- 1College of Biological Science and Technology, Shenyang Agricultural University, Shenyang, China
- 2College of Life Engineering, Shenyang Institute of Technology, Fushun, China
- 3Institute of Edible Fungi, Shanghai Academy of Agricultural Sciences, Shanghai, China
- 4Alpine Fungarium, Tibet Plateau Institute of Biology, Lasa, China
The Chinese name “Lingzhi” refers to Ganoderma genus, which are increasingly used in the food and medical industries. Ganoderma species are often used interchangeably since the differences in their composition are not known. To find compositional metabolite differences among Ganoderma species, we conducted a widely targeted metabolomics analysis of four commonly used edible and medicinal Ganoderma species based on ultra performance liquid chromatography-electrospray ionization-tandem mass spectrometry. Through pairwise comparisons, we identified 575–764 significant differential metabolites among the species, most of which exhibited large fold differences. We screened and analyzed the composition and functionality of the advantageous metabolites in each species. Ganoderma lingzhi advantageous metabolites were mostly related to amino acids and derivatives, as well as terpenes, G. sinense to terpenes, and G. leucocontextum and G. tsugae to nucleotides and derivatives, alkaloids, and lipids. Network pharmacological analysis showed that SRC, GAPDH, TNF, and AKT1 were the key targets of high-degree advantage metabolites among the four Ganoderma species. Analysis of Gene Ontology and Kyoto Encyclopedia of Genes and Genomes demonstrated that the advantage metabolites in the four Ganoderma species may regulate and participate in signaling pathways associated with diverse cancers, Alzheimer’s disease, and diabetes. Our findings contribute to more targeted development of Ganoderma products in the food and medical industries.
1 Introduction
“Lingzhi” is the Chinese term for a certain type of edible and medicinal fungi with a long history, generally including Ganoderma lingzhi, Ganoderma sinense, Ganoderma tsugae, and Ganoderma leucocontextum (1–3). Ganoderma contains a diverse range of bioactive compounds, including polysaccharides, triterpenoids, polyphenols, nucleotides, amino acids, alkaloids, and vitamins (4–6). These active compounds exert remarkable pharmacological effects and are therefore intensively researched and widely applied in the medical field (7). The pharmacological properties of Ganoderma have been demonstrated in clinical trials and therapeutic applications, encompassing its anticancer, antioxidant, immunomodulatory, hypoglycemic, cardioprotective, anti-inflammatory, antiviral, and neuroprotective effects (8–11).
Ganoderma is also highly favored in the food industry (12). For thousands of years, it has been utilized as a health-promoting food supplement renowned for its properties of relaxation, mental clarity, and physical well-being (13, 14). Currently, Ganoderma is primarily marketed in health products and nutritional supplements, such as teas, alcoholic beverages, beverages, capsules, oral solutions (12, 14). Ganoderma and derived products represent a multibillion-dollar industry worldwide (15). Thus, Ganoderma has great potential in the food and medical industries.
However, there remain some prominent and challenging issues in the development of the Ganoderma industry. The most significant issue is that it is currently uncertain whether there are differences in the composition and contents of active components among different species. If so, it may be possible to develop different Ganoderma products based on the advantages of each species in future, which would be of great importance for the further development of Ganoderma for the food and medical industries. Widely targeted metabolomics tools are suitable for addressing the aforementioned issues as they offer high throughput, high sensitivity, and high qualitative accuracy, and comprehensive databases are available (16).
In this study, we collected samples of the four commonly cultivated Ganoderma species: G. lingzhi, G. sinense, G. tsugae, and G. leucocontextum (17). We analyzed the composition of the active components in each species and identified significantly differential metabolites using widely targeted metabolomics based on ultra performance liquid chromatography-electrospray ionization-tandem mass spectrometry (UPLC-ESI-MS/MS). The research findings are beneficial for the development of Ganoderma in the food and medical industries.
2 Materials and methods
2.1 Sampling and sample extraction
The abbreviations used for the fungal species in this manuscript and the production areas of the four Ganoderma species are presented in Table 1. The fruiting bodies of all Ganoderma species are harvested upon reaching maturity through artificial cultivation. The criteria for determining Ganoderma fruiting body maturity are disappearance of the white growth ring of the fungus cap, cessation of expansion of the fungus cap and continuous thickening, and browning of the edge of the fungus cap (24, 25). Three replicate samples (1 g) from fungal caps were collected for each species. Using vacuum freeze-drying technology, place the biological samples in a lyophilizer (Scientz-100F), then grinding (30 Hz, 1.5 min) the samples to powder form by using a grinder (MM 400, Retsch). Next, weigh 50 mg of sample powder using an electronic balance (MS105DM) and add 1200 μL of −20°C pre-cooled 70% methanolic aqueous internal standard extract (less than 50 mg added at the rate of 1200 μL extractant per 50 mg sample). Vortex once every 30 min for 30 sec, for a total of 6 times. After centrifugation (rotation speed 12000 rpm, 3 min), the supernatant was aspirated, and the sample was filtered through a microporous membrane (0.22 μm pore size) and stored in the injection vial for UPLC-MS/MS analysis.
2.2 UPLC conditions
The sample extracts were analyzed using an UPLC-ESI-MS/MS system (UPLC, ExionLC™ AD, https://sciex.com.cn/; MS, Applied Biosystems 6,500 Q TRAP, https://sciex.com.cn/). The analytical column was Agilent SB-C18 (1.8 μm, 2.1 mm × 100 mm), and the mobile phase comprised solvent A, pure water with 0.1% formic acid, and solvent B, acetonitrile with 0.1% formic acid. Sample measurements were performed with a gradient program comprising 95% A, 5% B, linear gradient to 5% A and 95% B within 9 min, 5% A and 95% B for 1 min, 95% A and 5.0% B within 1.1 min and kept for 2.9 min. The flow rate was 0.35 mL/min, column oven temperature was 40°C, and the injection volume was 2 μL. The effluent was alternatively connected to an ESI-triple quadrupole (QQQ)-linear ion trap (QTRAP)-MS.
2.3 ESI-q trap-MS/MS
The ESI source operation parameters were as follows: source temperature, 500°C; ion spray voltage (IS), 5,500 V (positive ion mode)/−4,500 V (negative ion mode); ion source gas I (GSI), gas II (GSII), curtain gas were 50, 60, and 25 psi, respectively; and collision-activated dissociation, high. QQQ scans were acquired under multiple reaction monitoring (MRM), with collision gas (nitrogen) set to medium. Declustering potential (DP) and collision energy (CE) analyses for individual MRM transitions were performed, with further DP and CE optimization. A specific set of MRM transitions was monitored for each period according to the metabolites eluted within this period.
2.4 Qualitative and quantitative analyses of metabolites
Crucial MS parameters, such as DP, CE, retention time (RT), Q1 (precursor ion), and Q3 (product ion), were used to identify metabolites from the Metware database (Wuhan Metware Biotechnology Co., Ltd.). After identifying the compounds, we conducted a comparative analysis against the database and classified them into Class I and Class II based on their structural characteristics. Class I represents the primary classification of compounds, while Class II provides a more detailed categorization of metabolites in Class I. Metabolites were quantified using the MRM mode for mass spectrum peaks of metabolites.
2.5 Reconstruction and analysis of protein–protein interaction networks
The canonical SMILES of Ganoderma metabolites were retrieved from the PubChem and NPASS databases. Subsequently, protein targets for each compound were predicted using SwissTargetPrediction, with a restriction to Homo sapiens. The identified targets of each dominant Ganoderma metabolite were merged, and duplicates were eliminated. PPI networks for all targets were predicted using information provided by the STRING database, with target genes restricted to “Homo sapiens” genetic symbols and saved as tsv files. The tsv files were visualized in Cytoscape v3.9.1 to depict the PPI network. To identify the key targets, we conducted target screening within the PPI network of all targets. The screening criteria were as follows: key targets were determined based on a degree value greater than twice the median, and betweenness centrality, and closeness centrality values exceeding the median. The selected key targets were subsequently visualized using STRING and Cytoscape v3.9.1, leading to the construction of a PPI network for each key target of each Ganoderma metabolite.
2.6 Gene ontology functional annotation and Kyoto encyclopedia of genes and genomes pathway analysis
To elucidate the biological processes and signaling transduction roles of key target proteins, we employed the ClueGO tool (a Cytoscape plug-in) for GO functional annotation and KEGG pathway analysis. The analysis was performed by inputting a list of human target gene names. The pathways wherein genes were located were filtered, retaining only GO items with p values <0.01, while ensuring that each pathway contains more than 20 genes and has a ratio higher than 20%. The top 20 pathways were selected based on their ratio, and a bubble plot visualization was generated using the ggplot2 package in R.1
2.7 Statistical analysis
Unsupervised principal component analysis (PCA) was performed using the statistics function prcomp in R. The data were unit variance-scaled before unsupervised PCA. Pearson correlation coefficients between two samples were calculated using the cor function in R and the data are presented as heatmaps. In differential metabolite analysis, differential metabolites between two groups were determined based on a value importance plot (VIP) value >1 and |Log2fold change (FC)| ≥ 1.0. VIP values were extracted from orthogonal projections to latent structures-discriminant analysis (OPLS-DA) results, which also contain score plots and permutation plots, generated using the R package MetaboAnalystR. The data were log-transformed and mean-centered before OPLS-DA. To avoid overfitting, a permutation test (200 permutations) was used.
3 Results and discussion
3.1 Metabolite composition of the four Ganoderma species
Widely targeted metabolomics analysis revealed the presence of 1,187 metabolites in the Gl, Gs, Gz, and Gt samples. All detected metabolites were categorized into 11 classes under Class I: amino acids and derivatives (387), lipids (140), alkaloids (119), organic acids (110), others (110), phenolic acids (92), terpenoids (91), nucleotides and derivatives (84), flavonoids (36), lignans and coumarins (10), and quinones (8). Polysaccharides and triterpenoids have been the focus of research on the pharmacological activities of Ganoderma (26, 27). The fruiting body is the most important pharmacological part of Ganoderma. Xie et al. (6) demonstrated that the fruiting body exhibited the highest abundance of metabolites compared to the fermentation broth, mycelium, and spores of G. lucidum. Additionally, most triterpenoids were exclusively detected in the fruiting body, thereby establishing the fruiting body as a more promising candidate for the development of anti-tumor and anti-AIDS drugs (6). However, the chemical composition of Ganoderma is remarkably complex, and efforts are underway to broaden the research scope of its pharmacological constituents (28, 29). We found that amino acids and their derivatives were the most abundant metabolites in each Ganoderma species, providing new insights for the development of Ganoderma products. Amino acids and derivatives play diverse specific physiological roles in human life activities, and derived products represent an established market globally (30–32). Our findings indicate that Ganoderma is suitable for developing amino acid-based health products, such as nutritional supplements and beverages. In addition, Ganoderma is rich in other functional components such as alkaloids and organic acids, but research on their composition and functionality is limited. Based on the detected metabolites, we analyzed the metabolite composition of each Ganoderma species.
3.2 Differential metabolite composition of each Ganoderma species
The upset Venn diagram in Figure 1 shows the numbers of metabolites in each species. We detected a total of 1,187 metabolites, including 1,117 common metabolites, in all four species, with Gt having the widest variety of metabolites (1,174), followed by Gl (1,169), Gz (1,162), and Gs (1,155). Thus, there were only minor differences in metabolite variety among the four Ganoderma species were.
The pie chart in Figure 2 shows the numbers of metabolites in the different categories in each Ganoderma species. Among all species, Gz was the most abundant in amino acids and derivatives, Gl in lipids, and Gt in alkaloids and terpenoids. Differences in the numbers of metabolites in other categories were relatively small (≤3).
The interrelationships in metabolite composition among all Ganoderma species were investigated using PCA (Figure 3). PC1 and PC2 contributed 35.73 and 23.46%, respectively, of the metabolic variation among all species. The central sample Qc was a mixture of equal amounts of all samples and served as a quality control. The three samples from each Ganoderma species clustered closely together, indicating good reproducibility of the triplicate measurements. The distance between Gz and Gs was short, whereas Gl and Gt were clustered further away from the other species. These findings indicated significant differences in metabolite composition among the different Ganoderma species.
3.3 Selection and analysis of advantageous metabolites in each Ganoderma species
3.3.1 OPLS-DA
To maximize the differentiation among groups and screen differential metabolites, an OPLS-DA model was established using multidimensional statistics (33). The results indicated that differential metabolites could be screened based on VIP values (Supplementary Figure S1).
3.3.2 Differential metabolite analysis among the four Ganoderma species
The volcano plots in Figure 4 show the numbers of differential metabolites (both significantly and insignificantly) in pairwise comparisons of all Ganoderma species. There most significantly differential metabolites (764) were found between Gs and Gt, and the least (565) between Gl and Gt (Table 2). The metabolites that were significantly more abundant in one species than in the other three were regarded advantageous metabolites and are represented in an upset Venn diagram (Figure 5). According to the numbers of advantageous metabolites, the species were ranked in the following order: Gl (179) > Gt (160) > Gz (129) > Gs (37).
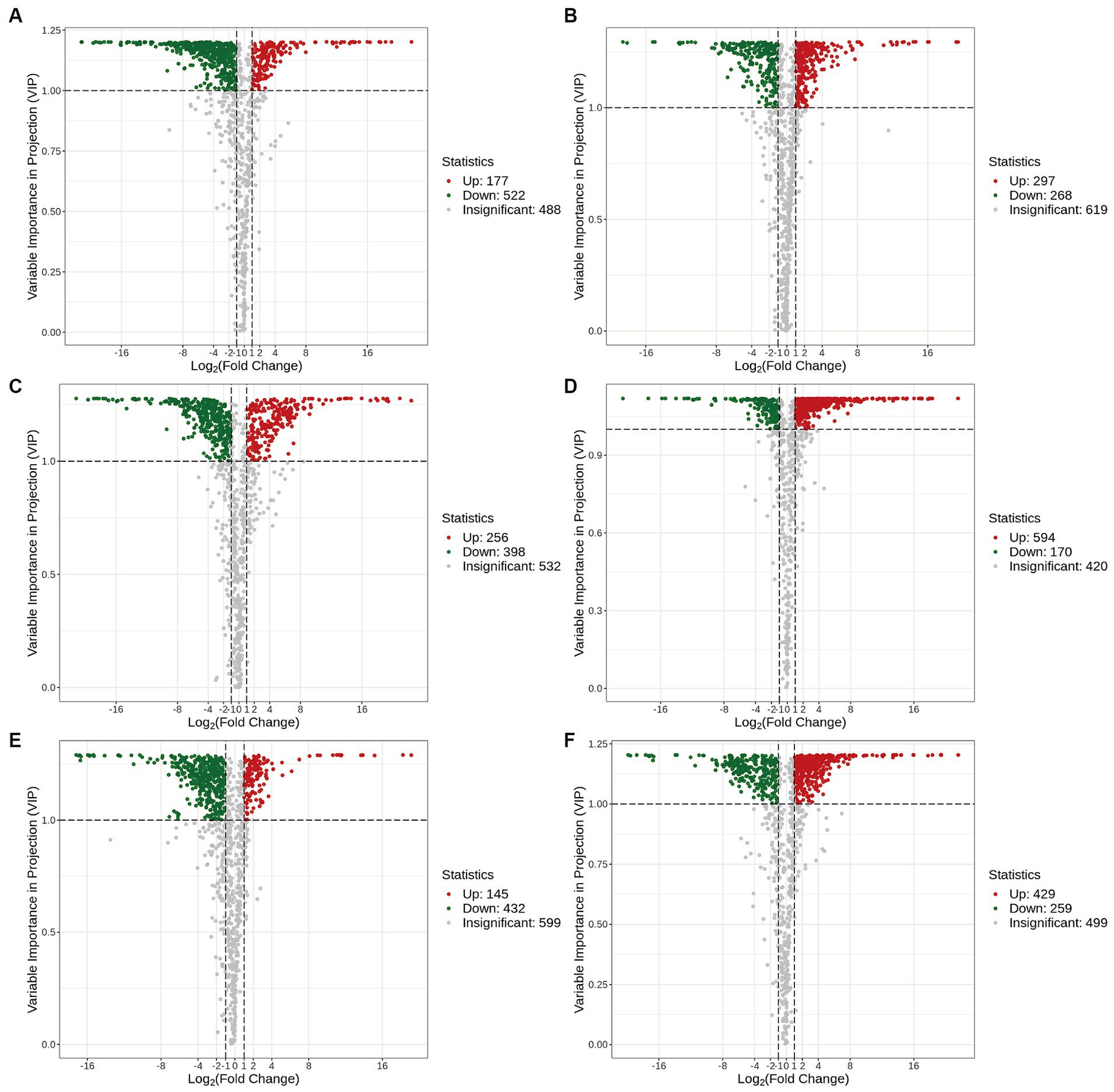
Figure 4. Volcano plots of the differential metabolites in the four Ganoderma species. (A) Gl vs. Gs; (B) Gl vs. Gt; (C) Gl vs. Gz; (D) Gs vs. Gt; (E) Gz vs. Gs; (F) Gz vs. Gt.

Table 2. Numbers of significant and non-significant differential metabolites among the four Ganoderma species.
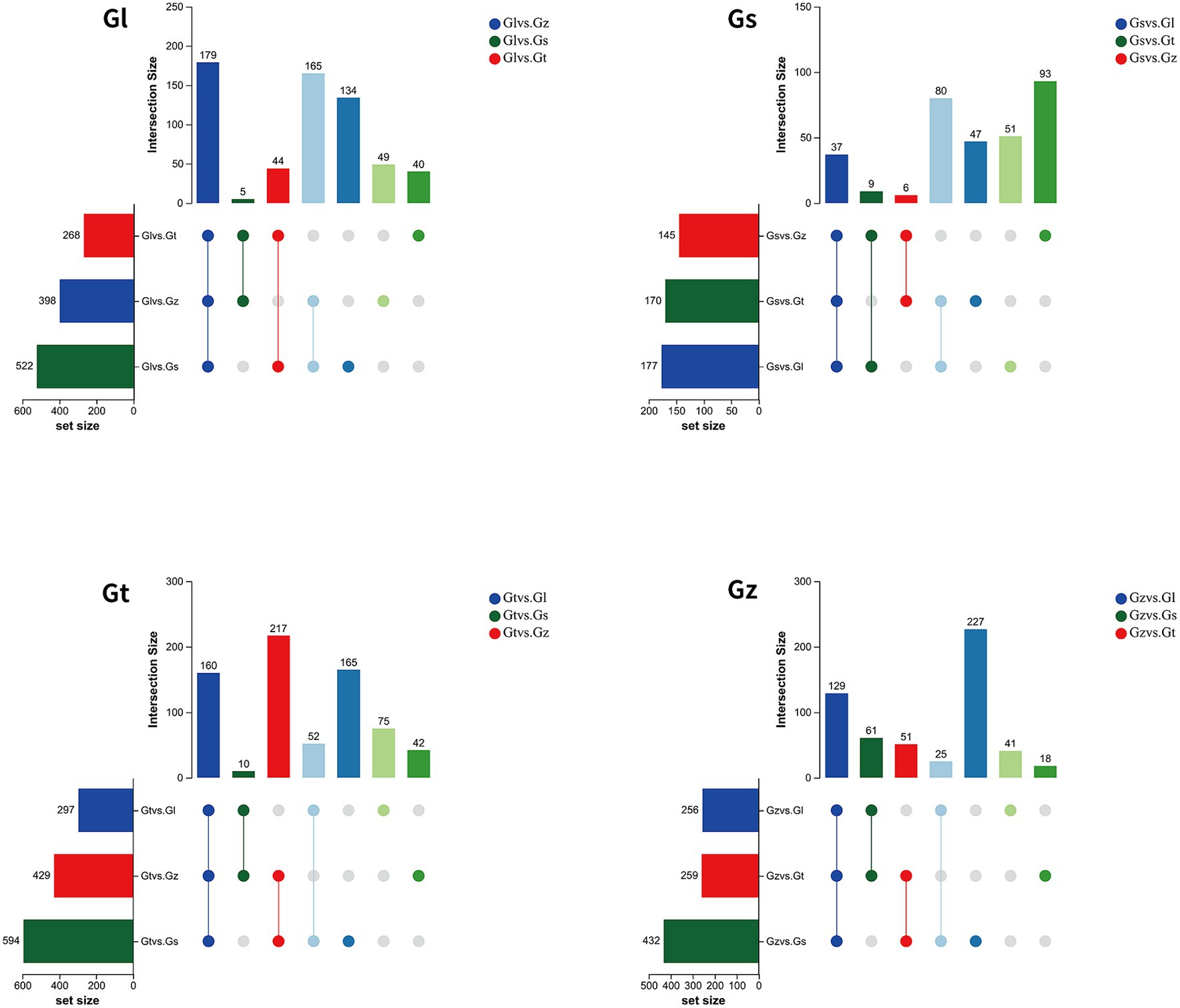
Figure 5. Upset Venn diagrams of metabolites specifically enriched in each of the four Ganoderma species.
3.3.3 FCs in advantageous metabolites among Ganoderma species
FCs represent differences in the relative abundance of metabolites. We statistically analyzed the FC of advantageous metabolites to evaluate their level of dominance (Figure 6). In general, the majority of advantageous metabolites in Gl and Gz were found in the larger FC range, whereas the majority of predominant metabolites in Gt and Gs showed smaller FCs. The advantageous metabolites with FC > 1,000 are listed in Supplementary Table S1. Most of these metabolites were unique to one species, as observed in pairwise comparisons. Some metabolites, e.g., terpenoids in Gs, exhibited a FC > 1,000-fold between two species.
3.4 Composition of advantageous metabolites of different categories among all Ganoderma species
Advantageous metabolites reflect the characteristics in which one Ganoderma species excels over the others. Therefore, we will discuss the advantageous metabolites of each species based on the classifications of the compounds. The pie charts in Supplementary Figures S2, S3 show the composition of advantageous metabolites in Classes I and II of each species.
Within Class I, the most advantageous metabolites in all species were amino acids and derivatives. According to the numbers of amino acids and derivatives among advantageous metabolites, the species were ranked in the following order: Gz (98) > Gl (71) > Gt (46) > Gs (15). Combining the results of Section 3.1 with these findings on dominant metabolites, it can be concluded that Gz is the most suitable among all Ganoderma species for developing amino acid products. Lipids were also found to be abundant in Ganoderma. According to the numbers of lipids, the species were ranked as follows: Gl (35) > Gt (33) > Gs (4) > Gz (1). The major lipids in Gl were comprised lysophosphatidylethanolamine and lysophosphatidylcholine, whereas those in Gt were mainly free fatty acids. Some fatty acids are essential because they cannot be synthesized by the human body. For instance, α-linolenic acid identified in this study has antithrombotic functions and is associated with reduced mortality rates of cardiovascular diseases and cancer (34, 35).
Terpenoids are considered one of the most important chemical compounds in Ganoderma (26, 36). According to the numbers of terpenoids among advantageous metabolites, the species were ranked as follows: Gs (9) = Gz (9) > Gt (2) > Gl (0). The contents of many terpenoids (91 in total) were relatively similar among all Ganoderma species; however, Gs and Gz were more abundant in advantageous terpenoids than the other species. Nearly all terpenoids found in Ganoderma have been demonstrated to possess beneficial physiological activities, such as anticancer and antioxidant properties (26, 36, 37). However, when we attempted to extract a large quantity of a specific terpenoid, we were unable to determine which species contains the highest amount and allows the highest extraction efficiency. Therefore, we presented the advantageous terpenoids in Supplementary Table S2 to facilitate better utilization of the terpenoids from each species.
Alkaloids are important chemical components of Ganoderma. Several studies have demonstrated that alkaloids in various Ganoderma products have remarkable health-promoting functions, including neuroprotection, renal protection, and anticancer effects (29, 38, 39). In total, 119 different alkaloids were identified in the four Ganoderma species, which calls for further research and development. According to the numbers of advantageous alkaloids among advantageous metabolites, the species were ranked as follows: Gl (20) > Gt (19) > Gz (5) > Gs (2). According to the pie chart in Supplementary Figure S2, Gl and Gt are more abundant in alkaloids than the other species. Compared to Gl, Gt has a wider variety of advantageous alkaloids. In addition to plumerane, which is also present in Gl, Gt contains pyridine alkaloids, pyrrole alkaloids, quinoline alkaloids, and piperidine alkaloids, which are absent in Gl. The alkaloids among the advantageous metabolites of all Ganoderma species are listed in Supplementary Table S2. N-Oleoylethanolamine exhibits weight-reducing and anti-atherosclerotic effects and is employed for the treatment of cardiovascular and metabolic disorders (40–42). O-Acetyl-L-carnitine functions in neuroprotection and the protection of brain development (43–45). Agmatine contributes to the regulation of glucose and lipid metabolism and has antidepressant, anticonvulsant, and neuroprotective effects (46, 47).
Nucleotides and derivatives are another important component of Ganoderma and derived health products; they exhibit pharmacological activities and health-promoting functions such as lipid-lowering effects (48–51). We identified 84 nucleotides and derivatives in the four Ganoderma species. According to the numbers of nucleotides and derivatives among advantageous metabolites, the species were ranked as follows: Gt (17) > Gl (14) > Gz (2) > Gs (0). The nucleotides and derivatives among advantageous metabolites of all Ganoderma species are listed in Supplementary Table S2. Vidarabine exhibits potent antiviral activity and is widely used as an anti-herpesvirus agent (52). Citicoline exerts neuroprotective effects and has anticonvulsant properties (53, 54). β-Nicotinamide mononucleotide possesses anti-aging properties (55, 56). Acadesine possesses antitumor activities in several cancer types (57, 58).
Flavonoids, phenolic acids, and organic acids among advantageous metabolites of four Ganoderma species are listed in Supplementary Table S2. Flavonoids are a research focus in Ganoderma studies and exhibit antioxidant and anti-inflammatory effects and cytotoxicity against cancer cells (59–62). Xanthohumol possesses antitumor activities and antiarrhythmic properties (63, 64). 2′,7-Dihydroxy-3′,4′-dimethoxyisoflavan possesses anti-inflammatory activity (65). The organic acid γ-aminobutyric acid improves sleep and reduces blood pressure (66–68). Shikimic acid has diverse biological activities and serves as an intermediate in the synthesis of anticancer drugs (69). The phenolic acids protocatechualdehyde, (E)-ethyl p-methoxycinnamate, and picein exhibit anticancer, anti-inflammatory, and antioxidant effects (70–73).
3.5 Network pharmacology analysis
3.5.1 PPI networks of key targets from the four Ganoderma species
PPI networks of key targets were constructed for advantageous metabolites of all Ganoderma species (Figure 7 and Supplementary Table S3). In each PPI network, SRC, GAPDH, TNF, and AKT1 were among the top five highest-degree targets. SRC is associated with increased tumor progression, invasion, and metastasis in many cancers (74, 75). TNF plays a crucial role in cancer treatment and autoimmunity (76, 77), while AKT1 is linked to breast cancer and ovarian cancer (78, 79). Despite differences in the advantageous metabolites from the four Ganoderma species, their mechanisms of tumor suppression are closely related to these targets.
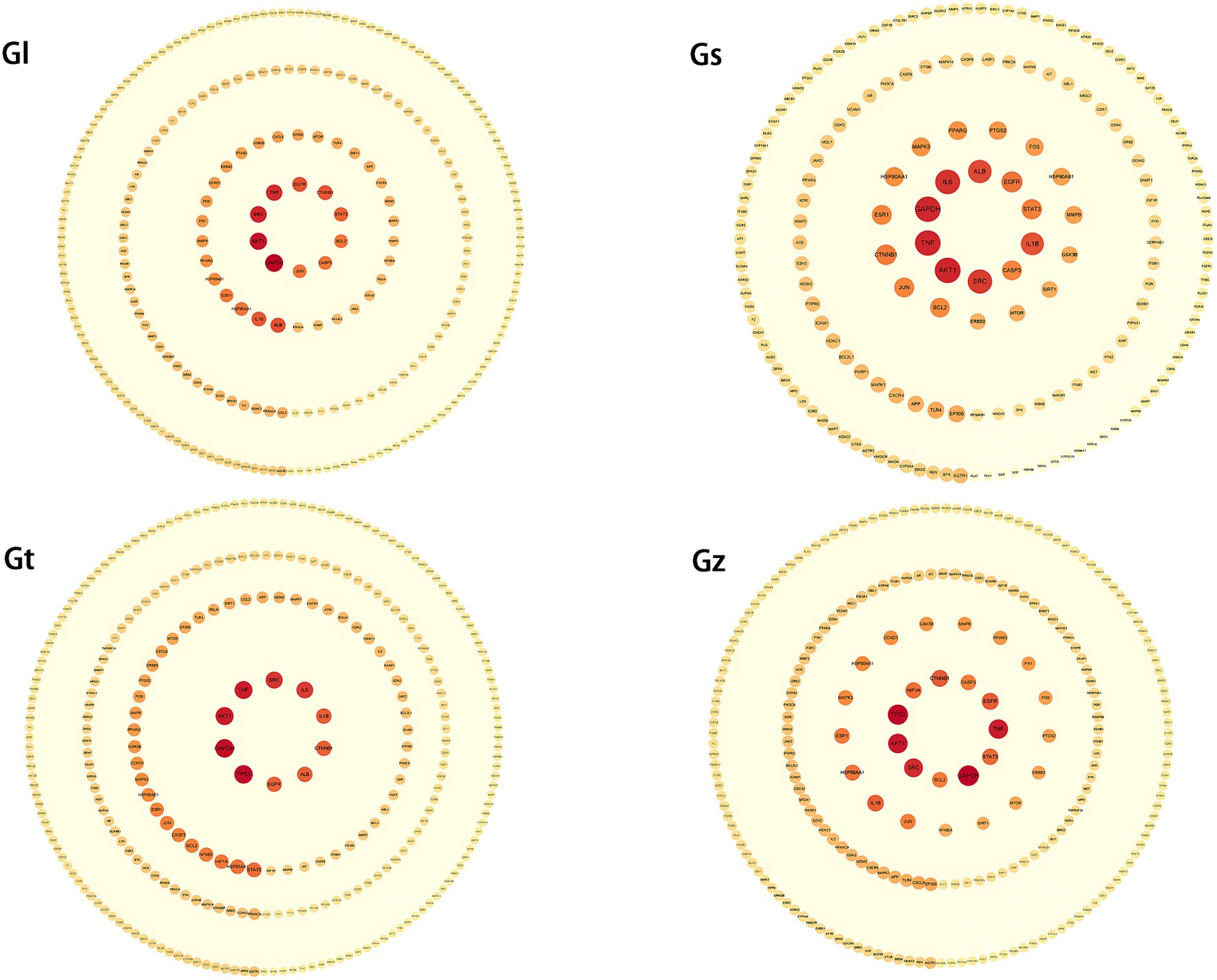
Figure 7. PPI networks of key targets from four Ganoderma species. The degree of the target increases proportionally with the darkness of the color and the size of the font.
The third highest-degree target of TP53 was identified in Gz and Gt, but not in Gl and Gs. TP53 functions as a crucial tumor suppressor, with its mutations being frequently observed in various malignant neoplasms (80, 81). This observation implies that the tumor-suppressive mechanisms of metabolites in Gz and Gt may exhibit greater prominence in regulating TP53 than those of metabolites in Gl and Gs. Albumin (ALB) exhibited a high degree in the PPI networks of Gl, Gs, and Gt. ALB is primarily synthesized by the liver in humans and serves as the predominant plasma protein (82). Extensive research has demonstrated that ALB functions as a tumor suppressor and plays a crucial role in hepatocellular carcinoma metastasis and invasion (83).
3.5.2 KEGG pathway and GO annotation analyses
GO and KEGG pathways were enriched and visualized by Cluego software (Figure 8). Among the top 20 pathways identified in the four Ganoderma species, several cancer-related pathways were prominently observed, including prostate cancer, non-small cell lung cancer, colorectal cancer, small cell lung cancer, pancreatic cancer, bladder cancer, and endometrial cancer. The advantageous metabolites of the four Ganoderma species exhibited a strong correlation with diverse cancer pathways, underscoring their potential as promising therapeutic agents. We also observed that some of the top 20 pathways were only found in certain species of Ganoderma, including cocaine addiction and B cell receptor signaling pathway in Gl; TNF signaling pathway, positive regulation of smooth muscle cell proliferation, IL-17 signaling pathway, C-type lectin receptor signaling pathway, pertussis, and neuroinflammatory response in Gs; and renal cell carcinoma in Gz. The findings facilitate a more precise investigation into the therapeutic pathways of different Ganoderma species.
The bubble plot was utilized to compare the number and ratio of genes in the pathways (Figure 9). Prostate cancer accounted for the highest ratio in all Gl and Gt pathways, reaching more than 44%. The proteasome exhibited a high ratio in both the Gz and Gt pathways. The response to amyloid-beta in the top 20 pathways of the four Ganoderma species exhibited a relatively high ratio. The neurotoxicity of amyloid-beta and its strong correlation with Alzheimer’s disease have been consistently demonstrated in recent studies (84, 85). Therefore, the neuroprotective effects of the four Ganoderma species may primarily be attributed to their modulation of the amyloid-beta pathway. Similar findings have also been corroborated in pharmacological analyses and validation studies involving G. lucidum (86, 87). The AGE-RAGE signaling pathway in diabetic complications had a high ratio in the four Ganoderma species. AGE-RAGE plays a crucial role in the development of diabetic complications, such as kidney disease and cardiovascular disease (88). Recent advancements in network pharmacological analysis and animal experiments have demonstrated that certain natural remedies, such as Radix Rehmanniae and Corni Fructus, can effectively regulate this pathway to combat diabetic complications (89); however, limited research has been conducted on Ganoderma.
4 Conclusion
We conducted a widely targeted metabolomics analysis of four Ganoderma species commonly used in the food and medical industries. The results indicate that although there are differences in the variety of metabolites among the four Ganoderma species, these differences are relatively small. However, there are significant differences in the content of metabolites among the four Ganoderma species. The relative amounts of many metabolites in different species of Ganoderma vary significantly by hundreds or even thousands of times. Therefore, even if the metabolite compositions of these four species of Ganoderma are similar, it is imperative to determine the difference in dosage when using them interchangeably. To achieve a more targeted application of Ganoderma in the medical and food fields and to facilitate further development and research of each Ganoderma species, we identified and discussed advantageous metabolites that are significantly more abundant in one Ganoderma species than in the others. Among the four Ganoderma species, Gz is the most suitable for the development of amino acid-based products. Gs and Gz are richer in terpenes, whereas Gl and Gt are more abundant in nucleotides and derivatives, alkaloids, and lipids than the other species. Network pharmacological analysis showed that the top 5 targets with high degree were similar, although the compositions of dominant metabolites in the four Ganoderma species were different. Simultaneously, certain discrepancies were also observed. For instance, among the highly correlated targets, TP53 was exclusively present in Gz and Gt, while ALB appeared in only Gl, Gs, and Gt. These variations may reflect the specificity of different Ganoderma species in targeting disease-related pathways. Furthermore, KEGG and GO analyses demonstrated that the advantageous metabolites of the four Ganoderma species have potential regulatory effects on various pathways associated with cancer, Alzheimer’s disease, and diabetes complications, among others. However, each Ganoderma species also displayed unique and significantly enriched pathways. The findings necessitate further comprehensive exploration and validation to facilitate the targeted utilization of diverse metabolites from Ganoderma. In the future, we should determine the absolute content of the advantageous metabolites of the four types of Ganoderma to determine their utilization value. At the same time, we should also study the thermal stability of the active ingredients in Ganoderma and explore the temperature range required to maintain their biological activity in daily processing.
Data availability statement
The original contributions presented in the study are included in the article/Supplementary material, further inquiries can be directed to the corresponding author.
Author contributions
LW-Y: Data curation, Formal analysis, Methodology, Software, Visualization, Writing – original draft, Writing – review & editing. GH-B: Conceptualization, Investigation, Supervision, Writing – original draft, Writing – review & editing, Formal analysis, Validation. YR-H: Investigation, Writing – review & editing. XA-G: Investigation, Writing – review & editing, Resources. ZJ-C: Visualization, Writing – original draft. YZ-Q: Writing – original draft, Resources. HW-J: Resources, Writing – original draft, Visualization. YX-D: Resources, Writing – original draft, Conceptualization, Funding acquisition, Investigation, Project administration, Supervision, Writing – review & editing.
Funding
The author(s) declare that financial support was received for the research, authorship, and/or publication of this article. This research was funded by the National Natural Science Foundation of China (No. 32370008), the Science and Technology Plan Project of Liaoning Province (2022-MS-418), Key Research and Development Plan of Tibet (XZ202201ZY0010N).
Conflict of interest
The authors declare that the research was conducted in the absence of any commercial or financial relationships that could be construed as a potential conflict of interest.
Publisher’s note
All claims expressed in this article are solely those of the authors and do not necessarily represent those of their affiliated organizations, or those of the publisher, the editors and the reviewers. Any product that may be evaluated in this article, or claim that may be made by its manufacturer, is not guaranteed or endorsed by the publisher.
Supplementary material
The Supplementary material for this article can be found online at: https://www.frontiersin.org/articles/10.3389/fnut.2024.1335538/full#supplementary-material
Footnotes
References
1. Cui, B, Pan, X, Pan, F, Sun, Y, Xing, J, and Dai, Y. Species diversity and resources of Ganoderma in China. Mycosystema. (2023) 42:170–8. doi: 10.13346/j.mycosystema.220216
2. Wu, DT, Deng, Y, Chen, LX, Zhao, J, Bzhelyansky, A, and Li, SP. Evaluation on quality consistency of Ganoderma lucidum dietary supplements collected in the United States. Sci Rep. (2017) 7:7792. doi: 10.1038/s41598-017-06336-3
3. Wu, Q, Liu, H, Shi, Y, Li, W, Huang, J, Xue, F, et al. Characteristics of the genome, transcriptome and Ganoderic acid of the medicinal fungus Ganoderma lingzhi. J Fungi. (2022) 8:1257. doi: 10.3390/jof8121257
4. Ahmad, MF . Ganoderma lucidum: persuasive biologically active constituents and their health endorsement. Biomed Pharmacother. (2018) 107:507–19. doi: 10.1016/j.biopha.2018.08.036
5. Chaturvedi, VK, Agarwal, S, Gupta, KK, Ramteke, PW, and Singh, MP. Medicinal mushroom: boon for therapeutic applications. 3 Biotech. (2018) 8:334. doi: 10.1007/s13205-018-1358-0
6. Xie, C, Yan, S, Zhang, Z, Gong, W, Zhu, Z, Zhou, Y, et al. Mapping the metabolic signatures of fermentation broth, mycelium, fruiting body and spores powder from Ganoderma lucidum by untargeted metabolomics. LWT. (2020) 129:109494. doi: 10.1016/j.lwt.2020.109494
7. Johnson, BM, Doonan, BP, Radwan, FF, and Haque, A. Ganoderic acid DM: an alternative agent for the treatment of advanced prostate cancer. Open Prostate Cancer J. (2010) 3:78–85. doi: 10.2174/1876822901003010078
8. Cör Andrejč, D, Knez, Ž, and Knez Marevci, M. Antioxidant, antibacterial, antitumor, antifungal, antiviral, anti-inflammatory, and nevro-protective activity of Ganoderma lucidum: an overview. Front Pharmacol. (2022) 13:934982. doi: 10.3389/fphar.2022.934982
9. Henao, SLD, Urrego, SA, Cano, AM, and Higuita, EA. Randomized clinical trial for the evaluation of immune modulation by yogurt enriched with β-glucans from Lingzhi or Reishi medicinal mushroom, Ganoderma lucidum (Agaricomycetes), in children from Medellin, Colombia. Int J Med Mushrooms. (2018) 20:705–16. doi: 10.1615/intjmedmushrooms.2018026986
10. Liu, Y, Lai, G, Guo, Y, Tang, X, Shuai, O, Xie, Y, et al. Protective effect of Ganoderma lucidum spore extract in trimethylamine-N-oxide-induced cardiac dysfunction in rats. J Food Sci. (2021) 86:546–62. doi: 10.1111/1750-3841.15575
11. Xiao, C, Wu, Q, Xie, Y, Tan, J, Ding, Y, and Bai, L. Hypoglycemic mechanisms of Ganoderma lucidum polysaccharides F31 in db/db mice via RNA-seq and iTRAQ. Food Funct. (2018) 9:6495–507. doi: 10.1039/c8fo01656a
12. Sheikha, A. FEl . (2022). Nutritional profile and health benefits of Ganoderma lucidum Lingzhi, Reishi, or Mannentake as functional foods: current scenario and future perspectives. Foods 11,:1030. doi: 10.3390/foods11071030
13. Fu, C . Development status and application prospects of derivative products of Ganoderma lucidum. Edible Med Mushrooms. (2023) 31:255–61.
14. Jian, Q, Ma, C, Wang, A, He, Z, and Ma, C. Analysis and reflection on food safety standards of Ganoderma lingzhi (pilot) products. Edible Med Mushrooms. (2023) 31:246–54.
15. Chang, ST . Ganoderma lucidum – a leader of edible and medicinal mushrooms. Int Agri Trade. (2004) 90:22–4.
16. Gauthier, L, Atanasova-Penichon, V, Chéreau, S, and Richard-Forget, F. Metabolomics to decipher the chemical defense of cereals against fusarium graminearum and Deoxynivalenol accumulation. Int J Mol Sci. (2015) 16:24839–72. doi: 10.3390/ijms161024839
17. Tan, W, Zhou, J, Zhang, B, Li, X, Miao, R, Huang, Z, et al. Investigation into the current status of Ganoderma cultivation and analysis of the demand for cultivation technology. Sichuan Agric Sci Technol. (2018) 1:11–2.
18. Cao, Y, Wu, S, and Dai, Y. Species clarification of the prize medicinal Ganoderma mushroom Lingzhi. Fungal Divers. (2012) 56:49–62. doi: 10.1007/s13225-012-0178-5
19. Hapuarachchi, K, Karunarathna, S, Phengsintham, P, Yang, H, Kakumyan, P, Hyde, K, et al. Ganodermataceae (Polyporales): diversity in greater Mekong subregion countries (China, Laos, Myanmar, Thailand and Vietnam). Mycosphere. (2019) 10:221–309. doi: 10.5943/mycosphere/10/1/6
20. Luangharn, T, Karunarathna, SC, Dutta, AK, Paloi, S, Promputtha, I, Hyde, KD, et al. Ganoderma (Ganodermataceae, Basidiomycota) species from the greater Mekong subregion. J Fungi. (2021) 7:819. doi: 10.3390/jof7100819
21. Cong, V . Ganoderma spp. – Biology, species and culture in Vietnam and in the Czech Republic. Dissertation’s thesis. Brno: Mendel University in Brno (2010).
23. Li, T, Hu, H, Deng, W, Wu, S, Wang, D, and Tsering, T. Ganoderma leucocontextum, a new member of the G. lucidum complex from southwestern China. Mycoscience. (2015) 56:81–5. doi: 10.1016/j.myc.2014.03.005
24. Li, G, Xu, Y, Pang, Q, Liang, X, Li, H, Hu, H, et al. Substitute cultivation techniques of Ganoderma tsugae in greenhouse. Protect For Sci Technol. (2018) 4:92-93+95. doi: 10.13601/j.issn.1005-5215.2018.04.037
25. Sun, H, and Gao, L. Planting adaptability of different Ganoderma lucidum strains in Lasa. Heilongjiang Agric Sci. (2024) 1:46–51.
26. Galappaththi, MCA, Patabendige, NM, Premarathne, BM, Hapuarachchi, KK, Tibpromma, S, Dai, DQ, et al. A review of Ganoderma triterpenoids and their bioactivities. Biomol Ther. (2022) 13:24. doi: 10.3390/biom13010024
27. Lu, J, He, R, Sun, P, Zhang, F, Linhardt, RJ, and Zhang, A. Molecular mechanisms of bioactive polysaccharides from Ganoderma lucidum (Lingzhi), a review. Int J Biol Macromol. (2020) 150:765–74. doi: 10.1016/j.ijbiomac.2020.02.035
28. Liu, J, Wang, H, Luo, Q, Qiu, S, He, Z, Liu, Z, et al. LingZhi oligopeptides amino acid sequence analysis and anticancer potency evaluation. RSC Adv. (2020) 10:8377–84. doi: 10.1039/c9ra10400c
29. Luo, Q, Cao, WW, and Cheng, YX. Alkaloids, sesquiterpenoids and hybrids of terpenoid with p-hydroxycinnamic acid from Ganoderma sinensis and their biological evaluation. Phytochemistry. (2022) 203:113379. doi: 10.1016/j.phytochem.2022.113379
30. Ellery, SJ, Walker, DW, and Dickinson, H. Creatine for women: a review of the relationship between creatine and the reproductive cycle and female-specific benefits of creatine therapy. Amino Acids. (2016) 48:1807–17. doi: 10.1007/s00726-016-2199-y
31. Rasmussen, B, Gilbert, E, Turki, A, Madden, K, and Elango, R. Determination of the safety of leucine supplementation in healthy elderly men. Amino Acids. (2016) 48:1707–16. doi: 10.1007/s00726-016-2241-0
32. Zhou, X, Dong, J, Zhuang, C, Chen, X, Chen, R, Chen, W, et al. Current situation and development trend of amino acid health products. Biotic Resourc. (2013) 35:69–72. doi: 10.14188/j.ajsh.2013.02.014
33. Jiang, N, Zhu, H, Liu, W, Fan, C, Jin, F, and Xiang, X. Metabolite differences of polyphenols in different Litchi cultivars (Litchi chinensis Sonn.), based on extensive targeted metabonomics. Molecules. (2021) 26:1181. doi: 10.3390/molecules26041181
34. Naghshi, S, Aune, D, Beyene, J, Mobarak, S, Asadi, M, and Sadeghi, O. Dietary intake and biomarkers of alpha linolenic acid and risk of all cause, cardiovascular, and cancer mortality: systematic review and dose-response meta-analysis of cohort studies. BMJ (Clinical Research Ed). (2021) 375:n2213. doi: 10.1136/bmj.n2213
35. Yang, Q, Cao, W, Zhou, X, Cao, W, Xie, Y, and Wang, S. Anti-thrombotic effects of α-linolenic acid isolated from Zanthoxylum bungeanum maxim seeds. BMC Complement Altern Med. (2014) 14:348. doi: 10.1186/1472-6882-14-348
36. Wu, GS, Guo, JJ, Bao, JL, Li, XW, Chen, XP, Lu, JJ, et al. Anti-cancer properties of triterpenoids isolated from Ganoderma lucidum – a review. Expert Opin Investig Drugs. (2013) 22:981–92. doi: 10.1517/13543784.2013.805202
37. Yan, S, Wu, Z, Xin, G, Xiao, X, Huang, M, and Meng, X. Progresses on synthesis and anti-tumor pharmacological a ctivity of Ganoderma lucidum triterpenoids. Life Sci Res. (2017) 21:454–7. doi: 10.16605/j.cnki.1007-7847.2017.05.015
38. Chen, Y, and Lan, P. Total syntheses and biological evaluation of the Ganoderma lucidum alkaloids Lucidimines B and C. ACS Omega. (2018) 3:3471–81. doi: 10.1021/acsomega.8b00295
39. Wang, XL, Dou, M, Luo, Q, Cheng, LZ, Yan, YM, Li, RT, et al. Racemic alkaloids from the fungus Ganoderma cochlear. Fitoterapia. (2017) 116:93–8. doi: 10.1016/j.fitote.2016.11.011
40. Gai, YT, Shu, Q, Chen, CX, Lai, YL, Li, WJ, Peng, L, et al. Anti-atherosclerosis role of N-oleoylethanolamine in CB2. Acta Pharm Sin. (2014) 49:316–21.
41. Wheal, AJ, Alexander, SP, and Randall, MD. Vasorelaxation to N-oleoylethanolamine in rat isolated arteries: mechanisms of action and modulation via cyclooxygenase activity. Br J Pharmacol. (2010) 160:701–11. doi: 10.1111/j.1476-5381.2010.00770.x
42. Xu, X, Guo, H, Jing, Z, Yang, L, Chen, C, Peng, L, et al. N-Oleoylethanolamine reduces inflammatory cytokines and adhesion molecules in TNF-α-induced human umbilical vein endothelial cells by activating CB2 and PPAR-α. J Cardiovasc Pharmacol. (2016) 68:280–91. doi: 10.1097/FJC.0000000000000413
43. Ferreira, GC, and McKenna, MC. L-carnitine and acetyl-L-carnitine roles and neuroprotection in developing brain. Neurochem Res. (2017) 42:1661–75. doi: 10.1007/s11064-017-2288-7
44. Martí-Carvajal, AJ, Gluud, C, Arevalo-Rodriguez, I, and Martí-Amarista, CE. Acetyl-L-carnitine for patients with hepatic encephalopathy. Cochrane Database Syst Rev. (2019) 2019:CD011451. doi: 10.1002/14651858.CD011451.pub2
45. Traina, G . The neurobiology of acetyl-L-carnitine. Front Biosci. (2016) 21:1314–29. doi: 10.2741/4459
46. Kotagale, NR, Taksande, BG, and Inamdar, NN. Neuroprotective offerings by agmatine. Neurotoxicology. (2019) 73:228–45. doi: 10.1016/j.neuro.2019.05.001
47. Uzbay, TI . The pharmacological importance of agmatine in the brain. Neurosci Biobehav Rev. (2012) 36:502–19. doi: 10.1016/j.neubiorev.2011.08.006
48. Gao, P . The technology research on the extraction of Lucidum adenosine and the culture of Lucidum mycelium with tea residues. Master’s thesis. Fujian: Fujian Agriculture and Forestry University (2009).
49. Li, H, Du, Y, Ji, H, Yang, Y, Xu, C, Li, Q, et al. Adenosine-rich extract of Ganoderma lucidum: a safe and effective lipid-lowering substance. iScience. (2022) 25:105214. doi: 10.1016/j.isci.2022.105214
50. Wen, Z, and Liu, L. Determination of adenosine in Ganoderma lucidum oral liquid by HPLC. J North Pharmacy. (2019) 16:1–2.
51. Xi, G . Determination of uridine and adenosine content of Ganoderma lucidum extract by HPLC. World Chin Med. (2014) 44:807. doi: 10.1016/j.jpba.2007.03.012
52. Suita, K, Fujita, T, Cai, W, Hidaka, Y, Jin, H, Prajapati, R, et al. Vidarabine, an anti-herpesvirus agent, prevents catecholamine-induced arrhythmias without adverse effect on heart function in mice. Pflugers Archiv Eur J Physiol. (2018) 470:923–35. doi: 10.1007/s00424-018-2121-4
53. Davinelli, S, Chiosi, F, Di Marco, R, Costagliola, C, and Scapagnini, G. Cytoprotective effects of Citicoline and Homotaurine against glutamate and high glucose neurotoxicity in primary cultured retinal cells. Oxidative Med Cell Longev. (2017) 2017:2825703. doi: 10.1155/2017/2825703
54. Karpova, MN, Zin’kovskii, KA, Kuznetsova, LV, and Klishina, NV. Increase of the seizure threshold in C57BL/6 mice after citicoline administration. Bull Exp Biol Med. (2015) 158:315–7. doi: 10.1007/s10517-015-2750-y
55. Gomes, AP, Price, NL, Ling, AJ, Moslehi, JJ, Montgomery, MK, Rajman, L, et al. Declining NAD(+) induces a pseudohypoxic state disrupting nuclear-mitochondrial communication during aging. Cell. (2013) 155:1624–38. doi: 10.1016/j.cell.2013.11.037
56. Tsubota, K . The first human clinical study for NMN has started in Japan. NPJ Aging Mechanisms Disease. (2016) 2:16021. doi: 10.1038/npjamd.2016.21
57. Pobbati, AV, and Hong, W. A combat with the YAP/TAZ-TEAD oncoproteins for cancer therapy. Theranostics. (2020) 10:3622–35. doi: 10.7150/thno.40889
58. Su, CC, Hsieh, KL, Liu, PL, Yeh, HC, Huang, SP, Fang, SH, et al. AICAR induces apoptosis and inhibits migration and invasion in prostate cancer cells through an AMPK/mTOR-dependent pathway. Int J Mol Sci. (2019) 20:1647. doi: 10.3390/ijms20071647
59. Cilerdžić, J, Vukojević, J, Stajić, M, Stanojković, T, and Glamočlija, J. Biological activity of Ganoderma lucidum basidiocarps cultivated on alternative and commercial substrate. J Ethnopharmacol. (2014) 155:312–9. doi: 10.1016/j.jep.2014.05.036
60. Dong, F, Zhong, J, and Li, P. Preparation and cytotoxic activity of total flavonoids extract from Ganoderma lucidum. Strait Pharmaceut J. (2022) 25:53–63. doi: 10.1615/IntJMedMushrooms.2023050232
61. Li, C . Extraction and determination of ganoderic acid and total flavonoids in the wild and planted Ganoderma lucidum. J Yanbian Univ. (2013) 39:269–72. doi: 10.16379/j.cnki.issn.1004-4353.2013.04.016
62. Park, MH, and Kim, M. Antioxidant and anti-inflammatory activity and cytotoxicity of ethanol extracts from Rhynchosia nulubilis cultivated with Ganoderma lucidum mycelium. Prevent Nutr Food Sci. (2018) 23:326–34. doi: 10.3746/pnf.2018.23.4.326
63. Arnaiz-Cot, JJ, Cleemann, L, and Morad, M. Xanthohumol modulates calcium signaling in rat ventricular myocytes: possible antiarrhythmic properties. J Pharmacol Exp Ther. (2017) 360:239–48. doi: 10.1124/jpet.116.236588
64. Chen, PH, Chang, CK, Shih, CM, Cheng, CH, Lin, CW, Lee, CC, et al. The miR-204-3p-targeted IGFBP2 pathway is involved in xanthohumol-induced glioma cell apoptotic death. Neuropharmacology. (2016) 110:362–75. doi: 10.1016/j.neuropharm.2016.07.038
65. Li, W, Sun, YN, Yan, XT, Yang, SY, Kim, S, Lee, YM, et al. Flavonoids from astragalus membranaceus and their inhibitory effects on LPS-stimulated pro-inflammatory cytokine production in bone marrow-derived dendritic cells. Arch Pharm Res. (2014) 37:186–92. doi: 10.1007/s12272-013-0174-7
66. Lin, Y, Tang, Q, Chu, M, Gu, M, Zhu, J, Ghenijian, O, et al. Research progress on function, production and food applica tation of γ-aminobutyric acid. China Condiment. (2021) 6:173–9.
67. Yamatsu, A, Yamashita, Y, Pandharipande, T, Maru, I, and Kim, M. Effect of oral γ-aminobutyric acid (GABA) administration on sleep and its absorption in humans. Food Sci Biotechnol. (2016) 25:547–51. doi: 10.1007/s10068-016-0076-9
68. Yang, S, Lu, Z, Lu, F, and Bie, X. Research Progress on microbial glutamate decarboxylase. Food Sci. (2005) 9:528–33.
69. Lee, MY, Hung, WP, and Tsai, SH. Improvement of shikimic acid production in Escherichia coli with growth phase-dependent regulation in the biosynthetic pathway from glycerol. World J Microbiol Biotechnol. (2017) 33:25. doi: 10.1007/s11274-016-2192-3
70. Choi, J, Jiang, X, Jeong, JB, and Lee, SH. Anticancer activity of protocatechualdehyde in human breast cancer cells. J Med Food. (2014) 17:842–8. doi: 10.1089/jmf.2013.0159
71. Kant, K, Walia, M, Agnihotri, VK, Pathania, V, and Singh, B. Evaluation of antioxidant activity of Picrorhiza kurroa (leaves) extracts. Indian J Pharm Sci. (2013) 75:324–9. doi: 10.4103/0250-474X.117438
72. Li, S, Yu, Y, Chen, J, Guo, B, Yang, L, and Ding, W. Evaluation of the antibacterial effects and mechanism of action of protocatechualdehyde against Ralstonia solanacearum. Molecules. (2016) 21:754. doi: 10.3390/molecules21060754
73. Umar, MI, Asmawi, MZ, Sadikun, A, Atangwho, IJ, Yam, MF, Altaf, R, et al. Bioactivity-guided isolation of ethyl-p-methoxycinnamate, an anti-inflammatory constituent, from Kaempferia galanga L extracts. Molecules. (2012) 17:8720–34. doi: 10.3390/molecules17078720
74. Raji, L, Tetteh, A, and Amin, R. Role of c-Src in carcinogenesis and drug resistance. Cancers. (2023) 16:32–2. doi: 10.3390/cancers16010032
75. Roskoski, R . Src protein–tyrosine kinase structure and regulation. Biochem Biophys Res Commun. (2004) 324:1155–64. doi: 10.1016/j.bbrc.2004.09.171
76. Balkwill, F . Tumour necrosis factor and cancer. Nat Rev Cancer. (2009) 9:361–71. doi: 10.1038/nrc2628
77. Holbrook, J, Lara-Reyna, S, Jarosz-Griffiths, H, and McDermott, MF. Tumour necrosis factor signalling in health and disease. F1000Research 8, F1000 faculty Rev-111. F1000Research. (2019) 1:17023. doi: 10.12688/f1000research.17023.1
78. George, B, Gui, B, Raguraman, R, Paul, AM, Nakshatri, H, Pillai, MR, et al. AKT1 transcriptomic landscape in breast cancer cells. Cells. (2022) 11:2290. doi: 10.3390/cells11152290
79. Shi, Z, Yuan, H, Cao, L, and Lin, Y. AKT1 participates in ferroptosis vulnerability by driving autophagic degradation of FTH1 in cisplatin-resistant ovarian cancer. Biochem Cell Biol. (2023) 101:422–31. doi: 10.1139/bcb-2022-0361
80. Olivier, M, Hollstein, M, and Hainaut, P. TP53 mutations in human cancers: origins, consequences, and clinical use. Cold Spring Harb Perspect Biol. (2009) 2:a001008. doi: 10.1101/cshperspect.a001008
81. Wang, Z, Strasser, A, and Kelly, GL. Should mutant TP53 be targeted for cancer therapy? Cell Death Differentiation. (2022) 29:911–20. doi: 10.1038/s41418-022-00962-9
82. Taguchi, K, Giam Chuang, VT, Maruyama, T, and Otagiri, M. Pharmaceutical aspects of the recombinant human serum albumin dimer: structural characteristics, biological properties, and medical applications. J Pharm Sci. (2012) 101:3033–46. doi: 10.1002/jps.23181
83. Fu, X, Yang, Y, and Zhang, D. Molecular mechanism of albumin in suppressing invasion and metastasis of hepatocellular carcinoma. Liver Int. (2021) 42:696–709. doi: 10.1111/liv.15115
84. Huang, Y, and Liu, R. The toxicity and polymorphism of β-amyloid oligomers. Int J Mol Sci. (2020) 21:4477. doi: 10.3390/ijms21124477
85. Itoh, S, and Okumura, H. Promotion and inhibition of amyloid-β peptide aggregation: molecular dynamics studies. Int J Mol Sci. (2021) 22:1859. doi: 10.3390/ijms22041859
86. Lai, CS, Yu, MS, Yuen, WH, So, KF, Zee, SY, and Chang, RC. Antagonizing β-amyloid peptide neurotoxicity of the anti-aging fungus Ganoderma lucidum. Brain Res. (2008) 1190:215–24. doi: 10.1016/j.brainres.2007.10.103
87. Luz, DA, Pinheiro, AM, Fontes-Júnior, EA, and Ferraz, S. Neuroprotective, neurogenic, and anticholinergic evidence of Ganoderma lucidum cognitive effects: crucial knowledge is still lacking. Med Res Rev. (2023) 43:1504–36. doi: 10.1002/med.21957
88. Sanajou, D, Ghorbani Haghjo, A, Argani, H, and Aslani, S. AGE-RAGE axis blockade in diabetic nephropathy: current status and future directions. Eur J Pharmacol. (2018) 833:158–64. doi: 10.1016/j.ejphar.2018.06.001
Keywords: widely targeted metabolomics, Ganoderma, Ganoderma lingzhi, Ganoderma sinense, Ganoderma leucocontextum, Ganoderma tsugae
Citation: Wei-Ye L, Hong-Bo G, Rui-Heng Y, Ai-Guo X, Jia-Chen Z, Zhao-Qian Y, Wen-Jun H and Xiao-Dan Y (2024) UPLC-ESI-MS/MS-based widely targeted metabolomics reveals differences in metabolite composition among four Ganoderma species. Front. Nutr. 11:1335538. doi: 10.3389/fnut.2024.1335538
Edited by:
Theodoros Chatzimitakos, University of Ioannina, GreeceReviewed by:
A. K. Güneş, Selçuk University, TürkiyeAttila Kiss, University of Debrecen, Hungary
Qi An, Langfang Normal University, China
Mei-Ling Han, Langfang Normal University, China
Gao-Qiang Liu, Central South University of Forestry and Technology, China
Copyright © 2024 Wei-Ye, Hong-Bo, Rui-Heng, Ai-Guo, Jia-Chen, Zhao-Qian, Wen-Jun and Xiao-Dan. This is an open-access article distributed under the terms of the Creative Commons Attribution License (CC BY). The use, distribution or reproduction in other forums is permitted, provided the original author(s) and the copyright owner(s) are credited and that the original publication in this journal is cited, in accordance with accepted academic practice. No use, distribution or reproduction is permitted which does not comply with these terms.
*Correspondence: Yu Xiao-Dan, eXV4ZDEyNkAxMjYuY29t
†These authors have contributed equally to this work