- 1School of Public Health, Shandong First Medical University and Shandong Academy of Medical Sciences, Ji’nan, Shandong, China
- 2State Key Laboratory of Food Nutrition and Safety, Tianjin University of Science and Technology, Tianjin, China
- 3School of Food Engineering and Biotechnology, Tianjin University of Science and Technology, Tianjin, China
The effects of the heating conditions and water content on the structure and digestibility of wheat starch (WS)-glycerol monopalmitin (GMP) complexes were investigated. The results showed that the higher water content and the heating conditions of 90°C for 60 min after 100°C for 10 min favor the formation of more WS-GMP complexes with the greater short-range order, although the thermal transition temperatures of GWS-GMP-100 complexes are not significantly affected by the water content. Only the type I complexes were formed under the heating conditions of 90°C for 60 min. The heating conditions of 90°C for 60 min after 100°C for 10 min facilitates the formation of type II complexes, and the amounts of type II complexes increased with increasing water content. The digestion rates of WS-GMP complexes decreased slightly with increasing water content, and the extent of starch amylolysis of WS-GMP complexes significantly decreased after heating further at 90°C compared with that only heating at 100°C. The digestibility of complexes is mainly related to structural order rather than the number of complexes. This study is helpful to further understand starch-lipid complexes by showing that heating conditions and water content influence the formation of WS-GMP complexes.
1 Introduction
Starch is the main component of green plants and provides most of the energy needed for people’s daily life. It is composed of two glucose polymers: amylopectin (AP), which has highly branched molecules, and amylose (AM), a linear polymer with a few branches (1–3). Lipids are added to food to enhance the flavor, mouth-feel and nutritive value during processing (4). Given the structural characteristics of AM with helical cavity, the hydrocarbon portion of guest molecules [e.g., fatty acids (FAs), monoglycerides (MGs)] can insert into this hydrophobic cavity to form AM-lipids complexes through hydrogen bonds and hydrophobic interaction (5–10). However, only a few AP can form complexes due to the larger steric hindrance of AP with more and shorter branch chain (1, 4, 11). During food processing, the interactions of AM and lipids can affect quality of finished food, such as texture and digestibility (12). Therefore, starch-lipids complexes have been a topic of wide concern.
There are many factors that can affect the formation of starch-lipid complexes, such as the type of starch and lipid, carbon chain lengths and the unsaturation degree of the lipids, amylose content and the polymerization degree of starch, reaction conditions, and methods of preparation (13, 14). Hydrothermal treatment is the most commonly used method to prepare starch-lipid complexes because most starchy foods need to be cooked with a certain amount of water (15). During this process, heating conditions (heating time and temperature) and moisture content can affect the formation of starch-lipid complexes. As increased water content, the amount of type II starch-lipid complexes with more ordered structure increased (16). However, the opposite results were observed in almond flour during extrusion cooking and rice with parboiling (17, 18). The difference in these results suggests that how does the water content affect complexes formation has not been elucidated clearly.
Glycerol monopalmitin (GMP), a linear molecule synthesized by esterification of palmitic acid and glycerol. In previous studies on the effects of temperature treatment (19), hydrophilic groups (20), different types of lipids (21), and different chain length of monoglycerides (22) on complex formation, the results showed that GMP can form complexes with starch and it has a greater emulsifying action and compounding ability with AM (21). Wheat starch is the main ingredient in staple of the daily diet (such as bread, noodles, biscuits, etc.), and GMP is added as an emulsifier in the processing of these foods to improve the food quality. The addition of GMP can interact with WS, and factors such as moisture content and heating conditions during processing can affect this interaction, which in turn affects the quality and functional properties of food. Therefore, GMP and WS were selected as representative of lipid and starch, respectively. In this study, we prepared wheat starch (WS)-glycerol monopalmitin (GMP) complexes through the control of water content under two different heating conditions. In one condition, WS and GMP were heated at 100°C for 10 min, and in the other condition, they were heated at 100°C for 10 min followed by 90°C for 60 min. We speculated that heating conditions and water content may influence the interaction and complexes formation between WS and GMP.
Hence, the effects of the heating conditions and water content on the formation, structure, and digestibility of WS-GMP complexes were investigated in this study. A better understanding of this topic will be helpful to understand deeply on the effect of the heating conditions and water content on the formation of WS-GMP complexes and modulate the functionality and nutrition of starchy food.
2 Materials and methods
2.1 Materials
Wheat starch (9.0% moisture content, 27.5% amylose content, and 0.18% lipid content), glycerol monopalmitin (GMP, C16:0) and α-amylase (Sigma, EC 3.2.1.1, type VI-B from porcine pancreas, 13 units/mg) were obtained from Sigma Chemical Co. (St. Louis, MO, USA). The Glucose Oxidase/Peroxidase Kit (GOPOD format) and Aspergillus niger amyloglucosidase (3260 units/mL) were purchased from Megazyme International Ireland, Ltd. (Bray Co., Wicklow, Ireland). All other chemical reagents were of analytical grade.
2.2 Preparation of starch-lipid complexes
Native wheat starch (WS, 2.0 g, wet weigh basis) was weighed and added distilled water to obtain starch suspensions with 50, 60, 70, 80, and 90% moisture contents (w/w, wet starch basis). Subsequently, 100 mg GMP was added and mixed thoroughly under magnetic stirring. And the partial mixtures were heated at 100°C for 10 min (designated WS (m%)-GMP-100), whereas other the mixtures of WS and GMP were firstly heated at 100°C for 10 min and then heated at 90°C for 60 min (WS (n%)-GMP-100-90). All above obtained samples were freeze-dried and ground into powders.
2.3 Differential scanning calorimetry (DSC)
Thermal properties of the WS-GMP complexes were examined using a differential scanning calorimeter (200 F3, Netzsch, Germany) according to the method described in the previous study with minor modification (23). Differently, samples were heated from 20 to 130°C at a heating rate of 10°C/min. The onset (To), peak (Tp), conclusion (Tc) temperatures, and gelatinization enthalpy change (ΔH) were obtained through data recording software.
2.4 X-ray diffraction analysis (XRD)
The crystalline structure of WS-GMP complexes was determined using a Bruker D8 Advance X-ray diffractometer (Bruker, Germany) operating at 40 kV and 40 mA. Samples were equilibrated over a saturated NaCl solution for 1 week before measurement. XRD patterns were obtained from 5 to 35° (2θ) at a rate of 2°/min and a step size of 0.06° (24).
2.5 Laser confocal micro-Raman spectroscopy
Renishaw Invia Raman microscope system (Renishaw, Gloucestershire, UK) equipped with a Leica microscope (Leica Biosystems, Wetzlar, Germany) was used to collect spectra in the range of 3200–100 cm–1 with a resolution of approximately 7 cm–1. The full width at half maximum of the band at 480 cm–1 (FWHM480) was calculated to characterize the short-range molecular order of WS-GMP complexes by the WIRE 2.0 software (21).
2.6 In vitro enzymatic digestion
In vitro enzymatic digestibility of WS-GMP complexes was determined according to the procedure described elsewhere (25). Differently, the WS-GMP complexes were incubated 4 h and determined the glucose content. The digestion curve was drawn with the release of glucose as a function of digestion time. The first-order rate coefficient (k) was fitted, and the content of rapidly digested starch (RDS, digested within 20 min), slowly digested starch (SDS, digested between 20 and 120 min) and resistant starch (RS, undigested after 120 min) were calculated based on the digestion curve.
2.7 Statistical analysis
In addition to XRD, all characterizations performed triplicate measurements, and the results in tables are reported as the mean values and standard deviations (SDs) obtained by the SPSS 17.0 Statistical Software Program (SPSS, Inc. Chicago, IL, USA). Pearson’s correlation analysis was also conducted by the SPSS 17.0 statistical software.
3 Results and discussion
3.1 Thermal properties of complex samples
Figure 1 shows the DSC thermograms of WS-GMP complexes. All WS-GMP-100 complex samples exhibit two endothermic peaks. The peak at 50–65°C attributed to the melting of uncomplexed GMP and the second at 85–103°C is the typical thermal transitions peak of the WS-GMP-100 complexes. Previous studies have reported that starch-lipid complexes exist in two distinct crystalline forms depending on crystallization temperature. Type I complexes consisting of non-crystalline helical segments and crystalline V-type packing has a lower thermal transition temperature between 95 and 105°C, and type II with distinct crystalline structures and amorphous regions has a higher melting temperature (14, 26). Different from WS-GMP-100 complex samples, WS-GMP-100-90 complex samples showed different thermal transitions according to the water content. WS (50%)-GMP-100-90 showed two endothermic transitions, which respectively represent the melting of uncomplexed GMP (around 53–60°C) and type I complexes (around 90–103°C). However, three endothermic transitions, related to the melting of uncomplexed GMP, type I complexes and type II complexes (around 110–120°C) were observed for other WS-GMP-100-90 complexes. These results suggest that the lower water content was more readily to form less stable type I WS-GMP-100-90 complexes, whereas the higher water content tended to form type I and type II WS-GMP-100-90 complexes. No type II complexes were found in WS-GMP-100 complexes even under conditions of higher moisture content, which may be due to the fact that the shorter heating time and the heating temperature of 100°C do not allow stable type II complexes to form.
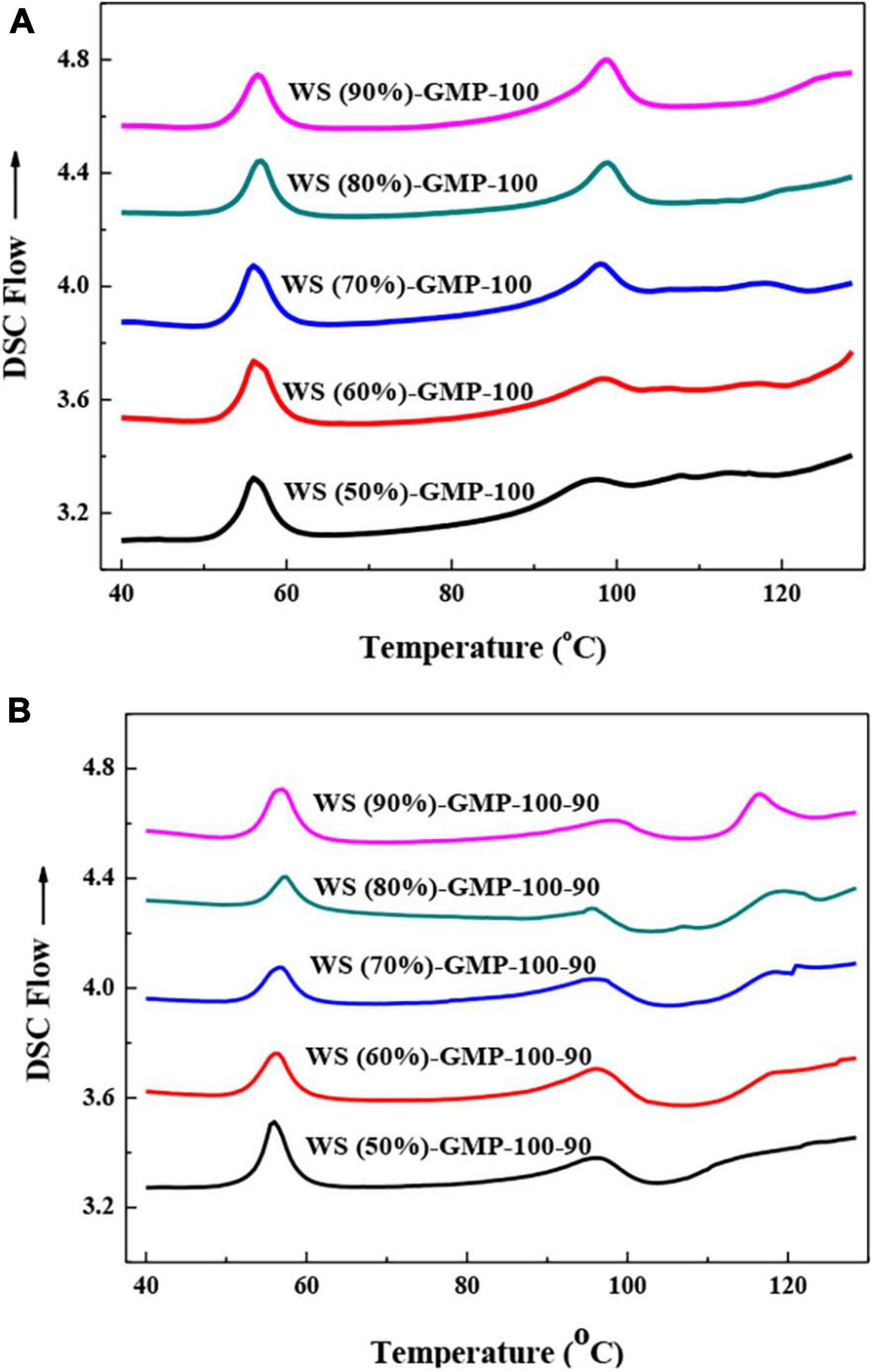
Figure 1. Differential scanning calorimetry (DSC) curves of WS-GMP complexes. (A) WS-GMP complexes were prepared at 100°C for 10 min. (B) WS-GMP complexes were prepared at 100°C for 10 min followed by complexing at 90°C for 60 min. WS (no/o), wheat starch with no/o water content; GMP, glycerol monopalmitin.
The thermal transition temperatures (To, Tp, Tc) and enthalpy change (ΔH) of WS-GMP complexes are presented in Table 1. The ΔH value can reflect the amounts of complexes (27). The total enthalpy change (ΔHt) of WS-GMP complexes prepared under two heating conditions increased with the increasing water content, for example, from 2.6 J/g for WS (50%)-GMP-100 to 5.3 J/g for WS (90%)-GMP-100, suggests that the complex formation during heating can be controlled by the water content, and the higher water content was more favorable for the formation of WS-GMP complexes. A previous study found that as the water content increased from 6 to 40%, the amount of complex formation increased, and as the water content further increased from 40 to 60%, the amount of complex decreased (28). The different results with this study may be related to processing methods and lipid types. The results of this study are due to that the better starch chain dispersibility of the WS with higher water content makes the GMP insert easily into the hydrophobic cavity of AM to form WS-GMP complexes (29). But thermal transition temperatures of WS-GMP-100 complexes seemed to be little affected by the water content. WS-GMP-100-90 complexes have higher ΔHt than the corresponding WS-GMP-100 complexes, indicating that the complexes were formed further after heating further at 90°C for 60 min. A previous study found that the heating conditions of 90°C for longer exhibited a decrease in the amount for type I polymorphs and an increase in type II polymorphs with increased thermal stability (10). The results of this study are attributed to the longer heating time and the slow nucleation of the complexes due to the further heating treatment at 90°C. More than that, the amounts of type II complexes were gradually increased with increasing water content for WS-GMP-100-90 complexes. More specifically, WS (50%)-GMP-100-90 had a ΔH1 value of 3.3 J/g from only type I complexes. As the water content increased, the ΔH1 values of type I complexes decreased from 4.6 to 3.3 J/g and the ΔH2 values of type II complexes increased from 0.9 to 3.7 J/g. At the condition of lower water content, dispersed starch chains in gelatinized starch interact strongly with each other, resulting in less availability of these chains for lipids to complex. Due to the limited space in the condition of the lower water content, the formed complexes were not ready to arrange into stable V-type crystalline structure. However, starch chains with the condition of higher water content are more dispersed and available for the lipids to complex, and the formed complexes are arranged more easily into stable type II complexes.
3.2 X-ray diffraction
Two stronger peaks at 12.9 and 19.8° (2θ) and one weak peak at 7.5° (2θ), representing V-type crystallites were showed in the X-ray diffraction patterns of WS-GMP complexes (Figures 2A, B). The XRD peaks with broad and flat reflections of complexes were proposed to represent the formation of type I complexes, differently, type II complexes have the narrow and sharp V-type peaks (30, 31). The obvious broad and flat peaks at 12.9 and 19.8° (2θ) of WS-GMP-100 complexes indicated the formation of type I complexes. The changes of broad-and-blunt into sharp-and-narrow XRD peaks of WS-GMP-100-90 complexes with increasing the water content indicate the transformation of less stable type I complexes into stable type II complexes. These results are consistent with the DSC results. Compared to WS-GMP-100 complexes, the WS-GMP-100-90 complexes showed shaper V-type diffraction peaks, suggesting the formation of more ordered complexes after heating further at 90°C.
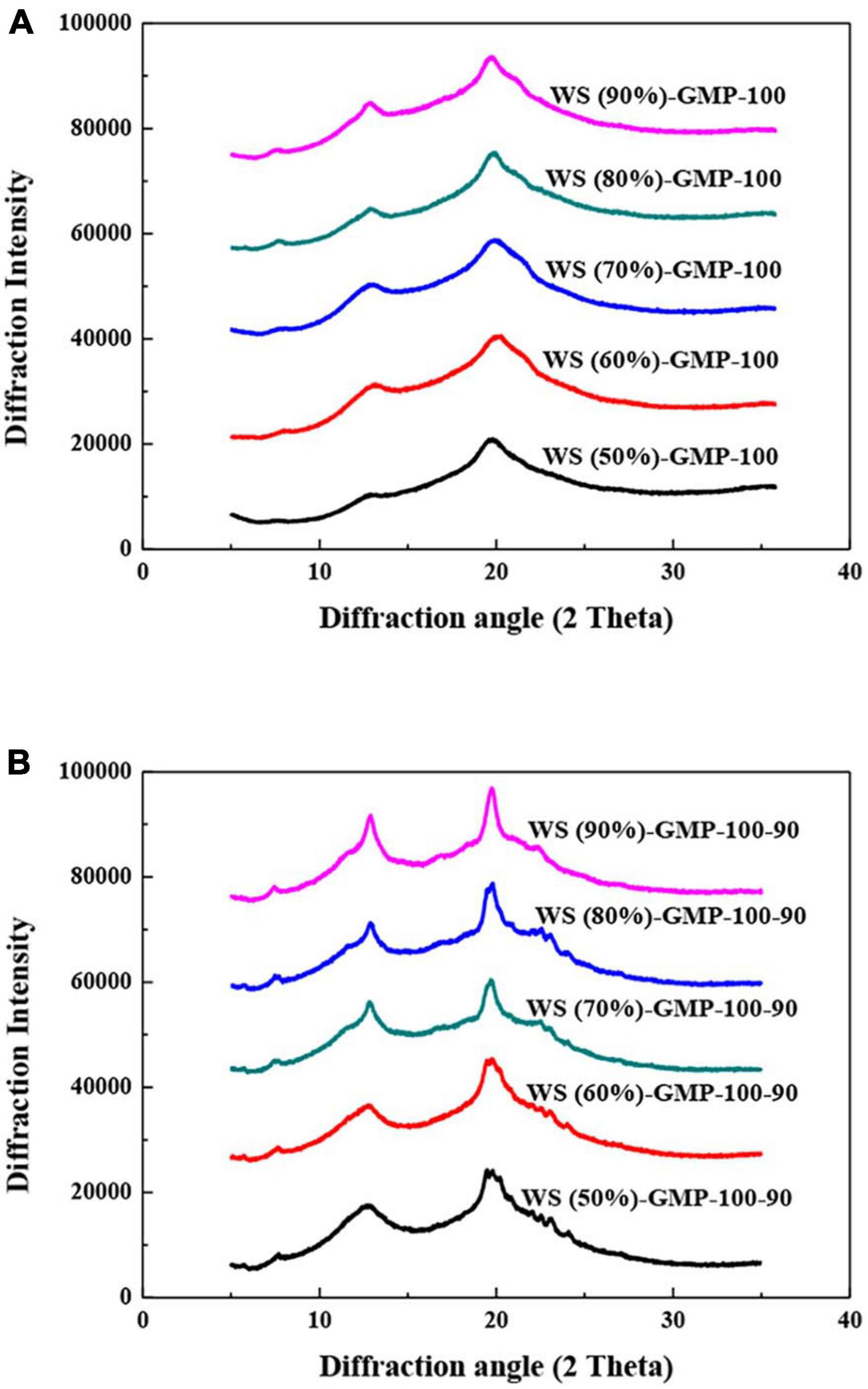
Figure 2. X-ray diffraction analysis (XRD) curves of WS-GMP complexes. (A) WS-GMP complexes were prepared at 100°C for 10 min. (B) WS-GMP complexes were prepared at 100°C for 10 min followed by complexing at 90°C for 60 min. WS (n%), wheat starch with n% water content; GMP, glycerol monopalmitin.
3.3 LCM-Raman spectroscopy
The short-range order of WS-GMP complexes was characterized by Raman spectra (Figures 3A, B). The lower FWHM480 means the better short-range ordered structure, that is to say, the more amount of ordered structure due to the formation of complexes (14). The value FWHM480 decreased from 16.67 for WS (50%)-GMP-100 to 15.34 for WS (90%)-GMP-100, and from 15.69 for WS (50%)-GMP-100-90 to 14.29 for WS (90%)-GMP-100-90 (Table 2), suggesting that the short-range order of complexes increased with increasing water content, which is generally consistent with XRD and DSC results. The FWHM480 of the WS-GMP-100-90 complexes samples is lower than that of the corresponding WS-GMP-100 complexes samples, suggesting that the short-range order of the complexes further increased after heating at 90°C.
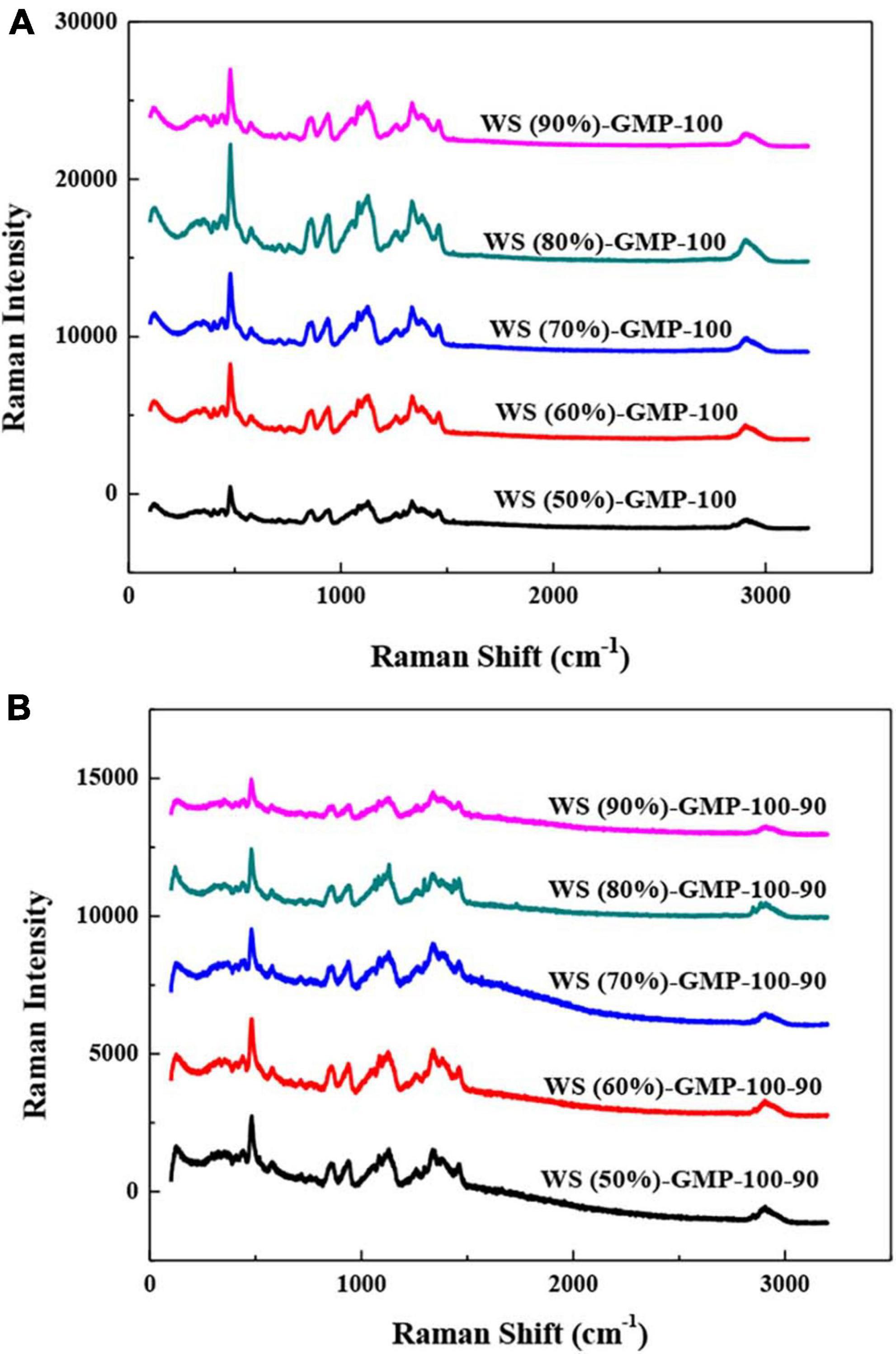
Figure 3. Laser confocal micro-Raman spectra of WS-GMP complexes. (A) WS-GMP complexes were prepared at 100°C for 10 min. (B) WS-GMP complexes were prepared at 100°C for 10 min followed by complexing at 90°C for 60 min. WS (n%), wheat starch with n% water content; GMP, glycerol monopalmitin.
3.4 In vitro digestibility of WS-GMP complexes
The digestograms of the WS-GMP complexes are presented in Figures 4A, B. All WS-GMP complexes reached a plateau after rapid digestion in the initial 80 min and the digestion percentage after 2 h was around 85 and 75%, respectively, for WS-GMP-100 and WS-GMP-100-90 complexes. The values of k decreased slightly with increasing water content for the WS-GMP complexes, and the k-value of the WS-GMP-100-90 complexes and the counterpart WS-GMP-100 complexes had little difference, for example, the k-values are 0.022 and 0.021 for the WS (90%)-GMP-100 complexes and WS (90%)-GMP-100-90 complexes, respectively. These results indicated that the final extent of starch amylolysis decreased after heating further at 90°C, but the digestion rate was little affected by the water content and the heating condition.
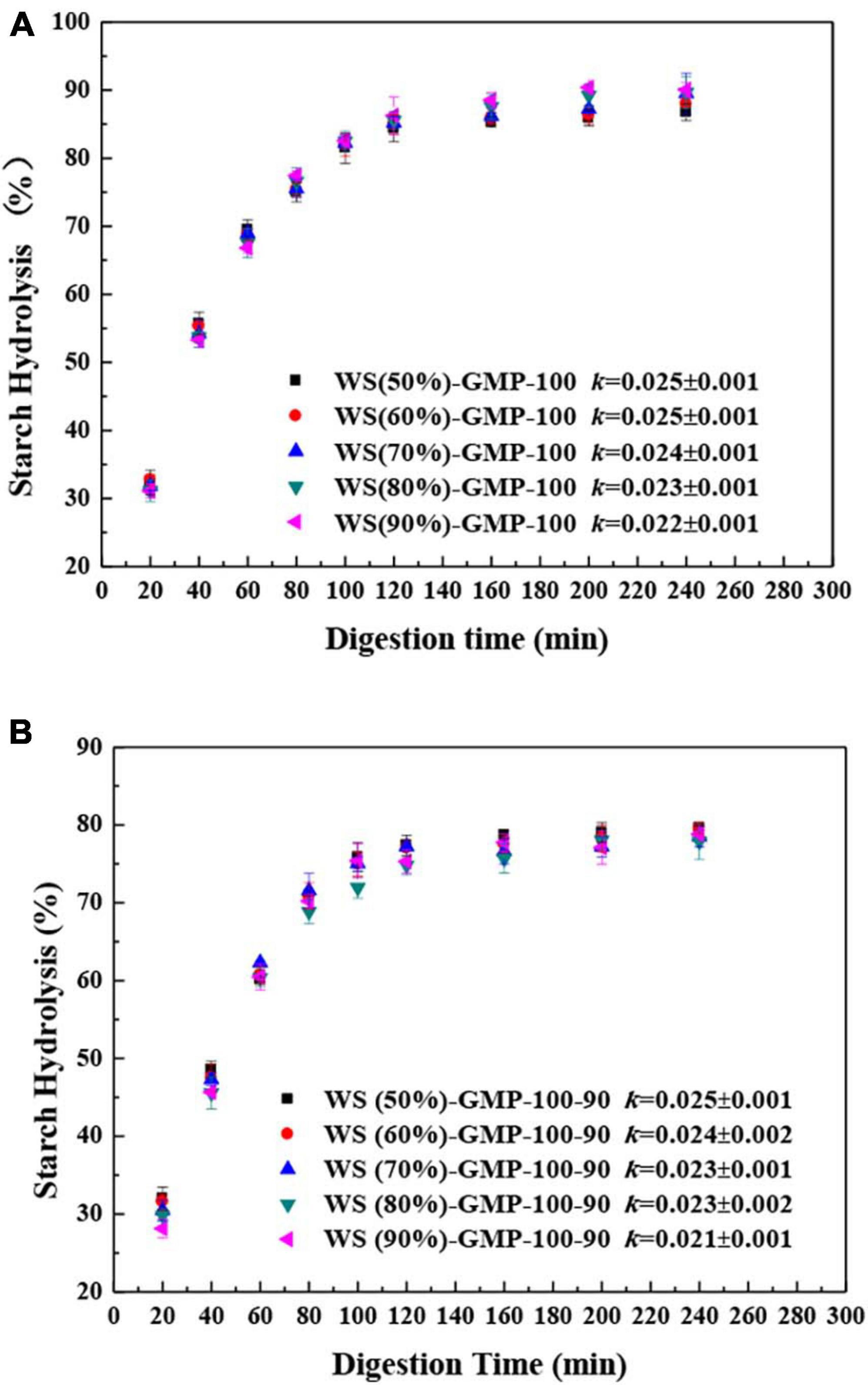
Figure 4. In vitro starch digestion of WS-GMP complexes. (A) WS-GMP complexes were prepared at 100°C for 10 min. (B) WS-GMP complexes were prepared at 100 °C for 10 min followed by complexing at 90°C for 60 min. WS (n%), wheat starch with n% water content; GMP, glycerol monopalmitin.
For further analysis of the digestibility of complexes, the RDS, SDS, and RS content were calculated (Table 3). They were little affected by the water content for the WS-GMP-100 complexes and WS-GMP-100-90 complexes. However, comparing with WS-GMP-100 complexes, WS-GMP-100-90 complex has lower RDS and SDS content and higher RS content. According to DSC and XRD results, the most obvious difference between the complexes obtained under two different processing conditions is the formation of type I and type II complexes with different structural stability. WS-GMP-100-90 complexes have more ordered type II complexes, resulting in obvious differences in RS, SDS, and RDS content between the WS-GMP-100 complexes and WS-GMP-100-90 complexes. The most obvious thing is that different amounts of complexes are obtained under the condition of different water content. Therefore, the formation of different crystal types complexes with different structural stability, rather than the number of complexes, have a more significant impact on the digestion of WS-GMP complexes.
3.5 The correlation between the structures and digestibility of complexes
Many studies have demonstrated that the formation and structure of starch-lipid complex are important factors in determining the resistance of starch to enzymatic hydrolysis (32, 33). In general, complexes with the greater structural order have the lower digestibility. In order to further analyze the relationships between the structures of WS-GMP complexes and in vitro starch digestibility, Pearson correlation analyses were performed (Table 4). Results showed that the total enthalpy changes (ΔHt) of WS-GMP complexes were negatively correlated with the RDS (r = −0.883, p < 0.01) and k-value (r = −0.845, p < 0.01), and positively correlated with the RS (r = 0.700, p < 0.05). Previous studies observed a strong positive correlation between enthalpy and RS contents (32). The FWHM480 of WS-GMP complexes positively correlated with the RDS (r = 0.900, p < 0.01) and k value (r = 0.800, p < 0.01), but negatively correlated with the RS (r = −0.807, p < 0.01). Compared with the correlation coefficient between RS/RDS and ΔHt, RS/RDS had stronger correlation with FWHM480. This result indicated that short-range molecular order plays a more significant role in the content of RS and RDS. Similar results of a stronger positive correlation between the short-range ordering and RS contents were found in previous studies (32, 34). The above results of correlation analyses also suggested that the decreased digestion of WS-GMP complexes is more related to the increasing ordered structure.
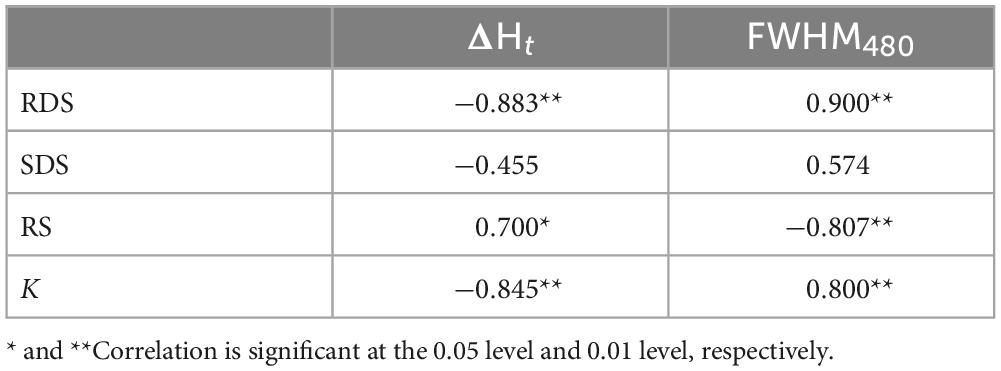
Table 4. Pearson’s correlation analysis between ΔHt, FWHM480 and digestion parameters of WS-GMP complexes.
4 Conclusion
The water content and heating conditions can affect the formation of starch-lipid complexes. The higher water content and the heating conditions of 90°C for 60 min after 100°C for 10 min are helpful for the formation of more complexes with the better short-range order. The heating conditions of 90°C for 60 min after 100°C for 10 min facilitates the formation of type II complexes, and the amounts of type II complexes increased with increasing water content. The digestion rate of WS-GMP complexes decreased slightly with increasing water content, however, the extent of starch digestion decreased significantly after further heating at 90°C for 60 min. The digestion difference of WS-GMP complexes are mainly determined by the structure order caused by different heating conditions rather than the number of complexes. This study provided new insights into the formation of starch-lipid complexes, which is of great significance for modulation of the functional and nutritive properties of starchy food.
Data availability statement
The original contributions presented in the study are included in the article/supplementary material, further inquiries can be directed to the corresponding author.
Author contributions
FJ: Data curation, Formal analysis, Investigation, Writing – original draft. ZL: Data curation, Formal analysis, Investigation, Writing – original draft. JN: Writing – review and editing. JY: Writing – review and editing. SW: Validation, Writing – review and editing. XL: Conceptualization, Formal analysis, Funding acquisition, Supervision, Writing – review and editing.
Funding
The author(s) declare financial support was received for the research, authorship, and/or publication of this article. This study was supported by the Shandong Provincial Natural Science Foundation (ZR2022QC015), Tai’an Science and Technology Innovation Development Project (Policy guidance, 2021GX006).
Acknowledgments
We thank the participants for their contribution to this study.
Conflict of interest
The authors declare that the research was conducted in the absence of any commercial or financial relationships that could be construed as a potential conflict of interest.
Publisher’s note
All claims expressed in this article are solely those of the authors and do not necessarily represent those of their affiliated organizations, or those of the publisher, the editors and the reviewers. Any product that may be evaluated in this article, or claim that may be made by its manufacturer, is not guaranteed or endorsed by the publisher.
References
1. Copeland L, Blazek J, Salman H, Tang MC. Form and functionality of starch. Food Hydrocolloids. (2009) 23:1527–34. doi: 10.1016/j.foodhyd.2008.09.016
2. Pérez S, Bertoft E. The molecular structures of starch components and their contribution to the architecture of starch granules: a comprehensive review. Starch. (2010) 62:389–420. doi: 10.1002/star.201000013
3. Wang S, Copeland L. Molecular disassembly of starch granules during gelatinization and its effect on starch digestibility: a review. Food Funct. (2013) 4:1564–80. doi: 10.1039/c3fo60258c
4. Wang S, Wang J, Yu J, Wang S. Effect of fatty acids on functional properties of normal wheat and waxy wheat starches: A structural basis. Food Chem. (2016) 190:285–92. doi: 10.1016/j.foodchem.2015.05.086
5. Garcia MC, Pereira-da-Silva MA, Taboga S, Franco CM. Structural characterization of complexes prepared with glycerol monoestearate and maize starches with different amylose contents. Carbohydr Polym. (2016) 148:371–9. doi: 10.1016/j.carbpol.2016.04.067
6. Marinopoulou A, Papastergiadis E, Raphaelides SN, Kontominas MG. Structural characterization and thermal properties of amylose-fatty acid complexes prepared at different temperatures. Food Hydrocoll. (2016) 58:224–34. doi: 10.1016/j.foodhyd.2016.02.034
7. Reddy CK, Lee D, Lim S, Park EY. Enzymatic debranching of starches from different botanical sources for complex formation with stearic acid. Food Hydrocoll. (2019) 89:856–63. doi: 10.1016/j.foodhyd.2018.11.059
8. Tan L, Kong L. Starch-guest inclusion complexes: Formation, structure, and enzymatic digestion. Crit Rev Food Sci Nutr. (2019) 60:1–11. doi: 10.1080/10408398.2018.1550739
9. Tufvesson F, Wahlgren M, Eliasson A. Formation of Amylose-lipid complexes and effects of temperature treatment. Part 2. Fatty acids. Starch. (2003) 55:138–49. doi: 10.1002/star.200390028
10. Seo TR, Kim JY, Lim ST. Preparation and characterization of crystalline complexes between amylose and C18 fatty acids. LWT – Food Sci Technol. (2015) 64:889–97. doi: 10.1016/j.lwt.2015.06.021
11. Conde-Petit B, Escher F, Nuessli J. Structural features of starch-flavor complexation in food model systems. Trends in Food Science & Technology. (2006) 17:227–35. doi: 10.1016/j.tifs.2005.11.007
12. Parada J, Santos JL. Interactions among starch, lipids, and proteins in foods: Microstructure control for glycemic response modulation. Crit Rev Food Sci Nutr. (2016) 56:2362–9. doi: 10.1080/10408398.2013.840260
13. Obiro WC, Sinha Ray S, Emmambux MN. V-amylose structural characteristics, methods of preparation, significance, and potential applications. Food Rev Int. (2012) 28:412–38. doi: 10.1080/87559129.2012.660718
14. Wang S, Chao C, Cai J, Niu B, Copeland L, Wang S. Starch–lipid and starch–lipid–protein complexes: A comprehensive review. Compreh Rev Food Sci Food Saf. (2020) 19:1056–79. doi: 10.1111/1541-4337.12550
15. Liu X, Huang S, Chao C, Yu J, Copeland L, Wang S. Changes of starch during thermal processing of foods: Current status and future directions. Trends Food Sci Technol. (2022) 119:320–37. doi: 10.1016/j.tifs.2021.12.011
16. Le Bail P, Bizot H, Ollivon M, Keller G, Bourgaux C, Buléon A. Monitoring the crystallization of amylose–lipid complexes during maize starch melting by synchrotron x-ray diffraction. Biopolymers. (1999) 50:99–110.
17. De Pilli T, Jouppila K, Ikonen J, Kansikas J, Derossi A, Severini C. Study on formation of starch–lipid complexes during extrusion-cooking of almond flour. J Food Eng. (2008) 87:495–504. doi: 10.1016/j.jfoodeng.2007.12.028
18. Derycke V, Vandeputte GE, Vermeylen R, Man WD, Goderis B, Koch MH, et al. Starch gelatinization and amylose–lipid interactions during rice parboiling investigated by temperature resolved wide angle X-ray scattering and differential scanning calorimetry. J Cereal Sci. (2005) 42:334–43. doi: 10.1016/j.jcs.2005.05.002
19. Tufvesson F, Wahlgren M, Eliasson A. Formation of Amylose-lipid complexes and effects of temperature treatment. Part 1. Monoglycerides. Starch. (2003) 55:61–71.
20. Li Q, Gao Y, Li Y, Du SK, Yu X. Effect of hydrophilic groups in lipids on the characteristics of starch–lipid complexes. Int JFood Sci Technol. (2022) doi: 10.1111/ijfs.15835 [Epub ahead of print].
21. Chao C, Yu J, Wang S, Copeland L, Wang S. Mechanisms underlying the formation of complexes between maize starch and lipids. J Agric Food Chem. (2018) 66:272–8. doi: 10.1021/acs.jafc.7b05025
22. Chao C, Cai J, Yu J, Copeland L, Wang S, Wang S. Toward a better understanding of starch-monoglyceride-protein interactions. J Agric Food Chem. (2018) 66:13253–9. doi: 10.1021/acs.jafc.8b04742
23. Qin R, Yu J, Li Y, Copeland L, Wang S, Wang S. Structural changes of starch-lipid complexes during postprocessing and their effect on in vitro enzymatic digestibility. J Agric Food Chem. (2019) 67:1530–6. doi: 10.1021/acs.jafc.8b06371
24. Liu X, Chao C, Yu J, Copeland L, Wang S. Mechanistic studies of starch retrogradation and its effects on starch gel properties. Food Hydrocolloids. (2021) 120:106914. doi: 10.1016/j.foodhyd.2021.106914
25. Wang S, Wang S, Liu L, Wang S, Copeland L. Structural orders of wheat starch do not determine the in vitro enzymatic digestibility. J Agric Food Chem. (2017) 65:1697–706. doi: 10.1021/acs.jafc.6b04044
26. Zhou J, Guo J, Gladden I, Contreras A, Kong L. Complexation ability and physicochemical properties of starch inclusion complexes with C18 fatty acids. Food Hydrocolloids. (2022) 123:107175. doi: 10.1016/j.foodhyd.2021.107175
27. Raphaelides S, Karkalas J. Thermal dissociation of amylose-fatty acid complexes. Carbohydr Res. (1988) 172:65–82. doi: 10.1016/S0008-6215(00)90843-7
28. Wang H, Wu Y, Wang N, Yang L, Zhou Y. Effect of water content of high-amylose corn starch and glutinous rice starch combined with lipids on formation of starch-lipid complexes during deep-fat frying. Food Chem. (2019) 278:515–22. doi: 10.1016/j.foodchem.2018.11.092
29. Liu X, Luan H, Yu J, Wang S, Wang S, Copeland L. A method for characterizing short-range molecular order in amorphous starch. Carbohydr Polym. (2020) 242:116405. doi: 10.1016/j.carbpol.2020.116405
30. Liu P, Kang X, Cui B, Gao W, Wu Z, Yu B. Effects of amylose content and enzymatic debranching on the properties of maize starch-glycerol monolaurate complexes. Carbohydr Polym. (2019) 222:115000. doi: 10.1016/j.carbpol.2019.115000
31. Luo S, Zeng Z, Mei Y, Huang K, Wu J, Liu C, et al. Improving ordered arrangement of the short-chain amylose-lipid complex by narrowing molecular weight distribution of short-chain amylose. Carbohydr Polym. (2020) 240:116359. doi: 10.1016/j.carbpol.2020.116359
32. Cui J, Zheng B, Liu Y, Chen L, Li B, Li L. Insights into the effect of structural alternations on the digestibility of rice starch-fatty acid complexes prepared by high-pressure homogenization. LWT. (2021) 136:110294. doi: 10.1016/j.lwt.2020.110294
33. Li Q, Dong Y, Gao Y, Du SK, Li W, Yu X. Functional properties and structural characteristics of starch-fatty acid complexes prepared at high temperature. J Agric Food Chem. (2021) 69:9076–85. doi: 10.1021/acs.jafc.1c00110
Keywords: heat condition, water content, wheat starch-glycerol monopalmitin complexes, structure, in vitro starch digestibility
Citation: Jiao F, Liu Z, Ni J, Yu J, Wang S and Liu X (2023) The structure and in vitro starch digestibility of wheat starch-glycerol monopalmitin complexes: the effect of heating conditions and water content. Front. Nutr. 10:1288067. doi: 10.3389/fnut.2023.1288067
Received: 03 September 2023; Accepted: 31 October 2023;
Published: 18 November 2023.
Edited by:
Tiantian Zhao, Agri-Food Research Institute Guangdong Academy of Agricultural Sciences, ChinaReviewed by:
Enbo Xu, Zhejiang University, ChinaJiayue Guo, University of California, Berkeley, United States
Copyright © 2023 Jiao, Liu, Ni, Yu, Wang and Liu. This is an open-access article distributed under the terms of the Creative Commons Attribution License (CC BY). The use, distribution or reproduction in other forums is permitted, provided the original author(s) and the copyright owner(s) are credited and that the original publication in this journal is cited, in accordance with accepted academic practice. No use, distribution or reproduction is permitted which does not comply with these terms.
*Correspondence: Xia Liu, liuxia@sdfmu.edu.cn
†These authors have contributed equally to this work and share first authorship