- Department of Food Science and Applied Nutrition, Addis Ababa Science and Technology University, Addis Ababa, Ethiopia
Introduction: The review titled Passion fruit by-products as a source of bioactive compounds for non-communicable disease prevention: extraction methods and mechanisms provide valuable insights into the health benefits and industrial applications of passion fruit waste. Passion fruits are a tropical and subtropical vine species, which produces edible fruits. Many food product types can be made from passion fruits. However, during passion fruit processing, large amounts of waste are released in to the environment. This review focuses on extraction methods of bioactive compounds from passion fruit by-products such as leaves, peels, seeds, and bagasse.
Methods: This comprehensive review focuses on the bioactive compounds present in passion fruit by-products, emphasis on their mechanisms of action on non-communicable diseases. It also provides a detailed analysis of the extraction methods used to obtain these bioactive compounds, their potential industrial applications, and the factors that affect extraction efficiency.
Results: This review encourages further research and innovation in utilization of passion fruit waste as a source of bioactive compounds for non- communicable disease prevention and their mechanisms of action. This can advance the circular economy. It also highlights the importance of sustainable and green extraction methods, which have gained attention due to environmental concerns.
Discussion: Unlike previous reviews, this comprehensive article explores the potential health benefits of multiple passion fruit waste products. It also examines the possible applications of these extracts for industrial goods such as food additives, colorants, nutraceuticals, natural antioxidants, and antimicrobial agents. Overall, it contributes new information emphasizing the potential of passion fruit by-products as a source of bioactive, and the findings have implications for the scientific community and industry, promoting a deeper understanding of the health benefits and sustainable practices associated with passion fruit waste utilization.
1 Introduction
Passion fruit, a tropical and subtropical vine, produces edible fruits and belongs to the Passi floraceae family (1, 2). Among more than 600 species of the passion fruit genus (Passiflora), the primary types of edible passion fruit include purple passion fruit (Passiflora edulis sims), Yellow passion fruit (Passiflora edulis f. flavicarpa Deg.), Sweet granadilla (Passiflora ligularis), and Granadilla (passiflora quadrangularis L) (3). Notably, Passiflora edulis Sims is the most significant species that produces yellow or purple fruit (3). Globally, Brazil, followed by Colombia and Indonesia, is the world's largest producer and consumer of passion fruit (4).
Passion fruits are rich in bioactive compounds such as carotenoids (b-carotene, lutein), polyphenols (gallic acid, piceatannol, neochlorogenic acid), and flavonoids (vetexi) (5). These natural phytochemicals and bioactive substances have gained attention for their antioxidant properties and potential health benefits (6). Passion fruits also exhibit anti-inflammatory properties, which can be beneficial against chronic diseases such as heart disease and diabetes (3).
Despite the popularity of passion fruit consumption, approximately 70% of the by-products produced during passion fruit juice production are discarded (7). However, extracts from waste have shown medicinal effects and a wide range of pharmacological activities against non-communicable diseases, including anti-diabetic, anti-cancer, wound repairing, anti-inflammatory, hypolipidemia, hypertensive capacities (8–14).
Passion fruit has gained global popularity due to its rich nutrient profile and flavonoids (3, 15). However, as compared to other tropical fruit groupings such as mangoes and guavas, passion fruit has received less attention. There are still gaps in understanding the mechanism of action of bioactive molecular compounds on non-communicable diseases, as well as research limitations in terms of efficacy, safety, and cost-effective and environment-friendly extraction methods.
Passion fruit wastes, including leaves, peels, bagasse, and seeds are an excellent source of bioactive compounds with potential applications in various industries, such as food, pharmaceuticals, and cosmetics. These compounds can be utilized as natural additives, flavorings, preservatives, drug formulations, and skin care products due to their different bioactive and skin benefits properties. That results in anti-inflammatory and skin-lightening effects. Therefore, further research is necessary to explore the potential of these compounds, and adopting sustainable eco-friendly extraction methods can reduce waste and minimize the harmful environmental impact associated with traditional extraction methods.
This comprehensive review focuses on the bioactive compounds present in passion fruit by-products, with emphasis on their mechanisms of action on non-communicable diseases. It also provides a detailed analysis of the extraction methods used to obtain these bioactive compounds, their potential industrial applications, and the factors that affect extraction efficiency.
2 Methodology
This review followed the methods of (16, 17) to ensure a rigorous and comprehensive approach. A relevant database, including Scopus, Google Scholar, PubMed, and Web of Science, was conducted to identify relevant papers. Specific keywords were created to develop a comprehensive search strategy. The initial search yielded a total of 356 papers. The screening process involved evaluating the relevance and quality of each paper based on the checklist. The checklist consisted of several items, including the title, abstract, introduction, methods, results, and conclusion. Each is assigned a high or low level of importance. Only papers that met the high-importance criteria were considered for inclusion. Based on this screening process, 108 papers were included in the final review, which provides a comprehensive overview of the current state of knowledge on the topic. Table 1 presents the screening criteria for inclusion of papers in a systematic review. It includes six items that need to be evaluated to determine the suitability of a paper for inclusion.
3 Passion fruit (Passiflora edulis f.) by-products
Passion fruit by-products refer to the residual materials generated during fruit processing. The processing of fruits and vegetables often leads to significant waste, which can account for 25%−30% of the total product (18). Kumar et al. (18) state that waste is generated after juice extraction in the case of citrus fruits. This accounts for approximately 50% of the fruit's wet mass (18). This finding is supported by (19). Similarly, passion fruits are estimated to produce substantial waste during juice processing. During this, over 60% of the fruit's weight is being discarded. This results in an annual waste amount of 1,050,000 million tons (19).
The main components of passion fruit waste include leaves, peels, seeds, and bagasse. The waste materials are rich in bioactive compounds such as carotenoids (β-carotene, lutein, polyphenols (gallic acid, piceatannol, neochlorogenic acid), and flavonoids (vitexin) (15). These bioactive compounds hold potential for the treatment of non-communicable diseases (20) and application in various industries. Efforts have been made to utilize these low-cost passion fruit wastes to create value-added products (21, 22). However, despite the ongoing initiatives to recycle industrial waste, a considerable number of passion fruit seeds remain underutilized (23, 24). This study supports the findings of Sudasinghe and Peiris (14) and Xie et al. (25). Moreover, passion fruit waste has been employed as a source of pectin and medicinal compounds in the production of wine and tea (13). Therefore, gaining insight into the current state of bioactive utilization from passion fruit waste is crucial. The leaf, peel, bagasse, and seeds have been identified as potential sources of valuable components derived from passion fruit.
Furthermore, the lack of consensus among different antioxidant assays and low assay specificity makes it difficult to establish a direct correlation between specific dietary antioxidants and their effects (26–28). These limitations underscore the critical importance of delving deeper into the bioactive mechanisms of actions and the effect of extraction. By addressing these challenges, a more comprehensive understanding of bioactive compounds in passion fruit by-products can be attained, ultimately contributing to the advancement of knowledge in this field.
4 Major bioactive compounds from by-products of passion fruits
The by-products of Passion fruit wastes are a rich source of bioactive compounds, making them excellent for various applications. However, it is important to acknowledge the changes in antioxidant activity and the bioavailability that occur during the consumption of these fruits (29). These changes play a crucial role in determining the pharmacological effects of passion fruit extract, including antioxidant, anti-inflammatory, antihypertensive, hypolipidemic, and antitumor activities (13, 30). To fully harness the potential bioactive compounds in passion fruit waste, it is essential to gain a comprehensive understanding of their mechanisms of action. These compounds include abundant polyphenols such as Gallic acid, and neochlorogenic acid, flavonoids like vetexi, stilbenoids (piceatannol), and carotenoids like carotene (beta-carotene) and xanthophyls (lutein). Understanding the function of these compounds is crucial for maximizing their utilization across various industries.
4.1 Polyphenols
Within passion fruit by-products, several important polyphenols can be found, including phenolic acids, flavonoids, and stilbenoids (piceatannol). These polyphenols exhibit excellent antioxidant capacity, which is beneficial for the prevention of diabetes (14, 31). After digestion, the overall phenolic content of the fruit significantly increases. The acidic and oxidized environment leads to the creation of new polyphenol molecules (32). Additionally, the digestion process releases some high-molecular-weight phenolic compounds from macromolecules (33, 34). Therefore, understanding the mechanism of action of these polyphenols enhances their utilization and promotes human health.
4.1.1 Phenolic acid
4.1.1.1 Gallic acid
Gallic acid is abundantly found in the peel of passion fruit, which is considered an agro-industrial waste (35). Additionally, Passiflora edulis, also, has been found to contain gallic acid in its fruits, specifically at a concentration of 31.20 mg/g of gallic acid equivalent. Gallic acid is one of the phenolic compounds that can inhibit lipid peroxidation and reduce lipid peroxyl radicals. They are antioxidant agents owing to their redox properties (36). Phenolic hydroxyl groups of GA can scavenge ROS and break the cycle of the generation of new radicals (37). The polyphenolic compounds gallic acid and its alkyl esters, which have antioxidative activity operate as a pro-oxidant that leads to copper-dependent DNA damage (38). When gallic acid and copper ions are applied to DNA from the plasmid pBR322 and calf thymus, strand scission and the production of 8-hydroxy-2′-deoxyguanosine occur. Catalase supplementation prevented the synthesis of 8-hydroxy-2′-deoxyguanosine, and the gallic acid-/copper-dependent strand breaks in DNA, indicating that the hydroxy radical may be involved in the DNA damage. They act as antioxidants and can inhibit the oxidation of lipids, DNA, proteins, and enzymes involved in radical generation (39).
4.1.1.2 Neochlorogenic acid
Neochlorogenic acid is found abundantly in all cultivars of passion fruit (40), as well as in vegetable wastes (41). This natural polyphenol can reduce endothelial cell aging via the Nrf2/HO-1 pathway. Chlorogenic acid, closely related to neochlorogenic acid, can modify the activity level of associated proteins and create protein–polyphenol complexes via hydrogen bonding with protein. It increases the protein antioxidant capability. Additionally, chlorogenic acid can form chelates with cadmium and limit its intestinal absorption. Furthermore, CGA has an excellent protective effect against oxidative stress. It is capable of inhibiting the formation of tyrosine, directly or indirectly promoting the expression and activation of antioxidant signal pathways, thus exerting a strong antioxidant effect.
4.1.2 Flavonoids compounds
Flavonoids are secondary metabolites synthesized mainly by plants (42). The general structure of flavonoids is a 15-carbon skeleton, containing two benzene rings connected by a 3-carbon linking chain. Therefore, they are depicted as C6-C3-C6 compounds. Flavonoid compounds include quercetin, apigenin, luteolin, and myricetin. Plants frequently contain polyphenolics called flavonoids, which have a variety of biological effects both in vitro and in vivo (43). Researchers have discovered that they possess antibacterial properties (44), antiviral, antiulcerogenic, cytotoxic, antineoplastic, mutagenic, antioxidant (43), antihepatotoxic, antihypertensive, hypolipidemic, antiplatelet, and anti-inflammatory properties (45). The flavonoid vitexin is an extract of Passiflora species—a promising anti-inflammatory and antioxidant substance in vitro and vivo studies (46).
4.1.2.1 Vitexin
Vitexin is an apigenin flavone glucoside—a compound found in the passion flower (47). After digestion, the total phenolic content and total flavonoid compounds increase, especially the vitexin and Gallic acid (48). Diabetes mellitus (DM) is a complex disorder that affects overall health and is characterized by elevated blood sugar levels (8, 9). The α-glucosidase enzyme located in the small intestine plays a role in the conversion of carbohydrates into glucose. Research conducted by (49) has shown that Passion fruit extracts have the potential to act as anti-diabetic agents. This finding aligns with the work of (50), who also reported the long-term benefits of fruit consumption for individuals with diabetes.
4.1.3 Stilbenoid compound
4.1.3.1 Piceatannol
Passion fruit seeds are reported to contain high levels of piceatannol, which is a type of stilbene (51). This compound has positive benefits including estrogenic, anti-cancer, anti-atherogenic, antioxidative, anti-inflammatory, and antimicrobial properties (52). This study aligns with the work of Piotrowska et al. (53). The stilbene compound with the formula ((HO)2 C6H3)2CH2 is made up of two phenolic rings connected by a styrene double bond (54). The double bond helps both the trans- and cis-isomeric forms (E) - and (Z) – diastereomers, respectively, to form the trans-isomers that are statically the more stable forms.
4.2 Carotenoids
The passion fruit pulp is reported to have lower levels of carotene compared to the seeds and peels, which have higher total phenolic content (55). Carotenoids in the diet are thought to have health benefits by lowering the risk of disease, particularly some malignancies and eye problems (56). Preclinical research has indicated that certain carotenoids exhibit powerful anti-cancer effects in vitro and in vivo (57). This aligns with the work of (58). The most researched carotenoids include β-carotene and lutein. These carotenoids are well studied and known for their positive effects, primarily attributed to their antioxidant activity and ability to be converted into vitamin A (57, 58). Furthermore, lutein may protect against eye disease by absorbing harmful blue light that enters the eye.
4.2.1 β-carotene
One of the carotenoids is β-carotene (59). The oxygen addition to β-carotene radical intermediates is assumed to be the mechanism of chain cleavage. Carotenoid β-carotene and other carotenoids are hypothesized to have disease-preventive properties by scavenging reactive free radicals (60). A substantial body of epidemiological research linked high β-carotene intake to a lower risk of cancer and cardiovascular disease. Rhodopsin is a G-protein-coupled receptor (GPCR) that is encoded by the RHO gene (61). It is a light-sensitive receptor protein, and the opsin of rod cells in the retina causes visual phototransduction in rods. Rhodopsin is particularly important because it mediates low-light vision. Rhodopsin is a complex protein composed of opsin and 11cis-retinal. Photo isomerization occurs when it absorbs light (62). During this isomerization process, rhodopsin can engage with transducer, which is in guanine nucleotide-binding proteins (Gp), and it can block Ca2+ from entering the retinal rod cells by engaging with rhodopsin, inhibiting exocytosis neurotransmitters via a more convoluted pathway. Non-provitamin—a carotenoid, which includes lycopenexanthrophyls (lutein) and carotenes (β-carotene), are other types of dietary pigments that are not transformed into vitamin A but may still have significant functions (63).
4.2.2 Lutein
Lutein is one of the carotenoid compounds commonly found in passion fruits. Carotenoids are phytochemicals that are classified as carotenes if they are exclusively hydrocarbons, but they are known as xanthophylls if they contain oxygen as a result of oxidation or enzymatic addition (64). This core system of conjugated carbon–carbon double bonds makes efficient quenchers of reactive oxygen species (ROS) and absorbers of potentially damaging visible light (65). Also, their functions are determined by their physical and chemical properties, functional groups, geometry, and varied structures. Traditionally, lutein is characterized by its blue light (66); this agrees with the research (67).
5 Bioactive compounds found in passion fruit and their biological activities
According to studies passion fruit waste contains bioactive compounds, such as flavonoids, phenolic compounds, and carotenoids, which have been associated with potential health benefits in preventing chronic non-communicable diseases, such as cancer and heart diseases (68–70). These bioactive compounds are found in passion fruit waste, including luteolin, chlorogenic acid, beta-carotene, lycopene, and neochlorogenic acid. These bioactive compounds have been recognized for their potential health-promoting properties (5).
Flavonoids are a type of bioactive compound found in passion fruit waste, which has been shown to possess antioxidant and anti-inflammatory properties. This may contribute to their potential protective effects against cancer and cardiovascular diseases (71). Phenolic compounds such as chlorogenic acid and neochlorogenic acid, which are found in passion fruit waste, have also demonstrated antioxidant and anti-inflammatory actions. This indicates their potential to reduce the risk of chronic diseases (72). Carotenoids, including beta carotene and lycopene, present in passion fruit waste, have been associated with a lower risk of certain types of cancer and heart diseases (73). However, further research is needed to fully understand the mechanism of action and determine the optimal dosage of these bioactive compounds for disease prevention.
5.1 Diabetic wound healing
Passion fruit waste contains gallic acid, quercetin, and β-carotene; these are used as anti-diabetic therapeutic (50). This is similar to extract from the peel of pineapple, which has α-glucosidase inhibitory activities. This is effective as an anti-diabetic therapeutic tool (74); this investigation is also supported by (75). Uncontrolled type 2 diabetes can cause severe impairment to organs such as the heart, blood vessels, eyes, kidneys, and nerves, resulting in complications such as cardiovascular disease (76). The anti-diabetic effect of passion fruits is associated with their high content of soluble fiber, as well as several polyphenols and carotenoids, like ellagic acid, epicatechin gallate, and β-carotene. Its leaves and stems improved liver function and oxidative stress parameters in alloxan-induced diabetic mice (50), which agreed with the work of Kandandapani et al. (49) and Chakraborty et al. (8).
Non-healing wounds, which are characterized by a lack of epithelial cell migration (77) and endothelial dysfunction, are frequently present in diabetes (78). Treating diabetes in vivo investigation P. suberosa extract from the leaves can manage blood sugar and associated cholesterol levels (14). The peel of Passiflora edulis is a good source of fiber and bioactive compounds. In young rats, supplementation prevents insulin resistance and hepatic steatosis caused by a low-fructose diet (79).
5.2 Mechanisms of anti-cancer cell
Cancer is a complex disease that arises from genetic mutations that disrupt the normal control mechanisms of cell growth and division. These mutations can be inherited or occur spontaneously during an individual's lifetime. Cancer cells originate from a single abnormal cell that undergoes uncontrolled replication due to genetic alterations. Prostate cancer (PCa), the second most common type of cancer in men, is influenced by factors such as androgens and inflammation, which contribute to its progression (80). Cancer cells exhibit aberrant behavior, including uncontrolled growth, invasion into surrounding tissue, and the ability to metastasize (37). These cells can combine to form a mass of tissue known as a tumor, which can be cancerous (malignant). The bioactive compounds including polyphenols have numerous health benefits in preventing the progression of cancer (37). This study is supported by the work of Baseggio et al. (81). According to Silva et al. (82), passion fruit leaf extract has an antioxidant activity that has the potential to be photoprotective. Xu et al. (22) state that bioactive compounds from passion fruit enhance nerve growth factor-induced neurite outgrowth and are used for antiaging and cosmetic formulation and to relieve stress and anxiety. On the other hand, the investigation of Ramirez et al. (83) indicates that these extracts are promising for cancer treatment. Therefore, understanding the mechanisms underlying the anti-cancer activity is crucial for the development of effective treatments.
5.3 Anti-inflammatory effects
Inflammation is a complex biological response that occurs when tissues are exposed to harmful stimuli such as infections, damaged cells, or irritants. It involves immune cells, blood vessels, and molecular mediators. Too little inflammation can lead to tissue destruction and compromise the survival of the organism. According to various studies (8, 84, 85), passion fruit waste extracts containing gallic acid have demonstrated anti-inflammatory effects by reducing levels of specific cytokines that promote inflammation. Those effects have the potential to alleviate conditions such as obesity and lipid peroxidation. Cytokines are signaling molecules that regulate immune responses and passion fruit waste extract may help modulate inflammation processes by targeting these cytokines. These extracts can also prevent DNA mutation induced by oxidative stress by acting as antioxidants. Furthermore, stress hormones such as glucocorticoids and catecholamines can stimulate the production of anti-inflammatory cytokines and enhance immune responses through the induction of TNF-alpha (86). Therefore, the consumption of passion fruits can help balance the levels of pro-inflammatory versus anti-inflammatory cytokines.
5.4 Antioxidant properties of passion fruit peel waste
Antioxidants are important species that can deactivate reactive oxygen species (ROS) by giving them their electrons, protecting essential molecules such as proteins, lipids, and DNA from damage due to oxidative stress (76). Passion fruit is known for its high phenolic content, which serves as the primary contributor to the antioxidant potential and associated health benefits of peel waste (9). These antioxidants work through enzymatic or non-enzymatic processes that occur inside organelles or within cells (87). They are essential for improving the by-products of physiological metabolism and can aid in preventing infection at wound sites (88). In the context of chronic wounds in humans, which often exhibit high concentrations of oxidative stress, the administration of antioxidants and antibacterial agents with treatment can help mitigate this stress. Passion fruit peel waste with its high phenolic content holds significant potential as a source of antioxidants and contributes to its health-promoting effects. Therefore, understanding the antioxidant properties of passion fruit peel waste is crucial for exploring and discovering its potential applications in wound healing and oxidative stress management.
6 Action mechanisms of bioactive compounds
Passion fruit contains various bioactive compounds, such as flavonoids, phenolic acids, and carotenoids, which have been shown to possess potent antioxidant, anti-diabetic, and anti-cancer properties. These compounds can activate the Keap1–Nrf2 pathway, leading to the transcription of a range of antioxidant and cytoprotective genes. The research conducted by de Melo Pereira et al. (89) provides an overview of the nutritional composition of passion fruit and discusses its potential health benefits, particularly its antioxidant effects. Whereas Adedayo et al. (90) conducted a study specifically on passion fruit peel extract, investigating its antioxidant and anti-inflammatory activities and exploring the potential mechanisms of action. Singh et al. (91) present a review article discussing the modulation of the Keap1–Nrf2 signaling pathway by bioactive phytochemicals, which could be relevant for understanding how passion fruit bioactive compounds trigger antioxidant effects. Furthermore, the review article by Serafini et al. (92) provides a comprehensive overview of dietary antioxidants and their role in preventing non-communicable diseases, including cancer, with a discussion on how antioxidants in fruits like passion fruit exert their effects. Overall, understanding the involvement of the Keap1–Nrf2 pathway in the antioxidant effects of passion fruit compounds contributes to the knowledge of their potential health benefits and highlights their importance in maintaining cellular redox balance.
Under normal conditions, the transcription factor Nrf2 (Nuclear factor erythroid 2-related factor 2) is sequestered in the cytoplasm by the Kelch-like ECH-associated protein 1 (Keap1). Keap1 acts as a negative regulator of Nrf2 by promoting its degradation. However, upon exposure to oxidative stress or the presence of certain bioactive compounds, Keap1–Nrf2 interactions are disrupted, leading to the stabilization and nuclear translocation of Nrf2. Once in the nucleus, Nrf2 forms a heterodimer with a small Maf protein and binds to antioxidant response elements (ARE) in the promoter regions of target genes. This binding activates the transcription of a wide array of genes involved in antioxidant defense, detoxification enzymes, and cellular stress response. For that matter, activation of the Keap1–Nrf2 pathway results in the upregulation of several key antioxidant enzymes, such as heme oxygenase-1 (HO-1), NAD(P)H:quinone oxidoreductase 1 (NQO1), glutathione peroxidase (GPx), and superoxide dismutase (SOD). These enzymes play crucial roles in scavenging reactive oxygen species (ROS) and preventing oxidative damage within cells. Finally, the induction of antioxidant enzymes through the Keap1–Nrf2 pathway enhances the cellular defense mechanisms against oxidative stress. By neutralizing ROS and reducing oxidative damage, passion fruit compounds mediated through the Keap1–Nrf2 pathway can protect cells from various pathological conditions associated with oxidative stress, such as inflammation, neurodegenerative diseases, and cardiovascular disorders. So understanding the involvement of the Keap1–Nrf2 pathway in the antioxidant effects of passion fruit compounds contributes to the knowledge of their potential health benefits and highlights their importance in maintaining cellular redox balance.
7 Bioactive mechanism of action in vivo and in vitro assay
In vitro and in vivo investigation, P. edulis leaf extract is a viable choice to increase the antioxidant supply and protect against oxidative stress (93); it can be used to manage blood glucose (14) and reduce cholesterol levels (85). Also, it has the maximum bio-accessibility of phenolic compounds and mono/oligosaccharides (94). The highest link with improved antioxidant capacity is seen with chlorogenic acid, quercetin, and xylose (95), although these compounds are often discarded as waste in different passion fruit juice processing industries. The bagasse of passion fruit makes an interesting source for the extraction of unsaturated fatty acids and carotenoids (96). On the other hand, passion fruit waste has a high content of phenolic acids (97). Among these, it mostly contains anthocyanin, flavonoids, and carotenoids (97).
Table 2 presents a summary of in vivo and in vitro tests conducted on various extracts obtained from passion fruit by-products. The table highlights the potential of passion fruit by-products as a source of bioactive compounds with various biological activities and potential applications in different fields. It also provides a useful summary of the various compounds that have been isolated and tested, along with their potential applications. This makes it easier for researchers and industries to identify suitable bioactive compounds for their specific needs. Additionally, the table lists the potential applications of the extract in different fields, including cosmetics, complementary therapy for cancer patients, wound repair, and prostate cancer progression.
The extracts of passion fruit waste have effects on different non-communicable diseases such as being potent to treat diabetes wound repair, gastrointestinal digestion improvement, prostate cancer therapy, and being used for cosmetic formulations. There is also the possibility of studying the healing efficacy of fruit peel flour fortification with different food types in vitro study (21). Bioactive compounds from the waste of passion fruits have an impact on in vitro gastrointestinal digestion, bioaccessibility, and antioxidant capacity of leaves and bagasse (26), which agrees with the work (46).
Leaf extracts from the Passiflora genus exhibit promising biological activity for treatment of cancer (83). They also enhance nerve growth factor-induced neurite outgrowth in protein Cell 12 cells (22). Methanolic acid and glycolic acid are used for anti-aging and cosmetic formulation (82). Additionally. C-di-deoxyhexosyl flavones from leaves are used to relieve stress (22). In vitro tests of the bioactive compounds and flavonoids from fruit leaf extract can improve antioxidant capacity and bioaccessibility (26). Peels are common wastes that are often thrown away (99). However, they are it is a source of natural antioxidants with potential industrial and culinary applications (21).
The passion fruit seeds contain the essential compound piceatannol, which can enhance the physicochemical properties and functionality of the product (54). Likewise, passion fruit seeds have essential oil (100), which is used for various purposes, particularly for diabetic and antiglycation treatment (52). The seeds are also known to contain a variety of polyphenols, particularly high levels of piceatannol (54). Numerous studies have demonstrated the physiological effects of passion fruit seed extracts and their compounds, including hypoglycaemic effects (54, 101). Generally, those co-products are notable for their strong antioxidant activity, attributed to their polyphenol and carotenoid content (85).
As Table 3 states, the study by FP da Araújo et al. (45) reveals that passion fruit peel extracts exhibit anti-cancer activity on colorectal cancer cells in vitro, reducing cell viability, inducing apoptosis, and arresting cell cycle progression. The research by da Silva et al. (102) demonstrates that passion fruit peel extract has anti-diabetic effects in rats, lowering blood glucose levels, increasing insulin secretion, and reducing oxidative stress markers. Similarly, Ojezele et al. (106) show that P. edulis, including the peel, exhibits anti-diabetic activity in a rat model, reducing blood glucose levels, improving insulin sensitivity, and suggesting its potential as a therapeutic agent for managing diabetes. The review article by de Melo Pereira et al. (107), highlights that passion fruit peel contains bioactive compounds with potential anti-cancer and anti-diabetic effects, providing an overview of these compounds, their potential health benefits, and future research prospects. These findings underscore the importance of further exploring passion fruit peel as a source of valuable bioactive compounds for potential therapeutic applications in cancer and diabetes management.
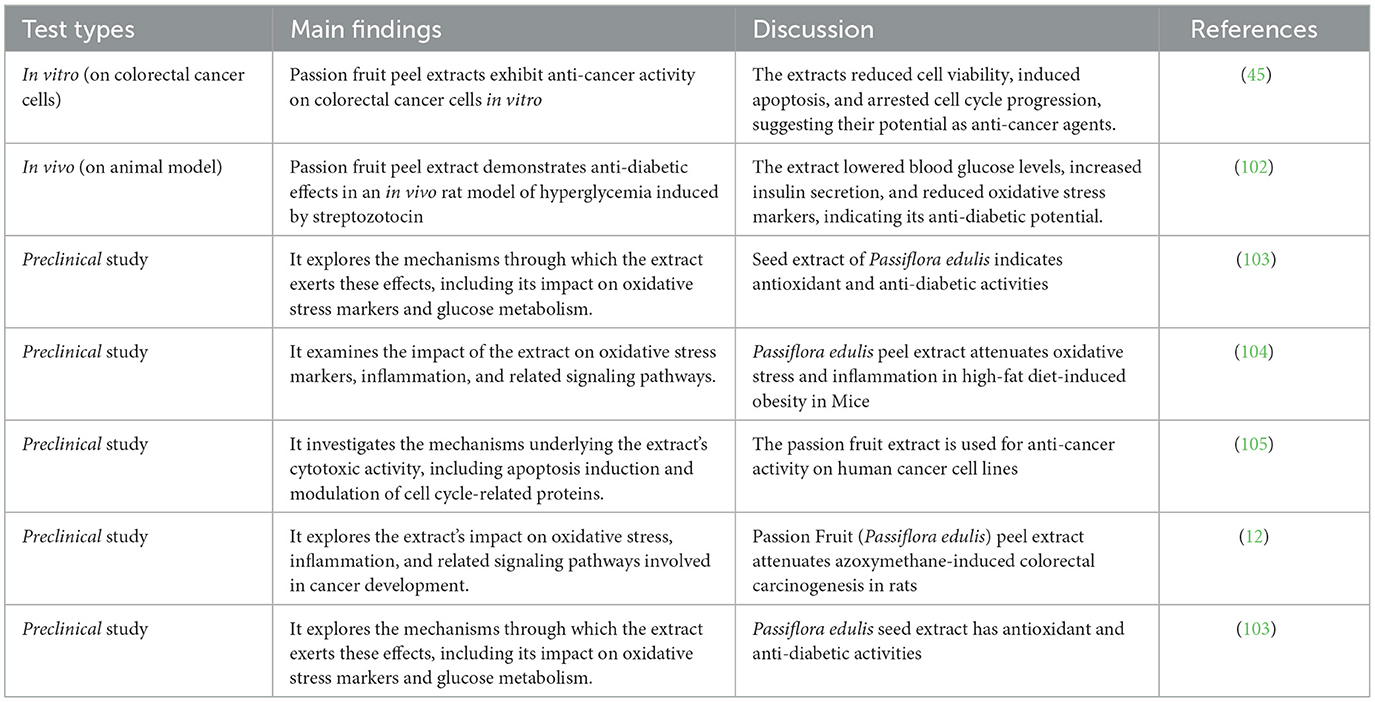
Table 3. Passion fruit peel extracts and its health benefits in vivo, in vitro and preclinical studies.
Table 3 also summarizes four preclinical studies on passion fruit extracts. The study by Ribeiro et al. (103) investigates the antioxidant and anti-diabetic activities of passion fruit seed extract, exploring its impact on oxidative stress markers and glucose metabolism (104). This study focuses on the antioxidant effects of passion fruit peel extract in a mouse model of high-fat diet-induced obesity, examining its influence on oxidative stress markers, inflammation, and related signaling pathways. Nascimento et al. (105) explore the anti-cancer effects of passion fruit extract on human cancer cell lines, investigating mechanisms such as apoptosis induction and modulation of cell cycle-related proteins (12). They also study the potential chemopreventive effects of passion fruit peel extract on colorectal carcinogenesis in rats, analyzing its impact on oxidative stress, inflammation, and related signaling pathways. Overall, these studies contribute to understanding the potential health benefits and mechanisms of action of passion fruit extracts.
8 Effect of technologies on bioactive extractions
The extraction of bioactive compounds from the by-product of passion fruit can be significantly influenced by various technologies. Advanced extraction techniques, such as ultrasound or microwave-assisted extraction, have demonstrated effectiveness in enhancing the recovery of bioactive compounds from food waste and plant sources (18). These technologies utilize physical effects such as cavitation thermal energy to facilitate the release and extraction of bioactive compounds, resulting in improved extraction efficiency and higher yields. Furthermore, the impact of drying technologies on the extraction and recovery of bioactive compounds is an essential consideration, particularly when used as a pretreatment before extraction (108). Proper drying techniques such as free-drying or hot air drying can help preserve the bioactive compounds in the passion fruit by-products and enhance their subsequent extraction (108). Drying can reduce the moisture content, stabilize the bioactive compound, and facilitate their release during extraction leading to increased extraction efficiency and improved overall yield.
It is worth noting that the combination of different technologies can have synergistic effects on the recovery of bioactive compounds. For instance, pretreatment with drying techniques followed by advanced extraction methods can result in even higher extraction yields and enhanced extraction efficiency. The sequential application of technologies can optimize the release and extraction of bioactive compounds, enabling their potential utilization in the food and pharmaceutical industries. To provide a more comprehensive understanding of strategies for different extraction technologies for passion fruit by-products. Factors such as temperature, extraction time, solvent selection, and sample-to-solvent ratio can significantly influence the extraction efficiency and quality of bioactive compounds. Additionally, comprehensive studies analyzing the effectiveness and cost-effectiveness of different extraction technologies would be valuable in guiding industrial-scale extraction processes.
Table 4 summarizes the different technologies utilized for the extraction of bioactive compounds from various parts of the passion fruit, along with the identified compounds and corresponding references. Silva et al. (10), studied the impact of freeze-drying on passion fruit residues and identified the presence of anthocyanins, flavonols, stilbenes, phenolic acids, flavonoids, and procyanidins. Viganó (110) investigated the use of pressurized liquids assisted by ultrasound on defatted passion fruit bagasse and identified the presence of polyphenols. It focused on agro-industrial waste and utilized high-pressure technologies to extract antioxidants, and it can be possible to extract piceatannol and sirtuin B from bagasse using sequential high-pressure extractions (110, 117).
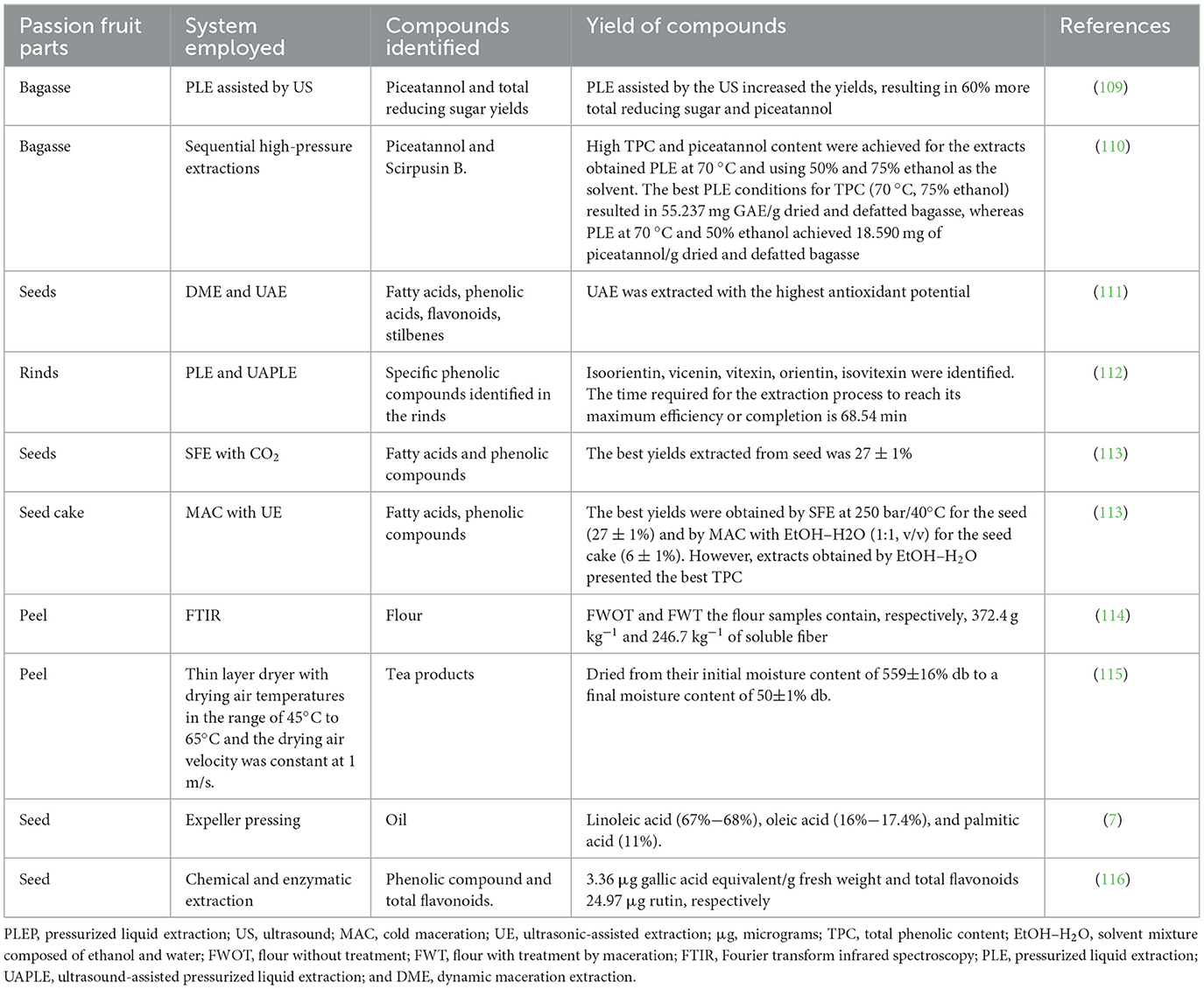
Table 4. Effect of extraction technologies employed for bioactive extraction from passion fruit by-products.
Another study by Viganó et al. (110) states that using supercritical fluid extraction assisted by CO2 uses to extract nonpolar fractions from bagasse extracts. Moreover, Vafaei et al. (118) utilized supercritical fluid extraction for extracting tocopherols and carotenoids. Pedro et al. (119) investigated the effect of the drying method on the adsorption isotherms and isosteric heat of pulp, while Ribeiro et al. (120) utilized pressurized ethanol and ultrasound for extracting oil from passion fruit pulp. Coelho et al. (114) demonstrated the process of converting passion fruit peel into flour. Sittipa et al. (115) studied the drying kinetics of passion fruit peel for preparing tea products. Yusuf (116) employed chemical and enzymatic extraction to obtain bioactive components from passion fruit seeds. The use of various technologies has effectively extracted bioactive compounds from different parts of the passion fruit, offering potential applications in functional food and nutraceutical industries.
9 Industrial application of passion fruits co-product bioactive compounds
9.1 Utilization of bioactive compounds for food preservation
The utilization of bioactive compounds for food preservation has been demonstrated in several studies. Powder extracts derived from passion fruit peel (P. edulis) have shown antibacterial and antioxidant qualities that make them beneficial for preserving meat products and enhancing their aroma. Specifically, studies by Ramli et al. (44) indicate that passion fruit peel extract can be applied for antibacterial and antioxidant activity on preserved meat products (6). These findings suggest the potential of bioactive compounds found in passion fruit to play a role in the development of safe, efficient, and natural methods for food preservation.
Table 5 presents the utilization of bioactive compounds derived from passion fruit co-products in various food applications. The table highlights different co-products, their specific utilization, extraction methods, and corresponding references. For instance, peel extract demonstrates antibacterial properties and aroma enhancement, when it is extracted through solvent extraction (44). While another peel extract is utilized for aroma enhancement using enzyme-assisted extraction (6). The peel itself finds application in antimicrobial bio-based films, nanoparticles, healing agents, and edible coatings through acid extraction (121). Both peel and pulp are used as food-flavoring ingredients in desserts, employing emerging technologies for extraction (122). Lastly, the seed is utilized for edible oil production, although the extraction method is not specified (123). Overall, the table provides a comprehensive overview of the diverse applications of passion fruit co-products in the food industry, demonstrating the versatility and potential uses of their bioactive compounds.
9.2 Utilization of extracts in cosmetics applications
The utilization of extracts in cosmetics applications has been investigated in various studies, with a focus on their antioxidant and photoprotective properties. Research by (82) suggests that Passiflora leaf extracts exhibit antioxidant activity and have the potential photoprotective application in cosmetics. Additionally, Chen et al. (9) highlight the variation in antioxidant and potential of phenolic compounds present in these extracts. Furthermore, studies by Wolde et al. (73) and Huang et al. (47) underscore the importance of reactive molecular species and antioxidant mechanisms in processes such as aging, carcinogenesis, and the normal aging of the skin. These findings highlight the potential of extracts, particularly, those derived from leaves, for the development of antioxidant and photo-protective cosmetic products.
Table 6 provides valuable insights into the utilization of passion fruit extracts in various cosmetics applications. The table presents different parts of the passion fruit, the specific cosmetic products derived from them, the yields obtained, and the corresponding references. For instance, seed extract demonstrates its potential in sun-protective makeup products, with SPF values of 18.09 ± 1.48 and 18.60 ± 1.21 for concealers containing 0.1% and 0.3% passion fruit extract, respectively (94). Passion fruit extracts are also known for their antibacterial properties, although the specific part used is not specified (44). Moreover, shell wastes of passion fruit contain phytoprostanes (PhytoPs) and oxylipins with multiple biological activities, including cinnamoyl acid derivatives and flavonoid compounds (125). Peel extract is utilized in anti-aging cosmetics due to its polyphenol compounds and flavonoids, which act as natural antioxidants to prevent premature aging (126). On the other hand passion fruit seeds finds application in antioxidative extracts for skin protection from aging, containing compounds such as quercetin, chlorogenic acid, gallic acid, kojic acid, ferulic acid, and caffeic acid (127). Seeds are also employed as skin anti-aging agents (28). Through surfactant-assisted aqueous extraction, passion fruit seed yields bioactive components, including phenols, squalene, β-sitosterol, and vitamin E, as well as significant free fatty acids such as linoleic, oleic, and palmitic acids (124). Overall, Table 6 showcases the diverse cosmetic applications of passion fruit extracts, highlighting their potential in sun protection, antibacterial properties, anti-aging cosmetics, and skin rejuvenation.
9.3 Utilization of pectin as a food ingredient
Pectin has extensive use in the food industry for various applications. It serves as a gelling agent in jams, jellies, and preserves—a jelly-like consistency. Additionally, pectin is employed as a thickener, stabilizer, and emulsifier in low-calorie foods such as juices, bakery goods, and dairy products. Furthermore, pectin has shown potential in other applications, including the creation of antimicrobial bio-based films, nanoparticles, healing agents, and an edible coating to protect food items, as demonstrated in a study conducted by Freitas et al. (121). Overall, pectin is a versatile ingredient utilized in the food industry.
9.3.1 Extraction of pectin from passion fruits by-products
Pectin extracted from passion fruit peel exhibits higher extraction efficiency (15.71%) compared to pectin extracted from soy husk (5.66%). However, it is lower than the result reported by dos Santos et al. (128), which indicates a pectin content of 37.37 g/100 g in passion fruit peels. Furthermore, passion fruit peel pectin demonstrates a higher galacturonic acid concentration (23.21%) compared to the content of orange pomace pectin (16.01%) (129). The composition, structural characterization, and emulsion stability of pectin can vary, resulting in these differences. Various extraction conditions such as the types of plant parts used, processing equipment or techniques employed, extraction time, and temperatures can have distinct effects on the quality characteristics of pectin extracted from passion fruit peel (P. edulis f. flavicarpa L.).
Table 7 presents the results of various studies that have examined the yields of pectin from different parts of plants. Enzymatically extracted passion fruit pectin, similar to commercial citrus pectin, has been reported to have a galacturonic acid content of 85 g/100 g and a degree of esterification of 68% (132), with supporting evidence from Nguyen et al. (94). However, the yield of pectin from sweet lemon peel (133) is even higher, accounting for the highest pectin yield at 25.31%.
However, extraction conditions have an effect on the quality and quantity characteristics of pectin from passion fruit peel (28). The extraction of pectin from passion fruit peel has been found to affect its composition, structural characterization, and emulsion stability (134). The content and physicochemical properties of pectin can vary across different plant species, depending on the source and degree of fruit maturity. In particular, passion fruit waste exhibits high amounts of pectin primarily in the peel, followed by the leaf and pulp. The structural, physicochemical, and rheological characteristics of pectin polysaccharides from fresh passion fruit peel are important for potential use in the food industry. Promising yields of total sugar and uronic acid suggest the potential for using plant-derived pectin as a valuable ingredient in the food industry. Overall, these findings highlight the value of exploring various plant sources for pectin extraction and utilization.
10 Conclusion and recommendation
The article concludes that passion fruit waste has potential health benefits and industrial applications due to its high content of bioactive compounds such as flavonoids, carotenoids, and polyphenols. Sustainable extraction methods can help minimize waste and reduce the negative environmental impact of the extraction process. While also meeting the increasing demand for ecofriendly and sustainable products in various industries. Extraction techniques such as enzymatic, microwave-assisted, ultrasound-assisted, and supercritical fluid extractions have shown promise in efficiently extracting bioactive compounds from passion fruit co-products. However, there is limited research on the mechanism of action and acceptable dosage of these compounds in human health, and further research is needed in this area. As a recommendation researching the pharmacokinetics (absorption, distribution, metabolism, and excretion) of passion fruit peel extracts in animal models and humans would be important for determining optimal dosage regimens. Understanding how the body processes these extracts can guide dosage recommendations for potential clinical applications. Additionally, conducting comprehensive long-term safety studies and assessing the potential toxicity of passion fruit waste extracts is essential before considering their widespread use as therapeutic agents. These studies could evaluate potential adverse effects, drug interactions, and any limitations or contraindications for specific populations. Overall, exploring the bioactive by-products of passion fruit has the potential to promote human health and reduce waste emissions.
Data availability statement
The original contributions presented in the study are included in the article/supplementary material, further inquiries can be directed to the corresponding authors.
Author contributions
GW: Conceptualization, Investigation, Methodology, Writing – original draft, Writing – review & editing. AB: Conceptualization, Supervision, Writing – review & editing. ET: Supervision, Validation, Visualization, Writing – review & editing.
Funding
The author(s) declare that no financial support was received for the research, authorship, and/or publication of this article.
Acknowledgments
The authors like to express our sincere appreciation to Addis Ababa Science and Technology University for providing the necessary facilities, such as an office and internet service, which enabled to conduct this research.
Conflict of interest
The authors declare that the research was conducted in the absence of any commercial or financial relationships that could be construed as a potential conflict of interest.
Publisher's note
All claims expressed in this article are solely those of the authors and do not necessarily represent those of their affiliated organizations, or those of the publisher, the editors and the reviewers. Any product that may be evaluated in this article, or claim that may be made by its manufacturer, is not guaranteed or endorsed by the publisher.
References
1. Anderson JD, Vidal RF, Brym M, Stafne ET, Resende Jr MF, Viana AP, et al. Genotyping-by-sequencing of passion fruit (Passiflora spp.) generates genomic resources for breeding and systematics. Genetic Res. Crop Evol. (2022) 69:2769–2786. doi: 10.1007/s10722-022-01397-4
2. Deshmukh N, Patel R, Okram S, Rymbai H, Roy S, Jha A. Passion fruit (Passiflora spp.). Magnesium. (2017) 100:200.
3. Duarte AE, Milenkovic D, Borges TK, de Oliveira L, Costa AM. Brazilian passion fruit as a new healthy food: from its composition to health properties and mechanisms of action. Food Funct. (2021) 12:11106–11120. doi: 10.1039/D1FO01976G
4. Gioppato HA, da Silva MB, Carrara S, Palermo BRZ, de Souza Moraes T, Dornelas MC. Genomic transcriptomic approaches to understand Passiflora physiology and to contribute to passionfruit breeding. Theoret Exp Plant Physiol. (2019) 31:173–81. doi: 10.1007/s40626-018-0134-1
5. Santos JL, Matsumoto SN, Oliveira PND, Oliveira LSD, Silva R. Morphophysiological analysis of passion fruit plants from different propagation methods and planting spacing. Revista Caatinga. (2016) 29:305–312. doi: 10.1590/1983-21252016v29n206rc
6. Ma D, Dong S, Zhang S, Wei X, Xie Q, Ding Q, et al. Chromosome-level reference genome assembly provides insights into aroma biosynthesis in passion fruit (Passiflora edulis). Mol Ecol Resour. (2021) 21:955–68. doi: 10.1111/1755-0998.13310
7. Antoniassi R, Wilhelm AE, Reis SLR, Regis SA, Faria-Machado AF, Bizzo HR, et al. Expeller pressing of passion fruit seed oil: Pressing efficiency and quality of oil. Braz J Food Technol. (2022) 25:16821. doi: 10.1590/1981-6723.16821
8. Chakraborty S, Bhattacharyya R, Banerjee D. Infections: a possible risk factor for type 2 diabetes. Adv Clin Chem. (2017) 80:227–51. doi: 10.1016/bs.acc.2016.11.004
9. Chen Z, Zhong B, Barrow CJ, Dunshea FR, Suleria HA. Identification of phenolic compounds in Australian grown dragon fruits by LC-ESI-QTOF-MS/MS and determination of their antioxidant potential. Arab J Chem. (2021) 14:103151. doi: 10.1016/j.arabjc.2021.103151
10. Silva NC, Santana RC, Duarte CR, Barrozo MA. Impact of freeze-drying on bioactive compounds of yellow passion fruit residues. J Food Process Eng. (2017) 40:e12514. doi: 10.1111/jfpe.12514
11. Gan Y-H. Host susceptibility factors to bacterial infections in type 2 diabetes. PLoS Pathog. (2013) 9:e1003794. doi: 10.1371/journal.ppat.1003794
12. Pereira DTV, Zabot GL, Reyes FGR, Iglesias AH, Martínez J. Integration of pressurized liquids and ultrasound in the extraction of bioactive compounds from passion fruit rinds: Impact on phenolic yield, extraction kinetics and technical-economic evaluation. Innov Food Sci Emerg Technol. (2021) 67:102549. doi: 10.1016/j.ifset.2020.102549
13. He X, Luan F, Yang Y, Wang Z, Zhao Z, Fang J, et al. Passiflora edulis: an insight into current researches on phytochemistry and pharmacology. Front Pharmacol. (2020) 11:617. doi: 10.3389/fphar.2020.00617
14. Sudasinghe H, Peiris D. Hypoglycemic hypolipidemic activity of aqueous leaf extract of Passiflora suberosa L. PeerJ. (2018) 6:e4389. doi: 10.7717/peerj.4389
15. Viera W, Shinohara T, Samaniego I, Sanada A, Terada N, Ron L, et al. Phytochemical composition and antioxidant activity of Passiflora spp. germplasm grown in ecuador. Plants. (2022) 11:328. doi: 10.3390/plants11030328
16. May CR, Cummings A, Girling M, Bracher M, Mair FS, May CM, et al. Using normalization process theory in feasibility studies and process evaluations of complex healthcare interventions: a systematic review. Implement Sci. (2018) 13:1–27. doi: 10.1186/s13012-018-0758-1
17. Pautasso M. Ten simple rules for writing a literature review. PLoS Comput Biol. (2013) 9:e1003149. doi: 10.1371/journal.pcbi.1003149
18. Kumar H, Bhardwaj K, Sharma R, Nepovimova E, Kuča K, Dhanjal DS, et al. Fruit and vegetable peels: utilization of high value horticultural waste in novel industrial applications. Molecules. (2020) 25:2812. doi: 10.3390/molecules25122812
19. Khuwijitjaru P, Klinchongkon K. Passion fruit. In: Valorization of Fruit Processing by-Products. London: Elsevier (2020). p. 183–201.
20. Liu M, Jiang Y, Wedow R, Li Y, Brazel DM, Chen F, et al. Association studies of up to 1.2 million individuals yield new insights into the genetic etiology of tobacco and alcohol use. Nat. Genet. (2019) 51:237–244. doi: 10.1038/s41588-018-0307-5
21. Ning X, Wu J, Luo Z, Chen Y, Mo Z, Luo R, et al. Cookies fortified with purple passion fruit epicarp flour: Impact on physical properties, nutrition, ” starch digestibility, antioxidant activity. Cereal Chem. (2021) 98:328–36. doi: 10.1002/cche.10367
22. Xu F, Wang C, Yang L, Luo H, Fan W, Zi C, et al. C-dideoxyhexosyl flavones from the stems and leaves of Passiflora edulis sims. Food Chem. (2013) 136:94–9. doi: 10.1016/j.foodchem.2012.07.101
23. Lourith N, Kanlayavattanakul M. Passion fruit seed: Its antioxidative extracts and potency in protection of skin aging. In: Aging. London: Elsevier (2020). p. 283–288.
24. Oliveira CCR, Feitosa BR, Souza VB, Tulini FL, Martins MV. Physicochemical sensory analyses of sequilhos produced with non-conventional food plants: arrowroot, licuri and wild passion fruit shell. Food Sci Technol. (2022) 42:721. doi: 10.1590/fst.42721
25. Xie X, Chen C, Fu X. Study on the bioaccessibility of phenolic compounds and bioactivities of passion fruit juices from different regions in vitro digestion. J Food Proc Preservat. (2021) 45:e15056. doi: 10.1111/jfpp.15056
26. Aguillón-Osma J, Luzardo-Ocampo I, Cuellar-Nuñez ML, Maldonado-Celis ME, Loango-Chamorro N, Campos-Vega R. Impact of in vitro gastrointestinal digestion on the bioaccessibility and antioxidant capacity of bioactive compounds from Passion fruit (Passiflora edulis) leaves and juice extracts. J Food Biochem. (2019) 43:e12879. doi: 10.1111/jfbc.12879
27. Alissa EM, Ferns GA. Dietary fruits and vegetables and cardiovascular diseases risk. Crit Rev Food Sci Nutr. (2017) 57:1950−62.
28. Santos E, Andrade R, Gouveia E. Utilization of the pectin and pulp of the passion fruit from Caatinga as probiotic food carriers. Food Biosci. (2017) 20:56–61. doi: 10.1016/j.fbio.2017.08.005
29. Gunathilake K, Ranaweera K, Rupasinghe H. Change of phenolics, carotenoids, and antioxidant capacity following simulated gastrointestinal digestion and dialysis of selected edible green leaves. Food Chem. (2018) 245:371–9. doi: 10.1016/j.foodchem.2017.10.096
30. Masson-Delmotte V, Zhai P, Pirani A, Connors SL, Péan C, Berger S, et al. Climate change 2021: the physical science basis. In: Contribution of Working Group I to the Sixth Assessment Report of the Intergovernmental Panel on Climate Change (2021). p. 2.
31. Oliveira PN, dos Santos Gomes PC, Alcarde AR, Bortoletto AM, Neta MTSL, Narain N, et al. Characterization and volatile profile of passion fruit spirit. Int J Gastron Food Sci. (2020) 21:100223. doi: 10.1016/j.ijgfs.2020.100223
32. Liu Y, Su Y, Quan X, Fan X, Chen S, Yu H, et al. Facile ammonia synthesis from electrocatalytic N2 reduction under ambient conditions on N-doped porous carbon. ACS Catal. (2018) 8:1186–91. doi: 10.1021/acscatal.7b02165
33. Putnik P, Bursać Kovačević D, Režek Jambrak A, Barba FJ, Cravotto G, Binello A, et al. Innovative “green” and novel strategies for the extraction of bioactive added value compounds from citrus wastes—a review. Molecules. (2017) 22:680. doi: 10.3390/molecules22050680
34. Roohinejad S, Koubaa M, Barba FJ, Saljoughian S, Amid M, Greiner R. Application of seaweeds to develop new food products with enhanced shelf-life, quality and health-related beneficial properties. Food Res Int. (2017) 99:1066–83. doi: 10.1016/j.foodres.2016.08.016
35. Suleria HA, Barrow CJ, Dunshea FR. Screening characterization of phenolic compounds and their antioxidant capacity in different fruit peels. Foods. (2020) 9:1206. doi: 10.3390/foods9091206
36. Afieroho OE, Afieroho MC. Evaluation of the nitric oxide scavenging potential of Harungana madagascariensis Lam ex poir (Hypericaceae) fruits oil. Pharma Innov. (2017) 6:171.
37. Ahmad N, Qamar M, Yuan Y, Nazir Y, Wilairatana P, Mubarak MS. Dietary polyphenols: Extraction, identification, bioavailability, and role for prevention and treatment of colorectal and prostate cancers. Molecules. (2022) 27:2831. doi: 10.3390/molecules27092831
38. Dludla PV, Nkambule BB, Jack B, Mkandla Z, Mutize T, Silvestri S, et al. Inflammation and oxidative stress in an obese state and the protective effects of gallic acid. Nutrients. (2018) 11:23. doi: 10.3390/nu11010023
39. M. Marques MP, Borges F, Sousa J, Calheiros R, Garrido J, et al. Cytotoxic and COX-2 inhibition properties of hydroxycinnamic derivatives. Lett Drug Design Discov. (2006) 3:316–20. doi: 10.2174/157018006777574212
40. Sayago-Ayerdi S, García-Martínez DL, Ramírez-Castillo AC, Ramírez-Concepción HR, Viuda-Martos M. Tropical fruits and their co-products as bioactive compounds and their health effects: a review. Foods. (2021) 10:1952. doi: 10.3390/foods10081952
41. Zeng Y, Zhou W, Yu J, Zhao L, Wang K, Hu Z, et al. By-products of fruit and vegetables: antioxidant properties of extractable and non-extractable phenolic compounds. Antioxidants. (2023) 12:418. doi: 10.3390/antiox12020418
42. Shi M, Ali MM, He Y, Ma S, Rizwan HM, Yang Q, et al. Flavonoids accumulation in fruit peel and expression profiling of related genes in purple (Passiflora edulis f. edulis) and yellow (Passiflora edulis f. flavicarpa) passion fruits Plants. (2021) 10:2240. doi: 10.3390/plants10112240
43. Domínguez-Rodríguez G, Marina ML, Plaza M. Rapid fingerprinting of extractable and non-extractable polyphenols from tropical fruit peels using direct analysis in real time coupled to orbitrap mass spectrometry. Food Chem. (2022) 371:131191. doi: 10.1016/j.foodchem.2021.131191
44. Ramli ANM, Manap NWA, Bhuyar P, Azelee NIW. Passion fruit (Passiflora edulis) peel powder extract and its application towards antibacterial and antioxidant activity on the preserved meat products. SN Appl Sci. (2020) 2:1–11. doi: 10.1007/s42452-020-03550-z
45. Araújo CLA, Salles BCC, Duarte SM, Rodrigues MR, Paula FBA. Passion fruit (Passiflora edulis) leaf extract modulates the oxidative metabolism of rat peritoneal neutrophils in a model of inflammation. Braz J Pharm Sci. (2020) 56:7362. doi: 10.1590/s2175-97902020000117362
46. Ożarowski M, Karpiński TM. Extracts flavonoids of Passiflora species as promising anti-inflammatory and antioxidant substances. Curr Pharm Des. (2021) 27:2582–604. doi: 10.2174/1381612826666200526150113
47. Huang H, Ullah F, Zhou D-X, Yi M, Zhao Y. Mechanisms of ROS regulation of plant development and stress responses. Front Plant Sci. (2019) 10:800. doi: 10.3389/fpls.2019.00800
48. Gökbulut A, Özhan O, Karacaoğlu M, Şarer E. Radical scavenging activity and vitexin content of Vitex agnus castus leaves and fruits. FABAD J Pharmaceut. (2012) 35:85–91.
49. Kandandapani S, Balaraman AK, Ahamed HN. Extracts of passion fruit peel and seed of Passiflora edulis (Passifloraceae) attenuate oxidative stress in diabetic rats. Chin J Nat Med. (2015) 13:680–6. doi: 10.1016/S1875-5364(15)30066-2
50. Holidah D, Dewi IP, Pusparini D, Rochayati D, Nurrizki AM. Effects of Passiflora edulis var. flavicarpa on liver functional parameters in alloxan induced diabetic mice. Med Plants. (2021) 13:493–8. doi: 10.5958/0975-6892.2021.00057.5
51. Krambeck K, Oliveira A, Santos D, Pintado MM, Baptista Silva J, Sousa Lobo JM, et al. Identification and quantification of stilbenes (Piceatannol and resveratrol) in Passiflora edulis by-products. Pharmaceuticals. (2020) 13:73. doi: 10.3390/ph13040073
52. Dos Santos FA, Xavier JA, da Silva FC, Merlin JJ, Goulart MO, Rupasinghe HV. Antidiabetic, antiglycation, and antioxidant activities of ethanolic seed extract of Passiflora edulis and piceatannol in vitro. Molecules. (2022) 27:4064. doi: 10.3390/molecules27134064
53. Piotrowska H, Kucinska M, Murias M. Biological activity of piceatannol: leaving the shadow of resveratrol. Mutat Res/Rev Mutat Res. (2012) 750:60–82. doi: 10.1016/j.mrrev.2011.11.001
54. Kawakami S, Morinaga M, Tsukamoto-Sen S, Mori S, Matsui Y, Kawama T. Constituent characteristics and functional properties of passion fruit seed extract. Life. (2021) 12:38. doi: 10.3390/life12010038
55. Allaqaband S, Dar AH, Patel U, Kumar N, Nayik GA, Khan SA, et al. Utilization of fruit seed-based bioactive compounds for formulating the nutraceuticals and functional food: a review. Front Nutr. (2022) 9:902554. doi: 10.3389/fnut.2022.902554
56. Karasawa MMG, Mohan C. Fruits as prospective reserves of bioactive compounds: a review. Nat Prod Bioprosp. (2018) 8:335–46. doi: 10.1007/s13659-018-0186-6
57. Tanaka T, Shnimizu M, Moriwaki H. Cancer chemoprevention by caroteno. Molecules. (2012) 17:3202–42. doi: 10.3390/molecules17033202
58. Nishino H, Murakoshi M, Ii T, Takemura M, Kuchide M, Kanazawa M, et al. Carotenoids in cancer chemoprevention. Cancer Metastasis Rev. (2002) 21:257–64. doi: 10.1023/A:1021206826750
59. Paiva SA, Russell RM. β-carotene other carotenoids as antioxidants. J Am Coll Nutr. (1999) 18:426–33. doi: 10.1080/07315724.1999.10718880
60. Stahl W, Sies H. β-Carotene and other carotenoids in protection from sunlight. Am Clini Nutr. (2012) 96:1179S−84S. doi: 10.3945/ajcn.112.034819
61. Bendich A. The safety of β-carotene. Nutr Cancer. (1988) 11:207–14. doi: 10.1080/01635588809513989
62. Rockett FC, de O, Schmidt H, Pagno CH, Possa J, Assis RQ, et al. Seven Brazilian native fruits as potential sources of bioactive compounds and antioxidants. Curr. Bioactive Comp. (2021) 17:120–129. doi: 10.2174/1573407216666200303110113
63. Barua AB, Furr HC, Olson JA, van Breemen RB. Vitamin A and carotenoids. In: Modern Chromatographic Analysis of Vitamins. Boca Raton, FL: CRC Press (2000) p. 8–75.
64. Bernstein PS, Li B, Vachali PP, Gorusupudi A, Shyam R, Henriksen BS, et al. Lutein, zeaxanthin, and meso-zeaxanthin: The basic and clinical science underlying carotenoid-based nutritional interventions against ocular disease. Prog Retin Eye Res. (2016) 50:34–66. doi: 10.1016/j.preteyeres.2015.10.003
65. Zhang J, Zhao Y, Chen C, Huang Y-C, Dong C-L, Chen C-J, et al. Tuning the coordination environment in single-atom catalysts to achieve highly efficient oxygen reduction reactions. J Am Chem Soc. (2019) 141:20118–26. doi: 10.1021/jacs.9b09352
66. Kijlstra A, Tian Y, Kelly ER, Berendschot TT. Lutein: more than just a filter for blue light. Prog Retin Eye Res. (2012) 31:303–15. doi: 10.1016/j.preteyeres.2012.03.002
67. Schalch W, Cohn W, Barker FM, Köpcke W, Mellerio J, Bird AC, et al. Xanthophyll accumulation in the human retina during supplementation with lutein or zeaxanthin–the LUXEA (LUtein Xanthophyll eye accumulation) study. Arch Biochem Biophys. (2007) 458:128–35. doi: 10.1016/j.abb.2006.09.032
68. Ignat I, Volf I, Popa VI. A critical review of methods for characterisation of polyphenolic compounds in fruits and vegetables. Food Chem. (2011) 126:1821–35. doi: 10.1016/j.foodchem.2010.12.026
69. Silva JK, Cazarin CBB, Colomeu TC, Batista ÂG, Meletti LM, Paschoal JAR, et al. Antioxidant activity of aqueous extract of passion fruit (Passiflora edulis) leaves: in vitro and in vivo study. Food Res Int. (2013) 53:882–90. doi: 10.1016/j.foodres.2012.12.043
70. Zucolotto SM, Fagundes C, Reginatto FH, Ramos FA, Castellanos L, Duque C, et al. Analysis of C-glycosyl flavonoids from South American Passiflora species by HPLC-DAD and HPLC-MS. Phytochem Anal. (2012) 23:232–9. doi: 10.1002/pca.1348
71. Silva NH, Rodrigues AF, Almeida IF, Costa PC, Rosado C, Neto CP, et al. Bacterial cellulose membranes as transdermal delivery systems for diclofenac: in vitro dissolution and permeation studies. Carbohydr Polym. (2014) 106:264–9. doi: 10.1016/j.carbpol.2014.02.014
72. Santos VG, Franchini E, Lima-Silva AE. Relationship between attack and skipping in taekwondo contests. J Strength Condit Res. (2011) 25:1743–51. doi: 10.1519/JSC.0b013e3181ddfb0f
73. Wolde HF, Gonete KA, Akalu TY, Baraki AG, Lakew AM. Factors affecting neonatal mortality in the general population: evidence from the 2016 Ethiopian Demographic and Health Survey (EDHS)—multilevel analysis. BMC Res Notes. (2019) 12:1–6. doi: 10.1186/s13104-019-4668-3
74. Azizan A, Lee AX, Abdul Hamid NA, Maulidiani M, Mediani A, Abdul Ghafar SZ, et al. Potentially bioactive metabolites from pineapple waste extracts and their antioxidant and α-glucosidase inhibitory activities by 1H NMR. Foods. (2020) 9:173. doi: 10.3390/foods9020173
75. Rodríguez-González S, Gutiérrez-Ruíz IM, Pérez-Ramírez IF, Mora O, Ramos-Gomez M, Reynoso-Camacho R. Mechanisms related to the anti-diabetic properties of mango (Mangifera indica L.) juice by-product. J Funct Foods. (2017) 37:190–9. doi: 10.1016/j.jff.2017.07.058
76. Xiao Y, Reis LA, Feric N, Knee EJ, Gu J, Cao S, et al. Diabetic wound regeneration using peptide-modified hydrogels to target re-epithelialization. Proc Nat Acad Sci. (2016) 113:E5792–801. doi: 10.1073/pnas.1612277113
77. Abdollahi Z, Zare EN, Salimi F, Goudarzi I, Tay FR, Makvandi P. Bioactive carboxymethyl starch-based hydrogels decorated with CuO nanoparticles: antioxidant and antimicrobial properties and accelerated wound healing in vivo. Int J Mol Sci. (2021) 22:2531. doi: 10.3390/ijms22052531
78. Lai Z, Li Y, Wang F, Cheng Y, Fan B, Yu JQ, et al. Arabidopsis sigma factor binding proteins are activators of the WRKY33 transcription factor in plant defense. Plant Cell. (2011) 23:3824–41. doi: 10.1105/tpc.111.090571
79. Goss M, Nunes M, Machado I, Merlin L, Macedo N, Silva A, et al. Peel flour of Passiflora edulis Var. Flavicarpa supplementation prevents the insulin resistance and hepatic steatosis induced by low-fructose-diet in young rats. Biomed Pharmacother. (2018) 102:848–54. doi: 10.1016/j.biopha.2018.03.137
80. Kido LA, Rossetto IMU, Baseggio AM, Chiarotto GB, Alves LF, Santos FR, et al. Brazilian berry extract differentially induces inflammatory and immune responses in androgen dependent and independent prostate cancer cells. J Cancer Prevent. (2022) 27:182–91. doi: 10.15430/JCP.2022.27.3.182
81. Baseggio AM, Kido LA, Viganó J, Carneiro MJ, Lamas C, Martínez J, et al. Systemic antioxidant and anti-inflammatory effects of yellow passion fruit bagasse extract during prostate cancer progression. J Food Biochem. (2022) 46:e13885. doi: 10.1111/jfbc.13885
82. Silva GC, Salvador MJ, Bottoli CBG. Towards the cosmetic application of Passiflora coccinea (Aubl.): antioxidant activity and photo protective capacity of the methanolic and glycolic leaf extracts. Braz J Pharm Sci. (2020) 56:7691. doi: 10.1590/s2175-97902019000317691
83. Ramirez V, Arango Varela SS, Maldonado Celis ME, Uribe Yunda DF, Aguillón Osma J, Quintero JP, et al. Biological activity of Passiflora edulis f. Flavicarpa ethanolic leaves extract on human colonic adenocarcinoma cells. J Appl Pharmaceut Sci. (2019) 9:64–71. doi: 10.7324/JAPS.2019.90209
84. de Albuquerque MAC, Levit R, Beres C, Bedani R, de LeBlanc A, Saad SMI, et al. Tropical fruit by-products water extracts as sources of soluble fibres and phenolic compounds with potential antioxidant, anti-inflammatory, functional properties. J Funct Foods. (2019) 52:724–733. doi: 10.1016/j.jff.2018.12.002
85. Vuolo MM, Lima GC, Batista ÂG, Carazin CBB, Cintra DE, Prado MA, et al. Passion fruit peel intake decreases inflammatory response and reverts lipid peroxidation and adiposity in diet-induced obese rats. Nutr Res. (2020) 76:106–17. doi: 10.1016/j.nutres.2019.08.007
86. Abdel Aziz NS, Elawady MY, Rizk SA, Hakim SA, Shahy EM, Abdel-Shafy E. Inflammatory cytokines, oxidative stress biomarkers and clinical manifestations of organophosphorus pesticides-exposed researchers. Egypt J Chem. (2021) 64:2235–45.
87. Zafar MS, Quarta A, Marradi M, Ragusa A. Recent developments in the reduction of oxidative stress through antioxidant polymeric formulations. Pharmaceutics. (2019) 11:505. doi: 10.3390/pharmaceutics11100505
88. Chávez-Reyes J, Escárcega-González CE, Chavira-Suárez E, León-Buitimea A, Vázquez-León P, Morones-Ramírez JR, et al. Susceptibility for some infectious diseases in patients with diabetes: the key role of glycemia. Front Public Health. (2021) 9:559595. doi: 10.3389/fpubh.2021.559595
89. de Melo Pereira GV, de Oliveira Coelho B, Júnior AIM, Thomaz-Soccol V, Soccol CR. How to select a probiotic? A review and update of methods and criteria. Biotechnol Adv. (2018) 36:2060–76. doi: 10.1016/j.biotechadv.2018.09.003
90. Adedayo AD, Stephen AO, Adekilekun TA, Daniel AT. Lead induces inflammation and neurodegenerative changes in the rat medial prefrontal cortex. Anatomy. (2017) 11:79–86. doi: 10.2399/ana.17.015
91. Singh JP, Kaur A, Shevkani K, Singh N. Composition, bioactive compounds and antioxidant activity of common Indian fruits and vegetables. J Food Sci Technol. (2016) 53:4056–66. doi: 10.1007/s13197-016-2412-8
92. Serafini B, Rosicarelli B, Veroni C, Mazzola GA, Aloisi F. Epstein-Barr virus-specific CD8 T cells selectively infiltrate the brain in multiple sclerosis and interact locally with virus-infected cells: clue for a virus-driven immunopathological mechanism. J Virol. (2019) 93:e00980–19. doi: 10.1128/JVI.00980-19
93. da Silva LN, Lima LKS, dos Santos IS, Sampaio SR, de Jesus ON. Multivariate strategies for selection of organic growing media to produce yellow passion fruit seedlings. Organic Agricult. (2022) 12:445–59. doi: 10.1007/s13165-022-00401-6
94. Nguyen TTT, Le TQ, Nguyen TTA, Nguyen LTM, Nguyen DTC, Van Tran T. Characterizations antibacterial activities of passion fruit peel pectin/chitosan composite films incorporated Piper betle L. leaf extract for preservation of purple eggplants. Heliyon. (2022) 8:e10096. doi: 10.1016/j.heliyon.2022.e10096
95. Partridge AW, Kaan HYK, Juang Y-C, Sadruddin A, Lim S, Brown CJ, et al. Incorporation of putative helix-breaking amino acids in the design of novel stapled peptides: exploring biophysical and cellular permeability properties. Molecules. (2019) 24:2292. doi: 10.3390/molecules24122292
96. Viganó J, Coutinho JP, Souza DS, Baroni NA, Godoy HT, Macedo JA, et al. Exploring the selectivity of supercritical CO2 to obtain nonpolar fractions of passion fruit bagasse extracts. J Supercrit Fluids. (2016) 110:1–10. doi: 10.1016/j.supflu.2015.12.001
97. Giambanelli E, Gómez-Caravaca AM, Ruiz-Torralba A, Guerra-Hernández EJ, Figueroa-Hurtado JG, García-Villanova B, et al. New advances in the determination of free and bound phenolic compounds of banana passion fruit pulp (Passiflora tripartita, var. Mollissima (Kunth) lh Bailey) and their in vitro antioxidant and hypoglycemic capacities. Antioxidants. (2020) 9:628. doi: 10.3390/antiox9070628
98. Ozarowski M, Piasecka A, Paszel-Jaworska A, de Chaves DSA, Romaniuk A, Rybczynska M, et al. Comparison of bioactive compounds content in leaf extracts of Passiflora incarnata, P. caerulea and P. alata and in vitro cytotoxic potential on leukemia cell lines. Revista Brasileira de Farmacognosia. (2018) 28:179–91. doi: 10.1016/j.bjp.2018.01.006
99. Fonseca AM, Geraldi MV, Junior MRM, Silvestre AJ, Rocha SM. Purple passion fruit (Passiflora edulis f. edulis): A comprehensive review on the nutritional value, phytochemical profile and associated health effects. Food Res Int. (2022) 111665. doi: 10.1016/j.foodres.2022.111665
100. Gupta P, Singh A, Singh N, Ali F, Tyagi A, Shanmugam SK. Healing potential of propolis extract–Passiflora edulis seed oil emulgel against excisional wound: biochemical, histopathological, and cytokines level evidence. Assay Drug Dev Technol. (2022) 20:300–16. doi: 10.1089/adt.2022.075
101. Sousa DF, Veras VS, Freire VE, Paula ML, Serra MA, Costa AC, et al. Effectiveness of passion fruit peel flour (Passiflora edulis L.) versus turmeric flour (Curcuma longa L.) on glycemic control: systematic review and meta-analysis. Curr Diab Rev. (2020) 16:450–456. doi: 10.2174/1573399815666191026125941
102. da Silva JK, Cazarin CBB, Batista ÂG, Maróstica M Jr. Effects of passion fruit (Passiflora edulis) byproduct intake in antioxidant status of Wistar rats tissues. LWT Food Sci Technol. (2014) 59:1213–9. doi: 10.1016/j.lwt.2014.06.060
103. Ribeiro JS, Santos MJMC, Silva LKR, Pereira LCL, Santos IA, da Silva Lannes SC, et al. Natural antioxidants used in meat products: a brief review. Meat Sci. (2019) 148:181–8. doi: 10.1016/j.meatsci.2018.10.016
104. Vargas-Mendoza N, Morales-González Á, Madrigal-Santillán EO, Madrigal-Bujaidar E, Álvarez-González I, García-Melo LF, et al. Antioxidant and adaptative response mediated by Nrf2 during physical exercise. Antioxidants. (2019) 8:196. doi: 10.3390/antiox8060196
105. Nascimento MSL, Albuquerque TDR, Nascimento AFS, Caldas IS, Do-Valle-Matta MA, Souto JT, et al. Impairment of interleukin-17A expression in canine visceral leishmaniosis is correlated with reduced interferon-γ and inducible nitric oxide synthase expression. J Comp Pathol. (2015) 153:197–205. doi: 10.1016/j.jcpa.2015.10.174
106. Ojezele, M, Agunbiade SO, Alao OO. Evaluation of the nutritional, phytochemical compositions and likely medicinal benefits of Vernomia amygdalina, Talinum triangulare and Ocimum basilicum leafy-vegetables. Adv Biol Res. (2015) 9:151–5.
107. de Melo Pereira GV, de Carvalho Neto DP, Júnior AIM, Vásquez ZS, Medeiros AB, Vandenberghe LP, et al. Exploring the impacts of postharvest processing on the aroma formation of coffee beans-A review. Food Chem. (2019) 272:441–52. doi: 10.1016/j.foodchem.2018.08.061
108. Tobar-Bolaños G, Casas-Forero N, Orellana-Palma P, Petzold G. Blueberry juice: Bioactive compounds, health impact, and concentration technologies—a review. J Food Sci. (2021) 86:5062–77. doi: 10.1111/1750-3841.15944
109. Viganó J, de Paula Assis BF, Náthia-Neves G, Dos Santos P, Meireles MAA, Veggi PC, et al. Extraction of bioactive compounds from defatted passion fruit bagasse (Passiflora edulis sp.) applying pressurized liquids assisted by ultrasound. Ultrason Sonochem. (2020) 64:104999. doi: 10.1016/j.ultsonch.2020.104999
110. Viganó J, Aguiar AC, Moraes DR, Jara JL, Eberlin MN, Cazarin CB, et al. Sequential high pressure extractions applied to recover piceatannol and scirpusin B from passion fruit bagasse. Food Res Int. (2016) 85:51–8. doi: 10.1016/j.foodres.2016.04.015
111. Santos-Jiménez JL, de Barros Montebianco C, Olivares FL, Canellas LP, Barreto-Bergter E, Rosa RCC, et al. Passion fruit plants treated with biostimulants induce defense-related and phytohormone-associated genes. Plant Gene. (2022) 30:100357. doi: 10.1016/j.plgene.2022.100357
112. Pereira SP, Oldfield L, Ney A, Hart PA, Keane MG, Pandol SJ, et al. Early detection of pancreatic cancer. Lancet Gastroenterol Hepatol. (2020) 5:698–710. doi: 10.1016/S2468-1253(19)30416-9
113. Oliveira DA, Angonese M, Gomes C, Ferreira SR. Valorization of passion fruit (Passiflora edulis sp.) by-products: sustainable recovery and biological activities. J Supercrit Fluids. (2016) 111:55–62. doi: 10.1016/j.supflu.2016.01.010
114. Coelho EM, Gomes RG, Machado BAS, Oliveira RS, dos Santos Lima M, de Azêvedo LC, et al. Passion fruit peel flour–technological properties and application in food products. Food Hydrocoll. (2017) 62:158–64. doi: 10.1016/j.foodhyd.2016.07.027
115. Sittipa C, Achariyaviriya S, Achariyaviriya A, Moran JC. Drying kinetics of passion fruit peel for tea products. In: E3S Web of Conferences, Vol. 187. EDP Sciences (2020). p. 04007. doi: 10.1051/e3sconf/202018704007
117. Li J, Pettinato M, Campardelli R, De Marco I, Perego P. High-pressure technologies for the recovery of bioactive molecules from agro-industrial waste. Appl Sci. (2022) 12:3642. doi: 10.3390/app12073642
118. Vafaei N, Rempel CB, Scanlon MG, Jones PJ, Eskin MN. Application of supercritical fluid extraction (SFE) of tocopherols and carotenoids (hydrophobic antioxidants) compared to non-SFE methods. Appl Chem. (2022) 2:68–92. doi: 10.3390/appliedchem2020005
119. Pedro MAM, Telis-Romero J, Telis VRN. Effect of drying method on the adsorption isotherms and isosteric heat of passion fruit pulp powder. Food Sci Technol. (2010) 30:993–1000. doi: 10.1590/S0101-20612010000400024
120. Ribeiro DN, Alves FMS, dos Santos Ramos VH, Alves P, Narain N, Vedoy DR, et al. Extraction of passion fruit (Passiflora cincinnata Mast.) pulp oil using pressurized ethanol and ultrasound: antioxidant activity and kinetics. J Supercriti Fluids. (2020) 165:104944. doi: 10.1016/j.supflu.2020.104944
121. Freitas C, Sousa R, Dias M, Coimbra J. Extraction of pectin from passion fruit peel. Food Eng Rev. (2020) 12:460–72. doi: 10.1007/s12393-020-09254-9
122. Salazar-Mendoza PS, Peralta-Aragón IE, Misailidis ML, Romero-Rivas LC, Strikis PC. Lance flies associated with sweet passion fruit and contributions to the knowledge on Lonchaeidae in Peru. Arquiv Inst Biol. (2019) 86:e0162019. doi: 10.1590/1808-1657000162019
123. Ong YY, Tan WS, Mohamad R, Sieo CC, Tey BT. Biochemical and molecular identification of Enterococcus spp. from red pitaya. Process Biochem. (2014) 49:563–8. doi: 10.1016/j.procbio.2014.01.019
124. Surlehan HF, Noor Azman NA, Zakaria R, Mohd Amin NA. Extraction of oil from passion fruit seeds using surfactant-assisted aqueous extraction. Food Res. (2019) 3:348–56. doi: 10.26656/fr.2017.3(4).146
125. Sonia S, Ruckmani K, Sivakumar M. Antimicrobial and antioxidant potentials of biosynthesized colloidal zinc oxide nanoparticles for a fortified cold cream formulation: a potent nanocosmeceutical application. Mater Sci Eng C. (2017) 79:581–9. doi: 10.1016/j.msec.2017.05.059
126. Wahdaningsih S, Rizkifani S, Utari EK. Anti-aging peel-off mask of dragon fruit peel extract (Hylocereus polyrhizus). J Farmasi Sains Praktis. (2023) 9. doi: 10.31603/pharmacy.v9i3.8837
127. Nattaya N, Poolchanuan P, Unagul P, Thongnest S, Wiyakrutta S, Mahidol C, et al. An anticonvulsive drug, valproic acid (valproate), has effects on the biosynthesis of fatty acids and polyketides in microorganisms. Sci Rep. (2020) 10:9300. doi: 10.1038/s41598-020-66251-y
128. dos Santos GJ, Defendi RO, Düsman E, Biffi MT, Berton GH, Tonin APP, et al. Valorization of wastes from the juice passion fruit production industry: extraction of bioactive compounds from seeds, antioxidant, photoprotective and antiproliferative activities. Waste Biomass Valoriz. (2022) 2022:1–18. doi: 10.1007/s12649-022-01937-0
129. de Moura FA, Macagnan FT, Dos Santos LR, Bizzani M, de Oliveira Petkowicz CL, Da Silva LP. Characterization physicochemical properties of pectins extracted from agroindustrial by-products. J Food Sci Technol. (2017) 54:3111–7. doi: 10.1007/s13197-017-2747-9
130. Liang Y, Yang Y, Zheng L, Zheng X, Xiao D, Wang S, et al. Extraction of pectin from passion fruit peel: composition, structural characterization and emulsion stability. Foods. (2022) 11:3995. doi: 10.3390/foods11243995
131. Kulkarni S, Vijayanand P. Effect of extraction conditions on the quality characteristics of pectin from passion fruit peel (Passiflora edulis f. flavicarpa L). LWT. (2010) 43:1026–31. doi: 10.1016/j.lwt.2009.11.006
132. Vasco-Correa J, Zapata ADZ. Enzymatic extraction of pectin from passion fruit peel (Passiflora edulis f. flavicarpa) at laboratory and bench scale. LWT. (2017) 80:280–5. doi: 10.1016/j.lwt.2017.02.024
133. Rahmani Z, Khodaiyan F, Kazemi M, Sharifan A. Optimization of microwave-assisted extraction and structural characterization of pectin from sweet lemon peel. Int J Biol Macromol. (2020) 147:1107–15. doi: 10.1016/j.ijbiomac.2019.10.079
Keywords: bioactive compounds, passion fruits, non-communicable diseases, extraction methods, by-product
Citation: Weyya G, Belay A and Tadesse E (2024) Passion fruit (Passiflora edulis Sims) by-products as a source of bioactive compounds for non-communicable disease prevention: extraction methods and mechanisms of action: a systematic review. Front. Nutr. 11:1340511. doi: 10.3389/fnut.2024.1340511
Received: 27 November 2023; Accepted: 21 May 2024;
Published: 06 June 2024.
Edited by:
Tița Ovidiu, Lucian Blaga University of Sibiu, RomaniaReviewed by:
Franklin Chamorro, University of Vigo, SpainAdriana Dabija, Ștefan cel Mare University of Suceava, Romania
Endre Mathe, University of Debrecen, Hungary
Copyright © 2024 Weyya, Belay and Tadesse. This is an open-access article distributed under the terms of the Creative Commons Attribution License (CC BY). The use, distribution or reproduction in other forums is permitted, provided the original author(s) and the copyright owner(s) are credited and that the original publication in this journal is cited, in accordance with accepted academic practice. No use, distribution or reproduction is permitted which does not comply with these terms.
*Correspondence: Getu Weyya, Z2V0dXdleWFAZ21haWwuY29t; Abera Belay, YWJlcmEuYmVsYXlAYWFzdHUuZWR1LmV0; Eneyew Tadesse, ZW5leWV3LnRhZGVzc2VAYWFzdHUuZWR1LmV0