- 1Nutrition and Bromatology Group, Department of Analytical Chemistry and Food Science, Instituto de Agroecoloxía e Alimentación (IAA)-CITEXVI, Universidade de Vigo, Vigo, Spain
- 2REQUIMTE/Serviço de Bromatologia, Faculdade de Farmácia da Universidade do Porto, Porto, Portugal
- 3LAQV@REQUIMTE, Department of Chemical Sciences, Faculdade de Farmácia, Universidade do Porto, Porto, Portugal
Consumers are increasingly interested in food products with high nutritional value and health benefits. For instance, fish consumption is linked with diverse positive health benefits and the prevention of certain widespread disorders, such as obesity, metabolic syndrome, or cardiovascular diseases. These benefits have been attributed to its excellent nutritional value (large amounts of high-quality fatty acids, proteins, vitamins, and minerals) and bioactive compounds, while being relatively low-caloric. Atlantic bluefin tuna (Thunnus tynnus) is one of the most consumed species worldwide, motivated by its good nutritional and organoleptic characteristics. Recently, some organizations have proposed limitations on its consumption due to the presence of contaminants, mainly heavy metals such as mercury. However, several studies have reported that most specimens hold lower levels of contaminants than the established limits and that their richness in selenium effectively limits the contaminants’ bioaccessibility in the human body. Considering this situation, this study aims to provide baseline data about the nutritional composition and the latest evidence regarding the beneficial effects of Atlantic bluefin tuna consumption. A review of the risk-benefit ratio was also conducted to evaluate the safety of its consumption, considering the current suggested limitations to this species’ consumption.
1 Introduction
Nowadays, consumers are increasingly aware of the beneficial effects on health of certain foods and the adoption of well-balanced diets. In this sense, most marine products, especially fish, are widely appealing for their high nutritional value (1). Fish consumption has been traditionally linked to many health benefits due to their high omega-3 polyunsaturated fatty acids (PUFAs) content (2), being of particular interest eicosapentaenoic acid (EPA) and docosahexaenoic acid (DHA) (3–6). Both compounds are well-known for their positive effects on the cardiovascular system and the nervous system, as well as the control of inflammatory processes in vertebrates, being beneficial in various human pathologies and disorders like obesity or metabolic syndrome (7, 8). More recently, fish proteins, peptides, and amino acids have harbored attention as they have shown properties similar to PUFAs (9). In addition, fish is also a significant source of vitamin B12 and vitamin D (10). Vitamin B12 is required to form red blood cells and DNA. Deficiency of vitamin D leads to rickets, a low bone mineral density and thereby to osteoporosis, among other pathologies. Fish is also an important source of essential minerals, like copper (Cu), manganese (Mn), zinc (Zn), and selenium (Se), which participate in many biological processes as part of numerous enzymes (10). Cu plays an important role as a catalytic cofactor in numerous critical enzyme reactions in metabolism (11). Mn deficiency results in poor reproductive performance, congenital malformations, growth retardation in offspring, and abnormal function of bone and cartilage (12). Zn is required in the stabilization of the structure of many proteins at all levels of cellular signal transduction (13). Finally, Se plays a fundamental role in reproduction, thyroid function, DNA replication and protection against microbes and oxidant compounds (14). Therefore, fish is considered one of the healthiest foods on global scale and is a fundamental part of a healthy and well-balanced diets.
However, in recent years, some national and international Food Safety Agencies, like the Spanish Agency for Consumer Affairs Food Safety and Nutrition (AECOSAN) and the US Food and Drug Administration (FDA), among others, have recommended limiting the consumption of certain species of fish in children and pregnant women (15). The reason for this limitation is the level of certain heavy metals, like mercury (Hg), found in some blue fish such as Prionace glauca (blue shark), Isurus oxyrinchus (blue pointer or bonito shark), Xiphias gladius (swordfish) and Thunnus thynnus (Atlantic bluefin tuna) (16–18). When Hg reaches the sea from soil or chemical industry, it accumulates in marine species throughout the food chain; the larger and longer predator fish are, the higher the levels found (19, 20). Thus, large fish such as swordfish, bluefin tuna, and sharks accumulate these compounds in their tissues since they feed on small fish. Despite this, some recent studies point out that the risk of Hg intake due to fishery products consumption is not as substantial as commonly believed (21, 22). The European Food Safety Agency (EFSA) has recently stated that limiting fish consumption due to Hg’s presence can lead to more significant health risks than moderate consumption (23). In fact, the European legislation (Commission Regulation (EU) No. 1881/2006) established maximum levels of Hg in fish (0.5–1 mg/kg) based on the level of consumer exposure (24), but the majority of fishery products currently show levels much lower than the limits set in the legislation (21, 22). This points to the current limitation on seafood consumption being somewhat exaggerated. In addition, several studies show that Se, an essential mineral commonly present in seafood, may also protect against the toxic effects of Hg, mainly its most dangerous form of organic methylmercury (25, 26). Thus, the Hg: Se ratio should also be considered when assessing the risk linked to fish intake (11).
The present study will be focused on the Atlantic bluefin tuna (ABFT) Thunnus thynnus (L., 1758), a top-level pelagic predator distributed throughout the Atlantic Ocean, from the Canary Islands to Ireland, with incursions to Norway and the North Sea, the Baltic, and the Barents Sea, Mediterranean and Black Sea, also in Canada and South America, along the Brazilian coast (27) (Figure 1). The species is very voracious and feeds on many other fishes, crustaceans, and cephalopods (28). The generic name of bluefin tuna incorporates three species: the ABFT Thunnus thynnus, the Pacific bluefin tuna Thunnus orientalis, and the southern bluefin tuna Thunnus maccoyii. Throughout history, bluefin tuna Thunnus thynnus has been exploited in the Mediterranean for thousands of years until the end of the 20th century (29). Research on bluefin tuna farming began in the 1970s in Japan, and numerous business initiatives for farming have been launched since then (30). Several studies have been carried out in various field of research such as reproduction, nutrition, genetics, pathology, diseases, and engineering, among others (31, 32). In addition, numerous projects have been launched to improve the captive reproduction of this species, both from the business and research sectors. In a recent study, the European Market Observatory for Fisheries and Aquaculture Products (EUMOFA) shows that tuna is Europe’s most consumed marine species, followed by cod, salmon, and Alaska pollock (33). The consumption of tuna in Europe is around 3.07 kg per capita, from which 99.2% is wild-caught and only 0.83% is farmed (33). There is a growing demand for fresh tuna Thunnus thynnus in Europe. Their production is currently limited to the Mediterranean Sea, mainly in Spain, France, Italy, and to a lesser extent, Portugal, Malta, Croatia, Cyprus, and Greece (34). There has been an essential economic contribution from the bluefin tuna fishing industry, with a value of sale of more than 875 million euros in the Mediterranean Sea since 2018 (35). However, it is necessary to improve the fisheries management to make fishing more sustainable from an environmental point of view. In this sense, the treatment and recovery of the waste originated in such an industry could reduce these environmental issues. By-products from bluefin tuna have several bioactive compounds of considerable economic value that can be extracted and obtained from this discarded biomass following the principles of the circular economy (36).
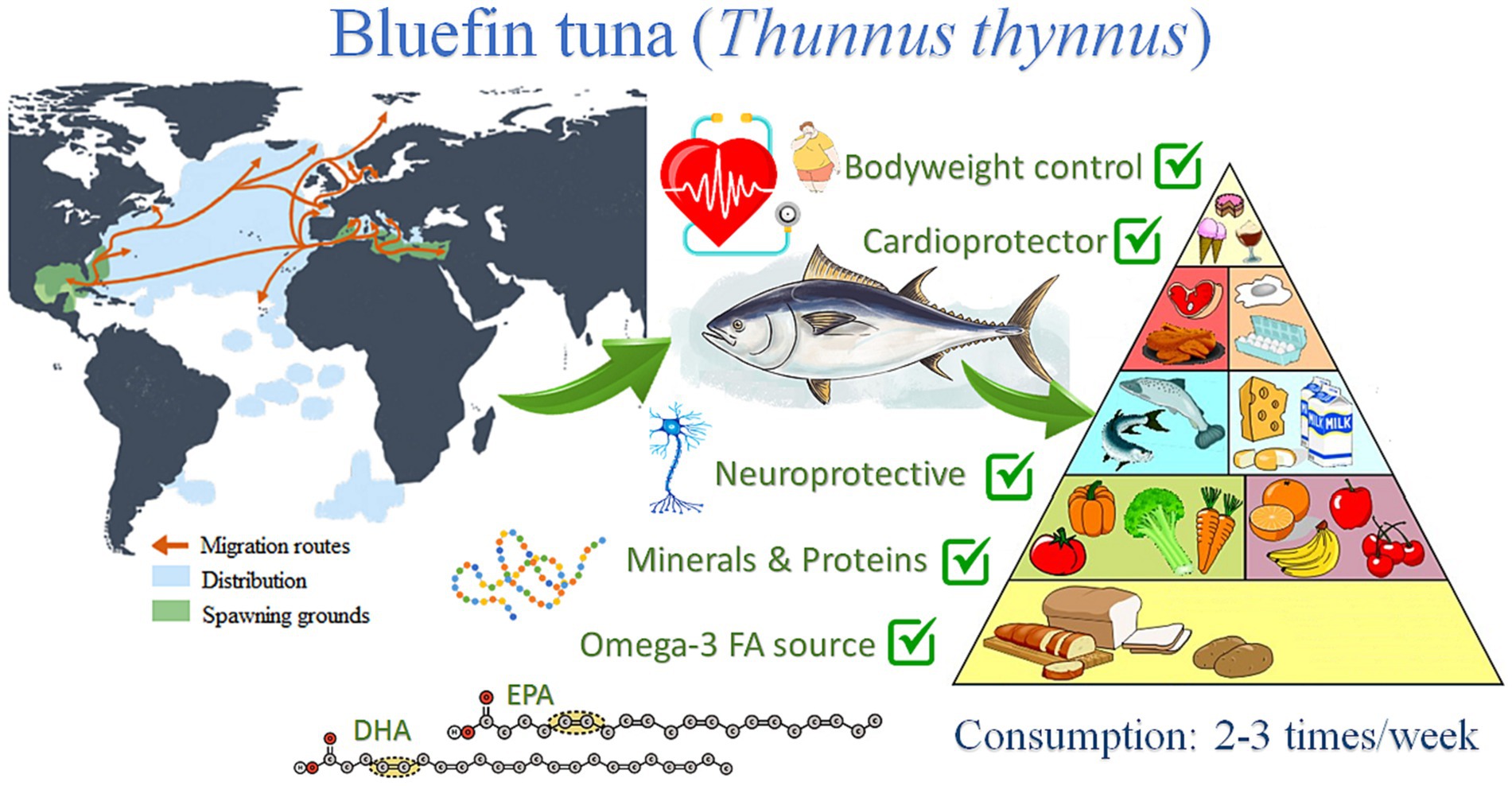
Figure 1. Schematic representation of bluefin tuna (Thunnus thynnus) distribution and their main health impact derived from their consumption.
In this context, the present study is focused on the nutritional composition and contaminants of ABFT Thunnus thynnus, including the latest evidence on human health impact and the assessment of the risk-benefit ratio of its consumption. Knowledge about the nutritional composition and risk-benefit ratio is valuable for consumers to change their diet conscientiously according to their life cycle stages.
2 Nutritional composition
It is well-known that fish consumption has numerous benefits for human health (37, 38). ABFT Thunnus thnunnus is valued as an excellent food worldwide due to its good nutritional and sensory quality, making it a favorite choice in the seafood market. Consequently, many organizations have been interested in developing aquaculture and processing technology to increase fishing and processing efficiency (39). In this section, we will address the nutritional composition of ABFT. Different databases were consulted to provide information about the approximate composition of fish and shellfish. Among them are the global database of FAO/INFOODS, the USDA, the United States National Marine Fisheries Service, and the United Kingdom Department of Health. Table 1 shows the composition of macro and micronutrients present in 100 g of ABFT meat, which is low in calories while providing high-quality proteins and lipids, fat-soluble vitamins, and various essential elements. In addition, the consumption of this species has been linked to a series of beneficial health effects due to the presence of bioactive compounds, including bioactive peptides present in proteins and PUFAs, mainly EPA and DHA (28, 44, 45). Nevertheless, it is important to underline that the nutritional composition of any fish may vary depending on environmental factors, age, sex, maturation stage, and the migratory behavior of each species.
2.1 Protein and amino acid profile
According to the data compiled, the protein content in bluefin tuna is 23 g/100 g of fresh product (Table 1). Considering that the usual protein range provided by fish is between 17–23 g, we find that bluefin tuna has a higher protein content when compared to other species. Similar results have been reported in farmed and wild bluefin tuna samples (21–23 g protein) (46, 47). In 2012, the Spanish Ministry of Agriculture, Food, and Environment published a guide on nutritional declarations and health properties of food products, where ABFT was considered a high-protein food. Additionally, in its health declarations, the European Parliament stated that these proteins contribute to increasing and conserving muscle mass and maintaining bones under normal conditions (40). Experimental studies in animals have demonstrated various benefits derived from fish protein intake. These benefits include hypocholesterolemic effects attributed to the amino acid composition of fish, although the mechanism is not clear (48); antihypertensive effects due to the presence of angiotensin-converting enzyme (ACE) inhibitor peptides (49, 50); and antiatherosclerotic effects, which are attributed to the antioxidant properties of peptides and fish protein hydrolysates (44). In addition, it has also been shown that proteins can improve insulin sensitivity, prevent metabolic syndrome, and reduce the risk of type 2 diabetes (44).
Fish proteins are better quality than red meat due to their lower collagen content and better digestibility, reported to be over 90% (6, 51). The nutritional value of a protein depends on the amino acid composition (score), the content of essential amino acids, and its susceptibility to digestion (52–54). Currently, the suggested method for assessing protein quality is a chemical score, or a protein digestibility corrected amino acid score (PDCAAS) (52–54). The amino acid profile of ABFT shows a high amount of histidine, isoleucine, leucine, lysine, threonine, tryptophan, valine, phenylalanine, and methionine (6). They are considered essential amino acids since humans do not have the ability to synthesize them, and must be incorporated into the diet (Table 1). Table 1 presents the contribution of ABFT regarding the reference daily intake. Just 100 g of ABTF cover between 44% and 69% of the requirements for all essential amino acids (55–58). Due to their amino acid profile, fish proteins can also benefit health, mainly through antioxidant and anti-inflammatory effects. For example, an adequate supply of histidine through the diet provides benefits against age-related neurodegenerative and cognitive disorders, metabolic syndrome, rheumatoid arthritis, and inflammatory bowel disease (59). The three branched-chain amino acids, leucine, isoleucine, and valine, also play a fundamental role in regulating energy homeostasis, metabolism, innate and adaptive immunity, and glucose metabolism, lipid and protein synthesis. Therefore, current evidence indicates that the adequate supply of these amino acids through the diet could positively affect the parameters associated with metabolic diseases (60). Another aspect to highlight in the amino acid content of bluefin tuna is the contribution of phenylalanine and tryptophan, as both amino acids are considered natural antidepressants (61). Tryptophan is additionally vital for the correct functionality of the brain–brain axis, gut, and immune system (62).
On the other hand, the protein content is essential from an organoleptic point of view since fish species containing small amounts of protein tend to lose a considerable amount of water during cooking, which ruins the texture of the meat (47). Thus, the high protein content of this species also contributes to its good organoleptic properties.
2.2 Lipid content: fatty acids profile and w-3/w-6 relation
Lipids are macronutrients needed in the human diet and can affect health depending on the type and proportion of the dietary fatty acids consumed. It has been stated that monosaturated fatty acids (MUFAs) and PUFAs exert beneficial properties in human health (63). The lipid content of ABFT corresponds to 12 g/100 g in both wild and farmed specimens (Table 1) (64). Due to its high lipidic content, this species is considered a bluefish (64). The guidelines published in 2012 by the Spanish Ministry of Agriculture, Food and Environment declared that ABFT is low in saturated fats and high in PUFAs and that the latest contribute to the functioning of a normal heart (40). The fatty acid profile of ABFT is shown in Figure 2. PUFAs represent the main contribution to the total content of fatty acids (3.58 g/100 g) in Atlantic bluefin tuna. Within this group, DHA (2.18 g/100 g), EPA (0.693 g/100 g), and DPA (0.306 g/100 g) are the most abundant. Regarding MUFAs, oleic acid (2.263 g/100 g) and palmitic acid (0.397 g/100 g) stand out (Figure 2). Several studies have reported similar results in farmed ABFT, with 3.6 g/100 g of PUFA (47, 64). One minor difference was that the leading group of fatty acids corresponded to MUFAs, accounting for 42% of the total lipid profile (1.2 g/100 g of oleic acid and 1.1 g/100 g of erucic acid). These differences could be due to the diet received by the species in cultivation, sex, or the size of the animals under study. Other factors that may influence the lipidic composition of fish include environmental factors, age, state of maturation, and migratory behavior (41). The lipids present in ABFT have exceptional quality indices: an excellent omega 3/omega 6 ratio (9/1), an adequate polyunsaturated/saturated fatty acids ratio (1.16), and an adequate polyunsaturated/monounsaturated/saturated fatty acids ratio (2.03) (41, 47, 64). Furthermore, low levels of atherogenicity indices (AI), thrombogenicity indices (TI), and a high ratio of hypocholesterolemic to hypercholesterolemic fatty acids (HH) have been reported, indicating that the intake of this fish may exert hypocholesterolemic effects (4, 64, 65). Therefore, the consumption of ABFT could be beneficial in preventing cardiovascular diseases (66).
Various organizations such as FAO (Food and Agriculture Organization), the Academy of Nutrition and Dietetics, and the European Association for Cardiovascular recommend a minimum intake of EPA and DHA of 250 mg for adults and, in the case of pregnant and lactating women, the amount of DHA should increase between 100–200 mg (67–70). In this sense, ABFT guarantees a good quantity of fatty acids. Hundred grams of tuna meat provides 0.693 and 2.18 g of EPA and DHA, respectively, contributing to more than 100% of the reference daily intake. Consumption of these fatty acids has essential roles in human health, including promoting cardiovascular health and protection against neurological and inflammatory conditions (68, 71). Observational studies demonstrated a protective effect of fish intake on cardiovascular disease risk. In agreement, various scientific organizations affirm that the consumption of at least two servings of fish per week, where at least 1 is an oily fish, is associated with a decreased risk of death from coronary heart disease of at least 25% compared to those who do not eat fish (67–70, 72).
2.3 Carbohydrates
Bluefin tuna tissue comprises lipids and proteins, so the proportions of carbohydrates are minor, almost insignificant. In Table 1 it is shown that the carbohydrate content is 0 g/100 g (73).
2.4 Vitamins
ABFT stands out for containing significant amounts of B complex vitamins, including thiamine (B1) 0.241 mg/100 g, niacin (B3) 17.8 mg/100 g, pyridoxine (B6) 0.46 mg/100 g and cobalamin (B12) 5 μg/100 g. Thus, 100 g of bluefin tuna provides between 25% and 50% of the reference daily intake of these vitamins (Table 1). The report published by the Spanish Ministry of Agriculture, Food and Environment in 2012 established that bluefin tuna is a good source of vitamins. Within the nutritional declaration, it is also indicated that thiamine contributes to the normal functioning of energy metabolism, the nervous system, the heart, and psychological functions; niacin contributes to the maintenance of the skin and mucosa and reduces fatigue; and pyridoxine and cobalamin vitamins contribute to the normal functioning of the immune system, formation of red blood cells, and the process of cell division (40).
Additionally, bluefin tuna is rich in fat-soluble vitamins such as vitamins A, D, and E, and its consumption can contribute between 25% and 80% of the reference daily intake. Consumption of these vitamins is important because they contribute to normal iron metabolism, immune system functioning, and cell differentiation process. In the particular case of vitamin D, it contributes to the maintenance of normal bones and teeth, the maintenance of normal calcium levels in the blood, and the normal absorption and utilization of calcium and phosphorus (74–76). On the other hand, although to a lesser extent, ABFT is also a source of vitamin E, which stands out for its powerful antioxidant role and free radical scavenger (77).
2.5 Minerals
Minerals have a crucial role in human health and metabolism, with intake through the diet being essential (78). In this context, ABFT constitutes an excellent food source of minerals. Table 1 reports the contribution of minerals in 100 g of tuna, highlighting 28 mg of Mg, and 82 mg of Se. According to the nutritional declarations published in 2012 by the Spanish Ministry of Agriculture, Food and Environment (40), ABFT is an excellent source of these minerals. Regarding human health, Mg contributes to normal energy metabolism, electrolyte balance, normal muscle and nervous system function, normal protein synthesis, and cell division (40, 79, 80). Se is attributed to different health benefits; among them is the contribution to the normal functioning of the immune system, normal thyroid function, and the protection of cells against oxidative damage since it is part of many selenoproteins, which are responsible for biological reactions of reduction-oxidation type, antioxidant defense, metabolism of thyroid hormone and immune responses (81, 82). Furthermore, various studies report that Se can protect against environmental contaminants, such as mercury (Hg), commonly found in some fish species (83–87), but this will be discussed later (see Table 2).
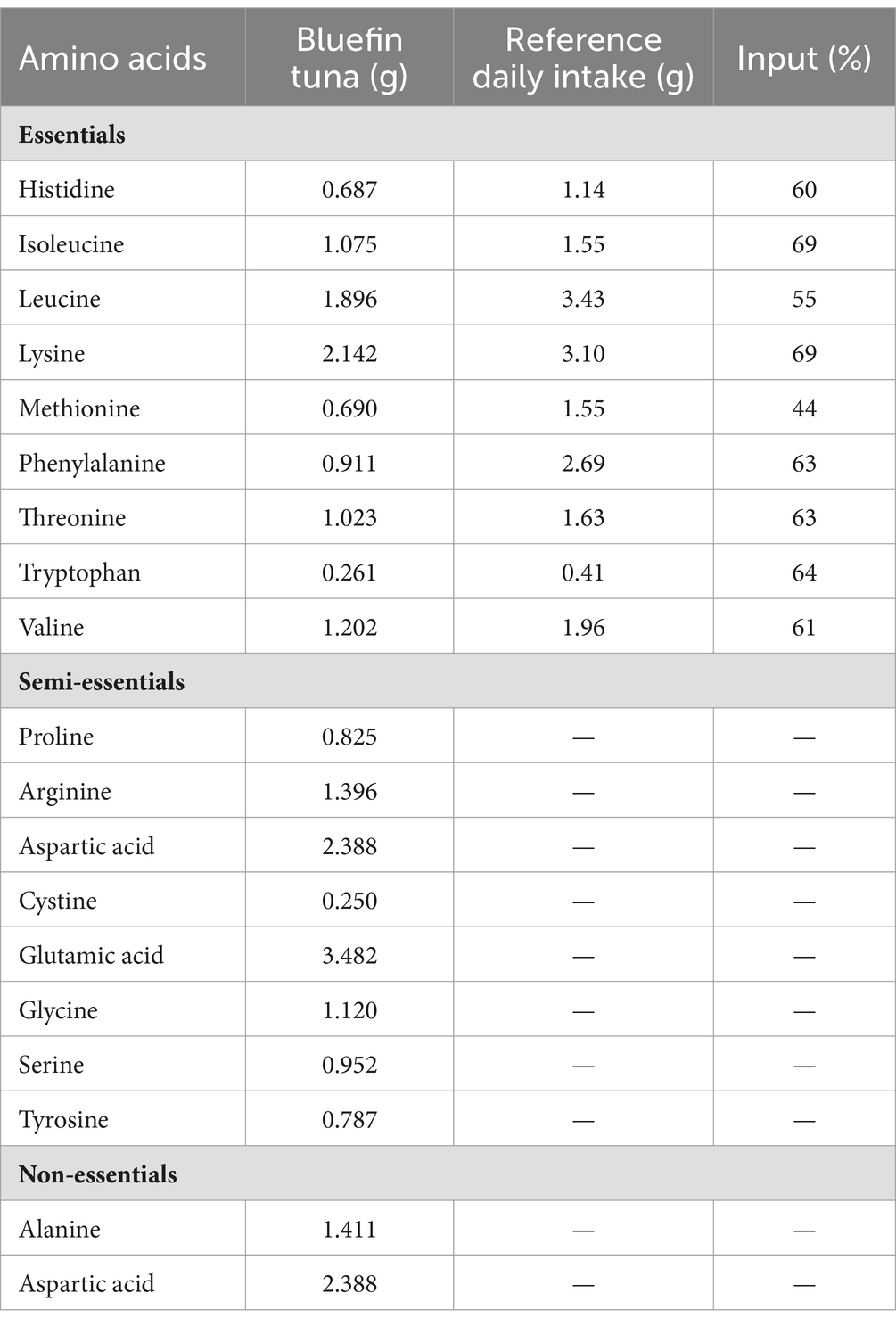
Table 2. Amino acid profile, recommended daily intake values and percentage of contribution to the daily diet of the amino acids present in bluefin tuna (55–58).
Regarding the reference daily intake, it has been observed that 100 g of bluefin tuna can contribute 149% of Se recommendation. Additionally, ABFT also contains iodine (36.7 μg/100 g), zinc (1.5 mg/100 g), and phosphorus (200 mg/100 g), being considered as a source of these minerals (42). On the other hand, ABFT has a low contribution of sodium (43 mg/100 g), the nutritional declaration naming it as a low-content source. Thus, its consumption is attractive for low-sodium or low-salt diets, recommended, for example, to patients with hypertension.
3 Health benefits associated with blue fish consumption
As previously mentioned, blue fish and ABFT are highly nutritious seafood products of great interest in the market and among health-conscious consumers (88). Numerous studies have linked the chemical composition of these foods with many biological properties and beneficial effects on health. These beneficial effects are mainly attributed to PUFAs, especially EPA and DHA. Additionally, fish provide other high-quality nutrients, such as proteins, vitamins, and minerals, that may have a synergic effect, reducing the incidence of certain diseases (89). The health benefits associated with fish consumption will be discussed in this section and are summarized in Table 3 and Figure 3.
3.1 Cardiovascular diseases
Globally, cardiovascular diseases (CVDs) are still the leading cause of mortality. According to the World Health Organization (WHO), about 17.9 million people died in 2019 from CVDs, which represents 32% of all global deaths (97). The major risk factors that may trigger CVDs include smoking, hypertension, obesity, dyslipidemia, psycho-social stress, and unhealthy and sedentary lifestyle (98). Current first-line treatments effectively reduce CVD risk; however, adherence to healthier dietary patterns is increasingly encouraged since certain nutrients can contribute to maintaining this risk to the minimum and can be used as a preventive tool (98, 99). In this context, fish represents an important cardioprotective dietary component, attributed to its high omega-3 long chain PUFAs content, especially EPA and DHA (99). Many studies have correlated a higher fish consumption to a lower risk of CVDs, including stroke (98), coronary heart disease (99), hypertension, arrhythmias (100), and cerebrovascular disease (101). Recently, a dose-response meta-analysis showed that fish intake of 20 g/day significantly reduced total CVD mortality (4%) (102). In a further study, these authors also found a significant association between a fish intake of 15 g/day and a reduction of myocardial infarction risk by 4% (99). Increasing fish consumption to 100–700 g/week was significantly associated with stroke risk reduction by 2%–12% (103). Some differences were observed in such association between geographical regions. While a pronounced inverse relationship between fish consumption and CVDs risk was found in Asian countries, studies conducted in Western countries reported a modest U-shaped association (102). This means that both low and high fish consumption could lead to higher CVDs risk. Possibly, this variation may be attributed to different cooking fish methods employed in Asian (mainly steaming and stir-frying) and Western countries (deep-frying) being the latter more unhealthy (102).
Many biological mechanisms are responsible for the cardioprotective effects attributed to omega-3 long chain PUFAs. Among them, are anti-inflammatory (104), and antioxidant action (105), antiarrhythmic and antithrombosis action, regulation of blood lipids level, protection of vascular endothelial cells, and immune-modulatory activity (99, 100, 106).
3.2 Neurological diseases
Bluefish consumption has also shown beneficial neuroprotective properties attributed to omega-3 long chain PUFAs composition. These compounds have a crucial role in proper brain development, neuro transmission, neuronal differentiation and growth, gene expression, and modulation of ion channels (107, 108). It has been stated that DHA can enhance blood flow, reduce inflammation and diminish amyloid-β pathology, thus preventing a primary cognitive decline (107). In addition, DHA has vital functions in different stages of the neuronal degeneration process since this compound can keep membrane fluidity, stimulate neurotrophic factors, diminish oxidative stress and cell death and exert anti-inflammatory activities (109). By contrast, DHA levels in the brain decrease with aging, resulting in cognitive decline (108). In a meta-analysis, the impact of DHA supplementation alone or in combination with EPA on specific memory domains (working, episodic and semantic) was studied in adults. These authors found that supplementation with 1 g/day DHA/EPA significantly improved episodic memory in adults with mild memory problems, while DHA supplementation alone induced changes in semantic and working memory to a lesser extent (110).
Regarding the incorporation of fish into diet as a good source of DHA and EPA, some authors found that moderate fish consumption and supplementation with omega-3 long-chain PUFAs (0.5–1 g/day) led to a significant reduction in depression prevalence with an U-shaped association, regardless of sex, cardiometabolic disturbances or lifestyle (111). Other study reported that a decreased ratio of omega-6/omega-3 PUFAs, a reduction of omega-6 PUFAs, and increased EPA and DHA levels in Mediterranean-style diet supplemented with fish oil significantly enhanced mental health in patients with depression over 3 and 6 months. The addition of fish oil to the diet improved omega-3 PUFAs levels while reducing the omega-6 ones (112).
3.3 Metabolic diseases
Metabolic syndrome is a multifactorial disorder resulting from the interaction between genetic, metabolic and environmental factors that can increase the risk of suffering CVDs, type-2 diabetes and all-cause mortality (113). It has been stated that fish consumption could inversely enhance metabolic syndrome features such as insulin resistance, abdominal obesity, hypertension, and dyslipidemia since fish containing omega-3 PUFA can reduce plasma triglycerides, blood pressure, fasting blood glucose while increasing high-density lipoprotein (HDL) cholesterol (113, 114). In addition to omega-3 PUFAs, fish also contain high-quality nutrients such as vitamins, minerals, and proteins, which could contribute to reducing metabolic syndrome (113). In a cross-sectional analysis, higher fish consumption in Norwegian adults was related to a better lipid profile with high HDL cholesterol levels and reduced triglyceride content. These authors also observed that participants consuming fish once a week (aged between 60 and 70 years) showed a 36% lower risk of suffering metabolic syndrome compared to those consuming fish at a low frequency (115). Similarly, in another cross-sectional study, higher fish consumption in Iranian female adults led to a lower prevalence of metabolic syndrome features like low blood pressure and high HDL cholesterol (113).
Many biological mechanisms have been proposed to understand the beneficial effects of omega-3 PUFAs on reducing metabolic syndrome. Among them, omega-3 PUFAs may alter transcription factors activity involved in inflammatory pathways and liver lipid metabolism (116). In this way, omega-3 PUFAs may promote triglyceride oxidation in the liver, adipose tissue and skeletal muscle, thus avoiding fat accumulation in these tissues (117). In addition, omega-3 PUFAs can enhance insulin sensitivity by reducing adipose tissue inflammation and synthesizing peroxisome proliferator-activated receptor alpha (117, 118).
3.4 Immunological system-related diseases
The immune system protects the host from infectious agents, bacteria, and viruses. This system involves various blood-borne factors and cells (119). The phospholipids of human immune cells hold a high concentration of omega-6 PUFAs (6%–10% linoleic acid, 1%–2% dihomo-γ-linoleic and 15%–25% arachidonic acid), while low concentrations of omega-3 PUFAs (<1% α-linoleic acid, 0.1%–0.8% of EPA, and 2%–4% of DHA). The immune processes are controlled by proteins, pro-inflammatory cytokines, eicosanoids, or miscellaneous compounds (120). It has been stated that arachidonic acid is the primary precursor of eicosanoids and leads to the production of inflammatory mediators, controlling inflammatory cell activities, cytokine production, and balance within the immune system (121). Eicosanoids are a family of bioactive mediators that modulate the intensity and duration of inflammatory and immune responses. Therefore, by altering the arachidonic acid concentration, cells will have less ability to produce eicosanoids (121–123).
Some studies concluded that omega-3 long-chain PUFAs, especially EPA and DHA, could reduce immune cells’ capacity to synthesize eicosanoids from arachidonic acid. The levels of eicosanoids are widely elevated when the amount of arachidonic acid is limited (122, 124). Thus, human diets rich in fish or fish oil may increase the concentration of EPA and DHA in immune cells. The anti-inflammatory activity attributed to omega-3 PUFAs may handle their immune function. Some studies conducted in animals, mainly in rats, demonstrated that omega-3 PUFAs affected the production of inflammatory cytokines (120, 121, 125). In fact, incorporating fish oil into the diet reduced the arachidonic acid proportion while increasing EPA and DHA levels in immune cell phospholipids (126, 127). Studies carried out in humans also demonstrated the immunomodulatory effects of omega-3 PUFAs, resulting in a significant decrease in the generation of pro-inflammatory leukotriene B4 and modulating cytokine production (128–130). Studies suggested that when sufficient concentrations of fish oil are consumed, significant anti-inflammatory effects are obtained. According to some authors, 1.35–2.7 g EPA per day is the threshold intake required to achieve a significant immunological effect (131). From these results, it may be concluded that n−3 fatty acids can be used as therapy for any type of inflammation that involves an undesirable immune response (121). Therefore, the regular intake of ABFT may lead to a reduction in the level of inflammation and exert a crucial immunomodulatory effect.
3.5 Bodyweight control
Obesity is considered an energy balance disorder leading to adipose tissue dysfunction. It is associated with high levels of inflammation and metabolic abnormalities (high levels of cytokines) (132). In fact, this disorder usually appears when omega-6:omega-3 ratio is increased, and serum phospholipid n−3 concentrations are decreased (93). Being overweight can lead to the development of other conditions, such as insulin resistance, type 2 diabetes, and some types of CVDs (133, 134). Women have a higher prevalence of obesity and overweight than men, and it increases with age (135). In 2017, approximately 39% of the world’s adult population was overweight, and 13% were obese (136). Although there are various strategies to treat obesity and overweight, such as pharmaceuticals, surgery, or dietary supplements, the prevalence of obesity continues to rise during this decade (117). For this reason, healthy strategies to help in weight loss and reduce body fat are needed. Omega-3 PUFAs might be a good candidate to treat obesity and its related side effects due to its important role as anti-inflammatory agent (117), reducing cytokines such as IL-1, IL-6, and TNF-α (137–139).
Numerous mechanisms have been proposed to explain the effects of omega-3 PUFAs, particularly EPA and DHA, on reducing body weight and enhancing the metabolic profile, including alterations in adipose tissue gene expression, changes in adipokine release, appetite suppression, alterations in carbohydrate metabolism and increase of fat oxidation, among others (117). Despite the knowledge of these mechanisms to reduce obesity, more studies are needed to reach a conclusion. Some works have assessed the effects of omega-3 PUFAs on body weight control both in animals and humans, concluding that EPA and DHA play a key role in promoting protection against body fat gain (140–142). For instance, incorporating omega-3 PUFAs into a rat diet for 3 weeks reduced up to 30% fat weight of subcutaneous and visceral adipose tissues (142). Similarly, other authors demonstrated that obese mice fed a diet rich in omega-3 PUFAs showed a significant loss of weight (143). Other studies dealt with the effects of supplementing the diet of overweight or obese young adult men with lean fish, fatty fish or fish oil capsules during 8 weeks (144, 145). They found a significantly higher weight loss when supplemented with fish-related capsules concerning a diet without fish. On the other hand, Schulz et al. (146) found that regular fish intake led to low weight loss in men and higher weight gain in women. Another study concluded that adopting a Mediterranean diet, including a higher consumption of fish rich in omega-3 PUFAs, did not lead to significant weight changes in men and women compared with lower fish consumption (47). Nonetheless, based on clinical studies, the impact of omega-3 PUFAs on body composition is still uncertain since there is little data available to reach a conclusion.
For this reason, there is still much controversy about whether omega-3 PUFAs exert significant anti-obesity effects (90, 93, 96). In this context, despite the anti-obesity effects of omega-3 PUFAs not yet being clear, incorporating these fatty acids into the diet may mitigate weight gain or maintain weight loss (117). Moreover, they clearly play a beneficial role in obese or overweight people in contributing to reducing inflammatory cytokines levels (137–139) and inflammatory processes (117).
4 Importance of fish consumption during the life cycle stages
4.1 Recommended intake per age group
Bluefish consumption during the life cycle stages is highly relevant. Starting with pregnant women, a sufficient intake of this type of fish is not reached to meet the recommended contributions, it can generate malformations in the fetus and defects in the neural tube. In fact, the EFSA recommends the consumption of blue fish because it can be positive in avoiding cardiovascular diseases. In the first 6 months of life and even in young children, insufficient consumption of blue fish can affect their cognitive development, causing adverse effects on brain and immune function (147, 148). However, there is still no specific information or data on the optimal amounts of ABFT in pregnant women and children under 3 years (149). In children between three and 12 years, the recommended consumption is between 50 g per week, with a total of 120 g per month (150). In adults, according to EFSA, the recommended intake is 125 g per week (148).
As mentioned, ABFT provides vitamins and minerals that stand out in its nutritional composition. ABFT is a source of vitamins B (B6, B3 and B12), D, and minerals such as phosphorus or selenium, which are high contents. For instance, one serving of tuna provides 250% of the recommended intake of vitamin D (151). Table 4 shows the nutritional contribution for each portion of 100 g of ABFT as well as the recommended daily intakes for different groups of age and also differentiated by sex. For instance, every portion of 100 g of this species provides 23 g of protein, which nearly accounts for half of the recommended daily intake. Similarly, a portion of ABFT contributes to fulfilling the recommended intake of minerals, as 100 g of ABFT provides 82 mg of Se (Table 4).
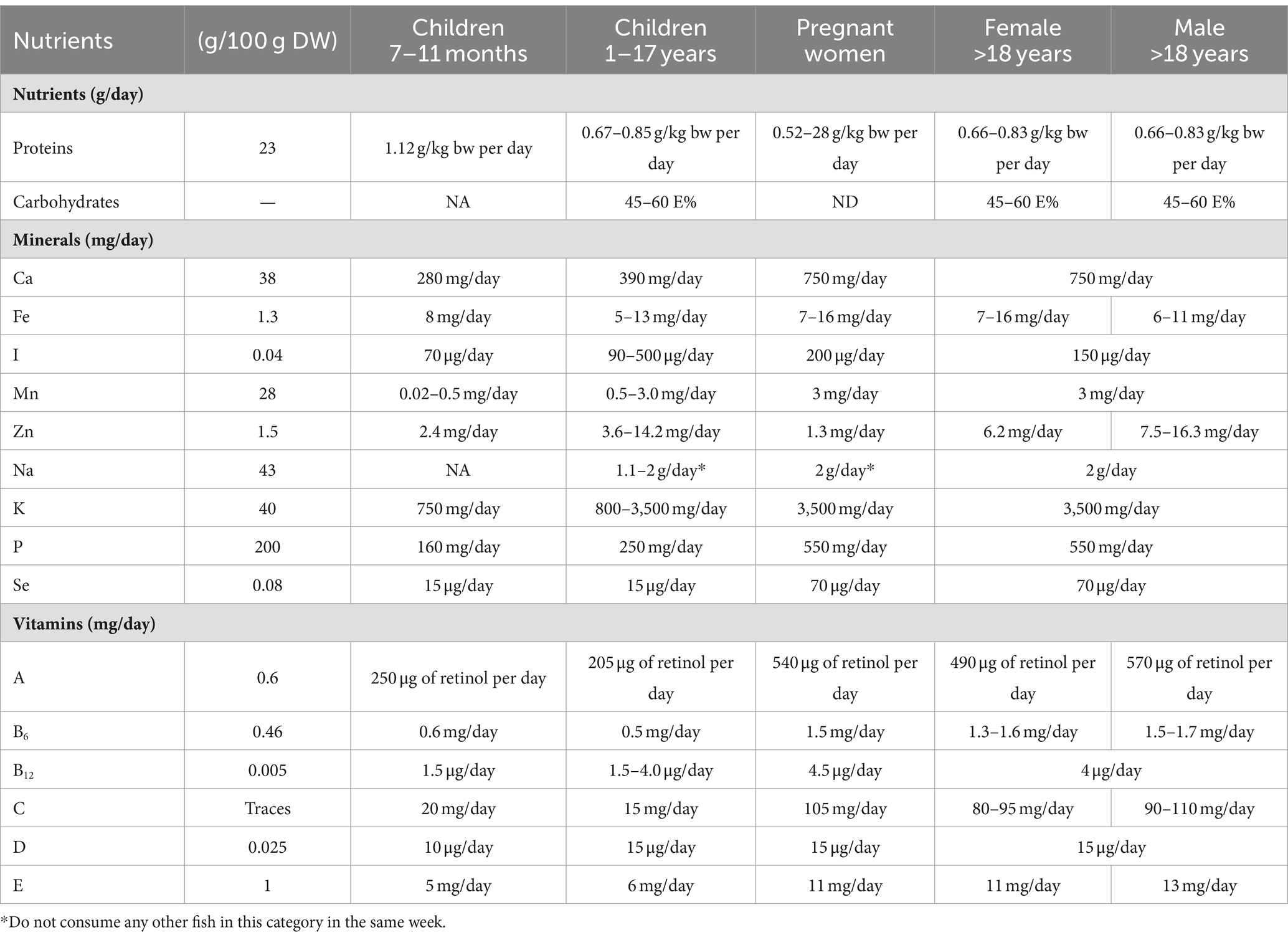
Table 4. Nutrition offered by 100 g of bluefin tuna Thunnus thynnus and the recommended daily value of certain nutrients to several different targeted populations (40–43, 152, 153).
It is important to note that the ingestion of toxic elements studied in different investigations from samples obtained from tuna do not pose any risk to the consumers health. However, regular, or excessive consumption of tuna species could exceed the recommended weekly intake or the lower confidence limit of the reference dose, which does not necessarily pose a significant risk to consumers (149).
4.2 Risk-benefit ratio: toxicological assessment
EFSA has provided risk-benefit assessments of fish consumption based on scientific resources that expose the beneficial effects of fish intake and the possible risks associated with some contaminants such as Hg or methylmercury (MeHg) (23, 150, 154, 155). In this sense, in 2012 EFSA updated the tolerable weekly intake (TWI) of MeHg, establishing the limit at 1.3 μg/kg of body weight and for inorganic Hg 4 μg/kg of body weight (150). These limits were adopted based on the assessment of different outcomes. Among them, several biomarkers were used to provide precise data for MeHg exposure such as red blood cells, hair, toenail, or fingernail whereas plasma and urine samples were preferred for Hg. Data obtained from in vivo assays based on different experimental animals and epidemiological studies from the Faroe Islands and Seychelles such as the Hg and MeHg toxicity in prenatal neurodevelopment, were also used as reference. To assess dietary exposure, it was assumed that the total content of Hg in fish was 100% as MeHg and a bioavailability in the body of 100%. Subsequently, EFSA made a scientific statement where panel members addressed the benefits of fish consumption, such as those due to the PUFAs content and its capacity to counteract to the risks of MeHg. Considering all this data and factors, EFSA concluded that an intake of 1 to 4 servings per week of fish was associated with beneficial effects in adults with coronary artery disease. In this range of fish consumption, health benefits outweigh risks, especially compared to people who do not consume fish (23). In addition, the EFSA stated that this frequency of consumption (1–4 servings/week) has been associated with a lower risk of mortality from coronary heart disease in adults and is compatible with current intakes and recommendations in most European countries. This statement refers to fish per se and considers the beneficial and adverse effects of nutrients and non-nutrients, including contaminants such as MeHg, which may be present in fish (23). However, in the risk assessment, EFSA considers children under 10 years of age and women during pregnancy, lactation or expecting to get pregnant as sensitive populations to exposure of high levels of Hg or MeHg. Therefore, for these groups, the consumption of fish species with lower amounts of these contaminants is recommended (155). Indeed, various national food safety agencies have issued recommendations to limit the consumption of certain types of fishery products in these susceptible populations. For instance, AESAN recommends avoiding the consumption of swordfish, shark, bluefin tuna, and pike by these previously mentioned susceptible populations (156).
Various authors have pointed out that the risk-benefit assessment should consider the apparent protective effect of some nutrients such as PUFAs and Se against Hg and MeHg (83, 84, 87, 88, 157–159). Regarding the protective effect of PUFAs, DHA seems to protect against oxidative stress induced by MeHg in neuronal cells (160–162). In this sense, a study evaluated the dose-response between maternal fish consumption and the child’s verbal intelligence quotient (IQ). It was found that a maternal intake of 100 mg of DHA per day may prompt a gain of 2.8 points of verbal IQ in 18 months-old children (163). Similarly, other works reported that the continuous consumption of fish by pregnant women led to a laxer relationship between intrauterine exposure to MeHg and children’s IQ (164, 165). In accordance with the Scientific Opinion of EFSA regarding the risks for public health related to the presence of Hg and MeHg, omega 3-LC PUFAs, can counteract the negative effects of exposure to MeHg (150). In this line, the most studied nutrient for protection against MeHg appears to be Se. The bound affinity of Hg and Se is a million times greater than for sulfur in analogous forms. Indeed, several attempts have been made to design products with Hg-detox capacity using Se (e.g., Hg selenide). Possible protective modes of action of Se against MeHg toxicity include antioxidant effects, increased glutathione peroxidase activity, glutathione synthesis, elevated selenoprotein levels, and increased MeHg demethylation (157, 166). In this sense, it is suggested that a molar excess of Se compared to Hg can protect against its toxic effects. This could explain why studies of maternal populations exposed to foods that contain Hg in a molar excess of Se, such as pilot whale meat, have found adverse results in children, while populations exposed to Hg but showing a constant pattern of consumption of sea fish rich in Se showed lesser or none adverse effects (167). Subsequently, a new criterion was proposed to assess the risks of Hg exposure, the Se Health Benefit Value (HBVSe), which simultaneously evaluates Hg exposures and dietary Se intakes, particularly regarding Se consumption during pregnancy (157). Another risk assessment proposal is the benefit-risk value (BRV), this equation attempts to reflect either excess Hg or excess Se, in which case it can be assessed with respect to adequate Se intake. Various studies have shown that benefits outweigh risks when it comes to bluefin tuna consumption, as the molar ratio of Se:Hg oscillates between 1.3 and 20 and always implies a molar excess of Se compared to Hg (Table 5). In addition, HBVSe values are reported to oscillate between 7.9 and 296 (Table 5); therefore, it is likely that the high Se content against Hg prevents the toxicity induced by Hg (88, 176, 178, 179).
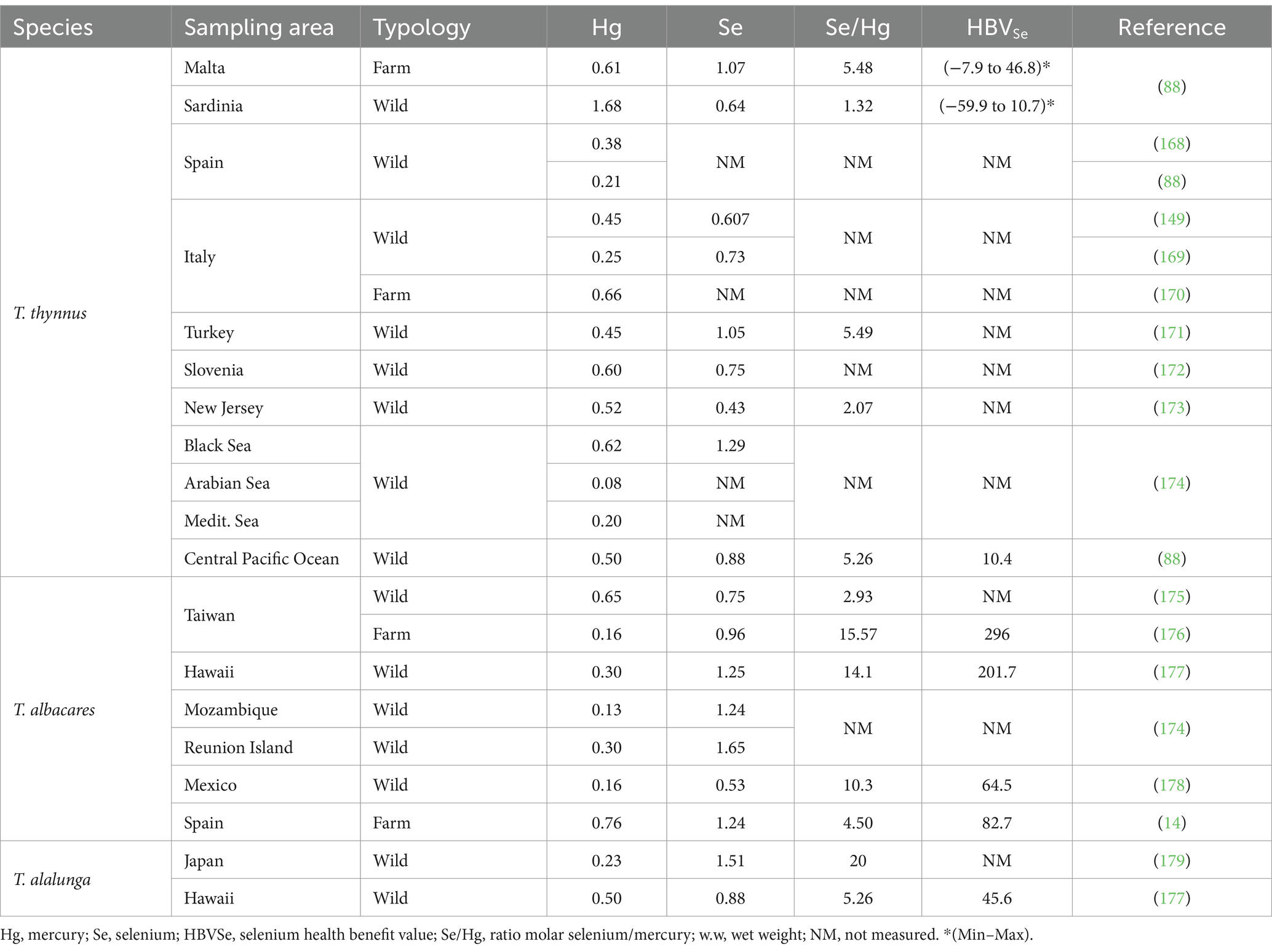
Table 5. Comparison of Hg and Se concentrations (mg kg − 1 w.w.) and relation molar ratios Se/Hg and HBVSe in farmed or wild Thunnus sp. samples.
On the other hand, some authors suggest considering the bioaccessible fraction of Se and Hg to provide a more accurate risk assessment (180–182). In this line in vitro gastrointestinal digestion techniques provide valuable data about the bioaccessibility of Hg and MeHg which can get decreased after cooking to around half of the original concentration (181). This change in bioaccessibility has been attributed to the effect of the temperature in the structural conformation of fish muscle proteins, which may cause loss of native protein structure. These alterations could prevent the access of the enzymes used in in vitro gastrointestinal digestion models to the structures to which Hg is bound such as thiol groups (181). In agreement with these outcomes, another work also found up to 40% reductions in the bioaccessible fraction of Hg in fish after cooking it (183). Therefore, for a more accurate risk assessment, all the criteria mentioned above must be considered Nevertheless, further research in the area is necessary to study the synergistic effects between the different variables, to improve the understanding of the repercussions on health regarding the intake of fish and shellfish.
5 Conclusion
Atlantic bluefin tuna, Thunnus thynnus, is a highly nutritious species rich in high-quality proteins, lipids, fat-soluble vitamins, and various essential elements essential for the proper functioning of the body. Among the nutritional composition, bioactive peptides and the omega-3-polyunsatturated fatty acids EPA and DHA have been linked to beneficial effects. In this sense, several population studies have reported the positive effects of fish consumption on human health, including protection against cardiovascular, neurological, metabolic, and immune diseases and body weight regulation. Besides, consuming this species helps achieve the intake recommendations of several vitamins and minerals. However, some limitations for some vulnerable population groups, such as young children and pregnant women, should be considered due to the presence of contaminants, especially mercury and methylmercury. However, several authors have pointed to high selenium levels’ capacity to counteract the negative effects of these contaminants. Selenium has been suggested to form complexes that reduce the bioaccessibility of mercury and methylmercury and so it would decrease their harmful effects. In this sense, some studies have evaluated this species’ risk-benefit ratio, showing a minimal risk in most cases. Nevertheless, further research and assessments of the risk of tuna consumption is still necessary to provide reliable data and help safeguard the health of humans, especially about the bioaccessibility of heavy metals, toxicity of selenium complexes or deeper evaluation of risk-benefits and exposure. These outcomes would reinforce and increase the current knowledge about Atlantic bluefin tuna consumption safety and try to define more accurate consumption recommendations.
Author contributions
FC: Methodology, Supervision, Validation, Conceptualization, Data curation, Investigation, Writing – original draft, Writing – review & editing. LC: Investigation, Writing – review & editing, Supervision. PG-O: Conceptualization, Methodology, Project administration, Supervision, Validation, Writing – original draft. MB-M: Investigation, Writing – original draft. AJ: Conceptualization, Investigation, Methodology, Writing – original draft. AP: Conceptualization, Investigation, Methodology, Writing – original draft. PO: Writing – original draft, Writing – review & editing. MF-C: Methodology, Supervision, Writing – review & editing. MAP: Writing – review & editing, Supervision. MP: Formal analysis, Funding acquisition, Methodology, Project administration, Resources, Supervision, Validation, Visualization, Writing – review & editing.
Funding
The author(s) declare financial support was received for the research, authorship, and/or publication of this article. The research leading to these results was supported by MICINN supporting the Ramón y Cajal grant for MAP (RYC-2017-22891) that supports the work of PG-O and the Juan de la Cierva Incorporación for Hui Cao (IJC2020-046055-I); by Xunta de Galicia for supporting the program EXCELENCIA-ED431F 2020/12, the post-doctoral grants of MF-C (ED481B-2019/096), and LC (ED481B-2021/152).
Acknowledgments
The authors thank the program Uvigo Project Number-CO-0019-2021 which supports the work of FC. Authors are grateful to the Ibero-American Program on Science and Technology (CYTED—AQUA-CIBUS, P317RT0003), to the Bio Based Industries Joint Undertaking (JU) under grant agreement No. 888003 UP4HEALTH Project (H2020-BBI-JTI-2019) that supports the work of PO.
Conflict of interest
The authors declare that the research was conducted in the absence of any commercial or financial relationships that could be construed as a potential conflict of interest.
The author(s) declared that they were an editorial board member of Frontiers, at the time of submission. This had no impact on the peer review process and the final decision.
Publisher’s note
All claims expressed in this article are solely those of the authors and do not necessarily represent those of their affiliated organizations, or those of the publisher, the editors and the reviewers. Any product that may be evaluated in this article, or claim that may be made by its manufacturer, is not guaranteed or endorsed by the publisher.
References
1. Kumar, V, Sinha, AK, Uka, A, Antonacci, A, Scognamiglio, V, Mazzaracchio, V, et al. Multi-potential biomarkers for seafood quality assessment: global wide implication for human health monitoring. Trends Anal Chem. (2020) 132:116056. doi: 10.1016/j.trac.2020.116056
2. Tilami, SK, Sampels, S, Zajíc, T, Krejsa, J, Másílko, J, and Mráz, J. Nutritional value of several commercially important river fish species from the Czech Republic. PeerJ. (2018) 6:e5729–16. doi: 10.7717/peerj.5729
3. Smida, MAB, Marzouk, B, and El Cafsi, M. The composition of fatty acids in the tissues of Tunisian swordfish (Xiphias gladius). Food Chem. (2009) 115:522–8. doi: 10.1016/j.foodchem.2008.12.084
4. Garaffo, MA, Vassallo-Agius, R, Nengas, Y, Lembo, E, Rando, R, Maisano, R, et al. Fatty acids profile, atherogenic (IA) and thrombogenic (IT) health lipid indices, of raw roe of blue fin tuna (Thunnus thynnus L.) and their salted product bottarga. Food Nutr Sci. (2011) 2:736–43. doi: 10.4236/fns.2011.27101
5. Ferdosh, S, Sarker, ZI, Norulaini, N, Oliveira, A, Yunus, K, Chowdury, AJ, et al. Quality of tuna fish oils extracted from processing the by-products of three species of neritic tuna using supercritical carbon dioxide. J Food Process Preserv. (2015) 39:432–41. doi: 10.1111/jfpp.12248
6. Weichselbaum, E, Coe, S, Buttriss, J, and Stanner, S. Fish in the diet: a review. Nutr Bull. (2013) 38:128–77. doi: 10.1111/nbu.12021
7. Swanson, D, Block, R, and Mousa, SA. Omega-3 fatty acids EPA and DHA: health benefits throughout life. Adv Nutr. (2012) 3:1–7. doi: 10.3945/an.111.000893
8. Calder, PC. Omega-3 fatty acids and inflammatory processes. Nutrients. (2010) 2:355–74. doi: 10.3390/nu2030355
9. Mohanty, BP, Mahanty, A, Ganguly, S, Mitra, T, Karunakaran, D, and Anandan, R. Nutritional composition of food fishes and their importance in providing food and nutritional security. Food Chem. (2019) 293:561–70. doi: 10.1016/j.foodchem.2017.11.039
10. Khalili Tilami, S, and Sampels, S. Nutritional value of fish: lipids, proteins, vitamins, and minerals. Rev Fish Sci Aquac. (2018) 26:243–53. doi: 10.1080/23308249.2017.1399104
11. Butz, AM, von Christopher, S, and Bartheld, JB. Getting the most out of pubchemfor virtual screening HHS public access. Physiol Behav. (2017) 176:139–48.
12. Horning, KJ, Caito, SW, Tipps, KG, Bowman, AB, and Aschner, M. Manganese is essential for neuronal health. Annu Rev Nutr. (2015) 35:71–108. doi: 10.1146/annurev-nutr-071714-034419
13. Maywald, M, Wessels, I, and Rink, L. Zinc signals and immunity. Int J Mol Sci. (2017) 18:2222. doi: 10.3390/ijms18102222
14. Melgar, MJ, Núñez, R, and García, MÁ. Selenium intake from tuna in Galicia (Spain): health risk assessment and protective role against exposure to mercury and inorganic arsenic. Sci Total Environ. (2019) 694:133716. doi: 10.1016/j.scitotenv.2019.133716
15. Taylor, CM, Emmett, PM, Emond, AM, and Golding, J. A review of guidance on fish consumption in pregnancy: is it fit for purpose? Public Health Nutr. (2018) 21:2149–59. doi: 10.1017/S1368980018000599
16. Jinadasa, BKKK, Edirisinghe, EMRKB, and Wickramasinghe, I. Total mercury content, weight and length relationship in swordfish (Xiphias gladius) in Sri Lanka. Food Addit Contam B. (2013) 6:244–8. doi: 10.1080/19393210.2013.807521
17. Biton-Porsmoguer, S, Bănaru, D, Boudouresque, CF, Dekeyser, I, Bouchoucha, M, Marco-Miralles, F, et al. Mercury in blue shark (Prionace glauca) and shortfin mako (Isurus oxyrinchus) from north-eastern Atlantic: implication for fishery management. Mar Pollut Bull. (2018) 127:131–8. doi: 10.1016/j.marpolbul.2017.12.006
18. Belmonte, A, Muñoz, P, Santos-Echeandía, J, and Romero, D. Tissue distribution of mercury and its relationship with selenium in Atlantic bluefin tuna (Thunnus thynnus l.). Int J Environ Res Public Health. (2021) 18:13376. doi: 10.3390/ijerph182413376
19. Hosseini, M, Nabavi, SMB, and Parsa, Y. Bioaccumulation of trace mercury in trophic levels of benthic, benthopelagic, pelagic fish species, and sea birds from Arvand River, Iran. Biol Trace Elem Res. (2013) 156:175–80. doi: 10.1007/s12011-013-9841-2
20. Harding, G, Dalziel, J, and Vass, P. Bioaccumulation of methylmercury within the marine food web of the outer Bay of Fundy, Gulf of Maine. PLoS One. (2018) 13:e0197220. doi: 10.1371/journal.pone.0197220
21. Kuras, R, Janasik, B, Stanislawska, M, Kozlowska, L, and Wasowicz, W. Assessment of mercury intake from fish meals based on intervention research in the Polish subpopulation. Biol Trace Elem Res. (2017) 179:23–31. doi: 10.1007/s12011-017-0939-9
22. Anual, ZF, Maher, W, Krikowa, F, Hakim, L, Ahmad, NI, and Foster, S. Mercury and risk assessment from consumption of crustaceans, cephalopods and fish from West Peninsular Malaysia. Microchem J. (2018) 140:214–21. doi: 10.1016/j.microc.2018.04.024
23. EFSA Dietetic Products, Nutrition, and Allergies (NDA). Scientific opinion on health benefits of seafood (fish and shellfish) consumption in relation to health risks associated with exposure to methylmercury. EFSA J. (2014) 12:3761. doi: 10.2903/j.efsa.2014.3761
24. European Union. Commission Regulation (EC) No. 1881/2006 setting maximum levels for certain contaminants in foodstuffs. Off J Eur Union. (2006) 364:5–24.
25. Choi, AL, Budtz-Jørgensen, E, Jørgensen, PJ, Steuerwald, U, Debes, F, Weihe, P, et al. Selenium as a potential protective factor against mercury developmental neurotoxicity. Environ Res. (2009) 107:45–52. doi: 10.1016/j.envres.2007.07.006.Selenium
26. Park, K, and Mozaffarian, D. Omega-3 fatty acids, mercury, and selenium in fish and the risk of cardiovascular diseases. Curr Atheroscler Rep. (2010) 12:414–22. doi: 10.1007/s11883-010-0138-z
27. Graham, JB, and Dickson, KA. Tuna comparative physiology. J Exp Biol. (2004) 207:4015–24. doi: 10.1242/jeb.01267
28. Fromentin, JM, and Powers, JE. Atlantic bluefin tuna: population dynamics, ecology, fisheries and management. Fish Fish. (2005) 6:281–306. doi: 10.1111/j.1467-2979.2005.00197.x
29. Mather, FJ III, Mason, JM Jr, and Jones, AC. Historical document: life history and fisheries of Atlantic bluefin tuna In: NOAA Technical Memorandum NMFS-SEFSC-370. Miami, FL: U.S. Department of Commerce, National Oceanic and Atmospheric Administration, National Marine Fisheries Service, Southeast Fisheries Science Center (1995)
30. Clark, B, Clausen, R, and Longo, SB. The tragedy of the commodity: oceans, fisheries, and aquaculture. USA: Rutgers University Press. (2015). 274 p.
31. Marisaldi, L, Iorillo, O, Basili, D, Gioacchini, G, Bobe, J, Thermes, V, et al. A comparison of reproductive performances in young and old females: a case study on the Atlantic bluefin tuna in the mediterranean sea. Animals. (2021) 11:3340. doi: 10.3390/ani11123340
32. Betancor, MB, Ortega, A, de la Gándara, F, Tocher, DR, and Mourente, G. Performance, feed utilization, and hepatic metabolic response of weaned juvenile Atlantic bluefin tuna (Thunnus thynnus L.): effects of dietary lipid level and source. Fish Physiol Biochem. (2019) 45:697–718. doi: 10.1007/s10695-018-0587-9
33. European Commission, Directorate-General for Maritime Affairs and Fisheries. The EU fish market—2019 edition. Belgium: Publications Office of the European Union (2019).
34. Irina, P. Bluefin tuna in the eastern Atlantic and the Mediterranean. Belgium: European Parliament (2020).
35. Cutajar, N, Lia, F, Deidun, A, Galdies, J, Arizza, V, and Zammit, MM. Turning waste into a resource: isolation and characterization of high-quality collagen and oils from Atlantic bluefin tuna discards. Appl Sci. (2022) 12:1542. doi: 10.3390/app12031542
36. Otero, P, Carpena, M, Fraga-Corral, M, Garcia-Oliveira, P, Soria-Lopez, A, Barba, F, et al. Aquaculture and agriculture-by products as sustainable sources of omega-3 fatty acids in the food industry. eFood. (2022) 2:209–33. doi: 10.53365/efood.k/144603
37. Ruxton, CHS. The benefits of fish consumption. Nutr Bull. (2011) 36:6–19. doi: 10.1111/j.1467-3010.2010.01869.x
38. Li, N, Wu, X, Zhuang, W, Xia, L, Chen, Y, Wu, C, et al. Fish consumption and multiple health outcomes: umbrella review. Trends Food Sci Technol. (2020) 99:273–83. doi: 10.1016/j.tifs.2020.02.033
39. Percin, F, Sogut, O, Altinelataman, C, and Soylak, M. Some trace elements in front and rear dorsal ordinary muscles of wild and farmed bluefin tuna (Thunnus thynnus L. 1758) in the Turkish part of the eastern Mediterranean Sea. Food Chem Toxicol. (2011) 49:1006–10. doi: 10.1016/j.fct.2011.01.007
40. Hermida, TA. Guía de las cualidades nutricionales de los productos procedentes de la pesca extractiva y de la acuicultura: Binomio riesgo-beneficio. Spain: Ministerio de Agricultura, Alimentación y Medio Ambiente (2012). 64 p.
41. Sprague, M, Dick, JR, Medina, A, Tocher, DR, Bell, JG, and Mourente, G. Lipid and fatty acid composition, and persistent organic pollutant levels in tissues of migrating Atlantic bluefin tuna (Thunnus thynnus, L.) broodstock. Environ Pollut. (2012) 171:61–71. doi: 10.1016/j.envpol.2012.07.021
42. Seguridad Alimentaria/Gestión de Riesgos/Seguridad Nutricional/Información alimentaria facilitada al consumidor Reglamento 1169/2011 sobre la información alimentaria facilitada al consumidor (2011) 18–63. Available at: https://www.aesan.gob.es/AECOSAN/web/seguridad_alimentaria/subdetalle/futura_legislacion.htm
43. Olga, M, Carbajal, A, Cabrera, L, and Cuadrado, C. Tablas de composicion de alimentos. 19th ed. Spain: Piramide Ediciones. (2018).
44. Chiesa, G, Busnelli, M, Manzini, S, and Parolini, C. Nutraceuticals and bioactive components from fish for dyslipidemia and cardiovascular risk reduction. Mar Drugs. (2016) 14:113. doi: 10.3390/md14060113
45. Ricardo Aguilar, P. (2008). Estudio sobre larvas de atún rojo. Campaña OCEANA-MarViva Mediterraneo. 1–76.
46. Yerlikaya, P, Gokoglu, N, Topuz, OK, and Gokoglu, M. Changes in the proximate composition of bluefin tuna (Thunnus thynnus) reared in the cages located on the Gulf of Antalya (Turkey’s Western Mediterranean coast) during the fattening period. Aquac Res. (2009) 40:1731–4. doi: 10.1111/j.1365-2109.2009.02276.x
47. Topic Popovic, N, Kozacinski, L, Strunjak-Perovic, I, Coz-Rakovac, R, Jadan, M, Cvrtila-Fleck, Z, et al. Fatty acid and proximate composition of bluefin tuna (Thunnus thynnus) muscle with regard to plasma lipids. Aquac Res. (2012) 43:722–9. doi: 10.1111/j.1365-2109.2011.02880.x
48. Wergedahl, H, Liaset, B, Gudbrandsen, OA, Lied, E, Espe, M, Muna, Z, et al. Fish protein hydrolysate reduces plasma total cholesterol, increases the proportion of HDL cholesterol, and lowers acyl-CoA:cholesterol acyltransferase activity in liver of Zucker rats. J Nutr. (2004) 134:1320–7. doi: 10.1093/jn/134.6.1320
49. Qian, ZJ, Je, JY, and Kim, SK. Antihypertensive effect of angiotensin I converting enzyme-inhibitory peptide from hydrolysates of bigeye tuna dark muscle, Thunnus obesus. J Agric Food Chem. (2007) 55:8398–403. doi: 10.1021/jf0710635
50. Unnikrishnan, P, Kizhakkethil, BP, George, JC, Aliyamveetil Abubacker, Z, Ninan, G, and Chandragiri, NR. Antioxidant peptides from dark meat of yellowfin tuna (Thunnus albacares): process optimization and characterization. Waste Biomass Valorization. (2021) 12:1845–60. doi: 10.1007/s12649-020-01129-8
51. Vijaykrishnaraj, M, and Prabhasankar, P. Marine protein hydrolysates: their present and future perspectives in food chemistry-a review. RSC Adv. (2015) 5:34864–77. doi: 10.1039/c4ra17205a
52. Bandyopadhyay, S, Kashyap, S, Calvez, J, Devi, S, Azzout-Marniche, D, Tomé, D, et al. Evaluation of protein quality in humans and insights on stable isotope approaches to measure digestibility—a review. Adv Nutr. (2021) 13:1131–43. doi: 10.1093/advances/nmab134
53. López, MMS, Kizlansky, A, and López, LB. Evaluación de la calidad de las proteínas en los alimentos calculando el escore de aminoácidos corregido por digestibilidad. Nutr Hosp. (2006) 21:47–51.
54. Centre for Genomic Pathogen Surveillance. Dietary protein quality evaluation in human nutrition: report of an FAO expert consultation, 31 March–2 April, 2011. Auckland, New Zealand: Food and Agriculture Organization of the United Nations (2020). 64 p.
55. Holt, LE Jr, and Snyderman, SE. Protein and amino acid requirements of infants and children. Nutr Abstr Rev. (2007) 35:1–13.
56. EFSA Panel on Dietetic Products, Nutrition and Allergies (NDA). Scientific opinion on dietary reference values for protein. EFSA J. (2014) 12:3759. doi: 10.2903/j.efsa.2014.3759
57. FAO Expert Consultation. Dietary protein quality evaluation in human nutrition In: Report of an FAO Expert Consultation. Rome: Food and Agriculture Organization of the United Nations (2013). 1–66.
58. FDA. Daily value and percent daily value: changes on the new nutrition and supplement facts labels. Daily value vs. % daily value. USA: FDA. (2022) 1–6.
59. Holeček, M. Histidine in health and disease: metabolism, physiological importance, and use as a supplement Milan. Nutrients. (2020) 12:848. doi: 10.3390/nu12030848
60. Nie, C, He, T, Zhang, W, Zhang, G, and Ma, X. Branched chain amino acids: beyond nutrition metabolism. Int J Mol Sci. (2018) 19:954. doi: 10.3390/ijms19040954
61. Akram, M, Daniyal, M, Ali, A, Zainab, R, Muhammad Ali Shah, S, Munir, N, et al. Role of phenylalanine and its metabolites in health and neurological disorders In: Synucleins—biochemistry and role in diseases. Pakistan: IntechOpen (2020). 1–13.
62. Kałużna-Czaplińska, J, Gątarek, P, Chirumbolo, S, Chartrand, MS, and Bjørklund, G. How important is tryptophan in human health? Crit Rev Food Sci Nutr. (2019) 59:72–88. doi: 10.1080/10408398.2017.1357534
63. Siriwardhana, N, Kalupahana, NS, and Moustaid-Moussa, N. Health benefits of n−3 polyunsaturated fatty acids: eicosapentaenoic acid and docosahexaenoic acid. Adv Food Nutr Res. (2012) 65:211–22. doi: 10.1016/B978-0-12-416003-3.00013-5
64. Truzzi, C, Annibaldi, A, Illuminati, S, Antonucci, M, Api, M, Scarponi, G, et al. Characterization of the fatty acid composition in cultivated Atlantic bluefin tuna (Thunnus thynnus L.) muscle by gas chromatography-mass spectrometry. Anal Lett. (2018) 51:2981–93. doi: 10.1080/00032719.2018.1467433
65. Özden, Ö, Erkan, N, Kaplan, M, and Karakulak, FS. Toxic metals and omega-3 fatty acids of bluefin tuna from aquaculture: health risk and benefits. Expo Health. (2020) 12:9–18. doi: 10.1007/s12403-018-0279-9
66. Vilavert, L, Borrell, F, Nadal, M, Jacobs, S, Minnens, F, Verbeke, W, et al. Health risk/benefit information for consumers of fish and shellfish: FishChoice, a new online tool. Food Chem Toxicol. (2017) 104:79–84. doi: 10.1016/j.fct.2017.02.004
67. FAO Food and Nutrition Paper. Fats and fatty acids in human nutrition. Report of an expert consultation. (2010). 1–166
68. EFSA Panel on Dietetic Products, Nutrition, and Allergies (NDA). Scientific opinion on dietary reference values for fats including saturated fatty acids, polyunsaturated fatty acids, monounsaturated fatty acids, trans fatty acids, and cholesterol. EFSA J. (2010) 8:1461. doi: 10.2903/j.efsa.2010.1461
69. Vannice, G, and Rasmussen, H. Position of the academy of nutrition and dietetics: dietary fatty acids for healthy adults. J Acad Nutr Diet. (2014) 114:136–53. doi: 10.1016/j.jand.2013.11.001
70. Reiner, Ž, Catapano, AL, De Backer, G, Graham, I, Taskinen, MR, Wiklund, O, et al. ESC/EAS Guidelines for the management of dyslipidaemias: the Task Force for the management of dyslipidaemias of the European Society of Cardiology (ESC) and the European Atherosclerosis Society (EAS). Eur Heart J. (2011) 32:1769–818. doi: 10.1093/eurheartj/ehr158
71. Shahidi, F, and Ambigaipalan, P. Omega-3 polyunsaturated fatty acids and their health benefits. Annu Rev Food Sci Technol. (2018) 9:345–81. doi: 10.1146/annurev-food-111317-095850
72. Saini, RK, and Keum, YS. Omega-3 and omega-6 polyunsaturated fatty acids: dietary sources, metabolism, and significance—a review. Life Sci. (2018) 203:255–67. doi: 10.1016/j.lfs.2018.04.049
73. Betancor, MB, Sprague, M, Ortega, A, de la Gándara, F, Tocher, DR, Ruth, R, et al. Central and peripheral clocks in Atlantic bluefin tuna (Thunnus thynnus, L.): daily rhythmicity of hepatic lipid metabolism and digestive genes. Aquaculture. (2020) 523:735220. doi: 10.1016/J.AQUACULTURE.2020.735220
74. Goltzman, D. Functions of vitamin D in bone. Histochem Cell Biol. (2018) 149:305–12. doi: 10.1007/s00418-018-1648-y
75. Cantorna, MT, Snyder, L, and Arora, J. Vitamin A and vitamin D regulate the microbial complexity, barrier function, and the mucosal immune responses to ensure intestinal homeostasis. Crit Rev Biochem Mol Biol. (2019) 54:184–92. doi: 10.1080/10409238.2019.1611734
76. Sassi, F, Tamone, C, and D’Amelio, P. Vitamin D: nutrient, hormone, and immunomodulator. Nutrients. (2018) 10:1656. doi: 10.3390/nu10111656
77. Miyazawa, T, Burdeos, GC, Itaya, M, Nakagawa, K, and Miyazawa, T. Vitamin E: regulatory redox interactions. IUBMB Life. (2019) 71:430–41. doi: 10.1002/iub.2008
78. Quintaes, KD, and Diez-Garcia, RW. The importance of minerals in the human diet In: Handbook of mineral elements in food. Spain: Willey Blackwell Editions. (2015). 1–21.
79. Romani, AMP. Magnesium in health and disease. Met Ions Life Sci. (2013) 13:49–79. doi: 10.1007/978-94-007-7500-8_3
80. Fiorentini, D, Cappadone, C, Farruggia, G, and Prata, C. Magnesium: Biochemistry, Nutrition, Detection, and Social Impact of Diseases Linked to Its Deficiency. Nutrients. (2021) 13:1136. doi: 10.3390/nu13041136
81. Kieliszek, M. Selenium-fascinating microelement, properties and sources in food. Molecules. (2019) 24:1298. doi: 10.3390/molecules24071298
82. Gać, P, Czerwińska, K, Macek, P, Jaremków, A, Mazur, G, Pawlas, K, et al. The importance of selenium and zinc deficiency in cardiovascular disorders. Environ Toxicol Pharmacol. (2021) 82:103553. doi: 10.1016/j.etap.2020.103553
83. Ralston, NVC, and Raymond, LJ. Selenium status and intake influences mercury exposure risk assessments In: Selenium in the environment and human health. London: Taylor and Francis Group (2014). 203–5.
84. Ralston, NVC, Blackwell, JL, and Raymond, LJ. Importance of molar ratios in selenium-dependent protection against methylmercury toxicity. Biol Trace Elem Res. (2007) 119:255–68. doi: 10.1007/s12011-007-8005-7
85. Gochfeld, M, and Burger, J. Mercury interactions with selenium and sulfur and the relevance of the Se:Hg molar ratio to fish consumption advice. Environ Sci Pollut Res. (2021) 28:18407–20. doi: 10.1007/s11356-021-12361-7
86. Cabañero, AI, Madrid, Y, and Cámara, C. Mercury-selenium species ratio in representative fish samples and their bioaccessibility by an in vitro digestion method. Biol Trace Elem Res. (2007) 119:195–211. doi: 10.1007/s12011-007-8007-5
87. Li, X, Yin, D, Yin, J, Chen, Q, and Wang, R. Dietary selenium protect against redox-mediated immune suppression induced by methylmercury exposure. Food Chem Toxicol. (2014) 72:169–77. doi: 10.1016/j.fct.2014.07.023
88. Annibaldi, A, Truzzi, C, Carnevali, O, Pignalosa, P, Api, M, Scarponi, G, et al. Determination of hg in farmed and wild Atlantic bluefin tuna (Thunnus thynnus L.) muscle. Molecules. (2019) 24:1273. doi: 10.3390/molecules24071273
89. Wan, Y, Zheng, J, Wang, F, and Li, D. Fish, long chain omega-3 polyunsaturated fatty acids consumption, and risk of all-cause mortality: a systematic review and dose-response meta-analysis from 23 independent prospective cohort studies. Asia Pac J Clin Nutr. (2017) 26:939–56. doi: 10.6133/apjcn.072017.01
90. He, K, Rimm, EB, Merchant, A, Rosner, BA, Stampfer, MJ, Willett, WC, et al. Fish consumption and risk of stroke in men. JAMA. (2002) 7:3130–6. doi: 10.1001/jama.288.24.3130
91. Iso, H, Rexrode, KM, Stampfer, MJ, Manson, JAE, Colditz, GA, Speizer, FE, et al. Intake of fish and omega-3 fatty acids and risk of stroke in women. JAMA. (2001) 285:304–12. doi: 10.1001/jama.285.3.304
92. Takata, Y, Zhang, X, Li, H, Gao, YT, Yang, G, Gao, J, et al. Fish intake and risks of total and cause-specific mortality in 2 population-based cohort studies of 134,296 men and women. Am J Epidemiol. (2013) 178:46–57. doi: 10.1093/aje/kws584
93. Micallef, M, Munro, I, Phang, M, and Garg, M. Plasma n−3 polyunsaturated fatty acids are negatively associated with obesity. Br J Nutr. (2009) 102:1370–4. doi: 10.1017/S0007114509382173
94. Villegas, R, Xiang, YB, Elasy, T, Li, HL, Yang, G, Cai, H, et al. Fish, shellfish, and long-chain n−3 fatty acid consumption and risk of incident type 2 diabetes in middle-aged Chinese men and women. Am J Clin Nutr. (2011) 94:543–51. doi: 10.3945/ajcn.111.013193
95. Chavarro, JE, Stampfer, MJ, Li, H, Campos, H, Kurth, T, and Ma, J. A prospective study of polyunsaturated fatty acid levels in blood and prostate cancer risk. Cancer Epidemiol Biomarkers Prev. (2007) 16:1364–70. doi: 10.1158/1055-9965.EPI-06-1033
96. Paoli, A, Moro, T, Bosco, G, Bianco, A, Grimaldi, KA, Camporesi, E, et al. Effects of n−3 polyunsaturated fatty acids (ω−3) supplementation on some cardiovascular risk factors with a ketogenic mediterranean diet. Mar Drugs. (2015) 13:996–1009. doi: 10.3390/md13020996
97. World Health Organization. Cardiovascular diseases (CVDs). (2021). Available at: https://www.who.int/news-room/fact-sheets/detail/cardiovascular-diseases-(cvds)
98. Zhang, B, Xiong, K, Cai, J, and Ma, A. Fish consumption and coronary heart disease: a meta-analysis. Nutrients. (2020) 12:2278. doi: 10.3390/nu12082278
99. Jayedi, A, Zargar, MS, and Shab-Bidar, S. Fish consumption and risk of myocardial infarction: a systematic review and dose-response meta-analysis suggests a regional difference. Nutr Res. (2019) 62:1–12. doi: 10.1016/j.nutres.2018.10.009
100. Liao, J, Xiong, Q, Yin, Y, Ling, Z, and Chen, S. The effects of fish oil on cardiovascular diseases: systematical evaluation and recent advance. Front Cardiovasc Med. (2022) 8:802306. doi: 10.3389/fcvm.2021.802306
101. Thomas, A, Crivello, F, Mazoyer, B, Debette, S, Tzourio, C, and Samieri, C. Fish intake and MRI burden of cerebrovascular disease in older adults. Neurology. (2021) 97:e2213–22. doi: 10.1212/WNL.0000000000012916
102. Jayedi, A, Shab-Bidar, S, Eimeri, S, and Djafarian, K. Fish consumption and risk of all-cause and cardiovascular mortality: a dose–response meta-analysis of prospective observational studies. Public Health Nutr. (2018) 21:1297–306. doi: 10.1017/S1368980017003834
103. Zhao, W, Tang, H, Yang, X, Luo, X, Wang, X, Shao, C, et al. Fish consumption and stroke risk: a meta-analysis of prospective cohort studies. J Stroke Cerebrovasc Dis. (2019) 28:604–11. doi: 10.1016/j.jstrokecerebrovasdis.2018.10.036
104. Yang, B, Ren, X, Li, Z, Shi, M, Ding, F, Su, K-P, et al. Lowering effects of fish oil supplementation on proinflammatory markers in hypertension: results from a randomized controlled trial. Food Funct. (2020) 11:1779–89. doi: 10.1039/C9FO03085A
105. Oppedisano, F, Macrì, R, Gliozzi, M, Musolino, V, Carresi, C, Maiuolo, J, et al. The anti-inflammatory and antioxidant properties of n−3 PUFAs: their role in cardiovascular protection. Biomedicines. (2020) 8:306. doi: 10.3390/biomedicines8090306
106. Jayedi, A, and Shab-Bidar, S. Fish consumption and the risk of chronic disease: an umbrella review of meta-analyses of prospective cohort studies. Adv Nutr. (2020) 11:1123–33. doi: 10.1093/advances/nmaa029
107. Fotuhi, M, Mohassel, P, and Yaffe, K. Fish consumption, long-chain omega-3 fatty acids and risk of cognitive decline or Alzheimer disease: a complex association. Nat Rev Neurol. (2009) 5:140–52. doi: 10.1038/ncpneuro1044
108. Suvarna, V, and Singh, V. DHA A Key supplement for neurologic health—an insight In: Omega fatty acids in brain and neurological health. India: Elsevier (2019). 409–19.
109. Balakrishnan, J, Kannan, S, and Govindasamy, A. Structured form of DHA prevents neurodegenerative disorders: A better insight into the pathophysiology and the mechanism of DHA transport to the brain. Nutr Res. (2021) 85:119–34. doi: 10.1016/j.nutres.2020.12.003
110. Yurko-Mauro, K, Alexander, DD, and Van Elswyk, ME. Docosahexaenoic acid and adult memory: a systematic review and meta-analysis. PLoS One. (2015) 10:e0120391. doi: 10.1371/journal.pone.0120391
111. Sánchez-Villegas, A, Álvarez-Pérez, J, Toledo, E, Salas-Salvadó, J, Ortega-Azorín, C, Zomeño, M, et al. Seafood consumption, omega-3 fatty acids intake, and life-time prevalence of depression in the PREDIMED-plus trial. Nutrients. (2018) 10:2000. doi: 10.3390/nu10122000
112. Parletta, N, Zarnowiecki, D, Cho, J, Wilson, A, Bogomolova, S, Villani, A, et al. A Mediterranean-style dietary intervention supplemented with fish oil improves diet quality and mental health in people with depression: a randomized controlled trial (HELFIMED). Nutr Neurosci. (2019) 22:474–87. doi: 10.1080/1028415X.2017.1411320
113. Zaribaf, F, Falahi, E, Barak, F, Heidari, M, Keshteli, AH, Yazdannik, A, et al. Fish consumption is inversely associated with the metabolic syndrome. Eur J Clin Nutr. (2014) 68:474–80. doi: 10.1038/ejcn.2014.5
114. Lorente-Cebrián, S, Costa, AGV, Navas-Carretero, S, Zabala, M, Martínez, JA, and Moreno-Aliaga, MJ. Role of omega-3 fatty acids in obesity, metabolic syndrome, and cardiovascular diseases: a review of the evidence. J Physiol Biochem. (2013) 69:633–51. doi: 10.1007/s13105-013-0265-4
115. Tørris, C, Molin, M, and Cvancarova, SM. Associations between fish consumption and metabolic syndrome. A large cross-sectional study from the Norwegian Tromsø study: Tromsø 4. Diabetol Metab Syndr. (2016) 8:18. doi: 10.1186/s13098-016-0137-5
116. Scorletti, E, and Byrne, CD. Omega-3 fatty acids and non-alcoholic fatty liver disease: evidence of efficacy and mechanism of action. Mol Asp Med. (2018) 64:135–46. doi: 10.1016/j.mam.2018.03.001
117. Albracht-Schulte, K, Kalupahana, NS, Ramalingam, L, Wang, S, Rahman, SM, Robert-McComb, J, et al. Omega-3 fatty acids in obesity and metabolic syndrome: a mechanistic update. J Nutr Biochem. (2018) 58:1–16. doi: 10.1016/j.jnutbio.2018.02.012
118. Belchior, T, Paschoal, VA, Magdalon, J, Chimin, P, Farias, TM, Chaves-Filho, AB, et al. Omega-3 fatty acids protect from diet-induced obesity, glucose intolerance, and adipose tissue inflammation through PPARγ-dependent and PPARγ-independent actions. Mol Nutr Food Res. (2015) 59:957–67. doi: 10.1002/mnfr.201400914
119. Calder, PC. Polyunsaturated fatty acids, inflammation, and immunity. Lipids. (2001) 36:1007–24. doi: 10.1007/s11745-001-0812-7
120. Grimble, RF. Dietary lipids and the inflammatory response. Proc Nutr Soc. (1998) 57:535–42. doi: 10.1079/pns19980078
121. Calder, PC, and Grimble, R. Omega 3 polyunsaturated fatty acids, inflammation and immunity. Eur J Clin Nutr. (2002) 56:14–9. doi: 10.1159/000059774
122. Calder, PC. Omega-3 fatty acids and inflammatory processes: from molecules to man. Biochem Soc Trans. (2017) 45:1105–15. doi: 10.1042/BST20160474
123. Gutiérrez, S, Svahn, SL, and Johansson, ME. Effects of omega-3 fatty acids on immune cells. Int J Mol Sci. (2019) 20:5028. doi: 10.3390/ijms20205028
124. Yaqoob, P, Pala, HS, Cortina-Borja, M, Newsholme, EA, and Calder, PC. Encapsultated fish oil enriched in α-tocopherol alters plasma phospholipid and mononuclear cell fatty acid compositions but not mononuclear cell functions. Eur J Clin Investig. (2000) 30:260–74. doi: 10.1046/j.1365-2362.2000.00623.x
125. Grimble, RF, and Grimble, GK. Immunonutrition: role of sulfur amino acids, related amino acids, and polyamines. Nutrition. (1998) 14:605–10. doi: 10.1016/S0899-9007(98)80041-5
126. Calder, P. n−3 fatty acids and mononuclear phagocyte function In: Medicinal fatty acids in inflammation. Progress in inflammation research. Basel: Birkhäuser (1998). 1–27.
127. Calder, PC. Dietary fatty acids and lymphocyte functions. Proc Nutr Soc. (1998) 57:487–502. doi: 10.1079/PNS19980073
128. Gadek, JE, DeMichele, SJ, Karlstad, MD, Pacht, ER, Donahoe, M, Albertson, TE, et al. Effect of enteral feeding with eicosapentaenoic acid, gamma-linolenic acid, and antioxidants in patients with acute respiratory distress syndrome. Crit Care Med. (1999) 27:1409–20. doi: 10.1097/00003246-199908000-00001
129. Gianotti, L, Braga, M, Fortis, C, Soldini, L, Vignali, A, Colombo, S, et al. A prospective, randomized clinical trial on perioperative feeding with an arginine‐, omega‐3 fatty acid‐, and RNA‐enriched enteral diet: effect on host response and nutritional status. JPEN J Parenter Enteral Nutr. (2015) 23:314–20. doi: 10.1177/0148607199023006314
130. Wachtler, P, Konig, W, Senkal, M, Kemen, M, and Koller, M. Influence of a total parenteral nutrition enriched with omega-3 fatty acids on leukotriene synthesis of peripheral leukocytes and systemic cytokine levels in patients with major surgery. J Trauma Inj Infect Crit Care. (1997) 42:191–8. doi: 10.1097/00005373-199702000-00004
131. Rees, D, Miles, EA, Banerjee, T, Wells, SJ, Roynette, CE, Wahle, KWJ, et al. Dose-related effects of eicosapentaenoic acid on innate immune function in healthy humans: a comparison of young and older men. Am J Clin Nutr. (2006) 83:331–42. doi: 10.1093/ajcn/83.2.331
132. Jakobsen, MU, Dethlefsen, C, Due, KM, May, AM, Romaguera, D, Vergnaud, AC, et al. Fish consumption and subsequent change in body weight in European women and men. Br J Nutr. (2013) 109:353–62. doi: 10.1017/S0007114512001079
133. Munro, IA, and Garg, ML. Dietary supplementation with long chain omega-3 polyunsaturated fatty acids and weight loss in obese adults. Obes Res Clin Pract. (2013) 7:e173–81. doi: 10.1016/j.orcp.2011.11.001
134. Kelly, T, Yang, W, Chen, CS, Reynolds, K, and He, J. Global burden of obesity in 2005 and projections to 2030. Int J Obes. (2008) 32:1431–7. doi: 10.1038/ijo.2008.102
135. Chooi, YC, Ding, C, and Magkos, F. The epidemiology of obesity. Metabolism. (2019) 92:6–10. doi: 10.1016/j.metabol.2018.09.005
136. WHO. Obesity and overweight (2017). Available at: https://www.who.int/news-room/fact-sheets/detail/obesity-and-overweight
137. Khalfoun, B, Thibault, F, Watier, H, Bardos, P, and Lebranchu, Y. Docosahexaenoic and eicosapentaenoic acids inhibit in vitro human endothelial cell production of interleukin-6. Adv Exp Med Biol. (1997) 400:589–97.
138. Endres, S, Ghorbani, R, Kelley, VE, Georgilis, K, Lonnemann, G, Der Meer, V, et al. The effect of dietary supplementation with n−3 polyunsaturated fatty acids on the synthesis of interleukin-1 and tumor necrosis factor by mononuclear cells. N Engl J Med. (1989) 320:265–71. doi: 10.1056/NEJM198902023200501
139. Kremer, JM, Lawrence, DA, Jubiz, W, Digiacomo, R, Rynes, R, Bartholomew, LE, et al. Dietary fish oil and olive oil supplementation in patients with rheumatoid arthritis clinical and immunologic effects. Arthritis Rheum. (1990) 33:810–20. doi: 10.1002/art.1780330607
140. Belzung, F, Raclot, T, and Groscolas, R. Fish oil n−3 fatty acids selectively limit the hypertrophy of abdominal fat depots in growing rats fed high-fat diets. Am J Phys. (1993) 264:R1111–8. doi: 10.1152/ajpregu.1993.264.6.R1111
141. Ruzickova, J, Rossmeisl, M, Prazak, T, Flachs, P, Sponarova, J, Vecka, M, et al. Omega-3 PUFA of marine origin limit diet-induced obesity in mice by reducing cellularity of adipose tissue. Lipids. (2004) 39:1177–85. doi: 10.1007/s11745-004-1345-9
142. Hajduch, E, Sebokova, E, Lombardo, Y, Tapsell, L, and Jenkins, A. Fish oil in a high lard diet prevents obesity, hyperlipemia, and adipocyte insulin resistance in rats. Ann N Y Acad Sci. (1993) 683:98–101. doi: 10.1111/j.1749-6632.1993.tb35696.x
143. Huang, X-F, Xin, X, McLennan, P, and Storlien, L. Role of fat amount and type in ameliorating diet-induced obesity: insights at the level of hypothalamic arcuate nucleus leptin receptor, neuropeptide Y and pro-opiomelanocortin mRNA expression. Diabetes Obes Metab. (2003) 6:35–44. doi: 10.1111/j.1463-1326.2004.00312.x
144. Thorsdottir, I, Tomasson, H, Gisladottir, E, Kiely, M, Parra, MD, Bandarra, NM, et al. Randomized trial of weight-loss-diets for young adults varying in fish and fish oil content. Int J Obes. (2007) 31:1560–6. doi: 10.1038/sj.ijo.0803643
145. Ramel, A, Jonsdottir, MT, and Thorsdottir, I. Consumption of cod and weight loss in young overweight and obese adults on an energy reduced diet for 8 weeks. Nutr Metab Cardiovasc Dis. (2009) 19:690–6. doi: 10.1016/j.numecd.2008.12.013
146. Schulz, M, Kroke, A, Liese, AD, Hoffmann, K, Bergmann, MM, and Boeing, H. Food groups as predictors for short-term weight changes in men and women of the EPIC-potsdam cohort. J Nutr. (2002) 132:1335–40. doi: 10.1093/jn/132.6.1335
147. Varsi, K, Bolann, B, Torsvik, I, Eik, TCR, Høl, PJ, and Bjørke-Monsen, AL. Impact of maternal selenium status on infant outcome during the first 6 months of life. Nutrients. (2017) 9:486. doi: 10.3390/nu9050486
148. European Food Safety Authority (EFSA). Opinion of the scientific panel on dietetic products, nutrition and allergies (NDA) related to the tolerable upper intake level of vanadium. EFSA J. (2004) 2:60. doi: 10.2903/j.efsa.2004.60
149. Di Bella, G, Potortì, AG, Lo Turco, V, Bua, D, Licata, P, Cicero, N, et al. Trace elements in Thunnus thynnus from Mediterranean sea and benefit-risk assessment for consumers. Food Addit Contam B. (2015) 8:175–81. doi: 10.1080/19393210.2015.1030347
150. EFSA Panel on Contaminants in the Food Chain (CONTAM). Scientific opinion on the risk for public health related to the presence of mercury and methylmercury in food. EFSA J. (2012) 10:2985. doi: 10.2903/j.efsa.2012.2985
151. Fundación Española de la Nutrición. Atún Fundación Española de la Nutrición (2010). 1 p Available at: https://www.fen.org.es/MercadoAlimentosFEN/pdfs/atun.pdf.
152. National Academies of Sciences, Engineering, and Medicine In: VA Stallings, M Harrison, and M Oria, editors. Dietary reference intakes for sodium and potassium. Washington, DC: The National Academies Press (2019). 594.
153. European Food Safety Authority. DVR finder database. (2024). Available att: https://multimedia.efsa.europa.eu/drvs/index.html. (Accessed January 1, 2024)
154. European Food Safety Authority (EFSA). Opinion of the scientific panel on contaminants in the food chain on a request from the commission related to mercury and methylmercury in food. EFSA J. (2004) 2:34. doi: 10.2903/j.efsa.2004.34
155. EFSA Scientific Committee. Statement on the benefits of fish/seafood consumption compared to the risks of methylmercury in fish/seafood. EFSA J. (2015) 13:3982. doi: 10.2903/j.efsa.2015.3982
156. AESAN. Recomendaciones de consumo de pescado (2019). Available at: https://www.aesan.gob.es/AECOSAN/docs/documentos/publicaciones/seguridad_alimentaria/RECOMENDACIONES_consumo_pescado_MERCURIO_AESAN_WEB.PDF
157. Ralston, NVC, Ralston, CR, and Raymond, LJ. Selenium health benefit values: updated criteria for mercury risk assessments. Biol Trace Elem Res. (2016) 171:262–9. doi: 10.1007/s12011-015-0516-z
158. Hatfield, DL, Berry, MJ, and Gladyshev, VN. Selenium status and intake influences mercury exposure risk assessments Selenium status and intake influences mercury exposure risk assessments. Selenium: its molecular biology and role in human health Springer New York, NY (2012). 598
159. Choi, AL, Mogensen, UB, Bjerve, KS, Debes, F, Weihe, P, Grandjean, P, et al. Negative confounding by essential fatty acids in methylmercury neurotoxicity associations. Neurotoxicol Teratol. (2008) 42:85–92. doi: 10.1016/j.ntt.2014.02.003
160. Kaur, G, Cameron-Smith, D, Garg, M, and Sinclair, AJ. Docosapentaenoic acid (22:5n−3): a review of its biological effects. Prog Lipid Res. (2011) 50:28–34. doi: 10.1016/j.plipres.2010.07.004
161. Oguro, A, Fujita, K, Ishihara, Y, Yamamoto, M, and Yamazaki, T. DHA and its metabolites have a protective role against methylmercury-induced neurotoxicity in mouse primary neuron and SH-SY5Y cells. Int J Mol Sci. (2021) 22:3213. doi: 10.3390/ijms22063213
162. Nisevic, JR, Prpic, I, Vukelic, P, Spiric, Z, and Horvat, M. Possible protective effects of docosahexaenoic acid (DHA) in combined prenatal exposure to methylmercury and long chain polyunsaturated fatty acids (LCPUFA). Eur J Paediatr Neurol. (2017) 21:e68. doi: 10.1016/j.ejpn.2017.04.1164
163. Axelrad, DA, Bellinger, DC, Ryan, LM, and Woodruff, TJ. Dose-response relationship of prenatal mercury exposure and IQ: an integrative analysis of epidemiologic data. Environ Health Perspect. (2007) 115:609–15. doi: 10.1289/ehp.9303
164. Golding, J, Taylor, C, Iles-Caven, Y, and Gregory, S. The benefits of fish intake: results concerning prenatal mercury exposure and child outcomes from the ALSPAC prebirth cohort. Neurotoxicology. (2022) 91:22–30. doi: 10.1016/j.neuro.2022.04.012
165. Golding, J, Hibbeln, JR, Gregory, SM, Iles-Caven, Y, Emond, A, and Taylor, CM. Maternal prenatal blood mercury is not adversely associated with offspring IQ at 8 years provided the mother eats fish: a British prebirth cohort study. Int J Hyg Environ Health. (2017) 220:1161–7. doi: 10.1016/j.ijheh.2017.07.004
166. Syversen, T, and Kaur, P. The toxicology of mercury and its compounds. J Trace Elem Med Biol. (2012) 26:215–26. doi: 10.1016/j.jtemb.2012.02.004
167. Ralston, NVC, and Raymond, LJ. Dietary selenium’s protective effects against methylmercury toxicity. Toxicology. (2010) 278:112–23. doi: 10.1016/j.tox.2010.06.004
168. Falcó, G, Llobet, JM, Bocio, A, and Domingo, JL. Daily intake of arsenic, cadmium, mercury, and lead by consumption of edible marine species. J Agric Food Chem. (2006) 54:6106–12. doi: 10.1021/jf0610110
169. Plessi, M, Bertelli, D, and Monzani, A. Mercury and selenium content in selected seafood. J Food Compos Anal. (2001) 14:461–7. doi: 10.1006/jfca.2001.1003
170. Renzi, M, Cau, A, Bianchi, N, and Focardi, SE. Levels of mercury and polychlorobiphenyls in bluefin tuna from the Western Mediterranean Sea: a food safety issue? J Environ Prot. (2014) 5:106–13. doi: 10.4236/jep.2014.52014
171. Ulusoy, Ş, Mol, S, Karakulak, FS, and Kahraman, AE. Selenium-mercury balance in commercial fish species from the Turkish waters. Biol Trace Elem Res. (2019) 191:207–13. doi: 10.1007/s12011-018-1609-2
172. Miklavčič, A, Stibilj, V, Heath, E, Polak, T, Tratnik, JS, Klavž, J, et al. Mercury, selenium, PCBs and fatty acids in fresh and canned fish available on the Slovenian market. Food Chem. (2011) 124:711–20. doi: 10.1016/j.foodchem.2010.06.040
173. Burger, J, and Gochfeld, M. Selenium and mercury molar ratios in saltwater fish from New Jersey: individual and species variability complicate use in human health fish consumption advisories. Environ Res. (2012) 114:12–23. doi: 10.1016/j.envres.2012.02.004
174. Kojadinovic, J, Potier, M, Le Corre, M, Cosson, RP, and Bustamante, P. Bioaccumulation of trace elements in pelagic fish from the Western Indian Ocean. Environ Pollut. (2007) 146:548–66. doi: 10.1016/j.envpol.2006.07.015
175. Burger, J, and Gochfeld, M. Selenium and mercury molar ratios in commercial fish from New Jersey and Illinois: variation within species and relevance to risk communication. Food Chem Toxicol. (2013) 57:235–45. doi: 10.1016/j.fct.2013.03.021
176. Fang, GC, Nam, DH, and Basu, N. Mercury and selenium content of Taiwanese seafood. Food Addit Contam B. (2011) 4:212–7. doi: 10.1080/19393210.2011.605526
177. Kaneko, JJ, and Ralston, NVC. Selenium and mercury in pelagic fish in the central North Pacific near Hawaii. Biol Trace Elem Res. (2007) 119:242–54. doi: 10.1007/s12011-007-8004-8
178. Ordiano-Flores, A, Rosíles-Martínez, R, and Galván-Magaña, F. Biomagnification of mercury and its antagonistic interaction with selenium in yellowfin tuna Thunnus albacares in the trophic web of Baja California Sur, Mexico. Ecotoxicol Environ Saf. (2012) 86:182–7. doi: 10.1016/j.ecoenv.2012.09.014
179. Yamashita, Y, Amlund, H, Suzuki, T, Hara, T, Hossain, MA, Yabu, T, et al. Selenoneine, total selenium, and total mercury content in the muscle of fishes. Fish Sci. (2011) 77:679–86. doi: 10.1007/s12562-011-0360-9
180. Jadán Piedra, C, Sánchez, V, Vélez, D, and Devesa, V. Reduction of mercury bioaccessibility using dietary strategies. LWT. (2016) 71:10–6. doi: 10.1016/j.lwt.2016.03.015
181. Torres-Escribano, S, Vélez, D, and Montoro, R. Mercury and methylmercury bioaccessibility in swordfish. Food Addit Contam A. (2010) 27:327–37. doi: 10.1080/19440040903365272
182. Cardoso, C, Afonso, C, Lourenço, H, Costa, S, and Nunes, ML. Bioaccessibility assessment methodologies and their consequences for the risk-benefit evaluation of food. Trends Food Sci Technol. (2015) 41:5–23. doi: 10.1016/j.tifs.2014.08.008
Keywords: Thynnus thunnus , nutritional composition, beneficial properties, risk-benefit ratio, food safety
Citation: Chamorro F, Cassani L, Garcia-Oliveira P, Barral-Martinez M, Jorge AOS, Pereira AG, Otero P, Fraga-Corral M, P. P. Oliveira MB and Prieto MA (2024) Health benefits of bluefin tuna consumption: (Thunnus thynnus) as a case study. Front. Nutr. 11:1340121. doi: 10.3389/fnut.2024.1340121
Edited by:
Laurent Dufossé, Université de la Réunion, FranceReviewed by:
Kashif Ameer, Chonnam National University, Republic of KoreaMiroslava Rossenova Atanassova, Møreforsking AS, Norway
Copyright © 2024 Chamorro, Cassani, Garcia-Oliveira, Barral-Martinez, Jorge, Pereira, Otero, Fraga-Corral, PP Oliveira and Prieto. This is an open-access article distributed under the terms of the Creative Commons Attribution License (CC BY). The use, distribution or reproduction in other forums is permitted, provided the original author(s) and the copyright owner(s) are credited and that the original publication in this journal is cited, in accordance with accepted academic practice. No use, distribution or reproduction is permitted which does not comply with these terms.
*Correspondence: M. Fraga-Corral, mfraga@uvigo.es; M. A. Prieto, mprieto@uvigo.es
†These authors have contributed equally to this work