- 1Clinical Nutrition Research Centre, Singapore Institute of Food and Biotechnology Innovation, Agency for Science, Technology and Research (A*STAR), Singapore, Singapore
- 2Faculty of Health and Social Sciences, Bournemouth University, Bournemouth, United Kingdom
As the global population continues to grow, the demand for dietary protein is rapidly increasing, necessitating the exploration of sustainable and nutritious protein sources. Algae has emerged as a promising food source due to their high value ingredients such as proteins, as well as for their environmental sustainability and abundance. However, knowledge gaps surrounding dietary recommendations and food applications restrict algae’s utilization as a viable protein source. This review aims to address these gaps by assessing the suitability of both microalgae and macroalgae as alternative/complementary protein sources and exploring their potential applications in food products. The first section examines the potential suitability of algae as a major food source by analyzing the composition and bioavailability of key components in algal biomass, including proteins, lipids, dietary fiber, and micronutrients. Secondly, the biological effects of algae, particularly their impact on metabolic health are investigated with an emphasis on available clinical evidence. While evidence reveals protective effects of algae on glucose and lipid homeostasis as well as anti-inflammatory properties, further research is required to understand the longer-term impact of consuming algal protein, protein isolates, and concentrates on metabolic health, including protein metabolism. The review then explores the potential of algal proteins in food applications, including ways to overcome their sensory limitations, such as their dark pigmentation, taste, and odor, in order to improve consumer acceptance. To maximize algae’s potential as a valuable protein source in the food sector, future research should prioritize the production of more acceptable algal biomass and explore new advances in food sciences and technology for improved consumer acceptance. Overall, this paper supports the potential utility of algae as a sustainable and healthy ingredient source for widespread use in future food production.
1. Introduction
It is projected that in 2050, the world’s population will reach 10 billion people (1, 2), necessitating a 35 to 56% increase in food production from 2010 to 2050 (1). Concurrently, due to shifts in dietary preferences toward protein-rich food consumption, demand for dietary protein continues to grow. Adding another level of complexity to this issue is the consideration of reducing environmental burdens while improving global nutrition, as outlined by the United Nations’ Sustainable Development Goals (3). In light of these factors, the exploration of alternative and complementary protein sources that are sustainable, nutritious, and affordable becomes crucial (4, 5).
Algae are a diverse group of photosynthetic eukaryotes. They encompass both microalgae, which are microscopic and unicellular, and complex macroalgae, which are commonly known as seaweed (6). Algae has gained attention as a potential solution to this increasing demand due to its sustainability advantages, excellent nutritional profile, and opportunities in food applications as well as nutraceuticals (7). Compared to most terrestrial plants, algae have a higher growth rate, productivity, and protein yield, making it a more sustainable source of protein (8). Algae cultivation is resource efficient in the already burdened agri-food system (7, 9), since it does not compete with traditional food sources for potable water and arable land (10–12). Algae can be cultivated in a wide range of environments, from oceans and lakes to controlled photobioreactors, either carbon-neutral photoautotrophically, or heterotrophically utilizing minimal carbon sources (8). This makes algae cultivation contribute to a lower environmental footprint compared to conventional protein sources (7, 13, 14). As part of the marine circular economy approach (12), algae’s potential in mitigating greenhouse gas emissions while generating high value organic nutrients or biofuel through CO2 biofixation (15–17) and industrial CO2 trapping (18) has been explored. Therefore, algae’s versatility extends beyond food production, making it a highly attractive alternative to conventional protein sources.
Despite the growing interest in algae, its widespread adoption in the food industry is still in its preliminary stages. While algae can be cultivated in controlled environments, scaling up production to meet the consistent quality and yield required for mainstream food production poses challenges (19). Additionally, with algae-based food products still being relatively new to the market for a substantial part of the population, consumer understanding and acceptance as well as the regulatory landscape for algae-based food products is still evolving. Limited knowledge regarding the suitability of algae in food applications, particularly from both nutrition and food technology perspectives, presents significant barriers to its integration into mainstream food systems. Similar to the introduction of all novel foods, increasing awareness and knowledge about algae as well as increasing the product range can encourage consumers to embrace new eating habits that include algae in their diets, which can ultimately lead to increased demand, economies of scale, and accessibility of algae products to a wider population. Taste, cost, and nutrition are essential characteristics of a successful food product, all of which impact consumer preferences and influence purchasing decisions. Algae has promising prospects in satisfying all three, which will be addressed in terms of algae’s nutritional features, followed by its sensory properties, and finally its prospective food uses.
While numerous studies have recognized algae’s potential to provide essential or high value nutrients such as fatty acids, minerals, or dietary fiber, this review aims to critically assess the suitability of algae to be consumed as a regular protein source. This work focuses on edible species of macroalgae and microalgae and considers their nutritional compositions, nutrient bioavailability, and accompanied health impacts based on clinical evidence. The second part of the review will explore the opportunities of incorporating algae in food applications and summarize the outcomes of previous attempts of algal food applications. Consumer acceptance and potential challenges will be examined to identify the sweet spot for successful algae incorporation in food products. Finally, future research directions will be discussed to address the identified challenges and prioritize further advancements in algae utilization in the food industry.
2. Edible algae
Despite being classified as a novel food, algae have been harvested and consumed by coastal populations for centuries, dating back to prehistoric times (20). The long-standing tradition of their consumption has been reviewed elsewhere (21, 22), highlighting their significance as an integral part of traditional diets and culinary practices, particularly in Latin American and Asian cultures.
While the phylogeny relationship of algae is continuing to be updated as new ones are discovered, algae is broadly classified into three groups depending on their pigments, namely green (chlorophytes), brown (phaeophytes), and red (rhodophytes) algae. Although not part of algae, cyanobacteria are often referred to as blue-green algae, which will also be included in this article. Figure 1 illustrates the broad classification of algae based on their pigments.
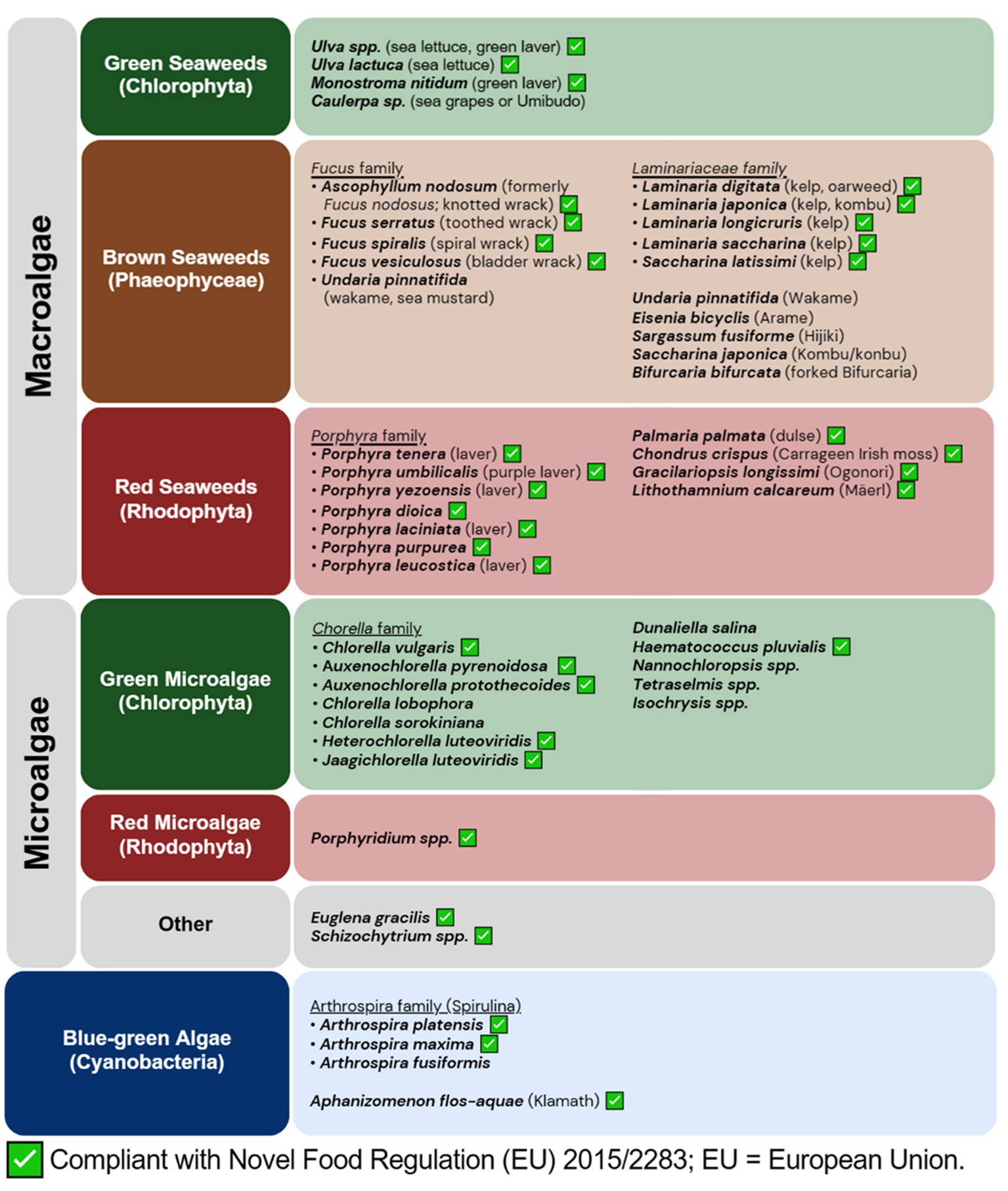
Figure 1. Overview of edible algae and their regulatory compliance. Common edible algae are broadly categorized according to their pigments. Cyanobacteria is included even though they are not considered as true algae. A green checkbox indicates compliance with the European Union’s Novel Food Regulation (EU 2015/2283). Data accurate as of June 20, 2023.
2.1. Macroalgae (seaweeds)
2.1.1. Green seaweeds (chlorophytes)
The green seaweeds (chlorophytes), such as sea lettuce (Ulva spp.) and sea grapes (Caulerpa spp.), are known for their vibrant green color and high protein content. Sea lettuce (Ulva spp.) is commonly used in salads and soups whereas sea grapes or Umibudo (Caulerpa spp.) are a popular edible green seaweed in Japan and Southeast Asian countries.
2.1.2. Brown seaweeds (phaeophytes)
Brown seaweeds (phaeophytes) feature varieties such as wakame (Undaria pinnatifida; referred hereafter as U. pinnatifida), arame (Eisenia bicyclis), hijiki (Sargassum fusiforme), kombu/konbu (Saccharina japonica; referred hereafter as S. japonica), and various kelp (Laminariaceae family). Wakame is a popular brown seaweed used in Asian cuisine, known for its tender texture and mild flavor. Arame and hijiki are commonly used in Japanese cuisine as well, adding distinct flavors and textures to various dishes. Kelp, with its large size and high concentrations of alginate, fucoxanthin, and fucoidan content, offers a wide range of functional and nutritional properties. Notable edible kelps include cochayuyo and kombu. Kombu is widely used in Japanese cuisine, providing a unique umami flavor.
2.1.3. Red seaweeds (rhodophytes)
Red seaweed (rhodophytes) includes commonly eaten seaweeds such as nori/laver (Porphyra spp.), dulse (Palmaria palmata; referred hereafter as P. palmata), and Irish moss (Chondrus crispus; referred hereafter as C. crispus). Nori is known for its use in sushi rolls. Dulse, commonly found in cool Artic temperatures, is known for its distinct flavor, and often used in soups, salads, and as a snack. Irish moss, also known as carrageen moss, is frequently used in food goods as a thickening ingredient. Red seaweeds are valued for their unique pigments like phycoerythrin.
2.2. Microalgae
Microalgae, on the other hand, are unicellular photosynthetic organisms that offer a range of nutritional benefits and have gained recognition as a dietary supplement. Notable edible species include Chlorella, and cyanobacteria (or blue-green algae) such as Spirulina and Aphanizomenon flos-aquae (referred hereafter as A. flos-aquae). Other candidates that have potential to be commercialized for human consumption are Tetraselmis, Isochrysis and Nannochloropsis (23). Each of these species has unique nutritional profiles and benefits (24) and is commonly consumed in the form of powdered supplements or pills, with recommended daily doses ranging from 1 to 5 grams (25).
Edible Chlorella spp. include C. vulgaris, Auxenochlorella pyrenoidosa (formerly known as C. protothecoides) (26), Auxenochlorella protothecoides (formerly known as C. protothecoides), and C. lobophora and C. sorokiniana which are green microalgae that possess a rich composition of protein, fat, carbohydrate, fiber, chlorophyll, vitamins, and minerals (27). However, Chlorella hard cell wall is indigestible by humans and must be broken down either by mechanical or enzymatic degradation before consumption (28). Once the cell wall is broken, Chlorella is typically consumed as a supplement in tablet, capsule, liquid, or powder form.
Commonly known as Spirulina, Arthrospira has been consumed by the Aztecs for its endurance-boosting properties. Edible Arthrospira spp., such as A. platensis and A. maxima are only remotely related to the Spirulina genera; yet they are still marketed under the commercial name “Spirulina” (29). It is known as a superfood rich in protein, vitamins, and minerals, making it an ideal dietary supplement for vegetarians or vegans (30).
2.3. Regulation of algae-derived novel foods
Concerning the regulatory features of novel foods derived from algae, the European Union mandates compliance with Novel Food Regulation (EU) 2015/2283 (31). To be exempt from the legislation, the product must have been in circulation as a food or food additive with substantial consumption prior to 15 May 1997. If not, a safety assessment of the novel food’s compositional, nutritional, toxicological, and allergenic attributes, as well as information regarding the production process, proposed uses, and dosage levels, is required before it can be approved for distribution on the food market (31). As of 20th June 2023, several microalgae species and ingredients have been approved for use in the European Union. These include various species from the family of Chlorella spp., such as C. vulgaris and those previously classified as Chlorella spp., such as Auxenchoella pyrenoidosa, Auxenchorella protothecoides, Heterochlorella luteoviridis and Jaagichlorella luteoviridis. Other approved microalgae include Spirulina (A. platensis), Klamath (A. flos-aquae), Euglena gracilis (E. gracilis), Haematococcus pluvialis, Schizochytrium spp., and red microalgae Porphyridium spp. (32).
For seaweeds, several green, red, and brown seaweed species are listed as approved (32). Approved green seaweeds include aonori or green laver (Ulva spp., previously known as Enteromorpha spp.) and sea lettuce (Ulva lactuca; referred hereafter as U. lactuca), and Monostroma nitidum. Approved red seaweeds include nori which is a family of Porphyra spp., such as Porphyra tenera, Porphyra umbilicalis, Porphyra yezoensis, Porphyra dioica, Porphyra laciniata, Porphyra purpurea, Porphyra leucostica (all referred hereafter with the genus name shortened to its first letter). Other approved red seaweeds include dulses (P. palmata), Irish moss or Carrageen moss (C. crispus), the agar-producing Ogonori or Gracilariopsis longissimi, and the calcium-rich Mäerl (Lithothamnium calcareum). Approved brown seaweeds include kelp from the Laminariaceae family, such as Laminaria digitata, Laminaria japonica, Laminaria longicruris, Laminaria saccharina, and Saccharina latissimi, as well as the Fucus family, such as Ascophyllum nodosum, Fucus nodosus, Fucus serratus, Fucus spiralis and Fucus vesiculosus (all referred hereafter with the genus name shortened to its first letter).
3. Assessing the suitability of microalgae and macroalgae as sources of alternative/complementary proteins
Algae are an ideal source of nutrients as they are rich in protein, lipids, vitamins, minerals, and essential fatty acids (25). Despite being consumed as a food source, seaweed’s potential to complement conventional protein sources is not widely recognized (33). In contrast, microalgae like Spirulina and Chlorella have gained popularity as dietary supplements mainly for their dietary fiber and other minor nutritional components. While the consumption of microalgae as dietary supplements is well-established, there is still much to be discovered and understood regarding their utilization as a primary protein source in the form of food (23, 33).
Section 3 will evaluate the suitability of algae as an alternative/complementary source of protein. This entails analyzing the major constituents of algae, including their macronutrient composition and bioavailability. Particularly, this section covers in-depth analyses that evaluate protein quality, amino acid composition, and briefly provides an overview of the presence of other nutrients such as carbohydrates/fiber, lipids, vitamins, and minerals as well as bioactive phytonutrients such as polyphenols and carotenoids. Furthermore, we will investigate the effects of algal consumption on digestion, metabolism, and overall health.
3.1. Overview of the nutritional composition of algae focusing on protein content and functionality
The nutritional composition and cellular structure of algae may vary depending on the strain, geographical region, and season, as well as the strain’s response to various factors such as light intensity, photoperiod, temperature, available nutrients, and growth phase (22, 34, 35).
In general, algae are abundant in dietary fiber, ω-3 and ω-6 fatty acids, vitamins (including A, C, D, E, K, and B vitamins), and essential minerals such as iron, calcium, magnesium, and potassium. Microalgae are abundant in protein, with species such as C. vulgaris, C. pyrenoidosa, A platensis, A. maxima containing 50–70% protein by dry weight, which comprises all nine essential amino acids (EAA) (25, 34). This makes algae valuable protein sources equivalent to those derived from animals (25, 34).
In addition to their rich nutritional profiles, algae contain primary metabolites such as polysaccharides and phycobiliproteins that may enhance the rheological and nutritional qualities of food. In addition, algae contains secondary metabolites including polyphenols and xanthophylls, which possess antioxidant and other bioactive properties (36). More details on the nutritional composition of algae are elaborated below.
3.1.1. Estimation of algal protein content
According to review articles published over the last 20 years, red seaweed is a rich source of protein, with laver Porphyra tenera containing up to 47% protein (37–42). However, tracking the origin of this high number revealed that it was based upon a study conducted in 1983 (43). Another study conducted by Admassu et al. (44) was able to corroborate a similar finding for red seaweed laver, albeit with a slightly lower (43%) reported percentage. However, both studies employed a nitrogen-to-protein conversion rate of 6.25 (44, 45), which results in an overestimation of protein content, owing to the presence of non-protein nitrogenous components, such as chlorophyll and inorganic nitrogen (46, 47).
Comparative studies have examined the protein content of algae using different methods (46, 47). These studies suggested that a more accurate nitrogen-to-protein conversion rate for algae is 5 (47, 48), or potentially closer to 4, depending on the specific species (46). After adjusting for the conversion rate, the highest recorded protein content in red seaweed should be 30 to 35%, which is closer to the typical protein content of Porphyra spp., which ranges from 23.7 to 30% (49–51).
The protein content of green seaweeds is comparable to that of red seaweeds, and brown seaweeds have a lower protein percentage of dry weight than these two, regardless of the protein determination technique (42, 48). According to a meta-analysis, the 5th to 95th percentile range for green seaweeds protein content is 4.6 to 32.2% dry weight, the range for red seaweeds is 2.0 to 28.7% dry weight, and the range for brown seaweeds is 3.3 to 15.9% dry weight (48). Nonetheless, it is crucial to acknowledge that variations in their concentrations have been observed, which can be attributable to factors like species, seasonal changes, and environmental conditions of growth (42, 52, 53).
As previously stated, microalgal species are known for their high protein content. Notably, species such as Chlorella spp., Spirulina, and Dunaliella salina (D. salina) have been extensively utilized, reporting a protein content of 38 to 70% dry weight (54–58). In contrast to seaweeds, protein content in cultivated microalgae remains consistent within standardized systems, although differences are observed within the same species based on the selected cultivation methodology (35, 59, 60).
The protein content of algae exceeds that of eggs (13%) and certain plant-based grains such as oats (13.5%) and wheat (15%), while being comparable to that of soy (38.6%) and chicken breast (31%) (61). Notably, it is important to acknowledge that algae protein encompasses the entire spectrum of EAA, which will be elaborated in Section 3.1.2.
3.1.2. Quality of algal protein: amino acids composition
The primary determinants of a protein’s nutritional quality are its amino acid composition, their relative quantities, and their bioavailability, which reflects the body’s ability to absorb and utilize them (62, 63). Among the 20 distinct proteinogenic amino acids present in the human body, nine are classified as essential amino acids (EAAs) due to the body’s inability to synthesize them in quantities that adequately fulfill physiological requirements, making them necessary to be acquired through dietary sources (64). These are tryptophan, histidine, isoleucine, leucine, lysine, methionine, phenylalanine, threonine, and valine.
On average, the composition of EAAs in algae meets the Food and Agriculture Organization (FAO) guidelines (65), and also closely resembles that of conventional protein sources, such as soybeans and eggs. Amino acid content of commercially available microalgae, such as Chlorella, Spirulina, Nannochloropsis spp., Tetraselmis spp., and macroalgae, dulse and Porphyra spp. are superior to that of the FAO guidelines (Supplementary Table S1), this is in contrast to many other plant-based proteins, which often fall short of meeting the recommended daily intake (66). On average, algae possess an EAA composition of 40 to 44%, which is marginally lower than soy at 48.6% and eggs at 49.3% (Figure 2).
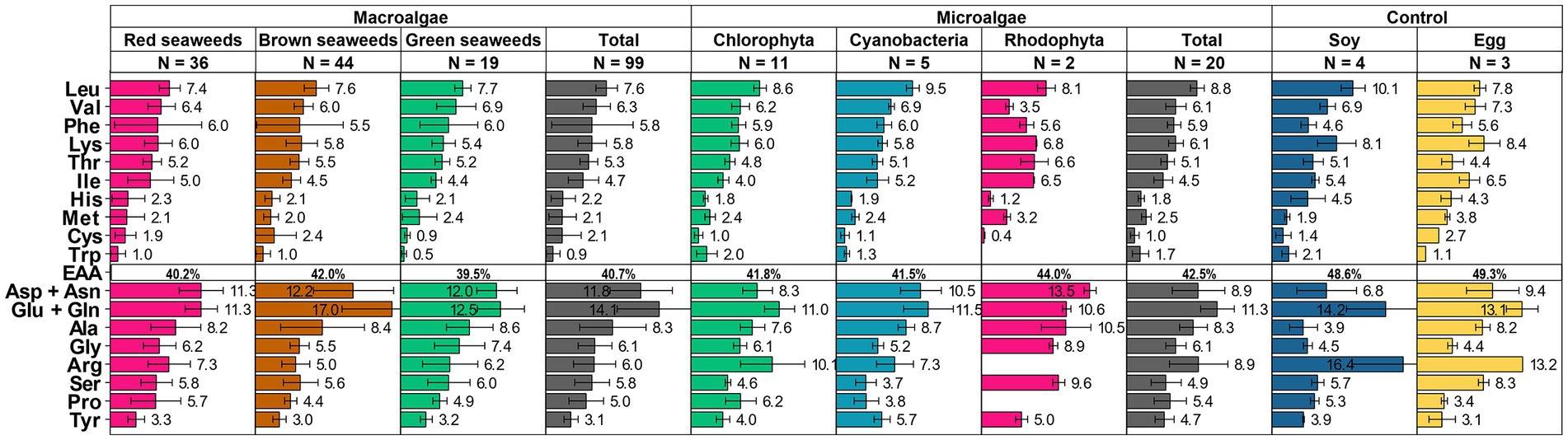
Figure 2. Amino acid compositions (expressed in grams per 100 grams of protein) of various macro- and microalgae were consolidated from scientific literature. The error bar represents the standard deviation (S.D.). N represents number of datapoints. Please refer to Supplementary Table S1 for the unprocessed data and corresponding scientific literature references.
A closer breakdown of amino acid composition is depicted in Figure 2. While amino acid profiles differ across algae species, the branched-chain amino acids (BCAAs)–namely leucine, isoleucine, and valine–demonstrate comparable levels to soy and eggs, making them a promising protein source for muscle health promotion.
However, algae are relatively deficient in sulfur-containing amino acids, such as cysteine and methionine. It is noteworthy that FAO/WHO/UNU recommends a daily intake of 13–15 mg/kg/day for these amino acids (67, 68), which is achievable with approximately 20 g of algal protein daily for an average weight adult. Algae also outperform soy in cysteine content, but not eggs. On the other hand, algae contain lower amounts of other EAAs like lysine and histidine compared to eggs and soy.
Conversely, algae are rich in aspartic acid and glutamic acid, especially in seaweed (Figure 2). High amounts of glutamic acid, typically ranging from 20 to 33% in certain genera like Sargassum and Laminaria (Supplementary Table S1), contribute to the distinctive ‘umami’ taste in seaweed, which can be further intensified when combined with 5′-ribonucleotides and alanine. Algae also have a higher content of alanine and glycine compared to soy and egg, which is important for physiological functions such as gluconeogenesis and as neurotransmitters. In addition, algae possess moderate levels of phenylalanine, tyrosine, and threonine, which are generally recognized as the limiting amino acids that restrict the nutritional value of plant proteins.
3.1.3. Algae as a viable alternative protein source: safety and availability
The effects of consuming seaweed as an alternative protein source are still largely unknown in humans, particularly in terms of protein bioavailability and cellular utilization. Nevertheless, the utilization of macroalgae or seaweed biomass as alternative feed resources for livestock has gained increasing interest in recent years, yielding valuable preliminary information for humans. A meta-analysis of six animal studies involving broiler chickens revealed that dietary seaweed enhanced body weight gain and feed conversion ratio without affecting intake (69). The types of seaweed used varied, as did the inclusion dose (2 to 30 g/kg) and intervention duration (21 to 42 days). Notably, one of the initial investigations that introduced seaweed as a dietary component for chickens demonstrated that Ulva seaweed feeding resulted in reduced growth when included as 10% of the total diet, owing to its substantial unmetabolizable polysaccharide content (70). At a lower incorporation, a 3% Ulva diet increased the methionine composition in the feeds, which contributed to the increased muscle yield and serum quality. However, no significant effects were observed on feed intake or growth (71, 72). For ducks however, the inclusion of 12 to 15% red seaweeds Polysiphonia improved carcass muscle quality (73). In swine, the inclusion of seaweed resulted in improved immune function responses (74), and at a 1% inclusion level of Ascophyllum, muscle quality and gut health were improved (75, 76). In vitro digestibility of seaweeds ranged from 77 to 82% (77).
In terms of microalgae, the rigid cell wall limits protein digestibility and bio-accessibility in poultry and swine (78). According to Spínola et al. (78), protein digestibility of Spirulina (A. platensis) ranges from 66.1 to 68.7% in poultry (at 1% microalgae incorporation) and from 75.4 to 80.6% in swine (at 10% microalgae incorporation). However, when these cell walls are mechanically or enzymatically broken down through methods like bead milling or enzymatic hydrolysis, the digestibility significantly improves, as shown by in vitro digestion. For example, digestibility in Nannochloropsis spp. increased from 54 to 79% (79) and from 62 to 78% (80), as well as in Chlorella and A. platensis, it increased from 35 and 50% to almost 70 and 97%, respectively (81).
The highest administration of algal biomass is reported in a 4-week human trial examining the impact of administering 48 g of brown seaweeds (wakame) daily in the form of tablets (82). There were no reported adverse effects but instead, several positive effects were observed (82), which will be further discussed in Section 4. Due to the limited availability of algal protein on the market, there have been no clinical studies evaluating its consumption effects. It must be noted that while algae are generally regarded as safe for consumption, there have been documented instances of allergic reactions in the Allergome database (allergome.org) for species including A. maxima, A. platensis, Chlorella (species not specified), and Laminaria. Nevertheless, the allergenicity of algae is yet to be biochemically characterized and studied (54).
Although studies on algae’s efficacy as a protein source for humans are limited, collective findings of livestock studies suggest that it could be beneficial for weight gain, cellular utilization, and gut health maintenance. A human trial involving 48 g high doses of brown seaweeds biomass had no adverse reactions reported. Also, even though microalgae may have limited protein availability due to their rigid cell walls, this can be improved by pre-treatments, particularly beads milling. However, more research is needed to confirm algae’s safety and efficacy as a protein source for human consumption.
3.1.4. Beyond protein: algae’s co-nutrients
While extracting pure protein from algae is challenging, algae’s rich co-nutrients such as carbohydrates, pigments, and lipids offer various health benefits beyond protein content (22, 35). These have been discussed elsewhere (83–92), and are not the focus of this study.
Regarding macronutrients, algae are rich in carbohydrate content due to their photosynthetic nature. Particularly in macroalgae, these saccharides appear as indigestible fibers, which act as prebiotics to promote peristalsis, enhance satiety, and slow down gastric emptying (22). Notably, saccharides in macroalgae such as Neoporphyra haitanensis (Chinese zicai), A. nodosum, F. vesiculosus and S. japonica are reported to maintain a healthy gut microbiome and modulate glycemic responses to starch intake (22, 93). Besides saccharides, lipids serve as energy stores as well as structural components in algae. Macroalgae typically contain less than 5% lipids, while some microalgae species contain 20 to 50% lipids (94, 95). The long-chain polyunsaturated fatty acids (PUFAs) found in algae hold particularly promising applications in nutraceuticals and pharmaceuticals as supplements, but their bioaccessibility might be limited by algal cell walls (96).
As for micronutrients, algae are considered superfoods as they contain a variety of vitamins including provitamin A, vitamins B1, B2, B6, B12, C, and E, as well as folic acid, niacin, and pantothenic acid. However, the bioavailability of algal vitamin B12 is questionable (97, 98). For example, dried laver/nori and Chlorella contain genuine vitamin B12 (97, 99, 100), whereas Spirulina contains mostly pseudo-B12, which is inactive in humans (101–104). Although pseudo-B12 is not harmful (105), it should be noted that other Spirulina components may interfere with vitamin B12 absorption (106), rendering it a less reliable B12 supplement. In addition, algae has the unique ability to form micelles (107, 108) and facilitate mineral uptake and absorption, as confirmed by in vitro digestion models (109, 110).
Bioactive compounds, such as carotenoids and polyphenols, are another important co-nutrient in microalgae and seaweeds. Notably, microalgae are a cost-effective source of a broad spectrum of carotenoids (111–113), including astaxanthin and lesser-known pigments like fucoxanthin and zeaxanthin (114, 115). Additionally, these organisms contain polyphenols such as phenolic acids, flavonoids, and lignans, which are potent antioxidants and anti-inflammatory agents (108–113, 116, 117) contributing to cellular protection from oxidative stress (23, 116, 118–123) and cardioprotective properties (124).
In summary, while the extraction of pure protein from algae may present challenges, algae offer a comprehensive array of macronutrients such as saccharides and lipids, and micronutrients such as vitamins, minerals, carotenoids, and polyphenols, which possess beneficial functions — from gut health enhancers to potential pharmaceutical resources. These highlight algae’s untapped potential in various health-related applications in metabolic effects, which will be discussed in Section 4.
4. Health effects of algae demonstrated in clinical trials
While in vitro and animal studies offer crucial insights into the prospective health benefits of algae — a topic thoroughly examined elsewhere (125) — clinical studies centered on the potential health benefits of incorporating algae into dietary routines present advantageous insights for nutritional science. To date, research has largely focused on examining the health effects of whole algal biomass while the health benefits of isolates, such as protein isolates, are unknown. Section 4 provides an overview of the health effects of algae demonstrated in human clinical trials with regards to glucose metabolism and satiety, lipid metabolism, and other health-promoting properties.
4.1. Effects of algae on glucose metabolism and satiety
Eleven studies that investigated effects of algae consumption on postprandial glucose homeostasis and satiety were reviewed (Table 1). Four were postprandial studies, two were short-term randomized controlled trials (RCTs), and five were longer-term RCTs. Findings showed disparities, with some studies demonstrating beneficial effects on glycaemia and satiety, while others found no significant impact.
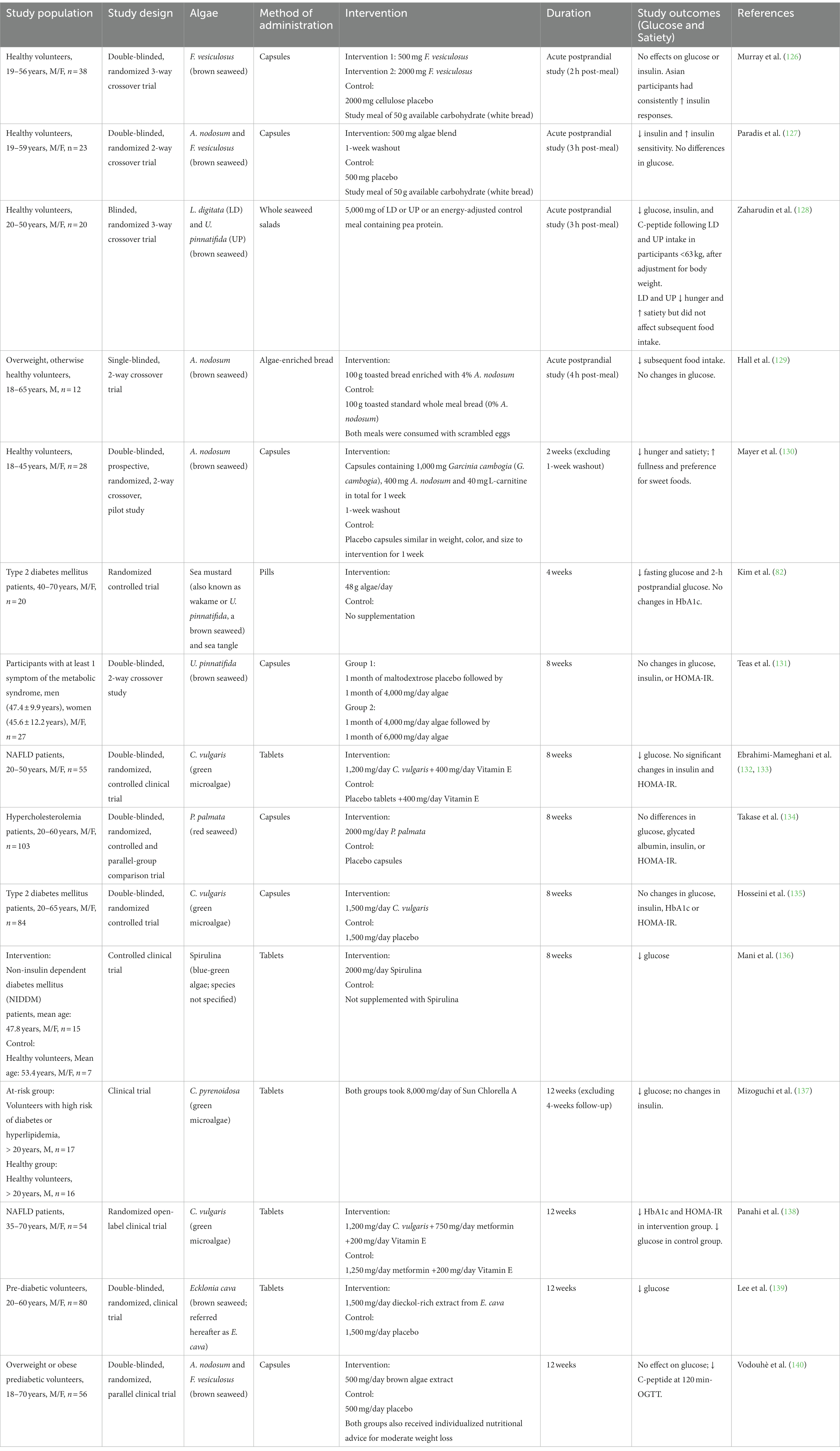
Table 1. Summary of human trials on effects of algae consumption on glucose homeostasis and satiety.
4.1.1. Postprandial studies
In acute postprandial crossover studies involving healthy participants, hypoglycemic effects of algae were more evident in participants of lower body weight and in studies lasting at least 3 h after consuming a carbohydrate-based meal (Table 1). For instance, Murray et al. found no discernible hypoglycemic effect within the first 2 h of consuming up to 2000 mg brown algae (126), whereas another study found evident hypoglycemic effects within 3 h following ingestion of 250 mg brown algae extract (127).
Satiating effects of algae were demonstrated, although potentially influenced by the macronutrient composition of the study meal. A longer, 4-h postprandial study found no changes in glycaemia but distinct changes in satiety (129). This may be due to the relatively small sample size (n = 12) and that the study meal served scrambled eggs with the algae-enriched bread, increasing the protein and fat content of the meal as compared to other studies that served algae with white bread (126, 127). Another 3-h postprandial study saw no hypoglycemic effects until body weight was factored into the statistical analysis (128). Consuming the algae also had satiating effects though it did not affect subsequent food intake.
4.1.2. Short-term randomized clinical trials
Two studies ranging from 2 to 4 weeks found algae to increase satiety, reduce fasting glucose and decrease 2-h postprandial glucose (Table 1). Both studies utilized brown algae, but varied in daily dosages and the health status of participants — ranging from 400 mg/day in healthy participants (130) to 48 g/day in diabetic patients (82). These variations, combined with the different metrics employed across studies, limit the comparability of these studies.
4.1.3. Longer-term randomized clinical trials
RCTs ranging from 8 to 12 weeks in duration found inconsistent results regarding hypoglycemic effects following algae intake (Table 1). However, reductions in glucose, HbA1c and HOMA-IR appeared more consistently in 12-week studies. The heterogeneity in these longer-term trials’ results could be attributable to suboptimal dosages of algae intake. Specifically, in the study conducted by Vodouhè et al. (140), the absence of effect on blood glucose could be due to the lower algae dose, compared to other studies of similar duration.
The study conducted by Mizoguchi et al. (137) was conducted in the absence of a proper control. It remains unclear whether the observed alterations in glycemic levels can be attributed to the consumption of Chlorella tablets or if they were influenced by the elevated susceptibility to disease. It also should be noted that the majority of these longer-term trials were conducted in individuals with or at high risk of chronic diseases. Hence, disease state and physiological differences may account for some inconsistencies in glycemic effects. Furthermore, the restricted scope of certain trials, such as those conducted among Mestizos in South America (131) or diabetes patients in clinical settings (136), may limit their generalizability.
Anti-diabetic properties of brown algae may be through polysaccharides, phlorotannins and polyphenolics that inhibit carbohydrate-digesting enzymes α-amylase and α-glucosidase and decrease hepatic gluconeogenesis. This has been demonstrated in vitro and in animal studies (141–143). Chlorella’s glucose-lowering properties may be attributed to decreasing non-esterified fatty acid levels, hence improving glucose uptake and utilization, supported by results in animal studies (144, 145). Antioxidants including lutein, α-tocopherol, ascorbic acid, and α- and β-carotene, may also improve insulin sensitivity and hepatoprotection (146–148).
Bromophenols found in red algae exhibited anti-diabetic activities in animal models by inhibiting α-glucosidase and PTP1B (149). Despite these promising findings, Takase et al. (134) found no changes in glucose homeostasis with 2000 mg/day red algae after 8 weeks although improvements in serum triglyceride (TG) after this first mention of triglyceride were seen in women. It could be that brown algae have more potent hypoglycemic effects.
However, anti-diabetic mechanisms of algae in humans are yet to be determined since findings have been inconsistent, such as consuming 500 mg algae extract resulting in hypoinsulinemic responses (127) but not after 2000 mg of algae (126). Moreover, studies have shown trends such as improvements in postprandial insulin but not in glucose levels (127).
Different algae dosages and compositions of the delivery medium could also explain variabilities in glycemic responses (126, 127), as capsules used in Paradis et al. contained algae extracts which were free of alginates and partially demineralized. In the study conducted by Zaharudin et al. (128), the relatively unprocessed whole seaweed salads used could explain the lack of postprandial glycemic responses before weight adjustment.
Taken together, there are demonstrated hypoglycemic and satiating effects of algae. However, results are inconsistent and further research is needed to establish more definitive conclusions and determine the optimal dosage and duration of algae consumption for glycemic control and effects on satiety.
4.2. Effects of algae on lipid metabolism
4.2.1. Short-term randomized clinical trials
Algae consumption has been associated with improvements in lipid profile across multiple studies (134, 136, 137, 150–154). In RCTs ranging 4 to 8 weeks, various forms of algae, including microalgae Spirulina and C. vulgaris, and seaweeds E. cava, Ulva, and sea mustard (U. pinnatifida), have demonstrated beneficial impacts on lipid profiles. Key outcomes across these studies highlight reductions in TG, total cholesterol, LDL-C, and VLDL-C.
4.2.2. Longer-term randomized clinical trials
All 12-week RCTs saw decreases in total cholesterol and LDL-cholesterol when administered with 2 to 8 g of microalgae (137, 150) or 400 mg of brown seaweed extract (153). An exception was Murray et al. (155) which provided 2000 mg brown seaweed extract but was underpowered to detect changes in lipid parameters. These findings span diverse populations, including healthy volunteers and at-risk populations with heart disease, diabetes, and those with hypercholesterolemia, reinforcing the potential broad-spectrum benefits of algae supplementation. These beneficial effects vary with the species and dosage of algae and the individual’s health status.
Notably, one study by Roach et al. (154) conducted a study investigating different dosages, revealing that lower doses (2 g) of sulfated xylorhamnoglucuronan-rich seaweed extract (Ulva sp. 84) yielded better outcomes than higher doses (4 g) in overweight volunteers. A subsequent study was then carried out with increased power to validate the reduction in non-HDL cholesterol seen, but results were not corroborated (154). The authors posited that it was potentially due to the baseline metabolic health of the study participants being less compromised (154). In addition, no washout period between the intervention arms might be another reason contributing to the unclear results of the trials. These findings underscore the need for personalized and precise dosing strategies in optimizing the lipid-lowering benefits of algae.
Specifically, the beneficial effect of Spirulina on lipid metabolism can be attributed to its high content of γ-linolenic acid, protein, and fiber. γ-linolenic acid inhibits the buildup of fat and cholesterol in the body. The protein and fiber composition of Spirulina lowers the production of VLDL and TG, as well as promotes the clearance of VLDL in the periphery, leading to declines in TG and VLDL after Spirulina supplementation. This in turn decreases the amount of LDL since the majority of these are generated from VLDL (136). It has been proposed that the positive effect of E. cava on blood lipid profiles is due to its polyphenol or phlorotannin content, although the mechanisms are unclear (153). The beneficial effect of SXRG84 on lipid levels can be attributed to the generation of short-chain fatty acid propionates by Akkermansia and Bacteroides, both of which increased in Study 1 (154). Further studies are necessary to explore and confirm the mechanisms behind the anti-dyslipidemic effects (Table 2).
4.3. Other health-promoting properties
Consuming algae has overall beneficial effects on inflammation and gut health in humans, although the available evidence varies in study design and algae dose administered. Nine studies were reviewed, with three of them investigating gut health (154, 156, 157).
4.3.1. Short-term randomized clinical trials
Four short-term studies showed improved anti-inflammatory markers and gut health after algae ingestion (Table 3). This was apart from one study that found algae-enriched bread to increase (as opposed to decrease) serum C-reactive protein (CRP) levels (158). As CRP levels remained within the normal clinical range, the authors stated that this was unlikely to impact health. Studies ranged from 2 to 4 weeks of intervention and were in generally healthy participants apart from one small study with Type 2 diabetes patients (82).
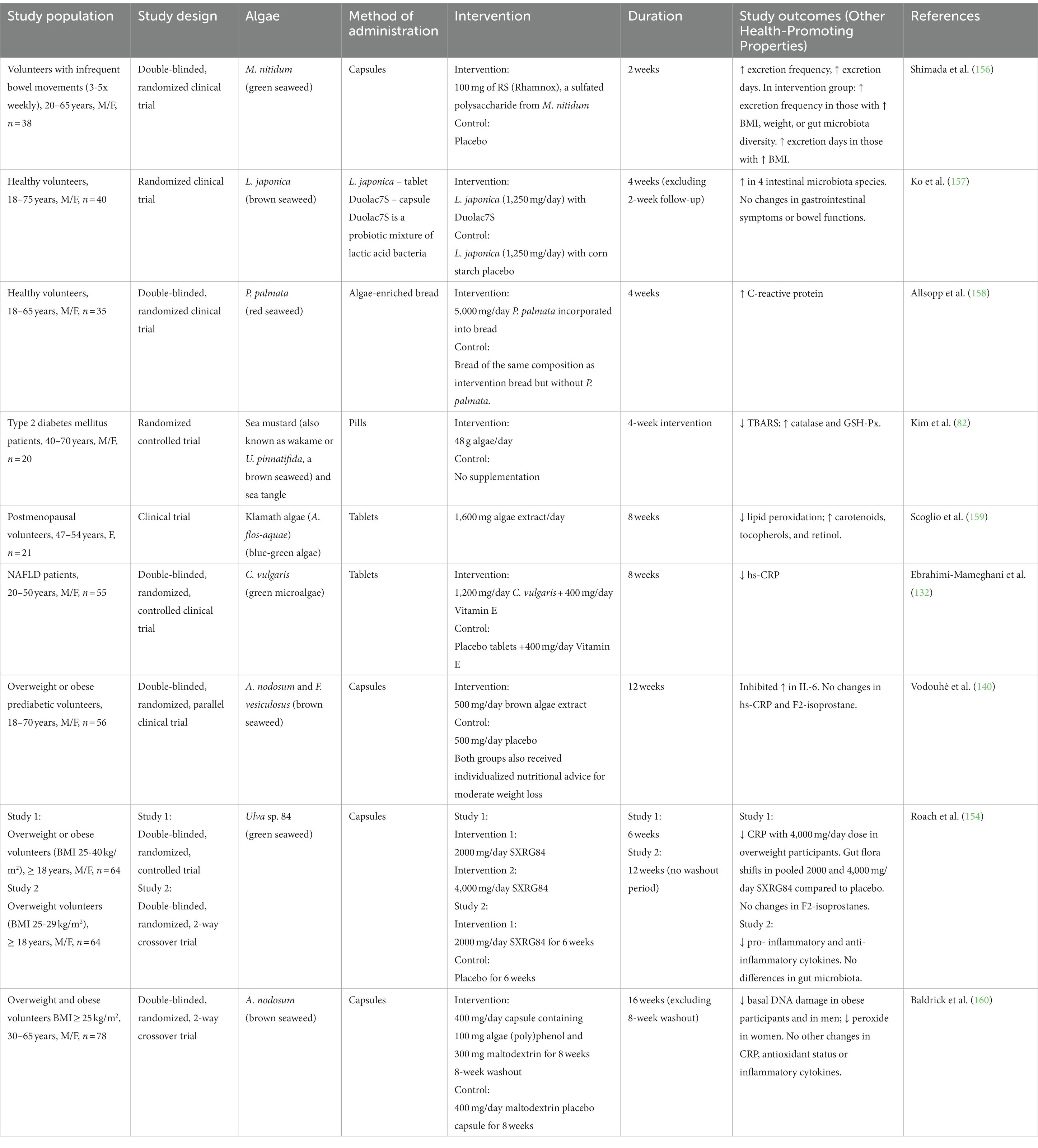
Table 3. Summary of human trials on effects of algae consumption on other health-promoting properties.
4.3.2. Longer-term randomized clinical trials
Longer term trials consistently demonstrated anti-inflammatory properties of algae, typically through reduced lipid peroxidation, lowered CRP, favorable changes in cytokines, increased antioxidant levels and reduced DNA damage (Table 3). Studies ranged from 6 to 16 weeks and were generally larger studies apart from one small trial (n = 21) (159). Roach et al. (154) found significant gut flora shifts after 6 weeks, although results were not replicated in a subsequent crossover trial. This was likely due to a lower algae dose (2000 mg/day) whereas the previous study used double the dose and pooled the intervention groups to see these microbiota changes. The subsequent crossover trial had no washout period between the two study arms however, increasing the likelihood of carry-over effects.
How algae reduce inflammation is unclear but in chemico, in vitro and in vivo animal studies suggest algae-derived bioactive compounds such as lipids have a role in mechanisms leading to downregulation of pro-inflammatory enzymes and mediators (89). The immunostimulatory activity observed by Allsopp and colleagues contrasts with previous in vitro and animal studies that have shown red algae to exert anti-inflammatory activity (161–164). Authors theorized that high temperatures in the baking process could have inactivated anti-inflammatory agents such as ω-3 PUFAs (158).
Beneficial effects of algae on gut health may be through their nutritional make up. The polysaccharide and fiber content of RS may explain its therapeutic effect on constipation, as well as causing functional alterations of cellular pathways (156). Authors noticed decreases in gut bacteria Clostridiales and increases in Negativicutes and Acidaminococcales which have been demonstrated to alleviate constipation (165, 166). In Roach et al. (154), gut flora changes were likely due to colonic bacteria responding positively to soluble dietary glycans, with some bacteria growing specifically on amine-polysaccharides provided during the study. Significant changes in intestinal microbiota however do not necessarily translate into improved gut health (157), although changes may be observed in studies of longer duration.
Studies varied greatly in algae dosages administered, from 100 mg to 48 g per day. Differing study durations and algae species may also explain why dose-dependent effects were not always observed. For example, 1,250 mg/day resulted in no gastrointestinal or bowel changes after 4 weeks (157) whereas constipation improved after 2 weeks of 100 mg/day of RS (156). Different algae species and a probiotic mixture given with the 1,250 mg/day of algae (157) may have led to distinct mechanisms explaining the differences in effects on the gut. A lower algae dose of 500 mg/day brown algae could explain why no changes in hs-CRP were seen after 12 weeks (though an improvement in IL-6 was seen) (140), when 1,200 mg/day C. vulgaris reduced hs-CRP after 8 weeks (132). Apart from Allsopp et al. (158), the reviewed studies investigating effects of algae on inflammation outcomes recruited diseased, postmenopausal, or overweight/obese patients who experience a basal level of inflammation which may not be present in healthy individuals. Hence factors such as disease state and physiological differences may explain some of the differences in observed anti-inflammatory changes in participants.
Several trials (135, 138, 140, 155, 158) measured blood pressure but found no difference between intervention and control groups. Teas et al. (131) observed reduced blood pressure though this was only in systolic blood pressure and in participants who consumed 6 g seaweed per day. However, significant reductions in systolic blood pressure were also seen in the placebo group but not in those consuming 4 g seaweed per day.
Algae has beneficial anti-inflammatory and prebiotic properties. However, studies with dose-dependent approaches and similar durations are needed to further define these effects and their underlying mechanisms. A recent meta-analysis of 31 trials found algae supplementation to significantly decrease HbA1c, HDL-C and TC and increase insulin, but not significantly change other glucose and lipid parameters (167). Results also suggested that algae supplementation could reduce 2-h post-meal glucose in Asians. Our findings support the decline in TC but observed discrepancies in the effects on other glycemic and lipid markers. These inconsistencies can potentially be explained by several factors, including the inclusion of different studies by Ding et al. in the meta-analysis, different algae species and dosages used, as well as dissimilar study populations. Moreover, several publications reviewed in the current work were published after December 2019 and therefore not included in the meta-analysis. However, Murray et al. (126) also observed ethnic-specific glucose metabolism responses in Asians versus non-Asians. Consistently elevated plasma insulin responses in Asian participants were found, irrespective of the algae dose (500 mg or 2000 mg) and even after a cellulose placebo, suggesting an increased risk of insulin resistance in this ethnic group compared with non-Asians. Hence, ethnicity may play a role in the effects of algae on postprandial glycaemia.
Taken altogether, clinical studies on algae consumption provide valuable insights into its potential health benefits and contribute to our understanding of the role of algae in promoting human well-being. From the clinical studies reviewed, algae intake has been shown to demonstrate hypoglycemic and satiating effects, improved lipid metabolism and beneficial effects on inflammation and gut health.
In spite of these encouraging results, additional research is required to determine the ideal dosage, long-term effects, processes, and potential treatments for increasing the bioavailability of nutrients from algae. A comparison of these metabolic effects utilizing the same algae in a dose-dependent manner with comparable study designs and durations is necessary due to the huge variances evident in prior research. Human clinical trials investigating similar effects for non-brown algae and algal extracts other than the total seaweed biomass, especially as protein extracts, are scarce and warrant further research efforts.
5. Incorporating algal proteins in future foods: challenges and opportunities
The food industry is witnessing an increase in innovative food products developed from algal biomass, marking the trend’s global progression (168). This interest stems from the nutritional characteristics that algae provide, as well as the previously noted health benefits (167–169).
In recent years, there has been a great deal of scientific interest in investigating the nutritional supplementation of algae in various cuisines. Due to the high operating costs associated with extracting and purifying algal proteins that maintain the nutritional and functional qualities of these proteins, the food industry focuses primarily on the utilization of algal whole biomass (19, 167, 168). Nonetheless, breakthroughs in the field of algal biomass incorporation in food production have revealed preliminary indicators of the modifications induced in the physiochemical features of final products and the resulting consequences, which will be elaborated below.
5.1. Algae-derived ingredients as flavor additives and seasonings
Incorporating algae-based ingredients in food additives and seasonings provides an opportunity to develop functional foods with added health benefits (22). Algae play a central role as ingredients in a wide range of dishes, providing unique flavors and a characteristic umami touch. Algae, in different forms, are extensively utilized in numerous recipes, particularly in health-conscious options. For example, algae can be added to citrus and fruity smoothies, salads, soups, and sushi recipes, enriching their nutritional value while imparting distinctive flavor profiles.
The edible kelp known as kombu, has gained significant popularity as food additives and flavor enhancers. Dr. Kikunae Ikeda, a prominent Japanese scientist, is credited with discovering the umami flavor from kombu dashi, a broth derived from kombu, in 1907. Kombu is a remarkable source of umami flavor, distinguished by its substantial content of all three key umami components: glutamate, inosinate, and guanylate. The notable attributes of kombu have resulted in its extensive utilization as a culinary enhancer across diverse global cuisines. Incorporating algae into homemade seasoning blends presents an intriguing opportunity to regulate sodium consumption, circumvent superfluous additives, and introduce the distinctive flavor characteristics inherent in algae.
5.2. Technofunctional properties of algal ingredients for food applications
Algae are significant in food technology due to their functional properties. Algal hydrocolloids like agar, carrageenan, xanthan, and guar gum are vital, serving as thickening, gelling, emulsifying, and stabilizing agents (170). Attributed to their ability to thrive in high stress living environments, algae produce an array of bioactive compounds, including antioxidants, polyphenols, and pigments.
In addition, algae, particularly microalgae, are abundant sources of various pigments, including chlorophyll, carotenoids, and phycobiliproteins (171). Due to the rising consumer demand for clean-label products and growing concerns about the potential health risks of synthetic colorants (172), algal pigments have become increasingly appealing to the food sector as natural alternatives (173, 174). Their application extends to various foods and beverages, ranging from chewing gum (175) to cookies (176).
Additionally, the natural encapsulation of algal pigments has developed into a revolutionary approach to protect pigments against deterioration and enhance their effectiveness in various food applications (177). Encapsulated pigments allow controlled and targeted release, preserving their stability and prolonging their functional properties in several dietary applications (177), such as antioxidant activities and other health-promoting effects (173, 177). Based on algal natural film-forming properties, algal-derived hydrocolloids have been developed into edible coatings and microcapsules for functional food products, thereby protecting bioactive ingredients from environmental damage, ensuring stability, and controlling release during consumption (178).
In addition to their effects on gut health, the dietary fibers present in algae, including polysaccharides, oligosaccharides, lignin, cellulose, and hemicellulose, have pivotal roles not only in the food industry but also in the cosmetic sector. These fibers contribute to the consistency of food products and act as stabilizing agents (22, 93, 179, 180).
5.3. Utility of algae in the production of meat analogs
Algal proteins also serve as a promising ingredient in meat analogs, not only for their physiochemical properties that serve as binders, fillers, and flavoring agents, but also for their amino acid profile that is promising in further bolstering nutritional value (181). Moreover, they provide a high-quality alternative for individuals allergic to soy or those adhering to vegan diets. Notable is the innovative utilization of algal ingredients to expand the selection of alternative plant-based meat products that are currently lacking on the market, particularly “pork” equivalents, such as dumplings and bacon, as well as seafood analogs (182, 183).
Algal biomass can be added in the production of meat analogs. Caporgno et al. demonstrated that mixing Chlorella-based microalgae with soy concentrates results in extrudates with a fibrous texture (184). Extrusion with up to 50% dried microalgal biomass affected fiber formation but could be mitigated by adjusting moisture levels. Microalgal-incorporated extrudates have a softer texture, due to the higher fat content in microalgae biomass resulting in lubrication effects while intact microalgae cells function as passive fillers —limiting access to intracellular proteins. Both features reduced texturing but increased tenderness compared to soy-based extrudates (184). Furthermore, the microalgae fortification enhanced the concentration of vitamins B and E in the extrudate, with over 95% preserved in the final product (184).
Similarly, incorporating Spirulina (A. platensis) biomass (10, 30% or 50%) in a texturized soy-based meat analog resulted in products with a black color and pronounced earthy flavor. However, 50% Spirulina (A. platensis) addition hindered the texture and led to reductions in the elasticity, fibrousness, and firmness of the extruded samples (183).
Interestingly, a red seaweed strain was patented for a strong bacon taste and later commercialized as a healthier vegan “bacon” product that is highly nutritious and sustainable (185, 186). For minced meat-based products, a commercially available, red-colored algal biomass known as Essential Red has been utilized. This biomass is derived from a specific microalgal strain with upregulated protoporphyrin IX and heme production pathways. Essential Red serves as the foundation for developing various algal-based animal-free “pork,” including vegan “meatballs,” pulled “pork” burgers, and dumplings with green-algal wrapping and red-algal filling (187).
Triton Algae Innovations, a company specializing in freshwater algae, exploits different strains of Chlamydomonas reinhardtii to produce algal-based biomass tailored to the needs of food companies it works with, including the Essential Red, Essential Green, and even colorless white algal biomass. The company selects strains with the desired biological traits, eliminating the need for genetic engineering, which is different from previous attempts in plant-based meat to mimic meat features (187, 188).
Besides plant-based proteins, algae’s utility in cultivated meat has been explored by Mewery, a food tech start-up venture that focuses on offering cultured pork products. Mewery has developed a novel prototype of cultivated meat composed of 75% pork cells and 25% microalgae cells. The incorporation of microalgae extracts in a hybrid culture medium provides the necessary conditions and nutrients for cell growth and division, allowing cells to develop into muscle and fat cells. Bioreactors have been built to scale up production and offer a diverse range of pork products, including meatballs, sausages, dumpling ground meat, and tenderloin (189, 190).
5.4. Algal protein fortification of carbohydrate-rich foods
5.4.1. Pastas and noodles
Numerous studies have demonstrated that the incorporation of algae into pasta or noodles significantly enhances its nutritional value. Specifically, improvements in protein and amino acid profile (191, 192), lipid profile (192, 193), minerals (192, 194) and antioxidants (195) have been documented.
Incorporating seaweeds such as sea lettuce (U. lactuca), nori (P. tenera), and wakame (U. pinnatifida) into pasta, despite a low supplementation level of 3%, resulted in noticeable color changes, shorter cooking times, and texture modifications, including decreased hardness and increased adhesiveness and resilience. These additions also increased the protein content and soluble fiber of the pasta (194).
Studies investigating microalgae supplementation into pasta production include Spirulina (A. platensis), D. salina (196), C. vulgaris (191), I. galbana and Diacronema vlkianum (D. vlkianum) (193). Spirulina supplementation at concentrations between 7 and 12.5% yields pastas with darker, greener colors but higher consumer acceptance scores (197–199). Concentrations exceeding 15%, however, impact the sensory attributes of the pasta (199, 200).
Taken altogether, approximately 10% microalgae supplementation represents an optimal concentration for nutritional enhancement without hindering sensory acceptability. However, it is noteworthy that the algae species selection significantly influences the taste and hence consumer preference, as observed for a perceived seafood taste caused by inclusion of I. galbana and D. vlkianum despite no other changes in sensory perception (193).
The incorporation of microalgae into noodles, however, has shown lower acceptability. There have been commercial activities to add Spirulina into noodles (201) including instant noodles (202), with quantities ranging from 0.1 to 2%. It was reported that the noodles were blue-green in color with a mild seafood taste and favorable mouthfeel (201). However, incorporating higher quantities of microalgae (2 to 8% A. platensis), has been associated with decreased overall acceptability (192). Within the range of 2 to 4% Spirulina (A. platensis) supplementation, acceptability scores did not exhibit significant disparities. However, as the proportion of Spirulina (A. platensis) exceeded 4%, there was a proportional decline in the overall acceptability of the noodle products (192).
5.4.2. Bakery products
5.4.2.1. Bread
Various attempts have been made to enhance the nutritional value of bread by incorporating microalgae at different concentrations. These efforts have resulted in improved nutritional quality, particularly in terms of increased protein content, minerals, and ash content (203–208). Different effects were reported for the levels of macronutrients such as fat, crude fiber, and carbohydrates, and compounds such as carotenoids and luteins, depending on the strain and concentration used (205–208).
Algal biomass pigments, such as chlorophylls and carotenoids, have a significant impact on the color of the fortified bread (205–208). This might be perceived negatively by consumers due to the unexpected color change in a traditionally pale product like bread. All sensory evaluations showed that a lower percentage (1 to 2%) of microalgae-supplemented bread was preferred in terms of color, compared to more enriched bread (3 to 5%) (205, 206, 208).
In terms of taste profile, there is no significant difference between bread with 1 and 4% Spirulina (species not specified) (206). Higher supplementation of Spirulina (10%) results in a stronger algal flavor in the bread which was overall, considered satisfactory (204).
In terms of bread rheology, only C. vulgaris has reported positive effects on dough rheology and viscoelastic characteristics of wheat flour bread when added at 1% (209) to 3% (210). Higher concentrations (5%) of microalgae resulted in negative effects on dough rheology, bread texture, and flavor (210). Cell wall disruption of C. vulgaris prior to incorporation did not significantly alter the viscoelastic characteristics, but improved the firmness of bread, suggesting positive effects of microalgae pre-treatment on bread texture (209).
Adding 1 and 3% Spirulina (A. fusiformis) biomass diluted gluten and starch in the dough, affecting the volume of bread (205). Addition of 12% of three different microalgae species Nannochloropsis gaditana (N. gaditana), T. chui, and C. vulgaris resulted in weaker dough strength, decreased bread volume, and increased crumb firmness (205). Nevertheless, all of these was improved if the biomass was pre-treated with ethanol (211). Ethanol pre-treatment reduced unpleasant smells and intense colors associated with the microalgae, but it should be noted that it affects overall protein digestibility negatively (211). Among microalgae species, N. gaditana combined with ethanol treatment exhibited the best effects for protein fortification of wheat bread (211).
Another study involving 79 sensory panelists revealed that incorporating 1% (w/w) A. nodosum into whole meal bread was acceptable (129). In the same study, Hall et al. conducted a crossover trial comparing the post-prandial effects, in which participants who consumed seaweed-enriched bread experienced significantly reduced energy intake (16.4%) in the next meal (129), suggesting that even a low incorporation rate of 1% seaweed in bread can have satiety-promoting effects (Table 1).
Besides nutrient fortification, fortification of seaweed for bioactive purposes has been investigated. Previous research found that adding 4% P. palmata protein hydrolysate to wheat bread retained its renin inhibitory bioactivity even after the baking process but did not significantly affect its texture or sensory properties, highlighting a potential for baked products to deliver bioactive protein hydrolysates (212).
5.4.2.2. Gluten-free bread
Algal supplementation has a positive influence on gluten-free bread, as opposed to substitution with plant-based, non-wheat proteins such as peas, potatoes, and zein isolates, which have been demonstrated to impair gluten-aggregation and bread properties (213, 214). Up to 4% Spirulina (A. platensis) supplementation in rice flour did not affect or even increase the volume and firmness of the bread (203, 206). But when 5% Spirulina (A. platensis) was added into bread flour, a 22% reduction in bread volume and a 113% increase in bread hardness was reported (203).
Two studies independently showed favorable results using N. gaditana in gluten-free bread (207, 215). Khemiri et al. (207) compared the effect of N. gaditana L2 and Chlamydomonas sp. EL5 incorporated into gluten-free bread and reported improved structure, nutritional value, and sensory evaluation (207). The 3% N. gaditana L2 bread received the highest score for overall purchase intention, despite the noticeable color changes (207). Qazi et al. compared the effect of adding 4% T. chui, C. vulgaris, or N. gaditana in gluten-free bread (215). Similarly, N. gaditana biomass with ethanol treatment produced gluten-free bread with improved technofunctional properties and sensory properties, making it a potential candidate for functional gluten-free bread development (215).
Brown seaweed biomass A. nodosum, when included in gluten-free bread ranging from 2 to 4%, resulted in favorable results, such as larger volume, improved texture, and increased antioxidant activity compared to the control bread (216). Further increasing the seaweed biomass resulted in undesirable changes in color, hardness, taste, and bread volume (216).
5.4.2.3. Patisseries
A study conducted by Massoud et al. (217) evaluated the impact of incorporating Spirulina (A. platensis) biomass into croissants at concentrations ranging from 0.5 to 1.5%. Results revealed several positive effects of Spirulina (A. platensis) fortification such as improved textural and organoleptic properties of the croissants, including increased protein and moisture levels, as well as water-holding capacity, which enhanced their overall quality (217). Notably, the study indicated that optimal sensory results were obtained when Spirulina (A. platensis) was incorporated at a concentration of 1% (217).
Khosravi-Darani et al. (218) conducted a study to explore the effects of Spirulina (A. platensis) addition on strudels at different levels (0 to 1.5% w/w). The results demonstrated that the enriched strudels had higher protein content and reduced peroxide value, as well as better color stability over a 45-day period. The sensory analysis indicated that the addition of 0.5 and 1% Spirulina (A. platensis) resulted in the most preferred texture and color.
Another study by Niccolai et al. (219) investigated the influence of Spirulina (A. platensis) biomass (2, 6, and 10% w/w) incorporation in “crostini,” a leavened bakery product commonly consumed in Italy and other parts of Europe. Results showed that the crostini doughs with Spirulina (A. platensis) reached a slightly lower, but still appropriate, volume after fermentation compared to the control. Furthermore, crostini fortified with 6 and 10% Spirulina (A. platensis) exhibited significantly higher protein content, antioxidant capacity and phenolic content, but slightly lower in vitro digestion.
5.4.2.4. Cookies and biscuits
Incorporating 4% Spirulina (A. platensis) into biscuits led to increased hardness and crispiness levels, and the highest sensory color scores (220). However, substituting wheat flour with 2% C. vulgaris biomass revealed no noticeable impact on the texture of wheat cookies, but resulted in the highest acceptance score. However, when the C. vulgaris content was increased to 6%, a significant rise in hardness was observed (221). In 4 species investigated, including Spirulina (A. platensis), C. vulgaris, Tetraselmis suecica, and P. tricornutum, 2% microalgal biomass had a significant impact on smell, taste, and overall acceptability (221). This effect was attributed to the presence of sulfuric compounds, diketones, α-ionone, and β-ionone (221). It is noteworthy that the addition of up to 6% C. vulgaris without affecting the overall sensorial properties was possible when the biomass was defatted (222). In fact, it was reported that the aroma of the cookies remained acceptable even with a 9% incorporation of defatted biomass (222).
From a nutritional perspective, incorporating algal biomass into cookies and biscuits increased the protein content, phenolic content, and antioxidant potential (220, 221). According to Batista et al., cookies prepared with C. vulgaris and A. platensis exhibited higher protein content compared to T. suecica and P. tricornutum (221). No significant difference in in vitro digestibility was found between microalgae cookies and the control (221).
5.4.3. Snacks
The dried biomass of E. gracilis has been explored for use in various food products for its rich β-glucan polysaccharides, including in grains, grain-based products, breakfast items, granola, and protein bars, as reviewed in (223). Its inclusion in these food items can contribute to their nutritional profile and provide the benefits associated with β-glucan polysaccharides.
Homemade roasted seaweed snacks, prepared from raw dried seaweed sheets, have gained popularity as a healthy, cost-effective alternative to packaged seaweed snacks. These snacks offer a convenient and nutritious option for consumers seeking a homemade, additive-free alternative.
Lucas et al. (224) developed snacks enriched with 2 and 6% Spirulina, which resulted in significant increases in protein content, and increased stability of the snacks during a 30-day storage period.
Da Silva et al. (225) conducted a study to examine the antioxidative properties of Spirulina in the context of snack production. The study compared the intact, hydrolysate, and hydrolyzed peptide fractions (<4 kDa or > 4 kDa) of Spirulina, evaluating their antioxidant potential and thermal stability when added to extruded snacks. The results demonstrated that snacks fortified with 2% bioactive peptides derived from Spirulina exhibited higher antioxidant activity, presenting a highly viable natural option to make functional food.
Incorporating 4% Spirulina (A. platensis) biomass into white chocolate significantly enhanced its nutritional value (226). Despite these changes, sensory scores did not show significant differences between nutrient-enriched products and control chocolate (226). These findings highlight the potential of Spirulina (A. platensis) biomass as a valuable enrichment ingredient for white chocolate production.
5.5. Algal supplementation in liquid food and beverages
Microalgae biomass, such as Spirulina, Chlorella, or Tetraselmis (all species not specified), when incorporated into a broccoli-based soup at concentrations ranging from 0.5 to 2.0%, have been found to increase viscosity, antioxidant capacity, and phenolic content of the soup (227). The most accepted formulation was the one containing 0.5% Tetraselmis, though increasing the percentage resulted in reduced sensorial acceptability compared to the control (227).
Another study involved the incorporation of Spirulina (A. platensis) in kefir and found supplementation with 0.05 and 0.1% Spirulina (A. platensis) to be most favorable, with these concentrations mildly elevating the protein content from 27 to 37 mg/mL (228). A more pronounced increase in amino acid content was observed in kefir enhanced with 1% Spirulina (A. platensis) (228).
A Dutch startup, Ful, has developed a bright blue soda made with Spirulina (species not specified), a blue-green algae, which is marketed as a nutritious and environmentally sustainable product with a reduced carbon footprint and which actively captures CO2 into ingestible nutrients (229).
Researchers have measured the antioxidant activity and total phenolic content of various juices containing Spirulina (A. platensis), such as apple juice, Japanese quince syrup, and cranberry syrup, and discovered that the resulting products have at least the same antioxidant activity as pure Spirulina (A. platensis) and a longer shelf life (230), indicating their potential for juice incorporation.
5.5.1. Milk and dairy products
Algae have been used as an additive for milk and yoghurt to enhance the viability of probiotics (231). Beheshtipour et al. (231) found that adding C. vulgaris at concentrations of ≤1.0% resulted in increased acidity and redox potential, without affecting pH levels significantly. However, sensory attributes, including oral texture, mouthfeel, appearance, and non-oral texture, were negatively affected (231). These findings suggest that microalgae addition into milk and dairy products may pose challenges in terms of palatability.
Incorporating microalgae into cheese has positive effects on nutritional values and product outcomes. Mohamed et al. (232) demonstrated that adding C. vulgaris at various concentrations (1 to 3% w/w) increased firmness and decreased oil separation indexes of cheese. Tohamy et al. (233) found that increasing C. vulgaris (2 to 6% w/w) led to an increase in pH and meltability of the spread cheeses compared to the control sample. In general, all supplementation reported better nutritional attributes in the product (232–234).
Similar conclusions were reached in a recent review article discussing the impact of adding microalgal biomass, such as C. vulgaris and A. platensis, on the composition, properties, texture, and sensory profile of dairy products like yogurt, cheese, and ice cream (235). The literature review reveals that increasing microalga content decreases yogurt palatability. In ice cream, microalgal biomass reduces melting time and replaces artificial dyes with stable natural ones. Cheese with microalgae has enhanced antioxidant capacity due to high phenolic and carotenoid contents. However, the incorporation of microalgae negatively affects the sensory attributes of these products, a major challenge that requires further research to strike a balance between improved nutrition and sensory quality (235).
Another noteworthy advancement is the use of concentrated microalgae flour to generate lactose-free plant-based milk with a nutritional profile comparable to that of cow’s milk and high-quality EAA (236, 237). In contrast to past efforts that added algal-based ingredients as a supplement or enhancement, this product is a pure algal-based dairy substitute created from microalgal-based protein-rich flour. The protein-rich flour can be modified by altering the ratio of water-soluble microalgae flour, allowing for variations in protein content and enhancing textural and sensory aspects, which has led to the development of microalgae-based vegan ice creams, yoghurts, and cheeses (238).
5.5.2. Beer
The marine species T. chui, renowned for its starch accumulation capabilities, has been studied as a potential active ingredient in beer brewing. Incorporating T. chui into the brewing process has the potential to influence the flavor profiles of beers as the compounds derived from microalgae can contribute to the complexity and uniqueness of flavors, providing consumers with novel sensory experiences. However, comprehensive studies investigating the specific flavor compounds and their impact on beer characteristics are still limited (239). Notably, one significant advantage of algal supplementation in beer brewing is its potential for carbon capture, which reduces their carbon footprint and contributes to climate change mitigation. Young Henrys, a craft brewery in Sydney, Australia, has been at the forefront of this approach and is credited as the first brewery to use algae for capturing CO2 during fermentation (240, 241).
5.6. Considerations when incorporating algae into future foods
Algae and its ingredients have demonstrated nutritional-enhancing properties, particularly in boosting protein content and antioxidant activity in food preservation. Multiple studies have found no significant changes in overall acceptability when supplementation was done at low percentages (< 5%) (192, 203, 206, 226), indicating the potential for supplementing into various food types.
However, results on the sensory qualities of greater amounts of algae in supplemented foods are less convincing, primarily due to the strong sensory characteristics that impair the appearance and flavor. In general, studies suggest that incorporating low percentages of algal biomass results in acceptable, and sometimes, preferred products, whereas high percentage incorporation can be undesirable. The optimal balance for carbohydrate-rich foods is struck when supplementing up to 6–7% of algae in biscuits, cookies (even up to 9%, if defatted), and snacks, as well as up to 12.5% for pasta. These percentages have been reported without compromising sensory evaluations, indicating potential for further research in carbohydrate-rich food.
Even if sensory evaluation might be compromised, low percentage of biomass incorporation can enhance textural, organoleptic, and rheological properties, rendering the final products acceptable. Regarding the rheology of baked goods, it is reported that algae fortification above 5% increases the hardness of wheat products (209, 221). Similarly, Sahni et al. reported that defatted C. vulgaris rendered flour blends with decreased pasting viscosities, which makes it suitable for cookies, but not for bread (222). Overall, this duality between sensory appeal and techno-functionality underpins the feasibility of incorporating algal biomass into carbohydrate staples such as pastas, noodles, cookies, snacks, and biscuits. For protein-rich food fortification and food innovations, algae improve the nutrition and sensory attributes of meat, seafood, and their corresponding animal-free analogs. Algae’s strong seafood attribute makes it an excellent seasoning and preservation agent in canned seafood. In contrast, adding algal biomass into milk and dairy products changes the texture, color, and taste which may result in less appetizing qualities.
5.7. Priorities for future research
Future research should focus on several key areas to fully unlock the potential utilization of microalgal and seaweed proteins for human nutrition. Firstly, optimization of sensory attributes to improve product acceptability remains a critical issue. Consumer’s perceptions of algae-based products are largely shaped by their unique taste and texture, which deviate from traditional food ingredients. Consequently, choosing the right cultivars and/or modifying growth conditions as well as modifying processing methods that fine-tune these sensory attributes are pivotal for enhancing product acceptability.
For instance, research by Caporgno et al. indicates that cultivating A. protothecoides under mixotrophic and heterotrophic conditions leads to reduced chlorophyll accumulation, thus generating a biomass with a less intense green hue (184). This is potentially more appealing to consumers who may find vibrant colors off-putting. Additionally, such biomass demonstrated superior emulsion properties compared to its phototrophically-produced counterparts.
From our observations, the continued success of algal supplementation hinges on efforts to customize and calibrate appropriate algal biomass for specific food applications. This entails not just modifying cultivation and processing methods but also selecting the appropriate strains and cultivars. Such tailored usage of algae should target to improve the acceptability of the final product as well as enhance the bioavailability of beneficial components, promoting improved health outcomes. Henceforth, it is imperative to prioritize the study of algal biology to enhance our comprehension of algae metabolism and facilitate advancements in biomass technologies. Subsequent to cultivation, downstream processing methods are able to further refine algae’s organoleptic properties. Numerous studies advocate for advanced processing techniques—such as protein extraction, ethanol treatment, and defatting—to enhance the end-product’s quality over that of crude biomass.
Next, optimization of extraction methods merits further investigation. Simple pre-processing methods like cell disruption can significantly elevate the bioavailability of nutrients, especially in algae strains with hard-to-digest cell walls. Defatting, another pre-processing method, can help remove the undesirable taste of algae. Continued exploration of effective extraction techniques such as ultrasound-assisted, microwave-assisted, and pulsed electric field extraction is necessary to overcome the challenges posed by cell wall polysaccharides. However, it is important to approach such preprocessing with caution, as the disruption of cells might also lead to the loss of some beneficial compounds or increased production cost. Striking the right balance in preprocessing will be an important aspect of future research and development in the field of algal food applications.
Lastly, future research should delve into the spatial and agroecological dimensions of algal farming. Optimizing the nutrient profiles of locally grown algae strains offers significant ecological benefits. A focus on localized cultivation enhances sustainability and allows for tailoring to local ecological conditions. This also streamlines the production process, potentially increasing crop yields and reducing transportation-related emissions. By aligning algae strains with local environmental factors, researchers could unlock avenues for more efficient and environmentally responsible protein production. Such an approach could also stimulate local economies and contribute to community resilience. Therefore, targeted research and planning in the spatial and agroecological aspects of algal farming represent a crucial step toward realizing algae’s full potential as a sustainable and nutritious protein source.
6. Conclusion
Taken together, this review has summarized multiple studies that underscore the potential of algae as a viable, nutritious and sustainable source of protein. Approximately 50% of certain microalgae species and around 25% of red and green seaweeds are constituted from proteins with amino acid profiles comparable to those of conventional protein sources. While there are challenges in extracting protein from algae, these do not preclude their applications in food as their co-nutrients (including dietary fiber, bioactive phytonutrients such as polyphenols and carotenoids) provide extra benefits, such as enhanced satiety and lipid profiles, as shown in human trials. Future trials should prioritize studies that assess the safety and protein bioavailability of algal biomass or protein extracts on humans.
The potential of algae as a prospective food source is rooted in its nutritional composition and associated health advantages, as well as its physicochemical attributes that make it suitable for various food-related purposes. The addition of algal proteins in various food products, such as protein-rich plant-based meats, seafood-related products, as well as hybrid meats and seafoods as well as baked goods, can improve the overall acceptability of the final products, although further efforts are needed to increase consumer acceptance and enjoyment of these products.
In conclusion, the incorporation of algae into our food system has great potential but requires further multidisciplinary investigations. Key areas for future research include the scalability of protein and nutrient extraction methods, localizing algal production focusing on life-cycle analyses, cost, and sustainability. Tailored strain selection and optimized cultivation, along with efficient downstream processing, can significantly improve both the desirability and nutritional quality of algae-derived food products.
Author contributions
JW: Writing – original draft, Writing – review & editing. RT: Writing – original draft, Writing – review & editing. HT: Writing – original draft. SH: Conceptualization, Funding acquisition, Supervision, Writing – review & editing.
Funding
The authors declare financial support was received for the research, authorship, and/or publication of this article. This project was funded by the Singapore-New Zealand Bilateral Program on Future Food and Singapore Institute of Food and Biotechnology Innovation (SIFBI) (Grant ID: A20D3b0072) and by A*STAR under its IAF-ICP funding (Award Number I1701E0011).
Conflict of interest
The authors declare that the research was conducted in the absence of any commercial or financial relationships that could be construed as a potential conflict of interest.
Publisher’s note
All claims expressed in this article are solely those of the authors and do not necessarily represent those of their affiliated organizations, or those of the publisher, the editors and the reviewers. Any product that may be evaluated in this article, or claim that may be made by its manufacturer, is not guaranteed or endorsed by the publisher.
Supplementary material
The Supplementary material for this article can be found online at: https://www.frontiersin.org/articles/10.3389/fnut.2023.1277343/full#supplementary-material
References
1. Van Dijk, M, Morley, T, Rau, ML, and Saghai, Y. A meta-analysis of projected global food demand and population at risk of hunger for the period 2010-2050. Nat Food. (2021) 2:494–501. doi: 10.1038/s43016-021-00322-9
2. Adam, D. How far will global population rise? Researchers can’t agree. Nature. (2021) 597:462–5. doi: 10.1038/d41586-021-02522-6
3. Clark, H, and Wu, H. The sustainable development goals: 17 goals to transform our world: United Nations, (2016).
4. Gephart, JA, Davis, KF, Emery, KA, Leach, AM, Galloway, JN, and Pace, ML. The environmental cost of subsistence: optimizing diets to minimize footprints. Sci Total Environ. (2016) 553:120–7. doi: 10.1016/j.scitotenv.2016.02.050
5. Willett, W, Rockström, J, Loken, B, Springmann, M, Lang, T, Vermeulen, S, et al. Food in the Anthropocene: the EAT–lancet commission on healthy diets from sustainable food systems. Lancet. (2019) 393:447–92. doi: 10.1016/S0140-6736(18)31788-4
6. Guiry, MD. How many species of algae are there? J Phycol. (2012) 48:1057–63. doi: 10.1111/j.1529-8817.2012.01222.x
7. De Mendonca, HV, Assemany, P, Abreu, M, Couto, E, Maciel, AM, Duarte, RL, et al. Microalgae in a global world: new solutions for old problems? Renew Energy. (2021) 165:842–62. doi: 10.1016/j.renene.2020.11.014
8. Klamczynska, B, and Mooney, W. Heterotrophic microalgae: A scalable and sustainable protein source. Sustainable protein sources. Amsterdam, Netherlands: Elsevier, pp. 327–339. (2017).
9. Garnett, T. Food sustainability: problems, perspectives and solutions. Proc Nutr Soc. (2013) 72:29–39. doi: 10.1017/S0029665112002947
10. Greene, CH, and Scott-Buechler, CM. Algal solutions: transforming marine aquaculture from the bottom up for a sustainable future. PLoS Biol. (2022) 20:e3001824. doi: 10.1371/journal.pbio.3001824
11. Greene, CH, Huntley, ME, Archibald, I, Gerber, LN, Sills, DL, Granados, J, et al. Marine microalgae: climate, energy, and food security from the sea. Oceanography. (2016) 29:10–5. doi: 10.5670/oceanog.2016.91
12. Greene, CH, Scott-Buechler, CM, Hausner, AL, Johnson, ZI, Lei, XG, and Huntley, ME. Transforming the future of marine aquaculture: a circular economy approach. Oceanography. (2022) 35:26–34. doi: 10.5670/oceanog.2022.213
13. Smetana, S, Sandmann, M, Rohn, S, Pleissner, D, and Heinz, V. Autotrophic and heterotrophic microalgae and cyanobacteria cultivation for food and feed: life cycle assessment. Bioresour Technol. (2017) 245:162–70. doi: 10.1016/j.biortech.2017.08.113
14. Matassa, S, Boon, N, Pikaar, I, and Verstraete, W. Microbial protein: future sustainable food supply route with low environmental footprint. Microb Biotechnol. (2016) 9:568–75. doi: 10.1111/1751-7915.12369
15. Price, GD, Pengelly, JJ, Forster, B, Du, J, Whitney, SM, von Caemmerer, S, et al. The cyanobacterial CCM as a source of genes for improving photosynthetic CO2 fixation in crop species. J Exp Bot. (2013) 64:753–68. doi: 10.1093/jxb/ers257
16. Tsai, DD, Ramaraj, R, and Chen, PH. Growth condition study of algae function in ecosystem for CO2 bio-fixation. J Photochem Photobiol B. (2012) 107:27–34. doi: 10.1016/j.jphotobiol.2011.11.005
17. Toledo-Cervantes, A, Morales, T, González, Á, Muñoz, R, and Lebrero, R. Long-term photosynthetic CO2 removal from biogas and flue-gas: exploring the potential of closed photobioreactors for high-value biomass production. Sci Total Environ. (2018) 640-641:1272–8. doi: 10.1016/j.scitotenv.2018.05.270
18. Iglina, T, Iglin, P, and Pashchenko, D. Industrial CO2 capture by algae: a review and recent advances. Sustainability. (2022) 319:137987. doi: 10.3390/su14073801
19. Bleakley, S, and Hayes, M. Algal proteins: extraction, application, and challenges concerning production. Foods. (2017) 6:33. doi: 10.3390/foods6050033
20. Dillehay, TD, Ramirez, C, Pino, M, Collins, MB, Rossen, J, and Pino-Navarro, JD. Monte Verde: seaweed, food, medicine, and the peopling of South America. Science. (2008) 320:784–6. doi: 10.1126/science.1156533
21. Mendes, MC, Navalho, S, Ferreira, A, Paulino, C, Figueiredo, D, Silva, D, et al. Algae as food in Europe: an overview of species diversity and their application. Foods. (2022) 11:1871. doi: 10.3390/foods11131871
22. Wells, ML, Potin, P, Craigie, JS, Raven, JA, Merchant, SS, Helliwell, KE, et al. Algae as nutritional and functional food sources: revisiting our understanding. J Appl Phycol. (2017) 29:949–82. doi: 10.1007/s10811-016-0974-5
23. Ramos-Romero, S, Torrella, JR, Pagès, T, Viscor, G, and Torres, JL. Edible microalgae and their bioactive compounds in the prevention and treatment of metabolic alterations. Nutrients. (2021) 13:563. doi: 10.3390/nu13020563
24. Leandro, A, Pereira, L, and Goncalves, AMM. Diverse applications of marine macroalgae. Mar Drugs. (2019) 18:17. doi: 10.3390/md18010017
25. Geada, P, Moreira, C, Silva, M, Nunes, R, Madureira, L, Rocha, CMR, et al. Algal proteins: production strategies and nutritional and functional properties. Bioresour Technol. (2021) 332:125125. doi: 10.1016/j.biortech.2021.125125
26. Kessler, E, and Huss, VA. Comparative physiology and biochemistry and taxonomic assignment of the Chlorella (Chlorophyceae) strains of the culture collection of University of Texas at Austin. J Phycol. (1992) 28:550–3. doi: 10.1111/j.0022-3646.1992.00550.x
27. Bito, T, Okumura, E, Fujishima, M, and Watanabe, F. Potential of Chlorella as a dietary supplement to promote human health. Nutrients. (2020) 12:2524. doi: 10.3390/nu12092524
28. Weber, S, Grande, PM, Blank, LM, and Klose, H. Insights into cell wall disintegration of Chlorella vulgaris. PLoS One. (2022) 17:e0262500. doi: 10.1371/journal.pone.0262500
29. Nowicka-Krawczyk, P, Mühlsteinová, R, and Hauer, T. Detailed characterization of the Arthrospira type species separating commercially grown taxa into the new genus Limnospira (Cyanobacteria). Sci Rep. (2019) 9:694. doi: 10.1038/s41598-018-36831-0
30. AlFadhly, NKZ, Alhelfi, N, Altemimi, AB, Verma, DK, Cacciola, F, and Narayanankutty, A. Trends and technological advancements in the possible food applications of Spirulina and their health benefits: a review. Molecules. (2022) 27:5584. doi: 10.3390/molecules27175584
31. European Parliament Council of the European Union. Regulation (EU) 2015/2283 of the European Parliament and of the council of 25 November 2015 on novel foods, amending regulation (EU) no 1169/2011 of the European Parliament and of the council and repealing regulation (EC) no 258/97 of the European Parliament and of the council and commission regulation (EC) no 1852/2001. Strasbourg, pp. 1–22. (2015).
32. EU Novel Food Catalogue. European commission. Available at: https://webgate.ec.europa.eu/fip/novel_food_catalogue/. (Accessed July 4, 2023).
33. Matos, ÂP, Novelli, E, and Tribuzi, G. Use of algae as food ingredient: sensory acceptance and commercial products. Front. Food Sci. Technol. (2022) 23:989801. doi: 10.3389/frfst.2022.989801
34. Barkia, I, Saari, N, and Manning, SR. Microalgae for high-value products towards human health and nutrition. Mar Drugs. (2019) 17:304. doi: 10.3390/md17050304
35. Metsoviti, MN, Papapolymerou, G, Karapanagiotidis, IT, and Katsoulas, N. Comparison of growth rate and nutrient content of five microalgae species cultivated in greenhouses. Plan Theory. (2019) 8:279. doi: 10.3390/plants8080279
36. Yang, Y, Hassan, SH, Awasthi, MK, Gajendran, B, Sharma, M, Ji, M-K, et al. The recent progress on the bioactive compounds from algal biomass for human health applications. Food. Bioscience. (2022):102267. doi: 10.1016/j.fbio.2022.102267
37. Brien, RO, Hayes, M, Sheldrake, G, Tiwari, B, and Walsh, P. Macroalgal proteins: a review. Foods. (2022) 11:571. doi: 10.3390/foods11040571
38. Thiviya, P, Gamage, A, Gama-Arachchige, NS, Merah, O, and Madhujith, T. Seaweeds as a source of functional proteins. Phycology. (2022) 2:216–43. doi: 10.3390/phycology2020012
39. Rajapakse, N, and Kim, S-K. Nutritional and digestive health benefits of seaweed. Adv Food Nutr Res. (2011) 64:17–28. doi: 10.1016/B978-0-12-387669-0.00002-8
40. Ito, K, and Hori, K. Seaweed: chemical composition and potential food uses. Food Rev Intl. (1989) 5:101–44. doi: 10.1080/87559128909540845
41. Rawiwan, P, Peng, Y, Paramayuda, IGPB, and Quek, SY. Red seaweed: a promising alternative protein source for global food sustainability. Trends Food Sci Technol. (2022) 123:37–56. doi: 10.1016/j.tifs.2022.03.003
42. Fleurence, J. Seaweed proteins: biochemical, nutritional aspects and potential uses. Trends Food Sci Technol. (1999) 10:25–8. doi: 10.1016/S0924-2244(99)00015-1
43. Arasaki, S, and Arasaki, T. Low calorie, high nutrition vegetables from the sea to help you look and feel. Better: Japan Publications (1983). 196 p.
44. Admassu, H, Abera, T, Abraha, B, Yang, R, and Zhao, W. Proximate, mineral and amino acid composition of dried laver (Porphyra spp.) seaweed. J Acad Ind Res. (2018) 6:149.
45. Fujiwara-Arasaki, T, Mino, N, and Kuroda, M. The protein value in human nutrition of edible marine algae in Japan. Eleventh International Seaweed Symposium: Proceedings of the Eleventh International Seaweed Symposium, held in Qingdao, People’s Republic of China, June 19–25, Springer, 1983. (1984).
46. Niemi, C, Mortensen, AM, Rautenberger, R, Matsson, S, Gorzsas, A, and Gentili, FG. Rapid and accurate determination of protein content in North Atlantic seaweed by NIR and FTIR spectroscopies. Food Chem. (2023) 404:134700. doi: 10.1016/j.foodchem.2022.134700
47. Lourenço, SO, Barbarino, E, De-Paula, JC, Pereira, LOS, and Marquez, UML. Amino acid composition, protein content and calculation of nitrogen-to-protein conversion factors for 19 tropical seaweeds. Phycol Res. (2002) 50:233–41. doi: 10.1111/j.1440-1835.2002.tb00156.x
48. Angell, AR, Mata, L, de Nys, R, and Paul, NA. The protein content of seaweeds: a universal nitrogen-to-protein conversion factor of five. J Appl Phycol. (2016) 28:511–24. doi: 10.1007/s10811-015-0650-1
49. Rupérez, P, and Saura-Calixto, F. Dietary fibre and physicochemical properties of edible Spanish seaweeds. Eur Food Res Technol. (2001) 212:349–54. doi: 10.1007/s002170000264
50. Campos, BM, Ramalho, E, Marmelo, I, Noronha, JP, Malfeito-Ferreira, M, Mata, P, et al. Proximate composition, physicochemical and microbiological characterization of edible seaweeds available in the Portuguese market. Frontiers in Bioscience-Elite. (2022) 14:26. doi: 10.31083/j.fbe1404026
51. Wahlström, N, Harrysson, H, Undeland, I, and Edlund, U. A strategy for the sequential recovery of biomacromolecules from red macroalgae Porphyra umbilicalis Kutzing. Ind Eng Chem Res. (2018) 57:42–53. doi: 10.1021/acs.iecr.7b03768
52. Xu, N, Wang, W, Xu, K, Xu, Y, Ji, D, Chen, C, et al. Cultivation of different seaweed species and seasonal changes cause divergence of the microbial community in coastal seawaters. Front Microbiol. (2022) 13:988743. doi: 10.3389/fmicb.2022.1063425
53. Bak, UG, Nielsen, CW, Marinho, GS, Gregersen, Ó, Jónsdóttir, R, and Holdt, SL. The seasonal variation in nitrogen, amino acid, protein and nitrogen-to-protein conversion factors of commercially cultivated Faroese Saccharina latissima. Algal Res. (2019) 42:101576. doi: 10.1016/j.algal.2019.101576
54. Janssen, M, Wijffels, RH, and Barbosa, MJ. Microalgae based production of single-cell protein. Curr Opin Biotechnol. (2022) 75:102705. doi: 10.1016/j.copbio.2022.102705
55. Xie, T, Xia, Y, Zeng, Y, Li, X, and Zhang, Y. Nitrate concentration-shift cultivation to enhance protein content of heterotrophic microalga Chlorella vulgaris: over-compensation strategy. Bioresour Technol. (2017) 233:247–55. doi: 10.1016/j.biortech.2017.02.099
56. Xiao, X, Zhou, Y, Liang, Z, Lin, R, Zheng, M, Chen, B, et al. A novel two-stage heterotrophic cultivation for starch-to-protein switch to efficiently enhance protein content of Chlorella sp. MBFJNU-17. Bioresour Technol. (2022) 344:126187. doi: 10.1016/j.biortech.2021.126187
57. Caporgno, MP, and Mathys, A. Trends in microalgae incorporation into innovative food products with potential health benefits. Front Nutr. (2018) 5:58. doi: 10.3389/fnut.2018.00058
58. Lupatini, AL, Colla, LM, Canan, C, and Colla, E. Potential application of microalga Spirulina platensis as a protein source. J Sci Food Agric. (2017) 97:724–32. doi: 10.1002/jsfa.7987
59. Wang, Y, Tibbetts, SM, and McGinn, PJ. Microalgae as sources of high-quality protein for human food and protein supplements. Foods. (2021) 10:3002. doi: 10.3390/foods10123002
60. Volkmann, H, Imianovsky, U, Oliveira, JL, and Sant'Anna, ES. Cultivation of Arthrospira (Spirulina) platensis in desalinator wastewater and salinated synthetic medium: protein content and amino-acid profile. Braz J Microbiol. (2008) 39:98–101. doi: 10.1590/S1517-83822008000100022
61. FoodData Central. US Department of Agriculture, Agricultural Research Service. (2019). Available at: fdc.nal.usda.gov. (Accessed July 7, 2023).
62. Day, L, Cakebread, JA, and Loveday, SM. Food proteins from animals and plants: differences in the nutritional and functional properties. Trends Food Sci Technol. (2022) 119:428–42. doi: 10.1016/j.tifs.2021.12.020
63. Gaudichon, C, and Calvez, J. Determinants of amino acid bioavailability from ingested protein in relation to gut health. Curr Opin Clin Nutr Metab Care. (2021) 24:55. doi: 10.1097/MCO.0000000000000708
64. Lopez, MJ, and Mohiuddin, SS. Biochemistry, essential amino acids Treasure Island. Florida: StatPearls Publishing (2020).
65. Food and Agriculture Organization. Dietary protein quality evaluation in human nutrition: report of an FAO expert consultation. FAO Food Nutr Pap. (2013) 92:1–66.
66. Mariotti, F, and Gardner, CD. Dietary protein and amino acids in vegetarian diets-a review. Nutrients. (2019) 11:2661. doi: 10.3390/nu11112661
67. World Health Organization. Protein and amino acid requirements in human nutrition. Geneva: World Health Organization (2007).
68. Young, VR, Wagner, DA, Burini, R, and Storch, KJ. Methionine kinetics and balance at the 1985 FAO/WHO/UNU intake requirement in adult men studied with L-[2H3-methyl-1-13C] methionine as a tracer. Am J Clin Nutr. (1991) 54:377–85. doi: 10.1093/ajcn/54.2.377
69. Andri, F, Dono, ND, Sasongko, H, and Zuprizal, Z. The effects of dietary seaweed inclusion on growth performance of broiler chickens: a systematic review and meta-analysis. F1000Res. (2020) 9:1087. doi: 10.12688/f1000research.25726.1
70. Ventura, M, Castanon, J, and McNab, J. Nutritional value of seaweed (Ulva rigida) for poultry. Anim Feed Sci Technol. (1994) 49:87–92. doi: 10.1016/0377-8401(94)90083-3
71. Abudabos, AM, Okab, AB, Aljumaah, RS, Samara, EM, Abdoun, KA, and Al-Haidary, AA. Nutritional value of green seaweed (Ulva lactuca) for broiler chickens. Ital J Anim Sci. (2013) 12:e28. doi: 10.4081/ijas.2013.e28
72. Nhlane, LT, Mnisi, CM, Mlambo, V, and Madibana, MJ. Effect of seaweed-containing diets on visceral organ sizes, carcass characteristics, and meat quality and stability of Boschveld indigenous hens. Poult Sci. (2021) 100:949–56. doi: 10.1016/j.psj.2020.11.038
73. El-Deek, A, and Brikaa, AM. Effect of different levels of seaweed in starter and finisher diets in pellet and mash form on performance and carcass quality of ducks. Int J Poult Sci. (2009) 8:1014–21. doi: 10.3923/ijps.2009.1014.1021
74. Katayama, M, Fukuda, T, Okamura, T, Suzuki, E, Tamura, K, Shimizu, Y, et al. Effect of dietary addition of seaweed and licorice on the immune performance of pigs. Anim Sci J. (2011) 82:274–81. doi: 10.1111/j.1740-0929.2010.00826.x
75. Dierick, N, Ovyn, A, and De Smet, S. Effect of feeding intact brown seaweed Ascophyllum nodosum on some digestive parameters and on iodine content in edible tissues in pigs. J Sci Food Agric. (2009) 89:584–94. doi: 10.1002/jsfa.3480
76. Venardou, B, O'Doherty, JV, Maher, S, Ryan, MT, Gath, V, Ravindran, R, et al. Potential of a fucoidan-rich Ascophyllum nodosum extract to reduce Salmonella shedding and improve gastrointestinal health in weaned pigs naturally infected with Salmonella. J Anim Sci Biotechnol. (2022) 13:39. doi: 10.1186/s40104-022-00685-4
77. De Bhowmick, G, and Hayes, M. In vitro protein digestibility of selected seaweeds. Foods. (2022) 11:289. doi: 10.3390/foods11030289
78. Spínola, MP, Costa, MM, and Prates, JA. Digestive constraints of Arthrospira platensis in poultry and swine feeding. Foods. (2022) 11:2984. doi: 10.3390/foods11192984
79. Wild, KJ, Steingaß, H, and Rodehutscord, M. Variability in nutrient composition and in vitro crude protein digestibility of 16 microalgae products. J Anim Physiol Anim Nutr. (2018) 102:1306–19. doi: 10.1111/jpn.12953
80. Teuling, E, Wierenga, PA, Agboola, JO, Gruppen, H, and Schrama, JW. Cell wall disruption increases bioavailability of Nannochloropsis gaditana nutrients for juvenile Nile tilapia (Oreochromis niloticus). Aquaculture. (2019) 499:269–82. doi: 10.1016/j.aquaculture.2018.09.047
81. Kose, A, Ozen, MO, Elibol, M, and Oncel, SS. Investigation of in vitro digestibility of dietary microalga Chlorella vulgaris and cyanobacterium Spirulina platensis as a nutritional supplement. 3 Biotech. (2017) 7:170. doi: 10.1007/s13205-017-0832-4
82. Kim, MS, Kim, JY, Choi, WH, and Lee, SS. Effects of seaweed supplementation on blood glucose concentration, lipid profile, and antioxidant enzyme activities in patients with type 2 diabetes mellitus. Nutr Res Pract. (2008) 2:62–7. doi: 10.4162/nrp.2008.2.2.62
83. Huang, W, Tan, H, and Nie, S. Beneficial effects of seaweed-derived dietary fiber: highlights of the sulfated polysaccharides. Food Chem. (2022) 373:131608. doi: 10.1016/j.foodchem.2021.131522
84. Hentati, F, Tounsi, L, Djomdi, D, Pierre, G, Delattre, C, Ursu, AV, et al. Bioactive polysaccharides from seaweeds. Molecules. (2020) 25:3152. doi: 10.3390/molecules25143152
85. Ran, W, Wang, H, Liu, Y, Qi, M, Xiang, Q, Yao, C, et al. Storage of starch and lipids in microalgae: biosynthesis and manipulation by nutrients. Bioresour Technol. (2019) 291:121894. doi: 10.1016/j.biortech.2019.121894
86. Admassu, H, Gasmalla, MAA, Yang, R, and Zhao, W. Bioactive peptides derived from seaweed protein and their health benefits: antihypertensive, antioxidant, and antidiabetic properties. J Food Sci. (2018) 83:6–16. doi: 10.1111/1750-3841.14011
87. De Jesus Raposo, MF, De Morais, RM, and De Morais, AM. Health applications of bioactive compounds from marine microalgae. Life Sci. (2013) 93:479–86. doi: 10.1016/j.lfs.2013.08.002
88. Mišurcová, L, Ambrožová, J, and Samek, D. Seaweed lipids as nutraceuticals. Adv Food Nutr Res. (2011) 64:339–55. doi: 10.1016/B978-0-12-387669-0.00027-2
89. Jaworowska, A, and Murtaza, A. Seaweed derived lipids are a potential anti-inflammatory agent: a review. Int J Environ Res Public Health. (2022) 20:730. doi: 10.3390/ijerph20010730
90. Park, E, Yu, H, Lim, JH, Hee Choi, J, Park, KJ, and Lee, J. Seaweed metabolomics: a review on its nutrients, bioactive compounds and changes in climate change. Food Res Int. (2023) 163:112221. doi: 10.1016/j.foodres.2022.112221
91. Jagtap, AS, Sankar, NPV, Ghori, RI, and Manohar, CS. Marine microbial enzymes for the production of algal oligosaccharides and its bioactive potential for application as nutritional supplements. Folia Microbiol (Praha). (2022) 67:175–91. doi: 10.1007/s12223-021-00943-4
92. Pereira, AG, Otero, P, Echave, J, Carreira-Casais, A, Chamorro, F, Collazo, N, et al. Xanthophylls from the sea: algae as source of bioactive carotenoids. Mar Drugs. (2021) 19:188. doi: 10.3390/md19040188
93. Mišurcová, L, Škrovánková, S, Samek, D, Ambrožová, J, and Machů, L. Health benefits of algal polysaccharides in human nutrition. Adv Food Nutr Res. (2012) 66:75–145. doi: 10.1016/B978-0-12-394597-6.00003-3
94. Spolaore, P, Joannis-Cassan, C, Duran, E, and Isambert, A. Commercial applications of microalgae. J Biosci Bioeng. (2006) 101:87–96. doi: 10.1263/jbb.101.87
96. Bonfanti, C, Cardoso, C, Afonso, C, Matos, J, Garcia, T, Tanni, S, et al. Potential of microalga Isochrysis galbana: bioactivity and bioaccessibility. Algal Res. (2018) 29:242–8. doi: 10.1016/j.algal.2017.11.035
97. Rauma, AL, Torronen, R, Hanninen, O, and Mykkanen, H. Vitamin B-12 status of long-term adherents of a strict uncooked vegan diet (“living food diet”) is compromised. J Nutr. (1995) 125:2511–5. doi: 10.1093/jn/125.10.2511
98. Dagnelie, PC, Van Staveren, WA, and Van den Berg, H. Vitamin B-12 from algae appears not to be bioavailable. Am J Clin Nutr. (1991) 53:695–7. doi: 10.1093/ajcn/53.3.695
99. Watanabe, F, Takenaka, S, Kittaka-Katsura, H, Ebara, S, and Miyamoto, E. Characterization and bioavailability of vitamin B12-compounds from edible algae. J Nutr Sci Vitaminol (Tokyo). (2002) 48:325–31. doi: 10.3177/jnsv.48.325
100. Yamada, S, Shibata, Y, Takayama, M, Narita, Y, Sugawara, K, and Fukuda, M. Content and characteristics of vitamin B12 in some seaweeds. J Nutr Sci Vitaminol (Tokyo). (1996) 42:497–505. doi: 10.3177/jnsv.42.497
101. Anantharajappa, K, Dharmesh, SM, and Ravi, S. Gastro-protective potentials of Spirulina: role of vitamin B(12). J Food Sci Technol. (2020) 57:745–53. doi: 10.1007/s13197-019-04107-8
102. Madhubalaji, CK, Rashmi, V, Chauhan, VS, Shylaja, MD, and Sarada, R. Improvement of vitamin B(12) status with Spirulina supplementation in Wistar rats validated through functional and circulatory markers. J Food Biochem. (2019) 43:e13038. doi: 10.1111/jfbc.13038
103. Watanabe, F. Vitamin B12 sources and bioavailability. Exp Biol Med (Maywood). (2007) 232:1266–74. doi: 10.3181/0703-MR-67
104. Watanabe, F, Katsura, H, Takenaka, S, Fujita, T, Abe, K, Tamura, Y, et al. Pseudovitamin B(12) is the predominant cobamide of an algal health food, spirulina tablets. J Agric Food Chem. (1999) 47:4736–41. doi: 10.1021/jf990541b
105. Takenaka, S, Sugiyama, S, Ebara, S, Miyamoto, E, Abe, K, Tamura, Y, et al. Feeding dried purple laver (nori) to vitamin B12-deficient rats significantly improves vitamin B12 status. Br J Nutr. (2001) 85:699–703. doi: 10.1079/BJN2001352
106. Miyamoto, E, Watanabe, F, Ebara, S, Takenaka, S, Takenaka, H, Yamaguchi, Y, et al. Characterization of a vitamin B12 compound from unicellular coccolithophorid alga (Pleurochrysis carterae). J Agric Food Chem. (2001) 49:3486–9. doi: 10.1021/jf0101556
107. Tan, Y, Zhou, H, and McClements, DJ. Application of static in vitro digestion models for assessing the bioaccessibility of hydrophobic bioactives: a review. Trends Food Sci Technol. (2022) 2022:28. doi: 10.1016/j.tifs.2022.02.028
108. Demarco, M, De Moraes, JO, Matos, ÂP, Derner, RB, De Farias, NF, and Tribuzi, G. Digestibility, bioaccessibility and bioactivity of compounds from algae. Trends Food Sci Technol. (2022) 2022:4. doi: 10.1016/j.tifs.2022.02.004
109. Hernandez-Hernandez, O, Olano, A, Rastall, RA, and Moreno, FJ. In vitro digestibility of dietary carbohydrates: toward a standardized methodology beyond amylolytic and microbial enzymes. Front Nutr. (2019) 6:61. doi: 10.3389/fnut.2019.00061
110. Guo, Y, Chen, X, Gong, P, Chen, F, Cui, D, and Wang, M. Advances in the in vitro digestion and fermentation of polysaccharides. Int J Food Sci Technol. (2021) 56:4970–82. doi: 10.1111/ijfs.15308
111. Ren, Y, Sun, H, Deng, J, Huang, J, and Chen, F. Carotenoid production from microalgae: biosynthesis, salinity responses and novel biotechnologies. Mar Drugs. (2021) 19:713. doi: 10.3390/md19120713
112. Sathasivam, R, and Ki, JS. A review of the biological activities of microalgal carotenoids and their potential use in healthcare and cosmetic industries. Mar Drugs. (2018) 16:26. doi: 10.3390/md16010026
113. Coesel, SN, Baumgartner, AC, Teles, LM, Ramos, AA, Henriques, NM, Cancela, L, et al. Nutrient limitation is the main regulatory factor for carotenoid accumulation and for Psy and Pds steady state transcript levels in Dunaliella salina (Chlorophyta) exposed to high light and salt stress. Mar Biotechnol. (2008) 10:602–11. doi: 10.1007/s10126-008-9100-2
114. Novoveska, L, Ross, ME, Stanley, MS, Pradelles, R, Wasiolek, V, and Sassi, JF. Microalgal carotenoids: a review of production, current markets, regulations, and future direction. Mar Drugs. (2019) 17:640. doi: 10.3390/md17110640
115. Wichuk, K, Brynjolfsson, S, and Fu, W. Biotechnological production of value-added carotenoids from microalgae: emerging technology and prospects. Bioengineered. (2014) 5:204–8. doi: 10.4161/bioe.28720
116. Cichoński, J, and Chrzanowski, G. Microalgae as a source of valuable phenolic compounds and carotenoids. Molecules. (2022) 2022:27. doi: 10.3390/molecules27248852
117. Le Goff, M, Le Ferrec, E, Mayer, C, Mimouni, V, Lagadic-Gossmann, D, Schoefs, B, et al. Microalgal carotenoids and phytosterols regulate biochemical mechanisms involved in human health and disease prevention. Biochimie. (2019) 167:106–18. doi: 10.1016/j.biochi.2019.09.012
118. Lomartire, S, and Gonçalves, AMM. Marine macroalgae polyphenols as potential neuroprotective antioxidants in neurodegenerative diseases. Mar Drugs. (2023) 21:261. doi: 10.3390/md21050261
119. Gunathilake, T, Akanbi TO, Suleria, HAR, Nalder, TD, Francis, DS, and Barrow, CJ. Seaweed Phenolics as natural antioxidants, Aquafeed additives, veterinary treatments and cross-linkers for microencapsulation. Mar Drugs. (2022) 20:445. doi: 10.3390/md20070445
120. Schepers, M, Martens, N, Tiane, A, Vanbrabant, K, Liu, HB, Lütjohann, D, et al. Edible seaweed-derived constituents: an undisclosed source of neuroprotective compounds. Neural Regen Res. (2020) 15:790–5. doi: 10.4103/1673-5374.268894
121. El-Beltagi, HS, Mohamed, AA, Mohamed, HI, Ramadan, KMA, Barqawi, AA, and Mansour, AT. Phytochemical and potential properties of seaweeds and their recent applications: a review. Mar Drugs. (2022) 20:342. doi: 10.3390/md20060342
122. Coulombier, N, Jauffrais, T, and Lebouvier, N. Antioxidant compounds from microalgae: a review. Mar Drugs. (2021) 19:549. doi: 10.3390/md19100549
123. Cirulis, JT, Scott, JA, and Ross, GM. Management of oxidative stress by microalgae. Can J Physiol Pharmacol. (2013) 91:15–21. doi: 10.1139/cjpp-2012-0249
124. Gomez-Guzman, M, Rodriguez-Nogales, A, Algieri, F, and Galvez, J. Potential role of seaweed polyphenols in cardiovascular-associated disorders. Mar Drugs. (2018) 16:250. doi: 10.3390/md16080250
125. Choudhary, B, Chauhan, OP, and Mishra, A. Edible seaweeds: a potential novel source of bioactive metabolites and nutraceuticals with human health benefits. Front Mar Sci. (2021) 8:740054. doi: 10.3389/fmars.2021.740054
126. Murray, M, Dordevic, AL, Ryan, L, and Bonham, MP. The impact of a single dose of a polyphenol-rich seaweed extract on postprandial Glycaemic control in healthy adults: a randomised cross-over trial. Nutrients. (2018) 10:270. doi: 10.3390/nu10030270
127. Paradis, ME, Couture, P, and Lamarche, B. A randomised crossover placebo-controlled trial investigating the effect of brown seaweed (Ascophyllum nodosum and Fucus vesiculosus) on postchallenge plasma glucose and insulin levels in men and women. Appl Physiol Nutr Metab. (2011) 36:913–9. doi: 10.1139/h11-115
128. Zaharudin, N, Tullin, M, Pekmez, CT, Sloth, JJ, Rasmussen, RR, and Dragsted, LO. Effects of brown seaweeds on postprandial glucose, insulin and appetite in humans - a randomized, 3-way, blinded, cross-over meal study. Clin Nutr. (2021) 40:830–8. doi: 10.1016/j.clnu.2020.08.027
129. Hall, AC, Fairclough, AC, Mahadevan, K, and Paxman, JR. Ascophyllum nodosum enriched bread reduces subsequent energy intake with no effect on post-prandial glucose and cholesterol in healthy, overweight males. A pilot study. Appetite. (2012) 58:379–86. doi: 10.1016/j.appet.2011.11.002
130. Mayer, MA, Finlayson, G, Fischman, D, de Paz, C, Telleriarte, MR, Ferrero, AJ, et al. Evaluation of the satiating properties of a nutraceutical product containing Garcinia cambogia and Ascophyllum nodosum extracts in healthy volunteers. Food Funct. (2014) 5:773–9. doi: 10.1039/c3fo60631g
131. Teas, J, Baldeón, ME, Chiriboga, DE, Davis, JR, Sarriés, AJ, and Braverman, LE. Could dietary seaweed reverse the metabolic syndrome? Asia Pac J Clin Nutr. (2009) 18:145–54.
132. Ebrahimi-Mameghani, M, Sadeghi, Z, Abbasalizad Farhangi, M, Vaghef-Mehrabany, E, and Aliashrafi, S. Glucose homeostasis, insulin resistance and inflammatory biomarkers in patients with non-alcoholic fatty liver disease: beneficial effects of supplementation with microalgae Chlorella vulgaris: a double-blind placebo-controlled randomized clinical trial. Clin Nutr. (2017) 36:1001–6. doi: 10.1016/j.clnu.2016.07.004
133. Ebrahimi-Mameghani, M, Aliashrafi, S, Javadzadeh, Y, and AsghariJafarabadi, M. The effect of Chlorella vulgaris supplementation on liver En-zymes, serum glucose and lipid profile in patients with non-alcoholic fatty liver disease. Health Promot Perspect. (2014) 4:107–15. doi: 10.5681/hpp.2014.014
134. Takase, T, Nakamura, A, Miyoshi, H, Koga, M, Toyomaki, A, Kusumi, I, et al. Effects of Palmaria palmata on lipid metabolism and glycemic control in participants with hypercholesterolemia in a randomized double-blind placebo-controlled trial. Phytother Res. (2020) 34:2303–12. doi: 10.1002/ptr.6680
135. Hosseini, AM, Keshavarz, SA, Nasli-Esfahani, E, Amiri, F, and Janani, L. The effects of Chlorella supplementation on glycemic control, lipid profile and anthropometric measures on patients with type 2 diabetes mellitus. Eur J Nutr. (2021) 60:3131–41. doi: 10.1007/s00394-021-02492-5
136. Mani, UV, Desai, S, and Iyer, U. Studies on the long-term effect of spirulina supplementation on serum lipid profile and glycated proteins in NIDDM patients. J Nutraceuticals Funct Med Foods. (2000) 2:25–32. doi: 10.1300/J133v02n03_03
137. Mizoguchi, T, Takehara, I, Masuzawa, T, Saito, T, and Naoki, Y. Nutrigenomic studies of effects of Chlorella on subjects with high-risk factors for lifestyle-related disease. J Med Food. (2008) 11:395–404. doi: 10.1089/jmf.2006.0180
138. Panahi, Y, Ghamarchehreh, ME, Beiraghdar, F, Zare, R, Jalalian, HR, and Sahebkar, A. Investigation of the effects of Chlorella vulgaris supplementation in patients with non-alcoholic fatty liver disease: a randomized clinical trial. Hepato-Gastroenterology. (2012) 59:2099–103. doi: 10.5754/hge10860
139. Lee, SH, and Jeon, YJ. Efficacy and safety of a dieckol-rich extract (AG-dieckol) of brown algae, Ecklonia cava, in pre-diabetic individuals: a double-blind, randomized, placebo-controlled clinical trial. Food Funct. (2015) 6:853–8. doi: 10.1039/C4FO00940A
140. Vodouhè, M, Marois, J, Guay, V, Leblanc, N, Weisnagel, SJ, Bilodeau, JF, et al. Marginal impact of Brown seaweed Ascophyllum nodosum and Fucus vesiculosus extract on metabolic and inflammatory response in overweight and obese Prediabetic subjects. Mar Drugs. (2022) 20:174. doi: 10.3390/md20030174
141. Lee, S-H, Yong, L, Karadeniz, F, Kim, M-M, and Kim, S-K. α-Glucosidase and α-amylase inhibitory activities of phloroglucinal derivatives from edible marine brown alga, Ecklonia cava. J Sci Food Agric. (2009) 89:1552–8. doi: 10.1002/jsfa.3623
142. Lee, S-H, Min, K-H, Han, J-S, Lee, D-H, Park, D-B, Jung, W-K, et al. Effects of brown alga, Ecklonia cava on glucose and lipid metabolism in C57BL/KsJ-db/db mice, a model of type 2 diabetes mellitus. Food Chem Toxicol. (2012) 50:575–82. doi: 10.1016/j.fct.2011.12.032
143. Lee, S-H, Park, M-H, Heo, S-J, Kang, S-M, Ko, S-C, Han, J-S, et al. Dieckol isolated from Ecklonia cava inhibits α-glucosidase and α-amylase in vitro and alleviates postprandial hyperglycemia in streptozotocin-induced diabetic mice. Food Chem Toxicol. (2010) 48:2633–7. doi: 10.1016/j.fct.2010.06.032
144. Cherng, J-Y, and Shih, M-F. Improving glycogenesis in Streptozocin (STZ) diabetic mice after administration of green algae Chlorella. Life Sci. (2006) 78:1181–6. doi: 10.1016/j.lfs.2005.06.050
145. Jong-Yuh, C, and Mei-Fen, S. Potential hypoglycemic effects of Chlorella in streptozotocin-induced diabetic mice. Life Sci. (2005) 77:980–90. doi: 10.1016/j.lfs.2004.12.036
146. Hashimoto, S, Setoyama, T, Yokokura, T, and Mutai, M. Effects of soybean phospholipid, chlorella phospholipid, and clofibrate on collagen and elastin synthesis in the aorta and on the serum and liver lipid contents in rats. Exp Mol Pathol. (1982) 36:99–106. doi: 10.1016/0014-4800(82)90082-X
147. Shibata, S, Oda, K, Onodera-Masuoka, N, Matsubara, S, Kikuchi-Hayakawa, H, Ishikawa, F, et al. Hypocholesterolemic effect of indigestible fraction of Chlorella regularis in cholesterol-fed rats. J Nutr Sci Vitaminol. (2001) 47:373–7. doi: 10.3177/jnsv.47.373
148. Shibata, S, Natori, Y, Nishihara, T, Tomisaka, K, Matsumoto, K, Sansawa, H, et al. Antioxidant and anti-cataract effects of <i>Chlorella</i> on rats with Streptozotocin-induced diabetes. J Nutr Sci Vitaminol. (2003) 49:334–9. doi: 10.3177/jnsv.49.334
149. Zhao, C, Yang, C, Liu, B, Lin, L, Sarker, SD, Nahar, L, et al. Bioactive compounds from marine macroalgae and their hypoglycemic benefits. Trends Food Sci Technol. (2018) 72:1–12. doi: 10.1016/j.tifs.2017.12.001
150. Ramamoorthy, A, and Premakumari, S. Effect of supplementation of Spirulina on hypercholesterolemic patients. J Food Sci Technol. (1996) 33:124–8. doi: 10.1590/S1516-89132008000200022y
151. Kim, S, Kim, J, Lim, Y, Kim, YJ, Kim, JY, and Kwon, O. A dietary cholesterol challenge study to assess Chlorella supplementation in maintaining healthy lipid levels in adults: a double-blinded, randomized, placebo-controlled study. Nutr J. (2016) 15:54. doi: 10.1186/s12937-016-0174-9
152. Sherafati, N, Bideshki, MV, Behzadi, M, Mobarak, S, Asadi, M, and Sadeghi, O. Effect of supplementation with Chlorella vulgaris on lipid profile in adults: a systematic review and dose-response meta-analysis of randomized controlled trials. Complement Ther Med. (2022) 66:102822. doi: 10.1016/j.ctim.2022.102822
153. Choi, EK, Park, SH, Ha, KC, Noh, SO, Jung, SJ, Chae, HJ, et al. Clinical trial of the hypolipidemic effects of a brown alga Ecklonia cava extract in patients with hypercholesterolemia. Int J Pharmacol. (2015) 11:798–805. doi: 10.3923/ijp.2015.798.805
154. Roach, LA, Meyer, BJ, Fitton, JH, and Winberg, P. Improved plasma lipids, anti-inflammatory activity, and microbiome shifts in overweight participants: two clinical studies on Oral supplementation with algal sulfated polysaccharide. Mar Drugs. (2022) 20:500. doi: 10.3390/md20080500
155. Murray, M, Dordevic, AL, Cox, K, Scholey, A, Ryan, L, and Bonham, MP. Twelve weeks’ treatment with a polyphenol-rich seaweed extract increased HDL cholesterol with no change in other biomarkers of chronic disease risk in overweight adults: a placebo-controlled randomized trial. J Nutr Biochem. (2021) 96:108777. doi: 10.1016/j.jnutbio.2021.108777
156. Shimada, Y, Terasawa, M, Okazaki, F, Nakayama, H, Zang, L, Nishiura, K, et al. Rhamnan sulphate from green algae Monostroma nitidum improves constipation with gut microbiome alteration in double-blind placebo-controlled trial. Sci Rep. (2021) 11:13384. doi: 10.1038/s41598-021-92459-7
157. Ko, S-J, Kim, J, Han, G, Kim, S-K, Kim, H-G, Yeo, I, et al. Laminaria japonica combined with probiotics improves intestinal microbiota: a randomized clinical trial. J Med Food. (2014) 17:76–82. doi: 10.1089/jmf.2013.3054
158. Allsopp, P, Crowe, W, Bahar, B, Harnedy, PA, Brown, ES, Taylor, SS, et al. The effect of consuming Palmaria palmata-enriched bread on inflammatory markers, antioxidant status, lipid profile and thyroid function in a randomised placebo-controlled intervention trial in healthy adults. Eur J Nutr. (2016) 55:1951–62. doi: 10.1007/s00394-015-1011-1
159. Scoglio, S, Benedetti, S, Canino, C, Santagni, S, Rattighieri, E, Chierchia, E, et al. Effect of a 2-month treatment with Klamin, a Klamath algae extract, on the general well-being, antioxidant profile and oxidative status of postmenopausal women. Gynecol Endocrinol. (2009) 25:235–40. doi: 10.1080/09513590802632506
160. Baldrick, FR, McFadden, K, Ibars, M, Sung, C, Moffatt, T, Megarry, K, et al. Impact of a (poly)phenol-rich extract from the brown algae Ascophyllum nodosum on DNA damage and antioxidant activity in an overweight or obese population: a randomized controlled trial. Am J Clin Nutr. (2018) 108:688–700. doi: 10.1093/ajcn/nqy147
161. Banskota, AH, Stefanova, R, Sperker, S, Lall, SP, Craigie, JS, Hafting, JT, et al. Polar lipids from the marine macroalga Palmaria palmata inhibit lipopolysaccharide-induced nitric oxide production in RAW264.7 macrophage cells. Phytochemistry. (2014) 101:101–8. doi: 10.1016/j.phytochem.2014.02.004
162. Cian, RE, López-Posadas, R, Drago, SR, Sánchez de Medina, F, and Martínez-Augustin, O. A Porphyra columbina hydrolysate upregulates IL-10 production in rat macrophages and lymphocytes through an NF-κB, and p38 and JNK dependent mechanism. Food Chem. (2012) 134:1982–90. doi: 10.1016/j.foodchem.2012.03.134
163. Gómez-Ordóñez, E, Jiménez-Escrig, A, and Rupérez, P. Effect of the red seaweed Mastocarpus stellatus intake on lipid metabolism and antioxidant status in healthy Wistar rats. Food Chem. (2012) 135:806–11. doi: 10.1016/j.foodchem.2012.04.138
164. Jung, W-K, Choi, I, Oh, S, Park, S-G, Seo, S-K, Lee, S-W, et al. Anti-asthmatic effect of marine red alga (Laurencia undulata) polyphenolic extracts in a murine model of asthma. Food Chem Toxicol. (2009) 47:293–7. doi: 10.1016/j.fct.2008.11.012
165. Jalanka, J, Major, G, Murray, K, Singh, G, Nowak, A, Kurtz, C, et al. The effect of psyllium husk on intestinal microbiota in constipated patients and healthy controls. Int J Mol Sci. (2019) 20:433. doi: 10.3390/ijms20020433
166. Tian, Y, Zuo, L, Guo, Q, Li, J, Hu, Z, Zhao, K, et al. Potential role of fecal microbiota in patients with constipation. Ther Adv Gastroenterol. (2020) 13:423. doi: 10.1177/1756284820968423
167. Ding, KX, Gao, TL, Xu, R, Cai, J, Zhang, HQ, Sun, YY, et al. Quantifying the effect of supplementation with algae and its extracts on glycolipid metabolism: a Meta-analysis of randomized controlled trials. Nutrients. (2020) 12:1712. doi: 10.3390/nu12061712
168. Lafarga, T. Effect of microalgal biomass incorporation into foods: nutritional and sensorial attributes of the end products. Algal Res. (2019) 41:101566. doi: 10.1016/j.algal.2019.101566
169. Park, SY, Seo, IS, Lee, SJ, and Lee, SP. Study on the health benefits of brown algae (Sargassum muticum) in volunteers. J Food Nutr Res. (2015) 3:126–30. doi: 10.12691/jfnr-3-2-9
170. Mohamed, S, Hashim, SN, and Rahman, HA. Seaweeds: a sustainable functional food for complementary and alternative therapy. Trends Food Sci Technol. (2012) 23:83–96. doi: 10.1016/j.tifs.2011.09.001
171. Nwoba, EG, Ogbonna, CN, Ishika, T, and Vadiveloo, A. Microalgal pigments: A source of natural food colors. Microalgae biotechnology for food, health and high value products. Berlin: Springer, pp. 81–123. (2020).
172. Kaur, G, Khattar, J, Singh, D, Singh, Y, and Nadda, J. Microalgae: a source of natural colours. Algal biol Biotechnol. (2009) 2009:129–49.
173. Viera, I, Pérez-Gálvez, A, and Roca, M. Green natural colorants. Molecules. (2019) 24:154. doi: 10.3390/molecules24010154
174. Solymosi, K, Latruffe, N, Morant-Manceau, A, and Schoefs, B. Food colour additives of natural origin. Colour additives for foods and beverages. Amsterdam, Netherlands: Elsevier, pp. 3–34. (2015).
175. Palabiyik, I, Durmaz, Y, Öner, B, Toker, OS, Coksari, G, Konar, N, et al. Using spray-dried microalgae as a natural coloring agent in chewing gum: effects on color, sensory, and textural properties. J Appl Phycol. (2018) 30:1031–9. doi: 10.1007/s10811-017-1324-y
176. Gouveia, L, Batista, AP, Miranda, A, Empis, J, and Raymundo, A. Chlorella vulgaris biomass used as colouring source in traditional butter cookies. Innovative Food Sci Emerg Technol. (2007) 8:433–6. doi: 10.1016/j.ifset.2007.03.026
177. Ghosh, S, Sarkar, T, Das, A, and Chakraborty, R. Natural colorants from plant pigments and their encapsulation: an emerging window for the food industry. LWT. (2022) 153:112527. doi: 10.1016/j.lwt.2021.112527
178. Zhang, D, Zhang, M, and Gu, X. Seaweed-derived hydrocolloids as food coating and encapsulation agents. Bioactive seaweeds for food applications. Amsterdam, Netherlands: Elsevier, p. 153–175. (2018).
179. Kim, JH, Lee, JE, Kim, KH, and Kang, NJ. Beneficial effects of marine algae-derived carbohydrates for skin health. Mar Drugs. (2018) 16:459. doi: 10.3390/md16110459
180. Udayangani, R, Somasiri, G, Wickramasinghe, I, and Kim, SK. Potential health benefits of sulfated polysaccharides from marine algae In: SK Kim, editor. Encyclopedia of Marine Biotechnology. Hoboken, NJ: Wiley (2020). 629–35.
181. Bhuva, V, Morya, S, and Borah, A. A review on meat analogue: is this time to see the algal proteins as a sustainable substitute for the meat proteins? Pharma Innov J. (2021) 10:1160–8.
182. Fu, Y, Chen, T, Chen, SHY, Liu, B, Sun, P, Sun, H, et al. The potentials and challenges of using microalgae as an ingredient to produce meat analogues. Trends Food Sci Technol. (2021) 112:188–200. doi: 10.1016/j.tifs.2021.03.050
183. Grahl, S, Palanisamy, M, Strack, M, Meier-Dinkel, L, Toepfl, S, and Mörlein, D. Towards more sustainable meat alternatives: how technical parameters affect the sensory properties of extrusion products derived from soy and algae. J Clean Prod. (2018) 198:962–71. doi: 10.1016/j.jclepro.2018.07.041
184. Caporgno, MP, Böcker, L, Müssner, C, Stirnemann, E, Haberkorn, I, Adelmann, H, et al. Extruded meat analogues based on yellow, heterotrophically cultivated Auxenochlorella protothecoides microalgae. Innovative Food Sci Emerg Technol. (2020) 59:102275. doi: 10.1016/j.ifset.2019.102275
185. The Fish Site. Seaweed-based bacon startup lands $3 million funding: the fish site (2022). Available at: https://thefishsite.com/articles/seaweed-based-bacon-startup-lands-3-million-funding-umaro. (Accessed March 25, 2022).
186. Buxton, A. Is seaweed bacon the next big plant-based thing? $3 million says it could Be: Green Queen; (2022). Available at: https://www.greenqueen.com.hk/umaro-seaweed-bacon-seed-funding/. (Accessed March 28, 2022)
187. CNN. Harnessing the power of an ancient organism to feed the planet: CNN (2023). Available from: https://www.cnn.com/videos/tv/2023/04/24/next-frontier-triton-algae-innovations-algae-dumplings-spc-intl.cnn. (Accessed April 24, 2023.
188. Watson, E. Tofurky teams up with Triton algae innovations for algae-fueled ‘meat innovations’ set to launch in Q1, 2022: food navigator USA; (2021). Available at: https://www.foodnavigator-usa.com/Article/2021/09/22/Tofurky-teams-up-with-Triton-Algae-Innovations-for-algae-fueled-meat-innovations-set-to-launch-in-Q1-2022. (Accessed September 22, 2021).
189. Southey, F. Pork and microalgae cells combined in cultivated meat an ‘industry first’. Foodnavigatorcom (2023).
190. Jones, G. Wery introduces microalgae cultivated meat. FoodBev media Ltd. Available at: https://www.foodbev.com/news/mewery-introduces-microalgae-cultivated-meat/. (Accessed February 14, 2023). (2023).
191. Fradique, M, Batista, AP, Nunes, MC, Gouveia, L, Bandarra, NM, and Raymundo, A. Incorporation of Chlorella vulgaris and Spirulina maxima biomass in pasta products. Part 1: preparation and evaluation. J Sci Food Agric. (2010) 90:1656–64. doi: 10.1002/jsfa.3999
192. Vatsala, S, and Sudesh, J. Quality characteristics of noodles enriched with Spirulina platensis powder. Int J Agric Sci. (2017) 9:4091–4.
193. Fradique, M, Batista, AP, Nunes, MC, Gouveia, L, Bandarra, NM, and Raymundo, A. Isochrysis galbana and Diacronema vlkianum biomass incorporation in pasta products as PUFA’s source. LWT Food Sci Technol. (2013) 50:312–9. doi: 10.1016/j.lwt.2012.05.006
194. Ainsa, A, Honrado, A, Marquina, P, Beltrán, JA, and Calanche, J. Influence of seaweeds on the quality of pasta as a plant-based innovative food. Foods. (2022) 11:2525. doi: 10.3390/foods11162525
195. Zouari, N, Abid, M, Fakhfakh, N, Ayadi, M, Zorgui, L, Ayadi, M, et al. Blue-green algae (Arthrospira platensis) as an ingredient in pasta: free radical scavenging activity, sensory and cooking characteristics evaluation. Int J Food Sci Nutr. (2011) 62:811–3. doi: 10.3109/09637486.2011.582461
196. El-Baz, FK, Abdo, SM, and Hussein, AM. Microalgae Dunaliella salina for use as food supplement to improve pasta quality. Int J Pharm Sci Rev Res. (2017) 46:45–51.
197. Raczyk, M, Polanowska, K, Kruszewski, B, Grygier, A, and Michałowska, D. Effect of spirulina (Arthrospira platensis) supplementation on physical and chemical properties of semolina (Triticum durum) based fresh pasta. Molecules. (2022) 27:355. doi: 10.3390/molecules27020355
198. Ozyurt, VH, and Ötles, S. Effect of food processing on the physicochemical properties of dietary fibre. Acta Sci Pol Technol Aliment. (2016) 15:233–45. doi: 10.17306/J.AFS.2016.3.23
199. Koli, DK, Rudra, SG, Bhowmik, A, and Pabbi, S. Nutritional, functional, textural and sensory evaluation of Spirulina enriched green pasta: a potential dietary and health supplement. Foods. (2022) 11:979. doi: 10.3390/foods11070979
200. De Marco, ER, Steffolani, ME, Martínez, CS, and León, AE. Effects of Spirulina biomass on the technological and nutritional quality of bread wheat pasta. LWT Food Sci Technol. (2014) 58:102–8. doi: 10.1016/j.lwt.2014.02.054
201. Chen, Y, and Li, Y. Development of nutritious Spirulina noodle. J Chin Cereals Oils Assoc. (1999) 14:13–5.
202. Xu, C. Inventor an instant algal noodle and its production method patent Chinese patent CN1077857A. (1993).
203. Figueira, FDS, Crizel, TDM, Silva, CR, and MD, S-M. Pão sem glúten enriquecido com a microalga Spirulina platensis. Braz J Food Technol. (2011) 14:308–16. doi: 10.4260/BJFT2011140400037
204. Ak, B, Avsaroglu, E, Isik, O, Özyurt, G, Kafkas, E, and Etyemez, M. Nutritional and physicochemical characteristics of bread enriched with microalgae Spirulina platensis. Int J Eng Res Appl. (2016) 6:30–8.
205. Achour, H, Doumandji, A, Sadi, S, and Saadi, S. Evaluation of nutritional and sensory properties of bread enriched with Spirulina. Ann Food Sci Technol. (2014) 15:270–5.
206. Selmo, M, and Salas-Mellado, M. Technological quality of bread from rice flour with Spirulina. Int Food Res J. (2014) 21:1523–8.
207. Khemiri, S, Khelifi, N, Nunes, MC, Ferreira, A, Gouveia, L, Smaali, I, et al. Microalgae biomass as an additional ingredient of gluten-free bread: dough rheology, texture quality and nutritional properties. Algal Res. (2020) 50:101998. doi: 10.1016/j.algal.2020.101998
208. Diprat, AB, Thys, RCS, Rodrigues, E, and Rech, R. Chlorella sorokiniana: a new alternative source of carotenoids and proteins for gluten-free bread. LWT. (2020) 134:109974. doi: 10.1016/j.lwt.2020.109974
209. Nunes, MC, Graça, C, Vlaisavljević, S, Tenreiro, A, Sousa, I, and Raymundo, A. Microalgal cell disruption: effect on the bioactivity and rheology of wheat bread. Algal Res. (2020) 45:101749. doi: 10.1016/j.algal.2019.101749
210. Graça, C, Fradinho, P, Sousa, I, and Raymundo, A. Impact of Chlorella vulgaris on the rheology of wheat flour dough and bread texture. LWT. (2018) 89:466–74. doi: 10.1016/j.lwt.2017.11.024
211. Qazi, WM, Ballance, S, Kousoulaki, K, Uhlen, AK, Kleinegris, DM, Skjånes, K, et al. Protein enrichment of wheat bread with microalgae: Microchloropsis gaditana, Tetraselmis chui and Chlorella vulgaris. Foods. (2021) 10:3078. doi: 10.3390/foods10123078
212. Fitzgerald, C, Gallagher, E, Doran, L, Auty, M, Prieto, J, and Hayes, M. Increasing the health benefits of bread: assessment of the physical and sensory qualities of bread formulated using a renin inhibitory Palmaria palmata protein hydrolysate. LWT Food Sci Technol. (2014) 56:398–405. doi: 10.1016/j.lwt.2013.11.031
213. Ziobro, R, Juszczak, L, Witczak, M, and Korus, J. Non-gluten proteins as structure forming agents in gluten free bread. J Food Sci Technol. (2016) 53:571–80. doi: 10.1007/s13197-015-2043-5
214. Skendi, A, Papageorgiou, M, and Varzakas, T. High protein substitutes for gluten in gluten-free bread. Foods. (2021) 10:1997. doi: 10.3390/foods10091997
215. Qazi, MW, De Sousa, IG, Nunes, MC, and Raymundo, A. Improving the nutritional, structural, and sensory properties of gluten-free bread with different species of microalgae. Foods. (2022) 11:397. doi: 10.3390/foods11030397
216. Różyło, R, Hameed Hassoon, W, Gawlik-Dziki, U, Siastała, M, and Dziki, D. Study on the physical and antioxidant properties of gluten-free bread with brown algae. CyTA-Journal of Food. (2017) 15:196–203. doi: 10.1080/19476337.2016.1236839
217. Massoud, R, Khosravi-Darani, K, Nakhsaz, F, and Varga, L. Evaluation of physicochemical, microbiological and sensory properties of croissants fortified with Arthrospira platensis (Spirulina). Czech J Food Sci. (2016) 34:350–5. doi: 10.17221/289/2015-CJFS
218. Khosravi-Darani, K, Gholami, Z, and Gouveia, L. Effect of Arthrospira platensis on the shelf life, sensorial and rheological properties of strudel. Rom Biotechnol Lett. (2017) 22:12250–8.
219. Niccolai, A, Venturi, M, Galli, V, Pini, N, Rodolfi, L, Biondi, N, et al. Development of new microalgae-based sourdough “crostini”: functional effects of Arthrospira platensis (Spirulina) addition. Sci Rep. (2019) 9:19433.
220. Şahin, OI. Effect of Spirulina biomass fortification for biscuits and chocolates. Turk J Agric Food Sci Technol. (2019) 7:583–7. doi: 10.24925/turjaf.v7i4.583-587.2204
221. Batista, AP, Niccolai, A, Fradinho, P, Fragoso, S, Bursic, I, Rodolfi, L, et al. Microalgae biomass as an alternative ingredient in cookies: sensory, physical and chemical properties, antioxidant activity and in vitro digestibility. Algal Res. (2017) 26:161–71. doi: 10.1016/j.algal.2017.07.017
222. Sahni, P, Sharma, S, and Singh, B. Evaluation and quality assessment of defatted microalgae meal of Chlorella as an alternative food ingredient in cookies. Nutr Food Sci. (2018) 49:221–31.
223. Gissibl, A, Sun, A, Care, A, Nevalainen, H, and Sunna, A. Bioproducts from Euglena gracilis: synthesis and applications. Front Bioeng Biotechnol. (2019) 7:108. doi: 10.3389/fbioe.2019.00108
224. Lucas, BF, De Morais, MG, Santos, TD, and Costa, JAV. Spirulina for snack enrichment: nutritional, physical and sensory evaluations. LWT. (2018) 90:270–6. doi: 10.1016/j.lwt.2017.12.032
225. Da Silva, PC, Toledo, T, Brião, V, Bertolin, TE, and Costa, JAV. Development of extruded snacks enriched by bioactive peptides from microalga Spirulina sp. LEB 18. Food Biosci. (2021) 42:101031
226. Özbal, B, Çelekli, A, Gün, D, and Bozkurt, H. Effect of Arthrospira platensis incorporation on nutritional and sensory attributes of white chocolate. Int J Gastron Food Sci. (2022) 28:100544. doi: 10.1016/j.ijgfs.2022.100544
227. Lafarga, T, Acién-Fernández, FG, Castellari, M, Villaró, S, Bobo, G, and Aguiló-Aguayo, I. Effect of microalgae incorporation on the physicochemical, nutritional, and sensorial properties of an innovative broccoli soup. LWT. (2019) 111:167–74. doi: 10.1016/j.lwt.2019.05.037
228. Üstün-Aytekin, Ö, Çoban, I, and Aktaş, B. Nutritional value, sensory properties, and antioxidant activity of a traditional kefir produced with Arthrospira platensis. J Food Proc Preserv. (2022) 46:e16380. doi: 10.1111/jfpp.16380
229. Peters, A. Why the future of soda is blue: fast company. (2022). Available at: https://www.fastcompany.com/90720721/why-is-this-nutritious-soda-bright-blue-algae. (Accessed February 11, 2022).
230. Stunda-Zujeva, A, Berele, M, Lece, A, and Šķesters, A. Comparison of antioxidant activity in various spirulina containing products and factors affecting it. Sci Rep. (2023) 13:4529.
231. Beheshtipour, H, Mortazavian, AM, Haratian, P, and Darani, KK. Effects of Chlorella vulgaris and Arthrospira platensis addition on viability of probiotic bacteria in yogurt and its biochemical properties. Eur Food Res Technol. (2012) 235:719–28. doi: 10.1007/s00217-012-1798-4
232. Mohamed, A, Abo-El-Khair, B, and Shalaby, SM. Quality of novel healthy processed cheese analogue enhanced with marine microalgae Chlorella vulgaris biomass. World Appl Sci J. (2013) 23:914–25. doi: 10.5829/idosi.wasj.2013.23.07.13122
233. Tohamy, MM, Ali, MA, Shaaban, HA, Mohamad, AG, and Hasanain, AM. Production of functional spreadable processed cheese using Chlorella vulgaris. Acta Sci Pol Technol Aliment. (2018) 17:347–58. doi: 10.17306/J.AFS.0589
234. Zanganeh, E, Mirzaei, H, Jafari, SM, Javadi, A, and Afshar Mogaddam, MR. Spirulina platensis extract Nanoliposomes: preparation, characterization, and application to white cheese. J AOAC Int. (2022) 105:827–34. doi: 10.1093/jaoacint/qsab162
235. Hernández, H, Nunes, MC, Prista, C, and Raymundo, A. Innovative and healthier dairy products through the addition of microalgae: a review. Foods. (2022) 11:755. doi: 10.3390/foods11050755
236. Cornall, J. Sophie’s bionutrients develops world's first dairy-free micro-algae based milk alternative: dairy reporter. (2021). Available at: https://www.dairyreporter.com/Article/2021/05/04/Sophie-s-Bionutrients-develops-world-s-first-dairy-free-micro-algae-based-milk-alternative. (Accessed May 4, 2021).
237. Ho, S. Sophie’s bionutrients unveils World’s first microalgae-based allergen-free Milk alternative: green queen (2021). Available at: https://www.greenqueen.com.hk/sophies-bionutrients-unveils-worlds-first-microalgae-based-allergen-free-milk-alternative/. (Accessed February 25, 2022).
238. Southey, F. Microalgae-based vegan ice cream developed with ‘complete nutritional profile’: food navigator Europe (2022). Available at: https://www.foodnavigator.com/Article/2022/11/08/microalgae-based-vegan-ice-cream-developed-with-complete-nutritional-profile. (Accessed November 8, 2022).
239. Carnovale, G, Leivers, S, Rosa, F, Norli, H-R, Hortemo, E, Wicklund, T, et al. Starch-rich microalgae as an active ingredient in beer brewing. Foods. (2022) 11:1449. doi: 10.3390/foods11101449
240. University of Technology Sydney. Algae cures popular brewer’s carbon hangover 2020. Available at: https://www.uts.edu.au/research-and-teaching/research/explore/impact/algae-cures-popular-brewers-carbon-hangover. (Accessed December 22, 2020).
241. Tatham, H Young henrys craft brewery team up with scientists to investigate if micro-algae can reduce methane emissions: ABC. (2021). Available at: https://www.abc.net.au/news/2021-10-26/sydney-brewery-algae-reducing-methane-from-cattle-experiment/100567164. (Accessed October 27, 2021).
Keywords: seaweed, algae, microalgae, alternative protein, nutrition, metabolic health, food applications, protein quality
Citation: Wu JY, Tso R, Teo HS and Haldar S (2023) The utility of algae as sources of high value nutritional ingredients, particularly for alternative/complementary proteins to improve human health. Front. Nutr. 10:1277343. doi: 10.3389/fnut.2023.1277343
Edited by:
Jose Manuel Miranda, University of Santiago de Compostela, SpainReviewed by:
Kit Leong Cheong, Guangdong Ocean University, ChinaEva Maria Santos, Autonomous University of the State of Hidalgo, Mexico
Copyright © 2023 Wu, Tso, Teo and Haldar. This is an open-access article distributed under the terms of the Creative Commons Attribution License (CC BY). The use, distribution or reproduction in other forums is permitted, provided the original author(s) and the copyright owner(s) are credited and that the original publication in this journal is cited, in accordance with accepted academic practice. No use, distribution or reproduction is permitted which does not comply with these terms.
*Correspondence: Sumanto Haldar, c3VtYW50b19oYWxkYXJfZnJvbS5CVUBzaWZiaS5hLXN0YXIuZWR1LnNn
†These authors have contributed equally to this work