- 1College of Physical Education, Shaanxi University of Technology, Hanzhong, China
- 2Shaanxi Province Key Laboratory of Bioresources, QinLing-Bashan Mountains Bioresources Comprehensive Development C. I. C., Qinba State Key Laboratory of Biological Resources and Ecological Environment, College of Bioscience and Bioengineering, Shaanxi University of Technology, Hanzhong, Shaanxi, China
- 3College of Health Management, Shangluo University, Shangluo, Shaanxi, China
- 4ShaanxiUnion Research Center of University and Enterprise for Health Food Ingredient and Walnut Industry, Shangluo, Shaanxi, China
- 5Pak-Austria Fachhochschule lnstitute of Applied Sciences and Technology, Haripur, Pakistan
- 6Department of Chemical Technology,Faculty of Science, Chulalongkorn University, Bangkok, Thailand
- 7Department of Pharmacology, Faculty of Veterinary Medicine, Cairo University, Giza, Egypt
- 8Department of Medical Pharmacology, Faculty of Medicine, Atatürk University, Erzurum, Türkiye
Introduction: Cornus officinalis sieb. et zucc, a deciduous tree or shrub, is renowned for its “Cornus flesh” fruit, which is widely acknowledged for its medicinal value when matured and dried. Leveraging C. officinalis as a foundational ingredient opens avenues for the development of environmentally friendly health foods, ranging from beverages and jams to preserves and canned products. Packed with diverse bioactive compounds, this species manifests a spectrum of pharmacological effects, including anti-inflammatory, antioxidant, antidiabetic, immunomodulatory, neuroprotective, and cardiovascular protective properties.
Methods: This study employs CiteSpace visual analysis software and a bibliometric analysis platform, drawing upon the Web of Science (WOS) database for literature spanning the last decade. Through a comprehensive analysis of available literature from WOS and Google Scholar, we present a thorough summary of the health benefits, phytochemistry, active compounds, and pharmacological effects of C. officinalis. Particular emphasis is placed on its potential in developing functional drugs and foods.
Results and Discussion: While this review enhances our understanding of C. officinalis as a prospective therapeutic agent, its clinical applicability underscores the need for further research and clinical studies to validate findings and establish safe and effective clinical applications.
1 Introduction
Cornus officinalis, also known as Fructus corni or dogwood, belongs to the Cornaceae family and can be found as a tree or shrub. It originated from the Caucasus region and subsequently spread across Turkey, Romania, Bulgaria, and other parts of continental Europe (1, 2). This species thrives in warm climates, with optimal growth occurring at temperatures between 20 and 30°C, while growth is hindered at temperatures exceeding 35°C. Dogwood flowers blossom during the spring season, and the fruits of the dogwood tree transform into a vibrant red color during autumn. The leaves of C. officinalis are arranged opposite to each other, resembling elm leaves, but they are more pronounced and lack serrations. The fruit of this plant is oval-shaped and possesses a sour taste.
The growth of dogwoods can be categorized into four stages: the juvenile stage, early fruiting stage, fruiting stage, and senescence stage (3). C. officinalis holds a significant position in traditional Chinese medicine, boasting a rich history in China (4). It is one of the key components of “Liu Wei Di Huang Wan” and contains various beneficial substances, such as ursolic acid, gallic acid, malic acid, saponins, phenols, resins, vitamin A, vitamin C, and others (5, 6). These components contribute to its biofunctionality, which includes safeguarding the cardiovascular system, boosting the immune system, and exhibiting anti-inflammatory, antibacterial, and antioxidant properties, as well as aiding in lowering blood lipids and enhancing human memory. Despite the abundance of active substances in C. officinalis and its positive effects on the body, it possesses a sour taste. The primary forms in which Cornus officinalis is consumed include dried fruit, used as a traditional functional seasoning, and jams (Figure 1). While C. officinalis holds considerable nutritional value, further research and development in the food industry are still needed. This review aims to elucidate the biological activity, health benefits, and potential applications of C. officinalis in food and medicine while also providing an overview of the current research status and future prospects for C. officinalis.
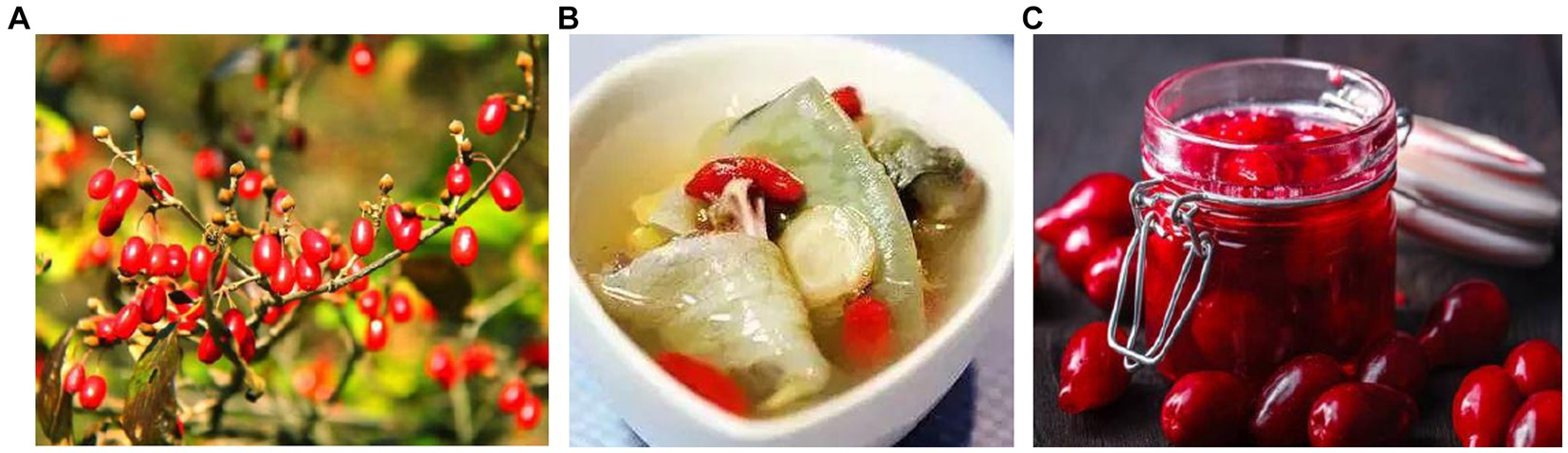
Figure 1. Cornus officinalis and its main products. (A) Fresh fruit; (B) dry fruit as functional seasoning; (C) Jam.
2 Literature analysis of Cornus officinalis research
CiteSpace software, developed by Dr. Chenchaomei at Redsell University in the United States, is a powerful visual analysis tool designed to explore the multidimensional, temporal, and dynamic aspects of scientific literature (7–10). In this study, the Web of Science (WOS) database serves as the primary source of information. The CiteSpace analysis tool (11, 12) was employed to investigate the current developmental status and identify the research hotspots related to C. officinalis. Herein, we utilized the Web of Science (WOS) database as the retrieval platform and conducted a literature search using “Cornus officinalis,” “ingredients,” or “activities” or “pharmacology” as the keyword. The search encompassed papers published between 2013 and 2023. Following the screening process, a total of 233 papers focusing on the bioactivity of C. officinalis were obtained. The selected literature was exported and saved for further analysis. CiteSpace software was employed to study and cluster the authors and keywords. To gain deeper insights into the research field of C. officinalis, we utilized both the WOS platform1 and the bibliometric analysis platform2 (13–15). By leveraging these platforms, we examined and analyzed the collaborative relationships and the number of published documents within the field of C. officinalis research (14, 16, 17).
2.1 Analysis of annual document issuance
The annual publication count serves as an indicator of the developmental trajectory and dynamics within a specific research field, providing insights into its level of popularity and interest (18). In Figure 2, we present the fitted and analyzed data showing the number of publications focused on the bioactivity of C. officinalis research from 2013 to 2023. Since 2017, there has been a notable increase in the core literature on C. officinalis, driven by a growing demand for health products and the expanding herbal medicine sector. However, in 2023, there appears to be a relative decline in the number of publications on dogwood. This decline could be attributed to the extensive research conducted in recent years on the chemical constituents and pharmacological effects of C. officinalis, leading to a comprehensive understanding of its biological properties both domestically and internationally. Consequently, there has been a relative deceleration in C. officinalis research post-2023. Additionally, in 2023, the primary production region of dogwood experienced extreme weather conditions, with temperatures dropping from 25 to 28°C to 1–3°C below zero. This climatic shift resulted in a reduction in dogwood production, significantly impacting the overall national output. The study’s findings shed light on the current status of C. officinalis research and its future outlook.
Through statistical analysis of the global publication count on the bioactivity of C. officinalis, as well as the publication counts from different countries, notable findings emerged. As illustrated on the right side of Figure 3, the top 10 countries in terms of publication volume during this decade were China, South Korea, the USA, Japan, Poland, Germany, Australia, India, the Czech Republic, and Malaysia. Notably, China had a significantly higher number of publications than the second-ranked countries, South Korea and the United States. From 2013 to 2020, the number of publications on the bioactivity of C. officinalis remained relatively stable. However, a substantial increase was observed from 2021 to 2023, primarily driven by China and South Korea. Particularly in 2021, there was a sudden surge in the number of publications related to C. officinalis bioactivity, with China being the primary contributor. Over the past 10 years, China has demonstrated rapid and prominent development in C. officinalis bioactivity research, consistently leading the field and making significant contributions.
2.2 Analysis of published periodicals
The research focus in this field can be inferred from the volume of literature published in relevant journals (19). Analysis of the industry fields of the selected documents in the WOS database (Figure 4) reveals that the research direction primarily lies within the domains of pharmacology, pharmacy, medicinal chemistry, and plant sciences. Table 1 presents the analysis of selected journals obtained through the bibliometric online analysis platform. The top three journals in terms of publication volume are JOURNAL OF ETHNOPHARMACOLOGY, FRONTIERS IN PHARMACOLOGY, and MOLECULES. The dominance of these journals, along with other top-ranking journals in terms of publication volume, reflects the significant academic influence of C. officinalis bioactivity research within leading national journals. Notably, the most published and cited paper is from the JOURNAL OF ETHNOPHARMACOLOGY. Although the study of C. officinalis bioactivity has gained international recognition, there is still ample room for further research in this field. This holds great significance for the development of the C. officinalis industry.
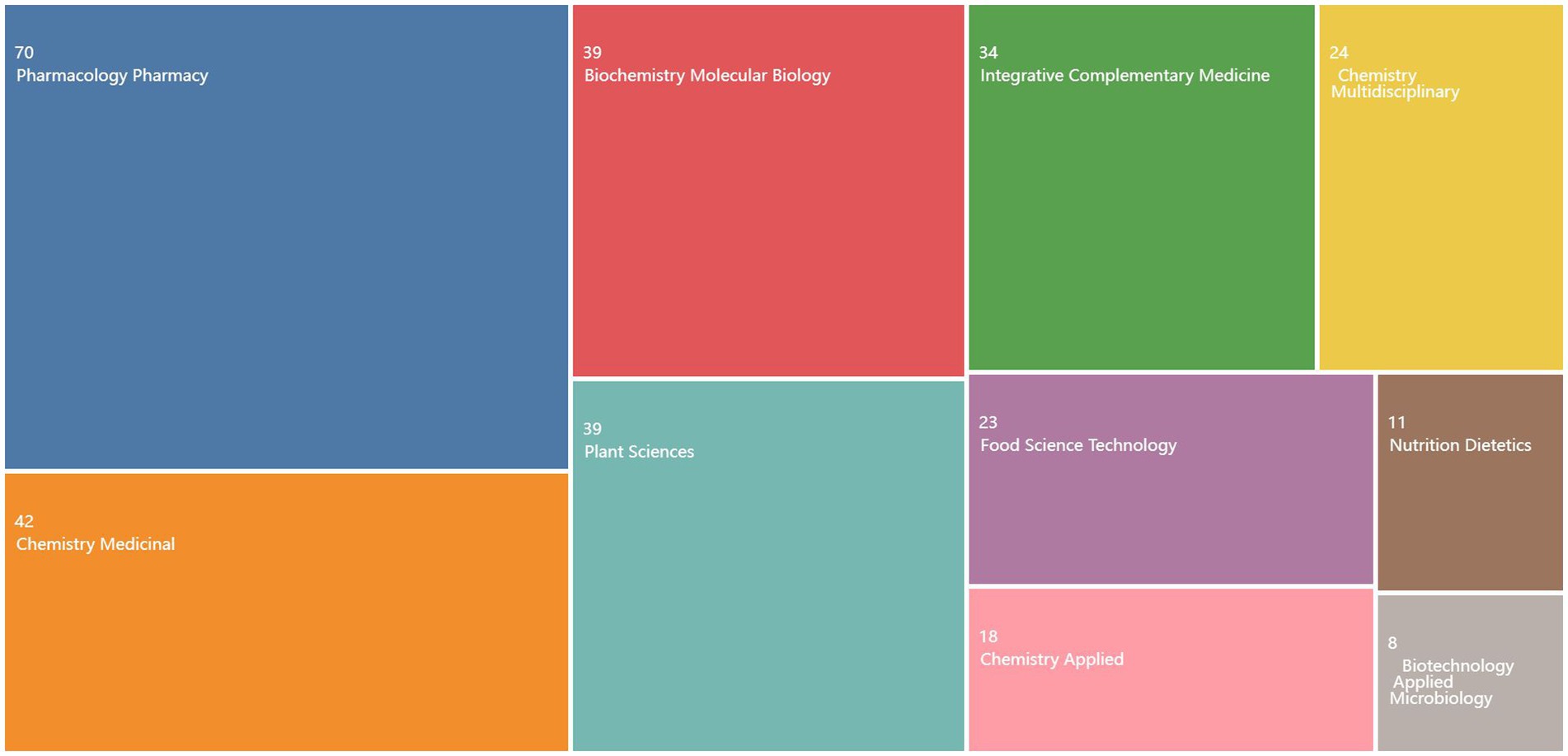
Figure 4. Analysis of global journal publications on the bioactivity of C. officinalis from 2013 to 2023.
2.3 Analysis of hotspot research
This section examines the research hotspots in the field of biological activity of C. officinalis by analyzing the keywords used in scholarly studies. The visualization of these keywords is presented in Figure 5. The identified keywords related to the biological activities of Cornus officinalis included “loganin,” “morroniside,” “expression,” “oxidative stress,” “inflammation,” “iridoid glycoside,” “fructus,” “identification,” “activation,” and “cell,” in addition to the keyword “Cornus officinalis” itself. This progressive exploration of the biological activity of C. officinalis holds significant importance for the development of the C. officinalis industry.
Figure 6 presents the cluster analysis of bioactive keywords related to C. officinalis in the WOS database. This analysis helps to elucidate the interrelationships among keywords. The clustering modules, as depicted in the figure, reveal that research on the biological activity of C. officinalis has evolved from plant extracts to encompass oxidative stress, morroniside, cornel iridoid glycoside, flavonoids, and other activities and mechanisms associated with C. officinalis products. This signifies a growing depth of research in this field.
Figure 7 presents an analysis of keyword occurrences in the WOS database pertaining to the bioactivity study of C. officinalis. The frequency of keywords provides insights into the evolving research focus over time. In earlier periods, there was a greater emphasis on investigating the composition of C. officinalis. However, starting in 2016, keywords such as iridoid glycoside and constituent gradually gained prominence. This suggests that research on C. officinalis extends beyond its basic components and encompasses the study of specific active substances within the plant. From 2019 onward, there was a notable increase in keywords such as protein, inflammation, and biological activity, indicating a deeper exploration of the diverse bioactivity of C. officinalis. This shift signifies a more comprehensive investigation into the potential medicinal properties of the plant.
3 Classification and distribution
Cornus officinalis, a species native to the northern United States, encompasses various subspecies, including European Cornus (C. mas), Japanese Cornus, Chinese Cornus (C. officinalis), and Pacific Cornus (C. nuttallii). In China, C. officinalis has a wide distribution, with areas such as Lin’an County in the Tianmu Mountains region of northwestern Zhejiang Province (30 N, 119E), Nanyang County in the Fuyu Mountains region of southwestern Henan Province (33 N, 112E), and Hanzhong County in the Qinlingba Mountains region of southwestern Shaanxi Province (32 N, 107E) (20–23). It is also found in certain regions of Japan and South Korea (24, 25). The variation in environmental factors such as climate, soil composition, temperature, and humidity contributes to differences in the content of active substances, pulp quality, and even taste of C. officinalis across different regions. These active substances play a crucial role in the pharmacological effects of C. officinalis, leading to varying outcomes in different geographical areas (22). Recognizing its unique biological activity and pharmacological effects, China has designated Cornus officinalis as a medicinal plant under national protection (23, 26).
Kingdom: Plants.
Phylum: Angiospermae.
Class: Monocotyledons.
Tribe: Cornus.
Suborder: Cornus.
Order: Cornus.
Family: Cornus.
Genus: Cornus.
Species: Cornus officinalis.
Distribution: China, Korea, USA, Japan.
4 Phytochemistry of Cornus officinalis
Ongoing investigation into C. officinalis has unveiled a multitude of bioactive substances with promising health advantages. Comprehensive research has identified hundreds of active compounds derived from different plant components (Table 2 provides details on some of these compounds).
Initially, the edible part of Cornus officinalis primarily consisted of fruit pulp (40). However, ongoing research has revealed the presence of varying levels of active substances in different parts of the plant. For instance, a study identified three active compounds, namely, 3,3′-di-O-methylellagic acid 4-(5″-acetyl)-α-l-arabinofuranoside, 6α-dihydrocornic acid, and 6β-dihydrocornic acid, extracted from the roots of C. officinalis (41, 42). Flavonoid substances such as β-sitosterol, glucose, and sucrose were extracted from the leaves (43, 44), and the ethanolic extract of the leaves yielded approximately 30–40 active substances, including phenols, esters, ketones, tannins, and organic acids (as listed in Table 3) (38, 51). Other studies have focused on the leaves, resulting in the extraction of three new iridoids, one of which exhibited inhibitory effects on the lung cancer cell line A-549 (52). Moreover, five substances with α-glucosidase inhibitory activity were isolated from the aqueous extract of C. officinalis fruits, named cornucadinoside A-E (1–5) (53). Additionally, research on C. officinalis seeds led to the isolation of eight substances, including various galloyl-glucose compounds and phenolic acids (54–59). Ursolic acid, obtained from the seeds or peels of C. officinalis, showed nontoxic properties in LD50 tests and single-cell gel electrophoresis (60, 61). While the majority of active substances are found in the fruit, studies have also identified new compounds, such as cornuside, cornusiin G, methyl malate, and sedoheptulose gallate, highlighting the presence of dimeric hydrolyzable tannins and other molecular components (62, 63). Furthermore, the fruits of C. officinalis yielded a new bisiridoid glucoside named cornutide, as well as 7β-O-dimethyl butanedioate morroniside and caffeoyltartaric acid dimethyl ester, which were structurally characterized using various spectroscopic analyses (64, 65).
5 Bioactive compounds in Cornus officinalis
5.1 Cyclic enol ether terpene glycosides in Cornus officinalis
Cyclic enol ether terpene glycosides are widely distributed in nature, primarily in dicotyledonous plants. Various herbal medicines, including Dihuang, Xuan Shen, Strychnos, Lonicera, and Gentian, contain cyclic enol ether glycosides (66, 67). Cyclic enol ether terpenes encompass different structural types, such as cyclic enol ether glycosides, cleaved cyclic enol ether terpene glycosides, and cyclic enol ether terpene esters. Cyclic enol ether terpene glycosides in C. officinalis are shown in Table 4 (75).
Cyclic enol ether glycosides are highly abundant bioactive compounds found in various parts of C. officinalis, including the fruit, peel, shell, and branches. Researchers have employed different extraction methods to isolate these compounds from C. officinalis (76). In one study, dried fruits were crushed and subjected to methanol extraction using an ultrasonic extraction method. The crude extract was further purified by passing it through macroporous resin to eliminate impurities such as pigments, resulting in a substantial yield of cyclic enol ether terpene glycosides. Another approach involved microwave irradiation for the extraction of these glycosides from C. officinalis. The optimized conditions included using 72% ethanol as the solvent, a liquid-to-material ratio of 15 mL/g, 10 min of microwave power at 400 W, and two consecutive extraction cycles. These extraction methods have proven effective in obtaining high concentrations of cyclic enol ether glycosides from C. officinalis. As research has progressed, a diverse array of cyclic enol ether terpene glycosides have been identified and extracted from C. officinalis. Among them are loganin, sweroside, morroniside, kingiside, loganic acid, 10-hydroxycornin, 7-ketologanin, 7-O-methylmorroniside, cornuside I, 8-epikingiside, secologanoside, 10-hydroxyhastatoside, hastatoside, dihydrocornin, swertimarin, secologanin, cornin, 7-O-methylmorroniside, cornuside II, and dehydromorroniside aglycone. These compounds represent a diverse range of cyclic enol ether terpene glycosides derived from C. officinalis (77–82) (Figure 8).
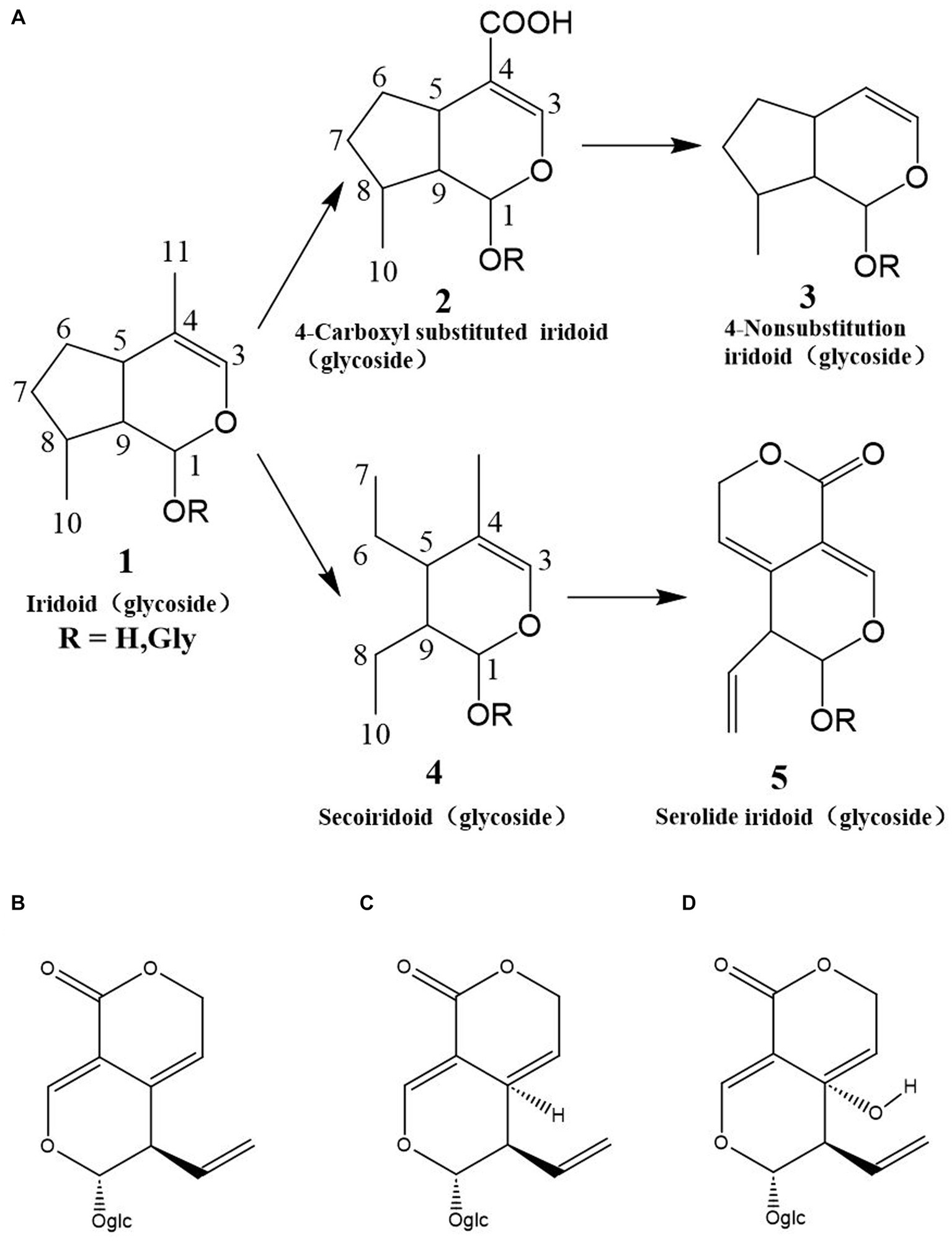
Figure 8. Classes of cyclic enolether glycosides and their interaction relationships. (A) Classification workframe; (B) Gentiopicri; (C) Sweroside; (D) Swertiamarin.
Morroniside, derived from the medicinal plant C. officinalis, is an atypical secoiridoid that possesses a unique six-membered cyclic endoether fragment. This compound belongs to the class of iridoid glycosides and has been found to exhibit potent antioxidant properties (83). In studies investigating the effects of morroniside on cytotoxicity induced by hydrogen peroxide in human neuroblastoma tumor SH-SY5Y cells, it was observed that morroniside effectively reduced intracellular calcium accumulation, mitigated hydrogen peroxide-induced mitochondrial membrane potential (MMP) disruption, and decreased the percentage of apoptosis triggered by hydrogen peroxide (84). Loganin, extracted from the fruit of C. officinalis, was evaluated in male mice subjected to destabilization of the medial meniscus of the right knee (DMM). Mice were treated with loganin at concentrations of 30 or 100 μg/mL injected into the osteoarthritic space for a period of 2–3 months. The results demonstrated that loganin exhibited a protective effect by slowing the progression of osteoarthritis, highlighting its potential for the treatment of this condition (83). The impact of cornelian cyclic enol ether terpene glycosides on platelet aggregation and bleeding time has been investigated in animal studies involving mice and rabbits. The findings revealed that these glycosides effectively inhibited platelet aggregation induced by adenosine diphosphate (ADP) both in vitro and in vivo. Moreover, the effects on bleeding time were examined using a tail break assay in mice, which demonstrated that high doses of cornelian cyclic enol ether terpene glycosides had the ability to prolong bleeding time in rats (85).
5.2 Flavonoids
Flavonoids are a class of phenolic compounds that are widely distributed in various plants, including flowers and vegetables. They are considered plant secondary metabolites and are characterized by their C6-C3-C6 structure (86, 87). This structure consists of two benzene rings (A and B rings) interconnected by a central three-carbon chain, and the C3 part can either form a six-membered ring with the C6 part or be a lipid chain. Flavonoids encompass a diverse group of compounds, and they are generally categorized into flavones and flavonols based on the basic structure of their parent nucleus. Additionally, there are other variations and derivatives of flavonoids, such as isoflavones, which have their B ring attached to the C3 position, and they can be further divided into isoflavones and dihydroisoflavones.
Flavonoids encompass a variety of compounds, including well-known subclasses such as anthocyanins, proanthocyanidins, isoflavones, and catechols. These compounds are commonly found in nature as glycosides or with carbon glycosyl groups attached to sugars. Flavonoids possess significant medicinal value and have been associated with benefits such as improving cardiovascular health, preventing arterial apoptosis, reducing the risk of cancer, and promoting overall well-being. Furthermore, they exhibit diverse bacteriostatic effects against various microorganisms, including gram-positive bacteria, gram-negative bacteria, and fungi (88, 89). Among the subclasses of flavonoids, flavones are particularly noteworthy. They are characterized by two phenolic hydroxyl groups on the benzene rings (A and B) connected by a three-carbon atom, forming a fundamental parent nucleus known as 2-phenylchromone. Flavonols, on the other hand, contain ketone groups. Some examples of flavonols include kaempferol, quercetin, prunetin, and fisetin. Foods such as apples, tomatoes, and kale are abundant sources of flavonols (90).
Cornus officinalis, including its fruits and leaves, is a rich source of flavonoids, which exhibit notable properties in terms of tumor inhibition and antioxidant activity. This characteristic makes C. officinalis highly promising for pharmacological development (91, 92). In one study, flavonoids were extracted from dried C. officinalis using a direct warm immersion method, where 70% ethanol was added to the plant material. The extraction process involved a constant water bath at 80°C for 2 h, followed by cooling and filtration. Another investigation explored the use of ultrasonic treatment with 70% ethanol at 80% power and 60°C for 20 min to extract flavonoids. The total flavonoid content in C. officinalis was determined by adding 1 mL of the test sample to a test tube, followed by the addition of 10 mL of diethylene glycol reagent and 1 mL of NaOH. The mixture was vigorously shaken and allowed to react in hot water at 37°C for 60 min. Finally, the absorbance at 420 nm was measured, and a standard curve was prepared (93).
An investigation focused on the activity-guided isolation of an 80% acetone extract from C. officinalis, which resulted in the identification of various flavonoids, including catechin, quercetin-3-O-β-D-glucuronide, quercetin-3-O-β-D-glucopyranoside, and kaempferol-3-O-β-D-glucopyranoside (94). Furthermore, several other flavonoids have been isolated from C. officinalis, such as kaempferol-3-O-β-D-galactoside, quercetin, rutin, quercetin-3-O-a-L-rhamnosyl-(1–6)-β-D-galactoside, and kaempferol-3-O-a-L-rhamnosyl-(1→6)-β-D-glucoside. These bioactive compounds are extracted from C. officinalis and exhibit promising potential for human health development.
A study was conducted to evaluate the antibacterial and antioxidant properties of flavonoids extracted from C. officinalis. The results demonstrated a significant impact of these flavonoids on inhibiting the activity of Salmonella (95). Moreover, the DPPH radical scavenging rate and hydroxyl radical scavenging rate of C. officinalis leaf flavonoids at a concentration of 1.2 mg/mL exceeded 75%, indicating strong antioxidant activity. The study also identified three different flavonoids, namely, 5-hydroxy-6,7,8,3′,4′,5′-hexamethoxyflavon-3-ol, demethyldigicitrin, and quercetin, extracted from the ethanolic extract of C. officinalis. Their EC50 values were measured as 2.74 ± 0.10, 3.41 ± 0.09, and 1.27 ± 0.25 μg/mL, while their IC50 values were 27.91 ± 0.18, 28.92 ± 0.12, and 81.38 ± 0.33 μg/mL, respectively. These findings provide empirical evidence supporting the medicinal potential of C. officinalis (96).
5.3 Polysaccharide
One of the primary active constituents of C. officinalis is polysaccharides (97). The dehydration and condensation of several monosaccharides lead to the formation of macromolecular polysaccharides, which are prevalent and significant in nature. In specific studies, C. officinalis polysaccharides have been modeled using artificial neural networks (ANN) and optimized through the application of genetic algorithms coupled with ANNs (GA-ANN). The optimal extraction parameters for C. officinalis polysaccharides were determined, and statistical techniques demonstrated that ANN could accurately estimate the yield of C. officinalis polysaccharides. The settings that yielded the best results (7.85 ± 0.09%) were as follows: 350 W of ultrasonic power, an extraction temperature of 51°C, a liquid–solid ratio of 17 mL/g, and an extraction time of 38 min (98). To optimize the extraction of polysaccharides from C. officinalis fruits and investigate the impact of various factors on the extractable content of polysaccharides, response surface methodology (RSM) has also been employed. The results indicated that after optimization, the polysaccharide extraction rate was 9.29 ± 0.31% at an extraction temperature of 55°C, ultrasound time of 97 min, pH of 4.2, and a dosage of 2.15% of the complex enzyme (99). The polysaccharides in C. officinalis were further purified using DEAE-52 and Sephadex G-100 chromatography, revealing the presence of glucose, arabinose, fucose, xylose, mannose, and rhamnose (100).
The diverse array of bioactivities and pharmacological effects exhibited by C. officinalis polysaccharides has captured the attention of researchers both domestically and internationally. These polysaccharides offer a range of benefits, including immunomodulatory, cardiovascular protective, antioxidant, hypoglycemic, and anticancer properties. In a study utilizing an acute myocardial infarction (AMI) rat model to investigate the impact of C. officinalis polysaccharides on AMI rats, the results, compared with the model group, revealed an increase in left ventricular systolic pressure (LVSP) and a significant reduction in the myocardial infarction area. This suggests that C. officinalis polysaccharides play a crucial role in enhancing cardiac function and reducing the size of myocardial infarcts, possibly through the activation of the GSK-3β signaling pathway, imparting a cardioprotective effect (101). Furthermore, research has explored the antibacterial and antioxidant properties of polysaccharides derived from C. officinalis leaves. The isolated polysaccharide fractions demonstrated robust antioxidant activity by effectively scavenging DPPH, hydroxyl, and superoxide anion radicals. Additionally, they exhibited antibacterial efficacy against Salmonella typhimurium, Bacillus subtilis, Escherichia coli, and Staphylococcus aureus (102).
5.4 Amino acids
The abundance of amino acids in C. officinalis is noteworthy. Metabolomic analysis conducted in a specific study identified the presence of 17 amino acids in C. officinalis fruits, encompassing all seven essential amino acids determined by biological techniques (103). Notably, the amino acid composition, with aspartic acid (Asp), glutamic acid (Glu), and leucine (Leu) being the predominant ones, exhibited pharmacological activity (3). These amino acids play a pivotal role in the metabolic control of glycolipids, kidney strengthening, and liver promotion, contributing to the functional aspects of C. officinalis (104, 105). Furthermore, current nutritional research suggests that foods with excessively high amino acid contents may diminish the nutritional value of proteins. Analyzing the amino acid content of Cornus sativus using the amino acid ratio coefficient method revealed that the quantity of amino acids in C. officinalis is reasonably balanced, aligning with the body’s requirements for effective absorption and utilization of amino acids (106, 107).
6 Pharmacology of Cornus officinalis
6.1 Antioxidant effect
A study was conducted to investigate the antioxidant properties of C. officinalis fruits. By measuring its total phenolic and flavonoid content, DPPH radical scavenging rate, and ABTS radical scavenging rate, the study determined that Cornus officinalis exhibited good antioxidant properties (108). The IC50 values for DPPH and ABTS radical scavenging rates were measured as 99.32 μg/mL and 138.51 μg/mL, respectively. This indicates the ability of C. officinalis to effectively scavenge free radicals and exhibit antioxidant activity. In another study focusing on the ethanolic extract of C. officinalis and its potential effect on atopic dermatitis (AD), the EC50 values for the DPPH radical scavenging rate, ferric reducing antioxidant capacity, and ABTS radical scavenging rate were measured as 1.82, 10.76, and 0.6 mg/mL, respectively. These findings suggest that the ethanolic extract of C. officinalis possesses antioxidant activity, further supporting its potential application in promoting skin health (109). Furthermore, a study investigated the antioxidant capacity of C. officinalis extract in protecting against oxidative damage induced by tert-butyl hydroperoxide (t-BHP) in Chang cells. The results demonstrated that C. officinalis extract exhibited antioxidant capacity by enhancing cell viability and preventing the generation of reactive oxygen species (110). Additionally, a study evaluated the antioxidant activity of 20 different varieties of C. officinalis plants using various photometric methods. The antioxidant activity, measured by the DPPH method (2,2-diphenyl-1-propenyl hydrazide) (μmol Trolox/g), ranged from 5.94 (Kozerog) to 16.56 (Kostia). The ABTS method (2,2-amino-3-ethylbenzothiazoline-6-sulfonic acid) yielded values ranging from 13.560 (Koralovyj Marka) to 33.96 (Semen). The FRAP method (ferric reducing antioxidant capacity) ranged from 8.45 (Koralovyj) to 22.49 (Kostia). These results indicate the presence of antioxidant activity in different varieties of C. officinalis (111).
6.2 Reproductive effects
Cornus officinalis is widely used in Chinese medicine as a tonic and has been recognized for its beneficial pharmacological effects on the reproductive system. It contains bioactive substances that can improve erectile dysfunction, enhance sperm quality, and treat urinary disorders. These effects were found to be safe (112). In a study, a new formulation was developed by combining C. officinalis extract with Psoralea corylifolia and evaluated for its effectiveness in treating benign prostatic hyperplasia (BPH). The results showed that the combination formulation exhibited superior efficacy compared to finasteride, suggesting its potential as a novel treatment option for BPH (113). Another study investigated the effects of intravenously administered C. officinalis extract on diabetic rats (DM rats) at high and low concentrations (30 mg/kg and 15 mg/kg, respectively). After a 12-week treatment, the rats showed improvements in various parameters, including body weight ratio, sperm rate, testosterone levels, luteinizing hormone (LH) and gonadotropin-releasing hormone (GnRH) levels, as well as reduced serum creatinine, urea nitrogen, and urinary protein excretion. Moreover, testicular morphology was also improved. These findings indicate that C. officinalis extract effectively ameliorated testicular damage induced by diabetes mellitus (114).
6.3 Anti-diabetic effects
The development of diabetes-related pathologies involves various factors, including the formation of advanced glycosylation end products (AGEs), increased flux in the polyol pathway, activation of protein kinase C isoforms, and hexosamine pathway flux. In a study, galloyl glucosides and lignans isolated from C. officinalis seeds demonstrated potent inhibitory activity against AGE formation, suggesting their potential for treating diabetes associated with AGEs (115). In a study involving mice with noninsulin-dependent diabetes mellitus (NIDDM), an ethanol extract of C. officinalis was orally administered daily, while a control group was established (116). The mRNA and protein expression in skeletal muscle was evaluated using Northern blot and Western blot methods after 1 month of treatment. Compared to the control group, the ethanol extract of C. officinalis significantly increased mRNA expression (p < 0.01), indicating its ability to promote islet proliferation and postprandial insulin secretion and enhance mRNA and protein expression in NIDDM mice (117). These results suggest that the extract may improve the high-glucose and high-fat conditions associated with type 2 diabetes, offering potential implications for its treatment (116). The secoiridoids present in C. officinalis fruit extract were investigated and found to hold promise as a novel approach for preventing or treating diabetes (118). Furthermore, the effect of oleanolic acid, found in C. officinalis, on insulin secretion in rats was studied by administering specific levels of oleanolic acid via intraperitoneal injection. The results demonstrated that oleanolic acid effectively lowered plasma glucose levels by promoting increased plasma insulin levels (119). In a comparative study on diabetic rats, the antidiabetic effect of C. officinalis extract was evaluated and compared with that of the drug glibenclamide. The results revealed that C. officinalis extract significantly reduced blood glucose levels and increased insulin levels, indicating its potential for facilitating diabetes treatment (120).
6.4 Anti-inflammatory activity
A study investigating the anti-inflammatory mechanisms of C. officinalis extracts in lipopolysaccharide (LPS)-stimulated macrophages and colitis mice demonstrated their ability to modulate the NF-κB and MAPK signaling pathways. By inhibiting the binding of LPS to TLR4 on immune cells, C. officinalis extracts exhibited anti-inflammatory activity. In this study, RAW 264.7 macrophages were treated with an ethanol-extracted extract of C. officinalis var. koreana Kitam (COE) at various concentrations (0, 50, 100, 200, and 400 μg/mL). Pretreatment with 100, 200, and 400 μg/mL COE significantly reduced lipopolysaccharide (LPS)-stimulated protein kinase B (Akt) phosphorylation (p ≤ 0.003), indicating that the inhibition of protein kinase B (Akt), potentially due to COE treatment, attenuated the inflammatory response induced by LPS in RAW 264.7 macrophages (121). Furthermore, four novel phenolic compounds derived from C. officinalis fruits displayed inhibitory effects on NO secretion in RAW 264.7 cells and demonstrated some anti-inflammatory activity against RAW 264.7 cells (122). Given the increasing prevalence of obesity worldwide, which often accompanies various inflammatory diseases, a study explored the combination of C. officinalis with other herbs to obtain potential anti-inflammatory drugs for obesity-related inflammation (123). Conducting a surgical examination on the knees of male rats with medial meniscus instability (DMM), loganin was subsequently injected into the osteoarthritic gap at concentrations of 30 or 100 μg/mL over a period of 2–3 months. The findings indicated a significant delay in the progression of the disease (124).
6.5 Potential protective effects of Cornus officinalis on kidney and liver health
The excessive accumulation of extracellular matrix (ECM) in renal cells is a characteristic of chronic kidney disease, with components such as collagen (Col IV), fibronectin (FN), and pro-inflammatory factors (IL-6) being detrimental to renal cells. However, C. officinalis has been found to inhibit the secretion of FN and IL-6 induced by high glucose in renal cells, potentially impacting the development of chronic kidney disease. Through pharmacological and chemical research methods, a bioactive compound called 5-methylfurfural (5-HMF) (125, 126) has been extracted from C. officinalis. Studies in mice have shown that 5-methylfurfural (5-HMF) can improve acute liver injury and protect human venous epidermal cells from the harmful effects of H2O2 and glucose, indicating its beneficial effects on liver and kidney protection (127). Additionally, a combination of iridoid glycosides (IGCO) and triterpene acids (TACO) from C. officinalis, along with iridoid glycosides (IGRR) from groundnut, may exert a protective effect on the kidneys through synergistic action (128). An iridoid compound present in C. officinalis has the potential to improve alcohol-induced intestinal microbial disorders, protect the gastrointestinal tract, and mitigate the hepatic damage caused by alcohol (129). Moreover, in a mouse model, the ethanol extract (ECO) derived from C. officinalis fruits exhibited hepatoprotective effects by preventing or mitigating oxidative stress, thereby reducing liver damage induced by acetaminophen (APAP) (130).
Furthermore, the effect of C. officinalis extract on human hepatocyte lines (L02) exposed to D-galactosamine (GalN) and tumor necrosis factor-α (TNF-α) injury was investigated. The findings indicate that CIG significantly enhances the viability of L02 cells subjected to GalN/TNF-α injury, highlighting its potential role in the treatment of liver diseases (131).
6.6 Antibacterial effect
Cornus officinalis extract has demonstrated a significant inhibitory effect on hepatitis C virus protease activity through bioguided distillation (29). In addition, the ethanolic extract of C. officinalis has shown varying degrees of inhibition against bacterial strains such as E. coli, Listeria monocytogenes, E. coli O157:H7, Staphylococcus aureus, Pseudomonas aeruginosa, and Salmonella typhi, as observed in a disk diffusion assay, where it produced inhibition zones ranging from 8.5 to 18.3 mm at 4000 μg/disc (132). Moreover, the use of 1,3-butanediol (1,3-BG) and ethanol (EtOH) as extraction solvents for the seeds of C. officinalis resulted in inhibitory activity against Staphylococcus aureus, Propionibacterium acnes, and Staphylococcus epidermidis. Notably, when comparing silver nanoparticles (AgNPs) synthesized using the aqueous extract of C. officinalis with AgNPs synthesized using chemical methods, the former exhibited significantly enhanced inhibition of E. coli and S. aureus, with 20–40 times greater inhibitory ability (133).
6.7 Anticancer activity
Cancer, a malignant tumor resulting from abnormal cell proliferation, is primarily caused by genetic abnormalities that lead to uncontrolled and accelerated cell growth. Although modern medicine has made significant advancements in cancer treatment, certain types of cancer, such as triple-negative breast cancer (TNBC), pose challenges due to limited effective treatments and the development of systemic toxicity and acquired tumor resistance during therapy. However, studies investigating the nutritional properties of medicinal herbs have revealed that extracts from C. officinalis exhibit inhibitory effects on TNBC cell cultures, leading to cell growth arrest. Another highly fatal cancer, hepatocellular carcinoma (HCC), predominantly affects the liver (134). In experiments using an aqueous extract of C. officinalis at a concentration of 100 μg/mL, all three human hepatocellular carcinoma cell lines (HepG2, SK-Hep1, and PLC/PRF/5) showed inhibition according to the cell activity assay (XTT). Furthermore, researchers isolated an active compound called SZYY from the acetone extract of C. officinalis leaves and studied its impact on the proliferation and migration of human malignant melanoma cells (A375) and STAT3 signaling. The findings demonstrated that SZYY can inhibit STAT3 signaling, thereby suppressing tumor angiogenesis and exerting anti-A375 activity (135).
6.8 Other effects
To investigate the antimenopausal effects of a combined extract of C. officinalis and Cockscomb (RF), the extract was administered to ovariectomized (OVX) mice. The study revealed several positive outcomes, including reduced deposition of fatty cells in the liver and abdominal visceral adipose tissue. Furthermore, there was a significant improvement in bone mineral density and content in the mice, indicating the potential of the CO + RF extract to exert anti-obesity and anti-osteoporosis effects (136). Through cytotoxicity assays, reverse transcriptase-polymerase chain reaction (RT–PCR), and Western blot analysis, it was demonstrated that C. officinalis effectively inhibits receptor activator-mediated osteoclast differentiation, thereby reducing the risk of fractures associated with osteoporosis (137). Additionally, C. officinalis fruit extracts were found to maintain stable calcium homeostasis by preventing excessive calcium accumulation induced by PM2.5 and protecting cells from PM2.5-induced DNA damage. These extracts also exhibited protective effects against oxidative stress in human HaCaT keratinocytes, preventing lipid peroxidation and protein carbonylation caused by PM2.5. Moreover, C. officinalis methanol extract (COME) was shown to impact melanin content in Melana cells, with a significant 36.1% increase observed after treatment with 12.5 μg/mL COME. This suggests the potential of COME in treating gray hair and promoting hair growth (138, 139). In a study on the oxidative mutagenic effect of C. officinalis on human neutrophils (PMNs), it was found that the extract inhibits the secretion of important chelators, such as IL-8, by human neutrophils and Caco-2 intestinal epithelial cells. This balancing effect on the immune system and epithelial cells is significant (140). Furthermore, the effects of C. officinalis extract on rats with cerebral infarction were investigated, revealing a reduction in the infarct area, NO content, NOS activity, and the number of NF-kappaB-positive cells in the cerebral cortex compared to the control group. These findings highlight the potential benefits of C. officinalis extract in the treatment of cerebral infarction (141).
7 Existing products in the market
Cornus officinalis possesses significant nutritional value, as indicated in Table 3. It primarily serves the pharmaceutical industry, where it is processed to form an essential component of drugs used to treat various ailments. As the national economy develops and people’s living standards improve, there is an increasing demand for functional health foods. C. officinalis dried fruit pulp is commonly utilized in the production of tea and wine (142, 143). Additionally, C. officinalis powder can be used for foot soaks or incorporated into foot patches, offering benefits for individuals with high blood pressure and chronic pharyngitis (144). The applications of C. officinalis extend beyond these examples and encompass the production of health drinks, jams, preserved fruits, canned fruit pulp, and more (145, 146). Moreover, studies have explored the preparation of C. officinalis, wolfberry, and danfeng peony as raw materials, with high-quality cooking wine as the base material, to create a flavonoid-rich and antioxidant-packed wine product through appropriate brewing techniques (147).
Cornus officinalis harbors a diverse range of endophytic bacteria (148). Previous research has focused on examining the beneficial properties of C. officinalis fruit fermentation, including acid and bile salt tolerance, antibacterial activity, self-aggregation, and cholesterol-lowering effects. From these fermented C. officinalis fruits, probiotic lactic acid bacteria (LAB) with desirable characteristics, such as acid and bile salt tolerance, antimicrobial activity, self-aggregation, and cholesterol-lowering capacity, have been identified. One such example is Lactobacillus plantarum. Lactobacillus plantarum is known for its positive impact on health, including immunomodulatory functions, maintenance of intestinal flora balance, promotion of nutrient absorption, alleviation of lactose intolerance, and inhibition of tumor cell formation. The LAB discovered from C. officinalis fermentation hold potential as probiotic candidates (148).
8 Conclusion and future perspectives
For centuries, the fruits of Cornus officinalis have been esteemed for their rich nutritional profile. This recognition is primarily attributed to the presence of diverse active components, including polyphenols, flavonoids, irises, anthocyanins, organic acids, and various bioactive compounds. Consequently, C. officinalis holds significant potential in promoting human health and combating various diseases, such as diabetes, kidney and liver disorders, and cancer. However, in-depth studies on the pharmacodynamics and pharmacotoxicology of C. officinalis fruits and their derived bioactive compounds are imperative to ascertain their efficacy and safety for human consumption.
The aforementioned insights collectively underscore extensive research on C. officinalis, revealing its advantageous effects on the reproductive system, anticancer properties, antidiabetic effects, and hypolipidemic effects. Additionally, noteworthy exploration has been undertaken to create novel food products with exceptional flavors that contribute to overall health. In Chinese culture, it has been a longstanding tradition to incorporate C. officinalis into porridge, wine-making, and soup preparation alongside other nutritional supplements. In recent years, the food industry has embraced the utilization of C. officinalis to develop healthy options, jams, wines, and jellies. Researchers have even introduced a C. officinalis complex crystal solid drink, with C. officinalis as the primary ingredient, sucrose as a sweetener, and other fruit juice concentrates as flavor enhancers. Looking ahead, there is a promising outlook for the development of additional compounds with remarkable nutritional value.
Data availability statement
The original contributions presented in the study are included in the article/supplementary material, further inquiries can be directed to the corresponding authors.
Author contributions
WD: Supervision, Writing – review & editing. YL: Investigation, Writing – original draft. YG: Supervision, Writing – review & editing. JC: Writing – review & editing. HA: Supervision, Writing – review & editing. MK: Supervision, Writing – review & editing. CP: Supervision, Writing – review & editing. JP: Resources, Supervision, Writing – review & editing. AE-A: Supervision, Writing – review & editing.
Funding
The author(s) declare financial support was received for the research, authorship, and/or publication of this article. This study was funded by the Key Basic Research Project of Shaanxi Provincial Department of Science and Technology (2023-JC-ZD-13), the Special Support Plan for High-Level Talents in Shaanxi Province (for JP), the Foreign Expert Project of the Ministry of Science and Technology, and the Project for Scientific and Technological Innovation Team in Shangluo City of Shaanxi Province (SK2019-72).
Conflict of interest
The authors declare that the research was conducted in the absence of any commercial or financial relationships that could be construed as a potential conflict of interest.
The author(s) declared that they were an editorial board member of Frontiers, at the time of submission. This had no impact on the peer review process and the final decision.
Publisher’s note
All claims expressed in this article are solely those of the authors and do not necessarily represent those of their affiliated organizations, or those of the publisher, the editors and the reviewers. Any product that may be evaluated in this article, or claim that may be made by its manufacturer, is not guaranteed or endorsed by the publisher.
Footnotes
References
1. Brindza, P, Brindza, J, Toth, D, Klimenko, S, and Grigorieva, O. Biological and commercial characteristics of cornelian cherry (Cornus mas L.) population in the Gemer region of Slovakia. Acta Hortic. (2009) 818:85–94. doi: 10.17660/ActaHortic.2009.818.11
2. Klymenko, S, Grygorieva, O, and Brindza, J. Less known species of fruIt crops. Nitra, Slovakia: Slovak University of Agriculture in Nitra (2017).
3. Park, CH, Sathasivam, R, Kim, TJ, Park, BB, Kim, JK, and Park, SU. Metabolic profiling and secondary metabolite accumulation during fruit development of Cornus officinalis Sieb. et Zucc. Ind Crop Prod. (2022) 189:115779. doi: 10.1016/j.indcrop.2022.115779
4. Ye, XS, Hao, J, Zhang, JL, Pang, XB, Zhang, L, Qiao, HY, et al. Study on chemical constituents of Cornus officinalis fruit. Chin J Chin Mat Med. (2016) 41:4605–9.
5. Li, Q, Hu, S, Huang, L, Zhang, J, and Cao, G. Evaluating the therapeutic mechanisms of selected active compounds in Cornus officinalis and Paeonia lactiflora in rheumatoid arthritis via network pharmacology analysis. Front Pharmacol. (2021) 12:648037. doi: 10.3389/fphar.2021.648037
6. Hwangbo, H, Jeung, JS, Kim, MY, Ji, SY, Yoon, S, Kim, TH, et al. A study on antioxidant and anti-inflammatory effects based on analysis of functional components of Cornus officinalis Siebold & Zucc. J Life Sci. (2021) 31:287–97. doi: 10.5352/JLS.2021.31.3.287
7. Zhang, X, Wang, J, Hu, J, Wang, Y, Lai, J, Zhou, LY, et al. Visual analysis of surimi research based on CiteSpace and bibliometric analysis platform. Food Sci. (2022):1–15.
8. Hua, T, and Jie, C. Research progress of global aged food based on bibliometric analysis of web of science. J Agric Engin. (2021) 37:324–32.
9. Hang, S, Yinghua, S, Moxiao, L, et al. Scientometrics analysis on the cross research of food safety and data science in China. Food Sci. (2020) 41:291–301.
10. Guangyang, L. The propagation track of CiteSpace's domestic applications -- statistical and visual analysis based on cross database data from 2006 to 2015. Library Inform Knowledge. (2017) 2:60–74. doi: 10.13366/j.dik.2017.02.060
11. Guo, S, Wang, L, Xie, Y, Luo, X, Zhang, S, Xiong, L, et al. Bibliometric and visualized analysis of stem cells therapy for spinal cord injury based on web of science and CiteSpace in the last 20 years. World Neurosurg. (2019) 132:e246–58. doi: 10.1016/j.wneu.2019.08.191
12. Chen, C. Science mapping: a systematic review of the literature. J Data Inform Sci. (2017) 2:1–40. doi: 10.1515/jdis-2017-0006
13. Saiqi, T, and Sheng, D. Knowledge map and progress analysis of tanning research based on wos and CiteSpace. Chinese Leather. (2022):1–8. doi: 10.13536/j.cnki.issn1001-6813.2022-006-006
14. Lian, W, and Xinjun, C. Based on bibliometric analysis of literature, the research status and development of scale headed canine tooth Antarctic fish. Marine Limnol Bullet. (2022) 44:82–90. doi: 10.13984/j.cnki.cn37-1141.2022.01.011
15. Wang, Lili, Zhang, Jiawei, and Yang, Jiaxi. Bibliometric analysis of Chinese aesthetics (1983-2020) based on CiteSpace. In Proceeding of the 5th International Conference on art research: a collection of papers on research, experience and education, (2021)
16. Chunjiao, Z, Mingxiao, J, Lu, M, et al. CiteSpace based visual analysis of the research status and trend of metabonomics in the field of traditional Chinese medicine. World Sci Technol. (2022):1–9.
17. Weichuan, K, Kun, L, Ye, J, et al. Current status, hot spots and trends of acupuncture in the treatment of spinal cord injury: CiteSpace based bibliometrics and visual analysis of knowledge map. Massage Rehabil Med. (2022) 13:68–73. doi: 10.19787/j.issn.1008-1879.2022.13.019
18. Sun, W, Huang, P, Song, H, and Feng, D. Bibliometric analysis of acute pancreatitis in web of science database based on CiteSpace software. Medicine. (2020) 99:e23208. doi: 10.1097/MD.0000000000023208
19. Liang, YD, Li, Y, Zhao, J, Wang, X-Y, Zhu, H-Z, and Chen, X-H. Study of acupuncture for low back pain in recent 20 years: a bibliometric analysis via CiteSpace. J Pain Res. (2017) 10:951–64. doi: 10.2147/JPR.S132808
20. Bai, CK, Yu, JR, Yu, F, and Zheng, P. Genetic diversity and construction of primary core germplasm in C. officinalis by ISSR marker. Acta Botanica Boreali-Occidentalia Sinica. (2009) 12:2401–7.
22. Guan, M, Bai, CK, Liu, J, and Wang, ZZ. Genetic diversity of C. officinalis accessions based on morphological and ISSR analysis. Mol Plant Breed. (2008) 5:912–20.
24. Baik, MC, Hoang, HD, and Hanmer, K. A checklist of the Korean cultivated plants. Kulturpflanze. (1986) 34:69–144. doi: 10.1007/BF02630485
25. P Hanelt, Institute of Plant Genetics and Crop Plant Research (IPK) eds. Mansfeld’s encyclopedia of agricultural and horticultural crops, vol. 3. Berlin: Springer (2001).
26. National Pharmacopoeia Committee. Pharmacopoeia of People’s republic of China. Beijing: Chemical Industry Press (2005). 20 p.
27. He, YQ, Yao, BH, Ma, ZY, and Wang, LJ. Chemical constituents from Cornus officinalis and their biological activity [sup] 1. Chronicles Young Scientists. (2012) 3:209–9. doi: 10.4103/2229-5186.99579
28. He, J, Ye, XS, Wang, XX, Yang, YN, Zhang, PC, Ma, BZ, et al. Four new iridoid glucosides containing the furan ring from the fruit of Cornus officinalis. Fitoterapia. (2017) 120:136–41. doi: 10.1016/j.fitote.2017.06.003
29. Yue, W, Zhengquan, L, Lirong, C, and Xiaojie, X. Antiviral compounds and one new iridoid glycoside from Cornus officinalis. Prog Nat Sci. (2006) 16:142–6. doi: 10.1080/10020070612331343205
30. Peng, Z, Wang, Y, He, J, Zhang, J, Pan, X, Ye, X, et al. Chemical constituents and their antioxidant and anti-inflammatory activities from edible Cornus officinalis fruits. Eur Food Res Technol. (2022) 248:1003–10. doi: 10.1007/s00217-021-03940-6
31. Liang, J, He, J, Zhu, S, Zhao, W, Zhang, Y, Ito, Y, et al. Preparation of main iridoid glycosides in Fructus corni by macroporous resin column chromatography and countercurrent chromatography. J Liq Chromatogr Relat Technol. (2013) 36:983–99. doi: 10.1080/10826076.2012.683914
32. Zhang, LL, Wang, YM, Xu, M, Wu, DM, and Chen, JH. Quantification of gallic acid and ellagic acid from the seed of Cornus officinalis by UHPLC method and their antioxidant activity. Chem Eng Commun. (2014) 201:545–56. doi: 10.1080/00986445.2013.780165
33. Yamabe, N, Kang, KS, Matsuo, Y, Tanaka, T, and Yokozawa, T. Identification of antidiabetic effect of iridoid glycosides and low molecular weight polyphenol fractions of Corni Fructus, a constituent of Hachimi-jio-Gan, in streptozotocin-induced diabetic rats. Biol Pharm Bull. (2007) 30:1289–96. doi: 10.1248/bpb.30.1289
34. Li, Y, Yang, J, Li, J, Liang, XH, and Sun, JL. Two new secoiridoid glucosides from the twigs of Cornus officinalis. Chem Nat Compd. (2016) 52:647–50. doi: 10.1007/s10600-016-1730-4
35. Seeram, NP, Schutzki, R, Chandra, A, and Nair, MG. Characterization, quantification, and bioactivities of anthocyanins in Cornus species. J Agric Food Chem. (2002) 50:2519–23. doi: 10.1021/jf0115903
36. Peng, W. Molecules and functions of Cornus officinalis bark volatiles. Emirates J Food Agric. (2018) 30:828–38. doi: 10.9755/ejfa.2018.v30.i10.1826
37. HATANO, T, OGAWA, N, KIRA, R, YASUHARA, T, and OKUDA, T. Tannins of cornaceous plants. I. Cornusiins a, B and C, dimeric monomeric and trimeric hydrolyzable tannins from Cornus officinalis, and orientation of valoneoyl group in related tannins. Chem Pharm Bull. (1989) 37:2083–90. doi: 10.1248/cpb.37.2083
38. Forman, V, Grančai, D, and Horváth, B. Constituents of the leaves of Sieb et Zucc. Ceska Slovenska Farmacie. (2016) 65:128–31.
39. Jinguang, H, Yanlin, L, and Jianqiang, S. Analysis of aroma components in fruit of Cornus officinalis Sieb. Et Zucc by GC–MS. J Northwest Agric Forestry Univ. (2009)
40. Cao, G, Zhang, C, Zhang, Y, Cong, X, Cai, H, and Cai, B. Screening and identification of potential active components in crude Fructus Croni using solid-phase extraction and LC-LTQ-linear ion trap mass spectrometry. Pharm Biol. (2012) 50:278–83. doi: 10.3109/13880209.2011.599036
41. Kucharska, AZ, Szumny, A, Sokół-Łetowska, A, Piórecki, N, and Klymenko, SV. Iridoids and anthocyanins in cornelian cherry (Cornus mas L.) cultivars. J Food Comp Anal. (2015) 40:95–102. doi: 10.1016/j.jfca.2014.12.016
42. Dong, Y, Feng, ZL, Chen, HB, Wang, FS, and Lu, JH. Corni fructus: a review of chemical constituents and pharmacological activities. Chin Med. (2018) 13:34. doi: 10.1186/s13020-018-0191-z
43. Ma, W, Wang, KJ, Cheng, CS, Yan, GQ, Lu, WL, Ge, JF, et al. Bioactive compounds from Cornus officinalis fruits and their effects on diabetic nephropathy. J Ethnopharmacol. (2014) 153:840–5. doi: 10.1016/j.jep.2014.03.051
44. Yang, JC. A study on the cosmetic preservative effects of Cornus officinalis seed extracts. J Korean Appl Sci Technol. (2016) 33:333–41. doi: 10.12925/jkocs.2016.33.2.333
45. Ding, X, Zhu, FS, Yu, ZL, Dong, LN, and Cai, BC. Comparative study on contents of amino acid and major and trace elements in Cornus officinalis before and after being processed. J Chinese Med Materials. (2007) 30:396–9.
46. Wang, LL, Zhang, T, Chen, SQ, and Shang, CL. Study on the correlation between the quality of Cornus officinalis and the contents of the inorganic elements in the planting soil. J Chin Med Materials. (2011) 34:1167–72.
47. Hou, D, Wang, W, Yang, M, Li, Z, Xu, J, Wang, L, et al. Molecular cloning and expression analysis of the transcription factor CobHLH40 from Cornus officinalis on the basis of de novo transcriptome sequencing. Plant Biotechnol Reports. (2020) 14:419–28. doi: 10.1007/s11816-020-00623-8
48. Miyazawa, M, and Kameoka, H. Volatile flavor components of CORNI FRUCTUS (Cornus officinalis Sieb. Et Zucc.). Agric Biol Chem. (1989) 53:3337–40.
49. Huang, F, Guo, H, Wei, Y, Zhao, X, Chen, Y, Lin, Z, et al. In silico network analysis of ingredients of Cornus officinalis in osteoporosis. Int Med J Experiment Clin Res. (2021) 27:e929219–1. doi: 10.12659/MSM.929219
50. Wu, MJ, Zhao, TZ, Zhang, HY, Yin, WP, and Fu, JG. Studies on chemical constituents of Syringa pubescens (I). Chin Tradit Herb Drug. (2003) 34:7–8.
51. Tanaka, N, Tanaka, T, Fujioka, T, Fujii, H, Mihashi, K, Shimomura, K, et al. An ellagic compound and iridoids from Cornus capitata root cultures. Phytochemistry. (2001) 57:1287–91. doi: 10.1016/S0031-9422(01)00179-0
52. Yue, X, Bi, H, Zheng, D, Zhao, Y, and Peng, W. Molecules of Cornus officinalis bark extractives. Ekoloji. (2019) 28:75–9.
53. He, J, Xu, J, Pan, X, Ye, XS, Gao, PY, Yan, Y, et al. Unusual cadinane-type sesquiterpene glycosides with α-glucosidase inhibitory activities from the fruit of Cornus officinalis Sieb. Et Zuuc. Bioorg Chem. (2019) 82:1–5. doi: 10.1016/j.bioorg.2018.09.026
54. Wang, KJ, Yang, CR, and Zhang, YJ. Phenolic antioxidants from Chinese toon (fresh young leaves and shoots of Toona sinensis). Food Chem. (2007) 101:365–71. doi: 10.1016/j.foodchem.2006.01.044
55. Wang, SJ, Wang, YH, Li, CH, et al. Analyses on oil content and fatty acid composition in seeds of Cornus officinalis from different locations. J Plant Resour Environ. (2016) 25:112–4.
56. Duan, D, Li, Z, Luo, H, Zhang, W, Chen, L, and Xu, X. Antiviral compounds from traditional Chinese medicines Galla Chinese as inhibitors of HCV NS3 protease. Bioorg Med Chem Lett. (2004) 14:6041–4. doi: 10.1016/j.bmcl.2004.09.067
57. Tanaka, T, Nonaka, GI, and Nishioka, I. Punicafolin, an ellagitannin from the leaves of Punica granatum. Phytochemistry. (1985) 24:2075–8. doi: 10.1016/S0031-9422(00)83125-8
58. Lee, HJ, Seong, YH, Bae, KH, Kwon, SH, Kwak, HM, Nho, SK, et al. β-Secretase (BACE1) inhibitors from Sanguisorbae Radix. Arch Pharm Res. (2005) 28:799–803. doi: 10.1007/BF02977345
59. Lu, Y, and Foo, LY. The polyphenol constituents of grape pomace. Food Chem. (1999) 65:1–8. doi: 10.1016/S0308-8146(98)00245-3
60. Gao, D, Li, J, and Liu, Z. Study of the extraction, purification and antidiabetic potential of ursolic acid from Cornus officinalis Sieb. Et Zucc. Clin Pract. (2008) 5:697.
61. Cao, G, Zhang, Y, Feng, J, Cai, H, Zhang, C, Ding, M, et al. A rapid and sensitive assay for determining the main components in processed Fructus corni by UPLC–Q-TOF-MS. Chromatographia. (2011) 73:135–41. doi: 10.1007/s10337-010-1825-1
62. He, J, Xu, Y, Xu, JK, Ye, XS, Zhang, XX, and Zhang, WK. Cornusglucosides a and B, two new Iridoid glucosides from the fruit of Cornus officinalis. Chem Biodivers. (2019) 16:e1900421. doi: 10.1002/cbdv.201900421
63. Zhang, Y, Chen, Y, and Zhao, S. A sedoheptulose gallate from the fruits of Cornus officinalis. Acta Pharm Sin. (1999) 34:153–5.
64. Zhao, SP, and Xue, Z. Studies on the chemical constituents of Cornus officinalis Sieb et Zucc. Yao Xue Xue Bao. (1992) 27:845–8.
65. Park, JY, Han, AR, Kil, YS, Kang, U, Kim, SH, Nam, SJ, et al. A new secoiridoid glycoside from the fruits of Cornus officinalis (Cornaceae). Nat Prod Res. (2016) 30:1504–10. doi: 10.1080/14786419.2015.1115996
66. Jiménez, O, de la Rosa, G, and Lavilla, R. Straightforward access to a structurally diverse set of oxacyclic scaffolds through a four-component reaction. Angew Chem Int Ed. (2005) 44:6521–5. doi: 10.1002/anie.200501548
67. Maicas, S, and Mateo, JJ. Hydrolysis of terpenyl glycosides in grape juice and other fruit juices: a review. Appl Microbiol Biotechnol. (2005) 67:322–35. doi: 10.1007/s00253-004-1806-0
68. Wang, X, Zhong, XJ, Zhou, N, Ji, LL, Li, JJ, Cai, N, et al. Secoiridoid glycosides from the fruits of Cornus officinalis. Nat Prod Res. (2022) 36:2329–35. doi: 10.1080/14786419.2020.1834547
69. Du, W, Cai, H, Wang, M, Ding, X, Yang, H, Cai, B, et al. Simultaneous determination of six active components in crude and processed Fructus corni by high-performance liquid chromatography. J Pharm Biomed Anal. (2008) 48:194–7. doi: 10.1016/j.jpba.2008.04.021
70. Liu, Z, Zhu, Z, Zhang, H, Tan, G, Chen, X, and Chai, Y. Qualitative and quantitative analysis of Fructus corni using ultrasound assisted microwave extraction and high-performance liquid chromatography coupled with diode array UV detection and time-of-flight mass spectrometry. J Pharm Biomed Anal. (2011) 55:557–62. doi: 10.1016/j.jpba.2011.02.007
71. Huang, J, Zhang, Y, Dong, L, Gao, Q, Yin, L, Quan, H, et al. Ethnopharmacology, phytochemistry, and pharmacology of Cornus officinalis Sieb. Et Zucc. J Ethnopharmacol. (2018) 213:280–301. doi: 10.1016/j.jep.2017.11.010
72. He, K, Song, S, Zou, Z, Feng, M, Wang, D, Wang, Y, et al. The hypoglycemic and synergistic effect of loganin, morroniside, and ursolic acid isolated from the fruits of Cornus officinalis. Phytother Res. (2016) 30:283–91. doi: 10.1002/ptr.5529
73. Ye, XS, He, J, Cheng, YC, Zhang, L, Qiao, HY, Pan, XG, et al. Cornusides A–O, bioactive Iridoid glucoside dimers from the fruit Of Cornus officinalis. J Nat Prod. (2017) 80:3103–11. doi: 10.1021/acs.jnatprod.6b01127
74. Chen, Y, Wu, Y, Gan, X, Liu, K, Lv, X, Shen, H, et al. Iridoid glycoside from Cornus officinalis ameliorated diabetes mellitus-induced testicular damage in male rats: involvement of suppression of the AGEs/RAGE/p38 MAPK signaling pathway. J Ethnopharmacol. (2016) 194:850–60. doi: 10.1016/j.jep.2016.10.079
75. Dong, TJ, Cui, YL, Tian, JS, et al. Advances in studies on natural iridoids. Chin Tradit Herb Drug. (2011) 42:185–94.
76. Castañeda-Ovando, A, Pacheco-Hernández, ML, Páez-Hernández, ME, Rodríguez, JA, and Galán-Vidal, CA. Chemical studies of anthocyanins: a review. Food Chem. (2009) 113:859–71. doi: 10.1016/j.foodchem.2008.09.001
77. Jeong, EJ, Kim, TB, Yang, H, Kang, SY, Kim, SY, Sung, SH, et al. Neuroprotective iridoid glycosides from Cornus officinalis fruits against glutamate-induced toxicity in HT22 hippocampal cells. Phytomedicine. (2012) 19:317–21. doi: 10.1016/j.phymed.2011.08.068
78. Wang, SF, Chen, XG, Hu, ZD, and Ju, Y. Analysis of three effective components in Fructus corni and its preparations by micellar electrokinetic capillary chromatography. Biomed Chrom. (2003) 17:306–11. doi: 10.1002/bmc.247
79. Ma, D, Luo, Y, Huang, R, Zhao, ZR, Zhang, L, Li, YL, et al. Cornel iridoid glycoside suppresses hyperactivity phenotype in rTg4510 mice through reducing tau pathology and improving synaptic dysfunction. Curr Med Sci. (2020) 40:1031–9. doi: 10.1007/s11596-020-2284-z
80. Park, CH, Noh, JS, Kim, JH, Tanaka, T, Zhao, Q, Matsumoto, K, et al. Evaluation of morroniside, iridoid glycoside from Corni Fructus, on diabetes-induced alterations such as oxidative stress, inflammation, and apoptosis in the liver of type 2 diabetic db/db mice. Biol Pharm Bull. (2011) 34:1559–65. doi: 10.1248/bpb.34.1559
81. Park, CH, Noh, JS, Tanaka, T, and Yokozawa, T. Effects of morroniside isolated from Corni Fructus on renal lipids and inflammation in type 2 diabetic mice. J Pharm Pharmacol. (2010) 62:374–80. doi: 10.1211/jpp.62.03.0013
82. Pi, WX, Feng, XP, Ye, LH, and Cai, BC. Combination of morroniside and diosgenin prevents high glucose-induced cardiomyocytes apoptosis. Molecules. (2017) 22:163. doi: 10.3390/molecules22010163
83. Wang, W, Xu, J, Li, L, Wang, P, Ji, X, Ai, H, et al. Neuroprotective effect of morroniside on focal cerebral ischemia in rats. Brain Res Bull. (2010) 83:196–201. doi: 10.1016/j.brainresbull.2010.07.003
84. Ji, L, Wang, X, Li, J, Zhong, XJ, Zhang, B, Juan, J, et al. New iridoid derivatives from the fruits of Cornus officinalis and their neuroprotective activities. Molecules. (2019) 24:625. doi: 10.3390/molecules24030625
85. Bai, Y, Wang, S, Zhang, L, et al. Effects of cornel iridoid glycoside on platelet aggregation and bleeding time in rabbits and rats. Chinese J Clin Pharmacol Therap. (2010) 15:1373.
86. Burak, M, and Imen, Y. Flavonoids and their antioxidant properties. Turkiye Klin Tip Bil Derg. (1999) 19:296–304.
87. Lee, Y, Yuk, D, Lee, J, Lee, SY, Ha, TY, Oh, KW, et al. Epigallocatechin-3-gallate prevents lipopolysaccharide-induced elevation of β-amyloid generation and memory deficiency. Brain Res. (2009) 1250:164–74. doi: 10.1016/j.brainres.2008.10.012
88. Metodiewa, D, Kochman, A, and Karolczak, S. Evidence for antiradical and antioxidant properties of four biologically active N, N, diethylaminoethyl ethers of flavanone oximes: a comparison with natural polyphenolic flavonoid (rutin) action. Biochem Mol Biol Int. (1997) 41:1067–75.
89. Walker, E, Pacold, M, Perisic, O, Stephens, L, Hawkins, PT, Wymann, MP, et al. Structural determinations of phosphoinositide 3-kinase inhibition by wortmannin, LY294002, quercetin, myricetin, and staurosporine. Mol Cell. (2000) 6:909–19. doi: 10.1016/S1097-2765(05)00089-4
90. Manach, C, Scalbert, A, Morand, C, Rémésy, C, and Jiménez, L. Polyphenols: food sources and bioavailability. Am J Clin Nutr. (2004) 79:727–47. doi: 10.1093/ajcn/79.5.727
91. Sozański, T, Kucharska, AZ, Rapak, A, Szumny, D, Trocha, M, Merwid-Ląd, A, et al. Iridoid–loganic acid versus anthocyanins from the Cornus mas fruits (cornelian cherry): common and different effects on diet-induced atherosclerosis, PPARs expression and inflammation. Atherosclerosis. (2016) 254:151–60. doi: 10.1016/j.atherosclerosis.2016.10.001
92. De Biaggi, M, Donno, D, Mellano, MG, Riondato, I, Rakotoniaina, EN, and Beccaro, GL. Cornus mas (L.) fruit as a potential source of natural health-promoting compounds: physico-chemical characterization of bioactive components. Plant Foods Hum Nutr. (2018) 73:89–94. doi: 10.1007/s11130-018-0663-4
93. Davies, R, Massey, RC, and Mcweeny, DJ. The catalysis of the N-nitrosation of secondary amines by nitrosophenols. Food Chem. (1980) 6:115–22. doi: 10.1016/0308-8146(80)90027-8
94. Lee, MW, Yin, J, and Park, KH. Antiproliferative effects of new dimeric ellagitannins from Cornus alba in prostate cancer cells. Planta Med. (2016) 81:S1–S381. doi: 10.1055/s-0036-1597063
95. Hayashi, T, Sawa, K, Kawasaki, M, Arisawa, M, Shimizu, M, and Morita, N. Inhibition of cow’s milk xanthine oxidase by flavonoids. J Nat Prod. (1988) 51:345–8. doi: 10.1021/np50056a030
96. Mavundza, EJ, Tshikalange, TE, Lall, N, Mudau, FN, and Hussein, AA. Antioxidant activity and cytotoxicity effect of flavonoids isolated from Athrixia phylicoides, vol. 76 (2010). 398 p.
97. Yang, L, Wang, Z, and Huang, L. Isolation and structural characterization of a polysaccharide FCAP1 from the fruit of Cornus officinalis. Carbohydr Res. (2010) 345:1909–13. doi: 10.1016/j.carres.2010.06.009
98. Tan, J, Cui, P, Ge, S, Cai, X, Li, Q, and Xue, H. Ultrasound assisted aqueous two-phase extraction of polysaccharides from Cornus officinalis fruit: modeling, optimization, purification, and characterization. Ultrason Sonochem. (2022) 84:105966. doi: 10.1016/j.ultsonch.2022.105966
99. You, Q, Yin, X, and Zhao, Y. Enzyme assisted extraction of polysaccharides from the fruit of Cornus officinalis. Carbohydrate Polymers. (2013) 98:607–10. doi: 10.1016/j.carbpol.2013.06.036
100. Yin, X, You, Q, Jiang, Z, and Zhou, X. Optimization for ultrasonic-microwave synergistic extraction of polysaccharides from Cornus officinalis and characterization of polysaccharides. Int J Biol Macromol. (2016) 83:226–32. doi: 10.1016/j.ijbiomac.2015.11.059
101. Chen, K, Li, J, Chen, K, Hou, X, Mai, H, and Xue, X. The mechanism of Cornus officinalis Total glycosides and Cornus polysaccharide on myocardial protection in rats with acute myocardial infarction. Chin Med. (2016) 7:45–54. doi: 10.4236/cm.2016.72007
102. Sun, J-R, Wang, D-H, Zhao, J-F, and Shao-Bin, G. Ultrasonic-assisted extraction, purification, antioxidant and antibacterial activity of polysaccharide from Cornus officinalis leaves. J Food Nutr Res. (2020) 8:496–505. doi: 10.12691/jfnr-8-9-6
103. Zhou, YC, Zhang, LJ, and Zhang, YL. New progress in chemical constituents and pharmacological action of Corni officinalis. Inform Tradit Chin Med. (2020) 37:114–20.
104. Pc, L, Lp, Z, Bf, G, et al. Nutritional constituents of Cornus officinalis. Food Ind. (2020) 41:319–23.
105. Luan, N, Wang, X, Zhong, X, Zhou, N, and Juan, J. Isolation, identification and neuroprotective effect of bioactive substances from Cornus officinalis. J Food Sci Technol. (2021) 39:126–36. doi: 10.12301/j.issn.2095-6002.2021.01.014
106. Yang, MM, Yuan, XX, Zhao, GQ, Yang, LM, and Zhang, WH. Research progress on chemical constituents and pharmacological effects of Cornus officinalis. J Chengde Med Univ. (2016) 33:398–400.
107. St, Z, and Wu, K. Evaluation of nutritional value of protein by amino acid ratio coefficient method. Acta Nutr Sin. (1988) 10:187–90.
108. Hwang, KA, Hwang, YJ, and Song, J. Antioxidant activities and oxidative stress inhibitory effects of ethanol extracts from Cornus officinalis on raw 264.7 cells. BMC Complement Altern Med. (2016) 16:1–9. doi: 10.1186/s12906-016-1172-3
109. Quah, Y, Lee, SJ, Lee, EB, Birhanu, BT, Ali, MS, Abbas, MA, et al. Cornus officinalis ethanolic extract with potential anti-allergic, anti-inflammatory, and antioxidant activities. Nutrients. (2020) 12:3317. doi: 10.3390/nu12113317
110. Kim, YS, Hwang, JW, Kang, SH, Kim, HR, Je, JY, Kim, EH, et al. Protective effects of Cornus walteri W. Extracts on t-BHP-induced cell damage through antioxidant activity. Biotechnol Bioprocess Eng. (2013) 18:819–26. doi: 10.1007/s12257-012-0623-z
111. Klymenko, S, Kucharska, AZ, Sokół-Łętowska, A, and Piórecki, N. Antioxidant activities and phenolic compounds in fruits of cultivars of cornelian cherry (Cornus mas L.). Agrobiodiversity. (2019):3.
112. Kam, SC, Choi, SM, Jeh, SU, Lee, SH, Hwa, JS, Jung, KH, et al. Efficacy and safety of a herbal formula that mainly consists of Cornus officinalis for erectile dysfunction: a double-blind, placebo-controlled study. Korean J Urol. (2007) 48:741–7. doi: 10.4111/kju.2007.48.7.741
113. Zhang, L, Ren, L, and Wen, J. Studies on antiarrhythmic portion in Cornus officinalis extract. Chin Tradit Herb Drug. (1994)
114. Damtoft, S, Franzyk, H, and Jensen, SR. Excelsioside, a secoiridoid glucoside from Fraxinus excelsior. Phytochemistry. (1992) 31:4197–201. doi: 10.1016/0031-9422(92)80442-H
115. Kim, J S. Seeds of Cornus officinalis and diabetic cataracts[M]//handbook of nutrition, diet and the eye. San Diego: Academic Press, (2014) 451–458.
116. Qian, DS, Zhu, YF, and Zhu, Q. Effect of alcohol extract of Cornus officinalis Sieb. Et Zucc on GLUT4 expression in skeletal muscle in type 2 (noninsulin-dependent) diabetic mellitus rats. China J Chin Materia Medica. (2001) 26:859–62.
117. Kutllovci, AH, Rizaj, D, and Bytyçi, F. The role of anthocyanin and Flavonoides in patient with diabetes mellitus-type II. Review Global Med Healthcare Res. 305.
118. Peng, ZC, He, J, Pan, XG, Zhang, J, Wang, YM, Ye, XS, et al. Secoiridoid dimers and their biogenetic precursors from the fruits of Cornus officinalis with potential therapeutic effects on type 2 diabetes. Bioorg Chem. (2021) 117:105399. doi: 10.1016/j.bioorg.2021.105399
119. Hsu, JH, Wu, YC, Liu, IM, and Cheng, JT. Release of acetylcholine to raise insulin secretion in Wistar rats by oleanolic acid, one of the active principles contained in Cornus officinalis. Neurosci Lett. (2006) 404:112–6. doi: 10.1016/j.neulet.2006.05.025
120. Shamsi, F, Asgari, S, Rafieian, M, Kazemi, S, and Adelnia, A. Effects of Cornus mas L. on blood glucose, insulin and histopathology of pancreas in alloxan-induced diabetic rats. J Isfahan Med School. (2011) 29:929–38.
121. Najjar, RS, Akhavan, NS, Pourafshar, S, Arjmandi, BH, and Feresin, RG. Cornus officinalis var. koreana Kitam polyphenol extract decreases pro-inflammatory markers in lipopolysaccharide (LPS)-induced RAW 264.7 macrophages by reducing Akt phosphorylation. J Ethnopharmacol. (2021) 270:113734. doi: 10.1016/j.jep.2020.113734
122. Thu, NT, The Hung, N, Thuy An, NT, Vinh, LB, Binh, BT, Thu, NTB, et al. Four new phenolic compounds from the fruit of Cornus officinalis (Cornaceae) and their anti-inflammatory activity in RAW 264.7 cells. Nat Prod Res. (2021) 36:3806–12. doi: 10.1080/14786419.2021.1887865
123. Han, SR, Paik, SH, Kwon, OJ, Ahn, YM, Lee, BC, Ahn, SY, et al. Effects in metabolism and adipocyte inflammation induced by the complex herbal medicine composed of Cornus officinalis, Dioscorea rhizoma, Aurantii fructus, Mori folium in obese type 2 diabetes mouse model. J Korean Med. (2012) 33:184–99.
124. Fernando, PDSM, Pia, MJ, Zhen, AX, Ahn, MJ, Yi, JM, Choi, YH, et al. Extract of Cornus officinalis protects keratinocytes from particulate matter-induced oxidative stress. Int J Med Sci. (2020) 17:63–70. doi: 10.7150/ijms.36476
125. Janzowski, C, Glaab, V, Samimi, E, Schlatter, J, and Eisenbrand, G. 5-Hydroxymethylfurfural: assessment of mutagenicity, DNA-damaging potential and reactivity toward cellular glutathione. Food Chem Toxicol. (2000) 38:801–9. doi: 10.1016/S0278-6915(00)00070-3
126. Zhang, XM, Chan, CC, Stamp, D, Minkin, S, Archer, M, and Bruce, WR. Initiation and promotion of colonic aberrant crypt foci in rats by 5-hydroxymethy1-2-furaldehyde in thermolyzed sucrose. Carcinogenesis. (1993) 14:773–5. doi: 10.1093/carcin/14.4.773
127. Ding, X, Wang, MY, Yu, ZL, Hu, W, and Cai, BC. Studies on separation, appraisal and the biological activity of 5-HMF in Cornus officinalis. China J Chin Materia Medica. (2008) 33:392–484.
128. Lv, X, Dai, G, Lv, G, Chen, Y, Wu, Y, Shen, H, et al. Synergistic interaction of effective parts in Rehmanniae Radix and Cornus officinalis ameliorates renal injury in C57BL/KsJ-db/db diabetic mice: involvement of suppression of AGEs/RAGE/SphK1 signaling pathway. J Ethnopharmacol. (2016) 185:110–9. doi: 10.1016/j.jep.2016.03.017
129. Han, X, Liu, J, Bai, Y, Hang, A, Lu, T, and Mao, C. An iridoid glycoside from Cornus officinalis balances intestinal microbiome disorder and alleviates alcohol-induced liver injury. J Funct Foods. (2021) 82:104488. doi: 10.1016/j.jff.2021.104488
130. Hu, J, Zhou, J, Wu, J, Chen, Q, du, W, Fu, F, et al. Loganin ameliorates cartilage degeneration and osteoarthritis development in an osteoarthritis mouse model through inhibition of NF-κB activity and pyroptosis in chondrocytes. J Ethnopharmacol. (2020) 247:112261. doi: 10.1016/j.jep.2019.112261
131. Jiang, Z, Ma, Y, Zhou, L, Jiang, H, Wang, M, and Zhan, X. Protective effect of cornel iridoid glycoside in D-galactosamine/tumor necrosis factor-α-injured L02 hepatocytes and its mechanism. J Intercult Ethnopharmacol. (2014) 3:201–5. doi: 10.5455/jice.20140916011549
132. Jeon, YH, Park, MH, and Kim, M. Antibacterial activity of the ethanol extract from Cornus officinalis against some bacteria related to foodborne illness and food spoilage. J East Asian Soc Dietary Life. (2012) 22:692–700.
133. Wei, SM. Synthesis and antibacterial activity of silver nanoparticles synthesized by Cornus officinalis aqueous extract. Chin Tradit Herb Drug. (2019) 50:52–8.
134. Pomfret, EA, Washburn, K, Wald, C, Nalesnik, MA, Douglas, D, Russo, M, et al. Report of a national conference on liver allocation in patients with hepatocellular carcinoma in the United States. Liver Transpl. (2010) 16:262–78. doi: 10.1002/lt.21999
135. Xu, R, Zeng, M, Wu, Y, Wang, S, Zhang, B, Zhang, J, et al. Acetone extract of Cornus officinalis leaves exerts anti-melanoma effects via inhibiting STAT3 signaling. Onco Targets Ther. (2021) 14:3487–501. doi: 10.2147/OTT.S308371
136. Park, E, Lim, E, Yeo, S, Yong, Y, Yang, J, and Jeong, SY. Anti-menopausal effects of Cornus officinalis and Ribes fasciculatum extract in vitro and in vivo. Nutrients. (2020) 12:369. doi: 10.3390/nu12020369
137. Kim, JY, Kim, YK, Choi, MK, Oh, J, Kwak, HB, and Kim, JJ. Effect of Cornus officinalis on receptor activator of nuclear factor-kappaB ligand (RANKL)-induced osteoclast differentiation. J Bone Metabolism. (2012) 19:121–7. doi: 10.11005/jbm.2012.19.2.121
138. An, YA, Hwang, JY, Lee, JS, and Kim, YC. Cornus officinalis methanol extract upregulates melanogenesis in melan-a cells. Toxicol Res. (2015) 31:165–72. doi: 10.5487/TR.2015.31.2.165
139. Park, JS, and Lee, JS. The promoting effect of Cornus officinalis fermented with Lactobacillus rhamnosus on hair growth. Korean J Pharmacognosy. (2011) 42:260–4.
140. Czerwińska, ME, Bobińska, A, Cichocka, K, Buchholz, T, Woliński, K, and Melzig, MF. Cornus mas and Cornus officinalis—a comparison of antioxidant and immunomodulatory activities of standardized fruit extracts in human neutrophils and Caco-2 models. Plan Theory. (2021) 10:2347. doi: 10.3390/plants10112347
141. Li, CY, Li, L, Li, YH, Ai, HX, and Zhang, L. Effects of extract from Cornus officinalis on nitric oxide and NF-kappaB in cortex of cerebral infarction rat model. China J Chin Materia Medica. (2005) 30:1667–70.
142. Nouska, C, Kazakos, S, Mantzourani, I, Alexopoulos, A, Bezirtzoglou, E, and Plessas, S. Fermentation of Cornus mas L. juice for functional low alcoholic beverage production. Curr Res Nutr Food Sci J. (2016) 4:119–24. doi: 10.12944/CRNFSJ.4.Special-Issue-October.16
143. Lihu, Q, Jianghong, R, Zhaorui, M, et al. Study on processing technology of Cornus officinalis goat milk fermented beverage. China Food Safety. (2014) 13:72–5.
144. Yongbin, Z. Development and utilization of Cornus officinalis health food. Agric Products Develop. (2001) 1:25–6.
145. Yang, J, Huang, M, Lu, F, and Gao, W. Optimization of fermented wine production from Cornus officinalis. J Beijing Forestry Univ. (2009) 31:131–6.
146. Rulan, QIN, and Qiao, WANG. Development of healthy jelly with the ex- tract of fructus corni. J Anhui Agric Sci. (2010) 38:4816–26.
147. Zhenjiang, F, and Wei, Z. Study on Cornus healthy vinegar beverage. Sichuan Food Fermentation. (2008) 44:68–70.
Keywords: Cornus officinalis , health characteristics, biological activity, pharmacological effects, Cornaceae
Citation: Deng W, Liu Y, Guo Y, Chen J, Abdu HI, Khan MRU, Palanisamy CP, Pei J and Abd El-Aty AM (2024) A comprehensive review of Cornus officinalis: health benefits, phytochemistry, and pharmacological effects for functional drug and food development. Front. Nutr. 10:1309963. doi: 10.3389/fnut.2023.1309963
Edited by:
Ding-Tao Wu, Chengdu University, ChinaReviewed by:
Hong-Yan Liu, Chinese Academy of Agricultural Sciences, ChinaHui Teng, Guangdong Ocean University, China
Copyright © 2024 Deng, Liu, Guo, Chen, Abdu, Khan, Palanisamy, Pei and Abd El-Aty. This is an open-access article distributed under the terms of the Creative Commons Attribution License (CC BY). The use, distribution or reproduction in other forums is permitted, provided the original author(s) and the copyright owner(s) are credited and that the original publication in this journal is cited, in accordance with accepted academic practice. No use, distribution or reproduction is permitted which does not comply with these terms.
*Correspondence: Wenhui Deng, ZGVuZ3doNTU1NTVAMTYzLmNvbQ==; Jinjin Pei, amluamlucGVpc2xnQHNudXQuZWR1LmNu; A. M. Abd El-Aty, YWJkZWxhdHk0NEBob3RtYWlsLmNvbQ==
†These authors share first authorship