- 1Key Laboratory of Respiratory Disease of Ningbo, Department of Respiratory and Critical Care Medicine, The First Affiliated Hospital of Ningbo University, Ningbo, China
- 2Department of Hepatology, The First Affiliated Hospital of Ningbo University, Ningbo, Zhejiang, China
Design: Ultra-processed foods (UPFs) have become a pressing global health concern, prompting investigations into their potential association with low muscle mass in adults.
Methods: This cross-sectional study analyzed data from 10,255 adults aged 20−59 years who participated in the National Health and Nutritional Examination Survey (NHANES) during cycles spanning from 2011 to 2018. The primary outcome, low muscle mass, was assessed using the Foundation for the National Institutes of Health (FNIH) definition, employing restricted cubic splines and weighted multivariate regression for analysis. Sensitivity analysis incorporated three other prevalent definitions to explore optimal cut points for muscle quality in the context of sarcopenia.
Results: The weighted prevalence of low muscle mass was 7.65%. Comparing the percentage of UPFs calories intake between individuals with normal and low muscle mass, the values were found to be similar (55.70 vs. 54.62%). Significantly linear associations were observed between UPFs consumption and low muscle mass (P for non-linear = 0.7915, P for total = 0.0117). Upon full adjustment for potential confounding factors, participants with the highest UPFs intake exhibited a 60% increased risk of low muscle mass (OR = 1.60, 95% CI: 1.13 to 2.26, P for trend = 0.003) and a decrease in ALM/BMI (β = −0.0176, 95% CI: −0.0274 to −0.0077, P for trend = 0.003). Sensitivity analysis confirmed the consistency of these associations, except for the International Working Group on Sarcopenia (IWGS) definition, where the observed association between the highest quartiles of UPFs (%Kcal) and low muscle mass did not attain statistical significance (OR = 1.35, 95% CI: 0.97 to 1.87, P for trend = 0.082).
Conclusion: Our study underscores a significant linear association between higher UPFs consumption and an elevated risk of low muscle mass in adults. These findings emphasize the potential adverse impact of UPFs on muscle health and emphasize the need to address UPFs consumption as a modifiable risk factor in the context of sarcopenia.
Introduction
Ultra-processed foods (UPFs) are highly processed and industrially manufactured products that typically contain high levels of additives, sugar, salt, and unhealthy fats. These foods often undergo multiple stages of cooking, refining, and packaging to extend shelf life and provide convenience (1). They are easy to obtain and are known for their appealing taste, leading to their increasingly widespread availability and increased consumption of UPFs by more than half over the past several years (2, 3). UPFs typically consist of processed sugars, proteins, fats, as well as low-cost industrial raw materials, additives and processing methods that are rarely used in traditional cooking. They are considered to decrease the overall quality of the diet (4) and result in various health problems such as obesity (5), type-2 diabetes (6), and cardiovascular diseases (7). For this reason, public health organizations have widely expressed the need to limit UPFs consumption (8).
Sarcopenia is a medical condition closely linked to the process of aging. As individuals grow older, their metabolic functions gradually decline, leading to an inevitable reduction in muscle mass and muscle strength (9). Research indicates that without resistance training, muscle mass decline may commence after the age of 30, and by the age of 60, muscle atrophy can reach 20−40% (10). Currently, the precise definition of sarcopenia remains a topic of debate and varies across different criteria (9, 11–13). However, a prevailing characteristic among most definitions is the presence of low muscle mass (9). This particular attribute is currently recognized as a significant determinant of various health outcomes and an elevated risk of mortality (14, 15). Numerous studies have established that low muscle mass is influenced by a multitude of individual factors, encompassing genetic predisposition, birth weight, breastfeeding history, levels of physical activity, dietary habits, socio-economic statuses, and diseases (16, 17). Nowadays, resistance training is considered one of the best methods for preventing/treating sarcopenia (18). Regularly engaging in resistance training programs throughout adulthood, especially during young adulthood and midlife, can mitigate age-related changes in the musculoskeletal system and lessen their impact on metabolism and the aging process (18, 19). While dietary choices represent another crucial modifiable aspect, it is noteworthy that low muscle mass also holds significance in the diagnosis of malnutrition and constitutes one of the three phenotypic criteria considered under the auspices of the Global Leadership Initiative on Malnutrition (GLIM) criteria (20, 21). Given the marked prevalence of UPFs consumption in Western countries (2), the potential connection between UPFs intake and muscle mass has been an area of interest. Excessive consumption of UPFs not only leads to concerns about muscle health due to nutritional deficiencies and excessive intake of food additives but also evidence suggests that it may be linked to muscle-skeletal damage through the disruption of the gut microbiota ecosystem, potentially via the gut-muscle and gut-brain axes (22). While older adults are at a higher risk of sarcopenia, maintaining optimal muscle health during younger and middle-aged years is pivotal for muscle conditions in later life. Studying the relationship between muscle mass decline during these age groups and modifiable dietary patterns provides crucial insights for preventive strategies. Therefore, we conducted an assessment of the association between the proportion of UPFs in the total daily caloric intake and low muscle mass, utilizing data sourced from a nationally representative sample of U.S. adults, with measurements based on the Dual-Energy X-ray Absorptiometry (DXA) method. Our study aims to shed light on the intricate interactions between UPFs consumption and muscle health, using robust data and accounting for crucial dietary and physiological factors.
Methods
Design
This cross-sectional study drew upon data publicly available from the National Health and Nutrition Examination Survey (NHANES) to analyze the relationship between UPFs and muscle mass. As adults aged ≥60 years were not eligible for DXA examination, participants aged 20−59 years who responded to relevant questions concerning demographics, socioeconomic factors, dietary intake, chronic diseases, body measurements from the 2011−2018 cycles were included in the analysis. After applying additional inclusion and exclusion criteria, this left 10,255 participants available for study. Detailed information is represented in Figure 1.
UPFs
In this study, we used the United States Department of Agriculture (USDA) Automated Multiple-Pass Method to collect 24-hour dietary recalls in-person from trained interviewers. The NOVA food classification system was applied to the Food and Nutrient Database for Dietary Studies (FNDDS) data, and all food items that matched the features of UPFs were considered. The proportion of the UPFs energy intake in total daily energy intake (%Kcal UPFs) was calculated based on the two-day average as an indicator for UPFs intake, and the UPFs consumption was divided into quartiles as the exposure variable. A more detailed description of NOVA classification can be found in Supplementary Table 1.
Previous studies have demonstrated the validity of the 24-hour dietary recall and that the UPFs classification and calorie estimation used in this study are consistent and accurate (23, 24).
Also, because many food additives cannot be measured in terms of energy, the proportion of the UPFs grams intake in total daily grams intake (%Gram UPFs) was also collected and used as an indicator for sensitivity analysis.
Low muscle mass
Height (m), weight (kg), and body mass index (BMI) (kg/m2) were measured for each survey cycle conducted between 2011−2018 in the Mobile Examination Center.
All eligible participants had their appendicular lean mass (ALM) assessed using DXA. ALM, an accepted proxy for skeletal muscle mass, was calculated by summing the lean mass (excluding bone mineral content) of the right and left leg and right and left arm as measured by DXA.
Because low muscle mass is another important basis for the diagnosis of sarcopenia in addition to reduced grip strength, different definitions exist and continue to be a source of controversy. We chose the previously published definition from Foundation for the National Institutes of Health (FNIH) - low muscle mass [ALM (kg)/BMI (kg/m2) < 0.789 for male and <0.512 for female] as the main outcome. Besides, the updated European Working Group on Sarcopenia in Older People (EWGSOP2) (12) definition [ALM (kg)/height2(m2) < 7.0 kg/m2 for male and <5.5°kg/m2 for female], Asian Working Group on Sarcopenia (2019, AWGS2) (11) definition [ALM (kg)/height2(m2) < 7.0 kg/m2 for male and <5.4°kg/m2 for female], and International Working Group on Sarcopenia (IWGS) definition [ALM (kg)/height2(m2) < 7.23 kg/m2 for male and <5.67°kg/m2 for female] were also included for the sensitivity analysis.
Covariates
The selection of potential confounding variables was based on prior literature findings, and these variables were subsequently adjusted in the multivariate models. These covariates included various socio-demographic characteristics, such as sex, age, race (e.g., non-Hispanic white, non-Hispanic black, Mexican American, and others), poverty income ratio [PIR, e.g., ≤1.3 categorized as low, >1.3 to ≤3.5 categorized as middle, >3.5 categorized as high (25)], marital status (e.g., never married, married or living with partner, and widowed, divorced, or separated), home status (e.g., rented, owned or being bought, and other arrangement), drinks (e.g., non-drinkers, 1−3 drinks/day, and ≥4 drinks/day), smoke status (categorized into active, former, and never based on responses to two questions: “Smoked at least 100 cigarettes in life?,” and “Do you now smoke cigarettes?”), and physical activity [categorized into inactive: less than 150 min of moderate-intensity physical activity per week, moderate: 150−300 min of moderate-intensity, or 75−150 min of vigorous-intensity aerobic physical activity per week, active: more than 300 min of moderate-intensity physical activity per week (26)]. In addition, certain chronic diseases, such as hypertension (yes or no), diabetes mellitus (DM) (yes or no), congestive heart failure (yes or no), heart attack (yes or no), stroke (yes or no), and cancer (yes or no) were included in this study. Moreover, in our multiple regression analysis, we incorporated the Urinary Albumin to Creatinine Ratio (UACR). The UACR was calculated using the following formula: UACR (mg/g) = Urinary albumin level (mg/dL)/Urinary creatinine level (g/dL). To obtain these values, spot urine samples were utilized. Additionally, estimations of glomerular filtration rate (eGFR), total energy intake (kcal), and protein intake (g) calculated as the average of 2°days were also taken into account as relevant covariates in our analysis.
Statistical analysis
The NHANES is a population-based survey that utilizes a non-random, stratified sampling design in order to effectively represent specific population subgroups. Sample weights are assigned to participants to account for non-response and other complexities associated with survey design. Our analysis follows the guidelines of NHANES, merging data from four separate cycles (2011−2018) into one 8-year dataset. In order to approximate standard errors for all continuous variables, we employed a Taylor Series Linearization approach. Subsequently, Student’s t-test was used to study associations of categorical variables, while weighted percentages, means [95% confidence intervals (CI)], and survey-weighted chi-squared tests were used to analyze categorical variables. Furthermore, we utilized restricted cubic splines, with four knots (20th, 40th, 60th, 80th percentiles) to flexibly model and visualize the relationship between UPFs and low muscle mass in multivariable logistic regression models. There was no evidence of departure from linearity observed in our analysis (Figure 2). Multiple regression models were then employed to calculate the adjusted odds ratio (OR) of low muscle mass across the quartile of UPFs (%Kcal) consumption. The adjusted differences of ALM/BMI across the quartile of UPFs (%Kcal) consumption were also estimated. Subgroup and interactive analyses were carried out in order to explore differences between selected subgroups.
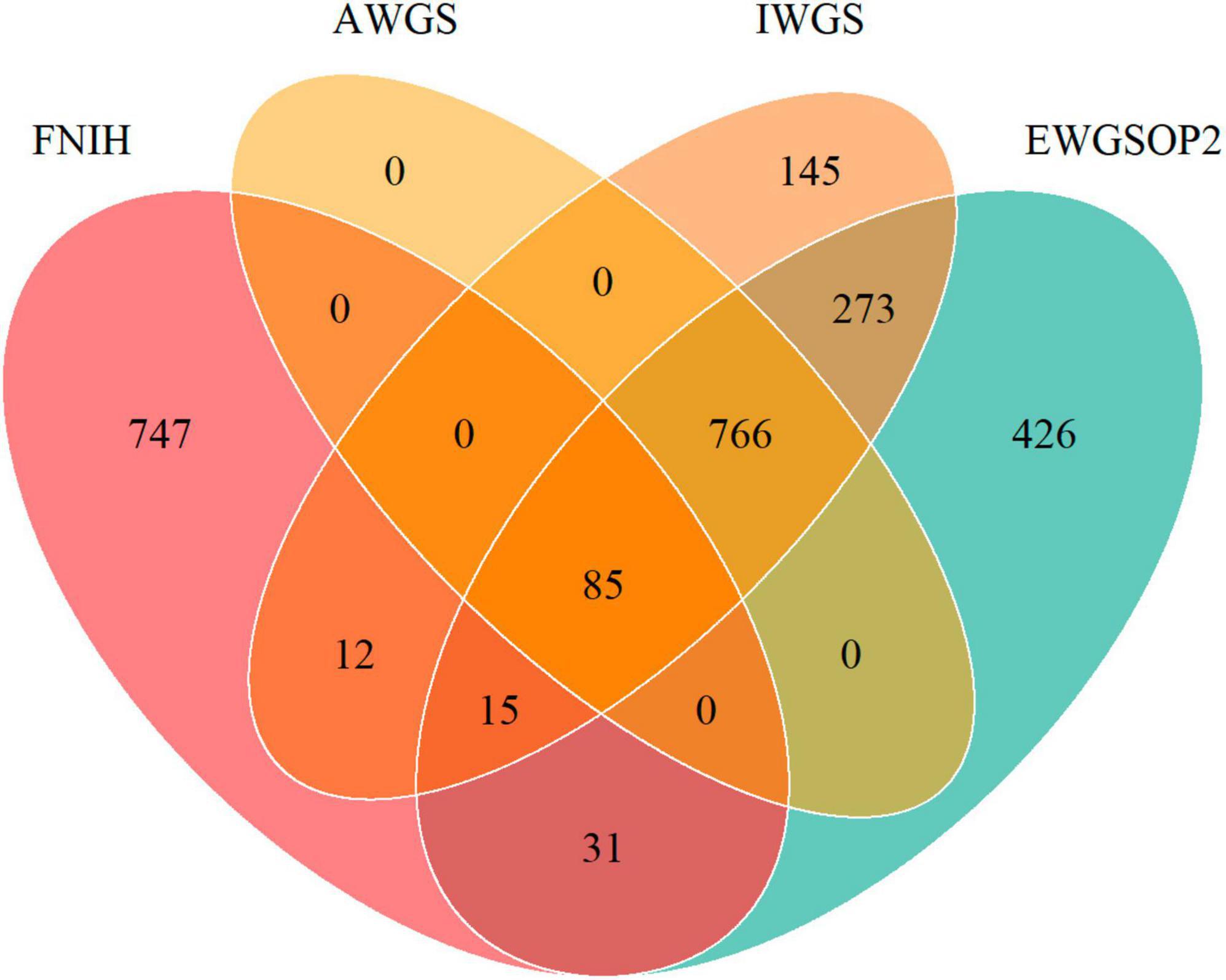
Figure 2. Venn diagram showing the overlap of prevalence of low muscle mass by different definitions of FNIH, EWGSOP2, AWGS, and IWGS.
Sensitivity analyses were then implemented in order to further explore the association between UPFs consumption and low muscle mass. The initial sensitivity analysis investigated the correlation between the proportion of UPFs by weight and muscle mass. This approach was motivated by previous studies suggesting that evaluating the percentage of UPFs based on the total weight of consumed foods and beverages might provide a more comprehensive understanding. By considering the weight of UPFs in relation to the entire diet, it allows for the inclusion of UPFs that do not contribute significantly to energy intake (e.g., artificially sweetened beverages) and those directly influenced by food processing characteristics rather than their nutritional attributes (27, 28). The intake of UPFs (%Gram) was categorized into quartiles, and its association with β value and ORs for muscle mass and low muscle mass was assessed (Supplementary Table 2). The second sensitivity analysis examined the association between the UPFs (%Kcal) and different low muscle mass definitions (AWGS2, EWGSOP2, and IWGS), as there are still debates about the cut point of low muscle mass for sarcopenia.
All data analysis was performed using software R (version 4.3.1) and the “survey” package.
Results
Our study encompassed a total of 10,255 participants (NHANES 2011-2018 cycles). The weighted prevalence of low muscle mass (FNIH) was found to be 7.65%. When comparing the consumption of UPFs in terms of both %Kcal and %Gram between individuals with normal muscle mass and those with low muscle mass, the proportions were similar, with values of 55.70% ± 0.38% versus 54.62% ± 1.11 (P = 0.3608) for %Kcal and 36.11% ± 0.36% versus 38.41% ± 1.10% (P = 0.0607) for %Gram, respectively. Upon further examination, individuals with low muscle mass exhibited several distinguishing characteristics in comparison to those with normal muscle mass. Specifically, the former group tended to be older, male, and with lower income as indicated by low PIR. Moreover, they were more likely to have completed education up to middle school or lower and high school, and were less likely to be regular consumers of alcoholic beverages. Additionally, individuals with low muscle mass had higher prevalence rates of various health conditions, including stroke, heart attack, congestive heart failure, coronary heart disease, angina, hypertension, and diabetes mellitus (DM). Moreover, they demonstrated lower total energy intake and protein intake, as well as reduced eGFR. On the other hand, their BMI and weight were higher. For further insight, the detailed demographic and behavioral characteristics of the study participants are provided in Table 1. And Supplementary Table 3 showed the characteristics of the study participants by quartiles of UPFs (%Kcal). Figure 2 depicts a Venn diagram illustrating the prevalence of low muscle mass as determined by various definitions.
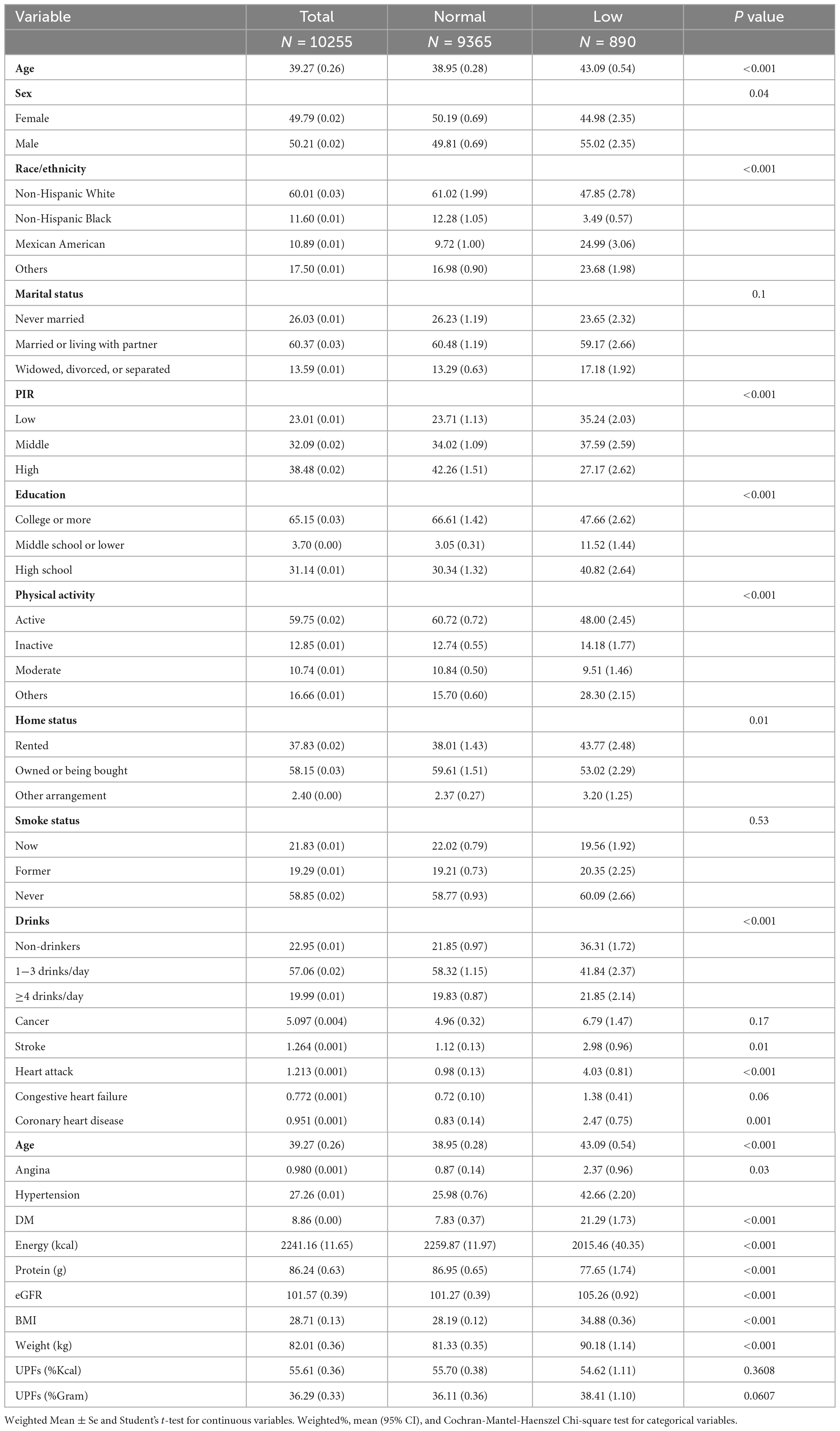
Table 1. Characteristics of the study participants by normal and low muscle mass among U.S adults aged 20–59 years.
The dose-response relationships between UPFs, expressed as a percentage of daily caloric intake, and the occurrence of low muscle mass was also estimated. To explore these relationships, we employed both linear and spline models, accounting for all potential confounding factors. Figure 3 serves as a visual representation of these dose-response relationships, capturing the interplay between UPFs and low muscle mass. Our meticulous analysis revealed a notable linear association between UPFs and low muscle mass (P for non-linear = 0.7915, P for total = 0.0117). This implies that as the proportion of UPFs in the daily caloric intake increases, there is a corresponding impact on the occurrence of low muscle mass.
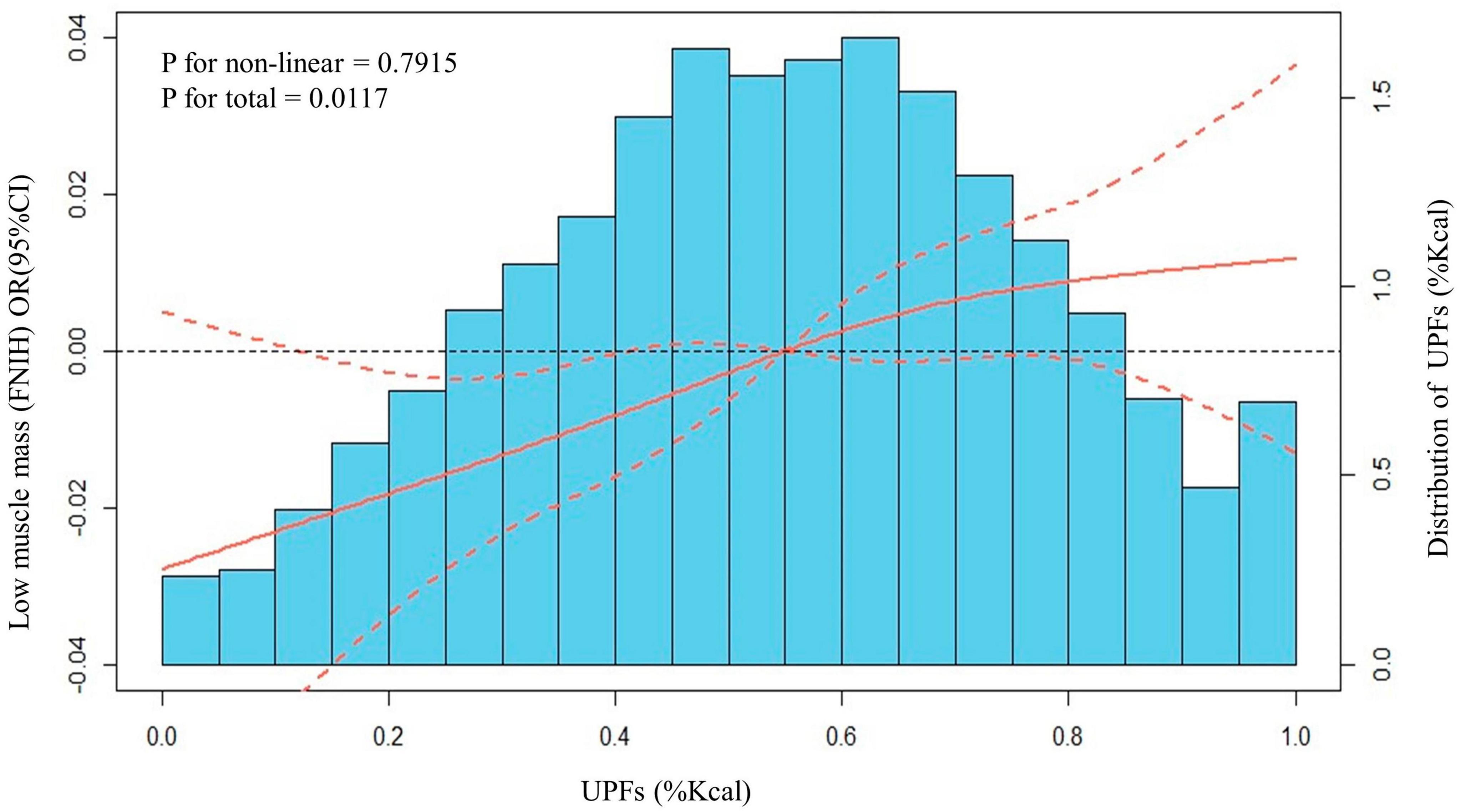
Figure 3. Distributions of frequency of UPFs (%Kcal) and dose–response relationship between UPFs (%Kcal) and low muscle mass in US adults 20–59 years (n = 10,255), NHANES 2011 to 2018. Values represent difference in predicted response in reference to a UPFs (%Kcal) of mean. Red solid lines and Red dotted line represent restricted cubic spline models and 95%CI, respectively. Multivariable logistic regression model is used to estimate the fully adjusted OR in low muscle mass (FNIH definition) and corresponding 95% CI. Model was adjusted by age, ethnicity, PIR, marital status, home status, education, physical activity, smoke status, drinks, eGFR, UACR, hypertension, DM, angina, coronary heart disease, congestive heart failure, heart attack, stroke, cancer, energy (Kcal), and protein (g).
Table 2 presents the weighted OR with 95%CI for low muscle mass categorized into quartiles of UPF calorie intakes, accounting for relevant covariates. Following adjustments for all selected covariates using multiple logistic regression (model 3), it was found that participants with the highest UPFs (%Kcal) intake faced a 60% higher risk of low muscle mass (OR = 1.60, 95% CI: 1.13 to 2.26, P for trend = 0.003) compared with the lowest quartile. Additionally, we explored the relationship between UPFs and ALM/BMI. After accounting for the specified covariates, it was observed that the highest UPFs intake compared to individuals with the lowest UPFs intake, there was a significant reduction in ALM/BMI (β = −0.0176, 95% CI: −0.0274 to −0.0077, P for trend = 0.03).
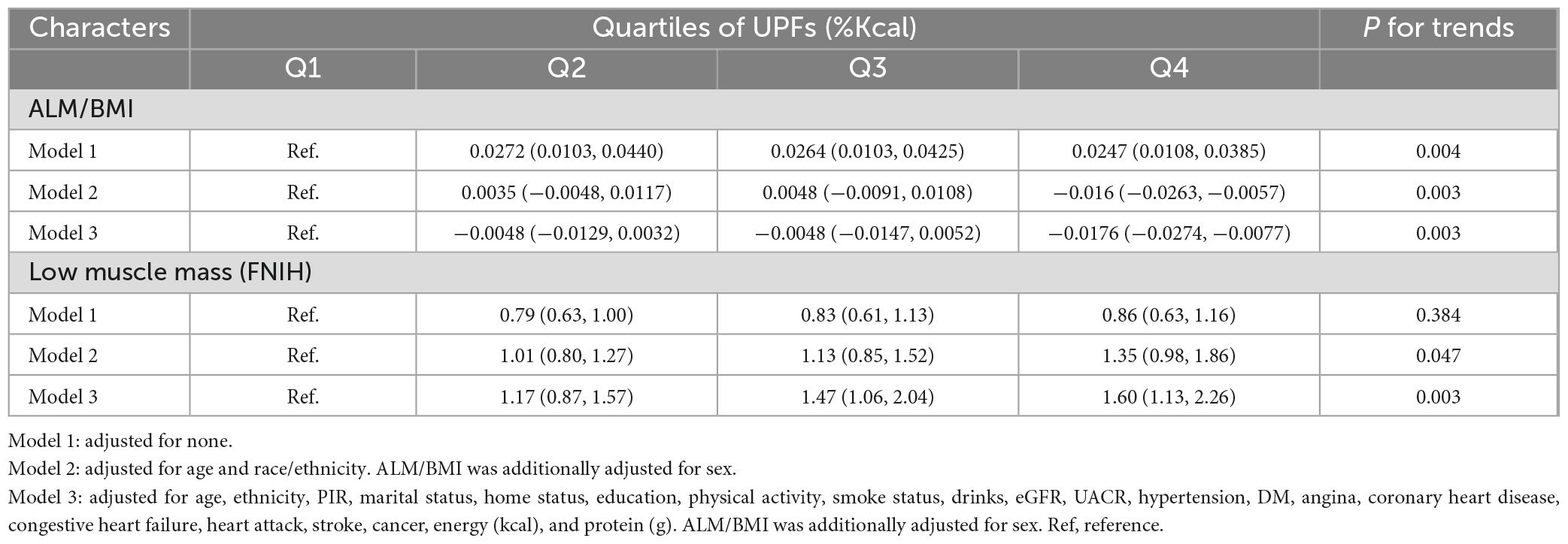
Table 2. Multivariable associations between quartiles of UPFs (%Kcal) and low muscle mass defined by FNIH.
Subsequently, we performed subgroup analyses and assessed potential interactions (Table 3). The results indicated that the associations between UPFs consumption and low muscle mass remained generally consistent across the selected subgroups, demonstrating stability in their patterns.
Moreover, in our sensitivity analyses, we undertook an examination of the relationship between UPFs consumption (%Gram) and low muscle mass, adhering to the definition provided by the FNIH. Additionally, we assessed the association between UPFs consumption (%Kcal) and low muscle mass using the criteria established by the AWGS2, EWGSOP2, and the IWGS. Across these sensitivity analyses, the fundamental patterns of association remained largely unchanged, except for the results obtained with the IWGS definition. In the case of IWGS, the observed association between the highest quartiles of UPFs (%Kcal) and low muscle mass did not attain statistical significance (OR = 1.35, 95% CI: 0.97 to 1.87, P for trend = 0.082) (Supplementary Tables 2, 4).
Discussion
Data from the NHANES nationally representative sample of adults aged 20−59 years reveals that UPFs constitute a noteworthy component of the adult diet and exhibit a linear correlation with low muscle mass. This correlation remains consistent across various cut points defining low muscle mass of sarcopenia context except for IWGS definition. Consequently, these findings imply that increased UPF consumption negatively impacts muscle mass in adults and represents a significant driving factor for sarcopenia.
Advances in the field of aging biology have shed light on the complex underlying processes involving myocyte, inflammatory, and hormonal mechanisms, contributing to body fat redistribution, decline in lean muscle mass, and reduced muscle strength (29, 30). Traditionally, sarcopenia has been primarily assessed based on muscle mass and grip strength. However, the heterogeneity within the sarcopenia patient population has led to controversies among professional societies, resulting in variations in cutoff values for grip strength and muscle mass, impacting the design of targeted interventions (31, 32). Furthermore, since muscle mass is one of the three criteria for diagnosing malnutrition, the consumption of UPFs, known for their low dietary quality, may increase the risk of malnutrition in adults.
Ultra-processed foods (UPFs) are gradually penetrating our traditional diets and have become dominant in high- and middle-income countries (2, 33). In the younger and middle-aged population, the integration of professional and personal responsibilities, coupled with a fast-paced work-life style, may inadvertently lead to increased sedentary behavior. Additionally, the appeal of UPFs’ convenience and extensive advertising further encourages individuals to choose these highly processed food options. Numerous observational studies have indicated that high UPF intake in adults is associated with various metabolic risk factors, including heightened body weight and BMI (34), elevated total cholesterol and low-density lipoprotein levels (35), and metabolic syndrome (23, 27). In France, the NutriNet-Santé cohort (27) survey further confirmed that higher UPFs consumption correlated with increased BMI and a greater risk of overweight or obesity. While epidemiological evidence on the association between UPFs and bone composition is lacking, animal studies have demonstrated that young rats consuming UPFs with high fat and sugar content experience growth retardation, decreased bone mineral density, damage to bone structural components, and growth plate impairment (36). Considering the potential links between body composition, fat mass, bone mass, and muscle mass (29), there is plausible reason to suspect that UPFs may adversely affect muscle mass. Previous research has revealed significant associations between UPFs and various markers of muscle health in different populations. Studies conducted in China involving adults over 40 years of age demonstrated a negative correlation between UPFs and grip strength (37), while investigations in Brazilian adolescents found associations between UPFs and body composition, particularly lean body mass (38). Furthermore, studies in Brazilian adults indicated that UPFs were linked to specific markers of muscle mass, such as arm circumference (39). These findings are consistent with our prior assumptions, which have been further corroborated by the current study, indicating a negative relationship between UPFs and muscle mass in adults.
However, when we reassessed the cutoff for low muscle mass based on different criteria for sarcopenia, we observed that only 9.6% of individuals were defined as having low muscle mass in all four definitions, whereas 16.3% of individuals in the IWGS definition were not classified as having low muscle mass in the other three definitions (Figure 2). Such substantial discrepancies result in considerable classification deviations among individuals and render the conclusions inconsistent. On one hand, this suggests that the diagnosis of sarcopenia necessitates additional data updates and support. On the other hand, the application of corresponding definitions should consider regional and population differences to avoid resulting biases (40).
The relationship between UPFs and the risk factors associated with low muscle mass is underpinned by several potential mechanisms. Previous studies have shed light on the impact of various dietary components, such as dietary fiber, red meat, oily fish, retinol, magnesium, and vitamins, on muscle mass and strength (41–44). For example, an adequate intake of protein, especially leucine-rich protein, can stimulate muscle protein synthesis and serve a preventive or interventional role in individuals facing muscle loss (45). Moreover, Omega-3-rich foods like fish, nuts, and grains possess anti-inflammatory properties that impede white blood cell migration toward sources of inflammation and hinder cell aggregation, thereby contributing to the preservation of muscle mass (46). Additionally, dietary fiber has been found to mitigate oxidative stress and inflammation. Conversely, UPFs often lack these crucial nutrients and are characterized by elevated levels of sugar, sodium, trans fats, saturated fats, and inadequate protein and essential nutrients (47). Furthermore, the process of over-processing UPFs, coupled with the addition of sugars and modifications to food additives and compositions, leads to overeating and an imbalance in intestinal flora. Research by Suez et al. (48) has substantiated that non-caloric artificial sweeteners can disrupt microbial metabolic pathways. Additionally, dietary emulsifiers, such as lecithin, fatty acid monoglycerides, and diglycerides, have been shown to enhance bacterial translocation across epithelial cells in vitro, promoting systemic inflammation (48, 49). This phenomenon reduces the diversity of intestinal microorganisms, decreases the abundance of Bacteroides, and increases the abundance of mucin-degrading microorganisms (Akkermansia muciniphila) and Proteobacteria. Furthermore, various other types of food additives, such as preservatives, nanoparticles, foaming agents, stabilizers, flavor enhancers, and others, individually or in combination with each other or with different types of food additives, have been found to potentially confer growth advantages or increased toxicity in host gut microbiota, including (opportunistic) pathogenic micro-organisms, leading to dysbiosis (50, 51). These changes in the microbiota contribute to disrupting the intestinal barrier’s function, compromising nutrient absorption, and increasing the glycemic load, thereby affecting overall metabolic function (52, 53). Additionally, compromised permeability, also known as “gut leaky syndrome” may lead to higher endotoxemia as harmful substances enter, thereby promoting systemic inflammation and a chronic state of immune activation. Ultimately, these processes may affect skeletal muscle through the gut-muscle axis or gut-brain axis, increasing the risk of musculoskeletal injury (22). One common scenario is when athletes increase their intake of sports energetic supplements for performance and recovery, potential microbiota dysbiosis and leaky gut may lead to gastrointestinal complications, a significant factor in poor endurance performance and dropout (54). Moreover, environmental pollutants from food packaging, such as phthalates and bisphenol, may also impact muscle mass and strength (55). Unfortunately, to date, research on the relationship between dietary patterns and sarcopenia has primarily focused on the elderly (>70 years old) (56). Limited evidence exists regarding the potential impact of overall diet quality on muscle mass and function decline in younger and middle-aged individuals. Some studies suggest that dietary patterns can affect muscle mass and function in middle-aged populations through inflammation, oxidative stress, and metabolic mechanisms (57). For instance, there is an association between pro-inflammatory diets and muscle mass decline in middle-aged individuals (58), and UPFs is linked to grip strength reduction in adults over 40 years old (37). Furthermore, there are indications suggesting that a higher quality diet during middle age may be beneficial for muscle mass and function 10−20 years later (58). The precise pathogenesis of skeletal muscle mass loss due to nutrition remains incompletely understood, highlighting the need for further research, particularly prospective intervention studies involving healthy young and middle-aged populations. Studies should specifically focus on cumulative exposure to dietary patterns to provide preventive opportunities.
This study, the first of its kind, assesses the association between UPFs and low muscle mass in a large, nationally representative sample of U.S. adults aged 20−59 years. The analysis considered individual consumption data, taking into account both caloric intake ratios and weight-defined UPF intake. The use of the DXA method for muscle mass measurement in NHANES addresses previous shortcomings related to body surface markers in other studies. Furthermore, the study considered multiple definitions of sarcopenia diagnosis from different guidelines, demonstrating a high level of agreement.
This study was limited in that it was a cross-sectional study which precludes the ability to make causal inferences. Additionally, while a 5-step interview was conducted, the accuracy of the type and amount of food intake was largely dependent on the participants’ recollections. Studies have indicated that 24-hour dietary recall may lead to an underestimation of energy intake by up to 11% (59), though this likely does not affect the dietary contributions established by the study. Furthermore, UPFs were classified by NOVA and some misclassification bias may have arisen due to the fact that the NHANES dietary survey was not designed to distinguish according to the NOVA system. Lastly, the simple classification of physical activity, may have overestimated the strength of the association due to the fact that higher UPF consumption often correlates to an overall unhealthy lifestyle, including a lessened daily activity and exercise.
Conclusion
In conclusion, this large-scale cross-sectional study establishes a significant linear correlation between UPFs and low muscle mass. The results underscore the significance of dietary pattern interventions in promoting optimal muscle health. Considering a healthier and higher-quality diet throughout the entire adult lifespan, associated with known benefits for various health outcomes, including the effective maintenance of muscle mass and function, reducing UPF intake could serve as an effective strategy to prevent low muscle mass in young and middle-aged adults, potentially contributing to better physical function in older age.
Data availability statement
The original contributions presented in this study are included in this article/Supplementary materials, further inquiries can be directed to the corresponding authors.
Ethics statement
The studies involving humans were approved by the CDC/NCHS Research Ethics Review Board. The studies were conducted in accordance with the local legislation and institutional requirements. The participants provided their written informed consent to participate in this study.
Author contributions
WK: Conceptualization, Data curation, Supervision, Visualization, Writing – original draft, Writing – review & editing. YX: Methodology, Validation, Writing – original draft. JH: Data curation, Formal Analysis, Writing – review & editing. WD: Investigation, Project administration, Software, Writing – review & editing. CC: Conceptualization, Supervision, Writing – review & editing.
Funding
The author(s) declare that no financial support was received for the research, authorship, and/or publication of this article.
Acknowledgments
We would like to thank NHANES for providing the public data used in this study.
Conflict of interest
The authors declare that the research was conducted in the absence of any commercial or financial relationships that could be construed as a potential conflict of interest.
Publisher’s note
All claims expressed in this article are solely those of the authors and do not necessarily represent those of their affiliated organizations, or those of the publisher, the editors and the reviewers. Any product that may be evaluated in this article, or claim that may be made by its manufacturer, is not guaranteed or endorsed by the publisher.
Supplementary material
The Supplementary Material for this article can be found online at: https://www.frontiersin.org/articles/10.3389/fnut.2024.1280665/full#supplementary-material
Abbreviations
UPFs, ultra-processed foods; DXA, Dual-Energy X-ray Absorptiometry; NHANES, National Health and Nutrition Examination Survey; FNIH, Foundation for the National Institutes of Health; BMI, body mass index (weight [kg]/height[m]2); OR, odds ratio; CI, confidence intervals; eGFR, estimations of glomerular filtration rate; USDA, United States Department of Agriculture; FNDDS, Food and Nutrient Database for Dietary Studies; UACR, Urinary Albumin to Creatinine Ratio; ALM, appendicular lean mass; DM, diabetes mellitus; EWGSOP2, updated European Working Group on Sarcopenia; AWGS2, Asian Working Group on Sarcopenia (2019); IWGS, International Working Group on Sarcopenia.
References
1. Gibney MJ. Ultra-processed foods: definitions and policy issues. Curr Dev Nutr. (2018) 3:nzy077. doi: 10.1093/cdn/nzy077
2. Wang L, Martínez Steele E, Du M, Pomeranz JL, O’Connor LE, Herrick KA, et al. Trends in consumption of ultraprocessed foods among US youths aged 2-19 years, 1999-2018. JAMA. (2021) 326:519–30. doi: 10.1001/jama.2021.10238
3. Gearhardt AN, Schulte EM. Is food addictive? A review of the science. Annu Rev Nutr. (2021) 41:387–310. doi: 10.1146/annurev-nutr-110420-111710
4. Koiwai K, Takemi Y, Hayashi F, Ogata H, Matsumoto S, Ozawa K, et al. Consumption of ultra-processed foods decreases the quality of the overall diet of middle-aged Japanese adults. Public Health Nutr. (2019) 22:2999–3008. doi: 10.1017/S1368980019001514
5. Wang Y, Wang K, Du M, Khandpur N, Rossato SL, Lo CH, et al. Maternal consumption of ultra-processed foods and subsequent risk of offspring overweight or obesity: results from three prospective cohort studies. BMJ. (2022) 379:e071767. doi: 10.1136/bmj-2022-071767
6. Chen Z, Khandpur N, Desjardins C, Wang L, Monteiro CA, Rossato SL, et al. Ultra-processed food consumption and risk of type 2 diabetes: three large prospective U.S. cohort studies. Diabetes Care. (2023) 46:1335–44. doi: 10.2337/dc22-1993
7. Srour B, Fezeu LK, Kesse-Guyot E, Allès B, Méjean C, Andrianasolo RM, et al. Ultra-processed food intake and risk of cardiovascular disease: prospective cohort study (NutriNet-Santé). BMJ. (2019) 365:l1451. doi: 10.1136/bmj.l1451
8. Lawrence M. Ultra-processed foods: a fit-for-purpose concept for nutrition policy activities to tackle unhealthy and unsustainable diets. Public Health Nutr. (2023) 26:1384–8. doi: 10.1017/S1368980022002117
9. Studenski SA, Peters KW, Alley DE, Cawthon PM, McLean RR, Harris TB, et al. The FNIH sarcopenia project: rationale, study description, conference recommendations, and final estimates. J Gerontol A Biol Sci Med Sci. (2014) 69:547–58. doi: 10.1093/gerona/glu010
10. Keller K, Engelhardt M. Strength and muscle mass loss with aging process. Age and strength loss. Muscles Ligaments Tendons J. (2014) 3:346–50.
11. Liang CK, Peng LN, Lin MH, Loh CH, Lee WJ, Hsiao FY, et al. Long-term mortality risk in older adults with sarcopenia: an 11-year prospective cohort study comparing AWGS 2014 and AWGS 2019 guidelines for enhanced clinical utility and accurate risk prediction. J Nutr Health Aging. (2023) 27(7):507–13. doi: 10.1007/s12603-023-1940-y
12. Cruz-Jentoft AJ, Bahat G, Bauer J, Boirie Y, Bruyère O, Cederholm T, et al. Sarcopenia: revised European consensus on definition and diagnosis. Age Ageing. (2019) 48:16–31. doi: 10.1093/ageing/afy169
13. Fielding RA, Vellas B, Evans WJ, Bhasin S, Morley JE, Newman AB, et al. Sarcopenia: an undiagnosed condition in older adults. Current consensus definition: prevalence, etiology, and consequences. International working group on sarcopenia. J Am Med Dir Assoc. (2011) 12:249–56. doi: 10.1016/j.jamda.2011.01.003
14. Kiss N, Prado CM, Daly RM, Denehy L, Edbrooke L, Baguley BJ, et al. Low muscle mass, malnutrition, sarcopenia, and associations with survival in adults with cancer in the UK Biobank cohort. J Cachexia Sarcopenia Muscle. (2023) 14:1775–88. doi: 10.1002/jcsm.13256
15. Tessier AJ, Wing SS, Rahme E, Morais JA, Chevalier S. Association of low muscle mass with cognitive function during a 3-year follow-up among adults aged 65 to 86 years in the Canadian longitudinal study on aging. JAMA Netw Open. (2022) 5:e2219926. doi: 10.1001/jamanetworkopen.2022.19926
16. Staatz CB, Kelly Y, Lacey RE, Hardy R. Area-level and family-level socioeconomic position and body composition trajectories: longitudinal analysis of the UK Millennium Cohort Study. Lancet Public Health. (2021) 6:e598–607. doi: 10.1016/S2468-2667(21)00134-1
17. Ganmaa D, Bromage S, Khudyakov P, Erdenenbaatar S, Delgererekh B, Martineau A. Influence of vitamin D supplementation on growth, body composition, and pubertal development among school-aged children in an area with a high prevalence of vitamin D deficiency: a randomized clinical trial. JAMA Pediatr. (2023) 177:32–41. doi: 10.1001/jamapediatrics.2022.4581
18. Ciolac EG, Rodrigues-da-Silva JM. Resistance training as a tool for preventing and treating musculoskeletal disorders. Sports Med. (2016) 46:1239–48. doi: 10.1007/s40279-016-0507-z
19. Nagata JM, Vittinghoff E, Pettee Gabriel K, Garber AK, Moran AE, Rana JS, et al. Moderate-to-vigorous intensity physical activity from young adulthood to middle age and metabolic disease: a 30-year population-based cohort study. Br J Sports Med. (2022) 56:847–53. doi: 10.1136/bjsports-2021-104231
20. Compher C, Cederholm T, Correia MI, Gonzalez MC, Higashiguch T, Shi HP, et al. Guidance for assessment of the muscle mass phenotypic criterion for the Global Leadership Initiative on Malnutrition diagnosis of malnutrition. J Parenter Enteral Nutr. (2022) 46:1232–42. doi: 10.1002/jpen.2366
21. Cederholm T, Jensen GL, Correia MI, Gonzalez MC, Fukushima R, Higashiguchi T, et al. GLIM criteria for the diagnosis of malnutrition - A consensus report from the global clinical nutrition community. J Cachexia Sarcopenia Muscle. (2019) 10:207–17. doi: 10.1002/jcsm.12383
22. Álvarez-Herms J, González A, Corbi F, Odriozola I, Odriozola A. Possible relationship between the gut leaky syndrome and musculoskeletal injuries: the important role of gut microbiota as indirect modulator. AIMS Public Health. (2023) 10:710–38. doi: 10.3934/publichealth.2023049
23. Zhang Z, Jackson SL, Martinez E, Gillespie C, Yang Q. Association between ultraprocessed food intake and cardiovascular health in US adults: a cross-sectional analysis of the NHANES 2011-2016. Am J Clin Nutr. (2021) 113:428–36. doi: 10.1093/ajcn/nqaa276
24. Steele EM, O’Connor LE, Juul F, Khandpur N, Galastri Baraldi L, Monteiro CA, et al. Identifying and estimating ultraprocessed food intake in the US NHANES according to the nova classification system of food processing. J Nutr. (2023) 153:225–41. doi: 10.1016/j.tjnut.2022.09.001
25. Bailey RL, Leidy HJ, Mattes RD, Heymsfield SB, Boushey CJ, Ahluwalia N, et al. Frequency of eating in the US population: a narrative review of the 2020 dietary guidelines advisory committee report. Curr Dev Nutr. (2022) 6:nzac132. doi: 10.1093/cdn/nzac132
26. Kim D, Vazquez-Montesino LM, Li AA, Cholankeril G, Ahmed A. Inadequate physical activity and sedentary behavior are independent predictors of nonalcoholic fatty liver disease. Hepatology. (2020) 72:1556–68. doi: 10.1002/hep.31158
27. Srour B, Fezeu LK, Kesse-Guyot E, Allès B, Debras C, Druesne-Pecollo N, et al. Ultraprocessed food consumption and risk of type 2 diabetes among participants of the nutrinet-santé prospective cohort. JAMA Intern Med. (2020) 180:283–91. doi: 10.1001/jamainternmed.2019.5942
28. Fiolet T, Srour B, Sellem L, Kesse-Guyot E, Allès B, Méjean C, et al. Consumption of ultra-processed foods and cancer risk: results from NutriNet-Santé prospective cohort. BMJ. (2018) 360:k322. doi: 10.1136/bmj.k322
29. Confortin SC, Aristizábal LY, Bragança ML, Cavalcante LC, Alves JD, Batista RF, et al. Are fat mass and lean mass associated with grip strength in adolescents? Nutrients. (2022) 14:3259. doi: 10.3390/nu14163259
30. Batsis JA, Villareal DT. Sarcopenic obesity in older adults: aetiology, epidemiology and treatment strategies. Nat Rev Endocrinol. (2018) 14:513–37. doi: 10.1038/s41574-018-0062-9
31. Salinas-Rodríguez A, Palazuelos-González R, Gonzalez-Bautista E, Manrique-Espinoza B. Editorial: sarcopenia, cognitive function, and the heterogeneity in aging. J Nutr Health Aging. (2023) 27:240–2. doi: 10.1007/s12603-023-1910-1
32. Attaway AH, Bellar A, Welch N, Sekar J, Kumar A, Mishra S, et al. Gene polymorphisms associated with heterogeneity and senescence characteristics of sarcopenia in chronic obstructive pulmonary disease. J Cachexia Sarcopenia Muscle. (2023) 14:1083–95. doi: 10.1002/jcsm.13198
33. Meyer KA, Taillie LS. Intake of ultraprocessed foods among US youths: health concerns and opportunities for research and policy. JAMA. (2021) 326:485–7. doi: 10.1001/jama.2021.9845
34. Vandevijvere S, Jaacks LM, Monteiro CA, Moubarac JC, Girling-Butcher M, Lee AC, et al. Global trends in ultraprocessed food and drink product sales and their association with adult body mass index trajectories. Obes Rev. (2019) 20 Suppl. 2:10–9. doi: 10.1111/obr.12860
35. Gadelha PC, de Arruda IK, Coelho PB, Queiroz PM, Maio R, da Silva Diniz A. Consumption of ultraprocessed foods, nutritional status, and dyslipidemia in schoolchildren: a cohort study. Eur J Clin Nutr. (2019) 73:1194–9. doi: 10.1038/s41430-019-0404-2
36. Travinsky-Shmul T, Beresh O, Zaretsky J, Griess-Fishheimer S, Rozner R, Kalev-Altman R, et al. Ultra-processed food impairs bone quality, increases marrow adiposity and alters gut microbiome in mice. Foods. (2021) 10:3107. doi: 10.3390/foods10123107
37. Zhang S, Gu Y, Rayamajhi S, Thapa A, Meng G, Zhang Q, et al. Ultra-processed food intake is associated with grip strength decline in middle-aged and older adults: a prospective analysis of the TCLSIH study. Eur J Nutr. (2022) 61:1331–41. doi: 10.1007/s00394-021-02737-3
38. Viola PC, Carvalho CA, Bragança ML, França AK, Alves MT, da Silva AA. High consumption of ultra-processed foods is associated with lower muscle mass in Brazilian adolescents in the RPS birth cohort. Nutrition. (2020) 79-80:110983. doi: 10.1016/j.nut.2020.110983
39. Monteles Nascimento L, de Carvalho Lavôr LC, Mendes Rodrigues BG, da Costa Campos F, de Almeida Fonseca Viola PC, Lucarini M, et al. Association between consumption of ultra-processed food and body composition of adults in a capital city of a Brazilian region. Nutrients. (2023) 15:3157. doi: 10.3390/nu15143157
40. Liu X, Hou L, Zhao W, Xia X, Hu F, Zhang G, et al. The comparison of sarcopenia diagnostic criteria using AWGS 2019 with the other five criteria in West China. Gerontology. (2021) 67:386–96. doi: 10.1159/000513247
41. Bizzozero-Peroni B, Martínez-Vizcaíno V, Garrido-Miguel M, Fernández-Rodríguez R, Torres-Costoso A, Ferri-Morales A, et al. The association between meat consumption and muscle strength index in young adults: the mediating role of total protein intake and lean mass percentage. Eur J Nutr. (2023) 62:673–83. doi: 10.1007/s00394-022-03014-7
42. Frampton J, Murphy KG, Frost G, Chambers ES. Higher dietary fibre intake is associated with increased skeletal muscle mass and strength in adults aged 40 years and older. J Cachexia Sarcopenia Muscle. (2021) 12:2134–44. doi: 10.1002/jcsm.12820
43. Ruiz A, Dror E, Handschin C, Furrer R, Perez-Schindler J, Bachmann C, et al. Over-expression of a retinol dehydrogenase (SRP35/DHRS7C) in skeletal muscle activates mTORC2, enhances glucose metabolism and muscle performance. Sci Rep. (2018) 8:636. doi: 10.1038/s41598-017-18844-3
44. Alonso N, Meinitzer A, Fritz-Petrin E, Enko D, Herrmann M. Role of vitamin K in bone and muscle metabolism. Calcif Tissue Int. (2023) 112:178–96. doi: 10.1007/s00223-022-00955-3
45. Oh GS, Lee JH, Byun K, Kim DI, Park KD. Effect of intake of leucine-rich protein supplement in parallel with resistance exercise on the body composition and function of healthy adults. Nutrients. (2022) 14:4501. doi: 10.3390/nu14214501
46. Taheri M, Chilibeck PD, Cornish SM. A brief narrative review of the underlying mechanisms whereby omega-3 fatty acids may influence skeletal muscle: from cell culture to human interventions. Nutrients. (2023) 15:2926. doi: 10.3390/nu15132926
47. Lawrence M. Ultra-processed foods: a fit-for-purpose concept for nutrition policy activities to tackle unhealthy and unsustainable diets. Br J Nutr. (2022) 129:1–4. doi: 10.1017/S000711452200280X
48. Suez J, Korem T, Zeevi D, Zilberman-Schapira G, Thaiss CA, Maza O, et al. Artificial sweeteners induce glucose intolerance by altering the gut microbiota. Nature. (2014) 514:181–6. doi: 10.1038/nature13793
49. Csáki KF. Synthetic surfactant food additives can cause intestinal barrier dysfunction. Med Hypotheses. (2011) 76:676–81. doi: 10.1016/j.mehy.2011.01.030
50. Ma Y, Zhang J, Yu N, Shi J, Zhang Y, Chen Z, et al. Effect of nanomaterials on gut microbiota. Toxics. (2023) 11:384. doi: 10.3390/toxics11040384
51. Abiega-Franyutti P, Freyre-Fonseca V. Chronic consumption of food-additives lead to changes via microbiota gut-brain axis. Toxicology. (2021) 464:153001. doi: 10.1016/j.tox.2021.153001
52. Song Z, Song R, Liu Y, Wu Z, Zhang X. Effects of ultra-processed foods on the microbiota-gut-brain axis: the bread-and-butter issue. Food Res Int. (2023) 167:112730. doi: 10.1016/j.foodres.2023.112730
53. Vrieze A, Holleman F, Zoetendal EG, de Vos WM, Hoekstra JB, Nieuwdorp M. The environment within: how gut microbiota may influence metabolism and body composition. Diabetologia. (2010) 53:606–13. doi: 10.1007/s00125-010-1662-7
54. Álvarez-Herms J, González-Benito A, Corbi F, Odriozola A. What if gastrointestinal complications in endurance athletes were gut injuries in response to a high consumption of ultra-processed foods? Please take care of your bugs if you want to improve endurance performance: a narrative review. Eur J Appl Physiol. (2023). doi: 10.1007/s00421-023-05331-z [Epub ahead of print].
55. Yang Y, Ju L, Fan J, Cai S, Sun L, Li Y. Association of urinary phthalate metabolites with sarcopenia in US adults: NHANES 1999-2006. Environ Sci Pollut Res Int. (2022) 29:7573–82. doi: 10.1007/s11356-021-16202-5
56. Robinson S, Granic A, Cruz-Jentoft AJ, Sayer AA. The role of nutrition in the prevention of sarcopenia. Am J Clin Nutr. (2023) 118:852–64. doi: 10.1016/j.ajcnut.2023.08.015
57. Van Elswyk ME, Teo L, Lau CS, Shanahan CJ. Dietary patterns and the risk of sarcopenia: a systematic review and meta-analysis. Curr Dev Nutr. (2022) 6:nzac001. doi: 10.1093/cdn/nzac001
58. Davis JA, Mohebbi M, Collier F, Loughman A, Staudacher H, Shivappa N, et al. The role of diet quality and dietary patterns in predicting muscle mass and function in men over a 15-year period. Osteoporos Int. (2021) 32:2193–203. doi: 10.1007/s00198-021-06012-3
Keywords: UPFs, low muscle mass, sarcopenia, ALM, NHANES
Citation: Kong W, Xie Y, Hu J, Ding W and Cao C (2024) Higher ultra processed foods intake is associated with low muscle mass in young to middle-aged adults: a cross-sectional NHANES study. Front. Nutr. 11:1280665. doi: 10.3389/fnut.2024.1280665
Received: 31 August 2023; Accepted: 09 February 2024;
Published: 19 February 2024.
Edited by:
Theocharis Ispoglou, Leeds Beckett University, United KingdomReviewed by:
Jesús Álvarez-Herms, Ministerio de Educación Cultura y Deporte, SpainDeaglan McCullough, Leeds Beckett University, United Kingdom
Copyright © 2024 Kong, Xie, Hu, Ding and Cao. This is an open-access article distributed under the terms of the Creative Commons Attribution License (CC BY). The use, distribution or reproduction in other forums is permitted, provided the original author(s) and the copyright owner(s) are credited and that the original publication in this journal is cited, in accordance with accepted academic practice. No use, distribution or reproduction is permitted which does not comply with these terms.
*Correspondence: Weiliang Kong, TGl2ZWtvbmdAaG90bWFpbC5jb20=; Chao Cao, Q2FvY2RvY3RvckAxNjMuY29t