- 1Department of Food Science and Technology, BOKU–University of Natural Resources and Life Sciences, Vienna, Austria
- 2Department of Agricultural, Food and Nutritional Science, University of Alberta, Edmonton, AB, Canada
- 3Department of Crop Sciences, BOKU–University of Natural Resources and Life Sciences, Tulln, Austria
- 4Institute for Animal Nutrition and Feed, AGES–Austrian Agency for Health and Food Safety, Vienna, Austria
- 5Department of Human Biology, School for Nutrition and Translational Research in Metabolism, Maastricht University, Maastricht, Netherlands
During the last decade, scientific interest in and consumer attention to sourdough fermentation in bread making has increased. On the one hand, this technology may favorably impact product quality, including flavor and shelf-life of bakery products; on the other hand, some cereal components, especially in wheat and rye, which are known to cause adverse reactions in a small subset of the population, can be partially modified or degraded. The latter potentially reduces their harmful effects, but depends strongly on the composition of sourdough microbiota, processing conditions and the resulting acidification. Tolerability, nutritional composition, potential health effects and consumer acceptance of sourdough bread are often suggested to be superior compared to yeast-leavened bread. However, the advantages of sourdough fermentation claimed in many publications rely mostly on data from chemical and in vitro analyzes, which raises questions about the actual impact on human nutrition. This review focuses on grain components, which may cause adverse effects in humans and the effect of sourdough microbiota on their structure, quantity and biological properties. Furthermore, presumed benefits of secondary metabolites and reduction of contaminants are discussed. The benefits claimed deriving from in vitro and in vivo experiments will be evaluated across a broader spectrum in terms of clinically relevant effects on human health. Accordingly, this critical review aims to contribute to a better understanding of the extent to which sourdough bread may result in measurable health benefits in humans.
Introduction
The application of sourdough fermentation for bakery products is increasing, especially in large-scale bread production. The microbiota of sourdough consists of lactic acid bacteria and yeasts, which is responsible for specific flavor and quality of the final products, including delayed staling and prevention of microbial spoilage. In addition, it is widely claimed that sourdough fermentation improves the nutritional profile of bread and reduces the content of components that may cause gastrointestinal complaints in susceptible individuals. These claims are predominantly based on in vitro studies that document the degradation of (potentially) harmful or anti-nutritive factors, or an altered digestibility of macronutrients and micronutrients (1–3). Effects on health inferred from in vitro observations, however, were not confirmed by randomized clinical trials. Probably other factors than sourdough fermentation per se, such as type of grain, flour coarseness and product structure play a determining role. Alternatively, effects of sourdough bread when measured in vivo may be too small to result in significant changes (4–7). A recent meta-analysis of studies investigating the effect of sourdough fermentation on the glycemic index (GI) of bread provided no convincing evidence of positive effects of sourdough bread on either reducing GI or improving glucose homeostasis (8).
To date, the limited and inconclusive clinical data on health benefits of sourdough bread also prevented the approval of health claims by regulatory agencies including the European Food Safety Agency (EFSA). Despite apparently favorable data, EFSA has not approved any of the requested sourdough benefit claims so far. For example, a health claim related to the reduction of post-prandial glycemic responses by high fiber rye sourdough bread was not approved because the supporting studies demonstrated a reduced glycemic response relative to glucose solutions but not to comparable bread produced without sourdough (9). Here we aim to review the current state of science and the potential difficulties that may underlie the discrepancy between assumed benefits of sourdough fermentation and the apparently insufficient substantiation of these benefits in clinical trials.
Much of the data presented in dossiers submitted to EFSA for approval of health claims are based on the study of ‘isolated factors’ in vitro, ex vivo and in animals. For a correct interpretation of data obtained from the variety of nutrition studies, one needs to understand all aspects of the food being tested. In the case of bread, the choice of the grain type, region and year of harvest, the degree of milling (fine or coarse), type of flour (wholemeal or refined flour) and the way the dough is being processed (conditions of the fermentation and baking process) influence its composition in terms of contents of nutrients and compounds that may cause intolerances in some individuals. Differences in these parameters may, in turn, affect digestion and absorption in the human body, exposure to cells and organs, and ultimately health. In this light, one may question what data from laboratory experiments mean with respect to the situation in which humans consume the tested food as part of their daily meals containing many other components.
When it is claimed that sourdough bread in comparison to straight dough bread with baker’s yeast as sole fermentation organism results in a ‘better nutritional profile’ or makes a bread ‘better digestible’, it is of crucial importance to define what these terms stand for. Dependent of the factor of measure (time or quantity) ‘better digestibility’ can relate to less time required for a component to be completely digested or that quantitatively more of the food is digested and consequently less undigested matter passes into the colon. Further, does a ‘better nutritional profile’ mean that the components that may cause adverse reactions are themselves reduced or that anti-nutritive factors are reduced; or does it mean that more nutrients are present. Further, the question arises if such changes really lead to measurable health benefits, which is the ultimate proof for claim approval. Depending on what we define as ‘digestibility’, the parameters to be studied can be very different, which will influence the conclusions regarding health benefits.
For components, which may cause adverse reactions, the degradation of anti-nutritive factors during sourdough fermentation or during proofing of straight dough bread can be quantified. This includes phytate, lectins, amylase-trypsin inhibitors (ATIs), rapidly fermentable non-absorbed carbohydrates (FODMAPs), and gluten (10–16). Similarly, one can measure the content of specific nutrients that have been synthesized by the fermentation medium or released from the food matrix in sourdough bread compared to straight dough bread, like vitamins, phenolic compounds and minerals. However, results from chemical analyzes or in vitro assays pointing to possible benefits for health are often not confirmed in vivo. Thus, a critical question arises: does enhanced digestion rate and quantity, or a favorable content of certain components, as measured in vitro, have a significant effect on human health in terms of measured clinical endpoints?
Animal vs. in vitro gastrointestinal models
To use in vitro and animal data as potentially valid for the prediction of the human in vivo situation, the consumption, gastrointestinal, hormonal and metabolic factors need to be at least ‘very similar’ to the human situation. Although frequently utilized, rats and mice have major differences. In contrast, swine have a digestive system that is similar to that of humans. This allows blood samples to be taken and calculations to be made on the distribution of nutrients throughout the body as well as their uptake by various organs. Data from the multiple cannulated swine model correlate well with human in vivo data (17–19). A significant drawback is the required surgery and high degree of specialization required, which makes its frequent use for multiple food testing impossible. To overcome these limitations, in vitro gastrointestinal models have been developed for rapid screening of potential digestibility and prediction of responses that are expected to occur in vivo, such as GI.
In this respect, a mechanical continuous digestion model, which includes the compartments of the stomach, duodenum, jejunum and ileum (TIM-1) and colon (TIM-2), has been developed (20). The TIM models have been improved over the last decade and it has been demonstrated that they produce valid test–retest data (21) as well as a high correlation when compared to both human and multi-cannulated pig data. In addition, the INFOGEST consortium has developed and validated a more straightforward statistical model (22–27).
Despite these promising developments, differences in the set-up and procedures of in vitro studies (e.g., duration of oral processing, type of enzymes used, exposure to the food sample) make direct quantitative comparisons of data across studies difficult. Furthermore, in vitro studies generally result in data on the nutrient content within the test digestion system. However, bio-accessibility is not the same as bio-availability and the two terms are often used interchangeably (Figure 1).
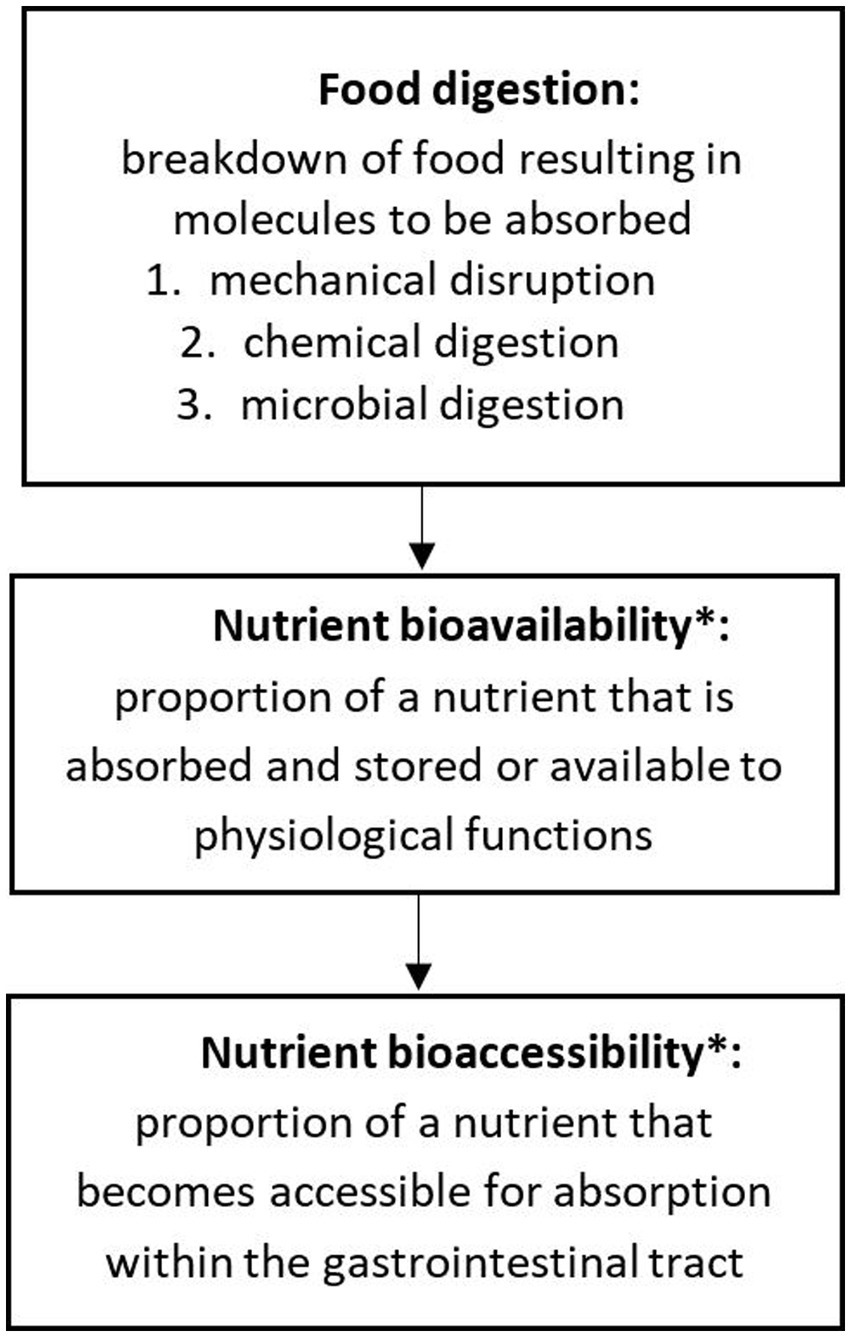
Figure 1. Definitions of the terms “food digestion,” “nutrient bioavailability” and “nutrient bioaccessibility.” *For detailed review see Dima et al. (4) and Fernandez-Garcia et al. (28).
In the in vitro dynamic model, small molecules derived from digestion can pass through a microdialysis membrane. The rate and quantity of passage are often used as estimates of potential absorption in the human body. However, the in vitro model lacks the cells of the intestinal epithelium that may either consume or convert such molecules to other compounds. For example, small peptides are readily degraded by enterocyte proteases and extremely small quantities may pass into the blood. There they become subjected to hydrolysis by proteases, resulting in very short half-lives and concentrations too low to induce a biological effect (29–31). Accordingly, the presence of peptides in an in vitro digest has little relevance for their in vivo absorption and postabsorption bioactivity. As a result, before drawing appropriate conclusions about in vivo in humans, in vitro data must be critically examined.
Adverse reaction to grain components
There are three main types of adverse reactions to grains, commonly known as: (1) celiac disease (CD), (2) wheat allergy, and (3) non-celiac wheat sensitivity (NCWS). Patients suffering from irritable bowel syndrome (IBS) show symptoms that strongly overlap with NCWS. Further, NCWS and IBS strongly overlap with fructose malabsorption (32). These disorders, except IBS, are summarized as gluten-related disorders (GRD) (33). An overview about components causing the mentioned disorders is given in Figure 2.
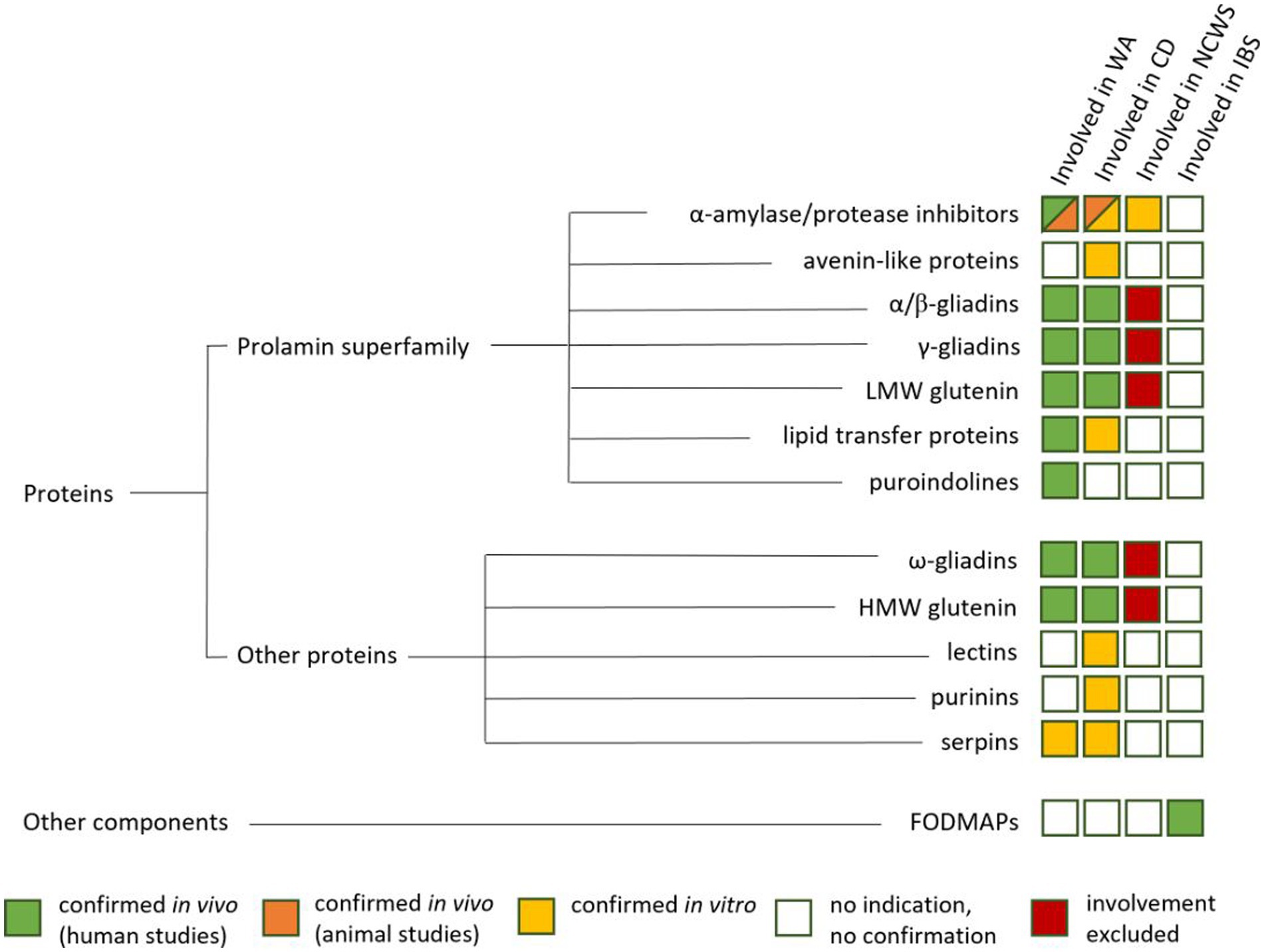
Figure 2. Proteins and other components and their involvement in wheat allergy (WA), Celiac Disease (CD), Non-Celiac Wheat Sensitivity (NCWS) and Irritable Bowle Sydrome (IBS) (34–45).
Celiac disease
CD is an autoimmune disorder that is triggered by consuming gluten-containing grains produced from wheat, rye, barley, and triticale. Undigested gluten peptides may pass the luminal side of the intestinal epithelium and enter the lamina propria via the transcellular or paracellular route. Subsequently, the enzyme tissue transglutaminase (tTG) deaminates the peptides, and dendritic cells recognize specific amino acid sequences on the surface of the antigen (called epitopes), resulting in binding to antigen-presenting cells and the initiation of immune and inflammatory responses (46).
Globally, approximately 1% of the population is diagnosed with CD, but regional prevalence may vary (47). Intestinal biopsy specimen analysis revealed that 0.7% (0.5–0.9%) of the population worldwide was positive. However, the pooled global CD prevalence was 1.4% (1.1–1.7%) (48). The Saharawi population in Sahara, Africa, had the highest CD prevalence in the world (5.6%) (49). It is well known that many people go undiagnosed due to unclear symptoms, which is also known as silent CD (50). CD is more prevalent in women than in men (60–40%). Dermatitis herpetiformis (DH) affects 10–15% of CD patients and is characterized by herpetiform clusters of pruritic, urticated papules and vesicles on the skin. Individuals suffering from CD have intestinal lining damage with the disappearance of the villi, resulting in a flattened surface and nutrient absorption limitations that cause deficiencies. The only remedy is a lifelong adherence to a strict gluten-free diet.
The role of oats in CD is still under discussion. Storage proteins in oat seeds differ from those in wheat, barley, and rye (51), and avenins, the oat prolamins, are significantly lower than in wheat and other gluten-containing cereals. Oats contain epitopes with the potential to trigger CD (52), albeit in low concentrations (53), as well as immune-reactivity (45). Some studies found differences in the immunological responses of different oat varieties (53, 54). Recent evidence suggests that oats cultivated and harvested under controlled conditions, avoiding gluten contamination, are safe for CD patients (55–57). Others, however, argue that the amount of oats consumed by people with CD should be limited for safety reasons (58). Nonetheless, gluten-free oat-based products can only be labeled gluten-free according to Commission Regulation (EC) 41/2009, if the gluten content is less than 20 ppm. Gluten contamination in the food chain is a significant challenge for the food industry, frequently leading to intake levels well above the legal limit (59–66). Further, the low fructan content in oats will potentially reduce fermentation-induced gas formation by microbiota in the colon, reducing the risk of intestinal symptoms in people with NCWS and IBS, while the high nutritional value of oats can help compensate for deficits that are often inherent in gluten-free diets. Other gluten-free flour alternatives such as amaranth, quinoa, rice, maize and potato are popular raw materials, with very low immunogenic potential and immune reactivity (45, 67). The gluten-free pseudocereal buckwheat, however, is known as a potentially highly allergenic grain (67, 68).
Wheat allergy
Wheat allergy is an allergic reaction to proteins found in wheat. This affects 0.2–1% of the population and appears to be more common in children than in adults (69). More than 80% of children outgrow their wheat allergy by the age of 8 years, and 96% before the age of 16 years (70). Accordingly, the number of adults affected by wheat allergy (0.25%) is much lower (71). Wheat allergy can be divided into several sub-categories such as baker’s asthma, contact urticaria, and wheat-dependent exercise-induced anaphylaxis (WDEIA). The prevalence of asthma (1–10%) and rhinitis (18–29%) is high among bakers who continuously inhale flour dust. For this reason, this is referred to as baker’s asthma and bakers associated rhinitis (69). Wheat-induced chronic urticaria was estimated to affect 0.5–5% of adults (72). WDEIA is a rare severe type of allergy, induced by the combination of wheat and exercise stress, but additional triggers may also be involved (97). WDEIA has a very low prevalence in children (0.017%) (98). and there are no reliable figures for its prevalence in adults (99). Noticeably, all food allergies, including wheat allergy, are more common in women than in men (100).
Non-celiac wheat sensitivity
NCWS is described as an unspecific immune-based reaction (sensitivity) following the consumption of wheat. There has been much discussion about the prevalence of patient-self reporting NCWS. It is assumed that the global prevalence is around 10% of the population, with high variations among different countries (82). The major reason for this is that self-reported symptoms may, to a large extent, be due to belief, which is strongly influenced by the effects of social media news as well as socio-economic factors. For example, four recently published studies from South America (Brazil, Chile, El Salvador and Paraguay) with similar criteria based on a self-administered questionnaire and more than 1,000 participants each revealed quite low prevalence of around 0.5% (101), 0.98% (102), and 1.71% (103) respectively, to moderate values of 5.2% (102). In contrast, two studies from Australia showed a prevalence of 13.9 and 14.9%, respectively (104, 105). Effects of geographic region and economic development status were confirmed by the review of (106) (Table 1), showing higher prevalence for industrial countries compared to countries with emerging economies. European studies show similar results. In England and the Netherlands, self-reported NCWS prevalence’s of 13 and 6.2% were observed (107, 108). Many of the self-reporting individuals appear non-reactive to gluten when tested in a controlled situation (109). studied in an IBS subcohort, who complained of gastrointestinal symptoms after the consumption of gluten-containing foods. 6.6% of these patients were diagnosed to suffer from CD and 0.5% from wheat allergy. The remaining people were all put on a 6-month strictly gluten-free (GF) diet, followed by a reintroduction of gluten-containing grains into their diet. Despite their self-diagnosis of being gluten-sensitive, only 6.88% of this IBS subcohort (approximately 1% of the general population) appeared to have verifiable symptoms, and 86% showed no specific reaction to gluten at all after reintroduction into the diet. Accordingly, it was concluded that self-perceived gluten-related symptoms are rarely indicative of the true presence of symptoms due to gluten consumption. Diagnosis and discrimination of NCWS and IBS are challenging due to overlapping symptoms and the absence of clear biomarkers (110–112).
Irritable bowel syndrome
According to the World Gastroenterology Organization Global Guidelines (113), IBS is a functional bowel disorder in which abdominal pain or discomfort is associated with defecation and/or a change in bowel habit. IBS represents the most commonly diagnosed functional gastrointestinal disorder with an estimated global prevalence of about 1 in 10, ranging from 7 to 21%. A recently published systematic review and meta-analysis reported a global prevalence of 9.2% based on 53 studies from 38 countries and comprising 395,385 participants using the Rome III criteria, which are used to diagnose and classify IBS (114–117). Typical symptoms, such as bloating, abdominal discomfort, diarrhea, and constipation, overlap significantly with those of CD and NCWS (110, 118).
The recently established Rome IV criteria, classifies IBS patients into specific subgroups based on their predominant bowel habits: (1) IBS with predominant constipation (IBS-C), (2) IBS with predominant diarrhea (IBS-D), (3) IBS with mixed bowel habits (IBS-M), or (4) unspecified/unsubtyped (IBS) (115). Because IBS is not a well-defined disease, but rather a cluster of symptoms caused by multiple pathologies, accurate diagnosis is difficult, and prevalence estimates are often based on self-diagnosis. Because there is still no standardized test or biomarker for diagnosis, an examination of the detailed patient history is essential for an appropriate diagnosis. Within its complexity, it is often impossible to define exact triggers of IBS. Most patients assume that their symptoms are caused by specific foods, in particular foods rich in rapidly fermentable carbohydrates such as fruits, vegetables, pulses and grains (119). In addition, IBS and fructose malabsorption appear to overlap (120) (121). suggested that more than 50% of patients with IBS might have an atypical food allergy, despite negative skin tests and serologic analysis of immunoglobulin E. People suffering from IBS or NCWS may benefit from a significant reduction in grain-based foods containing gluten and FODMAPs (80, 81, 122).
Components causing adverse reactions
Cereals consist mainly of carbohydrates, proteins and lipids, and are also a good source of fiber, minerals and antioxidants. While these components are the foundation of most people’s diet, wheat consumption may also induce adverse reactions in a small subset of the population. Several wheat components have been identified as potential allergens or triggers of CD and NCWS. Due to the presence of gluten proteins, even rye, barley and triticale have to be excluded from the diet. Many of these components have complex structures that are resistant to digestion and relatively stable to heat and acid exposure. Among the variety of grain components that cause wheat-related diseases, proteins are most frequently associated with wheat sensitivity. Wheat contains at least 114 allergenic proteins1 encoded by all three genomes (A, B and D). However, the contribution of individual components to wheat-related diseases has mostly been assessed only in in vitro or in animal models using isolated protein fractions, which often contain other unidentified components and/or do not reflect adulterations by processing (e.g., heat, hydrolysis by fermentation). In addition to proteins, so called FODMAPs (fermentable oligo-, di-, monosaccharides and polyols) are potential triggers of IBS and maybe even of NCWS (6, 80, 118, 123, 124). Further, a FODMAPs-rich diet is likely to exacerbate symptoms in NCWS (118).
Today, a gluten-free diet is considered the most commonly used therapy. An international threshold of 20 ppm gluten in food is generally considered to be safe for individuals suffering from CD and regulatory bodies have adopted this level for allowing food to be labeled “gluten free.” However, in severe cases of CD lower levels can result in adverse reactions. In addition, it has been shown that foods labeled to be gluten free may contain significantly more than 20 ppm due to contamination in the food chain (60, 66, 125, 126). Furthermore, gluten free-labeled foods may also contain significant amounts of FODMAPs and cause problems in patients who believe to be sensitive to gluten but in fact are sensitive to the effects of rapid carbohydrate fermentation and related intestinal discomfort (127).
Prolamin proteins
With the exception of oats and rice, the main storage proteins of all cereal grains are prolamins. This term is based on the fact that prolamins are high in proline and glutamine. During gastrointestinal digestion, the enzymes present in the human intestine only partially digest the proline-rich sections (128), which can easily trigger adverse reactions in predisposed individuals (122). Almost all food allergens are proteins that tend to be resistant to degradation by heat, proteases, or acid hydrolysis. Thus, a large number of wheat allergens can be found among the group of prolamins (41, 129). Gluten proteins and ATIs have been identified as components involved in CD and NCWS. Gluten peptides, which remain undigested, contain repeated amino acid sequences, also referred to as CD-active epitopes, which are recognized by immune cells and induce a cascade of immune and inflammatory responses when exposed to the gut epithelium. Among them is 33-mer, an α2-gliadin peptide that is highly resistant to proteolysis (130) and is often described as a very potent contributor to gluten immunotoxicity (131). The number, type, and distribution of epitopes may influence the potency of the inflammatory and immune responses involved in the etiology of CD (132).
Non-prolamin proteins
In addition to prolamins, cereals and cereal-based products contain a variety of other ingredients that may cause adverse physical reactions in sensitive people. Data from in vitro and animal studies suggest that non-prolamin proteins such as ATIs, serpins, lectins and others, also play or may play a role in triggering adverse gastrointestinal and extraintestinal reactions (40, 133, 134). With respect to wheat allergy, ATIs and non-specific lipid transfer proteins (nsLTPs) can sensitize susceptible atopic patients after ingestion or inhalation (37, 41, 129, 135–139). Similar to prolamins, the clinical relevance of ATIs and nsLTPs is attributed to their resistance to proteolysis and acid or heat exposure. In vitro studies revealed that, in addition to the well-recognized immune reaction to prolamins, CD is also associated with a humoral immune response directed against serpins (serine protease inhibitors), purinins, ATIs, globulins, farinins and lectins, also known as wheat germ agglutinin (40, 43, 44, 140). However, lectins are not heat stable and lose their adverse biological effects as a result of heat exposure, such as cooking or baking (140).
ATIs are thought to be involved in the etiology of NCWS by mediating intestinal inflammation via binding to the toll-like receptor 4 (TLR4) (45, 134). ATIs have attracted great research interest due to their suspected contribution to baker’s asthma (a respiratory form of wheat allergy), CD, and NCWS, as extensively reviewed by Geisslitz et al. (10). Although non-prolamin proteins make up a small proportion of cereal proteins, several of these proteins were suggested to be involved in adverse reactions and may contaminate protein preparations used for clinical trials, leading to erroneous conclusions about cause-effect relationships. There have been no studies to date, in which wheat-sensitive people have been tested in vivo for their response to individual non-prolamin components or combinations thereof.
FODMAPs
In addition to cereal proteins, wheat contains fructans that are indigestible carbohydrates. They are classified as FODMAPs, which include oligosaccharides (including fructans and raffinose-family oligosaccharides), disaccharides (lactose), monosaccharides (fructose), and polyols (e.g., sorbitol and mannitol). Lactose is conditionally digestible in humans, as approximately 70% of human adults are lactase-non-persistent, which means lactose is a non-digestible disaccharide due to the lack of brush border β-galactosidase. Approximately 15% of the population are fructose-malabsorbers, meaning that ingested fructose is not completely absorbed in the small intestine or is absorbed only completely when glucose is also present at the same time. In these individuals, unabsorbed fructose (excess of fructose in respect to glucose) is classified as FODMAPs (141). Although fructans of grammian type, they have both β(2–1) and β(2–6) fructosyl linkages and a rather complex, branched structure, are considered to be the most prevalent FODMAPs in monocot grains (142); other FODMAPs, particularly raffinose, are present in smaller amounts and mannitol is additionally produced during sourdough fermentation. Fermentable carbohydrates are beneficial to most people’s intestinal health because they are converted to health-beneficial short chain fatty acids, while also improving peristalsis and defecation. However, whether FODMAPs ingestion is beneficial or harmful is dose and individual dependent. A quantity of >15 g of FODMAPs per day results in quantitative effects on osmosis induced liquid accumulation in the terminal small intestine and on fermentation in the terminal cecum and colon, which may cause bloating, flatulence and abdominal discomfort in susceptible people. Patients suffering from IBS appear to develop central sensitization, manifesting as pain hypersensitivity (143), a reason why they may experience symptoms at lower doses of FODMAPs compared to non-sensitized individuals. It is estimated that cereal-based products may account for up to 70% of the daily fructan intake in the United States (144), with intakes varying across populations depending on the amount of bread and other grain-based foods consumed (145). Depending on other foods consumed that contribute to the FODMAPs intake (such as fruits, vegetables, pulses, artificially sweetened soft drinks, and apple juice), the contribution of grain fructans may be minimal to significant (141, 146–148). Low-fructan meals may help to reduce intestinal gas formation and alleviate symptoms in IBS patients (149–157).
Sourdough and microbial communities
Sourdough fermentation
In baking applications, sourdough is used for different technological reasons. Only few countries, mainly in the European Union, established a legal definition of sourdough and the approach to the definition of sourdough varies among different countries. France, Spain and the Netherlands define sourdough by specifying a maximum level of baker’s yeast addition and a minimum level of acidity as defined by either pH, total titratable activity or acetic acid concentration. Austria, the Czech Republic and Germany define sourdough as a dough with metabolically active lactic acid bacteria (158). An overview of the different use of sourdough in baking applications is presented in Table 2. Owing to the different use and definition of sourdough in baking application, the term “sourdough bread” includes fermentations that use diverse microbes and subject a different proportion of the flour to fermentation. With type I sourdoughs, sourdoughs that are used as sole leavening agent, 20–30% of the flour are fermented for more than 6 h and the time for proofing generally exceeds 2 h. With type II sourdoughs, sourdoughs that are used for dough acidification and/or for improved bread quality, usually 10–20% of the flour is fermented in the sourdough fermentation but sourdough microbes have a lower metabolic activity in the bread dough when compared to type I sourdoughs. Type III sourdoughs, dried sourdoughs without viable and metabolically active microbes, are added at a level of 3–5%. Here, only a small portion of the flour is fermented with lactic acid bacteria and metabolic activity in the bread dough is attributable only to baker’s yeast. Freeze dried starter cultures are commercially available but do not show appreciable metabolic activity in the bread dough unless they are propagated (159).
Microorganisms in sourdough
Currently, reliable data on the composition of more than 1,000 sourdoughs that have been used in bakeries or at the household level are available (160). Collectively, these data provide a comprehensive overview on microorganisms in sourdough. The geographical location has no impact on microbial communities in sourdoughs. The type of flour also has remarkably little influence on microbial communities in sourdough as long as whole flours or extracted flours from wheat and rye are used. In flour from other grains, the lack of maltogenic amylases and/or the presence of phenolic acids with antimicrobial activity selects against some sourdough microbes, particularly Fructilactobacillus sanfranciscensis (161, 162).
Overall, more than 100 species of lactic acid bacteria, by far predominantly species in the Lactobacillaceae (89) and several dozen yeast species have been isolated from sourdoughs. The genera Fructilactobacillus, Lactobacillus, Limosilactobacillus and Lactiplantibacillus dominate most sourdoughs. Companilactobacillus, Lacticaseibacillus, Latilactobacillus, Pediococcus, Levilactobacillus, Lentilactobacillus, Furfurilactobacillus, Weissella and Leuconostoc are frequently isolated. Species of other genera are not found in sourdoughs or were isolated in laboratory sourdoughs only. Generally, sourdough microbes include two to six major representative species, which represent stable associations of heterofermentative and homofermentative Lactobacillaceae with the former typically more abundant. Despite the apparent diversity of sourdough microbiota, several types of sourdough have a globally uniform microbial composition that converges at the genus or even species level. The composition of the microbial consortia depends on the fermentation conditions, which, in turn, are dictated by the technological aim of the fermentation (Table 2).
To use sourdough as sole leavening agent, sourdough microbes are maintained at their peak metabolic activity by frequent back-slopping. In bakeries, the process of frequent back-slopping is interrupted only on 1 day per week (163, 164). This fermentation scheme selects for those organisms that grow fastest in wheat or rye doughs and microbial consortia in type I sourdoughs are globally uniform at the species level. A vast majority of type I sourdoughs includes Fructilactobacillus sanfranciscensis as main bacterial representative and Kazachstania humilis as the main representative of yeasts. Other bacterial species that are frequently isolated include Lactiplantibacillus plantarum, Levilactobacillus species, Companilactobacillus species, Weissella species and Leuconostoc species (160). Extensive baking by farmer-bakers, i.e., four or fewer baking days per week, may result in selection of Kazachstania species other than K. humilis (165). Amateur bakers at the household level include more extensive interruptions of the continuous back-slopping process and use only 5–10 back-slopping cycles per month. The microbial consortia in these sourdoughs depends on the diverse fermentation conditions of the individual bakers. Lactobacilli other than Fl. sanfranciscensis are likely to predominate and S. cerevisiae is the most prevalent yeast (Table 2) (164). Acetic acid bacteria were also shown to occur in sourdough (166). As strict aerobes, their growth is restricted to the surface and their contribution decreases with increasing scale of the fermentation, corresponding to a smaller surface to volume ratio.
Most installations for industrial type II sourdough fermentations are found in Central, Northern and Eastern Europe where rye bread is common. In rye baking, dough acidification is essential to inhibit flour amylases, and to solubilize arabinoxylans, while leavening is achieved by addition of baker’s yeast (159). High levels of acidity are often achieved by fermentation at more than 30° C and long fermentation times; these conditions select for Limosilactobacillus and Lactobacillus species with Lm. panis and L. amylovorus being among the most frequent species (Table 2) (163, 167). Yeasts may or may not be present and, if present, S. cerevisiae is the most frequent organism (Table 2) (163, 168–170).
Freeze dried cultures are available as starter cultures (Table 2) but exhibit sufficient metabolic activity only after back-slopping and are therefore not widely used (Table 2). Dried sourdoughs are produced by ingredient suppliers for use in bakeries, which are either obtained by spray-or drum-drying with supersaturated steam (up to 130° C) (158, 171). Different producers of dried sourdoughs use either back-slopped sourdoughs that resemble type II sourdoughs, or inoculate the sourdoughs with defined strains. Dried sourdoughs are used at an inclusion level of 3–5% based on flour and, because sourdough microbes are killed during drying, they exhibit no metabolic activity in the bread dough. Nevertheless, aroma and shelf-life of bread are improved by key flavor compounds and lactic acid from dried sourdoughs (172).
Reduction of potentially harmful components by sourdough fermentation
The allergenic, immunogenic and inflammatory potential of wheat components is primarily due to their structure. Most processing procedures, including sourdough fermentation, have the potential to alter the quantity and structure of wheat components, which may affect the way these components are presented to the intestinal epithelium and immune system. Food processing affects wheat allergenicity (173); however, preclinical validations and human clinical studies are currently lacking. Although there is no validated strategy for producing cereal-based sourdough-fermented foods with lower levels of allergens and triggers of CD and NCWS, experimental sourdoughs have shown reductions that potentially may reduce adverse reactions (10, 13, 174–176).
Protease activity and changes in protein digestion
An extensive review of proteolytic aspects of grains, flours and fermentation media was provided by Gänzle et al. (175). In short, grains contain endogenous proteases (aspartic proteinases, carboxypeptidases, and cysteine proteinases) which are active at lower pH values and are important during the germination of the grain (seed) for the formation of amino acids required for the growth and development of the crop (177, 178). These enzymes are activated by the acidic conditions of dough making. Lactobacilli in sourdough express intracellular protease activity (175), which induces significant degradation of peptides, including toxic gluten epitopes, resulting in an almost linear increase in amino acids with fermentation time (179–184). This increase in free amino acids in sourdough contributes to the improved flavor and taste effects of baking sourdough bread (183). Only type I and type II exhibit significant proteolysis; in other types, either the dough pH never falls below 4.5, i.e., the pH below which cereal proteases are activated, or the proportion of flour fermented with sourdough is too low to affect the overall composition of the bread.
In addition to proteolytic activity, reducing power generated by sourdough microbes impacts protein stability and proteolytic degradation (Figure 3). Heterofermentative lactobacilli accumulate reduced glutathione, or produce other low-molecular weight thiols that interact with glutathione to reduce intra- and intermolecular disulfide bonds (185). Thus, gluten proteins depolymerize (175) and impacts bread volume (186, 187). The glutathione reductase of Fl. sanfranciscensis has been found to enhance the degradation of wheat germ agglutinin and allergenic ovotransferrin in sourdoughs (188, 189). The reduction of disulfide bonds to thiol groups decreases the resistance to proteases and potentially alleviates adverse events such as inflammation, immune and allergic responses that are dependent on proteins with an intact tertiary structure, either for activity or for passage through the intestinal tract. The reduction of intramolecular thiol groups during the breadmaking process, however, has been documented only for type I sourdoughs.
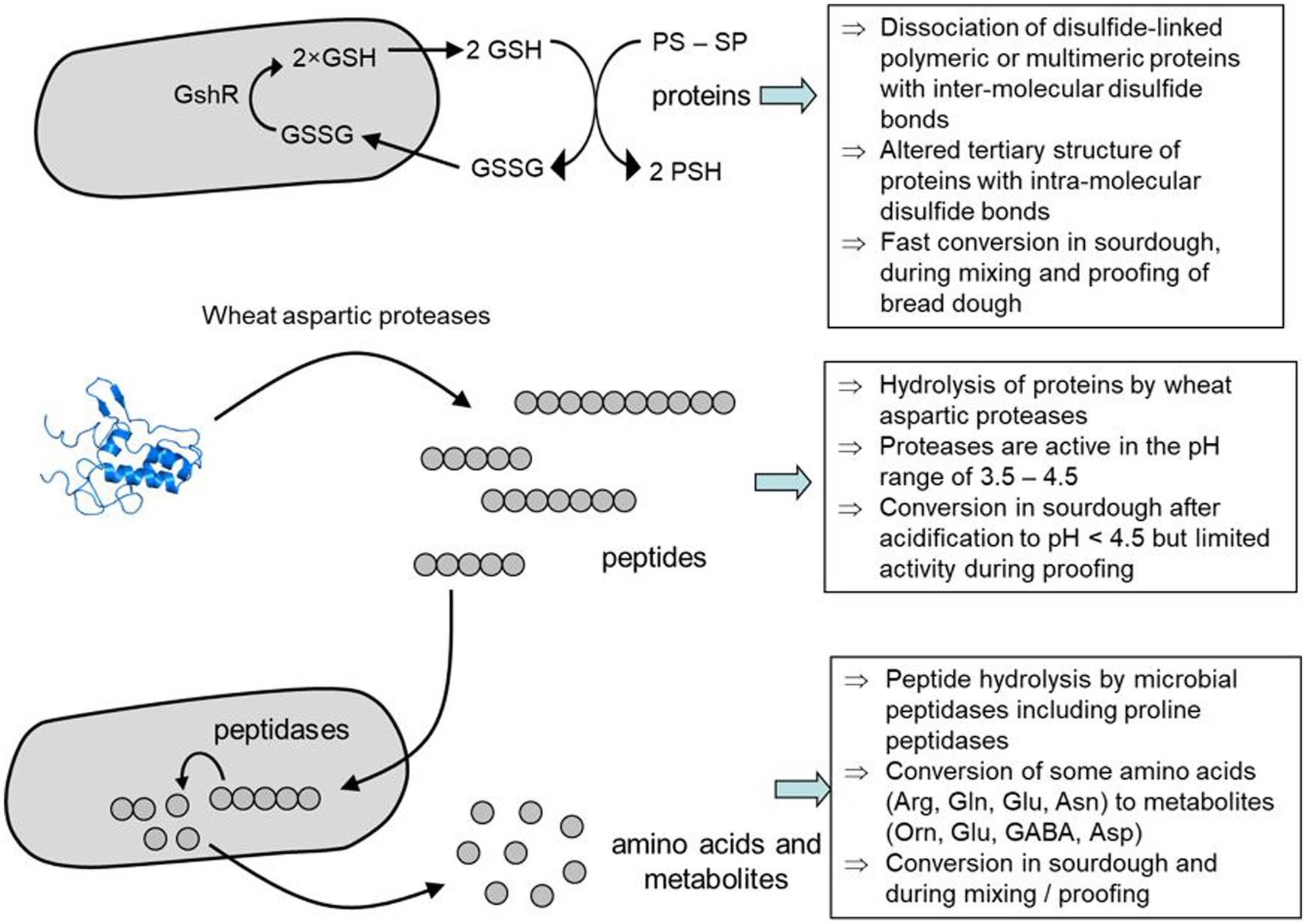
Figure 3. Mechanism of wheat protein degradation by sourdough fermentation. Modified from Gänzle et al. (175) with illustrations from https://www.ebi.ac.uk/pdbe/entry/pdb/1bip/protein/1.
Following food consumption, protein digestion takes place via the combined action of stomach acid and gastrointestinal enzymes (pepsin, trypsin, chymotrypsin), in combination with brush border peptidases. Post-consumption, specific food-derived proteins and peptides may resist degradation due to the lack of specific gastrointestinal enzymes required for their complete degradation. Grain proteins that are particularly high in disulfide bonds and/or proline, such as gluten proteins, are examples. The human gastrointestinal tract lacks the proline-specific peptidases required to cut the post-proline bonds in the gluten peptide chains (190), resulting in incomplete digestion and leaving proline-rich gluten fragments intact. These fragments induce immune responses and inflammation, causing CD. They are often referred to as toxic gluten peptides (epitopes) that can be recognized by the immune system. A reduction of gluten epitope levels in food by proline-specific peptidases during food processing, may help reduce adverse reactions. Many lactobacilli as well as fungi, including Aspergillus oryzae, express proline-specific peptidases that cleave peptide bonds adjacent to proline (191–195). However, sourdough products available on the market are generally not processed in this way, and there is no data to support the claim that sourdough breads reduce the risks of developing celiac disease.
Conversion of FODMAPs
Sourdough fermentation alone or in combination with yeast leavening is an effective approach to reduce FODMAP levels in wheat bread. The major FODMAPs in wheat flour are fructo-oligosaccharides with a degree of polymerization of 3 to 10, which are present at 1–3% and 3–5% in wheat and rye, respectively, and raffinose, which is present at 0.2–0.7% (95, 196, 197). An overview of enzymes that degrade or generate FODMAPs in breadmaking is presented in Table 3. Studies revealed that lactobacilli or yeasts with extracellular fructanases show the most extensive degradation, with a reduction of the fructan content in wheat flour by up to 95% during the breadmaking process. A sourdough that includes lactobacilli expressing the exceptional extracellular fructanase FruA, however, is maintained only by one bakery as reported by (85). Yeasts with extracellular fructanase activity have been used experimentally but not in commercial bread making (84). Fructan hydrolysis by extracellular fructanases generates fructose, which is included in the FODMAP definition when present in excess of glucose, which is not the case in bread after prolonged fermentation and baking (11, 198). Baker’s yeast converts up to 2.5% fructose to ethanol and carbon dioxide in the bread making process (85, 199). Yeast invertase is known to hydrolyze fructo-oligosaccharides but the specificity on oligosaccharides other than sucrose is highly strain-specific (11, 87) and it remains unknown whether yeasts that were domesticated in sourdough fermentations efficiently degrade fructans (200). Intracellular fructanase activity is present in many homofermentative lactobacilli but intracellular enzymes are active on oligosaccharides only after cell lysis (90). Sucrose phosphorylase, which is present in most lactobacilli and the main route of sucrose conversion in heterofermentative lactobacilli, is not active with fructo-oligosaccharides other than sucrose (91, 201). Heterofermentative lactobacilli convert fructose to mannitol but the mannitol concentrations in sourdough bread are generally below 0.3 g/kg and may be further reduced by homofermentative lactobacilli that utilize mannitol as carbon source (95). Raffinose is metabolized by levansucrase or sucrose-phosphorylase activity of lactobacilli in conjunction with α-galactosidase (92). Raffinose levels in wheat flour are reduced by more than 50% independent of the fermentation organisms (85, 202).
FODMAPs reduction of less than 50 to more than 95% have been reported. In yeast leavened bread, the extent of fructan degradation is mainly dependent on the fermentation time and the invertase activity of the baker’s yeast. Extended proofing times were shown to suffice to degrade a substantial part of wheat fructans. Sourdough bread is more commonly fermented with extended proofing times and the strain-specific capacity of sourdough lactobacilli for fructan hydrolysis additionally degrades FODMAPs (84, 85, 87, 196, 202, 203).
In conclusion, reducing FODMAPs through sourdough fermentation can help alleviate gastrointestinal distress in sensitive individuals. FODMAP levels in processed cereal products can be compared to cut-off values established by Varney et al. (141), which forms the foundation for classifying foods as being “low FODMAPs products.” Laatikainen et al. (6) investigated the effects of sourdough bread on IBS symptoms. Flatulence, abdominal pain, cramps, and stomach rumbling were all reduced after consuming low-FODMAPs rye sourdough bread. A subsequent study confirmed the degradation of ATIs and FODMAPs during sourdough fermentation, but sourdough bread was not better tolerated than straight dough bread (7). Furthermore, comparison of a regular rye sourdough with a specific sourdough containing unique lactobacilli that efficiently metabolize fructans showed no significant improvement of symptoms (124). Thus, the clinical relevance of sourdough bread for FODMAP-sensitive individuals remains unclear and the effects may be more dependent on the composition of the overall diet.
Reduction of anti-nutritive compounds and contaminants
Degradation of phytate, wheat germ agglutinin and amylase trypsin inhibitors
In many plant tissues, including grains, phytate or phytic acid (inositol-6-phosphate), stores phosphorus and minerals. High levels of phytate in cereals reduce mineral bioavailability in the small intestine because phytate chelates minerals such as Zn2+, Fe2+, Mg2+, Ca2+, Mn2+ and Cu2+. Furthermore, by binding amino acids, peptides, and enzymes, phytate may also influence amino acid and carbohydrate metabolism (204).
During sourdough fermentation, the dough is acidified to a pH less than 5.0, which solubilizes phytate salts of Fe2+, Mg2+ and Ca2+ while maintaining the activity of cereal phytases close to their optima (205–208). Improved bioacessibility after sourdough fermentation was confirmed by in vitro experiments (209). Consumption of sourdough bread increased mineral absorption in rats when compared to a diet based on yeast-fermented bread (210). The exact impact of sourdough on mineral absorption in humans can differ depending on a variety of factors, including the type of grain used, the fermentation time, and individual differences in gut physiology. However, low mineral intakes are common both in non-industrialized as well as industrialized countries; these individuals may benefit from improved bioavailability, as low Fe intake and/or bioavailability is a major risk factor for developing anemia (211, 212).
Wheat germ agglutinin, as present in raw cereal materials, is a lectin with the potential to reduce nutrient absorption and cause digestive problems (189, 213). Sourdough fermentation was reported to reduce WGA levels in dough, with the extent of degradation determined by thiol metabolism and redox potential rather than proteolytic activity (189). WGA reductions in the dough may also reduce adverse effects of lectin exposure in the intestine. Although this may be the case only for unprocessed flour or cereals, experimental studies have shown that heat exposure of more than 90° C, such as taking place during baking inactivates WGA leading to loss of bioactivity, as reviewed by (140).
ATIs are relatively heat and acid stable as well as digestion resistant. As a result, they remain largely intact during food processing and during gastrointestinal transit (10). ATIs have the ability to inhibit digestive enzymes as well as induce innate immune responses and inflammation, which is why they are suspected to play a significant role in the etiology of CD and NCWS (10, 214). Several studies documented the fate of ATIs during sourdough fermentation. However, these studies have only investigated the degradation resulting from fermentation in dough samples and not in the baked bread. Additionally, extraction protocols that do not account for denatured or aggregated proteins in bread were frequently used (14, 15, 175, 189) or model systems with protein fractions were examined (12). Therefore, data of effects of the consumption of ATIs by humans, as present in heat-processed cereal foods, analyzed appropriately, are required for drawing valid conclusions (215).
Reduction of acrylamide and mycotoxin levels
According to Council Regulation (EEC) No 315/93 acrylamide belongs to the group of contaminants and is a carcinogenic chemical hazard in foods formed during heat induced Maillard reaction (216). The concentration of acrylamide in baked foods such as bread is mainly affected by the presence of precursors such as reducing sugars and asparagine, as well as their ratio, which is mainly determined by flour quality such as type and degree of milling, and by fermentation conditions (217). Furthermore, thermal processing conditions (temperature, and time) and the water content influence acrylamide formation (218). Thiol groups reduced acrylamide formation during baking (219). A lower pH also resulted in lower acrylamide levels after addition of low or moderate amounts of dried sourdough for bread making (220).
Lower concentrations of acrylamide were observed in wholegrain sourdough breads compared to bread fermented with yeast only. The range of acrylamide contents in sourdough bread indicated a strain specific behavior. Significant relationships were also detected between pH, TTA and lactic acid, whereas no correlation was found between the amount of reducing sugars and amino acids (221). Other studies also reported a decrease of about 25–59% and strain specific effects. Pediococcus pentosaceus had the highest potential to reduce acrylamide levels (222). Other studies found lower acrylamide levels in sourdough fermentation as well, but observed a significant correlation with the amount of reducing sugars (223, 224). Contrary to the previously mentioned publications, Fredriksson et al. (217) found that sourdough fermentation did not diminish free asparagine and had an adverse impact of its utilization by yeast, which results in increased acrylamide levels. However, the fermentation reduced the acrylamide content significantly, which is commonly much longer in the case of sourdough fermentation. In short, conclusive evidence on mechanisms or metabolic activities of sourdough microbes that reduce acrylamide formation during baking remains elusive but, despite the increase of the concentration of asparagine during fermentation, sourdough fermentation decreases rather than increases acrylamide formation in bread production (217, 218, 221, 222). A reduced pH and an increased concentration of thiols may be main factors for the reduction of acrylamide levels but the impact on health and the cause of its reduction remains questionable.
The usage of raw materials and flours contaminated with fungal toxins is not possible from a legal point of view, irrespective of the reduction of mycotoxin concentrations by fermentation. COMMISION REGULATION (EC) No 1881/2006 established maximum levels for main mycotoxins in foodstuffs, including cereals and bakery products, which prohibits their usage even if the concentration of mycotoxins is strongly reduced by processing. Nevertheless, a few research groups examined potential mycotoxin degradation by sourdough fermentation, which was highlighted in two recently published reviews (225, 226). Results indicated degradation of mycotoxins in different intensities by sourdough fermentation, depending on applied microbiota and conditions. Although shown results of several studies revealed a high potential of sourdough fermentation for limiting mycotoxins exposure in bread consumption, the role of LAB might not be the predominant reason for lower mycotoxin levels. A study applying yeast fermentation only for bread making already showed a significant reduction in three fungal toxins spiked in wheat flour (227). Furthermore, the baking process and the induced heat impact had a strong effect on the mycotoxin contents, as mycotoxins were diminished in a higher extent in the crust than in the crumb of breads (228).
However, data about degradation products and its possible health hazards are missing. Adsorption mechanism of cells walls may be responsible for lower mycotoxins levels. Thus, release of adsorbed toxins during human digestion cannot be excluded. Detoxification of mycotoxins other than patulin by sourdough fermentation is not supported by reliable studies on metabolic pathways or enzymatic activities of sourdough microbes with activity on mycotoxins, or by in vivo studies, and is therefore unlikely to be relevant (225–227, 229–234). Milling strategies seem to be more suitable for mitigating mycotoxin exposure as they could be applied for flour production from a legal point of view (235).
Sourdough fermentation as a tool to obtain nutritional and health benefits
Bioactive peptides and amino acid derivatives
Long-term fermentation with a reduction of the pH to less than 4.5 results in a significant degradation of proteins with a corresponding increase of amino acids and peptides. If sourdough fermentation generating reducing power is combined with addition of fungal proteases, a virtually complete degradation of proteins to amino acids and peptides can be achieved (192, 236). Some of the peptides that are released from wheat proteins during sourdough fermentation and detected in sourdough or sourdough bread were proposed to have biological activity (236–242).
In order to exert systemic biological effects, intact food peptides must be absorbed in sufficient quantities and remain intact in blood or biofluids for sufficient time to have measurable biological activity (Figure 4). Bioactive gluten peptides were indeed shown to pass the disordered intestinal epithelium in CD patients by paracellular transport into blood after consumption of wheat protein (244). Untreated CD patients, however, have an inflamed, damaged intestinal barrier, allowing even macromolecules including intact proteins, peptides and bacteria to pass paracellularly from the intestinal lumen into blood (245–248). The presence of large peptides in blood under such specific pathophysiological circumstances does not prove that these are also absorbed under normal physiological conditions, during which most dietary peptides are rapidly hydrolysed by pancreatic enzymes and brush border peptidases. Few tri-and tetrapeptides that are rich in proline and thus resist degradation by brush border peptidases have been shown to translocate to the blood plasma; however, their concentration in plasma is less than 0.1% of the active concentration and the half-life of different peptides was determined as less than 15 min (29, 30, 249–251).
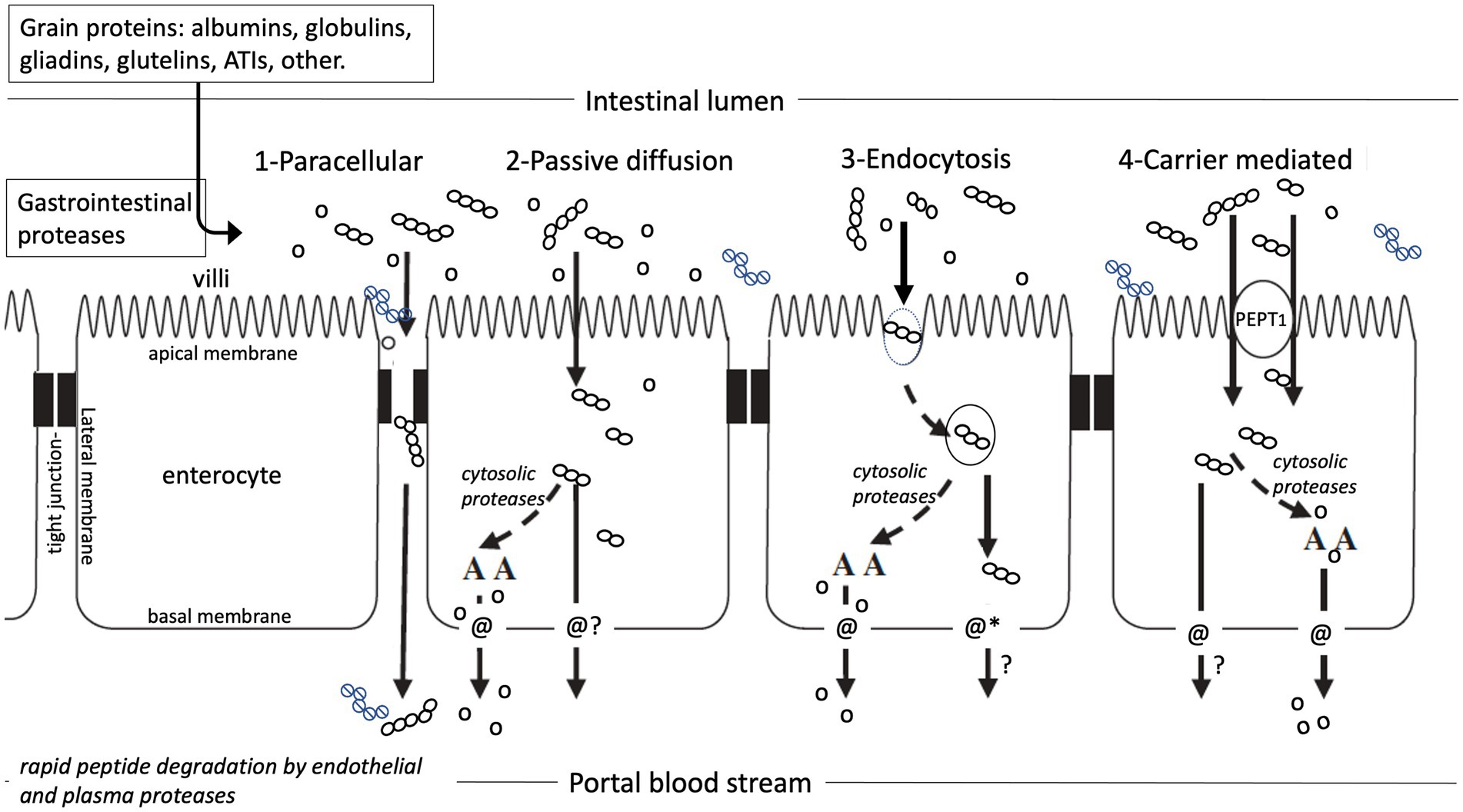
Figure 4. Potential pathways of amino acid and peptide absorption in the small intestine. (1) paracellular through widened tight junctions, (2) passive diffusion through the enterocyte, (3) endocytosis, followed by carrier transport or suggested peptide cargo*-permeability, (4) Transport carrier mediated passage *It is unlikely that peptides will passively diffuse across the cell membrane, but altering their physical properties (such as conformational flexibility and polarity), has been proposed to improve their permeability, also referred to as peptide cargo (243). Open dots represent amino acids, open dot-chains represent digestible peptides, closed dot-chains represent proteolysis resistant gluten peptides. Modified from Brouns and Shewry (202).
Despite the fact that dietary bioactive peptides do not pass into the bloodstream in biologically relevant concentrations, health benefits of few groups of bioactive peptides were consistently demonstrated in clinical trials. The ACE inhibitory peptides IPP and VPP reduced the systolic blood pressure in moderately hypertensive patients (252–255). Likewise, milk protein hydrolysates reduced the postprandial glucose response both when administered as a single dose, or over a period of several weeks (256–258). In both cases, the clinical outcomes likely relate to luminal or local (gastro-intestinal) effects rather than translocation of peptides to the blood. The biological effect of ACE inhibitory peptides was hypothesized to interact with the intestinal renin–angiotensin system, which regulates the passage of Na and fluid across the gut wall (30). A reduced postprandial glucose response may also be attributable to luminal effects, either to the inhibition of starch digestive enzymes by dietary peptides (259), or to interaction of dietary peptides with intestinal receptors that modulate the endocrine response and insulin secretion (260, 261).
Observations from animal and in vitro laboratory research, have led to the suggestion that the presence of gluten exorphins, peptides with 4–8 amino acids that have opioid activity, stimulates weight gain and decrease resting energy expenditure, resulting in weight gain, however, a critical assessment found no reliable evidence in support of this claim (262).
In summary, clinical evidence for health beneficial properties of bioactive peptides in sourdough bread is not available. When using a fermentation protocol that was optimized for proteolysis by including rye malt, fungal proteases and extended fermentation times, the angiotensin-converting enzyme inhibitors IPP and VPP were shown to be present in sourdough bread and sourdough steamed bread in clinically relevant concentrations (242). Whether their presence elicits a relevant physiological response, either alone or in conjunction with γ-aminobutyrate (GABA) or γ-glutamyl peptides, remains to be documented in future studies (192, 236, 263).
Effects of sourdough and yeast fermentation on starch digestion and blood glucose response
The effects of an undesired high and long elevated blood glucose drive a wide range of health-related factors (264, 265). GI is a measure of the potential of carbohydrates to raise the level of blood glucose and is determined by ingesting a food portion containing a calculated amount of 50 g of available carbohydrates followed by measuring the area under the blood glucose-response-curve above baseline (Table 4) (266). Influencing factors include the overall macronutrient composition, processing, matrix characteristics, and the content of active enzyme inhibitors (as illustrated in Figure 5).
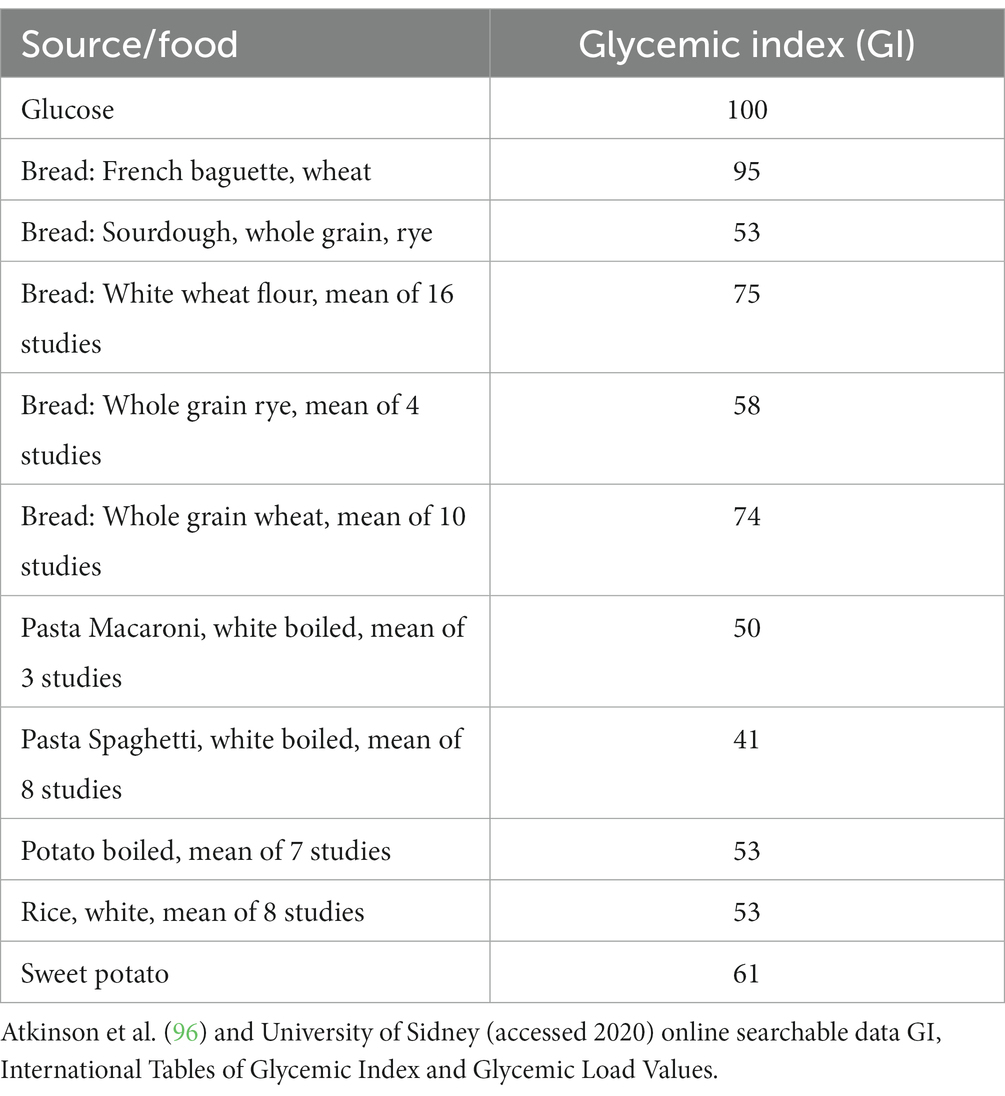
Table 4. Glycemic index value of bread and some other carbohydrate sources, as tested vs. glucose as reference-control.
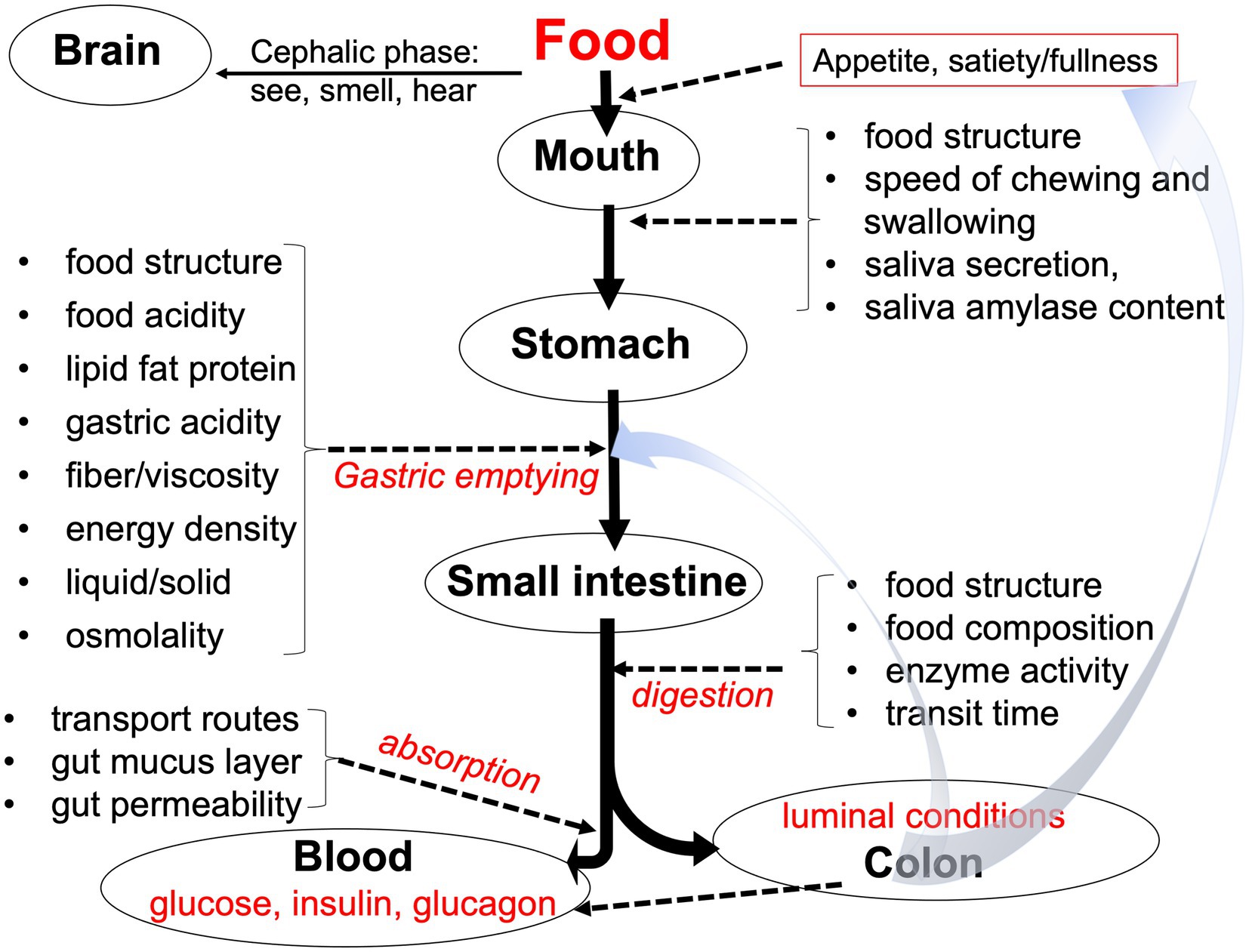
Figure 5. Factors that play a role in gastrointestinal meal transit, digestion, and absorption of nutrients. Adapted from Brouns (267).
Human GI testing must follow strict ethical guidelines, is time-consuming, and costly. Hence, in vitro digestion has been used to predict responses in humans. Data obtained from in vitro digestion of bread predicted in vivo GI values relatively well (268), which is in consistence with findings of others (269). However, when looking at absolute figures of the digestion rate (glucose release) or glycemia, there may be differences. Vangsøe et al. (270) showed that the in vitro digestion method ranked the tested diets in a similar relative manner as in vivo. The rate of glucose release in vitro, however, was much faster than the portal glucose appearance in vivo. In swine, the data on the in vitro release of glucose from starch correlated with portal glucose appearance rates only after correction for gastric emptying rate (271). Hence, the effects of gastric emptying have to be considered. Therefore, data from in vitro digestion and predicted GI should be considered as only indicative of what may be expected in vivo in humans. Different in vitro studies on different types of bread raise questions about the validity of benefit claims communicated in marketing. In fact, Korem et al. (5) found no significant effect of glycemic response after consumption of sourdough fermentation bread vs. yeast fermented bread in a crossover trial. While bread consumption did affect clinical parameters, the glycemic response to the different bread types varied greatly among the participants.
Effects of dietary fiber in kernels and flour on glycemic effects
The content and type of dietary fiber in the food matrix are known to influence the gastrointestinal transit, intestinal viscosity, and the rate and magnitude of macronutrient digestion and absorption. With respect to starch, this may result in a reduction of the blood glucose response (272). Hence, it is often suggested that wholemeal based breads result in a lower GI compared to white flour-based breads, low in fiber, which are based on observations that dietary fiber enriched sourdough-bread resulted in lower GI (< 55) values (273–276).
However, fibers of different cereals differ significantly in composition. Oat fiber consist of about 30% soluble and 70% insoluble fiber types, mainly cellulose, arabinoxylan, β-glucan, xyloglucan and fructan. The soluble fibers express viscous properties that are beneficial for slowing the glucose absorption rate in the intestine. This part of the fiber is also well fermentable and impacts on microbial production of short chain fatty acids, which are known to modulate metabolism and insulin sensitivity, in favor of overweight and diabetes risks reduction (277–280). A systematic review and meta-analysis addressing the effects of dietary fiber and whole grains in diabetes management concluded that higher-fiber diets are an important component of diabetes management (281). It appeared, however, that these effects were independent of the type of dietary fiber. In addition, the impact of fiber on health outcomes depends on the presence of other phytochemicals that are present in whole grains, particularly phenolic compounds (282). Because of these beneficial effects, adding fiber to the dough has become an important target of study. Owing to the beneficial impact of sourdough fermentation on the technological functionality of fiber-rich ingredients, whole grain sourdough bread is an optimal format for further studies.
The fate of resistant starch in sourdough fermented dough and bread
As a result of food processing, a fraction of digestible starch may be transformed into resistant starch, causing a decrease in the glycemic potential. The resistant starch fraction comes on top of a fraction of starch in bread that remains undigested during transit in the gastrointestinal tract (usually 3–5% of total starch) and passes on to the colon. These fractions will be completely fermented and will serve as an important substrate for the colonic formation of butyrate, which has been linked to gastrointestinal health and disease reduction (283, 284).
Available data show mixed outcomes in terms of the dough stage. De Angelis et al. (275) reported a fermentation-induced increase in resistant starch, whereas Liljeberg et al. (285) found no difference. Furthermore, Hefni et al. (268) observed no change in resistant starch content during dough formation but an approximately 3-fold increase during the subsequent step from proofed loaf to bread. A recent study used X-ray diffraction and FTIR to determine the crystallinity of starch from white breads fermented with yeast only or sourdough. Results indicated higher total crystallinity in sourdough bread due to the formation of resistant starch. To eliminate confounding factors, breads were baked identically and the temperature was set for storage experiments (286). Da Ros et al. (287) compared straight dough and sourdough bread baked under the same conditions and reported a higher resistant starch content in sourdough bread. Higher levels of RS in sourdough products were confirmed also by several other studies, but quantitatively the increases were low and not shown to result in measurable health effects (275, 288–290).
Data on different sourdough additions in tef bread making revealed a clear trend; resistant starch content increased significantly, whereas slowly and rapidly digestible starch decreased with increased sourdough addition to the bread recipe. However, after 5 days of storage, resistant starch values for all recipes were no longer different (291). In contrast, a single study discovered the lowest level of resistant starch in sourdough bread, with lactic acid having only a marginal effect on resistant starch content when compared to yeast-fermented control bread (292).
In summary, all studies with exception of Amaral et al. (292) showed a distinct but small increase in resistant starch in sourdough bread. Confounding factors such as different baking procedures can be excluded because the cited literature applied identical baking conditions (time and temperature) when comparing straight dough and sourdough bread (286). Nonetheless, the storage time may have a significant impact on the resistant starch content (291). The increase in resistant starch may be caused by the presence of acids, which promoted starch retrogradation after heat application during the baking step. However, the degree of gelatinization, porosity and other compounds may also have an impact (3).
Effect of fermentation on glycemic responses and role of induced bio-acids
Several studies reported a lower GI (3, 275, 285, 293, 294) and an improved insulin response (295) of sourdough bread. Several underlying mechanisms such as the previously discussed resistant starch content, have been proposed (see above). Furthermore, organic acids added to food have been shown to reduce post-ingestion glycemic response and have the potential to reduce the gastric emptying rate and increase meal-induced satiation (285, 296–299). However, using paracetamol adsorption (300) or the 13\u00B0C-octanoic acid breath-test (301) as a marker of gastric emptying, showed no differences in the rate of gastric emptying after ingesting breads made with different ferments. Polese et al. (302) observed that sourdough croissants have a faster gastric emptying when compared to croissants fermented with baker’s yeast only. In contrast, Rizzello et al. (303) showed that gastric emptying after consuming sourdough bread was faster compared to straight dough bread.
In addition to lower blood glucose responses, sourdough bread consumption was linked to lower insulin levels. For example, Juntunen et al. (295) supplied standardized amounts of sourdough (40% pre-dough) rye bread to provide 50 g of bioavailable starch and measured blood and insulin responses in 19 healthy postmenopausal women. The rye breads were made from wholemeal or white rye flour, with varying amounts of added fiber, and they were compared to white wheat bread as a control. The insulin responses were lower in the rye breads. Further, blood glucose responses did not differ between the sourdough rye breads and the control wheat bread, but less insulin was required to regulate blood sugar regardless of bran content. In an additional in vitro digestion test, the authors observed that starch hydrolysis was slower in all rye breads than in the white wheat control, and that the structure of the continuous matrix and starch granules differed between the rye and white wheat control breads (295). Apart from bio-acid effects, differences in matrix, starch granule structure, and resistant starch content may explain variations in blood glucose and insulin levels (304–307).
Although it is often suggested that sourdough bread results in a lower glycemic response than yeast fermented bread, data appear to be conflicting. This may result from improper control conditions, in which other variables than only sourdough vs. yeast dough making differ, such as comparing a rye sourdough bread with a yeast wheat bread. In such a comparison also grain type used, flour characteristics, fiber content and bread structure will impact on the glycemic response. Arora et al. (2) compiled data from 22 studies with in vivo challenges, and concluded that the average GI of sourdough bread was significantly lower compared to straight dough bread. However, none of these 22 study sources were cited making a critical evaluation of the study conditions and inclusion criteria for combining the results impossible. A recent systematic review and critical analysis of nutritional benefits of sourdough, by Ribet et al. (8), concluded that 50% of medical studies addressing the effects of sourdough fermentation in GI showed no effect, which leaves us to follow the conclusion that an effect of sourdough fermentation on GI is not substantiated.
Summary and conclusion
The definition of the term “sourdough” as a dough fermented with lactic acid bacteria in addition to yeasts is widely accepted in the scientific literature but there is no established and internationally recognized legal definition of sourdough bread. Descriptions and regulatory definitions differ among countries (158). In the world of artisanal bakers all have their own favorable ‘mother-sourdough’. Apart from commonalities, such as a high level of lactic acid bacteria and higher levels of activity, sourdoughs differ in composition with respect to microbiota subclasses, enzymes and metabolic activity, and the proportion of the flour that is fermented. Most research addressing the effects of sourdough fermentation on compositional changes, used experimental sourdoughs and fermentation conditions, usually leading to significant acidification of the dough to levels of pH < 4.5. Addition of specific microbiota or enzymes (e.g., isolated specific strains of lactobacilli or specific fungal enzymes) leads to a reduction of antinutritive or inflammatory compounds in the dough. However, results from small scale experiments can therefore not be generalized to sourdough breads that are commonly sold. In this respect, analysis of bread samples purchased in the market showed that most of these had pH levels of >5.0, substantially above desired levels of pH < 4.5 to obtain desired changes in composition (158). During several decades of research, compositional changes often suggested to be beneficial for health, such as phytate reduction and increased level of unbound minerals, decrease in FODMAPs and gluten immunogenic peptides, generation of bioactive peptides and increase in free amino acids, etc., in dough and bread, have been reported. Despite these observations, significant health effects on well-defined clinical endpoints such as diabetes risk reduction, improved weight management, reduction of gluten intolerances and improved bone mineral density as a result of sourdough bread consumption have not been clearly shown. Accordingly, there appears to be a clear discrepancy between observations in vitro/vivo tests with experimental sourdoughs and supporting data on health effects from human clinical studies. Because of this situation, health authorities such as EFSA (EU) or FDA (United States) have thus so far not approved the use of any sourdough health benefit claim.
Overall, we conclude that although a broad range of sourdough related health benefits are praised in publications, social media and by bakers, a sound evidence base for measurable effects on health related clinical endpoints has not been established. Our conclusions align with those of Ribet et al. (8), who pointed to conflicting evidence and inconsistent results or beneficial effects that appeared not to be significant. Both, sourdough fermentation and prolonged yeast fermentation result in a significant degradation of FODMAPs (range 50–85%) and inclusion of specific microbiota can induce almost complete degradation. Such reductions help mitigate gas formation related intestinal discomfort in IBS patients (8). In combination with raw materials delivering low amounts of harmful proteins, suitable products for patients with wheat related disorders can be produced by sourdough fermentation, e.g., products from oat for CD or einkorn for NCWS. Nevertheless, this conclusion has to be proven by in vivo tests and clinical studies. Sourdough fermentation might promote gut microbiota and health as well. However, a recent review pointed out eminent knowledge gaps which limits developments of a personalized nutrition (308). Although this review revealed that sourdough fermentation alone did not result in a direct and measurable health benefit, the sum of changes in structure of ingredients and metabolic products might have indirectly positive effects for human nutrition and wellbeing of consumers. Furthermore, increased shelf life of sourdough bread and reduced susceptibility for spoilage contribute to food safety.
Future sourdough studies should implement appropriate controls, with the only differing variable being the fermentation process (e.g., comparison of wholemeal wheat sourdough vs. wholemeal yeast bread) with identical ingredient recipes. Such studies should also include appropriate analysis of circulating target compounds in the intestine and in required biofluids in vivo. The latter is particularly important for determining the effects of potential bioactive peptides. To generalize effects of experimental sourdoughs to those sold in the market, they should have similar composition and processing.
We remain with consistent and reliable evidence that sourdough fermentation improves the sensory and textural quality of bread. Moreover, Sourdough fermentation particularly allows replacement of hyper-palatable baked goods, which are high in sugar, fat, and salt and were shown to increase the ad libitum food intake, with more wholesome recipes (309, 310). This beneficial impact on bread quality also relates to superior whole-grain or fiber-enriched products and Thus supports a healthy nutrition and lifestyle.
Author contributions
VD’A, MG, SD’A, and FB were mainly responsible for conceptualization, LC and HG assisted. VD’A, MG, LC, SD’A, and FB were preparing figures and tables. All authors wrote parts belonging to their expertise, read, and approved the final manuscript.
Acknowledgments
Maastricht University receives funds from the Dutch government (TKI1601P01) for the international research program “wellonwheat?” 2017-2023, addressing the effects of yeast and sourdough processing of bread wheat, spelt, and emmer and health effects in humans. Michael Gänzle acknowledges financial support from the Canada Research Chairs Program. Stefano D’Amico acknowledges the K-Center “Feed and Food Quality Safety and Innovation” (FFoQSI) funded by the COMET program of FFG. Heinrich Grausgruber and Lisa Call acknowledge funding by the Government of Lower Austria for work within the Danube Allergy Research Cluster (K3-T-74/001-2019).
Conflict of interest
The authors declare that the research was conducted in the absence of any commercial or financial relationships that could be construed as a potential conflict of interest.
Publisher’s note
All claims expressed in this article are solely those of the authors and do not necessarily represent those of their affiliated organizations, or those of the publisher, the editors and the reviewers. Any product that may be evaluated in this article, or claim that may be made by its manufacturer, is not guaranteed or endorsed by the publisher.
Footnotes
References
1. Arendt, EK, Moroni, A, and Zannini, E. Medical nutrition therapy: use of sourdough lactic acid Bacteria as a cell factory for delivering functional biomolecules and food ingredients in gluten free bread. Microb Cell Factories. (2011) 10:S15. doi: 10.1186/1475-2859-10-S1-S15
2. Arora, K, Ameur, H, Polo, A, Di Cagno, R, Rizzello, CG, and Gobbetti, M. Thirty years of knowledge on sourdough fermentation: a systematic Review. Trends Food Sci Technol. (2021) 108:71–83. doi: 10.1016/J.Tifs.2020.12.008
3. Poutanen, K, Flander, L, and Katina, K. Sourdough and cereal fermentation in a nutritional perspective. Food Microbiol. (2009) 26:693–9. doi: 10.1016/J.Fm.2009.07.011
4. Dima, C, Assadpour, E, Dima, S, and Jafari, SM. Bioavailability and bioaccessibility of food bioactive compounds; overview and assessment by in vitro methods. Compr Rev Food Sci Food Saf. (2020) 19:2862–84. doi: 10.1111/1541-4337.12623
5. Korem, T, Zeevi, D, Zmora, N, Weissbrod, O, Bar, N, Lotan-Pompan, M, et al. Bread affects clinical parameters and induces gut microbiome-associated personal glycemic responses. Cell Metab. (2017) 25:E5. doi: 10.1016/J.Cmet.2017.05.002
6. Laatikainen, R, Koskenpato, J, Hongisto, SM, Loponen, J, Poussa, T, Hillila, M, et al. Randomised clinical trial: low-Fodmap Rye bread vs. regular Rye bread to relieve the symptoms of irritable bowel syndrome. Aliment Pharmacol Ther. (2016) 44:460–70. doi: 10.1111/Apt.13726
7. Laatikainen, R, Koskenpato, J, Hongisto, SM, Loponen, J, Poussa, T, Huang, X, et al. Pilot study: comparison of sourdough wheat bread and yeast-fermented wheat bread in individuals with wheat sensitivity and irritable bowel syndrome. Nutrients. (2017) 9:1215. doi: 10.3390/Nu9111215
8. Ribet, L, Dessalles, R, Lesens, C, Brusselaers, N, and Durand-Dubief, M. Nutritional benefits of sourdoughs: a systematic Review. Adv Nutr. (2023) 14:22–9. doi: 10.1016/J.Advnut.2022.10.003
9. EFSA. Scientific opinion on the substantiation of a health claim related to high fibre sourdough Rye bread and reduction of post-prandial Glycaemic responses pursuant to article 13(5) of Regulation (Ec) no 1924/2006. EFSA J. (2014) 12:3837. doi: 10.2903/J.Efsa.2014.3837
10. Geisslitz, S, Shewry, P, Brouns, F, America, AHP, Caio, GPI, Daly, M, et al. Wheat Atis: characteristics and role in human disease. Front Nutr. (2021) 8:667370. doi: 10.3389/Fnut.2021.667370
11. Fraberger, V, Call, LM, Domig, KJ, and D'amico, S. Applicability of yeast fermentation to reduce Fructans and other Fodmaps. Nutrients. (2018) 10:1247. doi: 10.3390/Nu10091247
12. Fraberger, V, Ladurner, M, Nemec, A, Grunwald-Gruber, C, Call, LM, Hochegger, R, et al. Insights into the potential of sourdough-related lactic acid Bacteria to degrade proteins in wheat. Microorganisms. (2020) 8:1689. doi: 10.3390/Microorganisms8111689
13. Graça, C, Lima, A, Raymundo, A, and Sousa, I. Sourdough fermentation as a tool to improve the nutritional and health-promoting properties of its derived-products. Fermentation. (2021) 7:246. doi: 10.3390/Fermentation7040246
14. Huang, X, Schuppan, D, Rojas Tovar, LE, Zevallos, VF, Loponen, J, and Ganzle, M. Sourdough fermentation degrades wheat alpha-amylase/trypsin inhibitor (Ati) and reduces pro-inflammatory activity. Foods. (2020) 9:943. doi: 10.3390/Foods9070943
15. Won, S, Curtis, J, and Ganzle, M. Lc-Ms/Ms quantitation of alpha-amylase/trypsin inhibitor Cm3 and glutathione during wheat sourdough Breadmaking. J Appl Microbiol. (2022) 133:120–9. doi: 10.1111/Jam.15346
16. Yildirim, RM, and Arici, M. Effect of the fermentation temperature on the degradation of Phytic acid in whole-wheat sourdough bread. LWT. (2019) 112:108224. doi: 10.1016/J.Lwt.2019.05.122
17. Bach Knudsen, KE, Lærke, HN, Steenfeldt, S, Hedemann, MS, and Jørgensen, H. In vivo methods to study the digestion of starch in pigs and poultry. Anim Feed Sci Technol. (2006) 130:114–35. doi: 10.1016/J.Anifeedsci.2006.01.020
18. Henze, LJ, Koehl, NJ, O'shea, JP, Kostewicz, ES, Holm, R, and Griffin, BT. The pig as a preclinical model for predicting Oral bioavailability and in vivo performance of pharmaceutical Oral dosage forms: a Pearrl Review. J Pharm Pharmacol. (2019) 71:581–602. doi: 10.1111/Jphp.12912
19. Rowan, AM, Moughan, PJ, Wilson, MN, Maher, K, and Tasman-Jones, C. Comparison of the Ileal and Faecal digestibility of dietary amino acids in adult humans and evaluation of the pig as a model animal for digestion studies in man. Br J Nutr. (1994) 71:29–42. doi: 10.1079/Bjn19940108
20. Minekus, M, Marteau, P, Havenaar, R, and Veld, JHJ. A multicompartmental dynamic computer-controlled model simulating the stomach and Small intestine. Altern Lab Anim. (1995) 23:197–209. doi: 10.1177/026119299502300205
21. Minekus, M, Alminger, M, Alvito, P, Ballance, S, Bohn, T, Bourlieu, C, et al. A standardised static in vitro digestion method suitable for food - an international consensus. Food Funct. (2014) 5:1113–24. doi: 10.1039/C3fo60702j
22. Egger, L, Schlegel, P, Baumann, C, Stoffers, H, Guggisberg, D, Brugger, C, et al. Physiological comparability of the harmonized Infogest in vitro digestion method to in vivo pig digestion. Food Res Int. (2017) 102:567–74. doi: 10.1016/J.Foodres.2017.09.047
23. Fernandes, JM, Madalena, DA, Pinheiro, AC, and Vicente, AA. Rice in vitro digestion: application of Infogest harmonized protocol for glycemic index determination and starch morphological study. J Food Sci Technol. (2020) 57:1393–404. doi: 10.1007/S13197-019-04174-X
24. Mat, DJL, Le Feunteun, S, Michon, C, and Souchon, I. In vitro digestion of foods using Ph-stat and the Infogest protocol: impact of matrix structure on digestion kinetics of macronutrients, proteins and lipids. Food Res Int. (2016) 88:226–33. doi: 10.1016/J.Foodres.2015.12.002
25. Muleya, M, Young, SD, and Bailey, EH. A stable isotope approach to accurately determine Iron and zinc bioaccessibility in cereals and legumes based on a modified Infogest static in vitro digestion method. Food Res Int. (2021) 139:109948. doi: 10.1016/J.Foodres.2020.109948
26. Santos-Hernandez, M, Alfieri, F, Gallo, V, Miralles, B, Masi, P, Romano, A, et al. Compared digestibility of plant protein isolates by using the Infogest digestion protocol. Food Res Int. (2020) 137:109708. doi: 10.1016/J.Foodres.2020.109708
27. Tan, Y, Zhang, Z, Muriel Mundo, J, and Mcclements, DJ. Factors impacting lipid digestion and nutraceutical bioaccessibility assessed by standardized gastrointestinal model (Infogest): emulsifier type. Food Res Int. (2020) 137:109739. doi: 10.1016/J.Foodres.2020.109739
28. Fernandez-Garcia, E, Carvajal-Lerida, I, and Perez-Galvez, A. In vitro bioaccessibility assessment as a prediction tool of nutritional efficiency. Nutr Res. (2009) 29:751–60. doi: 10.1016/J.Nutres.2009.09.016
29. Foltz, M, Meynen, EE, Bianco, V, Van Platerink, C, Koning, TMMG, and Kloek, J. Angiotensin converting enzyme inhibitory peptides from a Lactotripeptide-enriched Milk beverage are absorbed intact into the Circulation1. J Nutr. (2007) 137:953–8. doi: 10.1093/Jn/137.4.953
30. Miner-Williams, WM, Stevens, BR, and Moughan, PJ. Are intact peptides absorbed from the healthy gut in the adult human? Nutr Res Rev. (2014) 27:308–29. doi: 10.1017/S0954422414000225
31. Van Der Pijl, PC, Kies, AK, Ten Have, GA, Duchateau, GS, and Deutz, NE. Pharmacokinetics of proline-rich tripeptides in the pig. Peptides. (2008) 29:2196–202. doi: 10.1016/J.Peptides.2008.08.011
32. Melchior, C, Douard, V, Coeffier, M, and Gourcerol, G. Fructose and irritable bowel syndrome. Nutr Res Rev. (2020) 33:235–43. doi: 10.1017/S0954422420000025
33. Asri, N, and Rostami-Nejad, M. Gluten-Related Disorders Definition In: M Rostami-Nejad, editor. Gluten-Related Disorders. Cambridge, MA: Academic Press (2022)
34. Scherf, KA. Immunoreactive cereal proteins in wheat allergy, non-celiac gluten/wheat sensitivity (Ncgs) and celiac disease. Curr Opin Food Sci. (2019) 25:35–41. doi: 10.1016/J.Cofs.2019.02.003
35. Algera, JP, Demir, D, Tornblom, H, Nybacka, S, Simren, M, and Storsrud, S. Low Fodmap diet reduces gastrointestinal symptoms in irritable bowel syndrome and clinical response could be predicted by symptom severity: a randomized crossover trial. Clin Nutr. (2022) 41:2792–800. doi: 10.1016/J.Clnu.2022.11.001
36. Armentia, A, Sanchez-Monge, R, Gomez, L, Barber, D, and Salcedo, G. In vivo allergenic activities of eleven purified members of a major allergen family from wheat and barley flour. Clin Exp Allergy. (1993) 23:410–5. doi: 10.1111/J.1365-2222.1993.Tb00347.X
37. Bellinghausen, I, Weigmann, B, Zevallos, V, Maxeiner, J, Reissig, S, Waisman, A, et al. Wheat amylase-trypsin inhibitors exacerbate intestinal and airway allergic immune responses in humanized mice. J Allergy Clin Immunol. (2019) 143:E4. doi: 10.1016/J.Jaci.2018.02.041
38. Biesiekierski, JR, Peters, SL, Newnham, ED, Rosella, O, Muir, JG, and Gibson, PR. No effects of gluten in patients with self-reported non-celiac gluten sensitivity after dietary reduction of fermentable, poorly absorbed, short-chain carbohydrates. Gastroenterology. (2013) 145:E1–3. doi: 10.1053/J.Gastro.2013.04.051
39. Crawley, C, Savino, N, Halby, C, Sander, SD, Andersen, AN, Arumugam, M, et al. The effect of gluten in adolescents and Young adults with gastrointestinal symptoms: a blinded randomised cross-over trial. Aliment Pharmacol Ther. (2022) 55:1116–27. doi: 10.1111/Apt.16914
40. Huebener, S, Tanaka, CK, Uhde, M, Zone, JJ, Vensel, WH, Kasarda, DD, et al. Specific nongluten proteins of wheat are novel target antigens in celiac disease humoral response. J Proteome Res. (2015) 14:503–11. doi: 10.1021/Pr500809b
41. Pastorello, EA, Farioli, L, Conti, A, Pravettoni, V, Bonomi, S, Iametti, S, et al. Wheat Ige-mediated food allergy in European patients: alpha-amylase inhibitors, lipid transfer proteins and low-molecular-weight Glutenins. Allergenic molecules recognized by double-blind, placebo-controlled food challenge. Int Arch Allergy Immunol. (2007) 144:10–22. doi: 10.1159/000102609
42. Peters, SL, Biesiekierski, JR, Yelland, GW, Muir, JG, and Gibson, PR. Randomised clinical trial: gluten may cause depression in subjects with non-coeliac gluten sensitivity - an exploratory clinical study. Aliment Pharmacol Ther. (2014) 39:1104–12. doi: 10.1111/Apt.12730
43. Sanchez, D, Stepanova Honzova, S, Hospodkova, M, Hoffmanova, I, Habova, V, Halada, P, et al. Occurrence of serum antibodies against wheat alpha-amylase inhibitor 0.19 in celiac disease. Physiol Res. (2018) 67:613–22. doi: 10.33549/Physiolres.933876
44. Yu, T, Hu, S, Min, F, Li, J, Shen, Y, Yuan, J, et al. Wheat amylase trypsin inhibitors aggravate intestinal inflammation associated with celiac disease mediated by gliadin in Balb/C mice. Foods. (2022) 11:1559. doi: 10.3390/Foods11111559
45. Zevallos, VF, Raker, V, Tenzer, S, Jimenez-Calvente, C, Ashfaq-Khan, M, Russel, N, et al. Nutritional wheat amylase-trypsin inhibitors promote intestinal inflammation via activation of myeloid cells. Gastroenterology. (2017) 152:E12. doi: 10.1053/J.Gastro.2016.12.006
46. Sollid, LM, Qiao, SW, Anderson, RP, Gianfrani, C, and Koning, F. Nomenclature and listing of celiac disease relevant gluten T-cell epitopes restricted by Hla-Dq molecules. Immunogenetics. (2012) 64:455–60. doi: 10.1007/S00251-012-0599-Z
47. Parzanese, I, Qehajaj, D, Patrinicola, F, Aralica, M, Chiriva-Internati, M, Stifter, S, et al. Celiac disease: from pathophysiology to treatment. World J Gastroenterol. (2017) 8:27–38. doi: 10.4291/Wjgp.V8.I2.27
48. Singh, P, Arora, A, Strand, TA, Leffler, DA, Catassi, C, Green, PH, et al. Global prevalence of celiac disease: systematic Review and Meta-analysis. Clin Gastroenterol Hepatol. (2018) 16:E2. doi: 10.1016/J.Cgh.2017.06.037
49. Catassi, C, Gatti, S, and Lionetti, E. World perspective and celiac disease epidemiology. Dig Dis Sci. (2015) 33:141–6. doi: 10.1159/000369518
50. Rubio-Tapia, A, Ludvigsson, JF, Brantner, TL, Murray, JA, and Everhart, JE. The prevalence of celiac disease in the United States. Am J Gastroenterol. (2012) 107:1538–44. doi: 10.1038/Ajg.2012.219
51. Smulders, MJM, Van De Wiel, CCM, Van Den Broeck, HC, Van Der Meer, IM, Israel-Hoevelaken, TPM, Timmer, RD, et al. Oats in healthy gluten-free and regular diets: a perspective. Food Res Int. (2018) 110:3–10. doi: 10.1016/J.Foodres.2017.11.031
52. Leisova-Svobodova, L, Sovova, T, and Dvoracek, V. Analysis of oat seed transcriptome with regards to proteins involved in celiac disease. Sci Rep. (2022) 12:8660. doi: 10.1038/S41598-022-12711-6
53. Mujico, JR, Mitea, C, Gilissen, LJWJ, De Ru, A, Van Veelen, P, Smulders, MJM, et al. Natural variation in Avenin epitopes among oat varieties: implications for celiac disease. J Cereal Sci. (2011) 54:8–12. doi: 10.1016/J.Jcs.2010.09.007
54. Comino, I, Real, A, De Lorenzo, L, Cornell, H, López-Casado, MÁ, Barro, F, et al. Diversity in oat potential immunogenicity: basis for the selection of oat varieties with no toxicity in coeliac disease. Gut. (2011) 60:915–22. doi: 10.1136/gut.2010.225268
55. Gilissen, L, Van Der Meer, IM, and Smulders, MJM. Why oats are safe and healthy for celiac disease patients. Med Sci (Basel). (2016) 4:21. doi: 10.3390/sMedsci4040021
56. Gilissen, LJWJ, Van Der Meer, IM, and Smulders, MJM. Reducing the incidence of allergy and intolerance to cereals. J Cereal Sci. (2014) 59:337–53. doi: 10.1016/J.Jcs.2014.01.005
57. Huang, X, Ahola, H, Daly, M, Nitride, C, Mills, EC, and Sontag-Strohm, T. Quantification of barley contaminants in gluten-free oats by four gluten Elisa kits. J Agric Food Chem. (2022) 70:2366–73. doi: 10.1021/Acs.Jafc.1c07715
58. Hoffmanova, I, Sanchez, D, Szczepankova, A, and Tlaskalova-Hogenova, H. The pros and cons of using oat in a gluten-free diet for celiac patients. Nutrients. (2019) 11:2345. doi: 10.3390/Nu11102345
59. Demirkesen, I, and Ozkaya, B. Recent strategies for tackling the problems in gluten-free diet and products. Crit Rev Food Sci Nutr. (2022) 62:571–97. doi: 10.1080/10408398.2020.1823814
60. Falcomer, AL, Santos Araujo, L, Farage, P, Santos Monteiro, J, Yoshio Nakano, E, and Puppin Zandonadi, R. Gluten contamination in food services and industry: a systematic Review. Crit Rev Food Sci Nutr. (2020) 60:479–93. doi: 10.1080/10408398.2018.1541864
61. Farage, P, De Medeiros Nobrega, YK, Pratesi, R, Gandolfi, L, Assuncao, P, and Zandonadi, RP. Gluten contamination in gluten-free bakery products: a risk for coeliac disease patients. Public Health Nutr. (2017) 20:413–6. doi: 10.1017/S1368980016002433
62. Farage, P, Zandonadi, RP, Gandolfi, L, Pratesi, R, Falcomer, AL, Araujo, LS, et al. Accidental gluten contamination in traditional lunch meals from food services in Brasilia, Brazil. Nutrients. (2019) 11:1924. doi: 10.3390/Nu11081924
63. Koerner, TB, Cleroux, C, Poirier, C, Cantin, I, La Vieille, S, Hayward, S, et al. Gluten contamination of naturally gluten-free flours and starches used by Canadians with celiac disease. Food Addit Contam Part A Chem Anal Control Expo Risk Assess. (2013) 30:2017–21. doi: 10.1080/19440049.2013.840744
64. Lee, HJ, Anderson, Z, and Ryu, D. Gluten contamination in foods labeled as "gluten free" in the United States. J Food Prot. (2014) 77:1830–3. doi: 10.4315/0362-028x.Jfp-14-149
65. Thompson, T, Lee, AR, and Grace, T. Gluten contamination of grains, seeds, and flours in the United States: a pilot study. J Am Diet Assoc. (2010) 110:937–40. doi: 10.1016/J.Jada.2010.03.014
66. Verma, AK, Gatti, S, Galeazzi, T, Monachesi, C, Padella, L, Baldo, GD, et al. Gluten contamination in naturally or labeled gluten-free products marketed in Italy. Nutrients. (2017) 9:115. doi: 10.3390/Nu9020115
67. D'amico, S, Schoenlechner, R, Tömösköszi, S, and Langó, B. Proteins and amino acids of kernels. Pseudocereals. (2017). doi: 10.1002/9781118938256.Ch5
68. Lee, S, Han, Y, Do, JR, and Oh, S. Allergenic potential and enzymatic resistance of buckwheat. Nutr Res Pract. (2013) 7:3–8. doi: 10.4162/Nrp.2013.7.1.3
69. Cianferoni, A. Wheat allergy: diagnosis and management. J Asthma Allergy. (2016) 9:13–25. doi: 10.2147/Jaa.S81550
70. Kotaniemi-Syrjanen, A, Palosuo, K, Jartti, T, Kuitunen, M, Pelkonen, AS, and Makela, MJ. The prognosis of wheat hypersensitivity in children. Pediatr Allergy Immunol. (2010) 21:E421–8. doi: 10.1111/J.1399-3038.2009.00946.X
71. Zuidmeer, L, Goldhahn, K, Rona, RJ, Gislason, D, Madsen, C, Summers, C, et al. The prevalence of plant food allergies: a systematic Review. J Allergy Clin Immunol. (2008) 121:E4. doi: 10.1016/J.Jaci.2008.02.019
72. Bernstein, JA, Lang, DM, Khan, DA, Craig, T, Dreyfus, D, Hsieh, F, et al. The diagnosis and management of acute and chronic Urticaria: 2014 update. J Allergy Clin Immunol. (2014) 133:1270–7. doi: 10.1016/J.Jaci.2014.02.036
73. Black, CJ, and Ford, AC. Global burden of irritable bowel syndrome: trends, predictions and risk factors. Nat Rev Gastroenterol Hepatol. (2020) 17:473–86. doi: 10.1038/S41575-020-0286-8
74. Ohman, L, and Simren, M. Pathogenesis of Ibs: role of inflammation, immunity and Neuroimmune interactions. Nat Rev Gastroenterol Hepatol. (2010) 7:163–73. doi: 10.1038/Nrgastro.2010.4
75. El-Salhy, M. Irritable bowel syndrome: diagnosis and pathogenesis. World J Gastroenterol. (2012) 18:5151–63. doi: 10.3748/Wjg.V18.I37.5151
76. Oswiecimska, J, Szymlak, A, Roczniak, W, Girczys-Poledniok, K, and Kwiecien, J. New insights into the pathogenesis and treatment of irritable bowel syndrome. Adv Med Sci. (2017) 62:17–30. doi: 10.1016/J.Advms.2016.11.001
77. Kucek, LK, Veenstra, LD, Amnuaycheewa, P, and Sorrells, ME. A grounded guide to gluten: how modern genotypes and processing impact wheat sensitivity. Compr Rev Food Sci Food Saf. (2015) 14:285–302. doi: 10.1111/1541-4337.12129
78. Infantino, M, Meacci, F, Grossi, V, Macchia, D, and Manfredi, M. Anti-gliadin antibodies in non-celiac gluten sensitivity. Minerva Gastroenterol Dietol. (2017) 63:1–4. doi: 10.23736/S1121-421x.16.02351-5
79. Infantino, M, Manfredi, M, Meacci, F, Grossi, V, Severino, M, Benucci, M, et al. Diagnostic accuracy of anti-gliadin antibodies in non celiac gluten sensitivity (Ncgs) patients: a dual statistical approach. Clin Chim Acta. (2015) 451:135–41. doi: 10.1016/J.Cca.2015.09.017
80. Sun, Y, Chen, X, Wang, S, Deng, M, Xie, Y, Wang, X, et al. Gluten-free diet reduces the risk of irritable bowel syndrome: a Mendelian randomization analysis. Front Genet. (2021) 12:684535. doi: 10.3389/Fgene.2021.684535
81. Reuze, A, Delvert, R, Perrin, L, Benamouzig, R, Sabate, JM, Bouchoucha, M, et al. Association between self-reported gluten avoidance and irritable bowel syndrome: findings of the Nutrinet-Sante study. Nutrients. (2021) 13:4147. doi: 10.3390/Nu13114147
82. Aziz, I. The global phenomenon of self-reported wheat sensitivity. Am J Gastroenterol. (2018) 113:945–8. doi: 10.1038/S41395-018-0103-Y
84. Struyf, N, Laurent, J, Verspreet, J, Verstrepen, KJ, and Courtin, CM. Saccharomyces cerevisiae and Kluyveromyces marxianus Cocultures allow reduction of fermentable oligo-, Di-, and monosaccharides and polyols levels in whole wheat bread. J Agric Food Chem. (2017) 65:8704–13. doi: 10.1021/Acs.Jafc.7b02793
85. Li, Q, Loponen, J, and Ganzle, MG. Characterization of the extracellular Fructanase FruA in Lactobacillus crispatus and its contribution to Fructan hydrolysis in Breadmaking. J Agric Food Chem. (2020) 68:8637–47. doi: 10.1021/Acs.Jafc.0c02313
86. Goh, YJ, Lee, JH, and Hutkins, RW. Functional analysis of the Fructooligosaccharide utilization operon in Lactobacillus Paracasei 1195. Appl Environ Microbiol. (2007) 73:5716–24. doi: 10.1128/Aem.00805-07
87. Laurent, J, Timmermans, E, Struyf, N, Verstrepen, KJ, and Courtin, CM. Variability in yeast Invertase activity determines the extent of Fructan hydrolysis during wheat dough fermentation and final Fodmap levels in bread. Int J Food Microbiol. (2020) 326:108648. doi: 10.1016/J.Ijfoodmicro.2020.108648
88. Nilsson, U, Öste, R, and Jägerstad, M. Cereal Fructans: hydrolysis by yeast Invertase, in vitro and during fermentation. J Cereal Sci. (1987) 6:53–60. doi: 10.1016/S0733-5210(87)80040-1
89. Zheng, J, Wittouck, S, Salvetti, E, Franz, C, Harris, HMB, Mattarelli, P, et al. A taxonomic note on the genus Lactobacillus: description of 23 novel genera, emended description of the genus Lactobacillus Beijerinck 1901, and union of Lactobacillaceae and Leuconostocaceae. Int J Syst Evol Microbiol. (2020) 70:2782–858. doi: 10.1099/Ijsem.0.004107
90. Ganzle, MG, and Follador, R. Metabolism of oligosaccharides and starch in lactobacilli: a Review. Front Microbiol. (2012) 3:340. doi: 10.3389/Fmicb.2012.00340
91. Teixeira, JS, Abdi, R, Su, MS, Schwab, C, and Ganzle, MG. Functional characterization of sucrose phosphorylase and Scrr, a regulator of sucrose metabolism in Lactobacillus Reuteri. Food Microbiol. (2013) 36:432–9. doi: 10.1016/J.Fm.2013.07.011
92. Teixeira, JS, Mcneill, V, and Ganzle, MG. Levansucrase and sucrose Phoshorylase contribute to Raffinose, Stachyose, and Verbascose metabolism by lactobacilli. Food Microbiol. (2012) 31:278–84. doi: 10.1016/J.Fm.2012.03.003
93. Gänzle, MG. Lactic metabolism revisited: metabolism of lactic acid Bacteria in food fermentations and food spoilage. Curr Opin Food Sci. (2015) 2:106–17. doi: 10.1016/J.Cofs.2015.03.001
94. Qiao, N, Wittouck, S, Mattarelli, P, Zheng, J, Lebeer, S, Felis, GE, et al. After the storm-perspectives on the taxonomy of Lactobacillaceae. JDS Commun. (2022) 3:222–7. doi: 10.3168/Jdsc.2021-0183
95. Loponen, J, and Ganzle, MG. Use of sourdough in low Fodmap baking. Foods. (2018) 7:96. doi: 10.3390/Foods7070096
96. Atkinson, FS, Foster-Powell, K, and Brand-Miller, JC. International tables of glycemic index and glycemic load values: 2008. Diabetes Care. (2008) 31:2281–3. doi: 10.2337/Dc08-1239
97. Scherf, KA, Brockow, K, Biedermann, T, Koehler, P, and Wieser, H. Wheat-dependent exercise-induced anaphylaxis. Clin Exp Allergy. (2016) 46:10–20. doi: 10.1111/Cea.12640
98. Aihara, Y, Takahashi, Y, Kotoyori, T, Mitsuda, T, Ito, R, Aihara, M, et al. Frequency of food-dependent, exercise-induced anaphylaxis in Japanese junior-high-school students. J Allergy Clin Immunol. (2001) 108:1035–9. doi: 10.1067/Mai.2001.119914
99. Baccioglu, A, Kalpaklıoglu, F, and Altan, G. Review of wheat dependent exercise induced anaphylaxis with two cases, and a new co-factor-myorelaxant. J Immunol Clin Res. (2017) 4:1045.
100. Afify, SM, and Pali-Scholl, I. Adverse reactions to food: the female dominance–a secondary publication and update. World Allergy Organ J. (2017) 10:43. doi: 10.1186/S40413-017-0174-Z
101. Araya, M, Bascuñán, KA, Alarcón-Sajarópulos, D, Cabrera-Chávez, F, Oyarzún, A, Fernández, A, et al. Living with gluten and other food intolerances: self-reported diagnoses and management. Nutrients. (2020) 12:1892. doi: 10.3390/Nu12061892
102. Ontiveros, N, Rodriguez-Bellegarrigue, CI, Galicia-Rodriguez, G, Vergara-Jimenez, MJ, Zepeda-Gomez, EM, Aramburo-Galvez, JG, et al. Prevalence of self-reported gluten-related disorders and adherence to a gluten-free diet in Salvadoran adult population. Int J Environ Res Public Health. (2018) 15:786. doi: 10.3390/Ijerph15040786
103. Aramburo-Galvez, JG, Beltran-Cardenas, CE, Geralda Andre, T, Carvalho Gomes, I, Macedo-Callou, MA, Braga-Rocha, EM, et al. Prevalence of adverse reactions to gluten and people going on a gluten-free diet: a survey study conducted in Brazil. Medicina (Kaunas). (2020) 56:163. doi: 10.3390/Medicina56040163
104. Potter, M, Jones, MP, Walker, MM, Koloski, NA, Keely, S, Holtmann, G, et al. Incidence and prevalence of self-reported non-coeliac wheat sensitivity and gluten avoidance in Australia. Med J Aust. (2020) 212:126–31. doi: 10.5694/Mja2.50458
105. Potter, MDE, Walker, MM, Jones, MP, Koloski, NA, Keely, S, and Talley, NJ. Wheat intolerance and chronic gastrointestinal symptoms in an Australian population-based study: association between wheat sensitivity, celiac disease and functional gastrointestinal disorders. Am J Gastroenterol. (2018) 113:1036–44. doi: 10.1038/S41395-018-0095-7
106. Cárdenas-Torres, FI, Cabrera-Chávez, F, Figueroa-Salcido, OG, and Ontiveros, N. Non-celiac gluten sensitivity: an update. Medicina. (2021) 57:526. doi: 10.3390/Medicina57060526
107. Aziz, I, Lewis, NR, Hadjivassiliou, M, Winfield, SN, Rugg, N, Kelsall, A, et al. A Uk study assessing the population prevalence of self-reported gluten sensitivity and referral characteristics to secondary care. Eur J Gastroenterol Hepatol. (2014) 26:33–9. doi: 10.1097/01.Meg.0000435546.87251.F7
108. Van Gils, T, Nijeboer, P, Ce, IJ, Sanders, DS, Mulder, CJ, and Bouma, G. Prevalence and characterization of self-reported gluten sensitivity in the Netherlands. Nutrients. (2016) 8:714. doi: 10.3390/Nu8110714
109. Capannolo, A, Viscido, A, Barkad, MA, Valerii, G, Ciccone, F, Melideo, D, et al. Non-celiac gluten sensitivity among patients perceiving gluten-related symptoms. Digestion. (2015) 92:8–13. doi: 10.1159/000430090
110. Catassi, C, Alaedini, A, Bojarski, C, Bonaz, B, Bouma, G, Carroccio, A, et al. The overlapping area of non-celiac gluten sensitivity (Ncgs) and wheat-sensitive irritable bowel syndrome (Ibs): an update. Nutrients. (2017) 9:1268. doi: 10.3390/Nu9111268
111. Makharia, A, Catassi, C, and Makharia, GK. The overlap between irritable bowel syndrome and non-celiac gluten sensitivity: a clinical dilemma. Nutrients. (2015) 7:10417–26. doi: 10.3390/Nu7125541
112. Usai-Satta, P, Bassotti, G, Bellini, M, Oppia, F, Lai, M, and Cabras, F. Irritable bowel syndrome and gluten-related disorders. Nutrients. (2020) 12:1117. doi: 10.3390/Nu12041117
113. Quigley, EM, Fried, M, Gwee, KA, Khalif, I, Hungin, AP, Lindberg, G, et al. World gastroenterology organisation global guidelines irritable bowel syndrome: a global perspective update September 2015. J Clin Gastroenterol. (2016) 50:704–13. doi: 10.1097/Mcg.0000000000000653
114. Barberio, B, Houghton, LA, Yiannakou, Y, Savarino, EV, Black, CJ, and Ford, AC. Symptom stability in Rome iv vs Rome iii irritable bowel syndrome. Am J Gastroenterol. (2021) 116:362–71. doi: 10.14309/Ajg.0000000000000946
115. Lacy, BE, and Patel, NK. Rome criteria and a diagnostic approach to irritable bowel syndrome. J Clin Med. (2017) 6:99. doi: 10.3390/Jcm6110099
116. Oka, P, Parr, H, Barberio, B, Black, CJ, Savarino, EV, and Ford, AC. Global prevalence of irritable bowel syndrome according to Rome iii or iv criteria: a systematic Review and Meta-analysis. Lancet Gastroenterol Hepatol. (2020) 5:908–17. doi: 10.1016/S2468-1253(20)30217-X
117. Sperber, AD. Review article: epidemiology of Ibs and other bowel disorders of gut-brain interaction (Dgbi). Aliment Pharmacol Ther. (2021) 54:S1–S11. doi: 10.1111/Apt.16582
118. De Giorgio, R, Volta, U, and Gibson, PR. Sensitivity to wheat, gluten and Fodmaps in Ibs: facts or fiction? Gut. (2016) 65:169–78. doi: 10.1136/Gutjnl-2015-309757
119. Fedewa, A, and Rao, SS. Dietary fructose intolerance, Fructan intolerance and Fodmaps. Curr Gastroenterol Rep. (2014) 16:370. doi: 10.1007/S11894-013-0370-0
120. Dinicolantonio, JJ, and Lucan, SC. Is fructose malabsorption a cause of irritable bowel syndrome? Med Hypotheses. (2015) 85:295–7. doi: 10.1016/J.Mehy.2015.05.019
121. Fritscher-Ravens, A, Pflaum, T, Mosinger, M, Ruchay, Z, Rocken, C, Milla, PJ, et al. Many patients with irritable bowel syndrome Have atypical food allergies not associated with immunoglobulin E. Gastroenterology. (2019) 157:E5. doi: 10.1053/J.Gastro.2019.03.046
122. Fasano, A, Sapone, A, Zevallos, V, and Schuppan, D. Nonceliac gluten sensitivity. Gastroenterology. (2015) 148:1195–204. doi: 10.1053/J.Gastro.2014.12.049
123. Algera, JP, Storsrud, S, Lindstrom, A, Simren, M, and Tornblom, H. Gluten and Fructan intake and their associations with gastrointestinal symptoms in irritable bowel syndrome: a food diary study. Clin Nutr. (2021) 40:5365–72. doi: 10.1016/J.Clnu.2021.09.002
124. Pirkola, L, Laatikainen, R, Loponen, J, Hongisto, SM, Hillila, M, Nuora, A, et al. Low-Fodmap vs regular Rye bread in irritable bowel syndrome: randomized Smartpill((R)) study. World J Gastroenterol. (2018) 24:1259–68. doi: 10.3748/Wjg.V24.I11.1259
125. Silvester, JA, Comino, I, Kelly, CP, Sousa, C, Duerksen, DR, Bernstein, CN, et al. Most patients with celiac disease on gluten-free diets consume measurable amounts of gluten. Gastroenterology. (2020) 158:E1. doi: 10.1053/J.Gastro.2019.12.016
126. Syage, JA, Kelly, CP, Dickason, MA, Ramirez, AC, Leon, F, Dominguez, R, et al. Determination of gluten consumption in celiac disease patients on a gluten-free diet. Am J Clin Nutr. (2018) 107:201–7. doi: 10.1093/Ajcn/Nqx049
127. Dieterich, W, and Zopf, Y. Gluten and Fodmaps-sense of a restriction/when is restriction necessary? Nutrients. (2019) 11:1957. doi: 10.3390/Nu11081957
128. Arentz-Hansen, H, Mcadam, SN, Molberg, O, Fleckenstein, B, Lundin, KE, Jorgensen, TJ, et al. Celiac lesion T cells recognize epitopes that cluster in regions of gliadins rich in proline residues. Gastroenterology. (2002) 123:803–9. doi: 10.1053/Gast.2002.35381
129. Tatham, AS, and Shewry, PR. Allergens to wheat and related cereals. Clin Exp Allergy. (2008) 38:1712–26. doi: 10.1111/J.1365-2222.2008.03101.X
130. Shan, L, Molberg, O, Parrot, I, Hausch, F, Filiz, F, Gray, GM, et al. Structural basis for gluten intolerance in celiac sprue. Science. (2002) 297:2275–9. doi: 10.1126/Science.1074129
131. Schalk, K, Lang, C, Wieser, H, Koehler, P, and Scherf, KA. Quantitation of the Immunodominant 33-Mer peptide from alpha-gliadin in wheat flours by liquid chromatography tandem mass spectrometry. Sci Rep. (2017) 7:45092. doi: 10.1038/Srep45092
132. Shewry, PR, and Tatham, AS. Improving wheat to remove coeliac epitopes but retain functionality. J Cereal Sci. (2016) 67:12–21. doi: 10.1016/J.Jcs.2015.06.005
133. Breiteneder, H, and Radauer, C. A classification of plant food allergens. J Allergy Clin Immunol. (2004) 113:821–30. doi: 10.1016/J.Jaci.2004.01.779
134. Junker, Y, Zeissig, S, Kim, SJ, Barisani, D, Wieser, H, Leffler, DA, et al. Wheat amylase trypsin inhibitors drive intestinal inflammation via activation of toll-like receptor 4. J Exp Med. (2012) 209:2395–408. doi: 10.1084/Jem.20102660
135. James, JM, Sixbey, JP, Helm, RM, Bannon, GA, and Burks, AW. Wheat alpha-amylase inhibitor: a second route of allergic sensitization. J Allergy Clin Immunol. (1997) 99:239–44. doi: 10.1016/S0091-6749(97)70103-9
136. Sanders, JW, Oomes, SJ, Membre, JM, Wegkamp, A, and Wels, M. Biodiversity of spoilage lactobacilli: phenotypic characterisation. Food Microbiol. (2015) 45:34–44. doi: 10.1016/J.Fm.2014.03.013
137. Tundo, S, Lupi, R, Lafond, M, Giardina, T, Larre, C, Denery-Papini, S, et al. Wheat Ati Cm3, Cm16 and 0.28 allergens produced in Pichia Pastoris display a different eliciting potential in food allergy to wheat (double dagger). Plants (Basel). (2018) 7:101. doi: 10.3390/Plants7040101
138. Van Ree, R. Clinical importance of non-specific lipid transfer proteins as food allergens. Biochem Soc Trans. (2002) 30:910–3. doi: 10.1042/Bst0300910
139. Weichel, M, Vergoossen, NJ, Bonomi, S, Scibilia, J, Ortolani, C, Ballmer-Weber, BK, et al. Screening the allergenic repertoires of wheat and maize with sera from double-blind, placebo-controlled food challenge positive patients. Allergy. (2006) 61:128–35. doi: 10.1111/J.1398-9995.2006.00999.X
140. Van Buul, VJ, and Brouns, FJPH. Health effects of wheat lectins: a Review. J Cereal Sci. (2014) 59:112–7. doi: 10.1016/J.Jcs.2014.01.010
141. Varney, J, Barrett, J, Scarlata, K, Catsos, P, Gibson, PR, and Muir, JG. Fodmaps: food composition, defining cutoff values and international application. J Gastroenterol Hepatol. (2017) 32:53–61. doi: 10.1111/Jgh.13698
142. Verspreet, J, Dornez, E, Van Den Ende, W, Delcour, JA, and Courtin, CM. Cereal grain Fructans: structure, variability and potential health effects. Trends Food Sci Technol. (2015) 43:32–42. doi: 10.1016/J.Tifs.2015.01.006
143. Midenfjord, I, Grinsvall, C, Koj, P, Carnerup, I, Törnblom, H, and Simrén, M. Central sensitization and severity of gastrointestinal symptoms in irritable bowel syndrome, chronic pain syndromes, and inflammatory bowel disease. Neurogastroenterol Motil. (2021) 33:E14156. doi: 10.1111/Nmo.14156
144. Moshfegh, AJ, Friday, JE, Goldman, JP, and Ahuja, JK. Presence of inulin and Oligofructose in the diets of Americans. J Nutr. (1999) 129:1407s–11s. doi: 10.1093/Jn/129.7.1407s
145. Whelan, K, Abrahmsohn, O, David, GJ, Staudacher, H, Irving, P, Lomer, MC, et al. Fructan content of commonly consumed wheat, Rye and gluten-free breads. Int J Food Sci Nutr. (2011) 62:498–503. doi: 10.3109/09637486.2011.553588
146. Biesiekierski, JR, Newnham, ED, Irving, PM, Barrett, JS, Haines, M, Doecke, JD, et al. Gluten causes gastrointestinal symptoms in subjects without celiac disease: a double-blind randomized placebo-controlled trial. Am J Gastroenterol. (2011) 106:508–14. doi: 10.1038/Ajg.2010.487
147. Muir, JG, Rose, R, Rosella, O, Liels, K, Barrett, JS, Shepherd, SJ, et al. Measurement of short-chain carbohydrates in common Australian vegetables and fruits by high-performance liquid chromatography (Hplc). J Agric Food Chem. (2009) 57:554–65. doi: 10.1021/Jf802700e
148. Muir, JG, Shepherd, SJ, Rosella, O, Rose, R, Barrett, JS, and Gibson, PR. Fructan and free fructose content of common Australian vegetables and fruit. J Agric Food Chem. (2007) 55:6619–27. doi: 10.1021/Jf070623x
149. Chumpitazi, BP, Hoffman, KL, Smith, DP, Mcmeans, AR, Musaad, S, Versalovic, J, et al. Fructan-sensitive children with irritable bowel syndrome Have distinct gut microbiome signatures. Aliment Pharmacol Ther. (2021) 53:499–509. doi: 10.1111/Apt.16204
150. Chumpitazi, BP, Mcmeans, AR, Vaughan, A, Ali, A, Orlando, S, Elsaadi, A, et al. Fructans exacerbate symptoms in a subset of children with irritable bowel syndrome. Clin Gastroenterol Hepatol. (2018) 16:E1. doi: 10.1016/J.Cgh.2017.09.043
151. Gibson, PR, and Shepherd, SJ. Personal view: food for thought--Western lifestyle and susceptibility to Crohn's disease. The Fodmap hypothesis. Aliment Pharmacol Ther. (2005) 21:1399–409. doi: 10.1111/J.1365-2036.2005.02506.X
152. Gibson, PR, and Shepherd, SJ. Evidence-based dietary management of functional gastrointestinal symptoms: the Fodmap approach. J Gastroenterol Hepatol. (2010) 25:252–8. doi: 10.1111/J.1440-1746.2009.06149.X
153. Hayes, PA, Fraher, MH, and Quigley, EM. Irritable bowel syndrome: the role of food in pathogenesis and management. Gastroenterol Hepatol (NY). (2014) 10:164–74.
154. Rao, SS, Yu, S, and Fedewa, A. Systematic Review: dietary fibre and Fodmap-restricted diet in the management of constipation and irritable bowel syndrome. Aliment Pharmacol Ther. (2015) 41:1256–70. doi: 10.1111/Apt.13167
155. Skodje, GI, Sarna, VK, Minelle, IH, Rolfsen, KL, Muir, JG, Gibson, PR, et al. Fructan, rather than gluten, induces symptoms in patients with self-reported non-celiac gluten sensitivity. Gastroenterology. (2018) 154:E2. doi: 10.1053/J.Gastro.2017.10.040
156. Staudacher, HM, Whelan, K, Irving, PM, and Lomer, MC. Comparison of symptom response following advice for a diet low in fermentable carbohydrates (Fodmaps) versus standard dietary advice in patients with irritable bowel syndrome. J Hum Nutr Diet. (2011) 24:487–95. doi: 10.1111/J.1365-277x.2011.01162.X
157. Whelan, K, Martin, LD, Staudacher, HM, and Lomer, MCE. The low Fodmap diet in the management of irritable bowel syndrome: an evidence-based Review of Fodmap restriction, reintroduction and personalisation in clinical practice. J Hum Nutr Diet. (2018) 31:239–55. doi: 10.1111/Jhn.12530
158. Brandt, M. Sourdough in a regulatory context In: M Gänzle and M Gobbetti, editors. Handbook of sourdough biotechnology. 2nd ed. Heidelberg: Springer International Publishing (2023)
159. Brandt, M, Loponen, L, and Capelle, S. Technology of sourdough fermentation and sourdough application In: M Gänzle and M Gobbetti, editors. Handbook of sourdough biotechnology. 2nd ed. Heidelberg: Springer International Publishing (2023)
160. De Vuyst, L, González-Alonso, V, Wardhana, YR, and Pradal, I. Taxonomy and species diversity of sourdough lactic acid Bacteria In: M Gobbetti and M Gänzle, editors. Handbook on sourdough biotechnology. Cham: Springer International Publishing (2023)
161. Dinardo, FR, Minervini, F, De Angelis, M, Gobbetti, M, and Gänzle, MG. Dynamics of Enterobacteriaceae and lactobacilli in model sourdoughs are driven by Ph and concentrations of sucrose and Ferulic acid. LWT. (2019) 114:108394. doi: 10.1016/J.Lwt.2019.108394
162. Sekwati-Monang, B, Valcheva, R, and Ganzle, MG. Microbial ecology of Sorghum sourdoughs: effect of substrate supply and phenolic compounds on composition of fermentation microbiota. Int J Food Microbiol. (2012) 159:240–6. doi: 10.1016/J.Ijfoodmicro.2012.09.013
163. Gänzle, MG, and Zheng, J. Lifestyles of sourdough lactobacilli - Do they matter for microbial ecology and bread quality? Int J Food Microbiol. (2019) 302:15–23. doi: 10.1016/J.Ijfoodmicro.2018.08.019
164. Landis, EA, Oliverio, AM, Mckenney, EA, Nichols, LM, Kfoury, N, Biango-Daniels, M, et al. The diversity and function of sourdough starter microbiomes. elife. (2021) 10:e61644. doi: 10.7554/Elife.61644
165. Urien, C, Legrand, J, Montalent, P, Casaregola, S, and Sicard, D. Fungal species diversity in French bread sourdoughs made of organic wheat flour. Front Microbiol. (2019) 10:201. doi: 10.3389/Fmicb.2019.00201
166. Comasio, A, Verce, M, Van Kerrebroeck, S, and De Vuyst, L. Diverse microbial composition of sourdoughs from different origins. Front Microbiol. (2020) 11:1212. doi: 10.3389/Fmicb.2020.01212
167. De Vuyst, L, Comasio, A, and Kerrebroeck, SV. Sourdough production: fermentation strategies, microbial ecology, and use of non-flour ingredients. Crit Rev Food Sci Nutr. (2023) 63:2447–79. doi: 10.1080/10408398.2021.1976100
168. De Vuyst, L, Harth, H, Van Kerrebroeck, S, and Leroy, F. Yeast diversity of sourdoughs and associated metabolic properties and functionalities. Int J Food Microbiol. (2016) 239:26–34. doi: 10.1016/J.Ijfoodmicro.2016.07.018
169. Lin, XB, and Ganzle, MG. Effect of lineage-specific metabolic traits of Lactobacillus Reuteri on sourdough microbial ecology. Appl Environ Microbiol. (2014) 80:5782–9. doi: 10.1128/Aem.01783-14
170. Meroth, CB, Walter, J, Hertel, C, Brandt, MJ, and Hammes, WP. Monitoring the bacterial population dynamics in sourdough fermentation processes by using Pcr-denaturing gradient gel electrophoresis. Appl Environ Microbiol. (2003) 69:475–82. doi: 10.1128/Aem.69.1.475-482.2003
171. Brandt, MJ. Sourdough products for convenient use in baking. Food Microbiol. (2007) 24:161–4. doi: 10.1016/J.Fm.2006.07.010
172. Albagli, G, Schwartz, IDM, Amaral, PFF, Ferreira, TF, and Finotelli, PV. How dried sourdough starter can enable and spread the use of sourdough bread. LWT. (2021) 149:111888. doi: 10.1016/J.Lwt.2021.111888
173. Jin, Y, Acharya, HG, Acharya, D, Jorgensen, R, Gao, H, Secord, J, et al. Advances in molecular mechanisms of wheat Allergenicity in animal models: a comprehensive Review. Molecules. (2019) 24:1142. doi: 10.3390/Molecules24061142
174. Fu, W, Xue, W, Liu, C, Tian, Y, Zhang, K, and Zhu, Z. Screening of lactic acid Bacteria and yeasts from sourdough as starter cultures for reduced Allergenicity wheat products. Foods. (2020) 9:751. doi: 10.3390/Foods9060751
175. Gänzle, MG, Loponen, J, and Gobbetti, M. Proteolysis in sourdough fermentations: mechanisms and potential for improved bread quality. Trends Food Sci Technol. (2008) 19:513–21. doi: 10.1016/J.Tifs.2008.04.002
176. Gobbetti, M, Giuseppe Rizzello, C, Di Cagno, R, and De Angelis, M. Sourdough lactobacilli and celiac disease. Food Microbiol. (2007) 24:187–96. doi: 10.1016/J.Fm.2006.07.014
177. Brijs, K, Bleukx, W, and Delcour, JA. Proteolytic activities in dormant Rye (Secale Cereale L.) grain. J Agric Food Chem. (1999) 47:3572–8. doi: 10.1021/Jf990070t
178. Mikola, L, and Saarinen, S. Occurrence of acid and neutral carboxypeptidases in germinating cereals. Physiol Plant. (1986) 67:557–61. doi: 10.1111/J.1399-3054.1986.Tb05055.X
179. Collar, C, Mascarós, AF, and Barber, CBD. Amino acid metabolism by yeasts and lactic acid Bacteria during bread dough fermentation. J Food Sci. (1992) 57:1423–7. doi: 10.1111/j.1365-2621.1992.tb06874.x
180. Gobbetti, M, Minervini, F, and Rizzello, CG. Angiotensin I-converting-enzyme-inhibitory and antimicrobial bioactive peptides. Int J Dairy Technol. (2004) 57:173–88. doi: 10.1111/J.1471-0307.2004.00139.X
181. Loponen, J, Mikola, M, Katina, K, Sontag-Strohm, T, and Salovaara, H. Degradation of Hmw Glutenins during wheat sourdough fermentations. Cereal Chem. (2004) 81:87–93. doi: 10.1094/Cchem.2004.81.1.87
182. Spicher, G, and Nierle, W. Proteolytic activity of sourdough Bacteria. Appl Microbiol Biotechnol. (1988) 28:487–92. doi: 10.1007/Bf00268220
183. Thiele, C, Gänzle, MG, and Vogel, RF. Contribution of sourdough lactobacilli, yeast, and cereal enzymes to the generation of amino acids in dough relevant for bread flavor. Cereal Chem J. (2002) 79:45–51. doi: 10.1094/Cchem.2002.79.1.45
184. Tuukkanen, K, Loponen, J, Mikola, M, Sontag-Strohm, T, and Salovaara, H. Degradation of Secalins during Rye sourdough fermentation. Cereal Chem. (2005) 82:677–82. doi: 10.1094/Cc-82-0677
185. Jansch, A, Korakli, M, Vogel, RF, and Ganzle, MG. Glutathione reductase from Lactobacillus Sanfranciscensis Dsm20451t: contribution to oxygen tolerance and thiol exchange reactions in wheat sourdoughs. Appl Environ Microbiol. (2007) 73:4469–76. doi: 10.1128/Aem.02322-06
186. Tang, KX, Zhao, CJ, and Ganzle, MG. Effect of glutathione on the taste and texture of type I sourdough bread. J Agric Food Chem. (2017) 65:4321–8. doi: 10.1021/Acs.Jafc.7b00897
187. Xu, D, Tang, K, Hu, Y, Xu, X, and Ganzle, MG. Effect of glutathione dehydrogenase of Lactobacillus Sanfranciscensis on gluten properties and bread volume in type I wheat sourdough bread. J Agric Food Chem. (2018) 66:9770–6. doi: 10.1021/Acs.Jafc.8b03298
188. Loponen, J, Konig, K, Wu, J, and Ganzle, MG. Influence of thiol metabolism of lactobacilli on egg white proteins in wheat sourdoughs. J Agric Food Chem. (2008) 56:3357–62. doi: 10.1021/Jf703600t
189. Tovar, LER, and Ganzle, MG. Degradation of wheat germ agglutinin during sourdough fermentation. Foods. (2021) 10:340. doi: 10.3390/Foods10020340
190. Cunningham, DF, and O'connor, B. Proline specific peptidases. Biochim Biophys Acta. (1997) 1343:160–86. doi: 10.1016/S0167-4838(97)00134-9
191. Di Cagno, R, De Angelis, M, Auricchio, S, Greco, L, Clarke, C, De Vincenzi, M, et al. Sourdough bread made from wheat and nontoxic flours and started with selected lactobacilli is tolerated in celiac sprue patients. Appl Environ Microbiol. (2004) 70:1088–96. doi: 10.1128/Aem.70.2.1088-1096.2004
192. Di Cagno, R, De Angelis, M, Lavermicocca, P, De Vincenzi, M, Giovannini, C, Faccia, M, et al. Proteolysis by sourdough lactic acid Bacteria: effects on wheat flour protein fractions and gliadin peptides involved in human cereal intolerance. Appl Environ Microbiol. (2002) 68:623–33. doi: 10.1128/Aem.68.2.623-633.2002
193. Guedon, E, Serror, P, Ehrlich, SD, Renault, P, and Delorme, C. Pleiotropic transcriptional repressor Cody senses the intracellular Pool of branched-chain amino acids in Lactococcus Lactis. Mol Microbiol. (2001) 40:1227–39. doi: 10.1046/J.1365-2958.2001.02470.X
194. Rizzello, CG, Curiel, JA, Nionelli, L, Vincentini, O, Di Cagno, R, Silano, M, et al. Use of fungal proteases and selected sourdough lactic acid Bacteria for making wheat bread with an intermediate content of gluten. Food Microbiol. (2014) 37:59–68. doi: 10.1016/J.Fm.2013.06.017
195. Rizzello, CG, De Angelis, M, Di Cagno, R, Camarca, A, Silano, M, Losito, I, et al. Highly efficient gluten degradation by lactobacilli and fungal proteases during food processing: new perspectives for celiac disease. Appl Environ Microbiol. (2007) 73:4499–507. doi: 10.1128/Aem.00260-07
196. Boakye, PG, Kougblenou, I, Murai, T, Okyere, AY, Anderson, J, Bajgain, P, et al. Impact of sourdough fermentation on Fodmaps and amylase-trypsin inhibitor levels in wheat dough. J Cereal Sci. (2022) 108:103574. doi: 10.1016/J.Jcs.2022.103574
197. Ziegler, JU, Steiner, D, Longin, CFH, Würschum, T, Schweiggert, RM, and Carle, R. Wheat and the irritable bowel syndrome – Fodmap levels of modern and ancient species and their retention during bread making. J Funct Foods. (2016) 25:257–66. doi: 10.1016/J.Jff.2016.05.019
198. Nyyssölä, A, Ellilä, S, Nordlund, E, and Poutanen, K. Reduction of Fodmap content by bioprocessing. Trends Food Sci Technol. (2020) 99:257–72. doi: 10.1016/J.Tifs.2020.03.004
199. Muller, DC, Nguyen, H, Li, Q, Schonlechner, R, Miescher Schwenninger, S, Wismer, W, et al. Enzymatic and microbial conversions to achieve sugar reduction in bread. Food Res Int. (2021) 143:110296. doi: 10.1016/J.Foodres.2021.110296
200. Bigey, F, Segond, D, Friedrich, A, Guezenec, S, Bourgais, A, Huyghe, L, et al. Evidence for two Main domestication trajectories in Saccharomyces Cerevisiae linked to distinct bread-making processes. Curr Biol. (2021) 31:E5. doi: 10.1016/J.Cub.2020.11.016
201. Zheng, J, Ruan, L, Sun, M, and Ganzle, M. A genomic view of lactobacilli and Pediococci demonstrates that phylogeny matches ecology and physiology. Appl Environ Microbiol. (2015) 81:7233–43. doi: 10.1128/Aem.02116-15
202. Shewry, PR, America, AHP, Lovegrove, A, Wood, AJ, Plummer, A, Evans, J, et al. Comparative compositions of metabolites and dietary fibre components in doughs and breads produced from bread wheat, emmer and spelt and using yeast and sourdough processes. Food Chem. (2022) 374:131710. doi: 10.1016/J.Foodchem.2021.131710
203. Menezes, LAA, Minervini, F, Filannino, P, Sardaro, MLS, Gatti, M, and Lindner, JD. Effects of sourdough on Fodmaps in bread and potential outcomes on irritable bowel syndrome patients and healthy subjects. Front Microbiol. (2018) 9:1972. doi: 10.3389/Fmicb.2018.01972
204. Brouns, F. Phytic acid and whole grains for health controversy. Nutrients. (2021) 14:25. doi: 10.3390/Nu14010025
205. Fretzdorff, B, and Brümmer, JM. Reduction of Phytic acid during Breadmaking of whole meal breads. Cereal Chem. (1992) 69:266–70.
206. Leenhardt, F, Levrat-Verny, MA, Chanliaud, E, and Remesy, C. Moderate decrease of Ph by sourdough fermentation is sufficient to reduce Phytate content of whole wheat flour through endogenous Phytase activity. J Agric Food Chem. (2005) 53:98–102. doi: 10.1021/Jf049193q
207. Lopez, HW, Krespine, V, Guy, C, Messager, A, Demigne, C, and Remesy, C. Prolonged fermentation of whole wheat sourdough reduces Phytate level and increases soluble magnesium. J Agric Food Chem. (2001) 49:2657–62. doi: 10.1021/Jf001255z
208. Tangkongchitr, U, Seib, PA, and Hoseney, RC. Phytic Acid. Iii. two barriers to the loss of phytate during breadmaking. Cereal Chem. (1982) 59:216–21.
209. Kwon, JG, Park, SH, Kwak, JE, Cho, JH, Kim, G, Lee, D, et al. Mouse feeding study and microbiome analysis of sourdough bread for evaluation of its health effects. Front Microbiol. (2022) 13:989421. doi: 10.3389/Fmicb.2022.989421
210. Lopez, HW, Duclos, V, Coudray, C, Krespine, V, Feillet-Coudray, C, Messager, A, et al. Making bread with sourdough improves mineral bioavailability from reconstituted whole wheat flour in rats. Nutrition. (2003) 19:524–30. doi: 10.1016/S0899-9007(02)01079-1
211. Allen, L, De Benoist, B, Dary, O, and Hurrell, R. Iron vitamin a and iodine. Guidelines on food fortification with micronutrients. Geneva: World Health Organization (2006).
212. Liberal, A, Pinela, J, Vivar-Quintana, AM, Ferreira, I, and Barros, L. Fighting Iron-deficiency Anemia: innovations in food Fortificants and biofortification strategies. Foods. (2020) 9:1817. doi: 10.3390/Foods9121871
213. De Punder, K, and Pruimboom, L. The dietary intake of wheat and other cereal grains and their role in inflammation. Nutrients. (2013) 5:771–87. doi: 10.3390/Nu5030771
214. Brouns, F, Van Rooy, G, Shewry, P, Rustgi, S, and Jonkers, D. Adverse reactions to wheat or wheat components. Compr Rev Food Sci Food Saf. (2019) 18:1437–52. doi: 10.1111/1541-4337.12475
215. Zhang, J, Chen, H, Fan, C, Gao, S, Zhang, Z, and Bo, L. Classification of the botanical and geographical origins of Chinese honey based on 1h Nmr profile with Chemometrics. Food Res Int. (2020) 137:109714. doi: 10.1016/J.Foodres.2020.109714
216. Mottram, DS, Wedzicha, BL, and Dodson, AT. Acrylamide is formed in the Maillard reaction. Nature. (2002) 419:448–9. doi: 10.1038/419448a
217. Fredriksson, H, Tallving, J, Rosén, J, and Åman, P. Fermentation reduces free asparagine in dough and acrylamide content in bread. Cereal Chem J. (2004) 81:650–3. doi: 10.1094/Cchem.2004.81.5.650
218. Sarion, C, Codina, GG, and Dabija, A. Acrylamide in bakery products: a Review on health risks, legal regulations and strategies to reduce its formation. Int J Environ Res Public Health. (2021) 18:4332. doi: 10.3390/Ijerph18084332
219. Claus, A, Carle, R, and Schieber, A. Acrylamide in cereal products: a Review. J Cereal Sci. (2008) 47:118–33. doi: 10.1016/J.Jcs.2007.06.016
220. Codină, GG, Sarion, C, and Dabija, A. Effects of dry sourdough on bread-making quality and acrylamide content. Agronomy. (2021) 11:1977. doi: 10.3390/Agronomy11101977
221. Nasiri Esfahani, B, Kadivar, M, Shahedi, M, and Soleimanian-Zad, S. Reduction of acrylamide in whole-wheat bread by combining lactobacilli and yeast fermentation. Food Addit Contam Part A Chem Anal Control Expo Risk Assess. (2017) 34:1904–14. doi: 10.1080/19440049.2017.1378444
222. Zhou, X, Duan, M, Gao, S, Wang, T, Wang, Y, Wang, X, et al. A strategy for reducing acrylamide content in wheat bread by combining acidification rate and prerequisite substance content of Lactobacillus and Saccharomyces Cerevisiae. Curr Res Food Sci. (2022) 5:1054–60. doi: 10.1016/J.Crfs.2022.06.005
223. Dastmalchi, F, Razavi, SH, Faraji, M, and Labbafi, M. Effect of Lactobacillus Casei-Casei and Lactobacillus Reuteri on acrylamide formation in flat bread and bread roll. J Food Sci Technol. (2016) 53:1531–9. doi: 10.1007/S13197-015-2118-3
224. Nachi, I, Fhoula, I, Smida, I, Ben Taher, I, Chouaibi, M, Jaunbergs, J, et al. Assessment of lactic acid Bacteria application for the reduction of acrylamide formation in bread. LWT. (2018) 92:435–41. doi: 10.1016/J.Lwt.2018.02.061
225. Muhialdin, BJ, Algboory, HL, Kadum, H, Mohammed, NK, Saari, N, Hassan, Z, et al. Antifungal activity determination for the peptides generated by Lactobacillus Plantarum Te10 against aspergillus Flavus in maize seeds. Food Control. (2020) 109:106898. doi: 10.1016/J.Foodcont.2019.106898
226. Nasrollahzadeh, A, Mokhtari, S, Khomeiri, M, and Saris, P. Mycotoxin detoxification of food by lactic acid Bacteria. Int J Food Contam. (2022) 9:1. doi: 10.1186/S40550-021-00087-W
227. Valle-Algarra, FM, Mateo, EM, Medina, A, Mateo, F, Gimeno-Adelantado, JV, and Jimenez, M. Changes in Ochratoxin a and type B Trichothecenes contained in wheat flour during dough fermentation and bread-baking. Food Addit Contam Part A Chem Anal Control Expo Risk Assess. (2009) 26:896–906. doi: 10.1080/02652030902788938
228. Bartkiene, E, Ozogul, F, and Rocha, JM. Bread sourdough lactic acid Bacteria-technological, antimicrobial, toxin-degrading, immune system-, and Faecal microbiota-modelling biological agents for the preparation of food, nutraceuticals feed. Foods. (2022) 11:452. doi: 10.3390/Foods11030452
229. Adebo, OA, Kayitesi, E, and Njobeh, PB. Reduction of mycotoxins during fermentation of whole grain Sorghum to whole grain ting (a Southern African food). Toxins (Basel). (2019) 11:180. doi: 10.3390/Toxins11030180
230. Adebo, OA, Njobeh, PB, and Mavumengwana, V. Degradation and detoxification of Afb1 by Staphylocococcus warneri, Sporosarcina Sp. Food Control. (2016) 68:92–6. doi: 10.1016/J.Foodcont.2016.03.021
231. Bartkiene, E, Zokaityte, E, Lele, V, Starkute, V, Zavistanaviciute, P, Klupsaite, D, et al. Combination of extrusion and fermentation with Lactobacillus Plantarum and L. uvarum strains for improving the safety characteristics of wheat bran. Toxins (Basel). (2021) 13:163. doi: 10.3390/Toxins13020163
232. Janic Hajnal, E, Pezo, L, Orcic, D, Saric, L, Plavsic, D, Kos, J, et al. Preliminary survey of Alternaria toxins reduction during fermentation of whole wheat dough. Microorganisms. (2020) 8:303. doi: 10.3390/Microorganisms8020303
233. Lim, E-S. Microbiological and chemical properties of sourdough fermented with probiotic lactic acid Bacteria. Korean J Microbiol. (2016) 52:84–97. doi: 10.7845/Kjm.2016.6012
234. Vidal, A, Marin, S, Morales, H, Ramos, AJ, and Sanchis, V. The fate of Deoxynivalenol and Ochratoxin a during the Breadmaking process, effects of sourdough use and bran content. Food Chem Toxicol. (2014) 68:53–60. doi: 10.1016/J.Fct.2014.03.006
235. Zadeike, D, Vaitkeviciene, R, Bartkevics, V, Bogdanova, E, Bartkiene, E, Lele, V, et al. The expedient application of microbial fermentation after whole-wheat milling and fractionation to mitigate mycotoxins in wheat-based products. LWT. (2021) 137:110440. doi: 10.1016/J.Lwt.2020.110440
236. Hu, Y, Stromeck, A, Loponen, J, Lopes-Lutz, D, Schieber, A, and Ganzle, MG. Lc-Ms/Ms quantification of bioactive angiotensin I-converting enzyme inhibitory peptides in Rye malt sourdoughs. J Agric Food Chem. (2011) 59:11983–9. doi: 10.1021/Jf2033329
237. Coda, R, Rizzello, CG, Pinto, D, and Gobbetti, M. Selected lactic acid Bacteria synthesize antioxidant peptides during sourdough fermentation of cereal flours. Appl Environ Microbiol. (2012) 78:1087–96. doi: 10.1128/Aem.06837-11
238. Luti, S, Galli, V, Venturi, M, Granchi, L, Paoli, P, and Pazzagli, L. Bioactive properties of breads made with sourdough of Hull-less barley or conventional and pigmented wheat flours. Appl Sci. (2021) 11:3291. doi: 10.3390/App11073291
239. Peñas, E, Diana, M, Frias, J, Quílez, J, and Martínez-Villaluenga, C. A multistrategic approach in the development of sourdough bread targeted towards blood pressure reduction. Plant Foods Hum Nutr. (2015) 70:97–103. doi: 10.1007/S11130-015-0469-6
240. Rizzello, CG, Cassone, A, Di Cagno, R, and Gobbetti, M. Synthesis of angiotensin I-converting enzyme (ace)-inhibitory peptides and gamma-aminobutyric acid (Gaba) during sourdough fermentation by selected lactic acid Bacteria. J Agric Food Chem. (2008) 56:6936–43. doi: 10.1021/Jf800512u
241. Rizzello, CG, Nionelli, L, Coda, R, and Gobbetti, M. Synthesis of the Cancer preventive peptide Lunasin by lactic acid Bacteria during sourdough fermentation. Nutr Cancer. (2012) 64:111–20. doi: 10.1080/01635581.2012.630159
242. Zhao, CJ, Hu, Y, Schieber, A, and Gänzle, M. Fate of ace-inhibitory peptides during the bread-making process: quantification of peptides in sourdough, bread crumb, steamed bread and soda crackers. J Cereal Sci. (2013) 57:514–9. doi: 10.1016/J.Jcs.2013.02.009
243. Yang, NJ, and Hinner, MJ. Getting across the cell membrane: an overview for Small molecules, peptides, and proteins. Methods Mol Biol. (2015) 1266:29–53. doi: 10.1007/978-1-4939-2272-7_3
244. Pennington, C, Dufresne, C, Fanciulli, G, and Wood, T. Detection of gluten Exorphin B 4 and B 5 in human blood by liquid chromatography-mass spectrometry/mass spectrometry. Open Spectr J. (2007) 1:9. doi: 10.2174/1874383800701010009
245. Castell, JV, Friedrich, G, Kuhn, CS, and Poppe, GE. Intestinal absorption of Undegraded proteins in men: presence of bromelain in plasma after Oral intake. Am J Physiol. (1997) 273:G139–46. doi: 10.1152/Ajpgi.1997.273.1.G139
246. Heyman, M. Symposium on ‘Dietary influences on mucosal Immunity’. How dietary antigens access the mucosal immune system. Proc Nutr Soc. (2001) 60:419–26. doi: 10.1079/PNS2001117
247. Heyman, M. Gut barrier dysfunction in food allergy. Eur J Gastroenterol Hepatol. (2005) 17:1279–85. doi: 10.1097/00042737-200512000-00003
248. Uhde, M, Ajamian, M, Caio, G, De Giorgio, R, Indart, A, Green, PH, et al. Intestinal cell damage and systemic immune activation in individuals reporting sensitivity to wheat in the absence of coeliac disease. Gut. (2016) 65:1930–7. doi: 10.1136/Gutjnl-2016-311964
249. Ozorio, L, Mellinger-Silva, C, Cabral, LMC, Jardin, J, Boudry, G, and Dupont, D. The influence of peptidases in intestinal brush border membranes on the absorption of oligopeptides from whey protein hydrolysate: an ex vivo study using an Ussing chamber. Foods. (2020) 9:E1415. doi: 10.3390/Foods9101415
250. Sanchez-Rivera, L, Ares, I, Miralles, B, Gomez-Ruiz, JA, Recio, I, Martinez-Larranaga, MR, et al. Bioavailability and kinetics of the antihypertensive casein-derived peptide Hlplp in rats. J Agric Food Chem. (2014) 62:11869–75. doi: 10.1021/Jf5035256
251. Xu, D, Zhang, Y, Tang, K, Hu, Y, Xu, X, and Ganzle, MG. Effect of mixed cultures of yeast and lactobacilli on the quality of wheat sourdough bread. Front Microbiol. (2019) 10:2113. doi: 10.3389/Fmicb.2019.02113
252. Cicero, AF, Colletti, A, Rosticci, M, Cagnati, M, Urso, R, Giovannini, M, et al. Effect of Lactotripeptides (isoleucine-proline-proline/valine-proline-proline) on blood pressure and arterial stiffness changes in subjects with suboptimal blood pressure control and metabolic syndrome: a double-blind, randomized, crossover clinical trial. Metab Syndr Relat Disord. (2016) 14:161–6. doi: 10.1089/Met.2015.0093
253. De Leeuw, PW, Van Der Zander, K, Kroon, AA, Rennenberg, RM, and Koning, MM. Dose-dependent lowering of blood pressure by dairy peptides in mildly hypertensive subjects. Blood Press. (2009) 18:44–50. doi: 10.1080/08037050902761209
254. Mizuno, S, Matsuura, K, Gotou, T, Nishimura, S, Kajimoto, O, Yabune, M, et al. Antihypertensive effect of casein hydrolysate in a placebo-controlled study in subjects with high-Normal blood pressure and mild hypertension. Br J Nutr. (2005) 94:84–91. doi: 10.1079/Bjn20051422
255. Van Der Zander, K, Jakel, M, Bianco, V, and Koning, MM. Fermented Lactotripeptides-containing Milk lowers daytime blood pressure in high Normal-to-mild hypertensive subjects. J Hum Hypertens. (2008) 22:804–6. doi: 10.1038/Jhh.2008.59
256. Chen, YC, Smith, HA, Hengist, A, Chrzanowski-Smith, OJ, Mikkelsen, UR, Carroll, HA, et al. Co-ingestion of whey protein hydrolysate with Milk minerals rich in calcium potently stimulates glucagon-like Peptide-1 secretion: an Rct in healthy adults. Eur J Nutr. (2020) 59:2449–62. doi: 10.1007/S00394-019-02092-4
257. Geerts, BF, Van Dongen, MG, Flameling, B, Moerland, MM, De Kam, ML, Cohen, AF, et al. Hydrolyzed casein decreases postprandial glucose concentrations in T2dm patients irrespective of leucine content. J Diet Suppl. (2011) 8:280–92. doi: 10.3109/19390211.2011.593617
258. Sartorius, T, Weidner, A, Dharsono, T, Boulier, A, Wilhelm, M, and Schon, C. Postprandial effects of a proprietary Milk protein hydrolysate containing bioactive peptides in Prediabetic subjects. Nutrients. (2019) 11:1700. doi: 10.3390/nu11071700
259. Althnaibat, RM, Bruce, HL, and Gӓnzle, MG. Identification of peptides from camel Milk that inhibit starch digestion. Int Dairy J. (2023) 141:105620. doi: 10.1016/J.Idairyj.2023.105620
260. Gunnerud, UJ, Ostman, EM, and Bjorck, IM. Effects of whey proteins on glycaemia and Insulinaemia to an Oral glucose load in healthy adults; a dose-response study. Eur J Clin Nutr. (2013) 67:749–53. doi: 10.1038/Ejcn.2013.88
261. Lan-Pidhainy, X, and Wolever, TM. The hypoglycemic effect of fat and protein is not attenuated by insulin resistance. Am J Clin Nutr. (2010) 91:98–105. doi: 10.3945/Ajcn.2009.28125
262. Brouns, F, and Shewry, P. Do gluten peptides stimulate weight gain in humans? Nutr Bull. (2022) 47:186–98. doi: 10.1111/nbu.12558
263. Guha, S, and Majumder, K. Comprehensive Review of gamma-Glutamyl peptides (gamma-Gps) and their effect on inflammation concerning cardiovascular health. J Agric Food Chem. (2022) 70:7851–70. doi: 10.1021/Acs.Jafc.2c01712
264. Blaak, EE, Antoine, JM, Benton, D, Bjorck, I, Bozzetto, L, Brouns, F, et al. Impact of postprandial glycaemia on health and prevention of disease. Obes Rev. (2012) 13:923–84. doi: 10.1111/J.1467-789x.2012.01011.X
265. Calder, PC, Ahluwalia, N, Brouns, F, Buetler, T, Clement, K, Cunningham, K, et al. Dietary factors and low-grade inflammation in relation to overweight and obesity. Br J Nutr. (2011) 106:S5–S78. doi: 10.1017/S0007114511005460
266. Brouns, F, Bjorck, I, Frayn, KN, Gibbs, AL, Lang, V, Slama, G, et al. Glycaemic index methodology. Nutr Res Rev. (2005) 18:145–71. doi: 10.1079/Nrr2005100
267. Brouns, F. Saccharide characteristics and their potential health effects in perspective. Front Nutr. (2020) 7:75. doi: 10.3389/Fnut.2020.00075
268. Hefni, ME, Thomsson, A, and Witthoft, CM. Bread making with sourdough and intact cereal and legume grains–effect on Glycaemic index and Glycaemic load. Int J Food Sci Nutr. (2021) 72:134–42. doi: 10.1080/09637486.2020.1769568
269. Woolnough, JW, Monro, JA, Brennan, CS, and Bird, AR. Simulating human carbohydrate Digestionin vitro: a Review of methods and the need for standardisation. Int J Food Sci Technol. (2008) 43:2245–56. doi: 10.1111/J.1365-2621.2008.01862.X
270. Vangsøe, CT, Ingerslev, AK, Theil, PK, Hedemann, MS, Lærke, HN, and Knudsen, KEB. In vitro starch digestion kinetics of diets varying in resistant starch and Arabinoxylan compared with in vivo portal appearance of glucose in pigs. Food Res Int. (2016) 88:199–206. doi: 10.1016/J.Foodres.2016.02.005
271. Van Kempen, TA, Regmi, PR, Matte, JJ, and Zijlstra, RT. In vitro starch digestion kinetics, corrected for estimated gastric emptying, predict portal glucose appearance in pigs. J Nutr. (2010) 140:1227–33. doi: 10.3945/Jn.109.120584
272. Weickert, MO, and Pfeiffer, AFH. Impact of dietary Fiber consumption on insulin resistance and the prevention of type 2 diabetes. J Nutr. (2018) 148:7–12. doi: 10.1093/Jn/Nxx0008
273. Alam, SA, Pentikainen, S, Narvainen, J, Katina, K, Poutanen, K, and Sozer, N. The effect of structure and texture on the breakdown pattern during mastication and impacts on in vitro starch digestibility of high fibre Rye Extrudates. Food Funct. (2019) 10:1958–73. doi: 10.1039/C8fo02188k
274. De Angelis, M, Damiano, N, Rizzello, CG, Cassone, A, Di Cagno, R, and Gobbetti, M. Sourdough fermentation as a tool for the manufacture of low-glycemic index white wheat bread enriched in dietary fibre. Eur Food Res Technol. (2009) 229:593–601. doi: 10.1007/S00217-009-1085-1
275. De Angelis, M, Rizzello, CG, Alfonsi, G, Arnault, P, Cappelle, S, Di Cagno, R, et al. Use of sourdough lactobacilli and oat fibre to decrease the Glycaemic index of white wheat bread. Br J Nutr. (2007) 98:1196–205. doi: 10.1017/S0007114507772689
276. Hartvigsen, ML, Gregersen, S, Laerke, HN, Holst, JJ, Bach Knudsen, KE, and Hermansen, K. Effects of concentrated Arabinoxylan and Beta-glucan compared with refined wheat and whole grain Rye on glucose and appetite in subjects with the metabolic syndrome: a randomized study. Eur J Clin Nutr. (2014) 68:84–90. doi: 10.1038/Ejcn.2013.236
277. Christensen, L, Vuholm, S, Roager, HM, Nielsen, DS, Krych, L, Kristensen, M, et al. Prevotella abundance predicts weight loss success in healthy, overweight adults consuming a whole-grain diet ad libitum: a post hoc analysis of a 6-Wk randomized controlled trial. J Nutr. (2019) 149:2174–81. doi: 10.1093/Jn/Nxz198
278. Interact, C. Dietary fibre and incidence of type 2 diabetes in eight European countries: the epic-Interact study and a Meta-analysis of prospective studies. Diabetologia. (2015) 58:1394–408. doi: 10.1007/S00125-015-3585-9
279. Jovanovski, E, Khayyat, R, Zurbau, A, Komishon, A, Mazhar, N, Sievenpiper, JL, et al. Should viscous Fiber supplements be considered in diabetes control? Results from a systematic Review and Meta-analysis of randomized controlled trials. Diabetes Care. (2019) 42:755–66. doi: 10.2337/Dc18-1126
280. Xu, C, Liu, J, Gao, J, Wu, X, Cui, C, Wei, H, et al. Combined soluble Fiber-mediated intestinal microbiota improve insulin sensitivity of obese mice. Nutrients. (2020) 12:351. doi: 10.3390/Nu12020351
281. Reynolds, AN, Akerman, AP, and Mann, J. Dietary fibre and whole grains in diabetes management: systematic Review and Meta-analyses. PLoS Med. (2020) 17:E1003053. doi: 10.1371/Journal.Pmed.1003053
282. Armet, AM, Deehan, EC, O’sullivan, AF, Mota, JF, Field, CJ, Prado, CM, et al. Rethinking healthy eating in light of the gut microbiome. Cell Host Microbe. (2022) 30:764–85. doi: 10.1016/J.Chom.2022.04.016
283. Canani, RB, Costanzo, MD, Leone, L, Pedata, M, Meli, R, and Calignano, A. Potential beneficial effects of butyrate in intestinal and Extraintestinal diseases. World J Gastroenterol. (2011) 17:1519–28. doi: 10.3748/Wjg.V17.I12.1519
284. Smith, C, Van Haute, MJ, and Rose, DJ. Processing has differential effects on microbiota-accessible carbohydrates in whole grains during in vitro fermentation. Appl Environ Microbiol. (2020) 86:e01705. doi: 10.1128/Aem.01705-20
285. Liljeberg, HG, Lonner, CH, and Bjorck, IM. Sourdough fermentation or addition of organic acids or corresponding salts to bread improves nutritional properties of starch in healthy humans. J Nutr. (1995) 125:1503–11. doi: 10.1093/Jn/125.6.1503
286. Kanazawa, S, Sanabria, M, and Monteiro, M. Influence of the fermentation methods on the resistant starch formation by X-Ray diffraction. SN Appl Sci. (2021) 3:191. doi: 10.1007/S42452-021-04240-0
287. Da Ros, A, Polo, A, Rizzello, CG, Acin-Albiac, M, Montemurro, M, Di Cagno, R, et al. Feeding with sustainably sourdough bread has the potential to promote the healthy microbiota metabolism at the Colon level. Microbiol Spectr. (2021) 9:E0049421. doi: 10.1128/Spectrum.00494-21
288. Buddrick, O, Jones, OAH, Hughes, JG, Kong, I, and Small, DM. The effect of fermentation and addition of vegetable oil on resistant starch formation in wholegrain breads. Food Chem. (2015) 180:181–5. doi: 10.1016/J.Foodchem.2015.02.044
289. Demirkesen-Bicak, H, Arici, M, Yaman, M, Karasu, S, and Sagdic, O. Effect of different fermentation condition on estimated glycemic index, in vitro starch digestibility, and textural and sensory properties of sourdough bread. Foods. (2021) 10:514. doi: 10.3390/Foods10030514
290. Scazzina, F, Del Rio, D, Pellegrini, N, and Brighenti, F. Sourdough bread: starch digestibility and postprandial glycemic response. J Cereal Sci. (2009) 49:419–21. doi: 10.1016/J.Jcs.2008.12.008
291. Shumoy, H, Van Bockstaele, F, Devecioglu, D, and Raes, K. Effect of sourdough addition and storage time on in vitro starch digestibility and estimated glycemic index of Tef bread. Food Chem. (2018) 264:34–40. doi: 10.1016/J.Foodchem.2018.05.019
292. Amaral, O, Guerreiro, CS, Gomes, A, and Cravo, M. Resistant starch production in wheat bread: effect of ingredients, baking conditions and storage. Eur Food Res Technol. (2016) 242:1747–53. doi: 10.1007/S00217-016-2674-4
293. Lappi, J, Aura, AM, Katina, K, Nordlund, E, Kolehmainen, M, Mykkanen, H, et al. Comparison of postprandial phenolic acid excretions and glucose responses after ingestion of breads with bioprocessed or native Rye bran. Food Funct. (2013) 4:972–81. doi: 10.1039/C3fo60078e
294. Maioli, M, Pes, GM, Sanna, M, Cherchi, S, Dettori, M, Manca, E, et al. Sourdough-leavened bread improves postprandial glucose and insulin plasma levels in subjects with impaired glucose tolerance. Acta Diabetol. (2008) 45:91–6. doi: 10.1007/S00592-008-0029-8
295. Juntunen, KS, Laaksonen, DE, Autio, K, Niskanen, LK, Holst, JJ, Savolainen, KE, et al. Structural differences between Rye and wheat breads but not Total Fiber content may explain the lower postprandial insulin response to Rye bread. Am J Clin Nutr. (2003) 78:957–64. doi: 10.1093/Ajcn/78.5.957
296. Liljeberg, H, Åkerberg, A, and Björck, I. Resistant starch formation in bread as influenced by choice of ingredients or baking conditions. Food Chem. (1996) 56:389–94. doi: 10.1016/0308-8146(95)00199-9
297. Liljeberg, H, Granfeldt, Y, and Bjorck, I. Metabolic responses to starch in bread containing intact kernels versus milled flour. Eur J Clin Nutr. (1992) 46:561–75.
298. Ostman, E, Granfeldt, Y, Persson, L, and Bjorck, I. Vinegar supplementation lowers glucose and insulin responses and increases satiety after a bread meal in healthy subjects. Eur J Clin Nutr. (2005) 59:983–8. doi: 10.1038/Sj.Ejcn.1602197
299. Zamaratskaia, G, Johansson, DP, Junqueira, MA, Deissler, L, Langton, M, Hellstrom, PM, et al. Impact of sourdough fermentation on appetite and postprandial metabolic responses - a randomised cross-over trial with whole grain Rye crispbread. Br J Nutr. (2017) 118:686–97. doi: 10.1017/S000711451700263x
300. Najjar, AM, Parsons, PM, Duncan, AM, Robinson, LE, Yada, RY, and Graham, TE. The acute impact of ingestion of breads of varying composition on blood glucose, insulin and incretins following first and second meals. Br J Nutr. (2009) 101:391–8. doi: 10.1017/S0007114508003085
301. Bondia-Pons, I, Nordlund, E, Mattila, I, Katina, K, Aura, AM, Kolehmainen, M, et al. Postprandial differences in the plasma metabolome of healthy Finnish subjects after intake of a sourdough fermented endosperm Rye bread versus white wheat bread. Nutr J. (2011) 10:116. doi: 10.1186/1475-2891-10-116
302. Polese, B, Nicolai, E, Genovese, D, Verlezza, V, La Sala, CN, Aiello, M, et al. Postprandial gastrointestinal function differs after acute administration of sourdough compared with Brewer's yeast bakery products in healthy adults. J Nutr. (2018) 148:202–8. doi: 10.1093/Jn/Nxx049
303. Rizzello, CG, Portincasa, P, Montemurro, M, Di Palo, DM, Lorusso, MP, De Angelis, M, et al. Sourdough fermented breads are more digestible than those started with Baker's yeast alone: an in vivo challenge dissecting distinct gastrointestinal responses. Nutrients. (2019) 11:2954. doi: 10.3390/Nu11122954
304. Bello-Perez, LA, Flores-Silva, PC, Agama-Acevedo, E, and Tovar, J. Starch digestibility: past, present, and future. J Sci Food Agric. (2020) 100:5009–16. doi: 10.1002/Jsfa.8955
305. Eelderink, C, Noort, MW, Sozer, N, Koehorst, M, Holst, JJ, Deacon, CF, et al. The structure of wheat bread influences the postprandial metabolic response in healthy men. Food Funct. (2015) 6:3236–48. doi: 10.1039/C5fo00354g
306. Magallanes-Cruz, PA, Flores-Silva, PC, and Bello-Perez, LA. Starch structure influences its digestibility: a Review. J Food Sci. (2017) 82:2016–23. doi: 10.1111/1750-3841.13809
307. Mishra, S, Hardacre, A, and Monro, J. Food structure and carbohydrate digestibility. Carbohydrates–Comprehensive Studies on Glycobiology and Glycotechnology (2012).
308. Wang, Y, Jian, C, Salonen, A, Dong, M, and Yang, Z. Designing healthier bread through the Lens of the gut microbiota. Trends Food Sci Technol. (2023) 134:13–28. doi: 10.1016/J.Tifs.2023.02.007
309. Fazzino, TL, Courville, AB, Guo, J, and Hall, KD. Ad libitum meal energy intake is positively influenced by energy density, eating rate and hyper-palatable food across four dietary patterns. Nat Food. (2023) 4:144–7. doi: 10.1038/S43016-022-00688-4
Keywords: sourdough fermentation, cereals, gastrointestinal disorders, immunogenic proteins, FODMAPs, health benefits, in vivo /in vitro/human studies
Citation: D’Amico V, Gänzle M, Call L, Zwirzitz B, Grausgruber H, D’Amico S and Brouns F (2023) Does sourdough bread provide clinically relevant health benefits? Front. Nutr. 10:1230043. doi: 10.3389/fnut.2023.1230043
Edited by:
Frank Thielecke, Swiss Distance University of Applied Sciences, SwitzerlandReviewed by:
Paucean Adriana, University of Agricultural Sciences and Veterinary Medicine of Cluj-Napoca, RomaniaEmiliano Salvucci, National Scientific and Technical Research Council (CONICET), Argentina
Copyright © 2023 D’Amico, Gänzle, Call, Zwirzitz, Grausgruber, D’Amico and Brouns. This is an open-access article distributed under the terms of the Creative Commons Attribution License (CC BY). The use, distribution or reproduction in other forums is permitted, provided the original author(s) and the copyright owner(s) are credited and that the original publication in this journal is cited, in accordance with accepted academic practice. No use, distribution or reproduction is permitted which does not comply with these terms.
*Correspondence: Stefano D’Amico, stefano.d-amico@ages.at