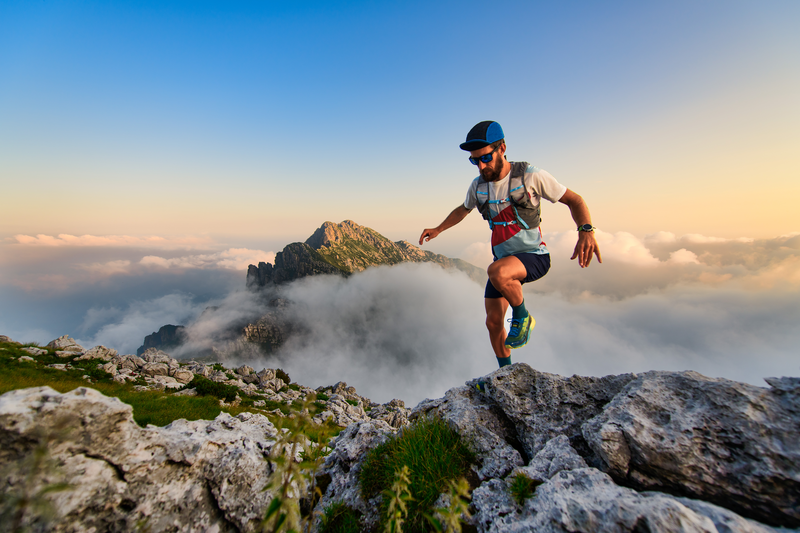
94% of researchers rate our articles as excellent or good
Learn more about the work of our research integrity team to safeguard the quality of each article we publish.
Find out more
ORIGINAL RESEARCH article
Front. Nutr. , 06 February 2024
Sec. Nutrition and Food Science Technology
Volume 11 - 2024 | https://doi.org/10.3389/fnut.2024.1325886
This article is part of the Research Topic Nutrition and Flavor During Food Processing: Change Patterns and Mechanisms View all 10 articles
To develop food flavorings with a delicious taste and an anti-oxidation effect, in this study, the glucose Maillard reaction was used for hydrolysates of Urechis unicinctus. The various biological activities of Maillard reaction products (MRPs) and their antioxidant capacity were evaluated. The results showed that the unique fishy odor substances of seafood in MRPs were reduced, indicating that the Maillard reaction improved the flavor of the hydrolysate of Urechis unicinctus. Meanwhile, MRPs exhibited more competitive radical scavenging activities compared to the hydrolysate. Moreover, MRPs demonstrated a considerable potential to protect against 2,2′-Azobis (2-methylpropionamidine) dihydrochloride (AAPH)-induced oxidative stress in a cell model in vitro and in a zebrafish model in vivo. Finally, a novel food flavoring was produced with MRPs as raw material, while the sensory qualities were deemed acceptable. In consequence, during industrial production, MRPs of Urechis unicinctus hydrolysate act as a high-quality raw material for functional flavorings and provide an effective way for the utilization of marine resources.
Flavorings are usually food ingredients that are used to be added in small quantities to other foods to improve their flavor (deodorize, enhance freshness, relieve grease, etc.) (1). The flavoring industry has a wide range of products and a large sales volume, especially in China and other south-central Asian countries. The flavoring industry is developing rapidly, and the annual production of flavoring can be more than ten million tons. Especially in recent years, with the rapid development of society, people’s living standards are improving at lightning speed, and the majority of consumers on the requirements for seasonings are also on the rise. Compared with the popular flavorings sold in the market now, people’s requirements for flavorings are not only reflected in the color and flavor but also in being natural and environmentally friendly, green and safe, with specific nutritional properties. Therefore, seasonings with certain functions have become a hot spot for research in the food industry and the mainstream development direction of China’s seasoning industry. Aquatic seasoning is becoming more and more popular because it retains the original unique flavor of aquatic products (2), and contains rich amino acids, nucleotides, small peptides, and other active ingredients, and it has shown a rapid growth trend in international market demand. The production and consumption of aquatic seasonings in foreign countries are mainly distributed in Southeast Asia, Japan, and the northern Philippines. At present, seafood seasonings account for about 60% of the Japanese seasoning market, and gradually replace traditional seasonings such as monosodium glutamate in Southeast Asia. Aquatic seasoning has gradually become the mainstream development direction of international condiments. China is rich in marine resources, and the development of functional marine food is the strategic goal of our country’s future development. Making full use of marine animal protein resources is an urgent problem for the aquatic product processing industry.
Urechis unicinctus, also known as the sea intestine, is a long cylindrical annelid. Its protein content is rich, accounting for about 71.01% of its dry weight, which is much higher than that of other aquatic products (mussel protein accounted for 58.5% and oyster protein accounted for 47.21%), and it also contains peptides, polysaccharides, and other physiologically active substances (3), which have the hypoglycemic effect, antihypertensive and antioxidant activity (4, 5). The muscle hydrolysate of Urechis unicinctus contained 18 amino acids, comprising five types of umami amino acids and eight types of necessary amino acids. The composition of amino acids was reasonable, and the umami amino acids representing 53.63% of the total amino acids. Therefore, Urechis unicinctus is an excellent source for the development of functional condiments. What’s more, the processing and utilization of Urechis unicinctus in China and other Southeast Asian countries is still in its infancy, mainly in the form of cooking. There are relatively few studies on flavor, especially in the development of seasonings. The development of seafood seasoning is one of the effective way to realize the high value utilization of marine resources, so the preparation of Urechis unicinctus seafood seasoning products has become the trend of the Urechis unicinctus industry. However, in general, as the freshness of seafood decreases, it will produce a unique smell, which may have a negative impact on the flavor of Urechis unicinctus. Therefore, improving this bad flavor is a prerequisite for the development of Urechis unicinctus seasoning. There are many methods to improve this unpleasant odor, such as adsorption, extraction, biological deodorization, and the Maillard reaction (MR), among which MR method is deeply favored by the food industry. The use of MR technology to treat the protein hydrolysate of aquatic products is a simple and low-cost process, and the purpose of deodorization and flavor enhancement can be achieved at the same time.
The Maillard reaction (MR), additionally referred as non-enzymatic browning, is a non-enzymatic reaction that takes place between the carbonyl group of reducing sugar and the amino group of amino acids, peptides, or proteins (6, 7), which can improve the disagreeable taste of food (8–12). The Maillard reaction has been extensively used in food processing, flavor chemistry, and other realms (13–15) and is essential for increasing the flavor and color of food products, resulting in distinctive color and flavor in pastry, cocoa bakery, and meat processing products (16). The Maillard reaction can not only improve flavor but also effectively improve antioxidant activity (17). For instance, Guérard et al. (18) reported that when casein peptone and cod offal hydrolysates were heated in the presence of glucose, the free radical scavenging effect increased by 75%. Some researchers have broadly divided the factors influencing the change in antioxidant activity into two categories: the type of reactants and the generation of some reaction products. Firstly, Hwang et al. (19) studied MRPs of more than 20 amino acids under the same conditions and found that MRPs produced by alanine, tyrosine, tryptophan, and asparagine had stronger free radical scavenging ability. Second, some antioxidant compounds are formed after the Maillard reaction, such as phenolics and acids (20–22). Consequently, we attempted to enhance the flavor and antioxidant capacity of the hydrolysate of Urechis unicinctus by means of the Maillard reaction, which supplied a foundation for the development of functional seasonings.
In this research, the enzymatic solution of Urechis unicinctus was reacted with glucose at 120°C for 2 h to prepare Maillard reaction products (MRPs). Compared to the hydrolysates, the components of MRPs may change. To evaluate these changes, high-performance liquid chromatography (HPLC) and gas chromatography–mass spectrometry (GC–MS) were utilized to detect the amino acid composition and flavor substances in the hydrolysates and MRPs (23). The antioxidant property of MRPs was evaluated by chemical approaches as well as the construction of L02 cells and zebrafish oxidative stress models. In addition, functional seasonings were prepared based on the Maillard reaction products of Urechis unicinctus hydrolysates.
Urechis unicinctus were obtained from Yantai Laishan District Aquatic Products Market (Shandong, China). Fetal bovine serum (FBS) was acquired from Zhejiang Tianhang Biotechnology Co., Ltd. (Zhejiang, China). 3-(4,5-dimethyl-2-thiazolyl) -2,5-diphenyl-2H-tetra-zolium bromide (MTT), dimethyl sulfoxide (DMSO), Acridine Orange, 1,3-bis (diphenylphosphine) propane (DPPP), and 2,2′-Azobis (2-methylpropionamidine) dihydrochloride (AAPH) were procured from Sigma-Aldrich (St Louis, MO, USA). Amino Acids Mixture Standard Solution was obtained from KGaA (Darmstadt, Germany). GSH assay kits and MDA assay kits were bought from Nanjing Jiancheng Bioengineering Institute (Nanjing, China). BCA Protein Assay Kit was supplied by the Beyotime Institute of Biotechnology (Beijing, China). Dulbecco’s Eagle’s medium (DMEM), phosphate-buffered solution (PBS, pH 7.2–7.4, 0.01 M), L-ascorbic acid, 2′,7′-dichlorodihydrofluorescein diacetate (DCFH-DA), HO• scavenging activity assay kit, DPPH• scavenging activity assay kit, ABTS• scavenging activity assay, and alkaline protease were procured from Solarbio Science & Technology Co., Ltd. (Beijing, China). Pepsin was obtained from Macklin Biochemical Co., Ltd. (Shanghai, China). Trypsin, papain, and flavourzyme were acquired from Aladdin Reagent Co., Ltd. (Shanghai, China). Salt, sodium glutamate, disodium nucleotide, yeast extract, and white granulated sugar were obtained from Yantai New world Department Store (Shandong, China).
After the viscera were removed, the fresh Urechis unicinctus was washed and stored in a refrigerator at −80°C. The cryopreserved Urechis unicinctus was placed in a freezing dryer. After 24 h, it was ground and crushed (24). A measured amount of distilled water was added and the pH was adjusted. After the hydrolysis was completed, the enzyme was killed at 95°C, cooled, centrifuged, and the supernatant was taken. The free amino acid was measured by the neutral formaldehyde method, and the total nitrogen content was detected by the Kjeldahl method (25). Based on the degree of hydrolysis, three proteases with the highest degree of hydrolysis were selected. Using the software Design-Expert1, the Simplex lattice experimental design method was utilized to identify the optimal ratio of compound protease. Then the optimal ultrasonic conditions and enzymatic hydrolysis conditions (4) were optimized by single factor test and response surface experiment. The enzymatic hydrolysate prepared under the optimal conditions was freeze-dried and placed in a refrigerator at −20°C for subsequent use.
Under the optimal conditions MRPs of the hydrolysate of Urechis unicinctus were prepared from the lyophilized powder of the enzymatic hydrolysate. The powder (10 g) was solved in 100 mL of water at a proportion of 1:10 (w/v), and then the glucose (4 g) was incorporated into the Maillard reaction mixture. Followed by heating at 120°C for 2 h (26), the samples were promptly freeze-dried and kept at −20°C for subsequent use.
The composition of amino acids was tested by HPLC utilizing a C18 column (5 μm, 4.6 × 250 mm). With this method, the composition of amino acids was analyzed separately for the Maillard reaction solution and the enzymatic digestion solution. The chromatographic conditions were as follows: Test wavelength was 254 nm, determination of column temperature was 40°C, injection volume was 10 μL, mobile phase: A phase was 0.1 mol/L sodium acetate solution (pH 6.50): acetonitrile = 93: 7, B phase was water: acetonitrile = 20: 80, gradient elution procedure: 0–0.01 min, 100% A; 0.01–35 min, 100 ~ 0% A; 35–42 min, 100% B; 42–45 min, 0–100% A, flow rate was 1.0 mL/min, and the total elution time was 60 min.
The changes in flavor substances of the hydrolysate and MRPs were determined separately by GC–MS. The samples were subjected to solid-phase microextraction at 80°C for 40 min and sorption at 250°C for 3 min, which were tested afterwards. The GC–MS analysis was carried out on a DB-5MS column under the following conditions: The temperature of the injection port was 250°C, the split ratio was 10:1, and the carrier gas was high-purity helium 6.0 flowing at a rate of 1 mL/min. The temperature program was adapted as follows: maintain 40°C for 2 min, rise to 200°C at 6°C/min, then rise to 300°C at 15°C/min for 2 min. The temperature of the ion source was 220°C, the surface temperature was 280°C, and the m/z scan range was 33 to 500 for the full scan.
The method of Li et al. (27) was utilized to evaluate the DPPH radical scavenging capacity of hydrolysates and MRPs with minor adjustment. DPPH working solution is obtained by dissolving DPPH powder in ethanol. The 20 μL sample was combined with 180 μL DPPH working solution (0.2 mM) and incubated in darkness at room temperature for 30 min. Subsequently, the absorbance of the mixed solution was detected at 517 nm utilizing a microplate with L-ascorbic acid as a positive control. The DPPH free radical scavenging rate is calculated as follows:
DPPH radical scavenging activity (%) = {[Ablank − (Asample − Acontrol)]/ Ablank} × 100%.
A minor modification was adapted to the procedure reported by Tan et al. (28) for sake of assessing ABTS radical scavenging capacity. The ABTS+ solution was formulated by combining 2.45 mM potassium persulfate solution and 7 mM ABTS mother liquor in an equal volume, which was then incubated for 12–16 h at room temperature away from light. PBS was applied to dilute the solution to an absorbance of 0.70 ± 0.02 at 734 nm, and the ABTS working solution was obtained. Subsequently, 200 μL of ABTS working solution was combined with 8 μL of the sample solution. The mixture was incubated under darkness at room temperature for 10 min before the absorbance was tested at 734 nm with L-ascorbic acid as the positive reference. The results of the calculations are expressed as the percentage of the scavenging rate using the following formula:
HO• scavenging activity was determined with reference to the testing means used by Liu et al. (29) with light revision. The HO• radical was produced by FeSO4 and H2O2, which was detected by its ability to hydroxylate salicylate. The reaction system (0.2 mL) included 50 μL FeSO4 (9 mM), 50 μL H2O2 (9 mM), 50 μL sodium salicylate (9 mM), and 50 μL different concentrations of hydrolysate or MRPs. After 1 h of incubation at 37°C, the absorbance at 520 nm was tested, and ascorbic acid was utilized as a positive control. The following is how the percentage elimination effect was computed:
L02 cells were cultivated in DMEM with 1% antibiotics (100 U/mL penicillin and 100 g/mL streptomycin) and 10% fetal bovine serum (FBS) at 37°C in a moist environment with 5% CO2. 0.25% trypsin was applied to subculture the cells when their confluence reached 80–90%.
The viability of L02 cells induced by AAPH was assessed by the MTT method. The cells (1 × 105/mL) were inoculated into 96-well plates and cultivated for 24 h. After that, the medium was renewed, and the cells were cultured in various doses of MRPs for 1 h. Afterwards, the cells were treated for 16 h in the absence or presence of AAPH (15 mM) (30). The final step was to incorporate 10 μL MTT solution (5 mg/mL). After 3 h of incubation, the purple formazan crystals were resolved in dimethyl sulfoxide (150 μL). With the use of a microplate reader (Molecular Devices, San Jose, CA, USA), the absorbance was measured at 570 nm.
Cells were inoculated in 96-well plates at a density of 1 × 104 per well and grown for 24 h. Cells were subsequently recieved treatment with AAPH (15 mM, 16 h) and pre-incubated with MRPs for 1 h. After that, according to the instructions for the ROS detection kit, the cells were covered with DCFH-DA (10 mM). After 30 min, the intracellular ROS content was assessed by quantifying the fluorescence emitted by DCFH-DA oxidation to 2′, 7′-dichlorofluorescein. A microplate reader was applied to examine the fluorescence intensity at wavelengths of 485 nm for the excitation and 525 nm for the emission.
To estimate the antioxidative effect of the MRPs, we constructed a zebrafish model of oxidative stress. Zebrafish embryos were cultivated in 6-well plates. The 24 hpf zebrafish embryos were firstly treated with various doses of MRPs (0, 25, 50, 100, 200, 400 μg/mL) with the aim of determining the concentration of MPRs used in subsequent experiments. After that, the concentration of AAPH-induced oxidative damage in 24 hpf zebrafish embryos was screened.
The selected 24 hpf zebrafish embryos were placed in 6-well plates, and the water was replaced once a day. The number of zebrafish heartbeats per minute was counted by microscope after 72 hpf of continuous administration and oxidative induction, and the average value was calculated.
Specific fluorescent probes were, respectively, used to stain zebrafish embryos with the purpose of detecting zebrafish cell mortality (acridine orange), intracellular ROS production rate (DCFH-DA), and lipid peroxidation production rate (DPPP). Zebrafish were rinsed with fresh embryonic medium after being incubated for a period of time in a dye-containing medium, then they were anesthetized. After that, we observed with a fluorescence microscope (Leica DMi8, Wetzlar, Germany), and images were taken with a digital camera. Finally, employing Image J software (National Institutes of Health, Bethesda, MD, USA), the fluorescence intensity of individual zebrafish was quantified.
After 96 h of exposure to MRPs in the presence or absence of AAPH, the spontaneous movement of embryos was tracked for 10 min in a 96-well plate at 27.5 ± 1°C between 9 a.m. and 12 a.m. under particularly suitable light conditions. The zebrafish behavior tracking system (Danio Vision, Noldus, Wageningen, Netherlands) was used to record the number of tail coil alternations every 10 min, and the spontaneous movement distance of 7 dpf embryos per minute was calculated.
The larvae were cleaned three times using pre-cooled PBS, and then 10 larvae were homogenized. After centrifugation at 4°C, based on the manufacturer’s instructions, the levels of malondialdehyde (MDA) and reductive glutathione (GSH) were detected by the assay kit supplied by Nanjing Jiancheng Bioengineering Institute (Nanjing, China).
To obtain high-quality seasonings, the enzymatic hydrolysate was used as raw material to prepare seasoning by adding salt, sodium glutamate, disodium nucleotide, yeast extract, and white granulated sugar. For purpose of exploring the concentration of raw materials in the composite seasoning, we first determined the optimum concentration of each raw material by single factor test. Then, MRPs, salt, white granulated sugar, and sodium glutamate were used as factors to do four-factor and three-level orthogonal experiments to determine the best compounding ratio. The ingredients were mixed in proportion, and the seafood seasoning was obtained after canning and sterilizing.
The evaluation of sensory was carried out in the way presented by Fu et al. (31) with minor changes. In the sensory evaluation process, the explanation of the sensory panel should be included: demographic characteristics of the panel, recruitment and training characteristics, and performance analysis results (32).
Select sensory evaluators based on interest, sensitivity, and familiarity with the sample. Sensory evaluators are not allergic to seafood products and pass olfactory and taste sensitivity tests. Sensory training was conducted for sensory evaluators a total of eight times, for about two hours each time, to establish consistent descriptive terms, improve the evaluators’ perception of descriptive words, and standardize the use of sensory scales. In the subsequent simulation test, 10 sensory evaluation team members (5 females and 5 males) were selected based on the difference of no more than 20% between individual team members.
Sensory evaluation indexes included taste, flavor, saltiness, total acceptance and so on. The samples were placed in a 50 mL sanitary cup, and the team members tasted the samples and scored them. Before evaluating each sample, team members were asked to rest for 30 s and were given clean water to rinse their mouths. In each test, 10 team members tested different samples three times.
All the data were displayed as means ± SD of three to six separate experiments. GraphPad Prism 8 software (GraphPad Software, San Diego, CA, USA) was applied to conduct statistical analyses using one-way ANOVA. p < 0.05 was deemed significant.
Under the optimum conditions, trypsin, alkaline protease, papain, pepsin, and flavorzyme were used to hydrolyze Urechis unicinctus. The three proteases with the highest degree of hydrolysis were trypsin, flavorzyme, and alkaline protease (Supplemenatry Figure S1). Then the simple lattice experimental design was used to obtain the best ratio of complex protease: trypsin: alkaline protease = 21.2: 8.8. Finally, the ultrasonic enzymolysis conditions were determined by the single factor experiment and the response surface experiment. The ultrasonic enzymolysis time was 54.95 min, the ratio of liquid to material was 52: 1, and the amount of enzyme was 3.17%. The optimum enzymatic hydrolysis conditions were that the enzymatic hydrolysis time was 3.16 h, the pH was 8.58, and the enzymatic hydrolysis temperature was 50.48°C. At this time, the degree of hydrolysis (DH) was 34.19%.
The hydrolysate of Urechis unicinctus was modified by the Maillard reaction when mainly amino acids were engaged in the reaction, which would lead to a significant change in amino acid composition. In this experiment, the amino acid composition of Urechis unicinctus hydrolysate was established by HPLC before and after the Maillard reaction. The changes in amino acid composition are shown in Table 1, where the total amino acid content of the hydrolysate of Urechis unicinctus decreased from 486.65 mg to 310.7 mg after MR, which occurred between reducing sugars and amino acids and gave the food its unique flavor. Studies have shown that bitter amino acids (leucine, lysine, valine, methionine, etc.) were consumed in large quantities during the Maillard reaction (33), so the content of bitter amino acids in the Maillard reaction products was significantly reduced.
Table 1. The amino acid composition of Urechis unicinctus hydrolysate and Urechis unicinctus hydrolysate MRPs.
GC–MS analysis was employed to study the changes of flavor substances in MRPs of enzymatic hydrolysates and hydrolysates of Urechis unicinctus. By comparing with the mass spectrometry database, we speculated on the types of flavor substances contained in the enzymatic hydrolysates and MRPs of enzymatic hydrolysates and analyzed the relative content changes of flavor substances in the enzymatic hydrolysate and the enzymatic hydrolysate MRPs with 2-methyl-3-heptanone as internal standard substance. It can be observed from Table 2 that the content of aldehydes decreased significantly after MR (from 14.21 to 3.33%), among which heptanal, hexanal, octanal, and 2-methyl-2-pentenal all decreased to 0%, nonanal decreased from 3.42 to 0.89%, and acetaldehyde decreased from 2.84 to 0.32%. The content of ketones also decreased (from 6.2 to 1.27%), especially acetone, nonanone, and undecane, which decreased to 0%.
Table 2. Comparison of flavor substances between Urechis unicinctus hydrolysate and hydrolysate MRPs.
The content of acid compounds raised after the Maillard reaction (from 24.91 to 34.9%), and the proportion of acid compounds contained in the enzymatic hydrolysate increased to a certain extent. Alcohols also increased from 16.54 to 27.88%, and the most significant change was ethanol (from 0.48 to 5.22%). In addition, phenolic compounds were achieved from nothingness to being, maltol (from 0 to 5.32%) and 5-methyl-2-isopropylphenol (from 0 to 2.23%) increased significantly.
The antioxidant properties of MRPs and Urechis unicinctus hydrolysate were determined by three methods, and it was observed that the scavenging ability of hydrolysates enhanced after MR and showed a concentration dependence (Figures 1A–C). In addition, it was shown that the difference between the antioxidant property of MRPs and the hydrolysate increased with growing concentration. For example, the enzymatic digest of 4 mg/mL showed no apparent difference after MR, and then it became larger. The results obtained by the three methods are consistent with each other, so they can be good proof of the enhanced antioxidant activity of the hydrolysate after the Maillard reaction.
Figure 1. Comparison of antioxidant ability between Urechis unicinctus hydrolysate and hydrolysate MRPs. DPPH• (A), ABTS• (B), and OH• (C) scavenging activity of hydrolysate and hydrolysate MRPs. n = 3, Data are expressed as mean ± SD.
To verify the antioxidant activity of the Urechis unicinctus hydrolysate MRPs, we established an AAPH-induced damage model based on previous studies (34, 35). AAPH is a free radical promoter that disintegrates at 37°C to form two free radicals with carbon atoms as the core. In the presence of oxygen, this triggers a free radical chain reaction to generate ROS, leading to cell damage. Cell viability after treatment with varying concentrations of MRPs was first measured using the MTT assay. MRPs did not show cytotoxicity at concentrations below 200 μg/mL compared to the control group (Figure 2A). MRPs at non-toxic concentrations were utilized in subsequent experiments to detect their protective effects against oxidative damage. Cells were incubated with varying doses of MRPs samples (0, 25, 50, 100, and 200 μg/mL) for 1 h, preceded by the addition of 15 μM AAPH for 16 h. As shown in Figure 2B, cell viability was drastically decreased in the AAPH group, and pretreatment of MRPs led to a positive response to the cell viability of oxidative stress injury in a concentration-dependent manner. Particularly, the cell viability treated with 200 μg/mL MRPs was markedly raised compared to the AAPH group.
Figure 2. MRPs attenuated AAPH-induced cell injury and ROS generation in L02 cells. (A) MTT assay to verify whether different concentrations of MRPs (25, 50, 100, 200 μg/mL) had cytotoxicity. (B) MRPs were added 1 h before AAPH. Effect of MRPs on AAPH-induced cell viability. (C) Effect of MRPs on the ROS levels of AAPH-induced L02 cells. n = 4, Data are expressed as mean ± SD. * p < 0.05, ** p < 0.01 vs. AAPH group, ns, not significant.
In addition, endo cellular ROS generation was assessed employing DCFH-DA fluorescence staining. In Figures 3B,C, the fluorescence intensity of the AAPH-exposed group was 98,963, which was dramatically higher than that in the control group. As expected, pretreatment with different dosages of MRPs remarkably reduced ROS overaccumulation in a dose-dependent manner, with fluorescence intensities reduced to 311,060, 253,936, 232,131, and 223,833, respectively. The results suggest that Urechis unicinctus hydrolysate MRPs can protect L02 cells by attenuating oxidative damage. The Urechis unicinctus hydrolysate MRPs could be a potential biomolecular candidate to inhibit cell oxidative stress and ROS generation.
Figure 3. Construction of AAPH-induced oxidative stress model in vivo. The effects of different concentrations of AAPH on the survival rate (A), yolk sac size (B,C), ROS production rate (D,E) and cell death rate (F,G) of zebrafish. n = 10, Data are presented as means ± SD and analyzed by one-way ANOV A followed. * p < 0.05, ** p < 0.01 vs. control, ns, not significant.
The antioxidant properties of an active substance can be verified by in vitro chemical methods, but these methods are not sufficient to support whether the substance has antioxidant properties in vivo. Therefore, an in vivo model of zebrafish was further used to investigate the protective impact of MRPs on AAPH-induced oxidative strain. The mortality rate of zebrafish embryos increased with increasing AAPH concentration in a dose-dependent manner. The survival rate of zebrafish embryos decreased to less than 50% when induced with 25 mM and 30 mM doses and was unsuitable for further index testing (Figure 3A). Zebrafish yolk sac size also increased with increasing AAPH concentration, showing distinct enlargement at 20 mM (Figure 3B). The fluorescence detection results of cellular ROS formation and cell death in zebrafish showed that AAPH induction could increase the ROS level in zebrafish, and the ROS generation rate was higher with increasing induction concentration (Figures 3B,C). The cell death rate of zebrafish also increased sharply with the increase of concentration (Figure 3D). In summary, we selected 20 mM AAPH to establish a zebrafish oxidative stress induction model for subsequent evaluation of the antioxidant property of MRPs.
The following methods were utilized to observe the impact of MRPs at various doses (25, 50, 100, 200 and 400 μg/mL) on the viability of zebrafish. MRPs in the dose range of 0–200 μg/mL had little effect on the zebrafish embryo survival rate, and the survival rate decreased when the concentration attained 400 μg/mL (Figure 4A). The heartbeat rate of zebrafish was significantly increased at a dose of 400 μg/mL compared to the control group (Figure 4B). As demonstrated in Figures 4B,C, in comparison with the control group, there was no obvious change in zebrafish cell mortality after treatment with MRPs below 200 μg/mL, while MRPs at 400 μg/mL caused a significant increase. Therefore, 25, 50, 100, and 200 μg/mL of MRPs were chosen for the subsequent experiments.
Figure 4. Screening of MRPs concentration. The effects of different concentrations of MRPs on the survival rate (A), heart rate (B) and cell death rate of zebrafish (C,D). n = 10, Data are presented as means ± SD and analyzed by one-way ANOV A followed. * p < 0.05, ** p < 0.01 vs. control, ns, not significant.
Oxidative injury may eventually result in cell death, overproduction of ROS and lipid peroxidation. In this study, the improved impacts of MRPs against AAPH-induced cell death, reduced locomotor activity, ROS production, and lipid peroxidation in zebrafish were examined. As indicated in Figures 5A,B), in comparison with the model group, zebrafish embryo survival and cell mortality were markedly reduced when MRPs were administered in a dose-dependent manner. The zebrafish heart rate and zebrafish yolk sac size could reflect the survival status of zebrafish. Treatment with MRPs reversed the increased heart rate and enlarged yolk sac size of zebrafish caused by AAPH. After treatment with only AAPH, the total distance of zebrafish movement was obviously decreased. However, MRPs showed a good improvement in locomotor activity in zebrafish (Figures 5B,C). The effects of MRPs on AAPH-induced ROS production and lipid peroxidation levels are indicated in Figures 5D,E), respectively. When zebrafish embryos were treated with MRPs in advance of AAPH treatment, a dose-dependent reduction in ROS and lipid peroxidation production was observed. Moreover, MRPs pretreatment ameliorated the increased MDA content and reduced GSH content in zebrafish caused by AAPH treatment (Figures 5F,G). These data indicated that MRPs pretreatment inhibited ROS generation and lipid peroxidation to protect zebrafish from oxidative injury.
Figure 5. Protective effects of MRPs against oxidative stress in zebrafish model. Effect of MRPs on the heart rate (A), survival rate (B), and yolk sac size (C) in AAPH-induced zebrafish embryos. Inhibitory effect of MRPs on AAPH-induced ROS production (D,E), lipid peroxidation (F,G), and cell death rate (H,I) in zebrafish embryos. They were measured after staining with DCFH-DA, DPPP, and acridine orange followed by image analysis and fluorescence microscopy. (J,K) Distance traveled of zebrafish larvae at 96 hpf after exposure to MRPs absence or presence of AAPH. Effect of MRPs on MDA (L) and GSH (M) in zebrafish. n = 10, Data are presented as means ± SD and analyzed by one-way ANOV A followed. * p < 0.05, ** p < 0.01 and *** p < 0.001 vs. AAPH group, ns, not significant.
There is no doubt that the most critical factor in seasoning is flavor. The single-factor experiment showed that the change of I + G and yeast extract content had little effect on the sensory scores. The main and secondary factors affecting the sensory score were salt, MRPs, sodium glutamate, and white sugar (Figure 6). The content of I + G was 0.8%, and yeast extract was 1.5% for subsequent experiments. With MRPs, salt, white sugar, and sodium glutamate as factors, the orthogonal experiment of four factors and three levels (Table 3) was conducted to determine the best formula process. The data showed that the optimal combination was MRPs (11%), sugar (11%), salt (25%), sodium glutamate (25%), I + G (0.8%), and yeast extract (1.5%) (Table 4). Finally, a high-end seasoning with hygienically qualified, white powder, unique seafood flavor, and pleasant taste was obtained.
With the improvement of people’s living standards, aquatic condiments with health care functions are becoming more popular (2). Urechis unicinctus, also known as “naked sea cucumber,” has the potential to prepare seafood condiments because of its low fat and high protein content. However, the hydrolysate of Urechis unicinctus has a bitter taste that is unique to seafood. MR can improve the flavor and color of food and in some cases, can enhance protein functionality (36, 37). Therefore, we attempted to use the enzymatic hydrolysate of Urechis unicinctus for MR with glucose and found that the bad flavor of the enzymatic hydrolysate was improved after the reaction. MRPs showed prosperous antioxidant capacity and the ability to alleviate oxidative damage in vitro and in vivo. Then, an umami-rich, nutritious, and healthy seasoning was prepared from the Maillard reaction product of Urechis unicinctus hydrolysate.
Urechis unicinctus has the potential to develop functional seafood seasonings due to its rich protein content, reasonable amino acid composition, and biological activities such as antioxidant and hypoglycemic. However, the enzymatic hydrolysate of Urechis unicinctus has the characteristic fishy flavor of aquatic products. Therefore, improving this unsatisfactory flavor has become the key to the preparation of Urechis unicinctus seasoning. The Maillard reaction treatment of aquatic product protein hydrolysates can achieve the purpose of deodorization and aroma enhancement, which is favored by the food industry. Therefore, firstly, in this study, the hydrolysate was reacted with glucose at 120°C for 2 h to obtain the MRPs of Urechis unicinctus hydrolysate. The total amino acid content decreased after the reaction, which was to be attributed to the fact that MR takes place mainly between reducing sugars and amino acids, giving the food a unique flavor (7, 37). Among them, leucine, lysine, methionine, valine, and other amino acids that produce a bitter taste (38, 39) were apparently reduced, which was similar to the findings obtained by Lan and Liu et al. in the study of the xylose-soybean peptide maillard reaction system (40). Hexanal, heptanal, octanal, nonanal, etc., which are responsible for the fishy smell of seafood (41, 42), were markedly reduced after MR, which was consistent with the study of Zhao et al. (43). After MR, the content of short-chain fatty acids such as acetic acid and propionic acid increased, and their thresholds were lower, which had a cheese flavor and could play a certain modification role. Additionally, the content of acetic acid and propionic acid increased after MR. Alcohols are mainly derived from amino acid reduction and fat oxidation, which usually have plant fragrances (44). Phenolic compounds are beneficial in encouraging the release of fishy odor components, reducing the fishy odor of aquatic products significantly (45), and harmonizing the overall flavor of the food. In summary, the findings indicated that MR enhanced the flavor of the hydrolysates of Urechis unicinctus.
Simultaneously, the MRPs showed more competitive free radical scavenging capacity in contrast to that of the hydrolysates. We speculated that the increase in free radical scavenging capacity might be related to changes in the composition of the hydrolysates, especially the increase in acids and phenolics in the MRPs. The antioxidant activity of phenolics and acids has been widely reported. Dini et al. (46) added 5-methyl-2-isopropyl phenol to polylactic acid at a certain concentration, and found that its antioxidant capacity in water was enhanced. Wang et al. (47) found that the antioxidant property of chitosan modified by fumaric acid increased from 63 to 85% in ionic liquid solution by the DPPH free radical scavenging method. Furthermore, oyster protein hydrolysate showed superior free radical scavenging ability due to the increased phenolic and acid content after MR (48). Therefore, we concluded that MR improved the antioxidant properties of the hydrolysates, and the change in composition was essential for the increase in antioxidant capacity.
L02 cells have been validated as an ideal cellular model for evaluating antioxidant activity (49, 50). For the sake of further verifying the antioxidant capacity of MRPs, we utilized L02 cells to evaluate their intracellular antioxidant efficacy. AAPH is a free radical initiator applied extensively in mimicking oxidative stress states (51). Therefore, AAPH was employed in this study to induce oxidative strain L02 cells. The viability of L02 cells exposed to AAPH was markedly reduced. This might be due to the fact that cellular damage attributed to ROS-induced oxidative stress usually impairs biomolecular functions and results in cell death (52, 53). However, cell death was inhibited with MRPs, indicating that MRPs can protect cells from AAPH-induced cytotoxicity. We utilized an oxidant-sensitive DCFH-DA fluorescence sensor to quantify the level of ROS generation in cells and determined that MRPs block AAPH-induced ROS generation and subsequent oxidative stress. These findings demonstrated that MRPs played a protective role in ROS-induced oxidative stress, thereby reducing cellular damage.
In accordance with recent reports, zebrafish can be utilized as a quick and straightforward model to evaluate anti-oxidative stress activity in vivo (34, 35). As a consequence, in this research, we made use of the zebrafish oxidative damage model to explore the antioxidant effect of MRPs in vivo. The outcomes indicated that AAPH dramatically raised cell death and ROS levels in zebrafish embryos. In contrast, MRPs suppressed this phenomenon. In the research, AAPH treatment significantly increased lipid peroxidation in zebrafish embryos, which was due to the fact that lipid peroxidation might be a type of cellular harm brought on by free radicals (54). However, MRPs effectively inhibited the formation of this lipid peroxidation. Accordingly, MRPs also exhibited satisfactory effects in inhibiting ROS overproduction and attenuating oxidative damage in a zebrafish model in vivo, further validating the antioxidant capacity and nutritional value of MRPs.
As stated previously, MRPs of the Urechis unicinctus hydrolysates showed strong aroma and favorable antioxidant effect, which were high-quality raw materials for preparing functional seafood seasonings. Consequently, we utilized MRPs as the raw material to determine the best formula by process optimization and sensory evaluation, and a high-end functional seasoning with a strong aroma and the antioxidant effect was prepared.
In summary, the Maillard reaction can improve the flavor of Urechis unicinctus hydrolysate and enhance its antioxidant property. Next, the MPRs of Urechis unicinctus hydrolysates can be utilized for industrial production of functional seafood seasonings and also provide an effective way to realize the high-value utilization of marine resources.
The original contributions presented in the study are included in the article/Supplementary material, further inquiries can be directed to the corresponding author.
The animal study was approved by Experimental Animal Ethics Committee of Yantai University. The study was conducted in accordance with the local legislation and institutional requirements.
MD: Conceptualization, Writing – original draft, Formal analysis, Methodology, Software, Validation. WY: Data curation, Formal analysis, Methodology, Resources, Validation, Writing – original draft. ND: Formal analysis, Software, Writing – original draft. MJ: Investigation, Visualization, Writing – original draft. YC: Funding acquisition, Project administration, Resources, Supervision, Writing – review & editing. JG: Funding acquisition, Project administration, Resources, Supervision, Writing – review & editing.
The author(s) declare financial support was received for the research, authorship, and/or publication of this article. This work was supported by the National Natural Science Foundation of China (32172161), the Natural Science Foundation of Shandong (ZR2022MC217). This work was partly supported by the Center for Mitochondria and Healthy Aging, College of Life Sciences, Yantai University.
The authors declare that the research was conducted in the absence of any commercial or financial relationships that could be construed as a potential conflict of interest.
All claims expressed in this article are solely those of the authors and do not necessarily represent those of their affiliated organizations, or those of the publisher, the editors and the reviewers. Any product that may be evaluated in this article, or claim that may be made by its manufacturer, is not guaranteed or endorsed by the publisher.
The Supplementary material for this article can be found online at: https://www.frontiersin.org/articles/10.3389/fnut.2024.1325886/full#supplementary-material
1. Spence, C. The psychology of condiments: a review. Intern J Gastronomy and Food Sci. (2018) 11:41–8. doi: 10.1016/j.ijgfs.2017.11.004
2. Wang, XY, Xie, J, and Chen, XJ. Applications of non-invasive and novel methods of low-field nuclear magnetic resonance and magnetic resonance imaging in aquatic products. Front Nutr. (2021) 8:651804. doi: 10.3389/fnut.2021.800489
3. Sung, WS, Park, SH, and Lee, DG. Antimicrobial effect and membrane-active mechanism of Urechistachykinins, neuropeptides derived from Urechis unicinctus. FEBS Lett. (2008) 582:2463–6. doi: 10.1016/j.febslet.2008.06.015
4. Li, JJ, Lu, JJ, Asakiya, C, Huang, KL, Zhou, XZ, Liu, QL, et al. Extraction and identification of three new Urechis unicinctus visceral peptides and their antioxidant activity. Mar Drugs. (2022) 20:293. doi: 10.3390/md20050293
5. Kang, HK, Lee, HH, Seo, CH, and Park, Y. Antimicrobial and immunomodulatory properties and applications of marine-derived proteins and peptides. Mar Drugs. (2019) 17:350. doi: 10.3390/md17060350
6. Kim, J-S, and Lee, Y-S. Study of Maillard reaction products derived from aqueous model systems with different peptide chain lengths. Food Chem. (2009) 116:846–53. doi: 10.1016/j.foodchem.2009.03.033
7. Nooshkam, M, Varidi, M, and Verma, DK. Functional and biological properties of Maillard conjugates and their potential application in medical and food: a review. Food Res Int. (2020) 131:109003. doi: 10.1016/j.foodres.2020.109003
8. Fu, Y, Zhang, Y, Soladoye, OP, and Aluko, RE. Maillard reaction products derived from food protein-derived peptides: insights into flavor and bioactivity. Crit Rev Food Sci Nutr. (2020) 60:3429–42. doi: 10.1080/10408398.2019.1691500
9. Song, S, Li, S, Fan, L, Hayat, K, Xiao, Z, Chen, L, et al. A novel method for beef bone protein extraction by lipase-pretreatment and its application in the Maillard reaction. Food Chem. (2016) 208:81–8. doi: 10.1016/j.foodchem.2016.03.062
10. Ren, GR, Zhao, LJ, Sun, Q, Xie, HJ, Lei, QF, and Fang, WJ. Explore the reaction mechanism of the Maillard reaction: a density functional theory study. J Mol Model. (2015) 21:132. doi: 10.1007/s00894-015-2674-5
11. Zhang, CL, Alashi, AM, Singh, N, Chelikani, P, and Aluko, RE. Glycated beef protein hydrolysates as sources of bitter taste modifiers. Nutrients. (2019) 11:2166. doi: 10.3390/nu11092166
12. Fu, BF, Xu, XB, Zhang, X, Cheng, SZ, El-Seedi, HR, and Du, M. Identification and characterisation of taste-enhancing peptides from oysters (Crassostrea gigas) via the Maillard reaction. Food Chem. (2023) 424:136412. doi: 10.1016/j.foodchem.2023.136412
13. Xu, D, Wang, X, Jiang, J, Yuan, F, and Gao, Y. Impact of whey protein – beet pectin conjugation on the physicochemical stability of β-carotene emulsions. Food Hydrocoll. (2012) 28:258–66. doi: 10.1016/j.foodhyd.2012.01.002
14. Simoes, D, Miguel, SP, Ribeiro, MP, Coutinho, P, Mendonca, AG, and Correia, IJ. Recent advances on antimicrobial wound dressing: a review. Eur J Pharm Biopharm. (2018) 127:130–41. doi: 10.1016/j.ejpb.2018.02.022
15. Li, XD, Teng, WD, Liu, GM, Guo, FY, Xing, HZ, Zhu, YH, et al. Allicin promoted reducing effect of garlic powder through acrylamide formation stage. Foods. (2022) 11:2394. doi: 10.3390/foods11162394
16. van Boekel, MA. Formation of flavour compounds in the Maillard reaction. Biotechnol Adv. (2006) 24:230–3. doi: 10.1016/j.biotechadv.2005.11.004
17. Cao, JR, Yan, HX, and Liu, L. Optimized preparation and antioxidant activity of glucose-lysine Maillard reaction products. Lwt-Food Science and Technology. (2022) 161:113343. doi: 10.1016/j.lwt.2022.113343
18. Guérard, F, and Sumaya-Martinez, MT. Antioxidant effects of protein hydrolysates in the reaction with glucose. Journal of the Oil Chemists’ Society. (2003) 80:467–70. doi: 10.1007/s11746-003-0721-1
19. Hwang, IG, Kim, HY, Woo, KS, Lee, J, and Jeong, HS. Biological activities of Maillard reaction products (MRPs) in a sugar–amino acid model system. Food Chem. (2011) 126:221–7. doi: 10.1016/j.foodchem.2010.10.103
20. Vinson, JA, Proch, J, and Zubik, L. Phenol antioxidant quantity and quality in foods: cocoa, dark chocolate, and Milk chocolate. J Agric Food Chem. (1999) 47:4821–4. doi: 10.1021/jf990312p
21. Long, LH, Kwee, DCK, and Halliwell, B. The antioxidant activities of seasonings used in Asian cooking. Powerful antioxidant activity of dark soy sauce revealed using the ABTS assay. Free Radic Res Commun. (2000) 32:181–6. doi: 10.1080/10715760000300181
22. Urbaniak, A, Szeląg, M, and Molski, M. Theoretical investigation of stereochemistry and solvent influence on antioxidant activity of ferulic acid. Computational and Theoretical Chemistry. (2013) 1012:33–40. doi: 10.1016/j.comptc.2013.02.018
23. Shi, XM, Wu, Q, Ren, DD, Wang, SY, and Xie, YF. Research of the determination method of furfurals and furosine in milk and the application in the quality evaluation of milk. Quality Assurance and Safety of Crops & Foods. (2022) 14:12–23. doi: 10.15586/qas.v14i1.929
24. Cunha, SA, de Castro, R, Coscueta, ER, and Pintado, M. Hydrolysate from mussel Mytilus galloprovincialis meat: enzymatic hydrolysis, optimization and bioactive properties. Molecules. (2021) 26:5228. doi: 10.3390/molecules26175228
25. Zhao, F, Qian, J, Liu, H, Wang, C, Wang, XJ, Wu, WX, et al. Quantification, identification and comparison of oligopeptides on five tea categories with different fermentation degree by Kjeldahl method and ultra-high performance liquid chromatography coupled with quadrupole-orbitrapultra-high resolution mass spectrometry. Food Chem. (2022) 378:132130. doi: 10.1016/j.foodchem.2022.132130
26. Wei, CK, Thakur, K, Liu, DH, Zhang, JG, and Wei, ZJ. Enzymatic hydrolysis of flaxseed (Linum usitatissimum L.) protein and sensory characterization of Maillard reaction products. Food Chem. (2018) 263:186–93. doi: 10.1016/j.foodchem.2018.04.120
27. Li, Q, Li, X, Ren, Z, Wang, R, Zhang, Y, Li, J, et al. Physicochemical properties and antioxidant activity of Maillard reaction products derived from Dioscorea opposita polysaccharides. Lwt. (2021) 149:111833. doi: 10.1016/j.lwt.2021.111833
28. Je, T, Liu, T, Yao, Y, Wu, N, Du, H, Xu, M, et al. Changes in physicochemical and antioxidant properties of egg white during the Maillard reaction induced by alkali. Lwt. (2021) 143:111151. doi: 10.1016/j.lwt.2021.111151
29. Liu, W, Wang, H, Pang, X, Yao, W, and Gao, X. Characterization and antioxidant activity of two low-molecular-weight polysaccharides purified from the fruiting bodies of Ganoderma lucidum. Int J Biol Macromol. (2010) 46:451–7. doi: 10.1016/j.ijbiomac.2010.02.006
30. Hseu, YC, Chen, SC, Yech, YJ, Wang, L, and Yang, HL. Antioxidant activity of Antrodia camphorata on free radical-induced endothelial cell damage. J Ethnopharmacol. (2008) 118:237–45. doi: 10.1016/j.jep.2008.04.004
31. Fu, Y, Liu, J, Hansen, ET, Bredie, WLP, and Lametsch, R. Structural characteristics of low bitter and high umami protein hydrolysates prepared from bovine muscle and porcine plasma. Food Chem. (2018) 257:163–71. doi: 10.1016/j.foodchem.2018.02.159
32. Djekic, I, Lorenzo, JM, Munekata, PES, and Tomasevic, I. Review on characteristics of trained sensory panels in food science. J Texture Stud. (2021) 52:501–9. doi: 10.1111/jtxs.12616
33. Liu, JB, Liu, MY, He, CC, Song, HL, and Chen, F. Effect of thermal treatment on the flavor generation from Maillard reaction of xylose and chicken peptide. Lwt-Food Science and Technology. (2015) 64:316–25. doi: 10.1016/j.lwt.2015.05.061
34. Kang, MC, Cha, SH, Wijesinghe, WA, Kang, SM, Lee, SH, Kim, EA, et al. Protective effect of marine algae phlorotannins against AAPH-induced oxidative stress in zebrafish embryo. Food Chem. (2013) 138:950–5. doi: 10.1016/j.foodchem.2012.11.005
35. Kim, EA, Lee, SH, Ko, CI, Cha, SH, Kang, MC, Kang, SM, et al. Protective effect of fucoidan against AAPH-induced oxidative stress in zebrafish model. Carbohydr Polym. (2014) 102:185–91. doi: 10.1016/j.carbpol.2013.11.022
36. Gu, F-L, Kim, JM, Abbas, S, Zhang, X-M, Xia, S-Q, and Chen, Z-X. Structure and antioxidant activity of high molecular weight Maillard reaction products from casein–glucose. Food Chem. (2010) 120:505–11. doi: 10.1016/j.foodchem.2009.10.044
37. Shakoor, A, Zhang, C, Xie, J, and Yang, X. Maillard reaction chemistry in formation of critical intermediates and flavour compounds and their antioxidant properties. Food Chem. (2022) 393:133416. doi: 10.1016/j.foodchem.2022.133416
38. Zhao, CJ, Schieber, A, and Ganzle, MG. Formation of taste-active amino acids, amino acid derivatives and peptides in food fermentations - a review. Food Res Int. (2016) 89:39–47. doi: 10.1016/j.foodres.2016.08.042
39. Ishibashi, N, Arita, Y, Kanehisa, H, Kouge, K, Okai, H, and Fukui, S. Bitterness of leucine-containing peptides. Agric Biol Chem. (2016) 51:2389–94. doi: 10.1080/00021369.1987.10868411
40. Lan, XH, Liu, P, Xia, SQ, Jia, CS, Mukunzi, D, Zhang, XM, et al. Temperature effect on the non-volatile compounds of Maillard reaction products derived from xylose-soybean peptide system: further insights into thermal degradation and cross-linking. Food Chem. (2010) 120:967–72. doi: 10.1016/j.foodchem.2009.11.033
41. Guan, W, Ren, X, Li, Y, and Mao, L. The beneficial effects of grape seed, sage and oregano extracts on the quality and volatile flavor component of hairtail fish balls during cold storage at 4 °C. Lwt. (2019) 101:25–31. doi: 10.1016/j.lwt.2018.11.024
42. Zhou, X, Chong, Y, Ding, Y, Gu, S, and Liu, L. Determination of the effects of different washing processes on aroma characteristics in silver carp mince by MMSE-GC-MS, e-nose and sensory evaluation. Food Chem. (2016) 207:205–13. doi: 10.1016/j.foodchem.2016.03.026
43. Zhao, TT, Zhang, Q, Wang, SG, Qiu, CY, Liu, Y, Su, GW, et al. Effects of Maillard reaction on bioactivities promotion of anchovy protein hydrolysate: the key role of MRPs and newly formed peptides with basic and aromatic amino acids. Lwt-Food Science and Technology. (2018) 97:245–53. doi: 10.1016/j.lwt.2018.06.051
44. Refsgaard, HHF, Haahr, AM, and BJJoA, J Chemistry F. Isolation and quantification of volatiles in fish by dynamic headspace sampling and mass spectrometry. J Agric Food Chem. (1999) 47:1114–8.
45. Huang, P, Wang, Z, Feng, X, and Kan, J. Promotion of fishy odor release by phenolic compounds through interactions with myofibrillar protein. Food Chem. (2022) 387:132852. doi: 10.1016/j.foodchem.2022.132852
46. Dini, I, and Laneri, S. Spices, condiments, extra virgin olive oil and aromas as not only flavorings, but precious allies for our wellbeing. Antioxidants (Basel). (2021) 10:868. doi: 10.3390/antiox10060868
47. Wang, Z, Zheng, L, Li, C, Zhang, D, Xiao, Y, Guan, G, et al. Modification of chitosan with monomethyl fumaric acid in an ionic liquid solution. Carbohydr Polym. (2015) 117:973–9. doi: 10.1016/j.carbpol.2014.10.021
48. He, S, Chen, YN, Brennan, C, Young, DJ, Chang, K, Wadewitz, P, et al. Antioxidative activity of oyster protein hydrolysates Maillard reaction products. Food Sci Nutr. (2020) 8:3274–86. doi: 10.1002/fsn3.1605
49. Liu, YP, Sun, XH, Jiang, WW, and Cao, XL. Protective effects of dimethylthiourea against hydrogen peroxide-induced oxidative stress in hepatic L02 cell. Int J Clin Exp Med. (2019) 12:5114–21.
50. Gao, XD, Jia, YN, Santhanam, RK, Wang, YJ, Lu, YP, Zhang, M, et al. Garlic flavonoids alleviate H2O2 induced oxidative damage in L02 cells and induced apoptosis in HepG2 cells by Bcl-2/caspase pathway. J Food Sci. (2021) 86:366–75. doi: 10.1111/1750-3841.15599
51. Ko, JY, Lee, JH, Samarakoon, K, Kim, JS, and Jeon, YJ. Purification and determination of two novel antioxidant peptides from flounder fish (Paralichthys olivaceus) using digestive proteases. Food Chem Toxicol. (2013) 52:113–20. doi: 10.1016/j.fct.2012.10.058
52. Rizwana, N, Agarwal, V, and Nune, M. Antioxidant for neurological diseases and Neurotrauma and bioengineering approaches. Antioxidants. (2022) 11:72. doi: 10.3390/antiox11010072
53. Finkel, T, Holbrook, N, and Nature, JJ. Oxidants, oxidative stress and the biology of ageing. Nature. (2000) 408:239.
Keywords: Urechis unicinctus, the Maillard reaction, ROS, antioxidation, functional condiment
Citation: Du M, Yu W, Ding N, Jian M, Cheng Y and Gan J (2024) Antioxidant, aroma, and sensory characteristics of Maillard reaction products from Urechis unicinctus hydrolysates: development of food flavorings. Front. Nutr. 11:1325886. doi: 10.3389/fnut.2024.1325886
Received: 22 October 2023; Accepted: 15 January 2024;
Published: 06 February 2024.
Edited by:
Dandan Pu, Beijing Technology and Business University, ChinaReviewed by:
Jinwang Li, Beijing Technology and Business University, ChinaCopyright © 2024 Du, Yu, Ding, Jian, Cheng and Gan. This is an open-access article distributed under the terms of the Creative Commons Attribution License (CC BY). The use, distribution or reproduction in other forums is permitted, provided the original author(s) and the copyright owner(s) are credited and that the original publication in this journal is cited, in accordance with accepted academic practice. No use, distribution or reproduction is permitted which does not comply with these terms.
*Correspondence: Jing Gan, Z2FuamluZ0B5dHUuZWR1LmNu
Disclaimer: All claims expressed in this article are solely those of the authors and do not necessarily represent those of their affiliated organizations, or those of the publisher, the editors and the reviewers. Any product that may be evaluated in this article or claim that may be made by its manufacturer is not guaranteed or endorsed by the publisher.
Research integrity at Frontiers
Learn more about the work of our research integrity team to safeguard the quality of each article we publish.