- School of Life Sciences, Technical University of Munich, Freising, Germany
Because of the indispensable amino acids dietary proteins are the most important macronutrients. Proper growth and body maintenance depends on the quantity and quality of protein intake and proteins have thus been most crucial throughout evolution with hominins living in quite diverse food ecosystems. Developments in agriculture and food science have increased availability and diversity of food including protein for a rapidly growing world population while nutrient deficiencies resulting in stunting in children for example have been reduced. Nevertheless, the developing world and growing population needs more protein of high quality – with around 400 million tons per annum estimated for 2050. In contrary, protein consumption in all developed countries exceeds meanwhile the recommended intakes considerably with consequences for health and the environment. There is a growing interest in dietary proteins driven by the quest for more sustainable diets and the increasing food demand for a growing world population. This brings new and novel sources such as algae, yeast, insects or bacteria into play in delivering the biomass but also new technologies such as precision fermentation or in vitro meat/fish or dairy. What needs to be considered when such new protein sources are explored is that proteins need to provide not only the required amino acids but also functionality in the food produced thereof. This review considers human physiology and metabolism in the context of protein intake from an evolutionary perspective and prospects on future protein production.
Introduction
Whereas carbohydrates and lipids received over time enormous public interest (sugar tax, low carb, low fat etc.), only recently have proteins experienced a similar public perception. This is fostered by popular press referring to higher protein intakes to help weight loss but also by the wide use of protein supplements in the fitness and bodybuilding scene that often suggest that just eating more protein provides a proper body shape. Markets have seen the emergence of products in almost all food categories with added protein to satisfy this protein-hype. With the high consumption rate of animal-based products and a large array of food items with higher protein contents, the daily protein intake exceeds already the recommended intake levels in all developed countries substantially.
With the awareness of an increasing food demand for the growing world population on background of climate change and expected reductions in agricultural yields and available land area, the interest in proteins from new sources increased drastically. The quest for more sustainable diets in which meat and animal-based food items are replaced has fostered the academic interest in “new proteins” and many research activities have been launched in recent years. These are often embedded into national strategic initiatives to make food systems more resilient and less dependent on imports. While the agri-food sector currently focuses on protein-plants, improved yields and better climate-adopted plant varieties, the biotech sector provides new avenues for food production with renewable energy used to generate hydrogen, acetate or methanol that can serve as energy substrates for biomass production (1, 2). Novel organisms such as chemolithotrophic bacteria have already proven to provide sufficient biomass for protein production by using hydrogen as energy source and by trapping CO2 from air (3). Cell culture techniques with stem cells as starters are in development to preplace conventional meat and fish products while “precision fermentation” with recombinant expression of target proteins in production strains of bacteria or yeast is used to provide for example dairy products based on bovine caseins or whey proteins by heterologous expression.
With the framing of “alternative proteins” we are thus exposed to a huge spectrum of approaches and numerous novel technologies that require the assessment of the safety and the nutritional and environmental quality of the new food items. It is the intension of the present review to provide a thoughtful reflection on the role of dietary proteins in human nutrition by taking an evolutionary perspective and by considering the new protein sources as part of future diets.
Proteins in the evolution of hominins
There can be no doubt that amongst the macronutrients, proteins with the essential amino acids are most crucial diet components in mammalian development. Whereas carbohydrates do not provide any essential entities, lipids have two fatty acids categorized as essential while there are 9 indispensable amino acids. Growth and development require these amino acids but their concentration in food items does not per se provide the best spectrum and in particular many plant proteins have individual amino acids in limited quantities. Therefore, combining plant foods to complement the amino acid profiles in the contained proteins is a principle and is realized in many kitchens all over the planet in which a few staple foods are available. This ensures that even with low overall protein contents in the diet optimal conditions for growth and development are realized. In contrast to plants, most animal food sources have amino acid patterns close to those of humans and thus those proteins have played an important role in human development. Although there is a long-lasting discussion on whether hominins evolved as carnivores or vegetarians or even frugivores, it has become obvious that depending on time and geographical location and climate, our ancestors have been consuming whatever the ecosystem provided and that was usually a mix of plant and animal-based products. In this respect it is misleading when argued that there is something as a “paleo-diet.” A new interest in diets of hunters and gatherers emerged in recent years in the biomedical scene when microbiome profiling of stools samples of isolated populations or even of paleosamples revealed that the diversity of gut microbial species was much higher than that found in samples from individuals living in industrialized countries. This is frequently explained with more diverse diets than those of modern times. However, stool analysis of paleosamples (4) but also stool samples collected from people living in rural areas in central Africa (5) showed a high density of parasites and this even correlated with microbiome diversity. This adaptation of bacterial diversity to parasites seems to be a more common phenomenon also in other species (6).
In the Hazda hunter and gatherer society careful analysis of diets – that vary considerably by season – revealed that they have periods in which up to half of their caloric intake comes from honey whereas in other month´ and season up to 65% of daily calorie consumption derives from animal products (7). Such diet patterns we usually consider as most unhealthy but strikingly, Hazda people show low incidence of non-communicable diseases such as cardiovascular diseases (8). But these modern hunter and gatherer groups also provide a good example of how variable human diets have been and can be and how much those depend on the ecosystem setting.
As a more general finding it has been observed that the percentage of animal products as part of diets increases with a more northern latitude (7). For central and northern Europe that also applies to diets in early hominins. In remains of neanderthal species (age around 40.000 years) and other palaeolithic humans living in Europe, isotope analysis of 15N in bone collagen revealed a signature that was even higher than that of pure carnivore species of the same regions suggesting that early humans had a very high rate of animal protein in the respective diets (9). The migration from a rather warm savanna ecosystem into a cold tundra-like landscape in northern Europe and Asia (see Figure 1) was likely associated with a marked change in diet and a high animal protein and fat consumption. The high protein quality of meat was clearly beneficial for development and that was even further enhanced when fire was introduced for food processing estimated as to happened about a million years ago. Raw diets consumed by humans are known to provide insufficient energy and nutrients leading to impairments in the female cycle or even loss of reproductive function (10). Heat treatment of food increases significantly the energy that can be absorbed from the small intestine (11, 12) and this is thought to have changed the anatomy and morphology of the gastrointestinal tract with markedly reduced mass relative to total body mass – leaving more nutrients and energy for brain development and overall body size. With the neolithic revolution and a more constant food supply by farming and animal husbandry, diets may have shifted to a higher proportion of plants while animals still delivered a considerable fraction of protein and fat. Domestication brought new protein sources such as bovine milk into the diet that was driving the adaptaion of humans to intestinal lactase persistance.
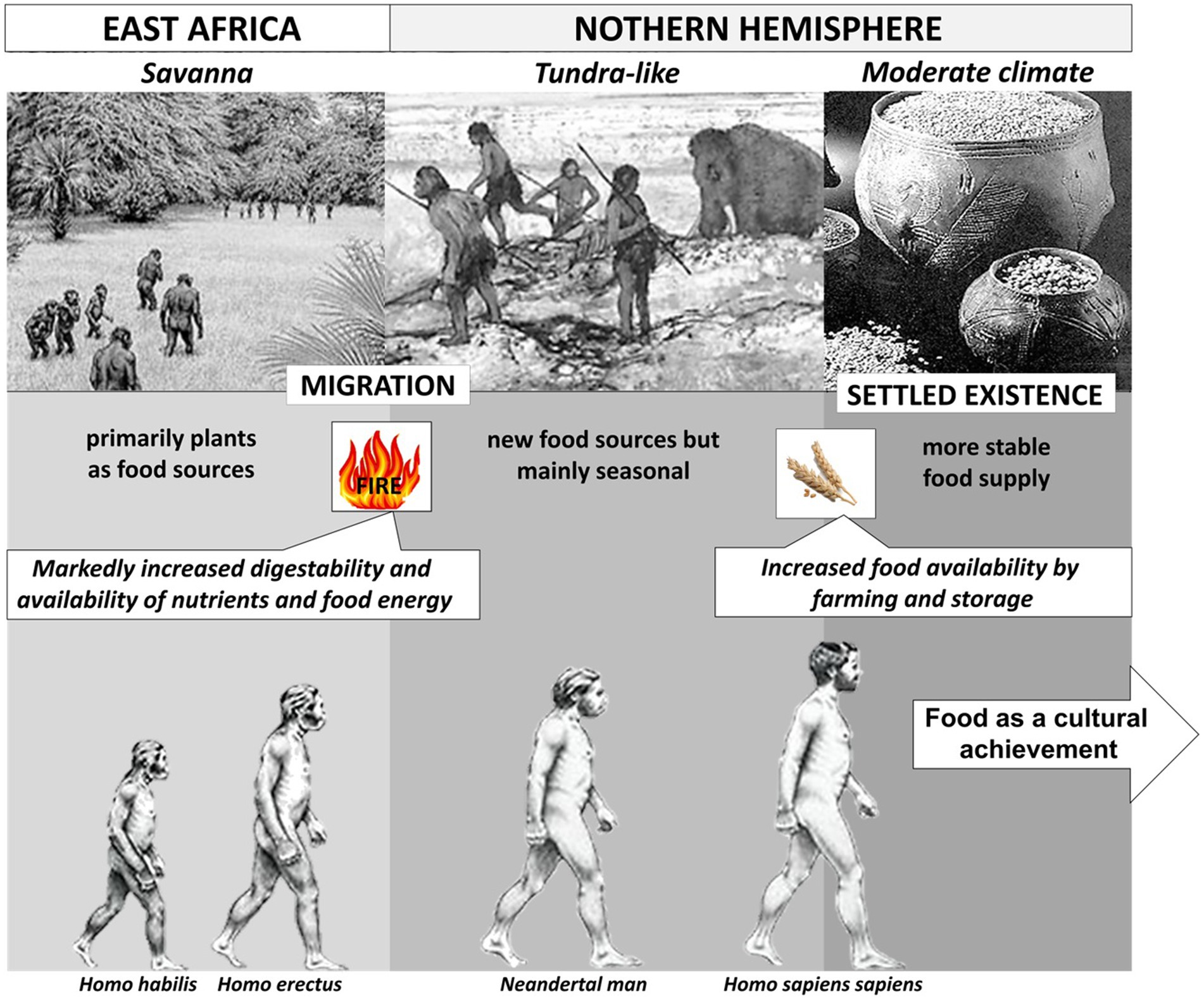
Figure 1. Milestones in the evolution of hominins and the role of diet as derived from different ecosystems.
Most strikingly, daily protein intake across the world correlates strongly with average male and female body height and even with the phenotype frequency of lactose tolerance (13) suggesting that the high biological quality of dairy protein introduced in the neolithic period also promotes extra growth. Taken together, diets of hominins throughout evolution varied considerably by time, geography, season and ecosystem. Protein supply as well was highly variable but with the indispensable amino acids their contribution of the development of Homo sapiens sapiens has been most important for growth and development and similarly, food processing techniques that improved supply and digestibility as well shaped human physiology.
Proteins in the diet-health relationship
The quantity and quality of dietary proteins has been a key determinant in human development over millennia. Whereas a low protein supply is still a large problem in many low-income countries, daily protein intakes in developed countries exceed meanwhile significantly the recommended intakes (14). In addition, protein quality is no more a critical factor as food availability is unrestricted and with the enormous number of food items – available around the year – and with a high percentage of animal-based product this high protein supply status is just a measure of wealth without a need. Protein intakes are around 90 to 100 g per day in man and around 70 to 75 g per day in woman (14). Required minimum protein intakes when providing high quality proteins to maintain an equilibrium in humans have been estimated to range between 0.3 and 0.5 g/kg body weight/day with the medium requirement defined as 0.66 g leading to a recommended intake of 0.8 or 0.83 g/kg/day as safe population intake in adults and of 1.05 g/kg/day for senior citizens (15). Total protein synthesis in an adult is around 300 g per day with muscle, liver and renewal of circulating blood cells accounting for most of the newly synthesized protein quantities. There is a constant protein breakdown leading to release of free amino acids into a pool in which dietary amino acids enter as well with this pool serving as the resource for de novo protein synthesis (see Figure 2). When amino acid intake exceeds the needs, amino acids need to be used as energy substrates leaving nitrogen and sulphur for excretion – mainly via urine.
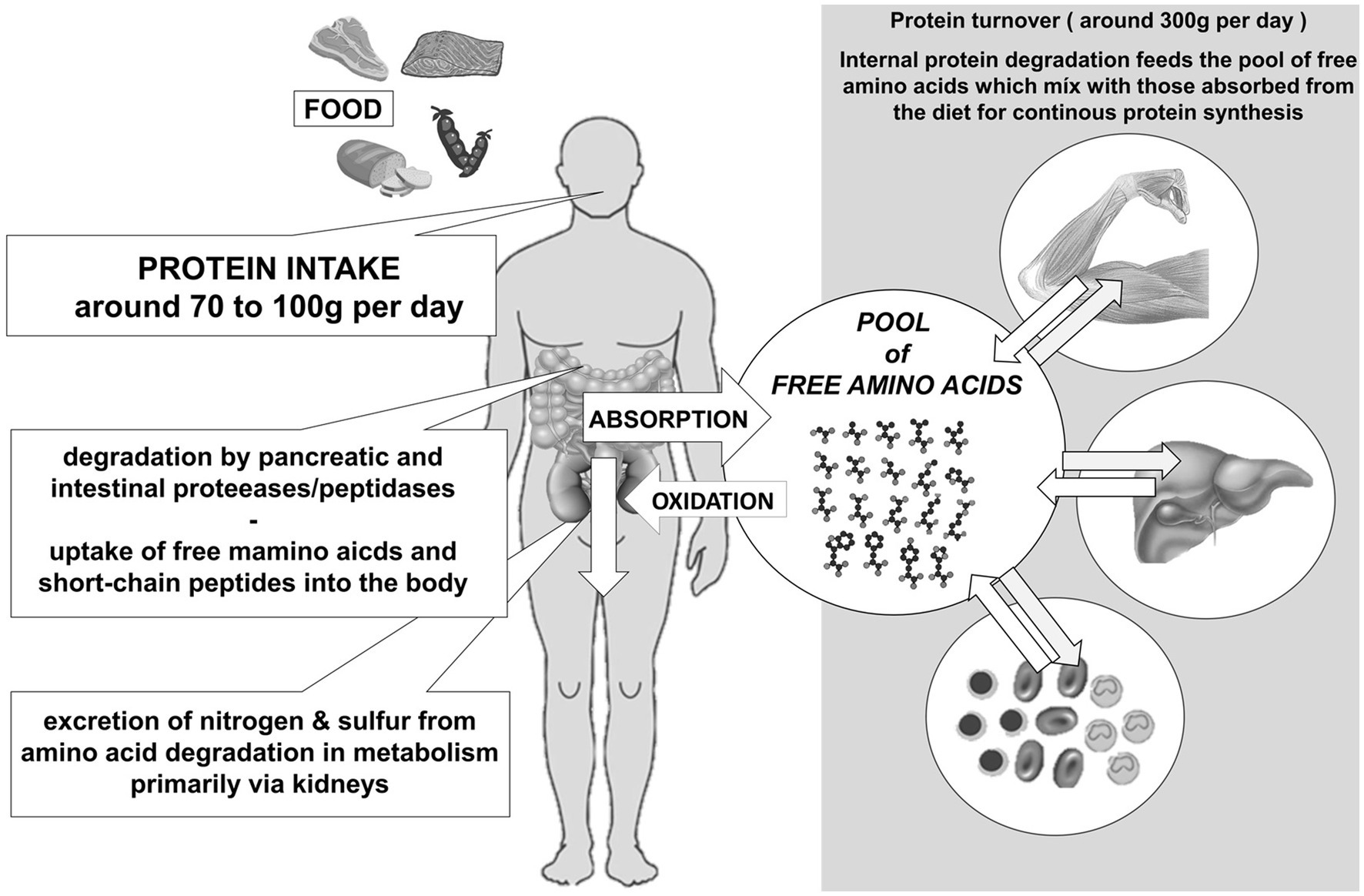
Figure 2. Fate of dietary proteins and turnover of body protein and utilisation of amino acids (per day) in adult humans.
High protein intakes in high income countries that exceed recommended intake by around 20 to 40 g per individual and day, require that amino acids are immediately oxidised as the capacity for storage of a surplus of amino acids is very limited. Amino acid oxidation leaves nitrogen and sulphur for detoxification which also results in a substantial flux of these entities through the large intestine with effects on the microbiome and not yet adequately defined health consequences (16, 17). Moreover, the surplus of protein with the excess of nitrogen that needs to be excreted has been identified as a problem for the environment (18).
Recently markets have seen a real “protein hype” with many products enriched with an extra portion of protein which further increases total daily protein intake above needed and recommended quantities. This trend is mainly based on the concept that high protein diets reduce caloric intake by a higher satiety signal and thus promote weight loss (19). Indeed, in randomised controlled trials (RCTs) with energy-restricted diets, high protein intake (27–35% of energy intake) provided a greater weight loss (of around 0.8 to 1.2 kg over 1-to-3-month periods) than low protein diets (20). But, meta-analysis of similar RCTs not always confirm such an effect (21) and it seems that as longer the study lasts, as smaller the effects of the high protein diets become (22). In RCTs with non-energy-restricted diets effects on body weight were even smaller with 0.36 kg in >1 month study periods as an example (23) In addition to weight-loss as an endpoint, meta-analysis of many RCTs employing high protein diets could not demonstrate any (21) or only very small beneficial metabolic effects – except for modest reductions in blood triglyceride levels (20, 23). Taken together, long-term effects of high protein diets for improved weight management are scarce and small (19). Benefits of high protein products and diets claimed in the public domain thus often overstate the effect sizes.
Like never before has health become the most crucial criterion in assessing the impact of diets, individual nutrients or even individual food items or any ingredient. In view of the association of protein quantity and protein origin (plant-based or animal product-based) with various disease end-points and all-cause mortality as derived from observational studies it may be concluded that daily protein intakes in the range of up to 30% of energy intake are save with no significant risks for diseases such as cancers or cardiovascular disease (24). If specifically assessed for plant-based or animal-based proteins, it becomes obvious that a high intake of animal protein (up to 20% of energy intake) is associated with a modest increase in disease risks and increased all-cause mortality. This is mainly driven by red meat and processed red meat for which disease-associations have been demonstrated to be dose-dependent (25, 26). Plant-proteins on the contrary appear to have protective effects for most disease endpoints (26).
High protein consumption has for a long time been linked to negative effects on bone-health based on a high production of sulfuric acid when sulphur-containing amino acids are oxidised in metabolism (27, 28). However, recent meta-analysis and umbrella reviews conclude that even with protein intake rates exceeding 1.5 g/kg body weight per day bone mineral density or osteoporosis risk are not impaired – neither in younger nor in older individuals (29). That provides a safe basis for recommendations towards higher protein intakes in senior citizens to prevent or fight sarcopenia (29). Taken together, there is a large body of evidence that higher protein intakes (up to 30% of energy) are safe with some evidence that intake of red meat and processed red meat but not of other meat varieties nor plant proteins increase disease risk (for diabetes, certain cancers, CVD) and all-cause mortality (30).
When we assess environmental footprints of diets or of individual food items, we have an issue with protein intake rates exceeding those recommended. When amino acid intake exceeds the quantity needed for body protein synthesis, amino acids are immediately oxidised and that leaves large quantities of nitrogen for excretion. Urea and ammonia as end-products of this energetic utilisation of the surplus of amino acids are excreted in urine and faeces and this is a burden for the environment. A recent analysis of this nitrogen flow resulting form high protein diets exceeding recommended intake rates estimated for the US (18) a contribution of 28% of all N-emissions into the environment and mainly into the surface water. According to the FAO Statistical Year Book (31) the mean protein supply per day in the US and Europe is similar and N-emissions derived from the surplus of protein intake should therefore be very similar.
New proteins with new technologies
There can be no doubt that the growing world population needs more protein and that is frequently used as the key argument for initiatives to isolate proteins from any source and with new biomass production approaches employing novel organisms and technologies. But the growing number of humans also need calories and even more so essential micronutrients such as vitamins and trace elements. Based on a model analysis it was concluded that the world produces already enough protein to satisfy the needs of 10 billion people by 2050 – when protein could be distributed evenly (32) whereas micronutrient supply will remain as most critical for billions of people. Iron-deficiency in particular is currently the most important individual nutrient deficiency leading to anaemia in around 500 million woman (31) and providing sufficient dietary iron will remain as one of the key problems in feeding the growing population.
Although there are many new sources for proteins such as micro-and macroalgae like spirulina, chlorella or kelp, mycoproteins, various insect species or new legume varieties (32), none of those sources deliver all essential nutrients that humans need (33). And when used as protein isolates or concentrates, they usually contain also some anti-nutritive compounds such as phytates, protease inhibitors, lectins or other unwanted compounds. Plant-based diets are known to reduce mineral and trace-element bioavailability and that has recently also been raised as concern for the so called “planetary health diet” as a model for future diets with minimal effects on environment but maximal effects on health (34). Moreover, plant-based protein blends (35) or burger patty substitutes (36) were shown to generate lower plasma levels of essential amino acids (EAAS) in postprandial states when provided in identical quantities as the animal-based reference protein. Although human studies in young healthy volunteers ingesting plant-based proteins or protein blends also revealed lower plasma levels of EAAS, differences in post-prandial protein synthesis could not be observed (37). However, in elderly in which postprandial protein synthesis is most important, a lower synthesis rate based on plant-protein rich food may be a problem (35, 38).
That means that when those protein sources are used in food production – mainly for replacing meat or dairy products – essential nutrients such as vitamins, minerals and trace elements need to be added to new food items created. That brings new products into the categories of so called ultraprocessed food (39). The growing consumer demands for plant-based replacers of meat, meat products and dairy has initiated a wide range of activities that go from protein isolation from side-streams or utilisation of new sources such as gras or hemp, to insects, mushroom-mycelium or novel production organisms such as chemo-lithotrophic bacteria.
What is not often considered is the functionality of the protein-concentrates and-isolates (PCIs) when used in production of new food items. Proteins provide many features required in food technology such as solubility, coagulation, emulsification, binding, foaming, gelation or whipping. And most importantly, products based on PCIs need to provide good taste or at least should not bring in off-flavours. Functionality of the various protein preparations is key for market success and that fosters studies that take plant PCIs and test their functional features.
An alternative route could be the synthesis of proteins with a defined functionality via biotechnology. With a better understanding of how amino acid sequence and structure of individual proteins translate into functionality, these proteins can be produced in large scale via expression in yeast – favourably via the methylotrophic yeast Pichia pastoris (40). Currently such proteins are produced as modular structures with known functionalities that mimic natural proteins such as collagen. They display interesting technological features such as undergoing temperature-or pH-dependent transitions between liquid and gel states (40). Other examples may be antifreeze-proteins that are employed in certain food categories such as ice-cream (41) or sweet-tasting proteins such as Thaumatin, Brazzein or Monellin (42).
Life science has generated over the last decades functional proteins as tools in cell biology that allow life cell-imaging for example or deliver/capture ligands upon activation by exposure to a defined wavelength of light. Such “protein cages” (43) could as well (given their safety) find numerous applications in food technology but are currently a science fiction domain. However, with the rapid development in artificial intelligence (AI) tools that predict 3D-protein structures from any given sequence and forecasting functional properties, the generation of in silico amino acid sequences for de novo synthesis of such novel proteins of a defined function may also become an option for food biotech applications of tomorrow.
Author contributions
HD: Conceptualization, Data curation, Formal analysis, Funding acquisition, Investigation, Methodology, Project administration, Resources, Software, Supervision, Validation, Visualization, Writing – original draft, Writing – review & editing.
Funding
The author(s) declare that no financial support was received for the research, authorship, and/or publication of this article.
Conflict of interest
The author declares that the research was conducted in the absence of any commercial or financial relationships that could be construed as a potential conflict of interest.
Publisher’s note
All claims expressed in this article are solely those of the authors and do not necessarily represent those of their affiliated organizations, or those of the publisher, the editors and the reviewers. Any product that may be evaluated in this article, or claim that may be made by its manufacturer, is not guaranteed or endorsed by the publisher.
References
1. Hann, EC , Overa, S , Harland-Dunaway, M , Narvaez, AF , Le, DN , Orozco-Cárdenas, ML, et al. A hybrid inorganic-biological artificial photosynthesis system for energy-efficient food production. Nat Food. (2022) 3:461–71. doi: 10.1038/s43016-022-00530-x
2. Leger, D , Matassa, S , Noor, E , Shepon, A , Milo, R , and Bar-Even, A . Photovoltaic-driven microbial protein production can use land and sunlight more efficiently than conventional crops. Proc Natl Acad Sci USA. (2021) 118, 118:e2015025118. doi: 10.1073/pnas.2015025118
3. Nyyssölä, A , Ojala, LS , Wuokko, M , Peddinti, G , Tamminen, A , Tsitko, I, et al. Production of endotoxin-free microbial biomass for food applications by gas fermentation of gram-positive H2-oxidizing Bacteria. ACS Food Sci Technol. (2021) 1:470–9. doi: 10.1021/acsfoodscitech.0c00129
4. Wibowo, MC , Yang, Z , Borry, M , Hübner, A , Huang, KD , Tierney, BT, et al. Reconstruction of ancient microbial genomes from the human gut. Nature. (2021) 594:234–9. doi: 10.1038/s41586-021-03532-0
5. Rubel, MA , Abbas, A , Taylor, LJ , Connell, A , Tanes, C , Bittinger, K, et al. Lifestyle and the presence of helminths is associated with gut microbiome composition in Cameroonians. Genome Biol. (2020) 21:122. doi: 10.1186/s13059-020-02020-4
6. Loke, P , and Harris, NL . Networking between helminths, microbes, and mammals. Cell Host Microbe. (2023) 31:464–71. doi: 10.1016/j.chom.2023.02.008
7. Pontzer, H , and Wood, BM . Effects of evolution, ecology, and economy on human diet: insights from hunter-gatherers and other small-scale societies. Annu Rev Nutr. (2021) 41:363–85. doi: 10.1146/annurev-nutr-111120-105520
8. Pontzer, H , Wood, BM , and Raichlen, DA . Hunter-gatherers as models in public health. Obes Rev. (2018) 19:24–35. doi: 10.1111/obr.12785
9. Lee-Thorp, J , and Sponheimer, M . Contributions of biogeochemistry to understanding hominin dietary ecology. Yearb Phys Anthropol. (2006) 49:131–48. doi: 10.1002/ajpa.20519
10. Koebnick, C , Strassner, C , Hoffmann, I , and Leitzmann, C . Consequences of a long-term raw food diet on body weight and menstruation: results of a questionnaire survey. Ann Nutr Metab. (1999) 43:69–79. doi: 10.1159/000012770
11. Groopman, EE , Carmody, RN , and Wrangham, RW . Cooking increases net energy gain from a lipid-rich food. Am J Phys Anthropol. (2015) 156:11–8. doi: 10.1002/ajpa.22622
12. Carmody, RN , Weintraub, GS , and Wrangham, RW . Energetic consequences of thermal and nonthermal food processing. Proc Natl Acad Sci USA. (2011) 108:19199–203. doi: 10.1073/pnas.1112128108
13. Grasgruber, P , and Hrazdíra, E . Nutritional and socio-economic predictors of adult height in 152 world populations. Econ Hum Biol. (2020) 37:100848. doi: 10.1016/j.ehb.2020.100848
14. Dietary Protein . Overview of protein intake in European countries. Dataset, EU commission, Science Hub (2021). Available at: https://knowledge4policy.ec.europa.eu/health-promotion-knowledge-gateway/dietary-protein-overview-countries-6_en
15. Millward, DJ . Identifying recommended dietary allowances for protein and amino acids: a critique of the 2007 WHO/FAO/UNU report. Br J Nutr. (2012) 108:S3–S21. doi: 10.1017/S0007114512002450
16. Yao, CK , Muir, JG , and Gibson, PR . Insights into colonic protein fermentation, its modulation and potential health implications. Aliment Pharmacol Ther. (2016) 43:181–96. doi: 10.1111/apt.13456
17. Windey, K , De Preter, V , and Verbeke, K . Relevance of protein fermentation to gut health. Mol Nutr Food Res. (2012) 56:184–96. doi: 10.1002/mnfr.201100542
18. Almaraz, M , Kuempel, CD , Salter, AM , and Halpern, BS . The impact of excessive protein consumption on human wastewater nitrogen loading of US waters. Front Ecol Environment. (2022) 20:8, 452–58. doi: 10.1002/fee.2531
19. Leidy, HJ , Clifton, PM , Astrup, A , Wycherley, TP , Westerterp-Plantenga, MS , Luscombe-Marsh, ND, et al. The role of protein in weight loss and maintenance. Am J Clin Nutr. (2015) 101:1320S–9S. doi: 10.3945/ajcn.114.084038
20. Wycherley, TP , Moran, LJ , Clifton, PM , Noakes, M , and Brinkworth, GD . Effects of energy-restricted high-protein, low-fat compared with standard-protein, low-fat diets: a meta-analysis of randomized controlled trials. Am J Clin Nutr. (2012) 96:1281–98. doi: 10.3945/ajcn.112.044321
21. Schwingshackl, L , and Hoffmann, G . Long-term effects of low-fat diets either low or high in protein on cardiovascular and metabolic risk factors: a systematic review and meta-analysis. Nutr J. (2013) 12:48. doi: 10.1186/1475-2891-12-48
22. Magkos, F . The role of dietary protein in obesity. Rev Endocr Metab Disord. (2020) 21:329–40. doi: 10.1007/s11154-020-09576-3
23. Santesso, N , Akl, EA , Bianchi, M , Mente, A , Mustafa, R , Heels-Ansdell, D, et al. Effects of higher-versus lower-protein diets on health outcomes: a systematic review and meta-analysis. Eur J Clin Nutr. (2012) 66:780–8. doi: 10.1038/ejcn.2012.37
24. Naghshi, S , Sadeghi, O , Willett, WC , and Esmaillzadeh, A . Dietary intake of total, animal, and plant proteins and risk of all cause, cardiovascular, and cancer mortality: systematic review and dose-response meta-analysis of prospective cohort studies. BMJ. (2020) 370:m2412. doi: 10.1136/bmj.m2412
25. Lescinsky, H , Afshin, A , Ashbaugh, C , Bisignano, C , Brauer, M , Ferrara, G, et al. Health effects associated with consumption of unprocessed red meat: a burden of proof study. Nat Med. (2022) 28:2075–82. doi: 10.1038/s41591-022-01968-z
26. Stanaway, JD , Afshin, A , Ashbaugh, C , Bisignano, C , Brauer, M , Ferrara, G, et al. Health effects associated with vegetable consumption: a burden of proof study. Nat Med. (2022) 28:2066–74. doi: 10.1038/s41591-022-01970-5
27. Shams-White, MM , Chung, M , Du, M , Fu, Z , Insogna, KL , Karlsen, MC, et al. Dietary protein and bone health: a systematic review and meta-analysis from the National Osteoporosis Foundation. Am J Clin Nutr. (2017) 105:1528–43. doi: 10.3945/ajcn.116.145110
28. Darling, AL , Millward, DJ , and Lanham-New, SA . Dietary protein and bone health: towards a synthesised view. Proc Nutr Soc. (2021) 80:165–72. doi: 10.1017/S0029665120007909
29. Zittermann, A , Schmidt, A , Haardt, J , Kalotai, N , Lehmann, A , Egert, S, et al. Protein intake and bone health: an umbrella review of systematic reviews for the evidence-based guideline of the German nutrition society. Osteoporos Int. (2023) 34:1335–53. doi: 10.1007/s00198-023-06709-7
30. Schwingshackl, L , Schwedhelm, C , Hoffmann, G , Lampousi, AM , Knüppel, S , Iqbal, K, et al. Food groups and risk of all-cause mortality: a systematic review and meta-analysis of prospective studies. Am J Clin Nutr. (2017) 105:1462–73. doi: 10.3945/ajcn.117.153148
31. FAO, IFAD, UNICEF, WFP and WHO . The state of food security and nutrition in the world (SOFI) (2023). Food and Agriculture Organziation (FAO) of the United Nations (UN).
32. Smith, NW , Fletcher, AJ , Dave, LA , Hill, JP , and McNabb, WC . Use of the DELTA model to understand the food system and global nutrition. J Nutr. (2021) 151:3253–61. doi: 10.1093/jn/nxab199
33. Parodi, A , Leip, A , De Boer, IJM , Slegers, PM , Ziegler, F , Temme, EHM, et al. The potential of future foods for sustainable and healthy diets. Nat Sustain. (2018) 1:782–9. doi: 10.1038/s41893-018-0189-7
34. Beal, T , Ortenzi, F , and Fanzo, J . Estimated micronutrient shortfalls of the EAT-lancet planetary health diet. Lancet Planet Health. (2023) 7:e233–7. doi: 10.1016/S2542-5196(23)00006-2
35. de Marco Castro, E , Valli, G , Buffière, C , Guillet, C , Mullen, B , Pratt, J, et al. Peripheral amino acid appearance is lower following plant protein fibre products, compared to whey protein and fibre ingestion, in healthy older adults despite optimised amino acid profile. Nutrients. (2022) 15:35. doi: 10.3390/nu15010035
36. Pham, T , Knowles, S , Bermingham, E , Brown, J , Hannaford, R , Cameron-Smith, D, et al. Plasma amino acid appearance and status of Appetite following a single meal of red meat or a plant-based meat analog: a randomized crossover clinical trial. Curr Dev Nutr. (2022) 6:nzac082. doi: 10.1093/cdn/nzac082
37. Pinckaers, PJM , Kouw, IWK , Gorissen, SHM , Houben, LHP , Senden, JM , Wodzig, WKHW, et al. The muscle protein synthetic response to the ingestion of a plant-derived protein blend does not differ from an equivalent amount of Milk protein in healthy young males. J Nutr. (2023) 152:2734–43. doi: 10.1093/jn/nxac222
38. Berrazaga, I , Micard, V , Gueugneau, M , and Walrand, S . The role of the anabolic properties of plant-versus animal-based protein sources in supporting muscle mass maintenance: a critical review. Nutrients. (2019) 11:1825. doi: 10.3390/nu11081825
39. Flint, M , Bowles, S , Lynn, A , and Paxman, JR . Novel plant-based meat alternatives: future opportunities and health considerations. Proc Nutr Soc. (2023) 82:370–85. doi: 10.1017/S0029665123000034
40. Werten, MWT , Eggink, G , Cohen, SMA , and de Wolf, FA . Production of protein-based polymers in Pichia pastoris. Biotechnol Adv. (2019) 37:642–66. doi: 10.1016/j.biotechadv.2019.03.012
41. Baskaran, A , Kaari, M , Venugopal, G , Manikkam, R , Joseph, J , and Bhaskar, PV . Anti freeze proteins (Afp): properties, sources and applications – a review. Int J Biol Macromol. (2021, 2021) 189:292–305. doi: 10.1016/j.ijbiomac.2021.08.105
42. Zhao, X , Wang, C , Zheng, Y , and Liu, B . New insight into the structure-activity relationship of sweet-tasting proteins: protein sector and its role for sweet properties. Front Nutr. (2021) 8:691368. doi: 10.3389/fnut.2021.691368
Keywords: diet, new proteins, physiology, food biotechnology, evolution
Citation: Daniel H (2024) Dietary proteins: from evolution to engineering. Front. Nutr. 11:1366174. doi: 10.3389/fnut.2024.1366174
Edited by:
Giuseppe Poli, Department of Clinical and Biological Sciences, ItalyReviewed by:
I. Sam Saguy, The Hebrew University of Jerusalem, IsraelFederico Canzoneri, Soremartec Italia Srl, Italy
Copyright © 2024 Daniel. This is an open-access article distributed under the terms of the Creative Commons Attribution License (CC BY). The use, distribution or reproduction in other forums is permitted, provided the original author(s) and the copyright owner(s) are credited and that the original publication in this journal is cited, in accordance with accepted academic practice. No use, distribution or reproduction is permitted which does not comply with these terms.
*Correspondence: Hannelore Daniel, Y29udGFjdEBoZGFuaWVsLmRl