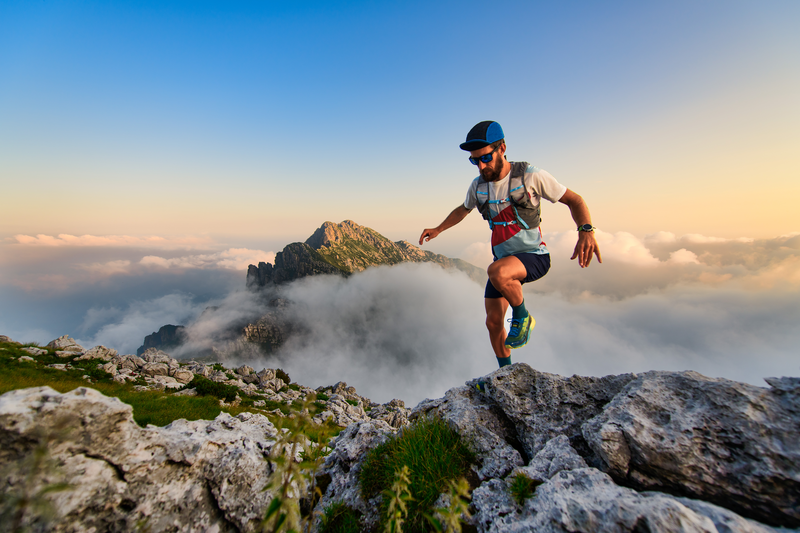
94% of researchers rate our articles as excellent or good
Learn more about the work of our research integrity team to safeguard the quality of each article we publish.
Find out more
ORIGINAL RESEARCH article
Front. Nutr. , 03 November 2023
Sec. Nutrition and Microbes
Volume 10 - 2023 | https://doi.org/10.3389/fnut.2023.1271648
Cantonese soy sauce is an important type of traditional Chinese brewed soy sauce that was developed in southern China, mainly in Guangdong. Due to the long fermentation period and complex microbiota in Cantonese soy sauce, there are few reports on the microbial metaproteomics of Cantonese soy sauce. In this study, integrative metagenomic and metaproteomic analyzes were used to identify the changes in the dominant microbiota and amino acid synthesis-related enzymes and metabolism during Cantonese soy sauce fermentation. Metagenomic analysis revealed that Tetragenococcus halophilus, Weissella confusa, Weissella paramesenteroides, Enterobacter hormaechei, and Aspergillus oryzae were the dominant microbiota. Using the Top 15 dominant microbiota identified by metagenomics as the database, LTQ Orbitrap Velos Pro ETD mass spectrometry was used to obtain metaproteomic information about the microbes in the soy sauce, and the results indicated that the active enzymes involved in the metabolism of amino acids were secreted by microorganisms such as A. oryzae, T. halophilus, and Zygosaccharomyces rouxii. During the Cantonese soy sauce fermentation process. Among them, early fermentation (0-15d) was dominated by A. oryzae and T. halophilus, mid-term fermentation (60-90d) was dominated by Z. rouxii, A. oryzae, and T. halophilus, and late fermentation (90-120d) was dominated by A. oryzae, Z. rouxii, and T. halophilus. Kyoto Encyclopedia of Genes and Genomes analysis revealed that the main enzymes involved in the metabolism of umami amino acids were aspartate aminotransferase, citrate synthase, aconitase, and isocitrate dehydrogenase, which were produced by Z. rouxii and A. oryzae during early fermentation (0–15 d) and the middle fermentation stage (60–90 d). This study constructed a regulatory network of enzymes potentially involved in the metabolism of flavor amino acids, which provided a theoretical basis for studying the amino acid metabolism of Cantonese soy sauce.
Cantonese soy sauce is an important type of traditional Chinese brewed soy sauce and was developed in southern China, mainly in Guangdong (1). With whole soybeans and wheat flour as the main raw materials, Cantonese soy sauce is naturally brewed by the traditional fermentation technique, such as steaming, koji making, fermentation, and post-processing. During the fermentation process, the sauce mash is placed in an open jar and subjected to natural fermentation for 3–6 months, which brine and sauce mash are periodically mixed. The type and content of amino acids in soy sauce are an important basis for determining soy sauce quality (2). During the soy sauce fermentation process, the population structure and interaction of microorganisms play a key role in the proportion and content of amino acids in the soy sauce (3, 4).
Most previous studies have focused on the analysis of amino acid composition in soy sauce or on the microbial dominant microbiota related to amino acid metabolism in soy-based fermented foods (5–8), while the mechanism of amino acid metabolism during soy sauce fermentation is rarely reported. With the rapid development of meta-omics technology (9, 10), metagenomics (11), metatranscriptomics (12), and metabolomics (13, 14) have been widely used in the study of fermented foods. Comparatively, metaproteomics is less used in the study of fermented foods but can be used to directly study the nature of microbial functions in specific environments and conditions and to elucidate the complex interactions between the substrate and microbial communities (15). Therefore, this study used metagenomic and metaproteomic analyzes to reveal the microorganisms and the main protease substances responsible for producing flavor amino acids during Cantonese soy sauce fermentation.
Soy sauce were obtained from Guangdong Meiweixian Flavouring Foods Co., Ltd. Three fermenters were randomly selected from 100 fermenters (Zhongshan, China), and three biological repeats were made for each sample. At 0 d, 15 d, 30 d, 45 d, 60 d, 90 d, and 120 d, 5 kg of soy sauce mash and 5 liters of salt water were taken from the fermentation tank as a sample, and three biological repeats were made for each sample.
50 g of soy sauce mash and 50 mL of salt water were mixed well and filtered with gauze. The filtrate was collected and centrifuged at 3000 × g for 5 min at 4°C, and the supernatant was discarded to obtain a bacterial precipitate, which was washed twice with sterile water.
According to the Kjeldahl method (16) and the National Food Safety Standard - Determination of amino acid nitrogen in food (GB5009.235–2016), the TN content and free amino acid nitrogen content in soy sauce samples at different fermentation stages were determined.
The preprocessed soy sauce samples were sequenced through the DNBSEQ sequencing platform, and in the original sequencing data obtained, reads containing 10% uncertain bases (N bases) and low-quality bases >40% (bases with Q < 20) were excluded, and the assembly software MEGAHIT (17) was used to perform metagenomic de novo assembly. Salmon software was used to quantify gene abundance, and Kraken was used to annotate species and calculate species abundance.
According to the improved bacterial extraction method of Bestbio Company (18), an equal volume of protein extraction solution (Bacterial Protein Extraction Kit, Bestbio Company) was added, and a bead mill was used at-20°C for 3 min, with grinding for 30 s and rest intervals of 20 s. The sample was placed on ice for 1 h and mixed every 10 min. The sample was sonicated on ice for 5 min and centrifuged for 15 min at 20,000 × g and 4°C. The supernatant was transferred to a new 1 mL precooled centrifuge tube to obtain a protein extract of the soy sauce sample. A protein sample (50 μg) was first removed and subjected to reduction and alkylation treatment, then digested in 25 mM NH4HCO3 (protein: trypsin = 1:50) overnight at 37°C. Polypeptide samples obtained after ultrafiltration were analyzed by LC-ESI-MS/MS using a Waters 2,695 nanoLC-LTQ Orbitrap Velos Pro ETD system (LTQ Orbitrap Velos Pro ETD mass spectrometer, Thermo Company, United States; Analytical column: C18 analytical column (50 μm × 2 μm × 15 cm), Thermo Company, USA; Waters 2,695 chromatograph: Waters Company, United States; LC-10A High Performance Liquid Chromatograph: Shimadzu Corporation, Japan). The peptide elution gradient is as follows: 0–10 min, 5% B; 10–100 min, 5–40% B; 100–105 min, 40–95% B; 105–115 min, 95% B; 115–116 min, 95–5% B; and 116–120 min, 5% B., mobile phase A was 0.1% (v/v) formic acid (FA), and mobile phase B was acetonitrile (ACN). The LTQ Orbitrap Velos Pro ETD mass spectrometry conditions were as follows: positive ion mode, electrospray ionization (ESI) source, spray voltage 2.0 kV, collision-induced dissociation (CID) mode (scanning in the range of 350–1800 m/z), and acquisition time 120 min.
For the downstream mass spectrometry data obtained from the LTQ Orbitrap Velos Pro ETD assay, the Proteome Discoverer 2.5 software was used for protein peptide matching and database search and identification (MS/MS spectra were searched with the SEQUEST engine against the UniProt database1 for the top 15 microorganisms in metagenomic abundance). The library search parameters were as follows: trypsin digestion, the maximum number of missed cleavage sites was 2, the mass deviation of the precursor ion was within 10–5, the mass deviation was 0.6 Da, and the false discovery rate (FDR) was <0.01.
Bioinformatics data for successfully identified proteins were obtained by Gene Ontology (GO) and Kyoto Encyclopedia of Genes and Genomes (KEGG) annotation. For the GO2 and KEGG pathway3 analyzes, the PartiGene program4 was used.
According to GB5009.235, the Kjeldahl method and the colorimetric method were used to determine the TN content and amino acid nitrogen content of each sample in the Cantonese soy sauce fermentation process, and results are shown in Figure 1. The TN content and amino acid nitrogen content increased rapidly within the first 15 d of fermentation and then remained steady until 120 d, with final concentrations of 1.51 g/100 mL and 0.98 g/100 mL, respectively. The amino acid nitrogen content accounted for 64.90% of the TN content. In the early stage of fermentation, due to sufficient nutrients in the fermenter, the microorganisms multiplied and metabolized rapidly, and the TN content and amino acid nitrogen content increased rapidly. After 15 d of fermentation, limited by the total volumes of the fermenter and soy sauce mash, the overall number of fermenting microorganisms gradually became saturated, and the TN content and amino acid nitrogen content produced by the metabolism of microorganisms stabilized, which is essentially consistent with the results of Bai (19) and Liu (20). However, the ratios of various amino acids were subject to change according to the dominant microbiota involved at different fermentation times and fermentation conditions.
Figure 1. TN content and amino acid nitrogen content of Cantonese soy sauce at different fermentation times.
The microorganisms identified in Cantonese soy sauce samples were classified by Kraken, and the results revealed that during the Cantonese soy sauce fermentation process, Tetragenococcus halophilus, Weissella confusa, Weissella paramesenteroides, Enterobacter hormaechei, and Aspergillus oryzae were the dominant species (Figure 2). This is basically consistent with the main microbiota groups reported in soy sauce in Southeast Asian countries, but the variation patterns and dominant bacterial groups vary from fermentation time to time (21, 22). One of the main reasons could be the different climatic conditions and fermentation processes of soy sauce producing countries. For example, Cantonese soy sauce is a high-salt dilute soy sauce, the concentration of salt water is 18.5 ~ 20.5%, the amount of salt water is 2 ~ 2.5 times of the mash, so sauce mash is floating on the salt water and in contact with the air, which is different from the high salt solid fermentation of Korean soy sauce and the relatively independent closed fermentation of Japanese soy sauce.
Figure 2. Histogram of microbial species abundance in different Cantonese soy sauce fermentation stages. The abscissa shows the sample name (A-G:0d-120d), and the ordinate shows the relative abundance of the annotated species.
In the early stage of fermentation, W. confusa, E. hormaechei, and A. oryzae were the dominant species; after 15 d of fermentation, the abundance of T. halophilus increased rapidly; after 30 d of fermentation, the abundance of E. hormaechei was reduced rapidly. In the middle stage of fermentation (30–60 d), Leuconostoc citreum gradually grew, and T. halophilus, W. confusa, W. paramesenteroides, and A. oryzae formed the dominant species; Pediococcus acidilactici grew rapidly at 90 d of fermentation and then declined rapidly at 120 d, which is possibly related to the Cantonese soy sauce fermentation process. For example, in the early stage of fermentation (0–15 d), after starter-making using A. oryzae and other species is completed, a large amount of salt water and starter are mixed, so T. halophilus could replace W. confusa, E. hormaechei, and A. oryzae as the dominant species. During the fermentation process, the mash and salt water are mixed regularly (every 15 or 30 days), which leads to a change in fermentation conditions at different fermentation times, resulting in changes in the dominant species and the production of various secondary metabolites (23, 24). Therefore, the time points where substantial changes occur in these microbial communities were the focus of this research.
Metaproteomics can be used to explore the functions and key enzymes of microorganisms in fermented foods at the protein level and to enhance the understanding of fermented food ecosystems (25–27). To date, there have been many proteomic studies on A. oryzae, the dominant specie in high-salt liquid-state fermentation soy sauce (HLFSS), and few studies on microbial metaproteomics in HLFSS (28, 29), possibly due to the long fermentation period of Cantonese soy sauce and its diverse microbial populations and complex metabolites, along with the lack of sample preparation methods for microbial proteomics analysis. The improved bacterial protein extraction method of Bestbio Company is a set of proteomic sample preparation methods suitable for soy sauce samples (18). Through the experimental steps such as gauze filtration and centrifugal precipitation, we mainly obtained the microorganisms in the soy sauce fermentation process, and the subsequent Metaproteomic analysis was based on the proteins in the microbial cells. In this study, the top 15 species in the metagenome were used as the comparison database. The pd2.5 software was used to identify 1,082 proteins in 21 samples (three biological replicates for each time point) at seven time points (0 d, 15 d, 30 d, 45 d, 60 d, 90 d, and 120 d) during soy sauce fermentation, and the intergroup differentially expressed proteins (DEPs) were compared to explore the molecular mechanisms of the soy sauce fermentation process. This study found that there are 76 upregulated proteins and 173 downregulated proteins at 30 d of fermentation, 186 upregulated proteins and 77 downregulated proteins at 60 d, 94 upregulated proteins and 189 downregulated proteins at 90 d, and 91 upregulated proteins and 134 downregulated proteins at 120 d. Overall, the differences between 0 d, 30 d, 60 d, and 90 d were obvious and varied. This is consistent with the predominant microbial populations detected by the metagenomic analysis, indicating that changes in microbial populations and enzymes during these periods may be closely related to the formation of the flavor of Cantonese soy sauce. All 1,082 identified proteins were imported into KEGG for analysis, and the annotation results of 897 enzymes were obtained, as shown in Figure 3. Among them, 39 enzymes were identified in the metabolic pathway of flavor amino acids, accounting for 4.35%. It indicates that amino acid metabolism plays a relatively important role in the entire metabolic pathway during the fermentation process of Cantonese soy sauce.
The seven dominant species with the highest abundance of enzymes involved in amino acid metabolism were selected, and the changes in enzymes involved in amino acid metabolism at different fermentation times during the Cantonese soy sauce fermentation process were compared, as shown in Figure 4. In the early fermentation stage (0–15 d), the protein abundance of T. halophilus and Zygosaccharomyces rouxii increased rapidly; in the middle fermentation stage (45–60 d), the protein abundance of Z. rouxii began to increase; and in the late fermentation stage (90–120 d), the protein abundance of A. oryzae and T. halophilus began to increase, while that of Z. rouxii decreased. The dominant species in different fermented foods or in different production processes of the same fermented foods are different, however, studying the relationship between the metabolism of dominant bacteria and the flavor of fermented food, we can simplify the complexity and quickly find some key enzymes related to flavor. Zhang et al. (30) and Xie et al. (31) reveal microbial assortments and key enzymes in Dajiang by metagenomic and metaproteomic analyzes. Belda et al. (32) proposed an overview of genetic and transcriptional studies to explain and interpret the effects of microorganisms in wine aroma and flavor. In this study, the amino acid metabolism enzymes involved in Cantonese soy sauce fermentation, A. oryzae and Weissella are dominant on Day 0; T. halophilus, A. oryzae, and Z. rouxii are dominant on Day 15; Z. rouxii, A. oryzae, and T. halophilus are dominant on Day 60; and A. oryzae, Z. rouxii, and T. halophilus are dominant on Day 120.
Figure 4. Abundance map of amino acid-metabolizing enzymes in Cantonese soy sauce at different fermentation times. The abscissa shows the fermentation time, and the ordinate shows the relative abundance of the seven dominant species at each fermentation stage.
According to the method of Tseng et al., flavor amino acids are divided into umami, sweet, bitter, and flavorless amino acids, and 39 related enzymes were identified in the metabolic pathways of umami, sweet, and bitter amino acids. The changes in abundance at different fermentation times are shown in Table 1. A regulatory network of these enzymes involved in the metabolism of flavor amino acids was constructed (Figure 5). However, due to the limitations of Label-Free quantitative proteomics, only preliminary inferences can be made based on large data sets to identify possible regulatory networks, and these inferred key enzymes still need further validation.
Table 1. Changes in the abundance of enzymes involved in the metabolism of flavor amino acids during Cantonese soy sauce fermentation.
Figure 5. Regulatory network diagram of flavor amino acid metabolism during Cantonese soy sauce fermentation. Red indicates umami amino acids, yellow indicates sweet amino acids, and green indicates bitter amino acids. Solid arrows indicate that the reaction can be completed in one step; dashed arrows indicate that the reaction can be completed in multiple steps.
Four enzymes were identified in the umami amino acid metabolism pathway: K14454 (aspartate aminotransferase (AST)), K01647 (citrate synthase (CS)), K01681 (aconitase (ACO)), and K00031 (isocitrate dehydrogenase (IDH)). K14454 (AST) is the key enzyme, derived from Z. rouxii, and is a reversible enzyme that mediates the conversion of α-ketoglutarate to glutamic acid and oxaloacetate to aspartic acid. Glutamic acid can produce gamma-aminobutyric acid (GABA) under the action of glutamic acid decarboxylase, which could enhance the functionality ofsoy sauce (33, 34) and the abundance of aspartic acid provides the material basis for the synthesis of alanine, threonine, and glycine (35). In terms of changes, the abundance of K14454 was the greatest on Day 60 of the Cantonese soy sauce fermentation process and then decreased rapidly. However, K14454 was largely undetected at 0–45 d, probably because the environment in the early stage of fermentation was not suitable for Z. rouxii growth; this result is in general agreement with the changing abundance of Z. rouxii detected by the metagenomic analysis, indicating that the period from 60 d to 90 d is the best time for Z. rouxii proliferation, and K14454 expression is the highest, thus leading to the accumulation of a large amount of umami amino acids. Therefore, we speculate that during Cantonese soy sauce fermentation, among the metabolic pathways related to the synthesis of umami amino acids, Z. rouxii and A. oryzae contribute the most, and in the period from 60 d to 90 d of fermentation, the abundance of the relevant enzymes reaches its maximum.
Five enzymes were identified in the metabolic pathways of three sweet amino acids. Under the action of K09758 (aspartate 4-decarboxylase (ASD)), aspartic acid is converted into alanine. K09758 (ASD) is secreted by T. halophilus, with the greatest abundance at the early fermentation stage (0 d, 15 d), and is continuously expressed at 45 d, 60 d, and 120 d. In the process of synthesizing threonine via homoserine with aspartic acid as the starting point, K00133 and K0003 are secreted by Z. rouxii, W. confusa, and A. oryzae; the abundance of Z. rouxii at 60 d and 90 d, W. confusa at 30 d and 60 d, and A. oryzae at 0 d is the highest. Taking threonine as the starting point, in the process of synthesizing glycine under the action of K01620, K01620 is produced by A. oryzae, and the expression level is the highest at 0 d and 90 d. Liu et al. (36) added T. halophilus and Z. rouxii to soy sauce mash during HLFSS fermentation to increase the umami and sweet amino acids by 34.0 and 27.0%, respectively. Studying the molecular mechanism of the production of flavor amino acids in the Cantonese soy sauce fermentation process can provide a theoretical basis for artificially regulating this process.
In conclusion, the enzymes related to the synthesis of umami and sweet amino acids were mainly produced by Z. rouxii, A. oryzae, T. halophilus, and W. confusa, and the expression levels were highest during early fermentation (0–15 d) and the middle fermentation stage (60–90 d). K14454 is the key enzyme that is closely related to the synthesis of glutamic acid and aspartic acid, with the highest expression at 60 d, and large quantities of synthesized aspartic acid provide the material basis for the synthesis of sweet amino acids such as alanine, threonine, and glycine.
The flavor of amino acids is closely related to the structure of the side chains. Most amino acids with strong hydrophobicity have a bitter taste (37). In this study, a total of 21 enzymes were detected in the anabolic pathways of five bitter amino acids, i.e., methionine, leucine, isoleucine, arginine, and histidine. K00549 (5-methyltetrahydropteroyltriglutamate--homocysteine methyltransferase) was detected in the methionine synthesis pathway and is synthesized by A. oryzae, Z. rouxii, and Limosilactobacillus fermentum. Whereas A. oryzae was abundantly expressed at 0 d and 120 d during fermentation, the other two bacteria were highly expressed at 90 d and 120 d of fermentation. K01649 (2-isopropylmalate synthase) and K00052 (3-isopropylmalate dehydrogenase) were detected in the leucine and isoleucine synthesis pathways, and both were produced by A. oryzae, with the highest abundance at Day 120. K01438 (acetylornithine deacetylase) and K00611 (ornithine carbamoyltransferase) were detected in the arginine synthesis pathway, and K01438 (acetylornithine deacetylase) was produced by A. oryzae, with the highest expression at 0 d and 15 d. The bacteria that can produce K00611 (ornithine carbamoyltransferase) were Pediococcus pentosaceus, T. halophilus, Leuconostoc, W. confusa, and A. oryzae 3.042; the abundance of the first three was the highest at 120 d, and the expression levels of the latter two were greatest during the early fermentation stage (0 d-45 d). K00615 (transketolase), K01807 (ribose 5-phosphate isomerase A), K01808 (ribose 5-phosphate isomerase B), K00765 (ATP phosphoribosyltransferase), K01814 (phosphoribosyl formimino-5-aminoimidazole carboxamide ribotide isomerase), and K04486 (histidinol-phosphatase) were detected in the histidine synthesis pathway. K00615 (transketolase) is produced by A. oryzae and T. halophilus, and the expression was relatively high in the middle and late fermentation stages (60–120 d), with the peak expression at 120 d. K01807 and K01808 were produced by A. oryzae and T. halophilus, and the expression levels were relatively high in the middle and late fermentation stages (60–120 d). K00765 was produced by A. oryzae, with the highest expression at 0 d and 60 d. K01814 was produced by Z. rouxii, with the highest expression at 15 d and 90 d. K04486 was produced by T. halophilus, with the highest expression at 30 d, 45 d and 60 d.
From the perspective of bacterial species, the enzyme-producing species related to bitterness were A. oryzae, Z. rouxii, T. halophilus, W. confusa, P. pentosaceus, and Leuconostoc. Controlled P. pentosaceus and Leuconostoc growth during the late fermentation stage (120 d) could provide a basis for reducing the amount of synthesized arginine. On the other hand, from the perspective of fermentation time, the relative abundance of enzymes related to the synthesis of bitter amino acids reached the maximum mainly during the late fermentation stage (90 d, 120 d); therefore, to control the content of bitter amino acids in Cantonese soy sauce, fermentation time optimization could be a suitable meth.
The Cantonese soy sauce fermentation process is complex. During large-scale production, the type and metabolism of various microorganisms involved in fermentation vary greatly. In this study, metagenomic and metaproteomic methods were used to explore the metabolic mechanism of flavor amino acids in industrially produced Cantonese soy sauce for the first time. The metaproteomic analysis of dominant species identified a total of 39 enzymes related to the metabolism of flavor amino acids during the Cantonese soy sauce fermentation process; the changes in abundance at different fermentation times were analyzed, and a regulatory network for the metabolism of flavor amino acids was constructed. Among the enzymes related to the synthesis of umami and sweet amino acids, K14454 is the key enzyme; it is closely related to the synthesis of sweet amino acids, such as glutamic acid, alanine, threonine, and glycine, and is produced by Z. rouxii, A. oryzae, T. halophilus, and W. confusa, with the highest expression levels during early fermentation (0–15 d) and the middle fermentation stage (60–90 d). Twenty-one enzymes related to the synthesis of bitter amino acids were identified, which are mainly produced in the later fermentation stage. The results of this study lay a theoretical foundation for the regulation of flavor amino acids in the large-scale production of Cantonese soy sauce. In future studies, the molecular mechanisms should also be further investigated using multi-omics approaches.
The data presented in the study are deposited in the iProX repository, accession number IPX0006854000.
CC: Data curation, Formal analysis, Investigation, Methodology, Writing – original draft, Writing – review & editing. LW: Formal analysis, Writing – original draft. LY: Software, Writing – original draft. JL: Writing – original draft. QK: Writing – original draft. TX: Writing – original draft. ZL: Writing – review & editing. JF: Writing – review & editing. YC: Conceptualization, Writing – review & editing.
The author(s) declare that no financial support was received for the research, authorship, and/or publication of this article.
CC was employed by Jonjee Hi-Tech Industrial and Commercial Holding Co., Ltd. TX, ZL, and JF were employed by Guangdong Meiweixian Flavouring Foods Co., Ltd.
The remaining authors declare that the research was conducted in the absence of any commercial or financial relationships that could be construed as a potential conflict of interest.
All claims expressed in this article are solely those of the authors and do not necessarily represent those of their affiliated organizations, or those of the publisher, the editors and the reviewers. Any product that may be evaluated in this article, or claim that may be made by its manufacturer, is not guaranteed or endorsed by the publisher.
2. ^http://www.geneontology.org
3. ^http://www.genome.jp/kegg/pathway.html
4. ^http://www.nematodes.org/bioinformatics/annot8r/index.shtml
1. Qian, B. Soy sauce science and brewing technology. Beijing: China Light Industry Press, pp. 102–105, (2011).
2. Liang, HQ, Chen, JG, Liu, W, Bao-Wen, MA, Rong, CY, and Cheng, C. Analysis of amino acids content and contribution to amino nitrogen in fermented soy sauce. Food Ferment Ind. (2018) 44:198–203. doi: 10.13995/j.cnki.11-1802/ts.016752
3. Wah, TT, Walaisri, S, Assavanig, A, Niamsiri, N, and Lertsiri, S. Co-culturing of Pichia guilliermondii enhanced volatile flavor compound formation by Zygosaccharomyces rouxii in the model system of Thai soy sauce fermentation. Int J Food Microbiol. (2013) 160:282–9. doi: 10.1016/j.ijfoodmicro.2012.10.022
4. Song, YR, Jeong, DY, and Baik, SH. Monitoring of yeast communities and volatile flavor changes during traditional Korean soy sauce fermentation. J Food Sci. (2015) 80:M2005–14. doi: 10.1111/1750-3841.12995
5. Geng, RD, and Wang, JH. Effect of flavoured amino acids and peptides on the flavour of fermented foods. China Condiment. (2019) 44:176–178+183. doi: 10.3969/j.issn.1000-9973.2019.07.040
6. Xie, MX, An, FY, Yue, XQ, Liu, Y, Shi, H, Yang, M, et al. Characterization and comparison of metaproteomes in traditional and commercial dajiang, a fermented soybean paste in Northeast China. Food Chem. (2019) 301:125270. doi: 10.1016/j.foodchem.2019.125270
7. Kim, KM, Lim, J, Lee, JJ, Hurh, BS, and Lee, I. Characterization of aspergillus sojae isolated from meju, Korean traditional fermented soybean brick. J Microbiol Biotechnol. (2017) 27:251–61. doi: 10.4014/jmb.1610.10013
8. Li, M, Wang, QQ, Song, XF, Guo, J, Wu, J, and Wu, R. iTRAQ-based proteomic analysis of responses of Lactobacillus plantarum FS5-5 to salt tolerance. Ann Microbiol. (2019) 69:377–94. doi: 10.1007/s13213-018-1425-0
9. Bertuzzi, AS, Walsh, AM, Sheehan, JJ, Cotter, PD, Crispie, F, McSweeney, P, et al. Omics-based insights into flavour development and microbial succession within surfaceripened cheese. mSystems. (2018) 3:e00211–7. doi: 10.1128/mSystems.00211-17
10. Chen, G, Chen, CC, and Lei, ZH. Meta-omics insights in the microbial community profiling and functional characterization of fermented foods. Trends Food Sci Technol. (2017) 65:23–31. doi: 10.1016/j.tifs.2017.05.002
11. Zhang, JC, Wang, XR, Huo, DX, Li, W, Hu, Q, Xu, CB, et al. Metagenomic approach reveals microbial diversity and predictive microbial metabolic pathways in Yucha, a traditional Li fermented food. Sci Rep. (2016) 6:32524. doi: 10.1038/srep32524
12. Song, Z, du, H, Zhang, Y, and Xu, Y. Unravelling core functional microbiota in traditional solid-state fermentation by high-throughput amplicons and metatranscriptomics sequencing. Front Microbiol. (2017) 8:1294. doi: 10.3389/fmicb.2017.01294
13. Chen, S, Liu, H, Zhao, X, Li, X, Shan, W, Wang, X, et al. Nontargeted metabolomics analysis reveals dynamic changes of volatile and nonvolatile metabolites during oolong tea manufacture. Food Res Int. (2020) 128:108778. doi: 10.1016/j.foodres.2019.108778
14. Dahlin, J, Holkenbrink, C, Marella, ER, Wang, G, Liebal, U, Lieven, C, et al. Multiomics analysis of fatty alcohol production in engineered yeasts Saccharomyces cerevisiae and Yarrowia lipolytica. Front Genet. (2019) 10:747. doi: 10.3389/fgene.2019.00747
15. Hettich, RL, Sharma, R, Chourey, K, and Giannone, RJ. Microbial metaproteomics: identifying the repertoire of proteins that microorganisms use to compete and cooperate in complex environmental communities. Curr Opin Microbiol. (2012) 15:373–80. doi: 10.1016/j.mib.2012.04.008
16. Yu, W, and Lyu, YQ. Automatic Kjeldahl nitrogen tester for the determination of protein in food. Chin J Health Lab Technol ISTIC. (2004) 11:610–11. doi: 10.3969/j.issn.1004-8685.2001.05.044
17. Li, DH, Li, C-M, Ruibang, L, Sadakane, K, and Lam, TW. MEGAHIT: An ultrafast single-node solution for large and complex metagenomics assembly via succinct de Bruijn graph. Bioinformatics. (2015) 31:1674–6. doi: 10.48550/arXiv.1409.7208
18. Chen, C, Li, J, and Wen, LF. Sample preparation for microbial proteomics analysis of Cantonese soy sauce. Mod Food Sci Technol ISTIC. (2004) 20:287–90. doi: 10.13982/j.mfst.1673-9078.2023.1.0287
19. Bai, XD, He, YY, and Chen, SF. Optimization of fermentation parameters based on the amino acid nitrogen content of soy sauce. Food Sci Technol. (2015) 6:5. doi: 10.13684/j.cnki.spkj.2015.06.070
20. Liu, HY, Jie, XW, Wang, RG, and Feng, JG. Discussion on instant brewing technique of soy sauce by liquid-state fermentation with high-salt. China Brewing. (2004) 1:3. doi: 10.3969/j.issn.0254-5071.2004.01.007
21. Ito, K, and Matsuyama, A. Koji molds for Japanese soy sauce brewing: characteristics and key enzymes. J Fungi Open Access Mycol J. (2021) 7:80658. doi: 10.3390/jof7080658
22. Chong, SY, Ilham, Z, Samsudin, NIP, Soumaya, S, and Wan-Mohtar, WA. Microbial consortia and up-to-date technologies in global soy sauce production: a review. Int Food Res J. (2023) 30:1–24. doi: 10.47836/ifrj.30.1.01
23. Yang, L. The study on the optimization and mechanism during high-salt soy sauce fermentation with liquid state [D]. Guangzhou: South China University of Technology (2010).
24. Chen, SJ. Key technology research on multi-strain symbiotic fermentation of high-salt liquid-state Cantonese soy sauce [D]. Guangzhou: Zhongkai University of Agriculture and Engineering (2014).
25. Wang, BW, Wu, Q, Xu, Y, and Sun, BG. Synergistic effect of multiple saccharifying enzymes on alcoholic fermentation for Chinese baijiu production. Appl Environ Microbiol. (2020) 86:20. doi: 10.1128/AEM.00013-20
26. Zheng, Q, Lin, BR, Wang, YB, Zhang, Q, He, X, Yang, P, et al. Proteomic and high-throughput analysis of protein expression and microbial diversity of microbes from 30-and 300-year pit muds of Chinese Luzhou-flavour liquor. Food Res Int. (2015) 75:305–14. doi: 10.1016/j.foodres.2015.06.029
27. Zhao, M, Su, XQ, Nian, B, Chen, LJ, Zhang, DL, Duan, SM, et al. Integrated meta-omics approaches to understand the microbiome of spontaneous fermentation of traditional Chinese pu-erh tea. MSystems. (2019) 4:e00680–19. doi: 10.1128/mSystems.00680-19
28. Zhao, G, Liu, C, Li, S, Wang, X, and Yao, Y. Exploring the flavor formation mechanism under osmotic conditions during soy sauce fermentation in aspergillus oryzae by proteomic analysis. Food Funct. (2020) 11:640–8. doi: 10.1039/c9fo02314c
29. Tong, J. Volatile flavour compounds and protease of high-salt liquid-state soy sauce fermented by aspergillus oryzae [D]. Fuzhou: Fujian Normal University (2018).
30. Zhang, P, Zhang, PF, Xie, MX, An, F, Qiu, B, and Wu, R. Metaproteomics of microbiota in naturally fermented soybean paste, da-jiang. J Food Sci. (2018) 83:1342–9. doi: 10.1111/1750-3841.14146
31. Xie, MX, An, FY, Wu, JR, Liu, Y, Shi, H, and Wu, R. Meta-omics reveal microbial assortments and key enzymes in bean sauce mash, a traditional fermented soybean product. J Sci Food Agric. (2019) 99:6522–34. doi: 10.1002/jsfa.9932
32. Belda, I, Ruiz, J, Esteban-Fernandez, A, Navascués, E, Marquina, D, Santos, A, et al. Microbial contribution to wine aroma and its intended use for wine quality improvement. Molecules. (2017) 22:189. doi: 10.3390/molecules22020189
33. Wanmohtar, WAA, Sohedein, MNA, Ibrahim, MF, Kadir, SA, Suan, OP, Loen, AW, et al. Isolation, identification, and optimization of γ--aminobutyric acid (GABA)-producing Bacillus cereus strain KBC from a commercial soy sauce moromi in submerged-liquid fermentation. PRO. (2020) 8:652. doi: 10.3390/pr8060652
34. Sassi, S, Ilham, Z, Jamaludin, NS, Halim-Lim, SA, Shin Yee, C, Weng Loen, AW, et al. Critical optimized conditions for gamma-aminobutyric acid (GABA)-producing Tetragenococcus halophilus strain KBC from a commercial soy sauce Moromi in batch fermentation. Fermentation. (2022) 8:409. doi: 10.3390/fermentation8080409
35. Van der Sluis, C, Smit, BA, Hartmans, S, Ter Schure, EG, Tramper, J, and Wijffels, RH. Regulation of aspartate-derived amino-acid metabolism in Zygosaccharomyces rouxii compared to Saccharomyces cerevisiae. Enzyme Microb Technol. (2000) 27:151–6. doi: 10.1016/S0141-0229(00)00199-X
36. Liu, JL, Zhou, CH, Li, TQ, Lu, LL, and Fang, F. Effect of Tetragenococcus halophilus on soy sauce quality prepared by fermentation. Food Ferment Ind. (2020) 46:96–106. doi: 10.13995/j.cnki.11-1802/ts.023200
Keywords: Cantonese soy sauce, fermentation, microbial amino acid metabolism, metagenomic, metaproteomic
Citation: Chen C, Wen LF, Yang LX, Li J, Kan QX, Xu T, Liu Z, Fu JY and Cao Y (2023) Metagenomic and metaproteomic analyses of microbial amino acid metabolism during Cantonese soy sauce fermentation. Front. Nutr. 10:1271648. doi: 10.3389/fnut.2023.1271648
Received: 04 August 2023; Accepted: 05 October 2023;
Published: 03 November 2023.
Edited by:
Michael Gänzle, University of Alberta, CanadaReviewed by:
Peng Liang, Fujian Agriculture and Forestry University, ChinaCopyright © 2023 Chen, Wen, Yang, Li, Kan, Xu, Liu, Fu and Cao. This is an open-access article distributed under the terms of the Creative Commons Attribution License (CC BY). The use, distribution or reproduction in other forums is permitted, provided the original author(s) and the copyright owner(s) are credited and that the original publication in this journal is cited, in accordance with accepted academic practice. No use, distribution or reproduction is permitted which does not comply with these terms.
*Correspondence: Yong Cao, Y2FveW9uZzIxODFAc2NhdS5lZHUuY24=; Zhan Liu, MTkxMTAzNDU1QHFxLmNvbQ==; Jiang Yan Fu, ZnVqaWFuZ3lhbjEyM0AxNjMuY29t
†These authors have contributed equally to this work
Disclaimer: All claims expressed in this article are solely those of the authors and do not necessarily represent those of their affiliated organizations, or those of the publisher, the editors and the reviewers. Any product that may be evaluated in this article or claim that may be made by its manufacturer is not guaranteed or endorsed by the publisher.
Research integrity at Frontiers
Learn more about the work of our research integrity team to safeguard the quality of each article we publish.