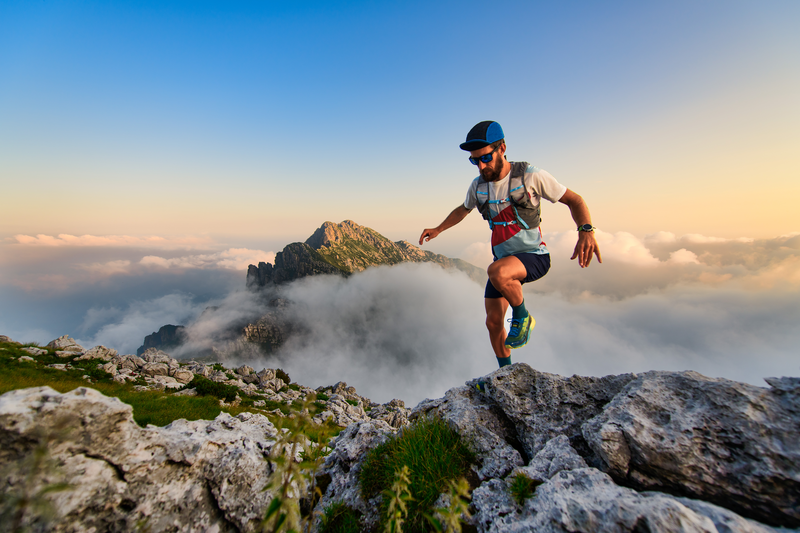
94% of researchers rate our articles as excellent or good
Learn more about the work of our research integrity team to safeguard the quality of each article we publish.
Find out more
ORIGINAL RESEARCH article
Front. Nutr. , 03 July 2024
Sec. Nutrition and Metabolism
Volume 11 - 2024 | https://doi.org/10.3389/fnut.2024.1356986
This article is part of the Research Topic Optimal Omegas View all 10 articles
Introduction: Previous studies have indicated that activity of fatty acid desaturase 1 (FADS1), is involved in cardiometabolic risk. Recent experimental data have shown that FADS1 knockdown can promote lipid accumulation and lipid droplet formation in liver cells. In this study, we aimed to characterize whether different FADS1 genotypes affect liver fat content, essential fatty acid content and free oxylipin mediators in the blood.
Methods: We analyzed the impact of FADS1 single-nucleotide polymorphisms (SNPs) rs174546, rs174547, and rs174550 on blood fatty acids and free oxylipins in a cohort of 85 patients from an academic metabolic medicine outpatient center. Patients were grouped based on their genotype into the homozygous major (derived) allele group, the heterozygous allele group, and the homozygous minor (ancestral) allele group. Omega-3 polyunsaturated fatty acids (n-3 PUFA) and omega-6 polyunsaturated fatty acids (n-6 PUFA) in the blood cell and plasma samples were analyzed by gas chromatography. Free Oxylipins in plasma samples were analyzed using HPLC–MS/MS. Liver fat content and fibrosis were evaluated using Fibroscan technology.
Results: Patients with the homozygous ancestral (minor) FADS1 genotype exhibited significantly lower blood levels of the n-6 PUFA arachidonic acid (AA), but no significant differences in the n-3 PUFAs eicosapentaenoic acid (EPA) and docosahexaenoic acid (DHA). There were no significant differences in liver fat content or arachidonic acid-derived lipid mediators, such as thromboxane B2 (TXB2), although there was a trend toward lower levels in the homozygous ancestral genotype group.
Discussion: Our findings suggest that FADS1 genotypes influence the blood levels of n-6 PUFAs, while not significantly affecting the n-3 PUFAs EPA and DHA. The lack of significant differences in liver fat content and arachidonic acid-derived lipid mediators suggests that the genotype-related variations in fatty acid levels may not directly translate to differences in liver fat or inflammatory lipid mediators in this cohort. However, the trend towards lower levels of certain lipid mediators in the homozygous ancestral genotype group warrants further investigation to elucidate the underlying mechanisms of different FADS1 genotypes and potential implications for cardiometabolic risk.
Metabolic-dysfunction associated fatty liver disease (MAFLD, steatosis hepatis) is a common disease with an estimated prevalence of 20–30% (1, 2). It is the most common liver disease in the Western world, with a rising trend. MAFLD can be considered as hepatic manifestation of the metabolic syndrome (MetS) (3, 4). MAFLD patients are at risk to develop persistent inflammation (Nonalcoholic steatohepatitis, NASH), which can lead to liver fibrosis, cirrhosis and is associated with an increased risk of developing hepatocellular carcinoma (5, 6).
Genes involved in the elongation and desaturation of long-chain (lc) PUFAs from short-chain precursors have been linked to diet-dependent risks of cardiovascular disease (CVD) and metabolic syndrome MetS) (7–9).
One of the rate-limiting steps in the synthesis of lc-PUFAs from sc-PUFAs is catalyzed by the fatty acid (FA) delta-5-desaturase (D5D; Figure 1) (10). D5D or fatty acid desaturase 1 (FADS1) is a membrane-bound desaturase that catalyzes the synthesis of lc omega-3 (n-3) and omega-6 (n-6) PUFAs from dietary linoleic acid (LA, 18:2 n-6) and α-linolenic acid (18:3 n-3) (11). The human D5D gene is located on chromosome 11 in the FADS region, where several polymorphisms are located. These FADS gene polymorphisms affect PUFA synthesis in addition to dietary regulation of FA supply and composition (10). Two alleles of the SNPs rs174546, rs 174547 and rs174550 are distinguished at the FADS1 locus. The major or derived allele corresponds to the following bases at these three SNPs: C at rs174546, T at rs 174547 and T at rs174550. The minor or ancestral allele corresponds to the following bases at the three SNPs analyzed here: T at rs174546, C at rs174547 and C at rs174550. The minor alleles of these SNPs in the FADS1 gene were found to be associated with lower blood concentrations of lc n–3 and n–6 PUFAs (12–14). Some data suggest that the major alleles are associated with an increased risk of atherosclerosis in the context of a diet high in animal fats (15), whereas other studies found that the minor alleles might confer an increased cardiovascular risk that could be alleviated by dietary measures (16).
It has been shown that the FADS1 minor ancestral allele has been the only relevant allele in human populations in Europe until approximately 8.500 years ago, when the derived allele was introduced (17).
The minor ancestral allele, which is characterized by lower FADS1 expression, has been implicated to confer increased metabolic risk and increased liver steatosis (18–21).
In this pilot study, we aimed to characterize liver fat content and lipidomics characteristics from patients enrolled in a routine setting of an outpatient metabolic medicine center for diagnosis, monitoring or treatment of MetS and MAFLD. We wanted to determine whether the homozygous minor (ancestral) FADS1 genotype is associated with higher liver fat in unselected patients presenting to our center, and whether different FADS1 genotypes affect essential fatty acid content and free oxylipin mediators in the blood.
Patients presenting to our metabolic disease clinic were recruited, containing patients with risk for, or manifest MAFLD, MetS, type 2 diabetes mellitus (T2DM), and/or dyslipidemia (elevation of triglycerides, total cholesterol, or LDL cholesterol or lowering of HDL cholesterol). Patients gave their informed consent for this research project investigating essential n-6 and n-3 fatty acids in the context of metabolic disease (approved by the institutional ethics committee, Nr. Z02-20170508). Only patients with signed informed consent were included in the study.
To assess PUFA levels in blood cells and plasma, blood samples were collected after at least 6 h of fasting from patients (n = 85) enrolled in a routine setting. All samples were centrifuged at 3500 rpm for 10 min at 4°C, separated blood cell and plasma samples were stored at −80°C until FA analysis. Extraction and quantification were carried out according to established protocols. According to a previous study, we expected a prevalence of patients homozygous for the minor FADS1 allele (that has lower D5D activity) of 11% (16), and aimed for 9–10 patients with this genotype to allow for meaningful statistical analysis of the FA and oxylipins.
In brief, FA from 50 μL erythrocytes and 75 μL plasma per sample were analyzed. Boron trifluoride (BF3) derivatization was applied for the blood cell fraction (22) and a combined BF3 + NaOH method for derivatization was used for the plasma samples (23, 24). FA values from blood cells are presented as percentage [%] of total FA content, FA concentration from plasma are presented in absolute amounts [μg/ml]. Free Oxylipins in plasma samples [ng/ml] were analyzed as described previously using LC–MS/MS (Lipidomix, Berlin) (25).
Sonographic evaluation was performed using FibroScan (Echosens, Paris), a standardized and reproducible point-of-care technique for quantification of steatosis and fibrosis as described previously (25). This is a non-invasive measurement method for quantifying a fatty liver, in which acoustically generated transient ultrasound waves cause the liver to vibrate intermittently. This method is based on shear wave elastography (SWE) (26).The cutoff value reported in the literature for the detection of hepatic steatosis ranges from 222 decibels per meter (dB/m) in a cohort of patients with chronic hepatitis C to 294 dB/m in a meta-analysis of patients with nonalcoholic fatty liver disease (NAFLD) (27). The examination was performed in all patients in a fasting state (the last meal should have been at least 6 h before the measurement). The examination was performed in the supine position with maximal abduction of the right arm and positioning of the right leg over the left to gain sufficient intercostal access. The probe was placed at the intersection of the xiphoid process and the midaxillary line. Patients weighing <100 kg were examined with the M probe (standard probe—transducer frequency 3.5 MHz) and patients weighing >100 kg were examined with the XL probe (transducer frequency 2.5 MHz). Reliable measurements were defined as: Median of 13 valid LS measurements with an interquartile range ≤ 30% (IQR/med = the difference between the 75 and 25th percentiles, i.e., the range of the middle 50% of the data relative to the median).
The FADS1 SNPs rs174546, rs174547 and rs174550 were characterized in venous blood collected from the patients in EDTA tubes using an Illumina Platform (Life and Brain, Bonn, Germany).
For statistical analysis FA or oxylipin values were tested for normal distribution using the Shapiro–Wilk test. For normally distributed values, a one-way ANOVA between the three groups and Tukey’s Honest Significance Difference (HSD) test as follow-up to assess significances between subsets of two groups was performed. For non-normally distributed values, testing for significant differences between the three groups was performed using the Kruskal-Wallis test and followed up with Dunn’s testing for subsets of the possible pairwise comparisons. Categorical values (age) were compared using Pearson Chi Square testing. Statistical analyses were done using GraphPad prism or Excel software. p ≤ 0.05 was considered as significant.
We aimed to include at least 10 patients homozygous for the minor (ancestral) FADS1 allele. This objective was achieved after having screened 85 patients for whom we performed liver fat determination, FA analyses and, of a subset, lipidomics analyses. Out of these 85 patients, 37 were homozygous for the major (derived) FADS1 alleles (rs174546 CC, rs174547 TT and rs174550 TT), 37 heterozygous (rs174546 CT, rs174547 CT and rs174550 CT), and 11 homozygous for the minor (ancestral) FADS1 allele (rs174546 TT, rs174547 CC and rs174550 CC). As shown in Table 1 there were no significant differences between the groups regarding age and sex. Furthermore, there were no significant differences between groups regarding steatosis and fibrosis, as assessed by Fibroscan.
In order to assess FADS1 activity the delta-5-desaturase index (D5D index) was calculated as the following ratio of arachidonic acid (AA) and its precursor dihomo-gamma-linolenic acid (DGLA) in FA and plasma (10, 28):
As expected from previously published data we found highly significant D5D index differences between genotypes containing the major allele (rs174546 CC and CT,) and the homozygous minor allele (rs174546 TT), supporting higher FADS1 gene activity with the major derived C allele, leading to higher levels of AA and lower levels of DGLA (Figures 2A,C). Indeed, there were significant differences in blood cell FA content for LA (18:2 n-6), DGLA (20:3 n-6), AA (20:4 n-6), and n-3 docosapentaenoic acid (DPA, 22:5 n-3). No significant differences were found for eicosapentaenoic acid (EPA, 20:5 n-3) and docosahexaenoic acid (DHA, 22:6 n-3; Figure 2C). The content of EPA plus DHA, determined in analogy to the well-established Omega-3-Index (29), was slightly lower in the homozygous minor allele group (Figure 2B).
Figure 2. Effect of the FADS1 rs174546 genotype on PUFAs in the blood cell fraction. (A) D5D index is significantly higher with the C allele carriers (CC > CT > TT). (B) Slightly lower EPA + DHA content (determined in analogy to the Omega-3-Index) in the blood cell fraction from patients with the homozygous ancestral FADS1 genotype (TT). (C) Significantly higher levels of n-6 PUFAs linoleic acid (LA) and dihomo-gamma-linolenic acid (DGLA), and lower levels of arachidonic acid (AA) and n-3 PUFA docosapentaenoic acid (DPA) with the minor TT genotype. n = 37 for the homozygous derived genotype CC, n = 37 for the heterozygous genotype CT, n = 11 for the homozygous ancestral genotype TT, *p < 0.05, **p < 0.01, ***p < 0.001, one-way ANOVA with subsequent Tukey’s HSD testing.
In a subset of patients, we also analyzed plasma fatty acids. These data confirmed the significantly higher D5D index, as well as higher levels of AA (20:4 n-6) in the derived genotype carriers (Figures 3A,C). There was a significant difference of D5D indices between the homozygous CC and TT allele carriers. In this subset we did not find significant differences for other plasma FAs. Interestingly, there was a higher plasma EPA/AA-ratio in patients with the homozygous ancestral genotype, although this finding did not reach statistical significance (Figure 3B). This parameter, with a cutoff at 0.4 and higher levels being beneficial, has been implicated as a risk stratification marker for cardiovascular protection (30, 31).
Figure 3. Effect of the FADS1 rs174546 genotype on plasma PUFAs content. (A) The D5D index is significantly higher with the CC alleles in comparison to TT alleles. (B) Slightly higher EPA/AA plasma ratio with the homozygous ancestral FADS1 genotype (ns, p = 0.067). (C) Slightly lower AA (20:4 n-6) levels with the homozygous ancestral FADS1 genotype. n = 19 for the homozygous derived genotype, n = 19 for the heterozygous genotype, n = 4 for the homozygous ancestral genotype, *p < 0.05, Kruskal-Wallis and subsequent Dunn’s testing.
There were no significant differences of plasma free oxylipin content between different FADS1 genotypes, with slightly higher, but not significantly different levels of metabolites in the homozygous ancestral genotype as compared to the other genotypes (Figures 4A–E). There were indications, however, that platelet-related mediators such as thromboxane B2 (TXB2) and 12-HETE tended to be higher with the derived genotype (Figures 4A,B). Potentially hepatoprotective epoxy metabolites also showing a trend to higher levels as compared to the minor homozygotes (Figure 4E).
Figure 4. Plasma free oxylipins in groups with FADS1 rs174546 genotypes. (A) Thromboxane B2 (TXB2), prostaglandin D2 (PGD2), prostaglandin E2 (PGE2) and lipoxin A4 (LXA4) contents in plasma of different FADS1 genotypes. (B) 12-Lipoxygenase-derived oxylipin contents from AA (12-hydroxyeicosatetraenoic acid, 12-HETE), EPA (12-hydroxyeicosapentaenoic acid, 12-HEPE) and DHA (14-hydroxydocosahexaenoic acid, 14-HDHA) in plasma of different FADS1 genotypes. (C) 15-Lipoxygenase-derived oxylipin contents from AA (15-HETE), EPA (15-HEPE) and DHA (17-HDHA). (D) 5-Lipoxygenase-derived oxylipins from AA (5-HETE), EPA (5-HEPE) and DHA (7-HDHA) in plasma of different FADS1 genotypes. (E) Cytochrome P450-derived epoxy metabolites from EPA (17,18-epoxyeicosatetraenoic acid 17,18-EEQ) and DHA (19,20-epoxydocosapentaenoic acid, 19,20-EDP) in plasma of different FADS1 genotypes. n = 36 for the homozygous derived genotype, n = 36 for the heteozygous genotype, n = 10 for the homozygous ancestral genotype. There were no significant differences between groups from Kruskal-Wallis testing.
The development of MetS is affected not only by lifestyle but also by genetics. In a retrospective study, the difference of dietary patterns was not enough to cause the change of MetS outcomes (32). Since genetic variants affect lipid metabolism which is closely associated with MetS (33), attention focused on the association between MetS and genetic factors (34–36), and FADS genotype has been found to contribute to biochemical and metabolic variations in the MetS. In our pilot study in a routine clinical setting, we chose the FADS1 SNP rs174546 which has been reported to be in linkage disequilibrium (LD) with other FADS1 variants as a representative variant for differences in the FADS1 genotype (12, 36) and confirmed this with the SNPs rs174547 and rs174550.
Carriers of the major allele are known to have higher FADS1 enzyme activities than homozygous carriers of the ancestral minor allele. This leads to higher substrate precursor concentrations (LA, 18:2 n-6), higher substrate concentrations (DGLA, 20:3 n-6) and lower product (AA, 20:4 n-6) concentrations in patients homozygous for the minor alleles (12, 16, 37).
We confirmed the previously described significantly lower D5D index and activity for patients with the homozygous minor (ancestral) FADS1 allele as compared to those homo- or heterozygous for the major (derived) allele in the blood cell fraction as well as in plasma for homozygous minor allele carriers in comparison with homozygous major allele carriers. Furthermore, the amounts of LA (18:2 n-6) and DGLA (20:3 n-6) were significantly higher in blood cells with the homozygous ancestral FADS1 genotype, whereas amounts of AA (20:4 n-6) and DPA (22:5 n-3) were significantly lower.
The lc n-3 PUFA concentrations in plasma and blood cells also tended to show higher concentrations in the derived allele carriers. This also indicates increased D5D activities in the derived allele carriers. We could not find any significance here except for n-3 DPA contents in blood cells, with higher values in the derived allele carriers. The observed reduction in D5D product concentrations of n-3 LC PUFAs, compared to the significant differences in n-6 LC PUFAs among derived allele carriers, may result from an excess of available linoleic acid (LA).
Our findings regarding D5D index and n-6 PUFAs are in agreement with earlier studies. EPIC, a multicenter prospective cohort study analyzing 2,653 patients, the TT genotype at SNP rs174546 was inversely related to D5D activity (39). Another large study also observed that D5D activity was significantly lower among carriers of the rs174546 minor T allele. In addition, this study also indicated higher LA and DGLA concentrations in patients with the TT genotype, while lower concentrations were observed for AA (40). In the PREOBE cohort, women who carried the T allele at rs174546 had higher DGLA and lower AA levels (41). Furthermore, higher serum phospholipid concentrations of LA were observed in people with the T allele of rs174546, while the lower serum phospholipid levels were observed for AA (42).
However, contrary to earlier studies (15, 43–45), we did not observe that FADS1 gene variants were associated with significant differences in blood levels of n-3 PUFAs EPA or DHA. Interestingly, other data also indicate that genetic differences at the FADS1 and FADS2 loci probably have stronger effects on n-6 PUFAs than n-3 PUFAs (46). Having said that, these differences could also be due to differences in dietary consumption of n-3 PUFA, for which there is more variation than for n-6 PUFA in western diet between individuals with high or low fish consumption, which we did not monitor in our study.
Liver steatosis and fibrosis assessed by Fibroscan (47) were not associated with FADS1 allele variations, which is in contrast to a pediatric study indicating that the minor FADS1 variant was associated with a higher degree of liver steatosis (19).
In order to expand knowledge and analyses regarding FADS1 genotype variations we also performed oxylipin analyses in plasma. While we did not observe significant differences between the groups, we found a trend toward higher thrombocyte AA-mediator formation in the derived group—which might indicate higher vascular risk and could fit with earlier observations that the derived FADS1 genotype is associated with increased cardiovascular risk (48, 49). Furthermore, we found a trend toward a lower plasma EPA/AA ratio in the derived group. At the same time n-3 PUFA derived epoxy metabolites that were identified as steatosis-protective factors in mouse experiments (50, 51), were lower in the ancestral group. This could be a mechanism involved in increased steatosis and metabolic risk that has been described for patients with the ancestral genotype (19, 21). Our data are not sufficient to prove these effects, however, they form the basis for hypotheses that now need to be tested in other studies.
Limiting factors of this observational study are mainly the heterogeneous group composed of a random selection of patients. In addition, the group of homozygous ancestral allele carriers is relatively small. In future studies it will be important to collect additional clinical data from patients, such as routine lipid parameters, blood pressure measurements and BMI, to further stratify risk constellation for steatosis hepatis and cardiovascular disease. Looking at the FADS1 gene alone is also a limitation. For example, Shetty et al. described an association of FADS2 gene polymorphisms with increased insulin resistance and type 2 diabetes (52). In the current cohort, diabetes mellitus status was not considered and thus the inclusion of this criterion is needed to better assess the risk constellation between diabetes mellitus and cardiovascular risk in MAFLD.
To further assess the effects of the minor, ancestral allele on PUFA and oxylipin concentrations, an increase in the study population is desirable.
In this pilot study in a routine clinical setting, we were able to confirm previous observations of significantly different blood fatty acid profiles depending on FADS1 genotype, with a lower D5D index in patients homozygous for the ancestral (minor) allele. Carriers of the derived genotype had a higher D5D index and higher AA concentrations and also showed a trend toward higher AA-derived thromboxane B2 and 12-HETE in blood plasma.
The original contributions presented in the study are included in the article, further inquiries can be directed to the corresponding authors.
The studies involving humans were approved by Ethics committee of Brandenburg Medical School. The studies were conducted in accordance with the local legislation and institutional requirements. The participants provided their written informed consent to participate in this study.
MRa: Data curation, Methodology, Writing – review & editing, Investigation. ZW: Data curation, Visualization, Writing – original draft, Writing – review & editing. CL: Data curation, Writing – review & editing. JE: Data curation, Writing – review & editing, Conceptualization, Methodology, Supervision. MRo: Methodology, Writing – review & editing, Formal analysis, Investigation. AJ: Methodology, Writing – review & editing, Data curation. CS: Writing – review & editing. UE: Writing – review & editing. KW: Writing – review & editing, Conceptualization, Data curation, Methodology, Project administration, Supervision, Validation, Visualization, Writing – original draft. AP: Writing – review & editing, Investigation, Methodology, Project administration, Supervision.
The author(s) declare that financial support was received for the research, authorship, and/or publication of this article. This project was supported by funds from the Ministry of Science, Research and Culture of the State of Brandenburg and by the Brandenburg Medical School (Medizinische Hochschule Brandenburg, MHB) publication fund supported by the German Research Foundation (Deutsche Forschungsgemeinschaft, DFG).
We thank our clinic staff for their support and the patients for their participation.
MRo is owner of Lipidomix GmbH.
The remaining authors declare that the research was conducted in the absence of any commercial or financial relationships that could be construed as a potential conflict of interest.
All claims expressed in this article are solely those of the authors and do not necessarily represent those of their affiliated organizations, or those of the publisher, the editors and the reviewers. Any product that may be evaluated in this article, or claim that may be made by its manufacturer, is not guaranteed or endorsed by the publisher.
1. Sangro, P, de la Torre Alaez, M, Sangro, B, and D'Avola, D. Metabolic dysfunction-associated fatty liver disease (MAFLD): an update of the recent advances in pharmacological treatment. J Physiol Biochem. (2023) 79:869–79. doi: 10.1007/s13105-023-00954-4
2. Younossi, ZM, Koenig, AB, Abdelatif, D, Fazel, Y, Henry, L, and Wymer, M. Global epidemiology of nonalcoholic fatty liver disease-Meta-analytic assessment of prevalence, incidence, and outcomes. Hepatology. (2016) 64:73–84. doi: 10.1002/hep.28431
3. Cornier, MA, Dabelea, D, Hernandez, TL, Lindstrom, RC, Steig, AJ, Stob, NR, et al. The metabolic syndrome. Endocr Rev. (2008) 29:777–822. doi: 10.1210/er.2008-0024
4. Godoy-Matos, AF, Silva Junior, WS, and Valerio, CM. NAFLD as a continuum: from obesity to metabolic syndrome and diabetes. Diabetol Metab Syndr. (2020) 12:60. doi: 10.1186/s13098-020-00570-y
5. Gu, W, Hortlik, H, Erasmus, HP, Schaaf, L, Zeleke, Y, Uschner, FE, et al. Trends and the course of liver cirrhosis and its complications in Germany: Nationwide population-based study (2005 to 2018). Lancet Reg Health Eur. (2022) 12:100240. doi: 10.1016/j.lanepe.2021.100240
6. Karlsen, TH, Sheron, N, Zelber-Sagi, S, Carrieri, P, Dusheiko, G, Bugianesi, E, et al. The EASL-lancet liver commission: protecting the next generation of Europeans against liver disease complications and premature mortality. Lancet. (2022) 399:61–116. doi: 10.1016/S0140-6736(21)01701-3
7. Aulchenko, YS, Ripatti, S, Lindqvist, I, Boomsma, D, Heid, IM, Pramstaller, PP, et al. Loci influencing lipid levels and coronary heart disease risk in 16 European population cohorts. Nat Genet. (2009) 41:47–55. doi: 10.1038/ng.269
8. Glaser, C, Heinrich, J, and Koletzko, B. Role of FADS1 and FADS2 polymorphisms in polyunsaturated fatty acid metabolism. Metabolism. (2010) 59:993–9. doi: 10.1016/j.metabol.2009.10.022
9. Walle, P, Takkunen, M, Mannisto, V, Vaittinen, M, Kakela, P, Agren, J, et al. Alterations in fatty acid metabolism in response to obesity surgery combined with dietary counseling. Nutr Diabetes. (2017) 7:e285. doi: 10.1038/nutd.2017.33
10. Lattka, E, Illig, T, Koletzko, B, and Heinrich, J. Genetic variants of the FADS1 FADS2 gene cluster as related to essential fatty acid metabolism. Curr Opin Lipidol. (2010) 21:64–9. doi: 10.1097/MOL.0b013e3283327ca8
11. Nakamura, MT, and Nara, TY. Structure, function, and dietary regulation of delta6, delta5, and delta9 desaturases. Annu Rev Nutr. (2004) 24:345–76. doi: 10.1146/annurev.nutr.24.121803.063211
12. Juan, J, Huang, H, Jiang, X, Ardisson Korat, AV, Song, M, Sun, Q, et al. Joint effects of fatty acid desaturase 1 polymorphisms and dietary polyunsaturated fatty acid intake on circulating fatty acid proportions. Am J Clin Nutr. (2018) 107:826–33. doi: 10.1093/ajcn/nqy025
13. Meuronen, T, Lankinen, MA, Kolmert, J, de Mello, VD, Sallinen, T, Agren, J, et al. The FADS1 rs174550 genotype modifies the n-3 and n-6 PUFA and lipid mediator responses to a high alpha-Linolenic acid and high linoleic acid diets. Mol Nutr Food Res. (2022) 66:e2200351. doi: 10.1002/mnfr.202200351
14. Wang, Y, Tang, Y, Ji, Y, Xu, W, Ullah, N, Yu, H, et al. Association between FADS1 rs174547 and levels of long-chain PUFA: a meta-analysis. Br J Nutr. (2021) 126:1121–9. doi: 10.1017/S0007114520005103
15. Ameur, A, Enroth, S, Johansson, A, Zaboli, G, Igl, W, Johansson, AC, et al. Genetic adaptation of fatty-acid metabolism: a human-specific haplotype increasing the biosynthesis of long-chain omega-3 and omega-6 fatty acids. Am J Hum Genet. (2012) 90:809–20. doi: 10.1016/j.ajhg.2012.03.014
16. Hellstrand, S, Ericson, U, Gullberg, B, Hedblad, B, Orho-Melander, M, and Sonestedt, E. Genetic variation in FADS1 has little effect on the association between dietary PUFA intake and cardiovascular disease. J Nutr. (2014) 144:1356–63. doi: 10.3945/jn.114.192708
17. Mathieson, S, and Mathieson, I. FADS1 and the timing of human adaptation to agriculture. Mol Biol Evol. (2018) 35:2957–70. doi: 10.1093/molbev/msy180
18. Athinarayanan, S, Fan, YY, Wang, X, Callaway, E, Cai, D, Chalasani, N, et al. Fatty acid desaturase 1 influences hepatic lipid homeostasis by modulating the PPARalpha-FGF21 Axis. Hepatol Commun. (2021) 5:461–77. doi: 10.1002/hep4.1629
19. Nobili, V, Alisi, A, Liu, Z, Liang, T, Crudele, A, Raponi, M, et al. In a pilot study, reduced fatty acid desaturase 1 function was associated with nonalcoholic fatty liver disease and response to treatment in children. Pediatr Res. (2018) 84:696–703. doi: 10.1038/s41390-018-0132-7
20. Wang, L, Athinarayanan, S, Jiang, G, Chalasani, N, Zhang, M, and Liu, W. Fatty acid desaturase 1 gene polymorphisms control human hepatic lipid composition. Hepatology. (2015) 61:119–28. doi: 10.1002/hep.27373
21. Yang, C, Hallmark, B, Chai, JC, O'Connor, TD, Reynolds, LM, Wood, AC, et al. Impact of Amerind ancestry and FADS genetic variation on omega-3 deficiency and cardiometabolic traits in Hispanic populations. Commun Biol. (2021) 4:918. doi: 10.1038/s42003-021-02431-4
22. Kang, JX, and Wang, J. A simplified method for analysis of polyunsaturated fatty acids. BMC Biochem. (2005) 6:5. doi: 10.1186/1471-2091-6-5
23. Ostermann, AI, Willenberg, I, Weylandt, K, and Schebb, NH. Development of an online-SPE-LC-MS/MS method for 26 hydroxylated polyunsaturated fatty acids as rapid targeted metabolomics approach for the LOX, CYP and autoxidation pathways of the arachidonic acid cascade. Chromatographia (2014). 78, 415–428.
24. Wang, C, Enssle, J, Pietzner, A, Schmocker, C, Weiland, L, Ritter, O, et al. Essential polyunsaturated fatty acids in blood from patients with and without catheter-proven coronary artery disease. Int J Mol Sci. (2022) 23:766. doi: 10.3390/ijms23020766
25. Xiao, Y, Pietzner, A, Rohwer, N, Jung, A, Rothe, M, Weylandt, KH, et al. Bioactive oxylipins in type 2 diabetes mellitus patients with and without hypertriglyceridemia. Front Endocrinol (Lausanne). (2023) 14:1195247. doi: 10.3389/fendo.2023.1195247
26. Sporea, I, Bende, F, Popescu, A, Lupusoru, R, Fofiu, R, and Sirli, R. Are there different cut-off values for staging liver fibrosis using 2D-SWE implemented on different systems from the same manufacturer? Med Ultrason. (2020) 1:7–12. doi: 10.11152/mu-2225
27. Ferraioli, G, Maiocchi, L, Savietto, G, Tinelli, C, Nichetti, M, Rondanelli, M, et al. Performance of the attenuation imaging Technology in the Detection of liver steatosis. J Ultrasound Med. (2021) 40:1325–32. doi: 10.1002/jum.15512
28. Warensjo, E, Sundstrom, J, Vessby, B, Cederholm, T, and Riserus, U. Markers of dietary fat quality and fatty acid desaturation as predictors of total and cardiovascular mortality: a population-based prospective study. Am J Clin Nutr. (2008) 88:203–9. doi: 10.1093/ajcn/88.1.203
29. von Schacky, C. Omega-3 index and cardiovascular health. Nutrients. (2014) 6:799–814. doi: 10.3390/nu6020799
30. Abstracts, AHA. Late-breaking science Abstracts and featured science Abstracts from the American Heart Association's scientific sessions 2022 and late-breaking Abstracts in resuscitation science from the resuscitation science symposium 2022. Circulation. (2022) 146:e569–605. doi: 10.1161/CIR.0000000000001116
31. Nishizaki, Y, Miyauchi, K, Iwata, H, Inoue, T, Hirayama, A, Kimura, K, et al. Study protocol and baseline characteristics of randomized trial for evaluation in secondary prevention efficacy of combination therapy-statin and Eicosapentaenoic acid: RESPECT-EPA, the combination of a randomized control trial and an observational biomarker study. Am Heart J. (2022) 257:1–8. doi: 10.1016/j.ahj.2022.11.008
32. Pandya, R, Abdelaal, R, Chen, JW, Masood, S, Talib, Z, Atamna, H, et al. Retrospective assessment of metabolic syndrome components in early adult life on vegetarian dietary status. Front Public Health. (2022) 10:945805. doi: 10.3389/fpubh.2022.945805
33. Nagrani, R, Foraita, R, Gianfagna, F, Iacoviello, L, Marild, S, Michels, N, et al. Common genetic variation in obesity, lipid transfer genes and risk of metabolic syndrome: results from IDEFICS/I.Family study and meta-analysis. Sci Rep. (2020) 10:7189. doi: 10.1038/s41598-020-64031-2
34. Lee, S, Kim, SA, Hong, J, Kim, Y, Hong, G, Baik, S, et al. Identification of genetic variants related to metabolic syndrome by next-generation sequencing. Diabetol Metab Syndr. (2022) 14:119. doi: 10.1186/s13098-022-00893-y
35. Tong, Z, Qi, J, Ma, W, Wang, D, Hu, B, Li, Y, et al. SUMO4 gene SNP rs237025 and the synergistic effect with weight management: a study of risk factors and interventions for MetS. Front Genet. (2021) 12:786393. doi: 10.3389/fgene.2021.786393
36. Zak, A, Jachymova, M, Burda, M, Stankova, B, Zeman, M, Slaby, A, et al. FADS polymorphisms affect the clinical and biochemical phenotypes of metabolic syndrome. Meta. (2022) 12:568. doi: 10.3390/metabo12060568
37. Hermant, X, Delay, C, Flaig, A, Luque-Bedregal, J, Briand, G, Bout, MA, et al. Identification of a functional FADS1 3'UTR variant associated with erythrocyte n-6 polyunsaturated fatty acids levels. J Clin Lipidol. (2018) 12:1280–9. doi: 10.1016/j.jacl.2018.07.012
38. Rodriguez, A, Sarda, P, Nessmann, C, Boulot, P, Leger, CL, and Descomps, B. Delta6- and delta5-desaturase activities in the human fetal liver: kinetic aspects. J Lipid Res. (1998) 39:1825–32. doi: 10.1016/S0022-2275(20)32170-2
39. Kroger, J, Zietemann, V, Enzenbach, C, Weikert, C, Jansen, EH, Doring, F, et al. Erythrocyte membrane phospholipid fatty acids, desaturase activity, and dietary fatty acids in relation to risk of type 2 diabetes in the European prospective investigation into Cancer and nutrition (EPIC)-Potsdam study. Am J Clin Nutr. (2011) 93:127–42. doi: 10.3945/ajcn.110.005447
40. Zietemann, V, Kroger, J, Enzenbach, C, Jansen, E, Fritsche, A, Weikert, C, et al. Genetic variation of the FADS1 FADS2 gene cluster and n-6 PUFA composition in erythrocyte membranes in the European prospective investigation into Cancer and nutrition-Potsdam study. Br J Nutr. (2010) 104:1748–59. doi: 10.1017/S0007114510002916
41. de la Garza Puentes, A, Montes Goyanes, R, Chisaguano Tonato, AM, Torres-Espinola, FJ, Arias Garcia, M, de Almeida, L, et al. Association of maternal weight with FADS and ELOVL genetic variants and fatty acid levels- the PREOBE follow-up. PLoS One. (2017) 12:e0179135. doi: 10.1371/journal.pone.0179135
42. Dumont, J, Huybrechts, I, Spinneker, A, Gottrand, F, Grammatikaki, E, Bevilacqua, N, et al. FADS1 genetic variability interacts with dietary alpha-linolenic acid intake to affect serum non-HDL-cholesterol concentrations in European adolescents. J Nutr. (2011) 141:1247–53. doi: 10.3945/jn.111.140392
43. Hallmann, J, Kolossa, S, Gedrich, K, Celis-Morales, C, Forster, H, O'Donovan, CB, et al. Predicting fatty acid profiles in blood based on food intake and the FADS1 rs174546 SNP. Mol Nutr Food Res. (2015) 59:2565–73. doi: 10.1002/mnfr.201500414
44. Molto-Puigmarti, C, Plat, J, Mensink, RP, Muller, A, Jansen, E, Zeegers, MP, et al. FADS1 FADS2 gene variants modify the association between fish intake and the docosahexaenoic acid proportions in human milk. Am J Clin Nutr. (2010) 91:1368–76. doi: 10.3945/ajcn.2009.28789
45. Santana, JDM, Pereira, M, Carvalho, GQ, Gouveia Peluzio, MDC, Drumond Louro, I, Santos, DBD, et al. FADS1 and FADS2 gene polymorphisms modulate the relationship of Omega-3 and Omega-6 fatty acid plasma concentrations in gestational weight gain: a NISAMI cohort study. Nutrients. (2022) 14:1056. doi: 10.3390/nu14051056
46. Nettleton, JA, and Salem, N Jr. International Society for the Study of fatty acids and lipids 2018 symposium: arachidonic and docosahexaenoic acids in infant development. Ann Nutr Metab. (2019) 74:83–91. doi: 10.1159/000495906
47. Dyson, JK, Anstee, QM, and McPherson, S. Non-alcoholic fatty liver disease: a practical approach to diagnosis and staging. Frontline Gastroenterol. (2014) 5:211–8. doi: 10.1136/flgastro-2013-100403
48. Martinelli, N, Girelli, D, Malerba, G, Guarini, P, Illig, T, Trabetti, E, et al. FADS genotypes and desaturase activity estimated by the ratio of arachidonic acid to linoleic acid are associated with inflammation and coronary artery disease. Am J Clin Nutr. (2008) 88:941–9. doi: 10.1093/ajcn/88.4.941
49. O'Neill, CM, and Minihane, AM. The impact of fatty acid desaturase genotype on fatty acid status and cardiovascular health in adults. Proc Nutr Soc. (2017) 76:64–75. doi: 10.1017/S0029665116000732
50. López-Vicario, C, Alcaraz-Quiles, J, García-Alonso, V, Rius, B, Hwang, SH, Titos, E, et al. Inhibition of soluble epoxide hydrolase modulates inflammation and autophagy in obese adipose tissue and liver: role for omega-3 epoxides. Proc Natl Acad Sci. (2015) 112:536–41. doi: 10.1073/pnas.1422590112
51. Lopez-Vicario, C, Gonzalez-Periz, A, Rius, B, Moran-Salvador, E, Garcia-Alonso, V, Lozano, JJ, et al. Molecular interplay between Delta5/Delta6 desaturases and long-chain fatty acids in the pathogenesis of non-alcoholic steatohepatitis. Gut. (2014) 63:344–55. doi: 10.1136/gutjnl-2012-303179
Keywords: steatosis hepatis, MAFLD, FADS1, Oxylipins, PUFA
Citation: Rabehl M, Wei Z, Leineweber CG, Enssle J, Rothe M, Jung A, Schmöcker C, Elbelt U, Weylandt KH and Pietzner A (2024) Effect of FADS1 SNPs rs174546, rs174547 and rs174550 on blood fatty acid profiles and plasma free oxylipins. Front. Nutr. 11:1356986. doi: 10.3389/fnut.2024.1356986
Received: 16 December 2023; Accepted: 06 June 2024;
Published: 03 July 2024.
Edited by:
Ivana Djuricic, University of Belgrade, SerbiaReviewed by:
Oscar Plunde, Karolinska Institutet (KI), SwedenCopyright © 2024 Rabehl, Wei, Leineweber, Enssle, Rothe, Jung, Schmöcker, Elbelt, Weylandt and Pietzner. This is an open-access article distributed under the terms of the Creative Commons Attribution License (CC BY). The use, distribution or reproduction in other forums is permitted, provided the original author(s) and the copyright owner(s) are credited and that the original publication in this journal is cited, in accordance with accepted academic practice. No use, distribution or reproduction is permitted which does not comply with these terms.
*Correspondence: Anne Pietzner, YW5uZS5waWV0em5lckBtaGItZm9udGFuZS5kZQ==; Karsten H. Weylandt, a2Fyc3Rlbi53ZXlsYW5kdEBtaGItZm9udGFuZS5kZQ==
†These authors have contributed equally to this work and share first authorship
Disclaimer: All claims expressed in this article are solely those of the authors and do not necessarily represent those of their affiliated organizations, or those of the publisher, the editors and the reviewers. Any product that may be evaluated in this article or claim that may be made by its manufacturer is not guaranteed or endorsed by the publisher.
Research integrity at Frontiers
Learn more about the work of our research integrity team to safeguard the quality of each article we publish.