- 1Exercise Psychophysiology Research Group, School of Arts, Science and Humanities, University of São Paulo, São Paulo, Brazil
- 2School of Physical Education and Sports, University of São Paulo, São Paulo, Brazil
- 3Department of Nutrition, University of Mogi das Cruzes, São Paulo, Brazil
- 4Department of Physical Education, Federal University of Paraná, Paraná, Brazil
- 5Department of Health Sciences and Human Performance, The University of Tampa, Tampa, FL, United States
We examined if carbohydrate (CHO) mouth rinse may reduce central fatigue and perceived exertion, thus improving maximal incremental test (MIT) performance. Nine recreational cyclists warmed up for 6 min before rinsing a carbohydrate (CHO) or placebo (PLA) solution in their mouth for 10 s in a double-blind, counterbalanced manner. Thereafter, they performed the MIT (25 W·min−1 increases until exhaustion) while cardiopulmonary and ratings of perceived exertion (RPE) responses were obtained. Pre- to post-MIT alterations in voluntary activation (VA) and peak twitch torque (Tw) were determined. Time-to-exhaustion (p = 0.24), peak power output (PPO; p = 0.45), and V̇O2MAX (p = 0.60) were comparable between conditions. Neither treatment main effect nor time–treatment interaction effect were observed in the first and second ventilatory threshold when expressed as absolute or relative V̇O2 (p = 0.78 and p = 0.96, respectively) and power output (p = 0.28 and p = 0.45, respectively) values, although with moderate-to-large effect sizes. RPE increased similarly throughout the tests and was comparable at the ventilatory thresholds (p = 0.56). Despite the time main effect revealing an MIT-induced central and peripheral fatigue as indicated by the reduced VA and Tw, CHO mouth rinse was ineffective in attenuating both fatigues. Hence, rinsing the mouth with CHO was ineffective in reducing central fatigue, lowering RPE, and improving MIT performance expressed as PPO and time-to-exhaustion. However, moderate-to-large effect sizes in power output values at VT1 and VT2 may suggest some beneficial CHO mouth rinse effects on these MIT outcomes.
1 Introduction
Carbohydrate (CHO) mouth rinse has been suggested as a potential aid to improve endurance performance in exercises with different durations (1, 2). The suggested mechanism underpinning the CHO mouth rinse effects on exercise performance is the likely enhanced neuromuscular activation and reduced rating of perceived exertion (RPE) (3, 4) when the CHO is sensed by oral cavity sensors (5, 6). For example, Gant et al. (6) found an increased force production in participants rinsing their mouths with CHO due to a facilitated motor output as a result of the enhanced corticospinal excitability. Others have observed that the improved cycling performance with CHO mouth rinse was associated with a reduced RPE during exercise (3). Thus, the overall suggestion is that the improved endurance motor output with CHO mouth rinse is due to an ameliorated neuromuscular activation and attenuated RPE (6).
Most studies reporting an improved endurance cycling performance with CHO mouth rinse have used trials lasting more than 30–60 min (2). These longer-lasting trials are characterized by submaximal power output values, that is, values usually ≤80% of the peak power output (PPO) attained in maximal incremental test (MIT), therefore eliciting central rather than peripheral fatigue (7). However, a previous study (8) observed that CHO mouth rinse failed to improve performance in a shorter-lasting cycling time trial (4 km distance), eliciting mean power output values closer to PPO values (i.e., ≥80% PPO) as peripheral rather than central fatigue would limit this type of effort (7, 9). Together, these results may suggest that CHO mouth rinse has the potential to improve endurance cycling performance during exercises requiring power output values ≤ 80% PPO, during which central rather than peripheral fatigue is limiting. Consequently, CHO mouth rinse would be ineffective in improving short-endurance cycling exercise performance with peripheral fatigue as a limiting factor (3).
Studies have reported a growing use of CHO mouth rinse as a strategy to improve performance in different endurance exercise modes such as cycling and running (2, 8, 10, 11). The fact that CHO mouth rinse induces no gastric discomfort, as no ingestion is necessary, may make this strategy a promising ergogenic aid for endurance sports competitions and training sessions (10). In this regard, trainers and cyclists may be interested in knowing if CHO mouth rinse affects outcomes used to prescribe and monitor endurance cycling training sessions, as this may overestimate training zone intensities when compared with non-supplemented conditions. For example, they may want to know if CHO mouth rinse changes the power output and RPE corresponding to the first and second ventilatory thresholds (VT1 and VT2, respectively) or the PPO associated with the maximal oxygen uptake (i.e., V̇O2MAX), as these variables have been traditionally used to determine endurance intensities training zones (12, 13). For example, the ameliorated neuromuscular activation and attenuated RPE with CHO mouth rinse (6) could lead to a higher power output and lowered RPE during exercise at VT1 and VT2. Unfortunately, studies on CHO mouth rinse effects have focused on cycling time trials (14–17), so we are unaware of studies assessing the effects of CHO mouth rinse on MIT performance and their underlying mechanisms. In contrast to short cycling time trials (8, 9), most of the MIT duration is performed under submaximal intensities-elicited central fatigue (≤80% PPO) (9); thus, one may argue that CHO mouth rinse may potentiate MIT performance due to an ameliorated neuromuscular activation and reduced RPE during most MIT intensities. Consequently, CHO mouth rinse may increase PPO and lower the RPE at VT1, VT2, and V̇O2MAX intensities during an MIT.
In the present study, we examined if CHO mouth rinse improved MIT performance, changing outcomes such as PPO and RPE at VT1, VT2, and V̇O2MAX due to attenuated central fatigue, as reported elsewhere (15). Due to submaximal power output values during most stages of the MIT, we hypothesized that CHO mouth rinse would attenuate central fatigue and reduce RPE during exercise, thus improving MIT performance outcomes.
2 Materials and methods
2.1 Participants
Nine recreational male cyclists ranked as Performance Level 2 (18) (36.9 ± 6.4 years, V̇O2MAX of 54.3 ± 9.3 mL·kg−1·min−1; body mass of 73.1 ± 13.0 kg; height of 180 ± 5 cm; PPO of 333 ± 29 W), and having >2 years of experience in regional competitions voluntarily participated in this study after signing the consent form. All procedures were approved by the ethics committee of the University of São Paulo (#0023.0.342.000-10) and conducted according to the Declaration of Helsinki. All participants were free from cardiovascular, neural, or musculoskeletal diseases/conditions that could interfere with the experimental protocol. Furthermore, they were asked to refrain from caffeine, alcohol, and other stimulant substances, as well as intense exercises, during the 24 h preceding the experimental tests.
2.2 Study design
The cyclists attended the laboratory on four occasions. During the first visit, the cyclists were familiarized with the maximal voluntary contraction (MVC) and twitch interpolation protocol, the 15-point RPE scale (19), and were asked to report their preceding 24-h alimentary intake. On the second visit, they were familiarized with the mouth rinses and performed another familiarization with the MVC before performing a preliminary MIT. During visits 3 and 4, they performed the MIT while rinsing their mouth either with CHO or with a placebo (PLA) solution. Visits 3 and 4 were performed in a randomized, counterbalanced, and double-blind design. Importantly, visits were interspaced by 3–7 days. After performing a 5-min self-paced exercise followed by 1 min controlled-pace exercise (100 W pedaling at 80 rpm), the MIT increased 25 W∙min−1 until exhaustion (i.e., the inability to maintain 80 rpm pedal cadence), while strong verbal encouragement was provided by the same researcher. The cyclists used the same speed bicycle (Giant® Thousand Oaks, CA, United States) individually adjusted before each test, connected to a cycle-simulator (Computrainer™ Lab 3D, RacerMate, Seattle, United States) calibrated according to the manufacturer’s recommendation.
2.3 Dietary control
We decided to evaluate the cyclists in a fed and normal muscle glycogen state, thus mimicking a condition met in practical situations, as individuals are frequently oriented to consume a balanced diet ~2 h before an MIT in practical situations such as in clinical and sports science environments. Thus, the cyclists followed an individual, one-day alimentary plan (~55% CHO, ~25% protein, and ~20% fat) based on the alimentary record previously provided in the first visit. Then, the cyclists were required to repeat the dietary plan before the experimental sessions, including a breakfast 2 h before the experimental trials. Upon their arrival at the laboratory, we asked them to confirm that they had followed the diet recommendations, mainly the individualized breakfast composed of ~55% CHO, ~25% protein, and ~20% fat.
2.4 Mouth rinse
The cyclists rinsed their mouths with 25 mL of CHO or PLA solution for the last 10 s of the controlled-pace warm-up, spitting the substance into a bowl immediately after the mouth rinse (20). The volume spat into the bowl was checked after the test to confirm they had not ingested the solution. Importantly, in addition to the practical approach as above clarified, an MIT is comparable to a high-intensity time trial (9) so that the vigorous body movement and hyperventilation after VT2 limit the use of mouth rinse. In contrast, removing the mask of the gas analyzer during the MIT would increase the likelihood of experimental error associated with gaseous exchanges. The CHO solution was composed of 64 g of glucose diluted in 1,000 mL of water (6.4%), while the PLA solution was made of 36.2 g of non-caloric saccharin-based sweetener diluted in 1,000 mL of water (3.6%). These concentrations are in agreement with previous results suggesting that a higher concentration than 6% of CHO produces no increment in exercise performance (21, 22). Importantly, we confirmed that participants were unable to distinguish the placebo from the CHO solution during the familiarization visit.
2.5 Twitch interpolation protocol
The pre- and post-knee extension torque measures (twitch torque and interpolated torque) were performed on the dominant limb through a Biodex System 3 (Biomedical Systems ®, Newark, CA, United States) immediately before and after the MIT (<40 s). Participants were seated on the chair, individually adjusted to maintain the hips and knees at 90° and 60°, respectively, from the horizontal plane. Importantly, the chest and hips were carefully fixed to avoid any accessory movements. The estimated center of rotation of the knee joint was visually aligned with the Biodex rotation center. Torque measures were assumed to be the torque produced by the Biodex motor, adjusted by gravity.
To impose supramaximal electrical stimuli, two 7.5 × 13-centimeter self-adhesive electrodes (Mundinter Dantec Clavis ®, Lisbon, Portugal) were positioned on the first- (cathode) and second-thirds (anode) of distance from the anterior superior iliac spine to the proximal border of the patella of the right thigh, respectively. Before the electrode’s placement, regions of the cathode and anode were shaved, abraded, and cleaned using alcohol to reduce skin impedance. Two pulses of 1 ms at 100 Hz were delivered over the muscle belly (Nicolet Viking Quest portable EMG apparatus; CareFusion ®, San Diego, CA, United States) using an individualized intensity determined during the maximum torque at rest (23). To perform the maximal torque test, the participants were instructed to perform a 5-s maximal MVC of the knee extensors. Electric stimuli were imposed at the maximal torque plateau (visually identified) (24), while the second stimulus was imposed 1.5 s after the end of the MVC (potentiated twitch). Torque values were controlled by visual feedback and saved for offline analysis (LabVIEW 2010, National Instruments, São Paulo, Brazil). Voluntary activation (VA) is described by the Equation (1) (25, 26). Delta of peak twitch torque (ΔTw) was measured using the potentiated 100 Hz doublet (after MVC), pre- and post-MIT to determine changes in peripheral fatigue (7, 15).
2.6 Measures and data analysis
2.6.1 Maximal incremental test performance
Time-to-exhaustion, PPO, and power output at VT1 and VT2 expressed as absolute and relative values (% PPO) were used as performance outcomes.
2.6.2 Cardiopulmonary responses
Cardiopulmonary responses such as oxygen uptake (V̇O2) and minute ventilation (V̇E) were assessed breath-by-breath through an open-system gas analyzer (Cosmed, Quark PT, Albano Laziale, Rome, Italy) calibrated before each test. Expired air volume was assessed through a bi-directional flow sensor calibrated before each test using a 3-L air syringe. The cyclists used a mask (Hans Rudolph R, Lenexa, KS, United States) connected to the gas analyzer to measure breath-by-breath gaseous exchange, which was filtered to 10 s epochs thereafter. V̇O2MAX was calculated as the average of the last 30 s of the test (27). Moreover, two evaluators visually identified VT1 and VT2 at the first and second breakpoints of the V̇E–time relationship, respectively. These breakpoints were confirmed by the breakpoint of the expired fractions of O2 (FeO2) and CO2 (FeCO2) responses (27). The PPO was calculated as suggested by De Pauw et al. (18) (Equation 2), where PO1 is the power output during the last fully completed stage, PO2 is the power output during the highest stage (not completed), T1 is the time (in min) of the previous stage duration, and T2 is the time spent at the last stage.
2.6.3 Ratings of perceived exertion
The RPE was assessed at the end of each MIT stage so that the RPE corresponding to VT1 and VT2 were reported. In addition, the slope of the RPE–time relationship (RPESLOPE) was used to verify how cyclists’ RPE increased throughout the MIT after CHO or PLA mouth rinse.
2.7 Statistical analysis
Data normality and homoscedasticity were initially checked through the Shapiro–Wilk and Levene tests, respectively. Outcomes such as V̇O2MAX, PPO, time-to-exhaustion, and RPESLOPE were compared using a paired Student’s t-test (CHO vs. PLA). Absolute and relative values of PPO and V̇O2 (% PPO or % V̇O2MAX) and RPE at VT1 and VT2 were compared through a 2 × 2 mixed model having time (VT1 vs. VT2) and treatment (CHO vs. PLA) as fixed factor, while subjects were the random factor. Accordingly, VA and Tw values obtained pre- and post-MIT were compared through a 2 × 2 mixed model having time (pre- vs. post-MIT) and treatment (CHO vs. PLA) as fixed factors, while the subjects were the random factor. Multiple comparisons were corrected through Bonferroni’s test when F values were significant. Effect size (ES) was calculated according to the family test used, expressed as Cohen’s d, to make possible comparisons with previous studies. Moreover, ES values were interpreted as proposed by Hopkins et al. (28) as Cohen’s d ≤ 0.1 is considered small, 0.1> and <0.3 moderate, 0.3> and <0.5 large, 0.5> and <0.9 very large, and >0.9 extremely large. Statistical significance was set at 5% (p < 0.05).
3 Results
3.1 MIT performance and outcomes
There was no significant difference in V̇O2MAX (t = 0.552, p = 0.60, d = 0.10, ES small), PPO (t = −0.785, p = 0.45, d = 0.08, ES small), and time-to-exhaustion (t = −1.259, p = 0.24, d = 0.12, ES small) between CHO and PLA mouth rinse. RPE increased throughout the MIT similarly in both CHO and PLA mouth rinse conditions, given the comparable RPESLOPE between conditions (t = −0.610, p = 0.56, d = 0.19, ES = moderate).
As expected, responses at VT1 were different from VT2; thus, we omitted time main effects from the manuscript to make the reading easier. There was no treatment main effect, so absolute V̇O2 (F = 0.08, p = 0.78, d = 0.15, ES small) and power output values (F = 1.33, p = 0.28, d = 0.58, ES large), as well as % V̇O2MAX (F = 0.003, p = 0.96, d = 0.03, ES small) and % PPO (F = 0.64, p = 0.45, d = 0.40, ES large), were comparable between CHO and PLA conditions. Additionally, no treatment-by-time interaction effect was found either in absolute V̇O2 (F = 0.10, p = 0.76, d = 0.17, ES small) and power output values (F = 0.26, p = 0.62, d = 0.26, ES moderate) or in % V̇O2MAX (F = 0.003, p = 0.96, d = 0.03, ES small) and % PPO values (F = 0.31, p = 0.58, d = 0.28, ES moderate). Accordingly, neither treatment main effect (F = 1.13, p = 0.31, d = 0.53, ES = large) nor time-by-treatment interaction effect (F = 0.79, p = 0.40, d = 0.44, ES = large) was observed in RPE. All these MIT outcomes were reported in Table 1.
3.2 Voluntary activation and twitch interpolation responses
There was neither the time-by-treatment interaction effect (F = 0.87, p = 0.37, d = 0.54, ES large) nor the treatment main effect (F = 0.22, p = 0.65, d = 0.27, ES moderate); however, a time main effect (F = 7.89, p = 0.01, d = 0.62, ES large) was detected on torque responses, indicating an exercise-induced reduction (Figure 1).
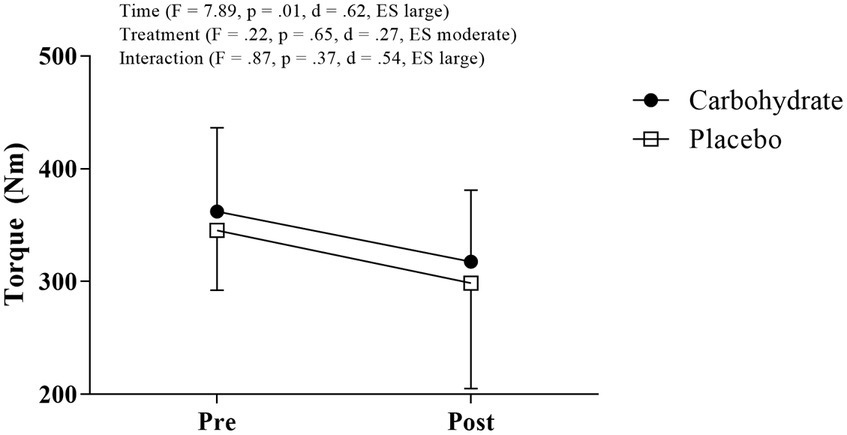
Figure 1. Torque measured before and after MIT in carbohydrate (black circle) and placebo (white square) mouth rinse trials.
Accordingly, neither the time-by-treatment interaction effect (F = 0.01, p = 0.94, d = 0.04, ES small) nor the treatment main effect (F = 0.06, p = 0.82, d = 0.14, ES moderate) was observed in VA, despite the borderline value of p and extremely large ES (F = 3.79, p = 0.07, d = 1.12, ES extremely large) suggesting an exercise-reduced VA (i.e., time main effect). Similarly, neither the time-by-treatment interaction effect (F = 1.50, p = 0.25, d = 0.71, ES very large) nor the treatment main effect (F = 0.62, p = 0.45, d = 0.45, ES large) were observed in ΔTw; however, the time main effect in ΔTw (F = 96.20, p < 0.01, d = 5.66, ES extremely large) evidenced exercise-increased peripheral fatigue. Figures 2A,B show central and peripheral fatigue responses.
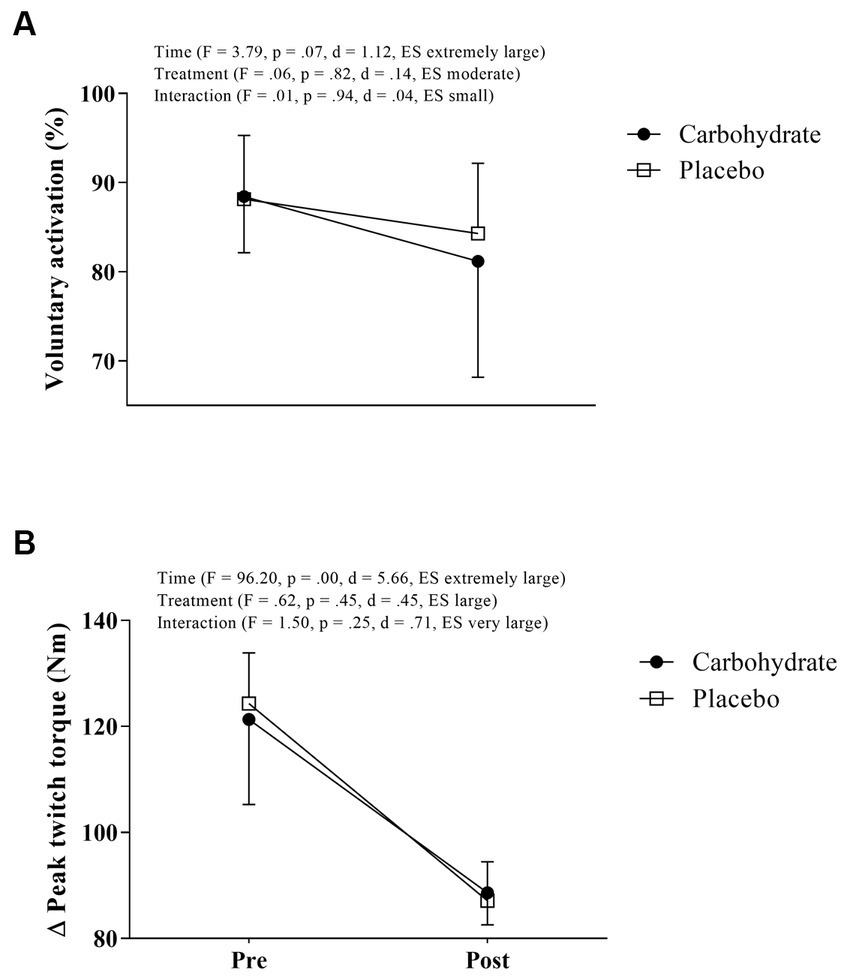
Figure 2. Voluntary activation (A) and peak twitch torque (B) measured before and after MIT in carbohydrate (black circle) and placebo (white square) mouth rinse trials.
4 Discussion
We investigated if MIT outcomes may be improved with CHO mouth rinse due to reduced central fatigue and RPE. In agreement with previous results in short-cycling exercises (8, 29), we found that CHO mouth rinse did not improve MIT outcomes such as VT1, VT2, PPO, and V̇O2MAX, although moderate-to-large effect sizes may suggest some beneficial effects in VT1 and VT2 outcomes. Despite the moderate-to-large effect size, we also found null effects of CHO mouth rinse in the central fatigue index, as reported elsewhere (8, 15). These results provide support to suggest that CHO mouth rinse has neglectable beneficial effects to reduce central fatigue and improve performance outcomes in MIT (8).
Several studies have suggested that CHO mouth rinse may improve different performance outcomes (3, 30), despite some null effects reported elsewhere (8, 14, 31). An earlier study showed improvements in ~1 h cycling time trial performance with CHO mouth rinse when participants were evaluated in fasted (↑ 3.4%) as well as fed state (↑ 1.8%), although the lower effect was in the fasted state (32). Thus, we used a logic-driven hypothesis to expect some improvement in MIT performance even though participants were in a fed state. In brief, we evaluated participants in a fed state to mimic practical situations from sports scenarios, as individuals are usually recommended to consume a balanced diet ~2 h before an MIT. Our results showed that CHO mouth rinse was ineffective at improving MIT performance outcomes such as time-to-exhaustion, PPO, and power output at VT1 and VT2. Consequently, no changes were observed in V̇O2 responses such as V̇O2MAX and V̇O2 at VT1 and VT2. Furthermore, PLA and CHO also showed comparable RPE responses throughout the MIT. These null results relate to the mechanisms suggested for the CHO mouth rinse ergogenic aid and to the exercise mode used in the present study.
Earlier studies by Chambers et al. (3) and Turner et al. (4) suggested that the presence of CHO in the oral cavity may increase the activation in motor planning cerebral areas associated with the reward circuit system and exercise regulation (33). Chambers et al. (3) showed that those cyclists who improved their time-trial performance at the same RPE in study 1 (greater average power output and reduced elapsed time in the last 75% of the cycling trial) elicited a further enhanced cerebral activation in frontal and premotor areas in study 2. Thus, they proposed that the enhanced activity in areas related to reward and exercise regulation in study 2 explained the improved performance and reduced RPE during exercise in study 1 (3, 4, 33). This mechanism may be potentiated in the participants in a fasted rather than fed state. Studies have observed that variations in the food-derived metabolic signals such as plasma ghrelin and leptin may play a role in the CHO mouth rinse-induced modulation in cerebral activity, as this signalization may induce greater cerebral responses mainly in periods of food abstinence longer than 4 h (10). Consequently, CHO mouth rinse-increased cerebral activation may not have been optimal in the present study, as participants were assessed in a fed state. Furthermore, the fact that the participants were allowed to have CHO meals while avoiding intense exercises during the 24 h before the experimental sessions likely preserved their muscle glycogen stores upon their arrival at the laboratory. This fact may have minimized the impact of an eventual improved cerebral activation due to the CHO mouth rinse, thus contributing to the null effects observed in MIT performance.
The exercise mode used in the present study may also have minimized the ergogenic aid effect of the CHO mouth rinse. We found no CHO mouth rinse effect on exercise-induced central fatigue, as reductions in VA after MIT were comparable between CHO (↓ ~3.01%) and PLA (↓ ~4.14%) mouth rinses. Similarly, RPE responses were comparable between both mouth rinses. CHO mouth rinse may reduce RPE and potentiate performance in long rather than short-term exercises, as the former is known to induce considerable levels of central fatigue (7). Thomas et al. (7) observed that short-lasting time trials such as 4 km induced greater peripheral fatigue as measured by potentiated quadriceps twitch force (i.e., ∆Tw) while longer-lasting ones (20 and 40 km) induced greater central fatigue. Hence, considering the central action of CHO mouth rinse, this strategy may be effective in attenuating central fatigue (34) and potentiating performance in cycling exercises longer than MIT exercises. Interestingly, we found that CHO mouth rinse failed to potentiate a 4-km cycling time trial despite the reduced central fatigue (8). Considering these exercise modes induce comparable physiological responses mainly from VT2 intensities (9), the ineffectiveness of the CHO mouth rinse to improve MIT outcomes may be possibly related to the fact that peripheral rather than central mechanisms limit performance in this exercise mode.
4.1 Methodological aspects and practical implications
Some methodological aspects should be highlighted. First, we are aware that neuromuscular fatigue recovers as soon as the exercise has been terminated so the time taken to obtain neuromuscular measures after the MIT may be a concern. However, we rehearsed the transition from the bike to the isokinetic dynamometer several times, which greatly decreased the transition time (~40 s). Although we may have missed some of the immediate changes post-MIT, in our experience, this time window is sufficient to detect neuromuscular changes as those reported in the literature (7, 8).
Most CHO mouth rinse studies used rinses at regular intervals, frequently at every 12.5% of the test duration, when investigating its effects on exercise performance (3, 11, 35). In contrast, in the present study, the participants rinsed their mouths once during the last 1 min of the cycling warm-up. This strategy was used for different reasons. First, the use of mouth rinse during an MIT, mainly from VT2 intensity, could preclude participants from the maximal effort, as they would have to rinse their mouth and spit the solution out while hyperventilating. Furthermore, as the gaseous exchange was assessed throughout the MIT, the mouth rinse would require removing the gas analyzer mask during exercise, thus increasing the likelihood of error on the gaseous exchange measure. Hence, considering the fact that CHO mouth rinse effects seem to be timely (6), the use of a single 10-s mouth rinse before the MIT commencement is apparently ineffective in improving MIT outcomes.
Moreover, despite controversial results indicating positive (32, 36) and null effects of CHO mouth rinse in both fed and fasted states (29, 37), Lane et al. (32) suggested that the magnitude of the CHO mouth rinse effect may be reduced when the subjects are fed. This suggestion challenges the utility of this strategy to potentiate exercise performance in clinical and sports sciences scenarios, as athletes and exercise practitioners are frequently recommended to avoid a fasted state when exercising (38). A systematic review found that the post-prandial state did not moderate the CHO mouth rinse effects on exercise performance (2), thus indicating that muscle glycogen availability may be the primary factor influencing the ergogenic benefit of CHO mouth rinse. Therefore, future studies are necessary to reveal if rinsing the mouth with CHO solution effectively reverses a depleted muscle glycogen-reduced MIT performance.
Anecdotal data suggest a growing use of this strategy to improve performance in different endurance sports, such as cycling (8). The fact that CHO mouth rinse neither induces gastric discomfort during exercise (as no ingestion is necessary) nor belongs to the banned substances list, according to the World Anti-Doping Agency (WADA), may put this strategy as a promising ergogenic aid in endurance sports scenarios. Consequently, trainers, coaches, and cyclists may be interested in knowing if CHO mouth rinse really influences MIT outcomes used to prescribe and monitor endurance cycling training sessions such as power output and RPE at VT1 and VT2 intensities. The results of the present study challenged the use of MIT with this purpose, although some clarification should be addressed at this moment. In brief, we did not calculate the sample size required to detect likely CHO mouth rinse effects according to the most probable effect size. In an earlier study (2), we calculated that CHO mouth rinses had only a small, despite significant effect size on cycling performance when expressed as Hedge’s coefficient (g = 0.25; p = 0.02), so that a very large and unrealistic sample size of 128 participants would be necessary to detect an eventual beneficial effect on cycling exercise performance in a paired T-test design as used in the present study. The post-hoc calculations revealed only a small CHO mouth rinse effect size on maximal parameters such as PPO and time-to-exhaustion. However, the moderate-to-large effect sizes found in power output values at VT1 and VT2 suggest that a sample size with nine participants was probably underpowered to detect beneficial CHO mouth rinse effects in these submaximal performance parameters. Therefore, despite the methodologically sound challenge of the use of CHO mouth rinse in MIT outcomes such as PPO and time-to-exhaustion, future studies with greater sample sizes are required to confirm the effectiveness of this nutritional strategy in VT1 and VT2 in participants in a fed state.
5 Conclusion
Our results showed that rinsing the mouth with CHO before commencing an MIT was ineffective in reducing central fatigue, lowering the RPE, and improving MIT performance expressed as PPO and time-to-exhaustion. However, moderate-to-large effect sizes in power output values at VT1 and VT2 may suggest some beneficial CHO mouth rinse effects on these MIT outcomes.
Data availability statement
All relevant data are within the paper. The raw data of this article may be made available by request.
Ethics statement
The studies involving humans were approved by Ethical Committee of the University of São Paulo (#0023.0.342.000-10). The studies were conducted in accordance with the local legislation and institutional requirements. The participants provided their written informed consent to participate in this study.
Author contributions
FOP: Conceptualization, Data curation, Formal Analysis, Funding acquisition, Methodology, Writing – original draft, Writing – review & editing. FAP: Formal Analysis, Investigation, Writing – review & editing. CB: Data curation, Formal Analysis, Investigation, Writing – original draft. PF-A: Data curation, Formal Analysis, Investigation, Writing – original draft. KV: Formal Analysis, Investigation, Writing – original draft. EM: Conceptualization, Investigation, Writing – original draft, Writing – review & editing. AR: Conceptualization, Project administration, Supervision, Writing – review & editing. CU: Conceptualization, Resources, Supervision, Writing – review & editing.
Funding
The author(s) declare financial support was received for the research, authorship, and/or publication of this article. The CNPq-Brazil sponsored FOP (#307212/2022-0) and CU (#307072/2016-9 and # 303085/2015-0, respectively) and CAPES-Brazil sponsored EM. This study was supported by FAPESP-Brazil (# 2010/01317-0, # 2016/164963, and 2020/14730-4), CAPES Brazil (PRODOC 29/2010), and CNPq-Brazil (#407307/2021-4).
Conflict of interest
The authors declare that the research was conducted in the absence of any commercial or financial relationships that could be construed as a potential conflict of interest.
Publisher’s note
All claims expressed in this article are solely those of the authors and do not necessarily represent those of their affiliated organizations, or those of the publisher, the editors and the reviewers. Any product that may be evaluated in this article, or claim that may be made by its manufacturer, is not guaranteed or endorsed by the publisher.
References
1. Rollo, IAN , Williams, C , and Nevill, M . Influence of ingesting versus mouth rinsing a carbohydrate solution during a 1-h run. Med Sci Sports Exerc. (2011) 43:468–75. doi: 10.1249/MSS.0b013e3181f1cda3
2. Brietzke, C , Franco-Alvarenga, PE , Coelho-Júnior, HJ , Silveira, R , Asano, RY , and Pires, FO . Effects of carbohydrate mouth rinse on cycling time trial performance: a systematic review and meta-analysis. Sports Med. (2019) 49:57–66. doi: 10.1007/s40279-018-1029-7
3. Chambers, ES , Bridge, MW , and Jones, DA . Carbohydrate sensing in the human mouth: effects on exercise performance and brain activity. J Physiol. (2009) 587:1779–94. doi: 10.1113/jphysiol.2008.164285
4. Turner, CE , Byblow, WD , Stinear, CM , and Gant, N . Carbohydrate in the mouth enhances activation of brain circuitry involved in motor performance and sensory perception. Appetite. (2014) 80:212–9. doi: 10.1016/j.appet.2014.05.020
5. de Araujo, IE , Simon, SA , De, AIE , and Simon, SA . The gustatory cortex and multisensory integration. Int J Obes. (2009) 33:S34–43. doi: 10.1038/ijo.2009.70
6. Gant, N , Stinear, CM , and Byblow, WD . Carbohydrate in the mouth immediately facilitates motor output. Brain Res. (2010) 1350:151–8. doi: 10.1016/j.brainres.2010.04.004
7. Thomas, K , Goodall, S , Stone, M , Howatson, G , St Clair Gibson, A , and Ansley, L . Central and peripheral fatigue in male cyclists after 4-, 20-, and 40-km time trials. Med Sci Sports Exerc. (2015) 47:537–46. doi: 10.1249/MSS.0000000000000448
8. Pires, FO , Brietzke, C , Pinheiro, FA , Veras, K , De Mattos, ECT , Rodacki, ALF, et al. Carbohydrate mouth rinse fails to improve four-kilometer cycling time trial performance. Nutrients. (2018) 10:342. doi: 10.3390/nu10030342
9. Pires, FO , Dos Anjos, CA , Covolan, RJM , Pinheiro, FA , St Clair Gibson, A , Noakes, TD, et al. Cerebral regulation in different maximal aerobic exercise modes. Front Physiol. (2016) 7:253. doi: 10.3389/fphys.2016.00253
10. Painelli, VS , Brietzke, C , Franco-Alvarenga, PE , Canestri, R , Vinícius, Í , and Pires, FO . A narrative review of current concerns and future perspectives of the carbohydrate mouth rinse effects on exercise performance. SAGE Open Med. (2022) 10:205031212210981. doi: 10.1177/20503121221098120
11. Fraga, C , Velasques, B , Koch, AJ , Machado, M , Paulucio, D , Ribeiro, P, et al. Carbohydrate mouth rinse enhances time to exhaustion during treadmill exercise. Clin Physiol Funct Imaging. (2015) 37:17–22. doi: 10.1111/cpf.12261
12. Bentley, DJ , Newell, J , and Bishop, D . Incremental exercise test design and analysis: implications for performance diagnostics in endurance athletes. Sports Med. (2007) 37:575–86. doi: 10.2165/00007256-200737070-00002
13. Shephard, RJ . Tests of maximum oxygen intake. A critical review. Sports Med. (1984) 1:99–124. doi: 10.2165/00007256-198401020-00002
14. Kulaksız, TN , Koşar, ŞN , Bulut, S , Güzel, Y , Willems, MET , Hazir, T, et al. Mouth rinsing with maltodextrin solutions fails to improve time trial endurance cycling rerformance in recreational athletes. Nutrients. (2016) 8:2–15. doi: 10.3390/nu8050269
15. Jeffers, R , Shave, R , Ross, E , Stevenson, EJ , and Goodall, S . The effect of a carbohydrate mouth-rinse on neuromuscular fatigue following cycling exercise. Appl Physiol Nutr Metab. (2015) 40:557–64. doi: 10.1139/apnm-2014-0393
16. Carter, JM , Jeukendrup, AE , and Jones, DA . The effect of carbohydrate mouth rinse on 1-h cycle time trial performance. Med Sci Sports Exerc. (2004) 36:2107–11. doi: 10.1249/01.MSS.0000147585.65709.6F
17. Rollo, I , Cole, M , Miller, R , and Williams, C . Influence of mouth rinsing a carbohydrate solution on 1-h running performance. Med Sci Sports Exerc. (2010) 42:798–804. doi: 10.1249/MSS.0b013e3181bac6e4
18. De Pauw, K , Roelands, B , Cheung, SS , de Geus, B , Rietjens, G , and Meeusen, R . Guidelines to classify subject groups in sport-science research. Int J Sports Physiol Perform. (2013) 8:111–22. doi: 10.1123/ijspp.8.2.111
19. Borg, GA . Psychophysical bases of perceived exertion. Med Sci Sports Exerc. (1982) 14:377–81. doi: 10.1249/00005768-198205000-00012
20. Sinclair, J , Bottoms, L , Flynn, C , Bradley, E , Alexander, G , McCullagh, S, et al. The effect of different durations of carbohydrate mouth rinse on cycling performance. Eur J Sport Sci. (2014) 14:259–64. doi: 10.1080/17461391.2013.785599
21. James, RM , Ritchie, S , Rollo, I , and James, LJ . No dose response effect of carbohydrate mouth rinse on cycling time trial performance. Int J Sport Nutr Exerc Metab. (2016) 27:25–31. doi: 10.1123/ijsnem.2016-0111
22. Devenney, S , Collins, K , and Shortall, M . Effects of various concentrations of carbohydrate mouth rinse on cycling performance in a fed state. Eur J Sport Sci. (2016) 16:1073–8. doi: 10.1080/17461391.2016.1196735
23. Place, N , Casartelli, N , Glatthorn, JF , and Maffiuletti, NA . Comparison of quadriceps inactivation between nerve and muscle stimulation. Muscle Nerve. (2010) 42:894–900. doi: 10.1002/mus.21776
24. Kent-Braun, JA . Central and peripheral contributions to muscle fatigue in humans during sustained maximal effort. Eur J Appl Physiol Occup Physiol. (1999) 80:57–63. doi: 10.1007/s004210050558
25. Shield, A , and Zhou, S . Assessing voluntary muscle activation with the twitch interpolation technique. Sports Med. (2004) 34:253–67. doi: 10.2165/00007256-200434040-00005
26. Pageaux, B , Marcora, SM , Rozand, V , and Lepers, R . Mental fatigue induced by prolonged self-regulation does not exacerbate central fatigue during subsequent whole-body endurance exercise. Front Hum Neurosci. (2015) 9:67. doi: 10.3389/fnhum.2015.00067
27. Weston, SB , Gray, AB , Schneider, DA , and Gass, GC . Effect of ramp slope on ventilation thresholds and V̇O2peak in male cyclists. Int J Sports Med. (2002) 23:22–7. doi: 10.1055/s-2002-19267
28. Hopkins, WG , Marshall, SW , Batterham, AM , and Hanin, J . Progressive statistics for studies in sports medicine and exercise science. Med Sci Sports Exerc. (2009) 41:3–12. doi: 10.1249/MSS.0b013e31818cb278
29. Beelen, M , Berghuis, J , Bonaparte, B , Ballak, SB , Jeukendrup, AE , and van Loon, LJC . Carbohydrate mouth rinsing in the fed state: lack of enhancement of time-trial performance. Int J Sport Nutr Exerc Metab. (2009) 19:400–9. doi: 10.1123/ijsnem.19.4.400
30. Murray, KO , Paris, HL , Fly, AD , Chapman, RF , and Mickleborough, TD . Carbohydrate mouth rinse improves cycling time-trial performance without altering plasma insulin concentration. J Sports Sci Med. (2018) 17:145–52.
31. Ali, A , Yoo, MJY , Moss, C , and Breier, BH . Carbohydrate mouth rinsing has no effect on power output during cycling in a glycogen-reduced state. J Int Soc Sports Nutr. (2016) 13:19. doi: 10.1186/s12970-016-0131-1
32. Lane, SC , Bird, SR , Burke, LM , and Hawley, JA . Effect of a carbohydrate mouth rinse on simulated cycling time-trial performance commenced in a fed or fasted state. Appl Physiol Nutr Metab. (2013) 38:134–9. doi: 10.1139/apnm-2012-0300
33. Meeusen, R , Pires, FO , Lutz, K , Cheung, SS , Perrey, S , Rauch, HGL, et al. A role for the prefrontal cortex in exercise tolerance and termination. J Appl Physiol. (2016) 120:464–6. doi: 10.1152/japplphysiol.00363.2015
34. Ridderinkhof, KR , van den Wildenberg, WPM , Segalowitz, SJ , and Carter, CS . Neurocognitive mechanisms of cognitive control: the role of prefrontal cortex in action selection, response inhibition, performance monitoring, and reward-based learning. Brain Cogn. (2004) 56:129–40. doi: 10.1016/J.BANDC.2004.09.016
35. Carter, JM , Jeukendrup, AE , Mann, CH , and Jones, DA . The effect of glucose infusion on glucose kinetics during a 1-h time trial. Med Sci Sports Exerc. (2004) 36:1543–50. doi: 10.1249/01.MSS.0000139892.69410.D8
36. Fares, E-JJM , and Kayser, B . Carbohydrate mouth rinse effects on exercise capacity in pre-and postprandial states. J Nutr Metab. (2011) 2011:385962. doi: 10.1155/2011/385962
37. Trommelen, J , Beelen, M , Mullers, M , Gibala, MJ , van Loon, LJC , and Cermak, NM . A sucrose mouth rinse does not improve 1-hr cycle time trial performance when performed in the fasted or fed state. Int J Sport Nutr Exerc Metab. (2015) 25:576–83. doi: 10.1123/ijsnem.2015-0094
Keywords: cycling, endurance performance, supplementation, brain, V̇O2MAX
Citation: Pires FO, Pinheiro FA, Brietzke C, Franco-Alvarenga PE, Veras K, de Matos ECT, Rodacki ALF and Ugrinowitsch C (2024) Carbohydrate mouth rinse failed to reduce central fatigue, lower perceived exertion, and improve performance during incremental exercise. Front. Nutr. 11:1329074. doi: 10.3389/fnut.2024.1329074
Edited by:
Mark Elisabeth Willems, University of Chichester, United KingdomReviewed by:
Ashril Yusof, University of Malaya, MalaysiaMichael Green, Troy University, United States
Ben Krings, University of Wisconsin–Platteville, United States
Copyright © 2024 Pires, Pinheiro, Brietzke, Franco-Alvarenga, Veras, de Matos, Rodacki and Ugrinowitsch. This is an open-access article distributed under the terms of the Creative Commons Attribution License (CC BY). The use, distribution or reproduction in other forums is permitted, provided the original author(s) and the copyright owner(s) are credited and that the original publication in this journal is cited, in accordance with accepted academic practice. No use, distribution or reproduction is permitted which does not comply with these terms.
*Correspondence: Flávio O. Pires, cGlyZXNmb0B1c3AuYnI=