- 1Laboratory of Functional Chemistry and Nutrition of Food, College of Food Science and Engineering, Northwest A&F University, Yangling, Shaanxi, China
- 2Northwest A&F University Shenzhen Research Institute, Shenzhen, Guangdong, China
- 3National Center of Technology Innovation for Dairy, Hohhot, Inner Mongolia, China
- 4Research and Development Center, Xi'an Yinqiao Dairy Technology Co., Ltd., Xi'an, Shaanxi, China
Central nervous system (CNS) disorders present a growing and costly global health challenge, accounting for over 11% of the diseases burden in high-income countries. Despite current treatments, patients often experience persistent symptoms that significantly affect their quality of life. Dietary polysaccharides have garnered attention for their potential as interventions for CNS disorders due to their diverse mechanisms of action, including antioxidant, anti-inflammatory, and neuroprotective effects. Through an analysis of research articles published between January 5, 2013 and August 30, 2023, encompassing the intervention effects of dietary polysaccharides on Alzheimer’s disease, Parkinson’s disease, depression, anxiety disorders, autism spectrum disorder, epilepsy, and stroke, we have conducted a comprehensive review with the aim of elucidating the role and mechanisms of dietary polysaccharides in various CNS diseases, spanning neurodegenerative, psychiatric, neurodevelopmental disorders, and neurological dysfunctions. At least four categories of mechanistic bases are included in the dietary polysaccharides’ intervention against CNS disease, which involves oxidative stress reduction, neuronal production, metabolic regulation, and gut barrier integrity. Notably, the ability of dietary polysaccharides to resist oxidation and modulate gut microbiota not only helps to curb the development of these diseases at an early stage, but also holds promise for the development of novel therapeutic agents for CNS diseases. In conclusion, this comprehensive review strives to advance therapeutic strategies for CNS disorders by elucidating the potential of dietary polysaccharides and advocating interdisciplinary collaboration to propel further research in this realm.
1 Introduction
Central nervous system (CNS) disorders are a growing and costly global health problem. According to the World Health Organization (WHO), CNS disorders account for more than 11% of the overall disease burden in high-income countries (1). These diseases not only affect the life quality of patients but also have a major impact on families, society, and global healthcare resources.
Concerning the high incidence and sophisticated outcome of CNS disorders, pharmacological intervention, surgical resection, and brain stimulation therapy are currently commonly used (2, 3). Despite recent advances in these treatments, many patients continue to experience relevant symptoms that significantly impact their work, relationships, and self-care (4, 5). Hence, scientific researchers are still continuously seeking more effective therapeutic strategies to reduce the burden of these diseases. In recent years, natural components of plants have sparked extensive research interest as a compelling intervention (6). Among them, polysaccharides, as a complex and diverse class of biomolecules, have a significant potential for the intervention of neurological disorders, and their multiple mechanisms of action, such as antioxidant, anti-inflammatory, and neuroprotective, offer new perspectives on therapeutics (6, 7).
By retrieving research articles about the neuroprotective effects of dietary polysaccharides published from 2013 to 2023 on databases such as PubMed, Web of Science, and Google Scholar, it was found that researchers have widely focused on Alzheimer’s disease, Parkinson’s disease, depression, anxiety disorders, autism spectrum disorder, stroke, and epilepsy. Although these diseases cover multiple fields such as neurodegenerative diseases, psychiatric disorders, neurodevelopmental disorders, and neurological dysfunctions, there is a lack of systematic reviews exploring the protective effects and related mechanisms of dietary polysaccharides against CNS diseases.
In this sense, the present review aims to comprehensively elucidate the potential roles, intrinsic mechanisms, and future research directions of dietary polysaccharides in various CNS diseases. Through this review, we hope to provide an in-depth and comprehensive research report for the nutritional disciplines to explore the prospect of dietary polysaccharides as potential therapeutic approaches for a wide range of CNS disorders. At the same time, we encourage interdisciplinary collaborations to promote in-depth research in these areas and make greater contributions to improving patients’ life quality and advancing medical science.
2 Polysaccharides: structures, sources, and biological activities
Polysaccharides are vital macromolecules found abundantly in nature, and serve as fundamental components in the cell walls of plants, fungi, and bacteria, providing structural support and protection (8). In addition, polysaccharides act as an essential energy storage form in plants and animals, offering a sustained and readily available energy source (9, 10). Polysaccharides can be categorized based on their sources, including plants, animals, microorganisms, and edible fungi. Plant-derived polysaccharides originate from various plants, encompassing medicinal herbs, fruits, and vegetables, and can be extracted from roots, stems, leaves, and fruits, or may naturally exist in plants, such as gum and cellulose. Animal-derived polysaccharides, are sourced from animal tissues, for instance, chondroitin sulfate from squid cartilage. Microbial-derived polysaccharides are obtained from microorganisms, such as Lactobacillus polysaccharides and yeast β-glucans. Additionally, there are polysaccharides derived from edible fungi, such as mushrooms, black fungus, and Ganoderma lucidum.
Polysaccharides are typically composed of more than ten repeating units of monosaccharides, such as glucose, fructose, or mannose, linked together in various configurations (11). The specific arrangement of these sugar units, as well as the presence of side chains and branching, contribute to the unique physicochemical properties and biological activities exhibited by polysaccharides (12). In recent years, there has been a growing interest in the study of dietary polysaccharides derived from plants, animals, and microorganisms, which have long been recognized for their valuable contributions to human health and nutrition, as they are believed to exhibit a wide range of biological activities (12).
Dietary polysaccharides have been shown to possess a wide array of health-promoting effects, including immunomodulatory, antioxidant, antitumor, anti-inflammatory, and antimicrobial activities. These bioactivities make them potential candidates for the development of natural medicines, functional foods, and nutraceuticals. Moreover, their ability to regulate various physiological processes, such as oxidative stress, lipid regulation, and gut microbiota modulation, highlights their potential in the prevention and management of CNS disorders (11). The exploration and biological evaluation of dietary polysaccharides have gained significant momentum in recent years, driven by advancements in extraction and purification techniques, like functional factors from the traditional Chinese medicine, marine organisms, edible fungus, and others. The study of dietary polysaccharides represents a fascinating and promising area of research, offering numerous possibilities for the development of novel therapeutic agents and functional foods.
3 Dietary polysaccharides and the interventions for Parkinson’s disease
Parkinson’s disease (PD) is a prevalent neurodegenerative movement disorder that commonly affects middle-aged and elderly individuals, characterized by clinical manifestations such as resting tremor, motor retardation, myotonia, and postural gait abnormalities (13). The major pathological features include the degenerative loss of dopaminergic neurons in the nigrostriatal circuit and the formation of Lewy bodies in multiple brain regions (14). As of 2015, the prevalence of PD in individuals aged over 60 years in China was 1.37% (15), in 2019, worldwide data disclosed over 8.5 million individuals were living with PD, the surge in disability and fatality rates due to PD outpaces all other neurological diseases, imposing a substantial burden on families and society for treatment (16). Currently, there is no cure for PD. Drugs such as Levodopa, Pramipexole, and others are commonly used to increase the level of dopamine or imitate its effects to improve or alleviate symptoms, however, long-term use can result in significant adverse reactions or varying degrees of reduced efficacy (17, 18).
PD’s etiology and pathogenesis remain unclear, but oxidative stress caused by reactive oxygen species (ROS) imbalance in mitochondria is closely implicated in the selective degeneration of dopaminergic neurons in the substantia nigra (19). It is known that dietary polysaccharides act as free radical scavengers, thus protecting mitochondria from oxidative damage and contributing to the normal function and health of the organism, which can be a practical strategy for preventing PD progression (20). Research has demonstrated that dietary polysaccharides (including Grifola frondosa extract (21), low molecular weight chitosan (22), inulin (23), fucoidan (24), astragalus polysaccharides (25), glycosaminoglycans (26), and polysaccharides from the starfish (27)) can significantly reduce ROS levels in animal or cell models of PD while preserving mitochondrial functionality, such as restoring mitochondrial membrane potential (ΔΨm), enhancing mitochondrial respiratory function, and increasing mitochondrial complex enzyme activity. Moreover, polymannuronic acid (28) can alleviate neuroinflammation caused by oxidative stress in PD mouse models. Notably, PD is pathologically based on the death of dopaminergic neurons leading to a reduction in dopamine levels (29). The intervention studies on dietary polysaccharides for PD focus on their direct effects on neural cells. It has been found that in rodent models of PD, polymannuronic acid administration prevented the loss of dopaminergic neurons in the substantia nigra pars compacta (28). Fucoidan mitigated the degeneration of dopaminergic neurons via the peroxisome proliferator-activated receptor gamma coactivator 1-alpha (PGC-1α) and nuclear transcription factor 2 (Nrf2) pathway (24). Astragalus polysaccharides and Ganoderma lucidum polysaccharides both enhanced the proportion of tyrosine hydroxylase-positive cells closely associated with dopaminergic neurons (25, 30).
In 2003, Braak first hypothesized that PD originated in the gastrointestinal tract, proposing that an unproven pathogen or toxin destroying the gastrointestinal mucosal barrier may cause α-synuclein to misfold and deposit in the enteric nerve plexus, and travel retrograde along the posterior intestinal neurons and vagus nerve into the CNS (31). Subsequently, a growing body of research has revealed the profound impact of abnormal microbiota and its metabolites on the pathogenesis and clinical manifestations of PD. Therefore, the regulation of gut microbiota represents another potential intervention method for PD. Polymannuronic acid from brown seaweed polysaccharide treatment caused changes in the gut microbial composition and dramatic changes in the digestion and metabolism of dietary protein and fat, leading to an increase in the content of short-chain fatty acids (SCFAs) in the feces of PD mice (28). Inulin intake, improved the abundance of Bifidobacterium and Lactobacillus, which are strongly positively associated with behavior in a model of gestational exposure to PD toxin (23).
Therefore, diverse dietary polysaccharides showed promise in reducing ROS and preserving mitochondria in PD models. Meanwhile, the effect of dietary polysaccharides on the gut microbiota may be a new avenue of intervention in PD. All these studies showed that dietary polysaccharides can be promising tools in the control of PD (Table 1).
4 Dietary polysaccharides and the interventions for Alzheimer’s disease
Alzheimer’s disease (AD) is a common neurodegenerative disease worldwide and one of the most prevalent forms of dementia in the elderly (32). As reported previously, the all-cause mortality rate for AD in individuals aged 70 years and older is 5.32% (32, 33). Currently, there are approximately 50 million reported cases of AD globally, which will be multiple times higher by 2050 as the population ages (32). The main symptoms of AD vary in severity and progression among individuals, while the most common symptoms include memory loss, cognitive decline, language and communication problems, behavioral and mood changes (33). Brain β-like amyloid (Aβ) deposition and neuronal fibrillary tangles (NFTs) formed by Tau hyperphosphorylation are considered important pathological indicators for AD (34).
Currently, there is no specific treatment for AD due to its unclear pathogenesis. In most cases, AD patients develop neurodegenerative disease as a result of genetics and environment, including apolipoprotein E genotype, metabolic syndrome, neuroinflammation, oxidative stress, and unhealthy diets (35). Oxidative stress, a common feature of neurodegenerative diseases, accelerates the progression of AD by causing mitochondrial dysfunction, neuron membrane damage, apoptosis, and neuroinflammation (36). Therefore, reducing oxidative stress with dietary polysaccharides is considered a prospective strategy to hinder AD pathology. Several studies have found Cistanche deserticola polysaccharides (37), poria cocos polysaccharides (38), non-saponin fraction with rich polysaccharides from Korean red ginseng (39), polysaccharides of Taxus chinensis var. mairei Cheng et L.K.Fu (40), astragalus polysaccharides (41, 42), Angelica sinensis polysaccharides (43), Schisandra chinensis (Turcz.) Baill. polysaccharide (44), Inonotus obliquus polysaccharide (45), Flammulina velutipes polysaccharides (46), Chondroitin sulfate E from squid cartilage (47), and low molecular weight chondroitin sulfate from shark cartilage (48) possessed the ability to inhibit oxidative stress and reduce neuroinflammation in animal models of AD. Additionally, Alpinia oxyphylla crude polysaccharides (49) and polysaccharides of Schisandra Chinensis Fructus (50) inhibited the inflammatory response in the AD mouse model and reduced the release of pro-inflammatory factors such as Interleukin-1 beta (IL-1β) and Tumor Necrosis Factor-alpha (TNF-α). Among them, Angelica sinensis polysaccharides (43), non-saponin fraction with rich polysaccharides from Korean red ginseng (39), polysaccharides from Lycium barbarum (51), poria cocos polysaccharides (38), Ganoderma lucidum polysaccharides (52) and Codonopsis pilosula polysaccharides (53) also reduced neuronal apoptosis, increased neuronal regeneration and restored synaptic dysfunction in some brain regions. Angelica sinensis polysaccharides stimulated the extracellular signal-regulated protein kinase (ERK) / cyclic AMP-responsive element-binding protein (CREB) signaling pathway, amplifying the expression of brain-derived neurotrophic factor (BDNF) and contributing to neuronal survival and regeneration (43).
Emerging evidence has suggested that metabolic dysregulations aggravate the occurrence and development of AD, around 80% of AD patients exhibit insulin resistance, which some scholars refer to as type III diabetes (54). In light of insulin-related signaling’s importance in energy homeostasis, neuronal survival, and memory processes, and the fact that insulin resistance is associated with memory impairment and other AD symptoms, substances that modulate insulin signaling should be considered as potential AD treatments (55). Notably, administering okra polysaccharides (56), yeast β-glucan (57), and Astragalus membranaceus polysaccharides (42) had shown effectiveness in alleviating insulin resistance and reducing cognitive impairment in AD model mice. Additionally, disturbances in amino acid metabolism can cause neurotoxicity, affecting neurotransmitter function, cognition, and emotional regulation, worsening neurodegeneration (58). Yet, treatment with Cistanche deserticola polysaccharides restored valine, L-methionine, uric acid and proline levels in serum, alleviated an amino acid imbalance, and enhanced cognitive function in D-galactose-induced AD mice (37). Furthermore, cholinergic metabolism, crucial for regulating neurotransmission, memory, and muscle movement, might also contribute to AD-related cognitive decline, possibly linked to abnormal cholinergic neuron count and function (59). Encouragingly, Angelica sinensis polysaccharides exhibited promise in reducing acetylcholinesterase (AChE) levels, elevating acetylcholine (ACh) and choline acetyltransferase (ChAT) levels, and improving memory impairment in AD rats through the BDNF/CREB pathway (43). Moreover, the hypothalamic–pituitary–adrenal (HPA) axis, also a key stress response component, significantly influences AD progression (60). These findings underscore the crucial roles of glucose and amino acid metabolism, cholinergic regulation, and HPA axis function in AD pathogenesis while highlighting the therapeutic potential of dietary polysaccharides in addressing AD-associated pathological processes.
Additionally, studies have found that the gut microbiota can influence the occurrence of AD through various pathways mediated by the gut-brain axis (61). Microbiota dysbiosis enhances immuno-senescence, oxidative stress, cytokine secretion, and neuroinflammation, which are involved in the early disease stages of AD (62). Studies have shown that Anoectochilus roxburghii (Wall.) Lindl. polysaccharides (63), Cistanche deserticola polysaccharides (37), inulin (64), fructan (65), and yeast β-glucan (57) could mitigate cognitive deficits and mental disorders by enriching beneficial bacteria, decreasing pathogenic bacteria, restoring the intestinal epithelial barrier, and augmenting SCFAs.
These findings collectively highlight those dietary polysaccharides are expected to intervene in the development and progression of AD by alleviating oxidative stress, neuroinflammation, metabolic dysregulation, and gut microbiota disorders (Table 2), presenting a promising direction for future therapeutic strategies.
5 Dietary polysaccharides and the interventions for depression and anxiety disorder
As a common psychiatric disorder, depression is characterized by feelings of sadness, guilt, and lack of interest in and self-worth; tiredness; poor concentration; poor appetite, and disturbed sleep (66). In 2017, the WHO reported that approximately 4.4% of the global population suffers from depression, and depression has become the leading contributor to suicide attempts (66). Anxiety disorder, another most common psychiatric disorder, is characterized by symptoms such as nervousness, restlessness, and vegetative dysfunction without a specific trigger (67). Up to 33.7% of the population suffers from anxiety disorders during their lifetime, and anxiety disorders are prone to combine with other mental disorders (67). Although depression and anxiety are distinct emotional states, they often co-occur and are highly comorbidity (68). Data suggests that approximately 85% of patients with depression also experience significant anxiety, while 90% of individuals with anxiety disorders also have symptoms of depression. Recent evidence suggests genetic and neurobiological similarities between depressive and anxiety disorders (69).
Due to its comparatively high oxygen utilization and lipid-rich constitution, the brain is considered particularly vulnerable to oxidative damage (70). Together with the pathological changes associated with many psychiatric syndromes, this intrinsic oxidative vulnerability suggests that oxidative damage could be a plausible pathogenic candidate for anxiety and depression (70). Oxidative stress-induced neuroinflammation not only affects individual neurons but also reaches synaptic connections, known as synapses (71). Abnormal structure and function of synapses, affect the communication between neurons and the balance of neurotransmitters, which contribute to the development and progression of depressive and anxious symptoms (72). However, dietary polysaccharides have demonstrated beneficial effects in improving depression and anxiety, with their antioxidative and anti-inflammatory properties playing significant roles. Administration of acidic polysaccharides from poria (73), inulin (74, 75), Polygonatum sibiricum F. Delaroche polysaccharides (76), Ganoderma lucidum polysaccharides (77), polysaccharide from okra (Abelmoschus esculentus (L) Moench) (78), ameliorated anxiety disorders and depressive behaviors, regulated the levels of multiple factors related to oxidative stress, reduced proinflammatory cytokine levels. At the same time, all the dietary polysaccharides mentioned above provided protective effects on neurons, such as reducing synaptic damage, enhancing synaptic activity, and regulating the expression of synapse-related proteins and genes. In addition, Lycium Barbarum polysaccharides (79) also alleviated the depression-like and social anxiety-like behavior by enhancing synaptic plasticity and maintaining the normal function of synapses.
The HPA axis is a crucial component of the neuroendocrine system. It becomes intensified in response to external stimuli, leading to the secretion of corticosterone by the adrenal glands, which helps the body adapt to the new environment (80). Hyperfunction of the HPA axis is considered an important factor in the development of depression and anxiety (81), although the regulation of other peptides or hormones within the HPA axis may differ between these two disorders (82). In addition, the intimate connection between the HPA axis and neurotransmitters also regulates the mood, cognition, and behavior in depression and anxiety patients (83, 84). Studies have shown that Polygonatum sibiricum F. Delaroche polysaccharides (76), inhibited the hyperfunctioning of the HPA axis. Moreover, partially hydrolyzed guar gum (85), acidic polysaccharides from poria (73), Polygonatum sibiricum F. Delaroche polysaccharides (76), total polysaccharides of Lilium lancifolium Thunberg (86), and polysaccharide from Ginkgo biloba leaves (87), regulated the neurotransmitter levels in multiple brain regions in rodent models of depression and anxiety.
There is increasing evidence that gut microbiota is associated with anxiety and depression. Although diversity findings were inconsistent, specific bacterial taxa were implicated according to clinical research findings: higher abundance of proinflammatory species (e.g., Enterobacteriaceae and Desulfovibrio), and lower SCFAs producing-bacteria (e.g., Faecalibacterium) in patients with anxiety/depressive disorders (88). An analysis of the composition of gut microbiota suggested that polysaccharides from okra (Abelmoschus esculentus (L) Moench) decreased the relative proportions of Bacteroidetes and Actinobacteria, while increasing Firmicutes at the phylum level in chronic unpredictable mild stress (CUMS)-induced depression mice. Simultaneously, the generation of SCFAs were also found to contribute positively to the antidepressant-like effect (78). Administration of partially hydrolyzed guar gum (85), inulin (74, 75), polydextrose (89), polysaccharide from Ginkgo biloba leaves (87), 3’Sialyllactose and 6’Sialyllactose (90) also had certain regulatory effects on the gut microbiota in rodent models of depression and anxiety.
The above studies demonstrate that polysaccharide compounds can improve and alleviate depression and anxiety disorders (Table 3). Nevertheless, the mechanisms by which dietary polysaccharides regulate the expression of synapse-associated proteins, reduce synaptic damage, and regulate the gut microbiota to prevent depression and anxiety need further research. In addition, the efficacy of dietary polysaccharides brought into the daily diet requires to be evaluated in further clinical trials.
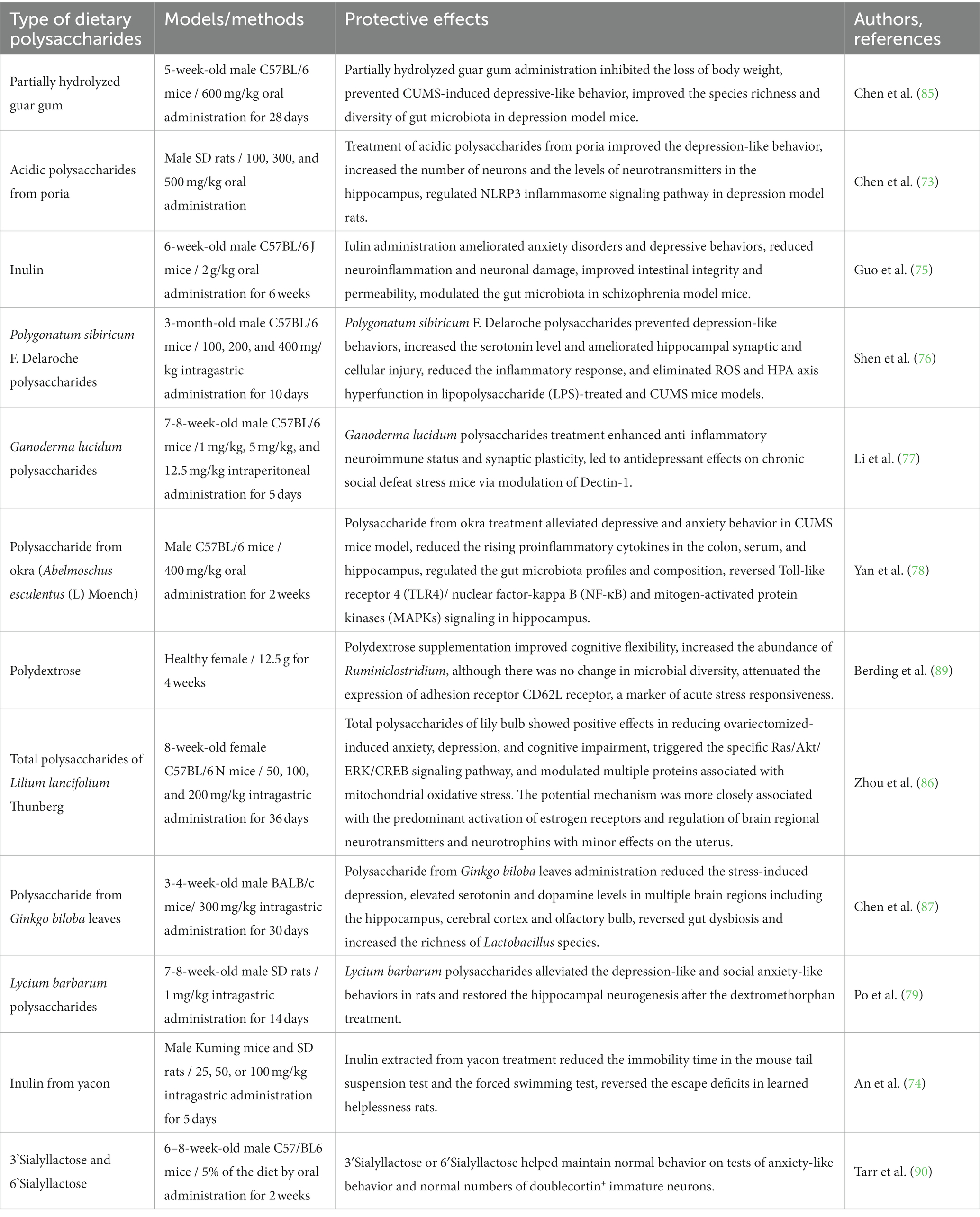
Table 3. Recent studies on neuroprotective effects of dietary polysaccharides on depression and anxiety disorder models.
6 Dietary polysaccharides and the interventions for autism spectrum disorder
Autism spectrum disorder (ASD) is a neurodevelopmental disorder believed to be caused by early brain changes and neuronal reorganization. Individuals with ASD exhibit a wide range of symptoms, but they share a common set of disorders, including impairments in social communication and repetitive sensory-motor behaviors (91). The average prevalence of ASD in Asia, Europe, and North America is estimated to be approximately 1%, males are more affected by autism than females, and comorbidity is common (more than 70% of people with autism have concurrent conditions) (92).
To date, the exact underlying causes of ASD are not yet fully understood. Clinical studies have shown that gastrointestinal symptoms such as constipation, diarrhea and gut microbiota imbalance are common in ASD patients (93). Unfortunately, there are no effective treatments for the core symptoms of ASD (94). Statistics show that approximately 50–70% of ASD patients resort to bio-related therapies such as antibiotics, antifungal and antiviral drugs, gastrointestinal medications, nutritional supplement therapy, and restrictive or special diets. However, most of these interventions lack comprehensive safety and efficacy assessments (94). With the deepening research on the relationship between human microbiome and ASD, scientists have gradually realized the importance of gut microbiota in affecting neurodevelopment and brain function. Partially hydrolyzed guar gum, a form of prebiotic dietary water-soluble fiber, has been shown to modulate the gut microbiota and stimulate the production of SCFAs in healthy adults. Moreover, the supplementation with partially hydrolyzed guar gum resulted in a trend toward decreased serum level of Interleukin-6 (IL-6) and TNF-α, which help improve behavioral irritability and constipation of children with ASD (95). Hence, polysaccharide intervention seems to help in the effective treatment of ASD. However, the mechanism of dietary polysaccharides to relieve constipation and gut microbiota dysbiosis caused by ASD also needs further study. Moreover, there are fewer dietary polysaccharides that can interfere with ASD, and it is the direction of our further research to find more dietary polysaccharides to treat children with ASD.
7 Dietary polysaccharides and the interventions for epilepsy
Epilepsy is a chronic non-communicable neurological dysfunctions disorder. It is primarily characterized by seizures, repetition, stereotypy, and transience, which belong to an involuntary movement involving a portion or the whole body, sometimes combined with the loss of consciousness and control of bowel or bladder function (96). Epilepsy can affect individuals of all ages, genders, races, income groups, and geographical regions (97). According to the WHO, approximately 5 million people worldwide are diagnosed with epilepsy each year. As of 2019, China alone had over 9 million people living with epilepsy, and the number of new cases continues to rise (98).
Seizures in epilepsy can be triggered by various factors that disrupt normal neuron activity, including illnesses, brain damage, abnormal brain development, imbalances in neurotransmitters, changes in ion channels, or a combination of these and other factors (99). Mutations in genes related to ion channels have been strongly linked to seizures, especially calcium ion channels (100). The effects of Ca2+ are typically mediated through its interaction with calmodulin (CaM) (101), epileptogenic factors can downregulate CaM, leading to increased neuronal activity and the development of epilepsy. CaM may also indirectly contribute to the pathological process of epilepsy by modulating calcium/CaM-dependent protein kinases (CaMK) (102). Studies have shown that Ganoderma lucidum polysaccharides inhibited the Ca2+ accumulation in neurons and subsequent stimulation of CaMK II α expression, which indicates a beneficial role in the prevention or treatment of epilepsy (103).
Mitochondrial dysfunction and oxidative stress have also been considered potential causes of epileptic seizures. Seizures can trigger neuroinflammatory responses that further directly impact the electrical activity of neurons and glial cells, and exacerbate CNS damage, forming the pathological basis for refractory epilepsy (104). Dietary polysaccharides have shown potential in reducing inflammatory responses, inhibiting the expression of neurotransmission-related genes, and improving hippocampal tissue damage in epilepsy models. Due to the antioxidant and anti-inflammatory properties of chondroitin sulfate, a significant reduction in seizure mice induced by pentylenetetrazole and pilocarpine was observed (105). Fructus corni polysaccharide treatment (106) decreased levels of ROS and malondialdehyde (MDA), increased superoxide dismutase (SOD) activity, and inhibited expressions of phosphorylated-Jun N-terminal Kinase (p-JNK), cytochrome C, and caspase-3 in an epileptic rat model. Angelica polysaccharide promoted cell proliferation, inhibited apoptosis, and suppressed IL-1β, TNF-α, and IL-6 production in epilepsy cell models (107). Thus, dietary polysaccharides intervention to improve epilepsy focuses on inflammatory response, oxidative stress, and ion channels and signaling pathways regulated by related genes (Table 4). In the future, combined with microbial studies, it is possible to explore whether dietary polysaccharides show their functional activities by regulating gut microbiota and intestinal microecology.
8 Dietary polysaccharides and the interventions for stroke
Stroke, an acute cerebrovascular disease, is another neurological disorder. About 80–85% of strokes are ischemic (the blockage of blood vessels preventing blood from flowing to the brain) (108), while about 15–20% are hemorrhagic (the sudden rupture of blood vessels in the brain) (109). The clinical manifestations of stroke include sudden weakness on one side of the body, fainting, unconsciousness, confusion, difficulty speaking or understanding, vision problems, and loss of balance or coordination (110). Over the past 20 years, the global burden of stroke has increased significantly, with an increase of 70.0% in incident strokes, 43.0% in deaths caused by stroke, 102.0% in prevalent strokes, and 143.0% in disability-adjusted life years (111). The prevalence of stroke in northern China in 2022 has nearly doubled compared to 2010 (112).
Stroke is a significant contributor to premature mortality, yet there is no effective treatment for stroke to improve blood circulation in the affected brain area and restore neurological function (113). Clinically, stroke symptoms are alleviated with medications targeting neuroprotection and cerebrovascular circulation enhancement. However, prolonged use of these drugs may adversely affect liver and kidney function, further impairing overall organ function (110). Consequently, dietary polysaccharides and their derivatives have been studied for their potential to alleviate the effects of stroke. Lycium barbarum polysaccharides exerted a neuroprotective effect against ischemic injury through dual actions of activating the N-methyl-D-aspartic acid receptor subunit 2A (NR2A) signaling pathway and inhibiting the N-methyl-D-aspartic acid receptor subunit 2B (NR2B) signaling pathway. This effect reduced the death of CA1 neurons after transient global cerebral ischemia and improved memory impairment in ischemic rats (114). Additionally, Momordica charantia polysaccharides provided neuroprotection against cerebral ischemia/reperfusion injury by inhibiting lipid peroxidation and preserving antioxidant enzyme activity (115). Furthermore, the neuroprotective effect of Ginkgo biloba polysaccharide is achieved by suppressing oxidative stress and reducing the concentration of inflammatory factors. This action decreased the cerebral infarction area in rats and ameliorates neurofunctional deficits (116). Hence, dietary polysaccharides play a beneficial role in ischemic stroke mainly by alleviating oxidative stress, reducing inflammatory factors, and promoting neuronal cell repair. These effects of dietary polysaccharides also suggest that the gut microbiota might play a crucial role in stroke, however, the exact impact of gut microbiota on stroke remains to be further studied. Table 5 provides examples of studies that have explored the use of dietary polysaccharides in improving cerebral stroke.
9 Remarks
In conclusion, the review sheds light on the role of dietary polysaccharides in neurodegenerative disorders, psychiatric disorders, neurodevelopmental disorders, and neurological dysfunctions. The potential mechanisms of dietary polysaccharides involved ameliorating oxidative stress, neuronal injury, metabolic abnormalities, and gut microbiota disorder (Figure 1). Multifaceted effects of dietary polysaccharides on these diseases are noteworthy, with a common thread being their antioxidant activity and gut microbiota regulation.
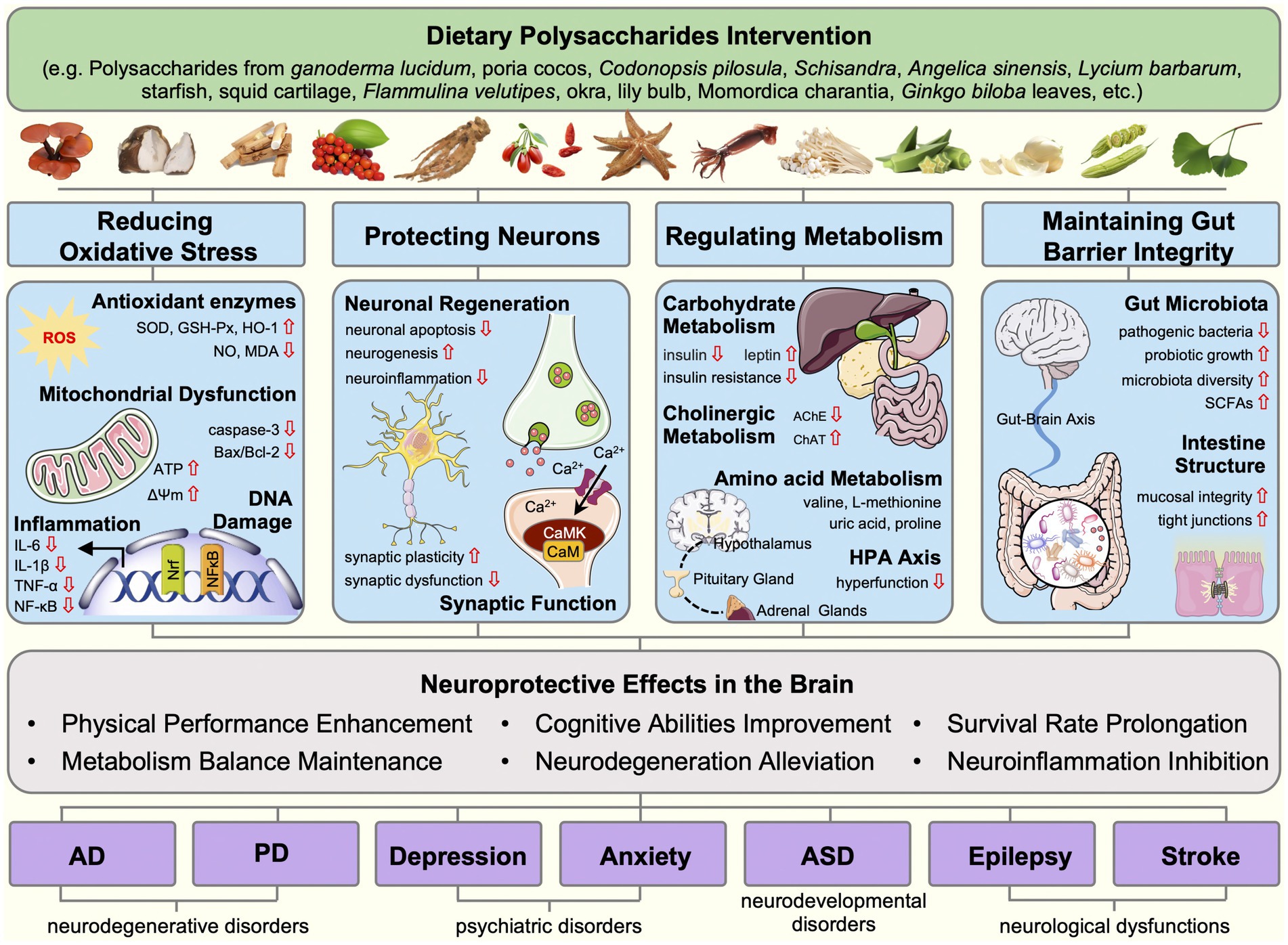
Figure 1. Mechanisms linking dietary polysaccharides and CNS diseases, Interventions by dietary polysaccharides in neurodegenerative diseases (Alzheimer’s disease, and Parkinson’s disease), mental disorders (depression and anxiety), neurodevelopmental disorders (autism spectrum disorders), and neurological dysfunctions (epilepsy and stroke) can be categorized into four mechanistic foundations, including the oxidative stress reduction, neuronal protection, metabolism regulation, and gut barrier integrity maintenance. Dietary polysaccharides reduce oxidative stress by enhancing antioxidant enzyme activity, restoring mitochondrial function, and reducing the production of inflammatory factors. Dietary polysaccharides restore the normal function of neurons by increasing neurogenesis, alleviating neuroinflammation, regulating ion channels, and enhancing synaptic plasticity. Dietary polysaccharides maintain metabolic balance and promote brain health by modulating carbohydrate metabolism, cholinergic metabolism, amino acid metabolism, HPA axis, and the neurotransmitter system. Furthermore, dietary polysaccharides exert neuroprotective effects by regulating gut microbiota and their metabolite composition, preserving gut mucosal barrier integrity, and influencing gut-brain communication.
In models of neurodegenerative diseases, such as PD or AD, dietary polysaccharides have been shown to provide neuroprotective effects by decreasing oxidative stress and modulating the gut microbiota. Dietary polysaccharides are found to reduce oxidative stress and inflammation, regulate the HPA axis and neurotransmitter system, and maintain gut microbiota balance in psychiatric disorders, such as anxiety and depression. By regulating the gut microbiota, dietary polysaccharides may also influence neurodevelopmental disorders (like ASD). In addition, dietary polysaccharides exert antiepileptic effects by controlling ion channels, reducing oxidative stress and neuroinflammation, and restoring mitochondrial function. The neuroprotective properties of dietary polysaccharides in the context of stroke further highlight their role in protecting neurons by inhibiting oxidative stress and anti-inflammatory mechanisms. Together, these comprehensive findings emphasize the critical role of oxidative stress as a key factor in the development of CNS diseases. Dietary polysaccharides are potential therapeutic agents for alleviating these diseases due to their outstanding antioxidant and anti-inflammatory properties. Notably, dietary polysaccharides can also exert beneficial effects by regulating the composition of gut microbiota and its metabolites, protecting the integrity of the intestinal mucosal barrier, and affecting gut-brain communication.
However, it is imperative to acknowledge that despite the range of diseases examined in this study, the full spectrum of CNS disorders susceptible to polysaccharide regulation remains incompletely explored, underscoring the need for further research initiatives. Additionally, this paper falls short of clarifying which specific components and structures in dietary polysaccharides work and how the metabolic fragments of dietary polysaccharides influence the organism. Moreover, it is crucial to recognize that a substantial portion of the studies reviewed are confined to in vitro or animal experimentation. Despite the intake of dietary polysaccharides from natural sources in the normal diet is generally recognized as safe (GRAS), a series of clinical trials is still imperative to determine the lowest effective dosage in human and to minimize the side effects. It is possible to convert the dosage between animals and human by body surface area or body weight, considering the appropriate dosage and safety of dietary polysaccharides in human beings (117). Thereby, advanced preclinical and clinical investigations are needed to substantiate the translational potential of dietary polysaccharides as intervention candidates for CNS disorders. Consequently, a more comprehensive understanding of dietary polysaccharides’ true potential in promoting CNS health hinges on a broader repertoire of research endeavors, including more extensive preclinical evaluations and compelling clinical trials. These collective endeavors are poised to shed light on the genuine capabilities of dietary polysaccharides in CNS health and provide a robust platform for the development of novel therapeutic strategies.
Author contributions
RG: Conceptualization, Writing – original draft. JP: Conceptualization, Writing – original draft. JZ: Conceptualization, Writing – original draft. XX: Writing – review & editing. JL: Writing – review & editing. JML: Writing – review & editing. WW: Writing – original draft. SZ: Writing – original draft. YZ: Writing – original draft. ZZ: Writing – review & editing. HC: Writing – review & editing. TY: Conceptualization, Writing – review & editing. SW: Writing – review & editing. ZL: Conceptualization, Writing – review & editing.
Funding
The author(s) declare financial support was received for the research, authorship, and/or publication of this article. This research was supported by the Sci-Tech Innovation 2030 Brain Science and Brain-Like Intelligence Technology Project (2022ZD0208100, ZL); Shenzhen Central Government Guidance Local Science and Technology Development Fund Project (2021Szvup119, ZL); the Regional Consolidated Fund-Youth Fund Project in Guangdong Province (2022A1515110717, RG); China Postdoctoral Science Foundation (2022 M72261, RG); Guangdong Basic and Applied Basic Research Foundation (2021A1515110813, TY); Shenzhen Fundamental Research Program (JYC20220530161401004, TY); National Center of Technology Innovation for Dairy (2021-NationalCenter of Technology Innovation for Dairy-5, SW). Additionally, the figures were partly generated using Servier Medical Art, provided by Servier, licensed under a Creative Commons Attribution 3.0 unported license.
Conflict of interest
SW was employed by Xi'an Yinqiao Dairy Technology Co., Ltd.
The remaining authors declare that the research was conducted in the absence of any commercial or financial relationships that could be construed as a potential conflict of interest.
Publisher’s note
All claims expressed in this article are solely those of the authors and do not necessarily represent those of their affiliated organizations, or those of the publisher, the editors and the reviewers. Any product that may be evaluated in this article, or claim that may be made by its manufacturer, is not guaranteed or endorsed by the publisher.
References
1. World Health Organization . (2020). Neurological disorders: public health challenges. Available at: https://www.who.int/publications/i/item/9789241563369.
2. Fung, TC, Olson, CA, and Hsiao, EY. Interactions between the microbiota, immune and nervous systems in health and disease. Nat Neurosci. (2017) 20:145–55. doi: 10.1038/nn.4476
3. Kim, YK, and Shin, C. The microbiota-gut-brain Axis in neuropsychiatric disorders: pathophysiological mechanisms and novel treatments. Curr Neuropharmacol. (2018) 16:559–73. doi: 10.2174/1570159X15666170915141036
4. Kalin, NH . Developing innovative and novel treatment strategies. Am J Psychiatry. (2019) 176:885–7. doi: 10.1176/appi.ajp.2019.19090952
5. Ceskova, E, and Silhan, P. Novel treatment options in depression and psychosis. Neuropsychiatr Dis Treat. (2018) 14:741–7. doi: 10.2147/NDT.S157475
6. Li, H, Ding, F, Xiao, L, Shi, R, Wang, H, Han, W, et al. Food-derived antioxidant polysaccharides and their pharmacological potential in neurodegenerative diseases. Nutrients. (2017) 9:778. doi: 10.3390/nu9070778
7. Xu, XL, Li, S, Zhang, R, and Le, WD. Neuroprotective effects of naturally sourced bioactive polysaccharides: an update. Neural Regen Res. (2022) 17:1907–12. doi: 10.4103/1673-5374.335142
8. Tudu, M, and Samanta, A. Natural polysaccharides: chemical properties and application in pharmaceutical formulations. Eur Polym J. (2023) 184:111801. doi: 10.1016/j.eurpolymj.2022.111801
9. Bilal, M, Gul, I, Basharat, A, and Qamar, SA. Polysaccharides-based bio-nanostructures and their potential food applications. Int J Biol Macromol. (2021) 176:540–57. doi: 10.1016/j.ijbiomac.2021.02.107
10. Liu, J, Willför, S, and Xu, C. A review of bioactive plant polysaccharides: biological activities, functionalization, and biomedical applications. Bioact Carbohydr Diet Fibre. (2015) 5:31–61. doi: 10.1016/j.bcdf.2014.12.001
11. Xu, J, Zhang, J, Sang, Y, Wei, Y, Chen, X, Wang, Y, et al. Polysaccharides from medicine and food homology materials: a review on their extraction, purification, structure, and biological activities. Molecules. (2022) 27:27(10). doi: 10.3390/molecules27103215
12. Xue, H, Wang, W, Bian, J, Gao, Y, Hao, Z, and Tan, J. Recent advances in medicinal and edible homologous polysaccharides: extraction, purification, structure, modification, and biological activities. Int J Biol Macromol. (2022) 222:1110–26. doi: 10.1016/j.ijbiomac.2022.09.227
13. de Lau, LM, and Breteler, MM. Epidemiology of Parkinson's disease. Lancet Neurol. (2006) 5:525–35. doi: 10.1016/S1474-4422(06)70471-9
14. Jankovic, J . Parkinson's disease: clinical features and diagnosis. J Neurol Neurosurg Psychiatry. (2008) 79:368–76. doi: 10.1136/jnnp.2007.131045
15. Qi, S, Yin, P, Wang, L, Qu, M, Kan, GL, Zhang, H, et al. Prevalence of Parkinson's disease: a community-based study in China. Mov Disord. (2021) 36:2940–4. doi: 10.1002/mds.28762
16. Nakmode, DD, Day, CM, Song, Y, and Garg, S. The management of Parkinson’s disease: an overview of the current advancements in drug delivery systems. Pharmaceutics. (2023) 15:1503. doi: 10.3390/pharmaceutics15051503
17. Brodsky, MA, Park, BS, and Nutt, JG. Effects of a dopamine agonist on the pharmacodynamics of levodopa in Parkinson disease. Arch Neurol. (2010) 67:27–32. doi: 10.1001/archneurol.2009.287
18. Zhang, PL, Wang, YX, Chen, Y, Zhang, CH, and Li, CH. The efficacy of homemade tolcapone in the treatment of patients with Parkinsons disease. Exp Ther Med. (2018) 15:127–30. doi: 10.3892/etm.2017.5377
19. Qin, L, Liu, Y, Hong, JS, and Crews, FT. NADPH oxidase and aging drive microglial activation, oxidative stress, and dopaminergic neurodegeneration following systemic LPS administration. Glia. (2013) 61:855–68. doi: 10.1002/glia.22479
20. Liao, C, Wu, L, Zhong, W, Zheng, Q, Tan, W, Feng, K, et al. Cellular antioxidant properties of Ischnoderma Resinosum polysaccharide. Molecules. (2022) 27:7717. doi: 10.3390/molecules27227717
21. Tripodi, F, Falletta, E, Leri, M, Angeloni, C, Beghelli, D, Giusti, L, et al. Anti-aging and neuroprotective properties of Grifola frondosa and Hericium erinaceus extracts. Nutrients. (2022) 14:4368. doi: 10.3390/nu14204368
22. Pramod Kumar, P, and Harish Prashanth, KV. Diet with low molecular weight chitosan exerts neuromodulation in rotenone induced Drosophila model of Parkinson's disease. Food Chem Toxicol. (2020) 146:111860. doi: 10.1016/j.fct.2020.111860
23. Krishna, G, Muralidhara . Oral supplements of inulin during gestation offsets rotenone-induced oxidative impairments and neurotoxicity in maternal and prenatal rat brain. Biomed Pharmacother. (2018) 104:751–62. doi: 10.1016/j.biopha.2018.05.107
24. Zhang, L, Hao, J, Zheng, Y, Su, R, Liao, Y, Gong, X, et al. Fucoidan protects dopaminergic neurons by enhancing the mitochondrial function in a rotenone-induced rat model of Parkinson's disease. Aging Dis. (2018) 9:590–604. doi: 10.14336/AD.2017.0831
25. Liu, H, Chen, S, Guo, C, Tang, W, Liu, W, and Liu, Y. Astragalus polysaccharide protects neurons and stabilizes mitochondrial in a mouse model of Parkinson disease. Med Sci Monit. (2018) 24:5192–9. doi: 10.12659/MSM.908021
26. Medeiros, TB, Cosendey, P, Gerin, DR, de Sousa, GF, Portal, TM, and Monteiro-de-Barros, C. The effect of the sulfation patterns of dermatan and chondroitin sulfate from vertebrates and ascidians on their neuritogenic and neuroprotective properties. Int J Biol Macromol. (2023) 247:125830. doi: 10.1016/j.ijbiomac.2023.125830
27. Zhang, W, Wang, J, Jin, W, and Zhang, Q. The antioxidant activities and neuroprotective effect of polysaccharides from the starfish Asterias rollestoni. Carbohydr Polym. (2013) 95:9–15. doi: 10.1016/j.carbpol.2013.02.035
28. Dong, X-L, Wang, X, Liu, F, Liu, X, Du, Z-R, Li, RW, et al. Polymannuronic acid prevents dopaminergic neuronal loss via brain-gut-microbiota axis in Parkinson's disease model. Int J Biol Macromol. (2020) 164:994–1005. doi: 10.1016/j.ijbiomac.2020.07.180
29. Emamzadeh, FN, and Surguchov, A. Parkinson's disease: biomarkers, treatment, and risk factors. Front Neurosci. (2018) 12:612. doi: 10.3389/fnins.2018.00612
30. Guo, SS, Cui, XL, and Rausch, WD. Ganoderma Lucidum polysaccharides protect against MPP(+) and rotenone-induced apoptosis in primary dopaminergic cell cultures through inhibiting oxidative stress. Am J Neurodegener Dis. (2016) 5:131–44.
31. Braak, H, Rüb, U, Gai, WP, and Del Tredici, K. Idiopathic Parkinson's disease: possible routes by which vulnerable neuronal types may be subject to neuroinvasion by an unknown pathogen. J Neural Transm (Vienna). (2003) 110:517–36. doi: 10.1007/s00702-002-0808-2
32. Breijyeh, Z, and Karaman, R. Comprehensive review on Alzheimer's disease: causes and treatment. Molecules. (2020) 25:5789. doi: 10.3390/molecules25245789
33. Zheng, LF, Li, GC, Gao, DW, Wang, S, Meng, XF, Wang, C, et al. Cognitive frailty as a predictor of dementia among older adults: a systematic review and meta-analysis. Arch Gerontol Geriatr. (2020) 87:103997. doi: 10.1016/j.archger.2019.103997
34. Erkkinen, MG, Kim, MO, and Geschwind, MD. Clinical neurology and epidemiology of the major neurodegenerative diseases. Cold Spring Harb Perspect Biol. (2018) 10:a033118. doi: 10.1101/cshperspect.a033118
35. Palmer, AM . Neuroprotective therapeutics for Alzheimer's disease: progress and prospects. Trends Pharmacol Sci. (2011) 32:141–7. doi: 10.1016/j.tips.2010.12.007
36. Chen, Z, and Zhong, C. Oxidative stress in Alzheimer's disease. Neurosci Bull. (2014) 30:271–81. doi: 10.1007/s12264-013-1423-y
37. Gao, Y, Li, B, Liu, H, Tian, Y, Gu, C, Du, X, et al. Cistanche deserticola polysaccharides alleviate cognitive decline in aging model mice by restoring the gut microbiota-brain axis. Aging. (2021) 13:15320–35. doi: 10.18632/aging.203090
38. Zhou, X, Zhang, Y, Jiang, Y, Zhou, C, and Ling, Y. Poria cocos polysaccharide attenuates damage of nervus in Alzheimer's disease rat model induced by D-galactose and aluminum trichloride. Neuroreport. (2021) 32:727–37. doi: 10.1097/WNR.0000000000001648
39. Shin, SJ, Nam, Y, Park, YH, Kim, MJ, Lee, E, Jeon, SG, et al. Therapeutic effects of non-saponin fraction with rich polysaccharide from Korean red ginseng on aging and Alzheimer's disease. Free Radic Biol Med. (2021) 164:233–48. doi: 10.1016/j.freeradbiomed.2020.12.454
40. Zhang, S, Li, L, Hu, J, Ma, P, and Zhu, H. Polysaccharide of Taxus chinensis var. mairei Cheng et L.K.Fu attenuates neurotoxicity and cognitive dysfunction in mice with Alzheimer's disease. Pharm Biol. (2020) 58:959–68. doi: 10.1080/13880209.2020.1817102
41. Qin, X, Hua, J, Lin, SJ, Zheng, HT, Wang, JJ, Li, W, et al. Astragalus polysaccharide alleviates cognitive impairment and β-amyloid accumulation in APP/PS1 mice via Nrf2 pathway. Biochem Biophys Res Commun. (2020) 531:431–7. doi: 10.1016/j.bbrc.2020.07.122
42. Huang, YC, Tsay, HJ, Lu, MK, Lin, CH, Yeh, CW, Liu, HK, et al. Astragalus membranaceus-polysaccharides ameliorates obesity, hepatic steatosis, neuroinflammation and cognition impairment without affecting amyloid deposition in metabolically stressed APPswe/PS1dE9 mice. Int J Mol Sci. (2017) 18:2746. doi: 10.3390/ijms18122746
43. Du, Q, Zhu, X, and Si, J. Angelica polysaccharide ameliorates memory impairment in Alzheimer's disease rat through activating BDNF/TrkB/CREB pathway. Exp Biol Med (Maywood). (2020) 245:1–10. doi: 10.1177/1535370219894558
44. Liu, Y, Liu, Z, Wei, M, Hu, M, Yue, K, Bi, R, et al. Pharmacodynamic and urinary metabolomics studies on the mechanism of Schisandra polysaccharide in the treatment of Alzheimer's disease. Food Funct. (2019) 10:432–47. doi: 10.1039/C8FO02067A
45. Han, Y, Nan, S, Fan, J, Chen, Q, and Zhang, Y. Inonotus obliquus polysaccharides protect against Alzheimer's disease by regulating Nrf2 signaling and exerting antioxidative and antiapoptotic effects. Int J Biol Macromol. (2019) 131:769–78. doi: 10.1016/j.ijbiomac.2019.03.033
46. Zhang, Y, Li, H, Yang, X, Jin, G, and Zhang, Y. Cognitive-enhancing effect of polysaccharides from Flammulina velutipes on Alzheimer's disease by compatibilizing with ginsenosides. Int J Biol Macromol. (2018) 112:788–95. doi: 10.1016/j.ijbiomac.2018.02.040
47. Wang, X, Yang, Y, Zou, J, Li, Y, and Zhang, XG. Chondroitin sulfate E alleviates β-amyloid toxicity in transgenic Caenorhabditis elegans by inhibiting its aggregation. Int J Biol Macromol. (2022) 209:1280–7. doi: 10.1016/j.ijbiomac.2022.04.124
48. Zhao, N, Wu, L, Zhang, X, Jiang, W, and Wang, F. Low molecular weight chondroitin sulfate ameliorates pathological changes in 5XFAD mice by improving various functions in the brain. Neuropharmacology. (2021) 199:108796. doi: 10.1016/j.neuropharm.2021.108796
49. Shi, W, Zhong, J, Zhang, Q, and Yan, C. Structural characterization and antineuroinflammatory activity of a novel heteropolysaccharide obtained from the fruits of Alpinia oxyphylla. Carbohydr Polym. (2020) 229:115405. doi: 10.1016/j.carbpol.2019.115405
50. Xu, M, Yan, T, Fan, K, Wang, M, Qi, Y, Xiao, F, et al. Polysaccharide of Schisandra Chinensis Fructus ameliorates cognitive decline in a mouse model of Alzheimer's disease. J Ethnopharmacol. (2019) 237:354–65. doi: 10.1016/j.jep.2019.02.046
51. Zhou, Y, Duan, Y, Huang, S, Zhou, X, Zhou, L, Hu, T, et al. Polysaccharides from Lycium barbarum ameliorate amyloid pathology and cognitive functions in APP/PS1 transgenic mice. Int J Biol Macromol. (2020) 144:1004–12. doi: 10.1016/j.ijbiomac.2019.09.177
52. Huang, S, Mao, J, Ding, K, Zhou, Y, Zeng, X, Yang, W, et al. Polysaccharides from Ganoderma lucidum promote cognitive function and neural progenitor proliferation in mouse model of Alzheimer's disease. Stem Cell Reports. (2017) 8:84–94. doi: 10.1016/j.stemcr.2016.12.007
53. Wan, L, Zhang, Q, Luo, H, Xu, Z, Huang, S, Yang, F, et al. Codonopsis pilosula polysaccharide attenuates Aβ toxicity and cognitive defects in APP/PS1 mice. Aging. (2020) 12:13422–36. doi: 10.18632/aging.103445
54. Yu, L, Jin, J, Xu, Y, and Zhu, X. Aberrant energy metabolism in Alzheimer's disease. J Transl Int Med. (2022) 10:197–206. doi: 10.2478/jtim-2022-0024
55. González, A, Calfío, C, Churruca, M, and Maccioni, RB. Glucose metabolism and AD: evidence for a potential diabetes type 3. Alzheimers Res Ther. (2022) 14:56. doi: 10.1186/s13195-022-00996-8
56. Yan, T, Nian, T, Wu, B, Xiao, F, He, B, Bi, K, et al. Okra polysaccharides can reverse the metabolic disorder induced by high-fat diet and cognitive function injury in Aβ1–42 mice. Exp Gerontol. (2020) 130:110802. doi: 10.1016/j.exger.2019.110802
57. Xu, M, Mo, X, Huang, H, Chen, X, Liu, H, Peng, Z, et al. Yeast β-glucan alleviates cognitive deficit by regulating gut microbiota and metabolites in Aβ(1)(−)(42)-induced AD-like mice. Int J Biol Macromol. (2020) 161:258–70. doi: 10.1016/j.ijbiomac.2020.05.180
58. Liu, R, Zhang, L, and You, H. Insulin resistance and impaired branched-chain amino acid metabolism in Alzheimer's disease. J Alzheimers Dis. (2023) 93:847–62. doi: 10.3233/JAD-221147
59. Berry, AS, and Harrison, TM. New perspectives on the basal forebrain cholinergic system in Alzheimer's disease. Neurosci Biobehav Rev. (2023) 150:105192. doi: 10.1016/j.neubiorev.2023.105192
60. Milligan Armstrong, A, Porter, T, Quek, H, White, A, Haynes, J, Jackaman, C, et al. Chronic stress and Alzheimer's disease: the interplay between the hypothalamic-pituitary-adrenal axis, genetics and microglia. Biol Rev Camb Philos Soc. (2021) 96:2209–28. doi: 10.1111/brv.12750
61. Pistollato, F, Sumalla Cano, S, Elio, I, Masias Vergara, M, Giampieri, F, and Battino, M. Role of gut microbiota and nutrients in amyloid formation and pathogenesis of Alzheimer disease. Nutr Rev. (2016) 74:624–34. doi: 10.1093/nutrit/nuw023
62. Varesi, A, Pierella, E, Romeo, M, Piccini, GB, Alfano, C, Bjørklund, G, et al. The potential role of gut microbiota in Alzheimer's disease: from diagnosis to treatment. Nutrients. (2022) 14:668. doi: 10.3390/nu14030668
63. Fu, L, Zhu, W, Tian, D, Tang, Y, Ye, Y, Wei, Q, et al. Dietary supplement of Anoectochilus roxburghii (wall.) Lindl. Polysaccharides ameliorates cognitive dysfunction induced by high fat diet via "gut-brain" Axis. Drug Des Devel Ther. (2022) 16:1931–45. doi: 10.2147/DDDT.S356934
64. Yanckello, LM, Hoffman, JD, Chang, YH, Lin, P, Nehra, G, Chlipala, G, et al. Apolipoprotein E genotype-dependent nutrigenetic effects to prebiotic inulin for modulating systemic metabolism and neuroprotection in mice via gut-brain axis. Nutr Neurosci. (2022) 25:1669–79. doi: 10.1080/1028415X.2021.1889452
65. Nishikawa, M, Brickman, AM, Manly, JJ, Schupf, N, Mayeux, RP, and Gu, Y. Association of dietary prebiotic consumption with reduced risk of Alzheimer's disease in a multiethnic population. Curr Alzheimer Res. (2021) 18:984–92. doi: 10.2174/1567205019666211222115142
66. World Health Organization . Depression and other common mental disorders: global health estimates World Health Organization (2017).
67. Bandelow, B, and Michaelis, S. Epidemiology of anxiety disorders in the 21st century. Dialogues Clin Neurosci. (2015) 17:327–35. doi: 10.31887/DCNS.2015.17.3/bbandelow
68. Coryell, W, Fiedorowicz, JG, Solomon, D, Leon, AC, Rice, JP, and Keller, MB. Effects of anxiety on the long-term course of depressive disorders. Br J Psychiatry. (2012) 200:210–5. doi: 10.1192/bjp.bp.110.081992
70. Ng, F, Berk, M, Dean, O, and Bush, AI. Oxidative stress in psychiatric disorders: evidence base and therapeutic implications. Int J Neuropsychopharmacol. (2008) 11:851–76. doi: 10.1017/S1461145707008401
71. Milton, VJ, and Sweeney, ST. Oxidative stress in synapse development and function. Dev Neurobiol. (2012) 72:100–10. doi: 10.1002/dneu.20957
72. Prada, I, Gabrielli, M, Turola, E, Iorio, A, D'Arrigo, G, Parolisi, R, et al. Glia-to-neuron transfer of miRNAs via extracellular vesicles: a new mechanism underlying inflammation-induced synaptic alterations. Acta Neuropathol. (2018) 135:529–50. doi: 10.1007/s00401-017-1803-x
73. Chen, KZ, Chen, S, Ren, JY, Lin, S, Xiao, MJ, Cheng, L, et al. Antidepressant effect of acidic polysaccharides from Poria and their regulation of neurotransmitters and NLRP3 pathway. Zhongguo Zhong Yao Za Zhi. (2021) 46:5088–95. doi: 10.19540/j.cnki.cjcmm.20210610.705
74. An, L, Yang, JC, Yin, H, Xue, R, Wang, Q, Sun, YC, et al. Inulin-type oligosaccharides extracted from Yacon produce antidepressant-like effects in behavioral models of depression. Phytother Res. (2016) 30:1937–42. doi: 10.1002/ptr.5698
75. Guo, L, Xiao, P, Zhang, X, Yang, Y, Yang, M, Wang, T, et al. Inulin ameliorates schizophrenia via modulation of the gut microbiota and anti-inflammation in mice. Food Funct. (2021) 12:1156–75. doi: 10.1039/D0FO02778B
76. Shen, F, Song, Z, Xie, P, Li, L, Wang, B, Peng, D, et al. Polygonatum sibiricum polysaccharide prevents depression-like behaviors by reducing oxidative stress, inflammation, and cellular and synaptic damage. J Ethnopharmacol. (2021) 275:114164. doi: 10.1016/j.jep.2021.114164
77. Li, H, Xiao, Y, Han, L, Jia, Y, Luo, S, Zhang, D, et al. Ganoderma lucidum polysaccharides ameliorated depression-like behaviors in the chronic social defeat stress depression model via modulation of Dectin-1 and the innate immune system. Brain Res Bull. (2021) 171:16–24. doi: 10.1016/j.brainresbull.2021.03.002
78. Yan, T, Nian, T, Liao, Z, Xiao, F, Wu, B, Bi, K, et al. Antidepressant effects of a polysaccharide from okra (Abelmoschus esculentus (L) Moench) by anti-inflammation and rebalancing the gut microbiota. Int J Biol Macromol. (2020) 144:427–40. doi: 10.1016/j.ijbiomac.2019.12.138
79. Po, KK, Leung, JW, Chan, JN, Fung, TK, Sánchez-Vidaña, DI, Sin, EL, et al. Protective effect of Lycium Barbarum polysaccharides on dextromethorphan-induced mood impairment and neurogenesis suppression. Brain Res Bull. (2017) 134:10–7. doi: 10.1016/j.brainresbull.2017.06.014
80. Menke, A . Is the HPA Axis as target for depression outdated, or is there a new Hope? Front Psych. (2019) 10:101. doi: 10.3389/fpsyt.2019.00101
81. Peng, GJ, Tian, JS, Gao, XX, Zhou, YZ, and Qin, XM. Research on the pathological mechanism and drug treatment mechanism of depression. Curr Neuropharmacol. (2015) 13:514–23. doi: 10.2174/1570159X1304150831120428
82. Boyer, P . Do anxiety and depression have a common pathophysiological mechanism? Acta Psychiatr Scand Suppl. (2000) 102:24–9. doi: 10.1111/j.0065-1591.2000.acp29[dash]04.x
83. Kim, YK, Na, KS, Myint, AM, and Leonard, BE. The role of pro-inflammatory cytokines in neuroinflammation, neurogenesis and the neuroendocrine system in major depression. Prog Neuro-Psychopharmacol Biol Psychiatry. (2016) 64:277–84. doi: 10.1016/j.pnpbp.2015.06.008
84. Gotlib, IH, Joormann, J, Minor, KL, and Hallmayer, J. HPA axis reactivity: a mechanism underlying the associations among 5-HTTLPR, stress, and depression. Biol Psychiatry. (2008) 63:847–51. doi: 10.1016/j.biopsych.2007.10.008
85. Chen, Y, Wan, M, Zhong, Y, Gao, T, Zhang, Y, Yan, F, et al. Partially hydrolyzed guar gum modulates gut microbiota, regulates the levels of neurotransmitters, and prevents CUMS-induced depressive-like behavior in mice. Mol Nutr Food Res. (2021) 65:e2100146. doi: 10.1002/mnfr.202100146
86. Zhou, XD, Zheng, Y, Sharma, R, and Zhang, ZJ. Total polysaccharides of lily bulb ameliorate menopause-like behavior in Ovariectomized mice: multiple mechanisms distinct from estrogen therapy. Oxidative Med Cell Longev. (2019) 2019:1–27. doi: 10.1155/2019/6869350
87. Chen, P, Hei, M, Kong, L, Liu, Y, Yang, Y, Mu, H, et al. One water-soluble polysaccharide from Ginkgo biloba leaves with antidepressant activities via modulation of the gut microbiome. Food Funct. (2019) 10:8161–71. doi: 10.1039/C9FO01178A
88. Simpson, CA, Diaz-Arteche, C, Eliby, D, Schwartz, OS, Simmons, JG, and Cowan, CSM. The gut microbiota in anxiety and depression – a systematic review. Clin Psychol Rev. (2021) 83:101943. doi: 10.1016/j.cpr.2020.101943
89. Berding, K, Long-Smith, CM, Carbia, C, Bastiaanssen, TFS, van de Wouw, M, Wiley, N, et al. A specific dietary fibre supplementation improves cognitive performance-an exploratory randomised, placebo-controlled, crossover study. Psychopharmacology. (2021) 238:149–63. doi: 10.1007/s00213-020-05665-y
90. Tarr, AJ, Galley, JD, Fisher, SE, Chichlowski, M, Berg, BM, and Bailey, MT. The prebiotics 3'Sialyllactose and 6'Sialyllactose diminish stressor-induced anxiety-like behavior and colonic microbiota alterations: evidence for effects on the gut-brain axis. Brain Behav Immun. (2015) 50:166–77. doi: 10.1016/j.bbi.2015.06.025
91. Lai, MC, Lombardo, MV, and Baron-Cohen, S. Autism. Lancet. (2014) 383:896–910. doi: 10.1016/S0140-6736(13)61539-1
92. Chiarotti, F, and Venerosi, A. Epidemiology of autism Spectrum disorders: a review of worldwide prevalence estimates since 2014. Brain Sci. (2020) 10:274. doi: 10.3390/brainsci10050274
93. Hughes, HK, Rose, D, and Ashwood, P. The gut microbiota and Dysbiosis in autism spectrum disorders. Curr Neurol Neurosci Rep. (2018) 18:81. doi: 10.1007/s11910-018-0887-6
94. Anagnostou, E, and Hansen, R. Medical treatment overview: traditional and novel psycho-pharmacological and complementary and alternative medications. Curr Opin Pediatr. (2011) 23:621–7. doi: 10.1097/MOP.0b013e32834cba3e
95. Inoue, R, Sakaue, Y, Kawada, Y, Tamaki, R, Yasukawa, Z, Ozeki, M, et al. Dietary supplementation with partially hydrolyzed guar gum helps improve constipation and gut dysbiosis symptoms and behavioral irritability in children with autism spectrum disorder. J Clin Biochem Nutr. (2019) 64:217–23. doi: 10.3164/jcbn.18-105
96. World Health Organization . (2022). Epilepsy. Available at: https://www.who.int/news-room/fact-sheets/detail/epilepsy.
97. Buainain, RP, Oliveira, CTP, Marson, FAL, and Ortega, MM. Epidemiologic profile of patients with epilepsy in a region of Southeast Brazil: data from a referral center. Front Neurol. (2022) 13:822537. doi: 10.3389/fneur.2022.822537
98. Li, L, Li, J, Wu, X, Zhu, Y, and Lei, D. Considering economic reality in calculating the financial burden of epilepsy in China. Epilepsia. (2011) 52:416–8. doi: 10.1111/j.1528-1167.2010.02913.x
99. Baram, TZ . The brain, seizures and epilepsy throughout life: understanding a moving target. Epilepsy Curr. (2012) 12:7–12. doi: 10.5698/1535-7511-12.4s.7
100. Maggi, L, Bonanno, S, Altamura, C, and Desaphy, JF. Ion channel gene mutations causing skeletal muscle disorders: pathomechanisms and opportunities for therapy. Cells. (2021) 10:1521. doi: 10.3390/cells10061521
101. Nairn, AC, and Picciotto, MR. Calcium/calmodulin-dependent protein kinases. Semin Cancer Biol. (1994) 5:295–303.
102. Xu, JH, and Tang, FR. Voltage-dependent calcium channels, calcium binding proteins, and their interaction in the pathological process of epilepsy. Int J Mol Sci. (2018) 19:2735. doi: 10.3390/ijms19092735
103. Wang, SQ, Li, XJ, Qiu, HB, Jiang, ZM, Simon, M, Ma, XR, et al. Anti-epileptic effect of Ganoderma lucidum polysaccharides by inhibition of intracellular calcium accumulation and stimulation of expression of CaMKII α in epileptic hippocampal neurons. PLoS One. (2014) 9:e102161. doi: 10.1371/journal.pone.0102161
104. van Vliet, EA, Aronica, E, Vezzani, A, and Ravizza, T. Review: neuroinflammatory pathways as treatment targets and biomarker candidates in epilepsy: emerging evidence from preclinical and clinical studies. Neuropathol Appl Neurobiol. (2018) 44:91–111. doi: 10.1111/nan.12444
105. Singh, S, Singh, TG, Singh, M, Najda, A, Nurzyńska-Wierdak, R, Almeer, R, et al. Anticonvulsive effects of chondroitin sulfate on pilocarpine and Pentylenetetrazole induced Epileptogenesis in mice. Molecules. (2021) 26:6773. doi: 10.3390/molecules26226773
106. Sun, X, Kong, L, and Zhou, L. Protective effect of Fructus corni polysaccharide on hippocampal tissues and its relevant mechanism in epileptic rats induced by lithium chloride-pilocarpine. Exp Ther Med. (2018) 16:445–51. doi: 10.3892/etm.2018.6142
107. Zhou, Y, Guo, X, Chen, W, and Liu, J. Angelica polysaccharide mitigates lipopolysaccharide-evoked inflammatory injury by regulating microRNA-10a in neuronal cell line HT22. Artif Cells Nanomed Biotechnol. (2019) 47:3194–201. doi: 10.1080/21691401.2019.1614595
108. Wang, CJ, Gu, HQ, Zhang, XM, Jiang, Y, Li, H, Bettger, JP, et al. Temporal trends and rural-urban disparities in cerebrovascular risk factors, in-hospital management and outcomes in ischaemic strokes in China from 2005 to 2015: a nationwide serial cross-sectional survey. Stroke Vasc Neurol. (2023) 8:34–50. doi: 10.1136/svn-2022-001552
109. Magid-Bernstein, J, Girard, R, Polster, S, Srinath, A, Romanos, S, Awad, IA, et al. Cerebral hemorrhage: pathophysiology, treatment, and future directions. Circ Res. (2022) 130:1204–29. doi: 10.1161/CIRCRESAHA.121.319949
110. Liu, Y, Wang, L, Yang, G, Chi, X, Liang, X, and Zhang, Y. Sirtuins: promising therapeutic targets to treat ischemic stroke. Biomol Ther. (2023) 13:1210. doi: 10.3390/biom13081210
111. Feigin, VL, Brainin, M, Norrving, B, Martins, S, Sacco, RL, Hacke, W, et al. World stroke organization (WSO): global stroke fact sheet 2022. Int J Stroke. (2022) 17:18–29. doi: 10.1177/17474930211065917
112. Ma, LY, Wang, XD, Liu, S, Gan, J, Hu, W, Chen, Z, et al. Prevalence and risk factors of stroke in a rural area of northern China: a 10-year comparative study. Aging Clin Exp Res. (2022) 34:1055–63. doi: 10.1007/s40520-021-02028-1
113. GBD 2015 Mortality and Causes of Death Collaborators . Global, regional, and national life expectancy, all-cause mortality, and cause-specific mortality for 249 causes of death, 1980–2015: a systematic analysis for the global burden of disease study 2015. Lancet. (2016) 388:1459–544. doi: 10.1016/S0140-6736(16)31012-1
114. Shi, Z, Zhu, L, Li, T, Tang, X, Xiang, Y, Han, X, et al. Neuroprotective mechanisms of Lycium barbarum polysaccharides against ischemic insults by regulating NR2B and NR2A containing NMDA receptor signaling pathways. Front Cell Neurosci. (2017) 11:288. doi: 10.3389/fncel.2017.00288
115. Gong, J, Sun, F, Li, Y, Zhou, X, Duan, Z, Duan, F, et al. Momordica charantia polysaccharides could protect against cerebral ischemia/reperfusion injury through inhibiting oxidative stress mediated c-Jun N-terminal kinase 3 signaling pathway. Neuropharmacology. (2015) 91:123–34. doi: 10.1016/j.neuropharm.2014.11.020
116. Yang, Y, Liu, P, Chen, L, Liu, Z, Zhang, H, Wang, J, et al. Therapeutic effect of Ginkgo biloba polysaccharide in rats with focal cerebral ischemia/reperfusion (I/R) injury. Carbohydr Polym. (2013) 98:1383–8. doi: 10.1016/j.carbpol.2013.07.045
117. Shannon, RS, Minakshi, N, and Nihal, A. Dose translation from animal to human studies revisited. FASEB J. (2008) 22:659–61. doi: 10.1096/fj.07-9574LSF
Glossary
Keywords: dietary polysaccharides, neuroprotection, central nervous system disorders, antioxidant, anti-inflammatory, gut microbiota, gut-brain axis
Citation: Guo R, Pang J, Zhao J, Xiao X, Li J, Li J, Wang W, Zhou S, Zhao Y, Zhang Z, Chen H, Yuan T, Wu S and Liu Z (2023) Unveiling the neuroprotective potential of dietary polysaccharides: a systematic review. Front. Nutr. 10:1299117. doi: 10.3389/fnut.2023.1299117
Edited by:
Bin Du, Hebei Normal University of Science and Technology, ChinaReviewed by:
Xin Liu, Xi’an Jiaotong University Health Science Center, ChinaJielun Hu, Nanchang University, China
Hong Chen, Sichuan Agricultural University, China
Copyright © 2023 Guo, Pang, Zhao, Xiao, Li, Li, Wang, Zhou, Zhao, Zhang, Chen, Yuan, Wu and Liu. This is an open-access article distributed under the terms of the Creative Commons Attribution License (CC BY). The use, distribution or reproduction in other forums is permitted, provided the original author(s) and the copyright owner(s) are credited and that the original publication in this journal is cited, in accordance with accepted academic practice. No use, distribution or reproduction is permitted which does not comply with these terms.
*Correspondence: Zhigang Liu, zhigangliu@nwsuaf.edu.cn; Shan Wu, shanwu331314@hotmail.com
†These authors have contributed equally to this work