologies for flavor formation in the processing of meat products: a review. Food Rev Int. (2021) 39:802–26. doi: 10.1080/87559129.2021.1926480
9. Wang, K, and Arntfield, SD. Binding of carbonyl flavours to canola, pea and wheat proteins using GC/MS approach. Food Chem. (2014) 157:364–72. doi: 10.1016/j.foodchem.2014.02.042
10. Lv, T, Wang, Y, Pan, D, Cao, J, Zhang, X, Sun, Y, et al. Effect of trypsin treatments on the structure and binding capacity of volatile compounds of myosin. Food Chem. (2017) 214:710–6. doi: 10.1016/j.foodchem.2016.07.115
11. Anantharamkrishnan, V, Hoye, T, and Reineccius, GA. Covalent adduct formation between flavor compounds of various functional group classes and the model protein β-lactoglobulin. J Agric Food Chem. (2020) 68:6395–402. doi: 10.1021/acs.jafc.0c01925
12. Bikaki, M, Shah, R, Müller, A, and Kuhnert, N. Heat induced hydrolytic cleavage of the peptide bond in dietary peptides and proteins in food processing. Food Chem. (2021) 357:129621. doi: 10.1016/j.foodchem.2021.129621
13. Chambers, ET, and Koppel, K. Associations of volatile compounds with sensory aroma and flavor: the complex nature of flavor. Molecules. (2013) 18:4887–905. doi: 10.3390/molecules18054887
14. Starowicz, M . Analysis of volatiles in food products. Separations. (2021) 8:157. doi: 10.3390/separations8090157
15. Pérez-Juan, M, Flores, M, and Toldra, F. Binding of aroma compounds by isolated myofibrillar proteins: effect of protein concentration and conformation. Food Chem. (2007) 105:932–9. doi: 10.1016/j.foodchem.2007.04.051
16. Dara, PK, Geetha, A, Mohanty, U, Raghavankutty, M, Mathew, S, Chandragiri Nagarajarao, R, et al. Extraction and characterization of myofibrillar proteins from different meat sources: a comparative study. J Bioresour Bioprod. (2021) 6:367–78. doi: 10.1016/j.jobab.2021.04.004
17. Cao, C, Xie, J, Hou, L, Zhao, J, Chen, F, Xiao, Q, et al. Effect of glycine on reaction of cysteine-xylose: insights on initial Maillard stage intermediates to develop meat flavor. Food Res Int. (2017) 99:444–53. doi: 10.1016/j.foodres.2017.06.012
18. Crocker, EC . Flavor of meat. J Food Sci. (1948) 13:179–83. doi: 10.1111/j.1365-2621.1948.tb16609.x
19. Fu, Y, Cao, S, Yang, L, and Li, Z. Flavor formation based on lipid in meat and meat products: a review. J Food Biochem. (2022) 46:e14439. doi: 10.1111/jfbc.14439
20. Shahidi, F, Rubin, LJ, D’Souza, LA, Teranishi, R, and Buttery, RG. Meat flavor volatiles: a review of the composition, techniques of analysis, and sensory evaluation. Crit Rev Food Sci Nutr. (1986) 24:141–243. doi: 10.1080/10408398609527435
21. Yu, YY, and Yang, YP. Research progress of meat aroma substances. China Condiment. (2019) 44:173–177. doi: 10.3969/j.issn.1000-9973.2019.10.039
22. Song, S., Fan, L., Xu, X., Xu, R., Jia, Q., and Feng, T. Aroma patterns characterization of braised pork obtained from a novel ingredient by sensory-guided analysis and gas-chromatography-olfactometry. Foods (2019), 8,:87, doi: 10.3390/foods8030087
23. Chiang, P-D, Yen, C-T, and Mau, J-L. Non-volatile taste components of various broth cubes. Food Chem. (2007) 101:932–7. doi: 10.1016/j.foodchem.2006.02.041
24. Shahidi, F, and Hossain, A. Role of lipids in food flavor generation. Molecules. (2022) 27:27. doi: 10.3390/molecules27155014
25. Anantharamkrishnan, V, and Reineccius, GA. Method to characterize and monitor covalent interactions of flavor compounds with β-lactoglobulin using mass spectrometry and proteomics. J Agric Food Chem. (2020) 68:13121–30. doi: 10.1021/acs.jafc.9b07978
26. Zhang, B, Zhang, J, Yu, X, Peng, J, Pan, L, and Tu, K. Evaluation of the adsorption capacity and mechanism of soy protein isolate for volatile flavor compounds: role of different oxygen-containing functional groups. Food Chem. (2022) 386:132745. doi: 10.1016/j.foodchem.2022.132745
27. Xu, B, He, W, Yi, Y, Wang, H, Xu, W, and Guo, D. The impacting mechanism of β-sanshool in Zanthoxylum bungeanum Maxim on the structure of myofibrillar protein in duck meat. LWT. (2023) 182:114838. doi: 10.1016/j.lwt.2023.114838
28. Wang, Z, He, Z, Zhang, D, Chen, X, and Li, H. The effect of linalool, limonene and sabinene on the thermal stability and structure of rabbit meat myofibrillar protein under malondialdehyde-induced oxidative stress. LWT. (2021) 148:111707. doi: 10.1016/j.lwt.2021.111707
29. Wang, Y, Zhou, X, Liu, M, Zang, H, Zhang, R, Yang, H, et al. Quality of chicken breast meat improved by dietary pterostilbene referring to up-regulated antioxidant capacity and enhanced protein structure. Food Chem. (2023) 405:134848. doi: 10.1016/j.foodchem.2022.134848
30. Zhang, Y., Li, H., Zhang, Y., Wang, L., Zhang, P., Jia, J., et al. Storage stability and flavor change of marinated pork. Foods (2022), 11,:1825, doi: 10.3390/foods11131825
31. Chen, Q., Zhang, Y., Jing, L., Xiao, N., Wu, X., and Shi, W. Changes in protein degradation and non-volatile flavor substances of swimming crab (Portunus trituberculatus) during steaming. Foods (2022), 11,:3502, doi: 10.3390/foods11213502
32. Xie, W, Huang, Y, Xiang, Y, Xiong, S, Manyande, A, and Du, H. Insights into the binding mechanism of polyphenols and fish myofibrillar proteins explored using multi-spectroscopic methods. Food Bioprocess Technol. (2020) 13:797–806. doi: 10.1007/s11947-020-02439-4
33. Tian, Y, Li, C, Zeng, F, Yu, C, Xia, Z, and Huang, Y. Study the interactions between multiple flavonoids and bovine serum albumin by the developed equilibrium dialysis. J Chromatogr B. (2022) 1212:123515. doi: 10.1016/j.jchromb.2022.123515
34. Li, H., Zheng, R., Zuo, F., Qian, C., Yao, Z., Dong, R., et al. Influence of proteolysis on the binding capacity of flavor compounds to myofibrillar proteins. Foods (2022), 11:891, doi: 10.3390/foods11060891
35. Xu, Y, Zhao, J, Wang, R, Li, X, Mi, H, and Li, J. Effect of heat treatment on the binding of selected flavor compounds to myofibrillar proteins. J Sci Food Agric. (2019) 99:5028–34. doi: 10.1002/jsfa.9744
36. Sun, X., Yu, Y., Saleh, A.S.M., Yang, X., Ma, J., Gao, Z., et al. Structural changes induced by ultrasound improve the ability of the myofibrillar protein to bind flavor compounds from spices. Ultrason Sonochem (2023), 98, doi: 10.1016/j.ultsonch.2023.106510: 106510
37. Zhang, Y, Bai, G, Jin, G, Wang, Y, Wang, J, Puolanne, E, et al. Role of low molecular additives in the myofibrillar protein gelation: underlying mechanisms and recent applications. Crit Rev Food Sci Nutr. (2022) 64:3604–22. doi: 10.1080/10408398.2022.2133078
38. Houde, M, Khodaei, N, and Karboune, S. Assessment of interaction of vanillin with barley, pea and whey proteins: binding properties and sensory characteristics. LWT. (2018) 91:133–42. doi: 10.1016/j.lwt.2018.01.022
39. Meynier, A, Rampon, V, Dalgalarrondo, M, and Genot, C. Hexanal and t-2-hexenal form covalent bonds with whey proteins and sodium caseinate in aqueous solution. Int Dairy J. (2004) 14:681–90. doi: 10.1016/j.idairyj.2004.01.003
40. Innocente, N, Marchesini, G, and Biasutti, M. Feasibility of the SPME method for the determination of the aroma retention capacity of proteose-peptone milk protein fraction at different pH values. Food Chem. (2011) 124:1249–57. doi: 10.1016/j.foodchem.2010.07.056
41. Dupuis, J.H., Wang, S., Song, C., and Yada, R.Y. The role of disulfide bonds in a Solanum tuberosum saposin-like protein investigated using molecular dynamics. PLoS One (2020), 15,:e0237884, doi: 10.1371/journal.pone.0237884
42. Pelletier, E, Sostmann, K, and Guichard, E. Measurement of interactions between β-lactoglobulin and flavor compounds (esters, acids, and pyrazines) by affinity and exclusion size chromatography. J Agric Food Chem. (1998) 46:1506–9. doi: 10.1021/jf970725v
43. Viry, O, Boom, R, Avison, S, Pascu, M, and Bodnar, I. A predictive model for flavor partitioning and protein-flavor interactions in fat-free dairy protein solutions. Food Res Int. (2018) 109:52–8. doi: 10.1016/j.foodres.2018.04.013
44. Pernollet, J-C, and Briand, L. Structural recognition between odorants, olfactory‐binding proteins and olfactory receptors—first events in odour coding. Agr food Sci (2004). 86–150.
45. Jacobson, D.R., and Saleh, O.A. Quantifying the ion atmosphere of unfolded, single-stranded nucleic acids using equilibrium dialysis and single-molecule methods. Nucleic Acids Res (2016), 44, 3763–3771, doi: 10.1093/nar/gkw196
46. Wulkersdorfer, B, Wicha, SG, Kurdina, E, Carrion Carrera, SF, Matzneller, P, Al Jalali, V, et al. Protein binding of clindamycin in vivo by means of intravascular microdialysis in healthy volunteers. J Antimicrob Chemother. (2021) 76:2106–13. doi: 10.1093/jac/dkab140
47. Högstedt, A., Farnebo, S., Tesselaar, E., and Ghafouri, B. Investigation of proteins important for microcirculation using in vivo microdialysis after glucose provocation: a proteomic study. Sci Rep (2021), 11,:19093, doi: 10.1038/s41598-021-98672-8
48. Iftekhar, S, Ovbude, ST, and Hage, DS. Kinetic analysis by affinity chromatography. Front Chem. (2019) 7:673. doi: 10.3389/fchem.2019.00673
49. Li, Z., Kim, J., and Regnier, F.E. Mobile affinity sorbent chromatography. Anal Chem (2018), 90, 1668–1676, doi: 10.1021/acs.analchem.7b03117
50. Li, R, Chen, P, Zhang, N, and Chen, B. Study on the affinity characteristics of proteins on the immobilized metal affinity chromatography column. Anal Methods. (2019) 11:4341–7. doi: 10.1039/C9AY01469A
51. Ni, L, Lu, Q, Hou, H, Zhang, L, and Luan, S. Isolation of volatiles in honeysuckle by multiple approaches with determination by gas chromatography-mass spectrometry (GC-MS) and headspace solid-phase microextraction-gas chromatography-mass spectrometry (HS-SPME/GC-MS). Anal Lett. (2023) 56:2359–70. doi: 10.1080/00032719.2023.2168687
52. Qi, D, Zhou, Y, Wang, J, Fei, T, Wu, D, and Lu, J. Determination of volatiles in flue-cured tobacco by gas chromatography-mass spectrometry (GC-MS) with chemometrics. Anal Lett. (2022) 55:1398–411. doi: 10.1080/00032719.2021.2006681
53. Leyva-Porras, C., Cruz-Alcantar, P., Espinosa-Solis, V., Martinez-Guerra, E., Balderrama, C.I.P., Martinez, I.C., et al. Application of differential scanning calorimetry (DSC) and modulated differential scanning calorimetry (MDSC) in food and drug industries. Polymers (2019), 12,:5, doi: 10.3390/polym12010005
54. Linkuvienė, V, Zubrienė, A, and Matulis, D. Intrinsic affinity of protein—ligand binding by differential scanning calorimetry. Biochim Biophys Acta. (1870) 1870:140830. doi: 10.1016/j.bbapap.2022.140830
55. Lv, X, Wang, S, Zhao, Y, and Shan, P. A reinforcement learning based method for protein’s differential scanning calorimetry signal separation. Measurement. (2022) 188:110391. doi: 10.1016/j.measurement.2021.110391
56. Baranauskiene, L, Kuo, T-C, Chen, W-Y, and Matulis, D. Isothermal titration calorimetry for characterization of recombinant proteins. Curr Opin Biotechnol. (2019) 55:9–15. doi: 10.1016/j.copbio.2018.06.003
57. Vu, HN, Situ, AJ, and Ulmer, TS. Isothermal titration calorimetry of membrane proteins. In: I Schmidt-Krey and JC Gumbart, editors. Structure and function of membrane proteins. New York, NY: Springer (2021). 69–79.
58. Rodler, A., Beyer, B., Ueberbacher, R., Hahn, R., and Jungbauer, A. Hydrophobic interaction chromatography of proteins: studies of unfolding upon adsorption by isothermal titration calorimetry. J Sep Sci (2018), 41, 3069–3080, doi: 10.1002/jssc.201800016
59. Rogers, DM, Jasim, SB, Dyer, NT, Auvray, F, Réfrégiers, M, and Hirst, JD. Electronic circular dichroism spectroscopy of proteins. Chem. (2019) 5:2751–74. doi: 10.1016/j.chempr.2019.07.008
60. Micsonai, A, Wien, F, Kun, J, Vadászi, H, Réfrégiers, M, and Kardos, J. Protein fold recognition by circular dichroism spectroscopy. Biophys J. (2018) 114:174a. doi: 10.1016/j.bpj.2017.11.970
61. Li, Y, Zhang, M, Zheng, C, Hu, L, Wang, C, Jiang, J, et al. Analysis of silver-associated proteins in pathogen via combination of native SDS-PAGE, fluorescent staining, and inductively coupled plasma mass spectrometry. J Chromatogr A. (2019) 1607:460393. doi: 10.1016/j.chroma.2019.460393
62. Heckmeier, PJ, and Langosch, D. Site-specific fragmentation of green fluorescent protein induced by blue light. Biochemistry. (2021) 60:2457–62. doi: 10.1021/acs.biochem.1c00248
63. Bupp, CR, and Wirth, MJ. Making sharper peaks for reverse-phase liquid chromatography of proteins. Annu Rev Anal Chem. (2020) 13:363–80. doi: 10.1146/annurev-anchem-061318-115009
64. Kartanas, T, Levin, A, Toprakcioglu, Z, Scheidt, T, Hakala, TA, Charmet, J, et al. Label-free protein analysis using liquid chromatography with gravimetric detection. Anal Chem. (2021) 93:2848–53. doi: 10.1021/acs.analchem.0c04149
65. de Moraes, MC, Cardoso, CL, and Cass, QB Solid-supported proteins in the liquid chromatography domain to probe ligand-target interactions. Front Chem (2019), doi: 10.3389/fchem.2019.00752,:752, 7
66. Ayed, C, Martins, S, Williamson, AM, and Guichard, E. Understanding fat, proteins and saliva impact on aroma release from flavoured ice creams. Food Chem. (2018) 267:132–9. doi: 10.1016/j.foodchem.2017.10.127
67. Relkin, P, Fabre, M, and Guichard, E. Effect of fat nature and aroma compound hydrophobicity on flavor release from complex food emulsions. J Agric Food Chem. (2004) 52:6257–63. doi: 10.1021/jf049477a
68. Gong, H, Yang, Z, Liu, M, Shi, Z, Li, J, Chen, W, et al. Time-dependent categorization of volatile aroma compound formation in stewed Chinese spicy beef using electron nose profile coupled with thermal desorption GC-MS detection. Food Sci Human Wellness. (2017) 6:137–46. doi: 10.1016/j.fshw.2017.07.001
69. Song, H, and Liu, J. GC-O-MS technique and its applications in food flavor analysis. Food Res Int. (2018) 114:187–98. doi: 10.1016/j.foodres.2018.07.037
70. Zhao, J, Wang, M, Xie, J, Zhao, M, Hou, L, Liang, J, et al. Volatile flavor constituents in the pork broth of black-pig. Food Chem. (2017) 226:51–60. doi: 10.1016/j.foodchem.2017.01.011
71. Chen, X, Xu, X, Liu, D, Zhou, G, Han, M, and Wang, P. Rheological behavior, conformational changes and interactions of water-soluble myofibrillar protein during heating. Food Hydrocoll. (2018) 77:524–33. doi: 10.1016/j.foodhyd.2017.10.030
72. Boyer, C, Joandel, S, Roussilhes, V, Culioli, J, and Ouali, A. Heat-induced gelation of myofibrillar proteins and myosin from fast- and slow-twitch rabbit muscles. J Food Sci. (1996) 61:1138–43. doi: 10.1111/j.1365-2621.1996.tb10948.x
73. Sun, X, Saleh, ASM, Wang, Z, Yu, Y, Li, W, and Zhang, D. Insights into the interactions between etheric compounds and myofibrillar proteins using multi-spectroscopy, molecular docking, and molecular dynamics simulation. Food Res Int. (2024) 175:113787. doi: 10.1016/j.foodres.2023.113787
74. Wang, K, and Arntfield, SD. Effect of salts and pH on selected ketone flavours binding to salt-extracted pea proteins: the role of non-covalent forces. Food Res Int. (2015) 77:1–9. doi: 10.1016/j.foodres.2015.03.017
75. Zhang, W, Xiao, S, and Ahn, DU. Protein oxidation: basic principles and implications for meat quality. Crit Rev Food Sci Nutr. (2013) 53:1191–201. doi: 10.1080/10408398.2011.577540
76. Gu, S., Dai, W., Chong, Y., Lyu, F., Zhou, X., and Ding, Y. The binding of key fishy off-flavor compounds to silver carp proteins: a thermodynamic analysis. RSC Adv (2020), 10, 11292–11299, doi: 10.1039/d0ra01365j
77. Adams, RL, Mottram, DS, Parker, JK, and Brown, HM. Flavor-protein binding: disulfide interchange reactions between ovalbumin and volatile disulfides. J Agric Food Chem. (2001) 49:4333–6. doi: 10.1021/jf0100797
78. Shen, H, Zhao, M, and Sun, W. Effect of pH on the interaction of porcine myofibrillar proteins with pyrazine compounds. Food Chem. (2019) 287:93–9. doi: 10.1016/j.foodchem.2019.02.060
79. Shimizu, M, Saito, M, and Yamauchi, K. Emulsifying and structural properties of β-lactoglobulin at different pHs. Agric Biol Chem. (1985) 49:189–94. doi: 10.1271/bbb1961.49.189
80. Gianelli, MP, Flores, M, and Toldrá, F. Interaction of soluble peptides and proteins from skeletal muscle with volatile compounds in model systems as affected by curing agents. J Agric Food Chem. (2005) 53:1670–7. doi: 10.1021/jf040357c
81. Zhang, Z, Zhang, R, Zou, L, and McClements, DJ. Protein encapsulation in alginate hydrogel beads: effect of pH on microgel stability, protein retention and protein release. Food Hydrocoll. (2016) 58:308–15. doi: 10.1016/j.foodhyd.2016.03.015
82. Ventanas, S, Mustonen, S, Puolanne, E, and Tuorila, H. Odour and flavour perception in flavoured model systems: influence of sodium chloride, umami compounds and serving temperature. Food Qual Prefer. (2010) 21:453–62. doi: 10.1016/j.foodqual.2009.11.003
83. Rios-Mera, JD, da Silva Pinto, JS, and Contreras-Castillo, CJ. Effect of ultimate pH and ageing on thermal denaturation of bovine muscle proteins. Meat Sci. (2017) 131:25–7. doi: 10.1016/j.meatsci.2017.04.017
84. Li, Z, Grün, IU, and Fernando, LN. Interaction of vanillin with soy and dairy proteins in aqueous model systems: a thermodynamic study. J Food Sci. (2000) 65:997–1001. doi: 10.1111/j.1365-2621.2000.tb09406.x
85. Chen, H, Zhao, G, Yu, X, Zhang, Q, Zhu, C, Tong, L, et al. Exploring in vitro gastrointestinal digestion of myofibrillar proteins at different heating temperatures. Food Chem. (2023) 414:135694. doi: 10.1016/j.foodchem.2023.135694
86. Zhou, C, Jiang, Y, Cao, J, Wang, Y, Chen, Y, Pan, D, et al. The effects of heat treatment time on the absorption capacity of duck myofibrillar protein gels on flavor compounds. Mod Food Sci Technol. (2016) 32:218–24. doi: 10.13982/j.mfst.1673-9078.2016.3.035
87. Xu, Y, Wang, R, Zhao, H, Zhao, J, Li, X, Yi, S, et al. Binding of aldehydes to myofibrillar proteins as affected by two-step heat treatments. J Sci Food Agric. (2020) 100:1195–203. doi: 10.1002/jsfa.10130
88. Setyabrata, D, Cooper, BR, Sobreira, TJP, Legako, JF, Martini, S, and Kim, YHB. Elucidating mechanisms involved in flavor generation of dry-aged beef loins using metabolomics approach. Food Res Int. (2021) 139:109969. doi: 10.1016/j.foodres.2020.109969
89. Zhang, Y, Zhang, D, Huang, Y, Chen, L, Bao, P, Fang, H, et al. L-arginine and L-lysine degrade troponin-T, and L-arginine dissociates actomyosin: their roles in improving the tenderness of chicken breast. Food Chem. (2020) 318:126516. doi: 10.1016/j.foodchem.2020.126516
90. Li, C, Al-Dalali, S, Wang, Z, Xu, B, and Zhou, H. Investigation of volatile flavor compounds and characterization of aroma-active compounds of water-boiled salted duck using GC-MS-O, GC-IMS, and E-nose. Food Chem. (2022) 386:132728. doi: 10.1016/j.foodchem.2022.132728
91. Kühn, J, Considine, T, and Singh, H. Interactions of milk proteins and volatile flavor compounds: implications in the development of protein foods. J Food Sci. (2006) 71:R72–82. doi: 10.1111/j.1750-3841.2006.00051.x
92. Bosse, R, Wirth, M, Konstanz, A, Becker, T, Weiss, J, and Gibis, M. Determination of volatile marker compounds in raw ham using headspace-trap gas chromatography. Food Chem. (2017) 219:249–59. doi: 10.1016/j.foodchem.2016.09.094
93. Cao, JX, Zhou, CY, Wang, Y, Sun, YY, and Pan, DD. The effect of oxidation on the structure of G-actin and its binding ability with aroma compounds in carp grass skeletal muscle. Food Chem. (2018) 240:346–53. doi: 10.1016/j.foodchem.2017.07.068
94. Wang, K, and Arntfield, SD. Binding of selected volatile flavour mixture to salt-extracted canola and pea proteins and effect of heat treatment on flavour binding. Food Hydrocoll. (2015) 43:410–7. doi: 10.1016/j.foodhyd.2014.06.011
95. Hansen, AP, and Heinis, JJ. Benzaldehyde, citral, and d-limonene flavor perception in the presence of casein and whey proteins1. J Dairy Sci. (1992) 75:1211–5. doi: 10.3168/jds.S0022-0302(92)77869-2
96. Han, Z, Cai, MJ, Cheng, JH, and Sun, DW. Effects of microwave and water bath heating on the interactions between myofibrillar protein from beef and ketone flavour compounds. Int J Food Sci Technol. (2019) 54:1787–93. doi: 10.1111/ijfs.14079
97. Weerawatanakorn, M., Wu, J.C., Pan, M.H., and Ho, C.T. Reactivity and stability of selected flavor compounds. J Food Drug Anal (2015), 23, 176–190, doi: 10.1016/j.jfda.2015.02.001
98. Liu, L, Abdullah,, Tian, W, Chen, Μ, Huang, Y, and Xiao, J. Oral sensation and gastrointestinal digestive profiles of bigels tuned by the mass ratio of konjac glucomannan to gelatin in the binary hydrogel matrix. Carbohydr Polym. (2023) 312:120765. doi: 10.1016/j.carbpol.2023.120765
99. Jousse, F, Jongen, T, Agterof, W, Russell, S, and Braat, P. Simplified kinetic scheme of flavor formation by the Maillard reaction. J Food Sci. (2002) 67:2534–42. doi: 10.1111/j.1365-2621.2002.tb08772.x
100. Chaijan, M, Benjakul, S, Visessanguan, W, Lee, S, and Faustman, C. Effect of ionic strength and temperature on interaction between fish myoglobin and myofibrillar proteins. J Food Sci. (2007) 72:C89–95. doi: 10.1111/j.1750-3841.2006.00236.x
101. Zhang, J, Kang, D, Zhang, W, and Lorenzo, JM. Recent advantage of interactions of protein-flavor in foods: perspective of theoretical models, protein properties and extrinsic factors. Trends Food Sci Technol. (2021) 111:405–25. doi: 10.1016/j.tifs.2021.02.060
102. Wang, K, and Arntfield, SD. Effect of protein-flavour binding on flavour delivery and protein functional properties: a special emphasis on plant-based proteins. Flavour Fragr J. (2017) 32:92–101. doi: 10.1002/ffj.3365
103. Lou, X, Yang, Q, Sun, Y, Pan, D, and Cao, J. The effect of microwave on the interaction of flavour compounds with G-actin from grass carp (Catenopharyngodon idella). J Sci Food Agric. (2017) 97:3917–22. doi: 10.1002/jsfa.8325
104. Ma, Y.J., Wu, J.H., Li, X., Xu, X.B., Wang, Z.Y., Wu, C., et al. Effect of alkyl distribution in pyrazine on pyrazine flavor release in bovine serum albumin solution. RSC Adv (2019), 9, 36951–36959, doi: 10.1039/c9ra06720e
105. Yin, X, Gao, M, Wang, H, Chen, Q, and Kong, B. Probing the interaction between selected furan derivatives and porcine myofibrillar proteins by spectroscopic and molecular docking approaches. Food Chem. (2022) 397:133776. doi: 10.1016/j.foodchem.2022.133776
106. Menezes, TM, Barros, MR, Ventura, GT, De Sa Pires Ferreira, D, Todeschini, AR, Borges, RM, et al. Insights on the interaction of furfural derivatives with BSA and HTF by applying multi-spectroscopic and molecular docking approaches. J Mol Liq. (2020) 317:114021. doi: 10.1016/j.molliq.2020.114021
107. Xie, J, Sun, B, Zheng, F, and Wang, S. Volatile flavor constituents in roasted pork of mini-pig. Food Chem. (2008) 109:506–14. doi: 10.1016/j.foodchem.2007.12.074
108. Scatchard, G . The attractions of proteins for small molecules and ions. Ann N Y Acad Sci. (1949) 51:660–72. doi: 10.1111/j.1749-6632.1949.tb27297.x
109. Harrison, M, and Hills, BP. Mathematical model of flavor release from liquids containing aroma-binding macromolecules. J Agric Food Chem. (1997) 45:1883–90. doi: 10.1021/jf9607876
110. Mohammadzadeh-Aghdash, H, Ezzati Nazhad Dolatabadi, J, Dehghan, P, Panahi-Azar, V, and Barzegar, A. Multi-spectroscopic and molecular modeling studies of bovine serum albumin interaction with sodium acetate food additive. Food Chem. (2017) 228:265–9. doi: 10.1016/j.foodchem.2017.01.149
111. Li, X-H, Chavali, PL, and Babu, MM. Capturing dynamic protein interactions. Science. (2018) 359:1105–6. doi: 10.1126/science.aat0576
112. Arroyo-Maya, IJ, Campos-Teran, J, Hernandez-Arana, A, and McClements, DJ. Characterization of flavonoid-protein interactions using fluorescence spectroscopy: binding of pelargonidin to dairy proteins. Food Chem. (2016) 213:431–9. doi: 10.1016/j.foodchem.2016.06.105
113. Mahmoudpour, M, Javaheri-Ghezeldizaj, F, Yekta, R, Torbati, M, Mohammadzadeh-Aghdash, H, Kashanian, S, et al. Thermodynamic analysis of albumin interaction with monosodium glutamate food additive: insights from multi-spectroscopic and molecular docking approaches. J Mol Struct. (2020) 1221:128785. doi: 10.1016/j.molstruc.2020.128785
114. Ross, PD, and Subramanian, S. Thermodynamics of protein association reactions: forces contributing to stability. Biochemistry. (1981) 20:3096–102. doi: 10.1021/bi00514a017
115. Kang, DC, Zou, YH, Cheng, YP, Xing, LJ, Zhou, GH, and Zhang, WG. Effects of power ultrasound on oxidation and structure of beef proteins during curing processing. Ultrason Sonochem. (2016) 33:47–53. doi: 10.1016/j.ultsonch.2016.04.024
116. Tan, Y, and Siebert, KJ. Modeling bovine serum albumin binding of flavor compounds (alcohols, aldehydes, esters, and ketones) as a function of molecular properties. J Food Sci. (2008) 73:S56–63. doi: 10.1111/j.1750-3841.2007.00591.x
117. Pérez-Juan, M, Flores, M, and Toldrá, F. Effect of ionic strength of different salts on the binding of volatile compounds to porcine soluble protein extracts in model systems. Food Res Int. (2007) 40:687–93. doi: 10.1016/j.foodres.2006.11.013
118. Shen, H, Huang, M, Zhao, M, and Sun, W. Interactions of selected ketone flavours with porcine myofibrillar proteins: the role of molecular structure of flavour compounds. Food Chem. (2019) 298:125060. doi: 10.1016/j.foodchem.2019.125060
119. Morris, CJ, and Corte, DD. Using molecular docking and molecular dynamics to investigate protein-ligand interactions. Mod Phys Lett B. (2021) 35:2130002. doi: 10.1142/S0217984921300027
120. Ren, W., Dokainish, H.M., Shinobu, A., Oshima, H., and Sugita, Y. Unraveling the coupling between conformational changes and ligand binding in ribose binding protein using multiscale molecular dynamics and free-energy calculations. J Phys Chem B (2021), 125, 2898–2909, doi: 10.1021/acs.jpcb.0c11600
Keywords: myofibrillar protein, flavor substance, binding interaction, volatile flavor, influencing factor
Citation: Qian R, Sun C, Bai T, Yan J, Cheng J and Zhang J (2024) Recent advances and challenges in the interaction between myofibrillar proteins and flavor substances. Front. Nutr. 11:1378884. doi: 10.3389/fnut.2024.1378884
Received: 30 January 2024; Accepted: 15 April 2024;
Published: 25 April 2024.
Edited by:
Chao-Hui Feng, Kitami Institute of Technology, JapanReviewed by:
Zongshuai Zhu, Henan Institute of Science and Technology, ChinaCopyright © 2024 Qian, Sun, Bai, Yan, Cheng and Zhang. This is an open-access article distributed under the terms of the Creative Commons Attribution License (CC BY). The use, distribution or reproduction in other forums is permitted, provided the original author(s) and the copyright owner(s) are credited and that the original publication in this journal is cited, in accordance with accepted academic practice. No use, distribution or reproduction is permitted which does not comply with these terms.
*Correspondence: Jie Cheng, Y2hlbmdqaWVAY2R1LmVkdS5jbg==; Jiamin Zhang, emhhbmdqaWFtaW5AY2R1LmVkdS5jbg==
Disclaimer: All claims expressed in this article are solely those of the authors and do not necessarily represent those of their affiliated organizations, or those of the publisher, the editors and the reviewers. Any product that may be evaluated in this article or claim that may be made by its manufacturer is not guaranteed or endorsed by the publisher.
Research integrity at Frontiers
Learn more about the work of our research integrity team to safeguard the quality of each article we publish.