- 1Division of Clinical Research, The First Hospital of Jilin University, Changchun, China
- 2School of Public Health, Jilin University, Changchun, China
- 3Department of Geriatrics, The First Hospital of Jilin University, Changchun, China
- 4Coll Comp Sci & Technol, Jilin University, Changchun, China
Background: Sarcopenia, a condition characterized by diminished skeletal muscle mass, strength, and function, accompanied by inflammation and oxidative stress, remains an area of limited exploration concerning its correlation with the Oxidative Balance Score (OBS).
Methods: Leveraging data from the 2011–2018 National Health and Nutrition Examination Survey (NHANES), we meticulously examined 16 dietary and four lifestyle factors to derive the OBS. Adjusting appendicular skeletal muscle mass (ASM) by body mass index (BMI) served as the designated marker for sarcopenia. To scrutinize the association between OBS and sarcopenia, we conducted weighted logistic regression and engaged in sensitivity analysis. Furthermore, we implemented subgroup analysis and interaction tests to gain comprehensive insights into the relationship across diverse populations.
Results: In a sample comprising 6,677 individuals aged 20–59, logistic regression illuminated a negative association between OBS and sarcopenia [OR = 0.942 (0.920, 0.964), p < 0.001]. Robust associations were also discerned between diseases and both dietary and lifestyle OBS. Subgroup analysis unveiled a more pronounced negative association in older, married/living with partner or more educated individuals. Moreover, this association persisted in populations grappling with comorbidities such as hypertension, diabetes, cancer, and arthritis.
Conclusion: Our study posits a perceptible link between OBS and the prevalence of sarcopenia among American adults.
1 Introduction
Sarcopenia is defined as a disease characterized by reduced skeletal muscle mass associated with decreased strength and function (1, 2). As an age-related disease, the disease occurs at all ages and results in adverse out-comes including recurrent falls, disability, fractures, frailty, poor quality of life, increased hospitalizations and mortality (3–7). Furthermore, studies have demonstrated that sarcopenia is associated with several diseases, including type 2 diabetes (8), osteoarthritis (9), cardiovascular disease (10–12), cirrhosis (13), some inflammatory disease (14). While the underlying causes of sarcopenia are not fully understood, most researchers believe that it results from a combination of factors, including changes in metabolic profile, malnutrition, prolonged sedentary time, hormonal, epigenetic and cellular changes (15–17). Oxidative stress and inflammation are crucial pathophysiological features of sarcopenia. Excessive oxidative stress impairs mitochondrial quality control, damaging mitochondrial membranes, DNA, proteins, and lipids. This damage compromises the structure and function of the mitochondria, which leads to increased production of reactive oxygen species (ROS) and further oxidative stress. This vicious cycle can result in muscle cell dysfunction and progressive loss of muscle mass (18–21).
Under normal physiological conditions, humans maintain a balance between oxidants and the antioxidant system. However, pro-oxidants can induce oxidative stress through the generation of reactive oxygen species (ROS) or the reduction of the defense activity of the antioxidant system (22). If the production of ROS exceeds the scavenging capacity of the antioxidant response system, it triggers oxidative damage, leading to cellular degeneration and reduced physiological function (23). Oxidative stress is implicated in several diseases (24), including metabolic syndrome (25), cardiovascular disease (CVD) (26), cancer (27, 28), Alzheimer’s disease (29). Due to the complex biological interactions between pro-oxidants and antioxidants, reliance on a single oxidative stress-related factor for assessment of overall oxidative homeostasis in vivo is inadequate. To overcome this limitation, researchers developed the Oxidative Balance Score (OBS), which calculated an individual’s pro-oxidant and total antioxidant exposure to assess overall antioxidant status, reflecting the impact of diet and lifestyle on the oxidative/antioxidant system (22, 30). Higher OBS scores indicate greater anti-oxidant exposure (31). Several studies have shown that OBS was negatively correlated with diabetes (32), cardiovascular disease (33), chronic kidney disease (34), osteoarthritis (34), non-alcoholic fatty liver disease (35), depression (36), and telomere length (31). OBS is a useful measure for assessing the cumulative impact of oxidative stress-related exposures in the study of chronic disease (37).
Since oxidative stress is closely associated with sarcopenia, it is crucial to use indicators that assess the overall oxidative/antioxidant system to evaluate the risk of the disease. Many current studies focus only on examining the relationship between inflammatory biomarkers such as CRP, IL-6, and TNF-α and muscle mass (14, 17, 38, 39). However, none of the studies have investigated the relationship of OBS with sarcopenia. Therefore, our study utilized data from the National Health and Nutrition Examination Survey (NHANES) with the aim of evaluating whether there is an association between the OBS index and sarcopenia in U.S. adults using a cross-sectional study approach. We hypothesize that an elevated OBS Index is associated with a lower risk of developing sarcopenia.
2 Materials and methods
2.1 Study population
This study was a cross-sectional study and the data were primarily obtained from the National Health and Nutrition Examination Survey (NHANES), a program that is widely used by scholar-researchers to assess the health and nutrition status of adults and children in the United States. The program consists of five main data sets collected through interviews and physical examinations, including demographic, dietary, medical assessment, laboratory, and questionnaire data (40). Each participant completed questionnaires and examinations at the Mobile Examination Center (MEC) over a one-year period to obtain important study variables, including physical condition, physiological and biochemical indices, and dietary habits at specific times of the year. Data collection is approved by the National Center for Health Statistics Research Ethics Review Board, and written informed consent was obtained from participants (41).
In this study, data from four cycles of the NHANES surveys (2011–2018) were used, which included a total of 39,156 participants. Individuals younger than 20 years (n = 16,539) were excluded, as shown in Figure 1. Furthermore, participants with missing sarcopenia index data (n = 11,791), incomplete OBS data (n = 3,451), and missing covariates (n = 698) were omitted. As a consequence, only 6,677 participants were eligible for complete case analysis.
2.2 Outcome variable
In this study, sarcopenia was the primary outcome variable, defined as a condition related to muscle mass. To measure sarcopenia, we assessed total appendicular lean mass (ALM) via Dual-energy X-ray absorptiometry (DEXA) within the NAHNES program. Those ineligible for DEXA testing due to a height exceeding 192.5 cm, weight exceeding 136.4 kg, or pregnancy were excluded.
Sarcopenia was objectively defined by the use of the sarcopenia index, which was a composite of appendicular skeletal muscle mass and BMI. The index was calculated by dividing the total mass of the appendicular skeletal muscle (in kg) by the BMI (in kg/m2) (42, 43). For the diagnosis of sarcopenia, the index (also known as sarcopenia index, BMI-adjusted ALM or ALMBMI) had different cut-off values for men (0.789) and women (0.512), according to guidelines from the National Institutes of Health (FNIH). These cut-off values were used to diagnose sarcopenia (43).
2.3 Exposure variable
The study required the calculation of the Oxidative Balance Score (OBS) through a compilation of pro-oxidant and antioxidant elements derived from dietary and lifestyle factors (31). The dietary-based OBS components comprising 16 nutrients, including dietary fiber, carotene, riboflavin, niacin, vitamin B6, total folate, vitamin B12, vitamin C, vitamin E, calcium, magnesium, zinc, copper, selenium, total fat and iron were obtained from 24-h dietary recall interviews. The interviews consisted of two sessions: the first one in person and the second one by phone 3–10 days later. However, this study only analyzed the data from the first interview as the main predictor. This was to ensure the reliability of the self-reported dietary intake and avoid confounding effects of variations in other physiological and biochemical markers and health conditions measured during the interview owing to the long time gap.
The lifestyle-based OBS components evaluated lifestyle factors such as physical activity, BMI, alcohol consumption and smoking status. Among them, total fat, iron, BMI, alcohol consumption, and smoking status were considered pro-oxidant, while the remaining variables were considered antioxidant.
To determine the physical activity score, we adhered to the NHANES guidelines and calculated metabolic equivalent (MET) scores. The scores included work-related (vigorous and moderate) and leisure-time physical activities (vigorous and moderate), as well as walking or bicycling for transportation. MET scores = weekly frequency of each physical activity * duration of each physical activity* each physical activity suggested MET Scores. The smoking status was evaluated using serum cotinine, a major metabolite of nicotine (44).
Following the method of calculating OBS by Zhang et al. (31), alcohol consumption was divided into three groups, heavy drinkers (≥15 g/d for women and ≥ 30 g/d for men), non-heavy drinkers (0–15 g/d for women and 0–30 g/d for men), and non-drinkers, who were assigned 0, 1, and 2 points, respectively.
Then, the components were grouped by gender and then divided into three groups on a weighted basis according to their tertiles, with antioxidants scoring 0–2 in groups 1–3, and pro-oxidants scoring 2–0 in groups 1–3, respectively (31, 45). The sum of OBSs varied from 0 to 40 points. For this study, we used total OBS, dietary OBS, and lifestyle OBS as variables. The detailed plan of OBS can be found in Table 1.
2.4 Covariates
Covariates in this study included demographic, examination, laboratory, and questionnaire data. In terms of demographics, we included age (<30, 30–40, 40–50, >50), gender, race (Mexican American, Hispanic, non-Hispanic white, non-Hispanic black, or others), education level (less than high school, high school or general educational development, more than high school), marital status (never married, married/living with partner, separated/divorced/widowed), poverty–income ratio (PIR; <1.3, 1.3–3.5, 3.5). For medical history, we considered diabetes, cardiovascular disease (CVD; including coronary heart disease, angina, heart failure, heart attack, and stroke), arthritis, cancer, and chronic kidney disease (CKD). In addition, we incorporated high-density lipoprotein cholesterol (HDL-C; mg/dL), total cholesterol (TC; mg/dL) and total energy intake (kcal). More details on variable collection methods can be found in the NHANES Survey Methods and Analysis Guide.
2.5 Statistical analysis
We took into account the recommended weights in our statistical analysis due to the specificities of NHANES, which gathered data based on a complex multistage cluster survey design, and used one-fourth of the examination weight as the new sample weight in line with NHANES’ weight selection guidelines. We present a statistical characterization of the baseline variable based on the presence or absence of Sarcopenia. Continuous variables were expressed as mean ± standard deviation, while categorical variables were presented as number (percentage). ANOVA tests were used for comparing continuous variables, and chi-square tests were employed to examine statistical differences in categorical variables between groups.
Furthermore, we conducted weighted multivariate logistic regression analyses to evaluate the association between OBS and sarcopenia by adjusting for potential confounders and calculating odds ratios (ORs) and 95% confidence intervals (CIs). Meanwhile, to investigate any linear correlation trends between OBS and sarcopenia, we converted OBS from a continuous variable to a categorical variable (quartiles). Survey weights were considered in all regression analyses. In addition, as OBS can be subdivided into dietary OBS and lifestyle OBS, and may also be strongly linked to sarcopenia, we conducted distinct studies investigating the association between the two types of OBS and sarcopenia. In the extended models, Model 1 was a crude model without covariate-adjusted; Model 2 was adjusted for age, gender, race/ethnicity, education level, PIR; Model 3 was Model 2 plus HDL-C, total cholesterol, total energy intake, diabetes, CVD, CKD, hypertension, arthritis, and cancer.
Subgroup analyses on the relationship between OBS and sarcopenia were executed in accordance with model 3, with stratified factors including gender (male/female), age (<30, 30–40, 40–50, >50), race (Mexican American, other Hispanic, non-Hispanic white, non-Hispanic black, and other), PIR (<1.3, 1.3–3.5, >3.5), educational level (<High school, High school, >High school), marital status (never married, married/living with partner, separated/divorced/widowed), hypertension (yes/no), diabetes (yes/no), cardiovascular disease (yes/no), and chronic kidney disease (yes/no), cancer (yes/no), arthritis (yes/no). Interaction tests were used to examine the consistency of the relationships between the different subgroups. Identical analyses were conducted for dietary OBS and lifestyle OBS and the results were then presented through forest plots.
Additionally, the stability of the findings was examined through sensitivity analyses. The association between OBS and sarcopenia was re-evaluated using multivariate logistic regressions after excluding extreme values of BMI below 15 kg/m2 and above 50 kg/m2. Secondly, we utilized ALMBMI, which refers to BMI-adjusted ALM, to evaluate muscle mass. We assessed the correlation between OBS and muscle mass through multiple linear regressions. Furthermore, we performed sensitivity analyses under unweighted conditions to assess the stability of the association between OBS and sarcopenia.
Statistical significance was determined when p < 0.05. All statistical analyses were conducted using R software (version 4.1.1).
3 Results
3.1 Characteristics of the study population
The characteristics of individuals grouped by sarcopenia are presented in Table 2. The study included 6,677 subjects, with a mean age (standard deviation) of 39.0 (11.71) years, comprising 3,401 (50.94%) males and 3,276 (49.06%) females. A total of 473 participants were diagnosed with Sarcopenia. Individuals with sarcopenia demonstrated a higher probability of being older, Mexican American or non-Hispanic white, having higher BMI, lower educational levels, lower income, reduced physical activity, lower HDL-C levels, and lower total energy intake (all p < 0.05), as compared to those without sarcopenia. OBS was lower in those with sarcopenia (17.456 ± 6.644) than in those without (20.606 ± 7.087), as were dietary OBS and lifestyle OBS. Furthermore, patients with sarcopenia were more prone to accompanying comorbidities such as diabetes, cardiovascular disease, arthritis, cancer, hypertension and chronic kidney disease.
Furthermore, after dividing the OBS into quartiles, it was observed that individuals in the top OBS quartile were more likely to be female, Mexican American, and non-Hispanic white in comparison to those in the bottom OBS quartile. Additionally, participants in the top OBS quartile showed higher levels of education and income in terms of socioeconomic status. Participants in the top OBS quartile were less likely to develop several diseases, including diabetes, cardiovascular disease, arthritis, hypertension, chronic kidney disease, and sarcopenia, indicating a healthier state (Supplementary Table S1).
3.2 Association between OBS and sarcopenia
As demonstrated in Table 3, we examined the relationship between OBS and risk of sarcopenia based on weighted logistic regression. When OBS was treated as a continuous variable, we observed that it was significantly associated with a lower incidence of developing sarcopenia in both crude model [OR = 0.938 (0.923, 0.954), p < 0.001] and fully adjusted model [OR = 0.942 (0.920, 0.964), p < 0.001]. When considering OBS as a categorical variable, the fully adjusted model (model 3) indicated that the highest quartile of OBS was linked to lower risk of sarcopenia prevalence when compared to the lowest quartile of OBS [OR = 0.389 (0.253, 0.599), p < 0.001]. This association persisted through various models. However, the second quartile of OBS did not demonstrate a significant association with sarcopenia risk in fully adjusted model. A trend test revealed a significant association between OBS and the risk of developing sarcopenia (p for trend<0.001).
3.3 Association between dietary OBS/lifestyle OBS and sarcopenia
After categorizing OBS into dietary and lifestyle OBS, we assessed their association with sarcopenia (Table 4). The results indicate that dietary OBS was statistically significant after adjusting for all confounders [OR = 0.961 (0.937, 0.986), p = 0.003], and a negative association with the disease was observed. Compared to lowest quartile of dietary OBS, the highest quartile of dietary OBS was significantly and negatively associated with sarcopenia [OR = 0.452 (0.280, 0.732), p = 0.003].
In the final adjusted model, lifestyle OBS was significantly associated with a reduced risk of developing sarcopenia [OR = 0.745 (0.688, 0.807), p < 0.001]. In addition, with the second, third and fourth quartiles all showing significant statistical associations, greater adherence to lifestyle OBS was associated with a lower risk of developing sarcopenia. Trend tests demonstrated that both OBS were linked to a statistically significant decreasing trend in the risk of disease prevalence (p = 0.004 and p < 0.001, respectively).
3.4 Subgroup analysis
To investigate the link between OBS and sarcopenia in diverse populations, we conducted analyses stratified by subgroups including sex, age, race, PIR, educational level, marital status, hypertension, diabetes, cardiovascular disease, chronic kidney disease, cancer and arthritis (Table 5). Our findings suggested that the degree of correlation varies among different populations. We found a significant interaction between education level and sarcopenia (p for interaction = 0.008), and the protective effect of association between OBS and sarcopenia was greater for those with higher education [OR = 0.887 (0.838, 0.940), p < 0.001] than for those with a high school education or less [OR = 0.946 (0.925, 0.968), p < 0.001]. There was also a significant interaction between arthritis and sarcopenia (p for interaction =0.027). The negative association effect of OBS with sarcopenia was significantly higher in individuals with arthritis [OR = 0.902 (0.850, 0.958), p = 0.002] compared to those without arthritis [OR = 0.951 (0.927, 0.976), p < 0.001]. For people over 50 years of age or married/living with partner, this indicator shows a significant protective effect. Individuals with comorbidities such as hypertension, diabetes, cancer were more protected from this association than those without such conditions though without significant interaction. Our results indicated that the association between OBS and sarcopenia remained negative in most subgroups, although no significant association was found in some subgroups.
In addition, significant interactions were also found in the education subgroups when examining the association between the dietary OBS or lifestyle OBS and sarcopenia. The protective effect of the associations was found to be better in more educated populations. Moreover, both indicators were negatively associated with sarcopenia in people with elder age, lower income, married/living with partner and co-morbid conditions such as hypertension, diabetes, and arthritis (Figures 2, 3).
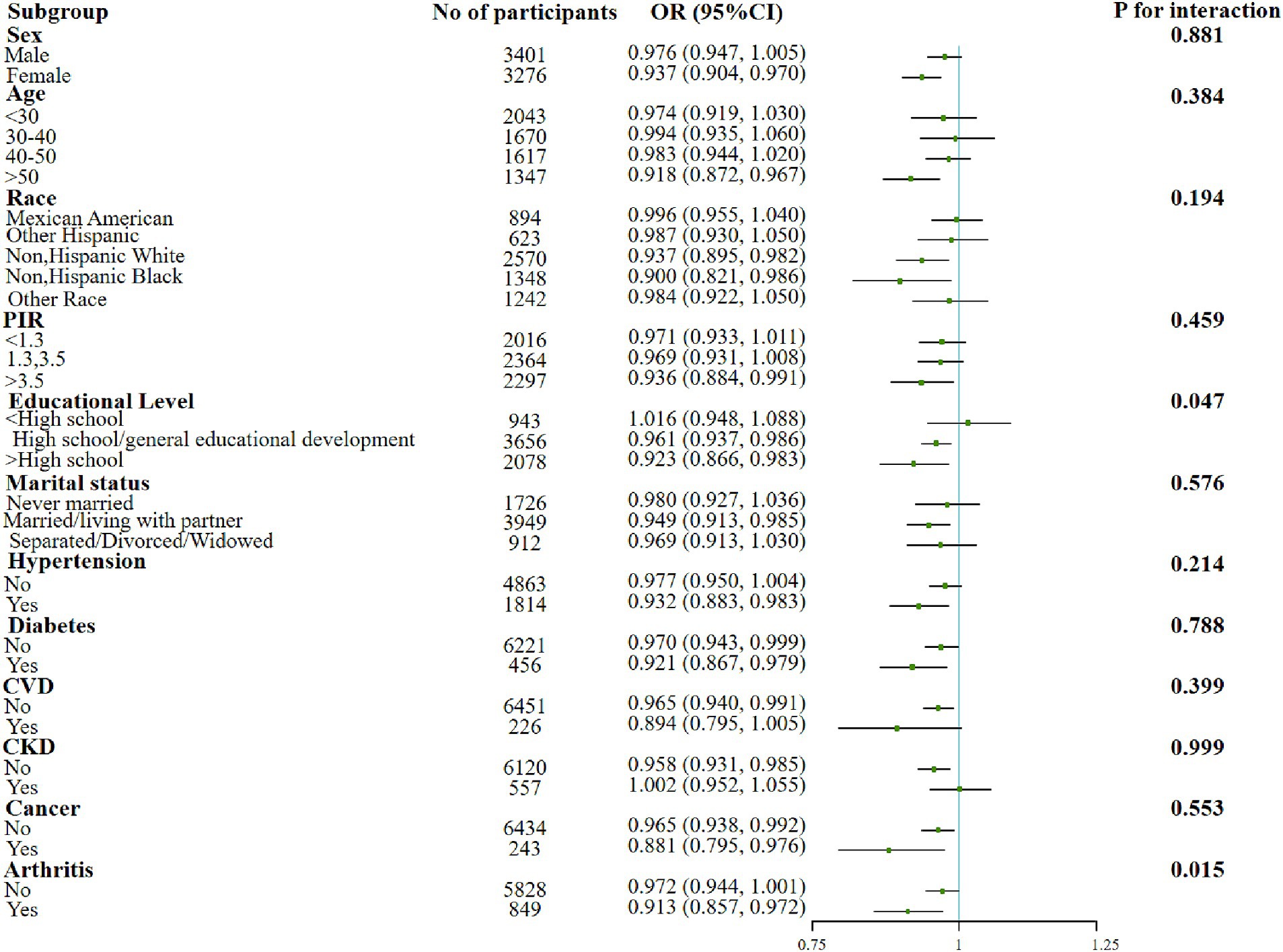
Figure 2. Forest plot for the effect of dietary OBS on sarcopenia according to different variables. After being adjusted for age, gender, race/ethnicity, education level, PIR, marital status, HDL-C, total cholesterol, total energy intake, diabetes, CVD, CKD, hypertension, arthritis, and cancer. Abbreviation: PIR, income to poverty ratio; CVD, Cardiovascular disease; CKD, Chronic Kidney Disease; HDL-C, high-density lipoprotein cholesterol; TC, total cholesterol; OBS, oxidative balance score.
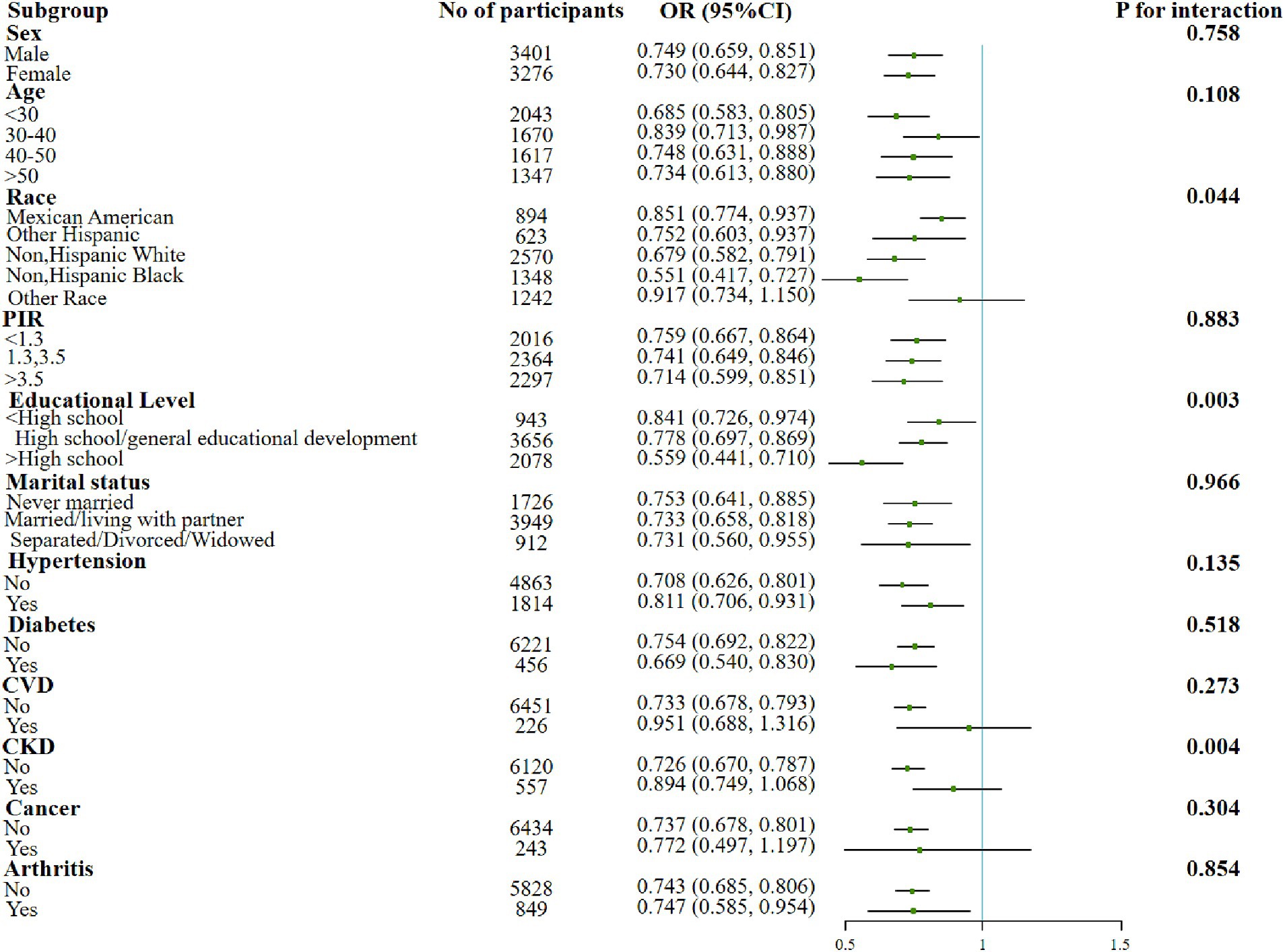
Figure 3. Forest plot for the effect of lifestyle OBS on sarcopenia according to different variables. After being adjusted for age, gender, race/ethnicity, education level, PIR, marital status, HDL-C, total cholesterol, total energy intake, diabetes, CVD, CKD, hypertension, arthritis, and cancer. Abbreviation: PIR, income to poverty ratio; CVD, Cardiovascular disease; CKD, Chronic Kidney Disease; HDL-C, high-density lipoprotein cholesterol; TC, total cholesterol; OBS, oxidative balance score.
3.5 Sensitivity analysis
We performed sensitivity analyses using multiple methods to determine the robustness of the results. Removal of BMI outliers (<15 kg/m2, n = 0) and (>60 kg/m2, n = 56; Supplementary Table S2) or under weighted condition did not significantly affect the results of correlation (Supplementary Table S3). In addition, when ALMBMI was used as a continuous variable to measure muscle mass, a significant positive association between OBS and muscle mass was observed after adjustment for confounders (p < 0.001; Supplementary Table S4). Higher OBS related to better muscle mass, indicating a lower risk of sarcopenia. The correlation be-tween OBS and muscle mass status would therefore be more effectively validated.
4 Discussion
To elucidate the association between OBS and sarcopenia, we conducted cross-sectional analyses on 6,677 individuals from the NHANES database. Our study revealed that total OBS, dietary OBS, and lifestyle OBS are all negatively correlated with the prevalence of sarcopenia. Furthermore, the high OBS category significantly reduced the risk of sarcopenia compared to the lowest category, even after accounting for potential confounding factors. Additionally, the link between OBS and sarcopenia was particularly pronounced in older age categories, lower incomes, higher education, and individuals suffering from hypertension, diabetes, cancer, and arthritis. In summary, a higher OBS score corresponded to a lower likelihood of developing sarcopenia. Our research also underscored the importance of maintaining a healthy diet and life-style, with notable implications for public healthcare.
Sarcopenia, an age-related muscle wasting disease, has become a serious public health concern. Its complexity defies easy explanation, despite many studies. Several lines of evidence implicate that oxidative stress and inflammation are linked to sarcopenia onset and development. Aging impairs muscle nutrition and mitochondrial function, boosting ROS levels. With aging, both muscle nutrition and mitochondrial function declined, boosting the levels of reactive oxygen species (ROS). The accumulation of ROS increases protein hydrolysis, resulting in loss of muscle mass (46–48). Elevated levels of inflammatory biomarkers, such as C-reactive protein (CRP), inter-leukin-6 (IL-6), and α-tumor necrosis factor (TNFα), have been implicated in promoting muscle loss and are associated with decreased muscle mass and strength in older adults. The detrimental impact of oxidative stress on muscle tissue is exacerbated by the increased expression of inflammatory cytokines (39, 49). Inflammatory diets may contribute to muscle loss by fostering oxidative stress, systemic inflammation, and reduced insulin (50). Consequently, maintaining low levels of systemic inflammation and reducing oxidative stress is pivotal in averting the risk of developing sarcopenia.
In this study, we explored the relationship between OBS and the risk of sarcopenia. OBS, functioning as a comprehensive measure of oxidative or antioxidant balance, has the potential to offer deeper insights into the overall oxidative stress status of the body. While OBS is constrained to external exposures like dietary and non-dietary lifestyle factors, multiple studies have demonstrated a correlation between OBS and inflammation markers (such as CRP) (51) and indicators of oxidative stress (such as F2-isoprostane) (52) and gamma glutamyl transferase, GGT (53), affirming the reliability of OBS in assessing oxidative homeostasis (35). Moreover, the emphasis on external exposures aligns with the daily lives of the general population, making it easier to provide relevant guidance to healthcare providers for enhancing population health. Our results indicated that higher oxidative balance score (OBS) might confer antioxidant advantage over pro-oxidant exposure and was significantly negatively associated with sarcopenia risk.
Our findings align with previous research indicating a correlation between dietary factors and sarcopenia. Diets abundant in fruits and vegetables are shown to prevent metabolic acidosis, diminish protein hydrolysis and amino acid catabolism, and lower the likelihood of sarcopenia (11). Diets high in fiber play a crucial role in reducing the risk of lower lean muscle mass (54). The Mediterranean diet is characterized by low levels of inflammation and antioxidants found in plant-based foods, healthy fats and whole grains, which have beneficial effects on muscle mass, physical function and the prevention of sarcopenia (55). On the flip side, the consumption of foods rich in saturated fat can be detrimental to muscle health. The risk of muscle loss may also be heightened by adhering to a Western dietary pattern, characterized by the frequent intake of flour, bread, potatoes, red meat, processed meat, eggs, and cheese (56).
Moreover, certain antioxidant and anti-inflammatory nutrients, such as vitamins E and C, omega-3 fatty acids, MUFA, PUFA, and caffeine, may contribute to a reduction in the prevalence of sarcopenia (57). The UK Biobank population-wide cross-sectional analysis study (58) and the Maastricht Sarcopenia Study (59) found a negative association between magnesium intake and sarcopenia, and older adults with sarcopenia had lower daily magnesium intakes. Another case–control study compared 66 older adults without decreased muscle mass with previously recruited adults with sarcopenia and found that selenium intake was significantly lower in the case group (60). In a cross-sectional analysis of 396,283 individuals from the UK Biobank, a higher in-take of calcium or potassium was associated with lower odds of having sarcopenia (58). Antioxidant-rich diets may alter the course of sarcopenia by modulating the phase angle (PA). As a measure of cell membrane integrity and cellular health, PA was positively correlated with dietary intake of antioxidants. Decreased PA values may indicate impaired cell membrane function, which is associated with muscle tissue degeneration and loss of muscle mass. Research supports the use of PA as a biomarker to predict sarcopenia. Lower PA values were associated with a higher risk of sarcopenia, which emphasizes the important role of antioxidant-rich diets in reducing the risk of this disease (61, 62).
Several studies also have examined the relationship between life-style and sarcopenia and muscle mass, and these variables were included in our OBS components. Two studies conducted in Korea have established a correlation between alcohol intake and a higher prevalence of sarcopenia among women (63, 64). Similar findings were reported in animal studies, indicating an association between alcohol consumption and skeletal muscle mass loss (65, 66). Alcohol caused a reduction in mammalian/rapamycin (mTOR) kinase activity inhibited overall protein synthesis, and led to reduced protein synthesis in skeletal muscle and dysregulated responses to anabolic stimuli in enzymatic or several anabolic stimuli (67–69). In addition, a study from the SarcoPhAge cohort found that individuals who smoked more had more severe sarcopenia (70), and Rom et al. (71) demonstrated that smoking contributes to the breakdown of muscle proteins by oxidative stress. In a study in mice, Nogueira et al. (72) illustrated that smoking-induced capillary deconditioning contributes to the decline in skeletal muscle function. Collectively, smoking and chronic alcohol abuse interfered with muscle protein metabolism pathways, reducing skeletal muscle mass and in-creasing the risk of sarcopenia. Furthermore, these habits contributed to conditions such as periodontal disease, chronic lung, and liver disease, amplifying the risk of musculoskeletal disorders (73).
Furthermore, a wealth of studies indicated that physical activity, encompassing aerobic, resistance, and multimodal exercise, has the potential to enhance or sustain muscle function in older adults with sarcopenia (74). Numerous meta-analyses further underscored a positive correlation between engaging in any form of physical activity and a reduced likelihood of developing sarcopenia, promoting the preservation of muscle mass (70). The act of muscle cells producing reactive oxygen species (ROS) in response to exercise can serve to either trigger or inhibit various genes. This process enhances antioxidant defenses and suppresses the activation of inflammatory pathways, thereby fortifying the organism’s resistance to free radicals (75–77). Consequently, the most effective strategy for improving sarcopenia and averting disability involves regular physical activity coupled with appropriate nutritional support (78).
As age advances, there is a concurrent rise in visceral adipose tissue and a decline in muscle mass, culminating in the emergence of sarcopenia coexisting with obesity, commonly referred to as sarcopenic obesity. Indications of this condition encompass a heightened body mass index and diminished muscle mass (79, 80). Independently, obesity is linked with oxidative stress, a factor that intensifies with age and increasing body mass index (BMI), consequently progressively weakening the body’s antioxidant status (81). Both low-grade systemic inflammation and the accumulation of intramuscular fat contribute to mitochondrial dysfunction and impaired mitochondrial beta-oxidation. This cascade results in elevated lipid peroxidation, leading to the increased accumulation of lipid intermediates and reactive oxygen metabolites (ROM), thereby escalating inflammation, oxidative stress, and insulin resistance (IR) (82). Additionally, myocytes in this state release myokines, including the muscle growth-inhibiting hormone, irisin, TNF-alpha, and interleukin (IL), exacerbating IR in adipose tissue and other tissues (82, 83). A recent meta-analysis has revealed a correlation between sarcopenic obesity, especially in men, and a higher rate of all-cause mortality (84). Increased oxidative stress in sarcopenia was associated with high risk of cardiovascular disease in sarcopenic obesity (85). To preempt disease, it is imperative to adopt a healthy diet and engage in regular exercise. However, weight loss must be coupled with adequate protein intake and exercise, recognizing that obesity is a multifactorial condition, and weight loss alone can exacerbate muscle loss (86–88).
Our findings suggest that the protective effect of the association between OBS and sarcopenia is more pronounced in higher age brackets. Emphasizing the significance of this association in preventing and predicting disease within the geriatric cohort is crucial due to the heightened likelihood of gradual skeletal muscle dysfunction and atrophy in middle-aged and older individuals as a consequence of aging (2, 79). Both male and female OBS were significantly associated with sarcopenia, but the protective effect is stronger for females than for males. This difference may be due to the greater expression of antioxidant genes and enzyme activities in women, which may result in better maintenance of antioxidant capacity and a reduction in disease risk (89, 90). Moreover, a correlation between OBS and sarcopenia that is influenced by the level of education is also indicated in our findings. Educational attainment serves as an indicator of the ability to translate nutritional knowledge into improved dietary habits (91). It is plausible that individuals with higher education levels place greater emphasis on their diet and lifestyle to ensure good health. Similarly, married or partnered individuals tend to adopt healthier lifestyles and diets with their partner’s or family’s support, which links lower oxidative stress and disease risk (92).
Additionally, significant correlations between OBS and sarcopenia were observed in individuals with diabetes, hypertension, or arthritis. Our investigation revealed an increased prevalence of sarcopenia in individuals with these conditions, demonstrating a robust association with pro-inflammatory elements, oxidative stress, and other pathological mechanisms (9, 79, 93–96). Moreover, each of these diseases has been negatively associated with OBS in previous studies (34, 97–99). Therefore, assessing the antioxidant status of patients with diabetes, hypertension, or arthritis via OBS holds significant public health implications for preventing muscle osteoporosis.
Our study had several strengths. Firstly, to our knowledge, it represents the inaugural investigation assessing the relationship between OBS and sarcopenia within the general population. Secondly, we leveraged the NHANES database, renowned for its extensive sample size and accurate national representation. This enabled us to adjust for additional confounding variables, ensuring the reliability of our outcomes. Thirdly, our study differentiated itself by concentrating on OBS, a composite indicator rather than a single component, in order to comprehensively understand the complex relationships among factors and thoroughly examine the outcomes of interest.
However, our study presents several limitations. Firstly, being a cross-sectional analysis, it poses challenges in establishing a clear causal relationship. Additionally, the reliance on self-reported medical conditions introduces the potential for recall bias. To ascertain the precise relationship between OBS and the risk of sarcopenia, further prospective studies are essential. Secondly, the overall OBS score may either underestimate or overestimate pro-oxidant and antioxidant biological effects due to the equal weighting of all components. Additionally, there is a likelihood of threshold effects with high antioxidant doses, potentially inducing toxic pro-oxidant activity. Thirdly, despite our efforts to adjust for several confounders, the possibility of additional con-founding in our analyses and subgroup analyses cannot be entirely excluded. Due to the limitations of the NHANES database for obtaining data regarding the assessment of sarcopenia, such as age, height, and weight restrictions, this study focused only on the eligible adult population. However, for the high-risk elderly population, the use of OBS to assess disease risk is particularly important and the relevance of OBS to the disease in this age group necessitates further analysis.
5 Conclusion
Our study revealed a noteworthy association between OBS and the prevalence of sarcopenia in US adults. It underscored the importance of adopting an antioxidant-rich diet and implementing lifestyle modifications to mitigate the prevalence of sarcopenia. Nevertheless, it was crucial to acknowledge that factors such as education level, income situation, or the presence of chronic diseases could influence the observed results. While the practicality of using OBS to assess antioxidant levels in elderly individuals and those with diabetes, hypertension, or arthritis is potentially possible, further studies are warranted to delve deeper into these associations.
Data availability statement
Publicly available datasets were analyzed in this study. This data can be found at: https://www.cdc.gov/nchs/nhanes/.
Ethics statement
The studies involving humans were approved by the National Center for Health Statistics Research Ethics Review Board. The studies were conducted in accordance with the local legislation and institutional requirements. The participants provided their written informed consent to participate in this study. Written informed consent was obtained from the individual(s) for the publication of any potentially identifiable images or data included in this article.
Author contributions
WX: Conceptualization, Data curation, Investigation, Software, Validation, Writing – original draft. DM: Conceptualization, Formal analysis, Funding acquisition, Investigation, Writing – original draft. YuW: Formal analysis, Funding acquisition, Investigation, Supervision, Writing – review & editing. YiW: Data curation, Funding acquisition, Investigation, Software, Supervision, Writing – review & editing. CW: Data curation, Methodology, Software, Writing – review & editing. XZ: Data curation, Supervision, Validation, Writing – review & editing.
Funding
The author(s) declare financial support was received for the research, authorship, and/or publication of this article. This research was supported by the National Natural Science Foundation of China (72374081), and the Science and Technology Development Plan of Jilin Province (YDZJ202301ZYTS001 and YDZJ202301ZYTS050).
Acknowledgments
The authors express sincere appreciation to all the participants and staff of the National Health and Nutrition Examination Survey for their invaluable contributions to the col-lection, administration, and publication of the data.
Conflict of interest
The authors declare that the research was conducted in the absence of any commercial or financial relationships that could be construed as a potential conflict of interest.
Publisher’s note
All claims expressed in this article are solely those of the authors and do not necessarily represent those of their affiliated organizations, or those of the publisher, the editors and the reviewers. Any product that may be evaluated in this article, or claim that may be made by its manufacturer, is not guaranteed or endorsed by the publisher.
Supplementary material
The Supplementary material for this article can be found online at: https://www.frontiersin.org/articles/10.3389/fnut.2024.1342113/full#supplementary-material
References
1. Rosenberg, IH. Sarcopenia: origins and clinical relevance. J Nutr. (1997) 127:990S–1S. doi: 10.1093/jn/127.5.990S
2. Tournadre, A, Vial, G, Capel, F, Soubrier, M, and Boirie, Y. Sarcopenia. Joint Bone Spine. (2019) 86:309–14. doi: 10.1016/j.jbspin.2018.08.001
3. Cruz-Jentoft, AJ, and Sayer, AA. Sarcopenia. Lancet. (2019) 393:2636–46. doi: 10.1016/s0140-6736(19)31138-9
4. Bloom, I, Shand, C, Cooper, C, Robinson, S, and Baird, J. Diet quality and sarcopenia in older adults: a systematic review. Nutrients. (2018) 10:308. doi: 10.3390/nu10030308
5. Yeung, SSY, Reijnierse, EM, Pham, VK, Trappenburg, MC, Lim, WK, Meskers, CGM, et al. Sarcopenia and its association with falls and fractures in older adults: a systematic review and Meta-analysis. J Cachexia Sarcopenia Muscle. (2019) 10:485–500. doi: 10.1002/jcsm.12411
6. Kamper, RS, Alcazar, J, Andersen, LL, Haddock, B, Jorgensen, NR, Hovind, P, et al. Associations between inflammatory markers, body composition, and physical function: the Copenhagen sarcopenia study. J Cachexia Sarcopenia Muscle. (2021) 12:1641–52. doi: 10.1002/jcsm.12832
7. Beaudart, C, Zaaria, M, Pasleau, F, Reginster, J-Y, and Bruyere, O. Health outcomes of sarcopenia: a systematic review and Meta-analysis. PLoS One. (2017) 12:e0169548. doi: 10.1371/journal.pone.0169548
8. Dai, S, Shu, D, Meng, F, Chen, Y, Wang, J, Liu, X, et al. Higher risk of sarcopenia in older adults with type 2 diabetes: Nhanes 1999-2018. Obes Facts. (2023) 16:237–48. doi: 10.1159/000530241
9. Chen, S, Han, H, Jin, J, Zhou, G, and Li, Z. Osteoarthritis and sarcopenia-related traits: the cross-sectional study from Nhanes 2011-2014 and Mendelian randomization study. J Orthop Surg Res. (2023) 18:502. doi: 10.1186/s13018-023-03960-w
10. Wathanavasin, W, Banjongjit, A, Avihingsanon, Y, Praditpornsilpa, K, Tungsanga, K, Eiam-Ong, S, et al. Prevalence of sarcopenia and its impact on cardiovascular events and mortality among Dialysis patients: a systematic review and Meta-analysis. Nutrients. (2022) 14:4077. doi: 10.3390/nu14194077
11. Chen, W, Shi, S, Jiang, Y, Chen, K, Liao, Y, Huang, R, et al. Association of Sarcopenia with ideal cardiovascular health metrics among us adults: a cross-sectional study of Nhanes data from 2011 to 2018. BMJ Open. (2022) 12:e061789. doi: 10.1136/bmjopen-2022-061789
12. Damluji, AA, Alfaraidhy, M, AlHajri, N, Rohant, NN, Kumar, M, Al Malouf, C, et al. Sarcopenia and cardiovascular diseases. Circulation. (2023) 147:1534–53. doi: 10.1161/circulationaha.123.064071
13. Kim, S-E, and Kim, DJ. Sarcopenia as a prognostic Indicator of liver cirrhosis. J Cachexia Sarcopenia Muscle. (2021) 13:8–10. doi: 10.1002/jcsm.12869
14. Tuttle, CSL, Thang, LAN, and Maier, AB. Markers of inflammation and their association with muscle strength and mass: a systematic review and Meta-analysis. Ageing Res Rev. (2020) 64:101185. doi: 10.1016/j.arr.2020.101185
15. Calvani, R, Marini, F, Cesari, M, Tosato, M, Picca, A, Anker, SD, et al. Biomarkers for physical frailty and sarcopenia. Aging Clin Exp Res. (2017) 29:29–34. doi: 10.1007/s40520-016-0708-1
16. Curcio, F, Ferro, G, Basile, C, Liguori, I, Parrella, P, Pirozzi, F, et al. Biomarkers in sarcopenia: a multifactorial approach. Exp Gerontol. (2016) 85:1–8. doi: 10.1016/j.exger.2016.09.007
17. Chhetri, JK, Barreto, PS, Fougere, B, Rolland, Y, Vellas, B, and Cesari, M. Chronic inflammation and sarcopenia: a regenerative cell therapy perspective. Exp Gerontol. (2018) 103:115–23. doi: 10.1016/j.exger.2017.12.023
18. Cannataro, R, Carbone, L, Petro, JL, Cione, E, Vargas, S, Angulo, H, et al. Sarcopenia: etiology, nutritional approaches, and Mirnas. Int J Mol Sci. (2021) 22:9724. doi: 10.3390/ijms22189724
19. Marzetti, E, Calvani, R, Cesari, M, Buford, TW, Lorenzi, M, Behnke, BJ, et al. Mitochondrial dysfunction and sarcopenia of aging: from signaling pathways to clinical trials. Int J Biochem Cell Biol. (2013) 45:2288–301. doi: 10.1016/j.biocel.2013.06.024
20. Danieli, MG, Antonelli, E, Piga, MA, Cozzi, MF, Allegra, A, and Gangemi, S. Oxidative stress, mitochondrial dysfunction, and respiratory chain enzyme defects in inflammatory myopathies. Autoimmun Rev. (2023) 22:103308. doi: 10.1016/j.autrev.2023.103308
21. Wu, D, Wang, H, Wang, W, Qing, C, Zhang, W, Gao, X, et al. Association between composite dietary antioxidant index and handgrip strength in American adults: Data from National Health and nutrition examination survey (Nhanes, 2011-2014). Front Nutr. (2023) 10:1147869. doi: 10.3389/fnut.2023.1147869
22. Hernandez-Ruiz, A, Garcia-Villanova, B, Guerra-Hernandez, EJ, Carrion-Garcia, CJ, Amiano, P, Sanchez, M-J, et al. Oxidative balance scores (Obss) integrating nutrient, food and lifestyle dimensions: development of the Nutrientl-Obs and Foodl-Obs. Antioxidants. (2022) 11:300. doi: 10.3390/antiox11020300
23. He, L, He, T, Farrar, S, Ji, L, Liu, T, and Ma, X. Antioxidants maintain cellular redox homeostasis by elimination of reactive oxygen species. Cell Physiol Biochem. (2017) 44:532–53. doi: 10.1159/000485089
24. Forman, HJ, and Zhang, H. Targeting oxidative stress in disease: promise and limitations of antioxidant therapy (Jun, 10.1038/S41573-021-00233-1, 2021). Nat Rev Drug Discov. (2021) 20:652. doi: 10.1038/s41573-021-00267-5
25. Rani, V, Deep, G, Singh, RK, Palle, K, and Yadav, UC. Oxidative stress and metabolic disorders: pathogenesis and therapeutic strategies. Life Sci. (2016) 148:183–93. doi: 10.1016/j.lfs.2016.02.002
26. Donia, T, and Khamis, A. Management of Oxidative Stress and Inflammation in cardiovascular diseases: mechanisms and challenges. Environ Sci Pollut Res. (2021) 28:34121–53. doi: 10.1007/s11356-021-14109-9
27. Chikara, S, Nagaprashantha, LD, Singhal, J, Horne, D, Awasthi, S, and Singhal, SS. Oxidative stress and dietary phytochemicals: role in Cancer chemoprevention and treatment. Cancer Lett. (2018) 413:122–34. doi: 10.1016/j.canlet.2017.11.002
28. Li, W, Guo, Y, Zhang, C, Wu, R, Yang, AY, Gaspar, J, et al. Dietary phytochemicals and Cancer chemoprevention: a perspective on oxidative stress, inflammation, and epigenetics. Chem Res Toxicol. (2016) 29:2071–95. doi: 10.1021/acs.chemrestox.6b00413
29. Schrag, M, Mueller, C, Zabel, M, Crofton, A, Kirsch, WM, Ghribi, O, et al. Oxidative stress in blood in Alzheimer’s disease and mild cognitive impairment: a Meta-analysis. Neurobiol Dis. (2013) 59:100–10. doi: 10.1016/j.nbd.2013.07.005
30. Goodman, M, Bostick, RM, Dash, C, Flanders, WD, and Mandel, JS. Hypothesis: oxidative stress score as a combined measure of pro-oxidant and antioxidant exposures. Ann Epidemiol. (2007) 17:394–9. doi: 10.1016/j.annepidem.2007.01.034
31. Zhang, W, Peng, S-F, Chen, L, Chen, H-M, Cheng, X-E, and Tang, Y-H. Association between the oxidative balance score and telomere length from the National Health and nutrition examination survey 1999-2002. Oxidative Med Cell Longev. (2022) 2022:1–11. doi: 10.1155/2022/1345071
32. Golmohammadi, M, Ayremlou, P, and Zarrin, R. Higher oxidative balance score is associated with better glycemic control among Iranian adults with Type-2 diabetes. Int J Vitamin Nutrition Res Internationale Zeitschrift fur Vitamin- und Ernahrungsforschung J I de vitaminologie et de nutrition. (2021) 91:31–9. doi: 10.1024/0300-9831/a000596
33. Ilori, TO, Wang, X, Huang, M, Gutierrez, OM, Narayan, KMV, Goodman, M, et al. Oxidative balance score and the risk of end-stage renal disease and cardiovascular disease. Am J Nephrol. (2017) 45:338–45. doi: 10.1159/000464257
34. Lee, J-H, Joo, YB, Han, M, Kwon, SR, Park, W, Park, K-S, et al. Relationship between oxidative balance score and quality of life in patients with osteoarthritis data from the Korea National Health and nutrition examination survey (2014-2015). Medicine. (2019) 98:e16355. doi: 10.1097/md.0000000000016355
35. Cho, AR, Kwon, Y-J, and Lee, J-H. Oxidative balance score is inversely associated with the incidence of non-alcoholic fatty liver disease. Clin Nutr. (2023) 42:1292–300. doi: 10.1016/j.clnu.2023.06.015
36. Li, H, Song, L, Cen, M, Fu, X, Gao, X, Zuo, Q, et al. Oxidative balance scores and depressive symptoms: mediating effects of oxidative stress and inflammatory factors. J Affect Disord. (2023) 334:205–12. doi: 10.1016/j.jad.2023.04.134
37. Hernandez-Ruiz, A, Garcia-Villanova, B, Guerra-Hernandez, E, Amiano, P, Ruiz-Canela, M, and Molina-Montes, E. A review of a priori defined oxidative balance scores relative to their components and impact on health outcomes. Nutrients. (2019) 11:774. doi: 10.3390/nu11040774
38. Picca, A, Lozanoska-Ochser, B, Calvani, R, Coelho-Junior, HJ, Leewenburgh, C, and Marzetti, E. Inflammatory, mitochondrial, and senescence-related markers: underlying biological pathways of muscle aging and new therapeutic targets. Exp Gerontol. (2023) 178:112204. doi: 10.1016/j.exger.2023.112204
39. Schaap, LA, Pluijm, SMF, Deeg, DJH, and Visser, M. Inflammatory markers and loss of muscle mass (sarcopenia) and strength. Am J Med. (2006) 119:526.e9–526.e17. doi: 10.1016/j.amjmed.2005.10.049
40. Centers for Disease Control and Prevention, National Center for Health Statistics. About the National Health and nutrition examination survey. (2023). Available at: https://www.Cdc.Gov/Nchs/Nhanes/About_Nhanes.Htm (Accessed August 22, 2023).
41. Zipf, G, Chiappa, M, Porter, KS, Ostchega, Y, Lewis, BG, and Dostal, J. National Health and nutrition examination survey: plan and operations, 1999-2010. Vital and health statistics Ser 1, Programs and collection procedures. (2013) 56:1–37.
42. Baumgartner, RN. Epidemiology of sarcopenia among the elderly in New Mexico. Am J Epidemiol. (1999) 147:755–63. doi: 10.1093/oxfordjournals.aje.a009520
43. Studenski, SA, Peters, KW, Alley, DE, Cawthon, PM, McLean, RR, Harris, TB, et al. The Fnih sarcopenia project: rationale, study description, conference recommendations, and final estimates. J Gerontol Series a-Biolog Sci Medical Sci. (2014) 69:547–58. doi: 10.1093/gerona/glu010
44. Tian, X, Xue, B, Wang, B, Lei, R, Shan, X, Niu, J, et al. Physical activity reduces the role of blood cadmium on depression: a cross-sectional analysis with nhanes data. Environmental pollution (Barking, Essex: 1987). (2022) 304:119211. doi: 10.1016/j.envpol.2022.119211
45. Lei, X, Xu, Z, and Chen, W. Association of Oxidative Balance Score with sleep quality: Nhanes 2007-2014. J Affect Disord. (2023) 339:435–42. doi: 10.1016/j.jad.2023.07.040
46. Szentesi, P, Csernoch, L, Dux, L, and Keller-Pinter, A. Changes in redox signaling in the skeletal muscle with aging. Oxidative Med Cell Longev. (2019) 2019:1–12. doi: 10.1155/2019/4617801
47. Jackson, MJ, and McArdle, A. Age-related changes in skeletal muscle reactive oxygen species generation and adaptive responses to reactive oxygen species. J Physiology-London. (2011) 589:2139–45. doi: 10.1113/jphysiol.2011.206623
48. Giorgi, C, Marchi, S, Simoes, ICM, Ren, Z, Morciano, G, Perrone, M, et al. Mitochondria and reactive oxygen species in aging and age-related diseases. Int. Rev. Cell Mol. Biol. (2018) 340:209–344. doi: 10.1016/bs.ircmb.2018.05.006
49. Morley, JE, and Baumgartner, RN. Cytokine-related aging process. J Gerontol A Biol Sci Med Sci. (2004) 59:M924–9. doi: 10.1093/gerona/59.9.m924
50. Huang, Y, Zeng, M, Zhang, L, Shi, J, Yang, Y, Liu, F, et al. Dietary inflammatory potential is associated with sarcopenia among chronic kidney disease population. Front Nutr. (2022) 9:9. doi: 10.3389/fnut.2022.856726
51. Kong, SYJ, Bostick, RM, Flanders, WD, McClellan, WM, Thyagarajan, B, Gross, MD, et al. Oxidative balance score, colorectal adenoma, and markers of oxidative stress and inflammation. Cancer Epidemiol Biomarkers Prev. (2014) 23:545–54. doi: 10.1158/1055-9965.Epi-13-0619
52. Lakkur, S, Bostick, RM, Roblin, D, Ndirangu, M, Okosun, I, Annor, F, et al. Oxidative balance score and oxidative stress biomarkers in a study of whites, African Americans, and African immigrants. Biomarkers. (2014) 19:471–80. doi: 10.3109/1354750x.2014.937361
53. Cho, AR, Kwon, Y-J, Lim, H-J, Lee, HS, Kim, S, Shim, J-Y, et al. Oxidative balance score and serum gamma-Glutamyltransferase level among Korean adults: a Nationwide population-based study. Eur J Nutr. (2018) 57:1237–44. doi: 10.1007/s00394-017-1407-1
54. Montiel-Rojas, D, Santoro, A, Nilsson, A, Franceschi, C, Capri, M, Bazzocchi, A, et al. Beneficial role of replacing dietary saturated fatty acids with polyunsaturated fatty acids in the prevention of sarcopenia: findings from the nu-age cohort. Nutrients. (2020) 12:3079. doi: 10.3390/nu12103079
55. Papadopoulou, SK, Detopoulou, P, Voulgaridou, G, Tsoumana, D, Spanoudaki, M, Sadikou, F, et al. Mediterranean diet and sarcopenia features in apparently healthy adults over 65 years: a systematic review. Nutrients. (2023) 15:1104. doi: 10.3390/nu15051104
56. Vijayakumar, A, Kim, Y, Kim, H, and Kwon, O. Western dietary pattern is associated with higher risk of lower lean muscle mass in Korean postmenopausal women: data from the Korea National Health and nutrition examination survey 2008-2011. Nutr Res Pract. (2021) 15:528–40. doi: 10.4162/nrp.2021.15.4.528
57. Papaioannou, K-G, Nilsson, A, Nilsson, LM, and Kadi, F. Healthy eating is associated with sarcopenia risk in physically active older adults. Nutrients. (2021) 13:2813. doi: 10.3390/nu13082813
58. Petermann-Rocha, F, Chen, M, Gray, SR, Ho, FK, Pell, JP, and Celis-Morales, C. Factors associated with sarcopenia: a cross-sectional analysis using Uk biobank. Maturitas. (2020) 133:60–7. doi: 10.1016/j.maturitas.2020.01.004
59. ter Borg, S, de Groot, LCPGM, Mijnarends, DM, de Vries, JHM, Verlaan, S, Meijboom, S, et al. Differences in nutrient intake and biochemical nutrient status between Sarcopenic and Nonsarcopenic older adults-results from the Maastricht sarcopenia study. J Am Med Dir Assoc. (2016) 17:393–401. doi: 10.1016/j.jamda.2015.12.015
60. Verlaan, S, Aspray, TJ, Bauer, JM, Cederholm, T, Hemsworth, J, Hill, TR, et al. Nutritional status, body composition, and quality of life in community dwelling Sarcopenic and non-Sarcopenic older adults: a case-control study. Clin Nutr. (2017) 36:267–74. doi: 10.1016/j.clnu.2015.11.013
61. Wu, H, Ding, P, Wu, J, Yang, P, Tian, Y, and Zhao, Q. Phase angle derived from bioelectrical impedance analysis as a marker for predicting sarcopenia. Front Nutr. (2022) 9:1060224. doi: 10.3389/fnut.2022.1060224
62. Detopoulou, P, Fragopoulou, E, Nomikos, T, and Antonopoulou, S. Associations of phase angle with platelet-activating factor metabolism and related dietary factors in healthy volunteers. Front Nutr. (2023) 10:1237086. doi: 10.3389/fnut.2023.1237086
63. Yoo, J-I, Ha, Y-C, Lee, Y-K, Hana, C, Yoo, M-J, and Koo, K-H. High prevalence of sarcopenia among binge drinking elderly women: a Nationwide population-based study. BMC Geriatr. (2017) 17:17. doi: 10.1186/s12877-017-0507-3
64. Kwon, Y-J, Lim, H-J, Lee, Y-J, Lee, H-S, Linton, JA, Lee, JW, et al. Associations between high-risk alcohol consumption and sarcopenia among postmenopausal women. Menopause-the J North American Menopause Society. (2017) 24:1022–7. doi: 10.1097/gme.0000000000000879
65. Steiner, JL, and Lang, CH. Alcohol impairs skeletal muscle protein synthesis and Mtor signaling in a time-dependent manner following electrically stimulated muscle contraction. J Appl Physiol (1985). (2014) 117:1170–9. doi: 10.1152/japplphysiol.00180.2014
66. Adachi, J, Asano, M, Ueno, Y, Niemela, O, Ohlendieck, K, Peters, TJ, et al. Alcoholic muscle disease and biomembrane perturbations (review). J Nutr Biochem. (2003) 14:616–25. doi: 10.1016/s0955-2863(03)00114-1
67. Lang, CH, Pruznak, AM, Nystrom, GJ, and Vary, TC. Alcohol-induced decrease in muscle protein synthesis associated with increased binding of Mtor and raptor: comparable effects in young and mature rats. Nutrition & metabolism. (2009) 6:4. doi: 10.1186/1743-7075-6-4
68. Steiner, JL, Kimball, SR, and Lang, CH. Acute alcohol-induced decrease in muscle protein synthesis in female mice is Redd-1 and Mtor-independent. Alcohol and alcoholism (Oxford, Oxfordshire). (2016) 51:242–50. doi: 10.1093/alcalc/agv105
69. Xia, X, Xiang, S, Hua, L, Sun, Q, and Wang, R. The relationship between lifestyles and sarcopenia-related traits: a two-sample Mendelian randomization study. Arch Gerontol Geriatr. (2023) 116:105169. doi: 10.1016/j.archger.2023.105169
70. Steffl, M, Bohannon, RW, Sontakova, L, Tufano, JJ, Shiells, K, and Holmerova, I. Relationship between sarcopenia and physical activity in older people: a systematic review and Meta-analysis. Clin Interv Aging. (2017) 12:835–45. doi: 10.2147/cia.S132940
71. Rom, O, Kaisari, S, Aizenbud, D, and Reznick, AZ. Sarcopenia and smoking: a possible cellular model of cigarette smoke effects on muscle protein breakdown. Ann. New York Acad. Sci. (2012) 1259:47–53. doi: 10.1111/j.1749-6632.2012.06532.x
72. Nogueira, L, Trisko, BM, Lima-Rosa, FL, Jackson, J, Lund-Palau, H, Yamaguchi, M, et al. Cigarette smoke directly impairs skeletal muscle function through capillary regression and altered Myofibre calcium kinetics in mice! J Physiology-London. (2018) 596:2901–16. doi: 10.1113/jp275888
73. Prokopidis, K, and Witard, OC. Understanding the role of smoking and chronic excess alcohol consumption on reduced caloric intake and the development of sarcopenia. Nutr Res Rev. (2022) 35:197–206. doi: 10.1017/s0954422421000135
74. Papadopoulou, SK. Sarcopenia: a contemporary health problem among older adult populations. Nutrients. (2020) 12:1293. doi: 10.3390/nu12051293
75. Jackson, MJ, Pye, D, and Palomero, J. The production of reactive oxygen and nitrogen species by skeletal muscle. J Appl Physiol. (2007) 102:1664–70. doi: 10.1152/japplphysiol.01102.2006
76. Powers, SK, Radak, Z, and Ji, LL. Exercise-induced oxidative stress: past, present and future. J Physiology-London. (2016) 594:5081–92. doi: 10.1113/jp270646
77. Gomes, MJ, Martinez, PF, Pagan, LU, Damatto, RL, Mariano Cezar, MD, Ruiz Lima, AR, et al. Skeletal muscle aging: influence of oxidative stress and physical exercise. Oncotarget. (2017) 8:20428–40. doi: 10.18632/oncotarget.14670
78. Calvani, R, Miccheli, A, Landi, F, Bossola, M, Cesari, M, Leeuwenburgh, C, et al. Current nutritional recommendations and novel dietary strategies to manage sarcopenia. J Frailty Aging. (2013) 2:38–53. doi: 10.14283/jfa.2013.7
79. Batsis, JA, and Villareal, DT. Sarcopenic obesity in older adults: Aetiology, epidemiology and treatment strategies. Nat Rev Endocrinol. (2018) 14:513–37. doi: 10.1038/s41574-018-0062-9
80. Marzetti, E, Calvani, R, Tosato, M, Cesari, M, Di Bari, M, Cherubini, A, et al. Physical activity and exercise as countermeasures to physical frailty and sarcopenia. Aging Clin Exp Res. (2017) 29:35–42. doi: 10.1007/s40520-016-0705-4
81. Wonisch, W, Falk, A, Sundl, I, Winklhofer-Roob, BM, and Lindschinger, M. Oxidative stress increases continuously with Bmi and age with Unfavourable profiles in males. Aging Male. (2012) 15:159–65. doi: 10.3109/13685538.2012.669436
82. Kalinkovich, A, and Livshits, G. Sarcopenic obesity or obese sarcopenia: a cross talk between age-associated adipose tissue and skeletal muscle inflammation as a Main mechanism of the pathogenesis. Ageing Res Rev. (2017) 35:200–21. doi: 10.1016/j.arr.2016.09.008
83. Wannamethee, SG, and Atkins, JL. Muscle loss and obesity: the health implications of sarcopenia and Sarcopenic obesity. Proc Nutr Soc. (2015) 74:405–12. doi: 10.1017/s002966511500169x
84. Tian, S, and Xu, Y. Association of Sarcopenic Obesity with the risk of all-cause mortality: a Meta-analysis of prospective cohort studies. Geriatr Gerontol Int. (2016) 16:155–66. doi: 10.1111/ggi.12579
85. Bellanti, F, Romano, AD, Lo Buglio, A, Castriotta, V, Guglielmi, G, Greco, A, et al. Oxidative stress is increased in sarcopenia and associated with cardiovascular disease risk in Sarcopenic obesity. Maturitas. (2018) 109:6–12. doi: 10.1016/j.maturitas.2017.12.002
86. Poggiogalle, E, Migliaccio, S, Lenzi, A, and Donini, LM. Treatment of body composition changes in obese and overweight older adults: insight into the phenotype of Sarcopenic obesity. Endocrine. (2014) 47:699–716. doi: 10.1007/s12020-014-0315-x
87. Weinheimer, EM, Sands, LP, and Campbell, WW. A systematic review of the separate and combined effects of energy restriction and exercise on fat-free mass in middle-aged and older adults: implications for Sarcopenic obesity. Nutr Rev. (2010) 68:375–88. doi: 10.1111/j.1753-4887.2010.00298.x
88. Polyzos, SA, and Margioris, AN. Sarcopenic obesity. Hormones-Int J Endocrinol Metabolism. (2018) 17:321–31. doi: 10.1007/s42000-018-0049-x
89. Borras, C, Sastre, J, Garcia-Sala, D, Lloret, A, Pallardo, FV, and Vina, J. Mitochondria from females exhibit higher antioxidant gene expression and lower oxidative damage than males. Free Radic Biol Med. (2003) 34:546–52. doi: 10.1016/s0891-5849(02)01356-4
90. Bhatia, K, Elmarakby, AA, El-Remessey, A, and Sullivan, JC. Oxidative stress contributes to sex differences in angiotensin ii-mediated hypertension in spontaneously hypertensive rats. Am J Phys Regul Integr Comp Phys. (2012) 302:R274–82. doi: 10.1152/ajpregu.00546.2011
91. Hiza, HAB, Casavale, KO, Guenther, PM, and Davis, CA. Diet quality of Americans differs by age, sex, race/ethnicity, income, and education level. J Acad Nutr Diet. (2013) 113:297–306. doi: 10.1016/j.jand.2012.08.011
92. Hai, S, Wang, H, Cao, L, Liu, P, Zhou, J, Yang, Y, et al. Association between sarcopenia with lifestyle and family function among community-dwelling Chinese aged 60 years and older. BMC Geriatr. (2017) 17:187. doi: 10.1186/s12877-017-0587-0
93. Bano, G, Trevisan, C, Carraro, S, Solmi, M, Luchini, C, Stubbs, B, et al. Inflammation and sarcopenia: a systematic review and Meta-analysis. Maturitas. (2017) 96:10–5. doi: 10.1016/j.maturitas.2016.11.006
94. Bai, T, Fang, F, Li, F, Ren, Y, Hu, J, and Cao, J. Sarcopenia is associated with hypertension in older adults: a systematic review and Meta-analysis. BMC Geriatr. (2020) 20:279. doi: 10.1186/s12877-020-01672-y
95. Izzo, A, Massimino, E, Riccardi, G, and Della, PG. A narrative review on sarcopenia in type 2 diabetes mellitus: prevalence and associated factors. Nutrients. (2021) 13:183. doi: 10.3390/nu13010183
96. Livshits, G, and Kalinkovich, A. Inflammaging as a common ground for the development and maintenance of sarcopenia, obesity, cardiomyopathy and Dysbiosis. Ageing Res Rev. (2019) 56:100980. doi: 10.1016/j.arr.2019.100980
97. Lee, JH, Son, DH, and Kwon, YJ. Association between oxidative balance score and new-onset hypertension in adults: a community-based prospective cohort study. Front Nutr. (2022) 9:1066159. doi: 10.3389/fnut.2022.1066159
98. Wu, C, Ren, C, Song, Y, Gao, H, Pang, X, and Zhang, L. Gender-specific effects of oxidative balance score on the prevalence of diabetes in the us population from Nhanes. Front Endocrinol. (2023) 14:1148417. doi: 10.3389/fendo.2023.1148417
Keywords: oxidative stress, oxidative balance score, sarcopenia, NHANES, skeletal muscle
Citation: Xu W, Mu D, Wang Y, Wang Y, Wang C and Zhang X (2024) Association between oxidative balance score and sarcopenia in US adults: NHANES 2011–2018. Front. Nutr. 11:1342113. doi: 10.3389/fnut.2024.1342113
Edited by:
Roberta Zupo, University of Bari Aldo Moro, ItalyReviewed by:
Orazio Valerio Giannico, Local Health Authority of Taranto, ItalyParaskevi Detopoulou, General Hospital Korgialenio Benakio, Greece
Copyright © 2024 Xu, Mu, Wang, Wang, Wang and Zhang. This is an open-access article distributed under the terms of the Creative Commons Attribution License (CC BY). The use, distribution or reproduction in other forums is permitted, provided the original author(s) and the copyright owner(s) are credited and that the original publication in this journal is cited, in accordance with accepted academic practice. No use, distribution or reproduction is permitted which does not comply with these terms.
*Correspondence: Dongmei Mu, moudm@jlu.edu.cn