Corrigendum: A randomized controlled trial to examine the impact of a multi-strain probiotic on self-reported indicators of depression, anxiety, mood, and associated biomarkers
- 1Exercise and Performance Nutrition Laboratory, Department of Kinesiology, College of Science, Technology, and Health, Lindenwood University, Saint Charles, MO, United States
- 2Increnovo LLC, Milwaukee, WI, United States
- 3Probiotical srl, Novara, Italy
Objective: To examine the efficacy of supplementing with a multi-strain probiotic (MSP) on changes associated with mood, anxiety, and neurotransmitter levels.
Method: In a randomized, double-blind, placebo-controlled fashion, 70 healthy men and women (31.0 ± 9.5 years, 173.0 ± 10.4 cm, 73.9 ± 13.8 kg, 24.6 ± 3.5 kg/m2) supplemented with a single capsule of MSP (a total daily dose of 4 × 109 live cells comprised of a 1 × 109 live cells dose from each of the following strains: Limosilactobacillus fermentum LF16, Lacticaseibacillus rhamnosus LR06, Lactiplantibacillus plantarum LP01, and Bifidobacterium longum 04, Probiotical S.p.A., Novara, Italy) or a maltodextrin placebo (PLA). After 0, 2, 4, and 6 weeks of supplementation and 3 weeks after ceasing supplementation, study participants completed the Beck Depression Inventory (BDI-II), State-Trait Anxiety Inventory (STAI), and Leiden Index of Depression Sensitivity (LEIDS-R) questionnaires and had plasma concentrations of cortisol, dopamine, serotonin, and C-reactive protein determined.
Results: BDI, STAI, and total LEIDS-R scores were reduced from baseline (p < 0.05) with MSP supplementation after 4 and 6 weeks of supplementation and 3 weeks after supplementation while no changes (p > 0.05) were reported in PLA. When compared to PLA, MSP scores for state anxiety, trait anxiety, and LEIDS-R (hopeless, aggression, rumination, and total score) were significantly lower (p < 0.05) after supplementation. Plasma serotonin concentrations in MSP were increased from baseline after 6 weeks of supplementation and 3 weeks after ceasing supplementation. No changes (p > 0.05) in plasma dopamine, C-reactive protein, or cortisol concentrations were observed between groups.
Conclusion: MSP supplementation resulted in widespread improvements in several questionnaires evaluating mood, anxiety, and depression in young, healthy men and women. MSP supplementation increased serotonin increased after 6 weeks of MSP supplementation with no change in dopamine, C-reactive protein, or cortisol.
Clinical trial registration: https://classic.clinicaltrials.gov/ct2/show/NCT05343533, NCT05343533.
Introduction
Recent scientific investigations have confirmed the presence of an established gut-brain axis, an integrated network of bidirectional communication that involves contributions from immune, neural, and endocrine systems (1–4). In this axis and as explained in a recent review by Ahmed et al. (5), bacterial communities found in the intestinal microbiota signal the brain through an associated network by modulating activation of sympathetic and parasympathetic intestinal neurons (6, 7), education of the immune system (8) and production of several neurotransmitters (9–11) and gut hormones (12, 13). Moreover, bacterial communities and their metabolites (e.g., short-chain fatty acids, etc.) can go on to function as neuromodulators (14, 15) while impacting neuroinflammation (16–18), inflammatory modulators (19), energetic regulation for the host (20, 21), and are implicated in brain functions associated with neurodevelopment (22) and maintenance of the integrity of the blood–brain barrier (5). Additionally, germ-free mouse models have illustrated that the gut microbiota is crucial for normal brain development and behavior (23, 24) into adolescence (25). For these reasons, strategies to influence or alter the intestinal microbial environment may be employed to favorably impact mood and cognitive function. Probiotics, defined as live microorganisms that, when administered in adequate amounts, confer a health benefit on the host (26), are a notable intervention for influencing the microbial environment. Initial probiotic research in animals suggested favorable outcomes whereby probiotic administration seemed to favorably impact different aspects of cognitive function (27), anxiety (6) and depression (6). Likewise, human investigations have provided additional evidence to support the potential for probiotics to favorably influence the function and communication of the gut-brain axis. For instance, a functional magnetic resonance imaging (fMRI) study demonstrated that 4 weeks of probiotic use in 39 healthy women impacts regions of the brain involving emotional processing (28). Another four-week investigation using a multi-species probiotic demonstrated a positive effect on cognitive reactivity to sad moods in 40 healthy adults (29). Marotta et al. (30) expanded upon these findings when they reported that supplementation with a multi-strain probiotic mixture for 6 weeks in 38 non-clinical healthy volunteers favorably impacted mood by reducing depression and anger, while improving fatigue and sleep quality, and little change on personality attributes.
As evidence accumulates demonstrating the potential positive impact of probiotic use on mood, depression, and anxiety, investigations have attempted to link possible mechanisms to the functional outcomes. While human research is limited, a growing interest is evident to explore outcomes associated with stress modulation, neurotransmission, and inflammation and how these changes regulate and influence the gut-brain axis (5). In this respect, a recent review article by Nobis and colleagues (31) highlighted several biomarkers connected with neurotransmission, inflammation, and oxidative stress that are modulated in clinical depressive conditions such as major depressive disorder. While more work in needed to firmly establish the presence of such links in non-clinical populations, the need to further explore approaches that influence gut-brain axis activity and regulation are of distinct interest. In this regard, Edebol Carlman and colleagues (32) reported that 4 weeks of supplementing with a multi-strain probiotic was linked to increased functional connectivity between regions of the brain, with a seemingly greater response on the arithmetic stress test. However, this increased connectivity did not impact cortisol concentrations or measures of cognitive performance. Alternatively, Kazemi et al. (33) randomized 81 subjects with major depressive disorder to probiotic supplementation (10 × 109 CFU L. helveticus R0052 and B. longum R01750) or placebo for 8 weeks and reported significant improvements in Beck Depression Inventory (BDI-II) scores and reductions in cortisol.
Serotonin, a neurotransmitter with hormone actions, is produced in the body and is closely associated with mood, stress, and anxiety (34). Approximately 95% of the body’s serotonin is produced in the gut (35). Connections between microbiome metabolites such as short-chain fatty acids and serotonin levels have been established, and as a result, probiotic administration has been used to modulate serotonin levels. For example, Riezzo and colleagues (36) randomized 56 patients with functional constipation to ingest Limosilactobacillus reuteri DSM 17938 or a placebo for 105 days to determine changes in serotonin and brain-derived neurotrophic factor. Serotonin levels decreased in response to supplementation while no changes were observed in the controls. Additional investigations examining Lactiplantibacillus plantarum DR7 have connected administration of this probiotic with improvements in serotonin pathway involvement (37). Other potential areas of efficacy for probiotics lies with their ability to support gut barrier function and integrity resulting in reductions in inflammation (38). This consideration is important as systemic inflammation is linked to the development and progression of many chronic health conditions. Systemic inflammation results from chronic activation of the immune system resulting in increased circulating levels of C-reactive protein, TNF-alpha, IL-6, and others (39–41). Ingestion of prebiotics, dietary fibers, and various probiotic strains (e.g., Bifido and lactobacillus) have been suggested to exert a favorable impact on short-chain fatty acid production and other inflammatory modulators through various mechanisms (19, 42, 43). In this respect, probiotics have previously been shown to prevent translocation of bacteria in the gut secondary to dysbiosis, which can help prevent immune cell activation and subsequent activation of inflammatory pathways (38). Romijn et al. (44) supplemented participants with L. helveticus R0052 (CNCM I-1722) and B. longum R0175 (CNCM I-3470) (≥3 × 109 CFU/day) for 8 weeks and found that neither depression ratings or serum levels of key inflammatory cytokines (TNF-alpha, IL-6, IL-1B) were impacted. Alternatively, Akkasheh and colleagues (45) reported decreases in C-reactive protein and Beck Depression scores after supplementing for 8 weeks with L. acidophilus, L. casei and B. bifidum (6 × 109 CFU/day).
While initial research in clinically stressed or depressed populations suggest probiotics may help to improve mood, depression, and anxiety, more research is needed to fully examine the potential of probiotic administration to impact mood, depression, personality, and anxiety including their ability to impact key hormones which may regulate mood, inflammation, and stress in healthy populations. As an individual’s mood, anxiety, personality, and stress levels can all intersect to influence how the brain and gut interact with each other, our study’s purpose was to examine the impact of supplementing with a multi-strain probiotic on changes in mood, depression, personality, and anxiety as well as circulating concentrations of cortisol, C-reactive protein, serotonin, and dopamine.
Methods
Overview of research design
This study followed a randomized, double-blind, placebo-controlled, parallel group design that was intended to evaluate the ability of a multi-strain probiotic to influence self-reported levels of mood, depression, anxiety, personality traits, and associated biomarkers (e.g., serotonin, cortisol, dopamine, and C-reactive protein). Healthy men and women between the ages of 18–50 years were assigned to one of two supplementation groups. The probiotic formulation (MSP) administered during the study was a blend of the four strains: Lactiplantibacillus plantarum LP01 (LMG P-21021), Limosilactobacillus fermentum LF16 (DSM 26956), Lacticaseibacillus rhamnosus LR06 (DSM 21981), and Bifidobacterium longum 04 (DSM 23233) blended with maltodextrin (2.5 g) all belonging to Probiotical S.p.A. collection. The clinical formula had a cell potency measured by Plate Count of >4 × 109 Colony Forming Units (CFU)/dose. (Biolab Research Method 014-06). Cell potency of the samples was also measured by flow cytometer (ISO 19344:2015 IDF 232:2015) resulting in values of >4 × 109 Active Fluorescent Units (AFU)/dose. The probiotic formulation was referred to 4 × 109 live cells/dose. Placebo (PLA) was composed of pure maltodextrins (2.5 g). Prior to study engagement, all participants signed an IRB-approved informed consent document (Lindenwood University: IRB-19-212, approval date: 7/17/19, conformed to the standards set by the latest revision of the Declaration of Helsinki), and completed a health history questionnaire, and a series of additional questions to determine study eligibility.
Based upon previous data of Marotta et al. (30) a priori sample size evaluation indicated that a sample size of 28–33 participants would be needed. This estimation assumes an effect size of 0.5–0.55, with an alpha (α) level of 0.05 and estimated power (1 – β) of 0.80. Participants were scheduled for four identical study visits between 06:00 and 10:00 h while attempts were made to standardize visit timing across the investigation. For all visits, subjects were instructed to undergo an 8–10 h fast (except water) and abstain from strenuous physical activity 48 h prior to each visit. All participants supplemented for 6 weeks followed by a 3-week washout period post-supplementation. Participants donated a venous blood sample at 0 and 6 weeks of supplementation, and after a 3-week washout and were evaluated for changes in mood, personality, depression, and anxiety at: 0, 2, 4, and 6 weeks of supplement administration, and again post 3-week washout. Dietary records (2 days) were collected after 0, 2, 4, and 6 weeks of supplementation in addition to 3 weeks after ceasing supplementation. This study protocol and design was registered on Clinicaltrials.gov on April 25, 2022, as NCT05343533.1 Primary outcomes for this study were determined to be the mood-related questionnaires [e.g., Beck Depression Inventory (BDI-II), State/Trait Anxiety (STAI), Leiden Index of Depression Sensitivity-Revisited (LEIDS-R)] and biomarkers (e.g., cortisol, serotonin, dopamine, and C-reactive protein) while secondary outcomes were determined to the personality-related questionnaires [e.g., Cope Orientation to the Problems Experienced (COPE), Behavioral Inhibition System and Behavioral Activation System Scale (BIS/BAS), and the Life Orientation Test-Revised (LOT-R)] (Table 1).
Study participants
A total of 70 healthy men and women (31.0 ± 9.5 years, 173.0 ± 10.4 cm, 73.9 ± 13.8 kg, 26.7 ± 7.1% fat, 24.6 ± 3.5 kg/m2) successfully completed the study protocol. A Consolidation Standards of Reporting Trials (CONSORT) diagram is provided in Figure 1. Participants were included in the study if they were between the ages of 18–50 years, had a body mass index (BMI) between 18.5–32 kg/m2 (if BMI was 30–32 kg/m2, participant was required to have body fat percentage < 25% for men and < 35% for women for inclusion), were weight stable for the past 3 months (<5% variation in body mass), and deemed healthy through completion of a health history questionnaire. Participants were excluded if they were diagnosed or were being treated for any cardiac, respiratory, endocrine, psychiatric, musculoskeletal, renal, hepatic, neuromuscular or metabolic disease or disorder that precluded safe participation or would contraindicate quality control over the collected data, were diagnosed with or being treated for celiac disease, lactose intolerance, digestive insufficiencies, or other gastrointestinal complications such as irritable bowel syndrome, ulcerative colitis, etc., reported being a current smoker or had quit within the past 6 months, reported having used any illicit or recreational drugs including anabolic steroids within the past 30 days, reported the intake of any prescription or over-the-counter medications (i.e., antibiotics) that may impact study outcomes, reported the current use of any dietary supplements known to impact digestion or sleep quality for the past 30 days, reported taking a probiotic within the past 30 days, had been actively trying to lose weight, or were currently following a ketogenic or low carbohydrate diet within the past 30 days. Further, antibiotic use at any point in the study protocol led to removal from the study. Women who were pregnant, lactating, or indicated during screening they were actively trying to become pregnant were excluded from the study.
Procedures
Baseline demographics and hemodynamics
Participants rested quietly for approximately 10 min before measuring resting heart rate and blood pressure (Omron BP785, Omron Corporation, Kyoto, Japan). Body mass was determined (Tanita BWB-627A, Tokyo, Japan) and recorded to the nearest ±0.1 kg. Height was measured using a standard wall-mounted stadiometer (Tanita, HR-200, Tokyo, Japan) and recorded to the nearest ±0.5 centimeter (cm).
Diet and physical activity controls
After the baseline visit and before each subsequent visit, study participants completed a hand-written two-day food record and were provided a copy to facilitate diet replication for the subsequent study period. In addition to the two-day food log, participants completed the automated self-administered 24-h dietary assessment tool (ASA24).2 Average energy, carbohydrate, fat, and protein intake were recorded and are presented in the current paper. In addition, Healthy Eating Index values were computed from the completed food records. The Healthy Eating Index is a measure of diet quality that can be used to assess compliance with the Dietary Guidelines for Americans. From the collected food intake information, 13 different food categories (seafood & plant proteins, fatty acids, refined grains, sodium added sugars, saturated fats, total fruits, whole fruits, total vegetables, greens & beans, whole grains, dairy, and total protein foods) are populated and the amount of food and fluid consumed that aligns with that food category is evaluated against the recommended intake.3 Each category is then assigned a percentage and from there a Healthy Eating Index composite score is determined. Radar plots have been generated to evaluate the difference in HEI values between groups and to evaluate the extent to which composite HEI values changed across time. Physical activity was not controlled throughout this study protocol. Eligible participants were instructed to maintain physical activity status throughout the study protocol, and to inform research staff if their physical activity habits changed throughout the study protocol.
Body composition (DEXA)
Body composition was obtained using dual-energy X-ray absorptiometry (DEXA). Calibration procedures were performed before each testing session and all scans were completed using a Hologic QDR Discovery A (HOLOGIC, Bedford, MA, USA) and analyzed using the accompanying software (Hologic APEX Software, Version 4.5.3; HOLOGIC) to obtain body composition parameters. The test–retest reliability (ICC and CV) of these procedures in 40 healthy college-aged men and women, was determined to be 0.99 and 1.26% for DEXA fat and 0.99 and 0.75% for DEXA fat-free mass (data not shown). All results were calculated using the NHANES correction factor.
Venous blood collection and processing
Blood samples were collected from the participants at weeks 0, and 6 weeks of supplementation, and after a three-week washout period. Each sample was collected using standard phlebotomy techniques into two serum separator (SST) and two ethylenediaminetetraacetic acid (EDTA) Vacutainer™ tubes. Following collection, tubes were gently inverted ten times. SST tubes were allowed to clot at room temperature for 30 min before being centrifuged, while EDTA tubes were immediately centrifuged at 4°C for 20 min at 2,000 revolutions per minute (rpm) (MegaFuge XFR, ThermoFisher Scientific, Waltham, MA, USA). After centrifugation, plasma and serum were aliquoted (600 μL) into separate micro-centrifuge tubes to minimize freeze–thaw cycles and labeled. Samples were then stored at -80°C for later analysis of serotonin, cortisol, dopamine, and C-reactive protein.
Mood-related questionnaires
Leiden Index of Depression Sensitivity – Revised
Leiden Index of Depression Sensitivity – Revised is a self-reported questionnaire that tests cognitive reactivity to sad mood (46). LEIDS-R consists of 34 statements describing different situations, and participants rated the extent to which each state applies to themselves.
State-Trait Anxiety Inventory
State-Trait Anxiety Inventory (STAI) is a self-reported questionnaire that measures the presence and severity of existing symptoms of anxiety and anxiety predisposition (47). STAI consists of two subscales, one for state anxiety (STAI – form Y1) and another for trait anxiety (STAI – form Y2). Each subscale consists of 20 questions. STAI – Y1 measures the current anxiety state by asking participants how they currently feel. STAI – Y2 measures anxiety predisposition by asking participants to rate how they generally feel in their life.
Beck Depression Inventory
Beck Depression Inventory is a self-reported questionnaire that measures the occurrence and severity of existing depressive symptoms (48). Participants chose statements that best describe their feelings over the past 2 weeks.
Personality-related questionnaires
Cope Orientation to the Problems Experienced
Cope Orientation to the Problems Experienced (COPE) is a self-reported questionnaire that evaluates coping strategies including cognitive and behavioral approaches people use to manage stressful situations (49). COPE includes 60 statements divided into five components: social support, avoidance strategies, positive attitude, problem-solving, and reliance on religion.
Behavioral Inhibition System and Behavioral Activation System Scale
Behavioral Inhibition System and Behavioral Activation System Scale is a self-reported questionnaire that assesses an individual’s sensitivity to behavioral inhibition and behavioral activation (50). The BIS/BAS consists of 24 statements related to punishment, reward anticipation, and control.
Life-Orientation Test-Revised
Life Orientation Test-Revised is a self-reported questionnaire that measures the dispositional optimism and pessimism (51) and consists of ten statements (n = 3 are worded positively, n = 3 are worded negatively, and n = 4 are control). Participants rated their agreement with statements regarding optimism and pessimism.
Biochemical analysis
Plasma samples were analyzed in duplicate using 96-well ELISA kits for serotonin, cortisol, dopamine, and C-reactive protein concentrations following the manufacturer’s instructions (DRG International, Springfield, NJ, USA). All samples exhibited absorbance values within the standard curve. Plate to plate controls were employed between all analyzed plates and exhibited coefficient of variations that ranged from 5 to 10% between the plates for each biomarker.
Supplementation protocol
Using a randomized, double-blind, placebo-controlled, parallel group fashion, participants were assigned to ingest a single capsule of either a maltodextrin PLA or a mixture of four probiotic strains (MSP). Each probiotic dose was delivered in capsules containing a 1 × 109 live cells dose of each of the following strains (total daily dose of 4 × 109 live cells): Limosilactobacillus fermentum LF16 (DSM 26956), Lacticaseibacillus rhamnosus LR06 (DSM21981), Lactiplantibacillus plantarum LP01 (LMG P-21021), and Bifidobacterium longum 04 (DSM 23233) (Probiotical S.p.A., Novara, Italy). Capsules for the placebo and probiotic strain were identical in color, shape, size, and transparency and were packaged into identical, bottled, containers that contained the same number of capsules. Participants were instructed to consume each dose at approximately the same time each day with 240–360 mL of water and within 2 h of consuming a meal. Product stability was monitored across the whole study up to 24 months at three different temperature conditions (refrigerated at 5°C, Zone II at 25°C and 65% relative humidity and Zone IVb at 30°C and 75% relative humidity) confirming the threshold values of 4 × 109 CFU at the end of the 24 months program (See Supplementary Data).
Adverse event reporting
Adverse events were collected via spontaneous reporting by the participants, clinical evaluation, interaction of a research team member with a participant, or through review of a participant’s research file throughout the entire duration of the protocol. The frequency of each adverse event was recorded along with corresponding severity ratings of ‘mild’, ‘moderate’, or ‘severe’.
Statistical analysis
Before any statistical tests were performed, data was screened for data entry and organization errors, and then analyzed for normality, skewness, and kurtosis. Any non-normally distributed value was normalized, if possible, using log-transformations. Parametric data is reported as means ± standard deviations while non-parametric data is reported as median ± interquartile range. For all statistical tests, data was considered statistically significant when the probability of type I error is 0.05 or less. A trend or a tendency for change was determined when the probability of type I error was p = 0.051 – ≤0.10. Parametric data was analyzed using mixed factorial ANOVA (group x time) with repeated measures on time. When significant group x time interactions were found, delta values (from baseline) were calculated and assessed using independent t-tests. Main effects of time were analyzed using single-factor ANOVA with repeated measures on time, and pairwise comparisons were evaluated using Bonferroni corrections. Non-parametric data was first assessed using a Friedman test for K-related samples, and if significant (p < 0.05), follow-up assessments were completed using a Wilcoxon signed-rank test between each baseline score and each subsequent follow-up timepoint. Bonferroni corrections were applied to evaluate pairwise comparisons (0.05 / # of comparisons being made). For all outcomes, a priori statistical approach was used to conduct a mixed gender analysis, as it was the primary aim identified for this investigation. When an outcome exhibited a significant group x time interaction, a three-way interaction was explored, including gender, to identify if any condition-specific outcomes were due to gender. Further, in a post-hoc fashion, changes in serotonin in participants assigned to MSP were dichotomized into ‘responders’ and ‘non-responders’, whereby responders were defined as those participants who exhibited an increase in circulating serotonin where non-responders exhibited no change or a decrease in circulating serotonin. Participants were then assigned a dummy code and the observed change in those outcomes where condition-specific differences were realized (Beck Depression, state anxiety, trait anxiety, LEDIS total, and LOT-R total score) and compared for differences. Pearson correlations were completed to evaluate the presence of any significant relationships. All analyses were completed using Microsoft Excel and the Statistical Package for the Social Sciences (v27; SPSS Inc.).
Results
Test product viability and stability
MSP was analyzed via plate count method as colony forming units (CFU) (Internal Method 014–06) and flow cytometry (ISO 19344, 2015: IDF 232: 2015) as Active Fluorescent Units (AFU) upon batch release (Biolab srl, Novara, Italy). To exclude product sample heterogeneity three random samples, withdrawn in triplicate during product manufacturing, were analyzed for total fluorescent units (TFU), and Relative Standard Deviation was <10%. Water activity of the product (aw) was monitored during the study to exclude any possible detrimental effects on probiotic cells or spoilage of the product due to the increase of water activity that was kept below 0.100. Product stability was monitored for 24 months at 5, 25°C and 30°C to be representative of a refrigerated condition, Zone II condition and Zone IVb conditions, respectively, according to ICH pharma guidelines.4 Figures of this data are provided as Supplementary Figures 1, 2.
Supplementation compliance
Participants reported excellent compliance to each supplementation period. Compliance in the MSP condition ranged from 76.2 and 100.0% compliance (mean ± SD: 93.7 ± 7.3%) while compliance in the PLA group ranged from 71.4 and 100.0% compliance (mean ± SD: 94.6 ± 7.1%).
Adverse event reporting
Adverse events were self-reported throughout the clinical trial. In totality, 12 mild or moderate adverse events were reported by participants assigned to the MSP condition (constipation, n = 1; tired in morning, n = 1, flatulence, n = 4, bloating, n = 3; lower back pain, n = 2; lower abdominal pain, n = 1) and 47 mild or moderate adverse events were reported by participants assigned to the PLA condition (constipation, n = 3; tired in morning, n = 1, burping, n = 3; flatulence, n = 6, bloating, n = 4; lower back pain, n = 2; lower abdominal pain, n = 1; acid reflux, n = 4; irritated esophagus, n = 2; skin irritation, n = 13, dry eyes, n = 4, fatigue, n = 2, and increased need to use bathroom, n = 1). No serious adverse events were reported.
Dietary replication and hemodynamics
Of the 70 participants who completed the current study, 60 of them provided suitable dietary data. Four additional participants provided suitable baseline dietary data, but did not provide a suitable follow-up measure. For these four cases, missing data was replaced by carrying the baseline value forward. No differences were identified between groups for energy (MSP: 1891 ± 569 kcals/day vs. PLA: 2050 ± 618 kcals/day, p = 0.27), protein (MSP: 87.2 ± 31.8 grams/day vs. PLA: 97.2 ± 37.6 grams/day, p = 0.23), fat (MSP: 77.1 ± 26.2 grams/day vs. PLA: 88.5 ± 29.6 grams/day, p = 0.09), and carbohydrates (MSP: 212.3 ± 66.2 vs. PLA: 213.0 ± 76.7 grams/day, p = 0.97) throughout the study. Figure 2 is a radar plot that illustrates various Healthy Eating Index (HEI) components as well as the composite HEI score.
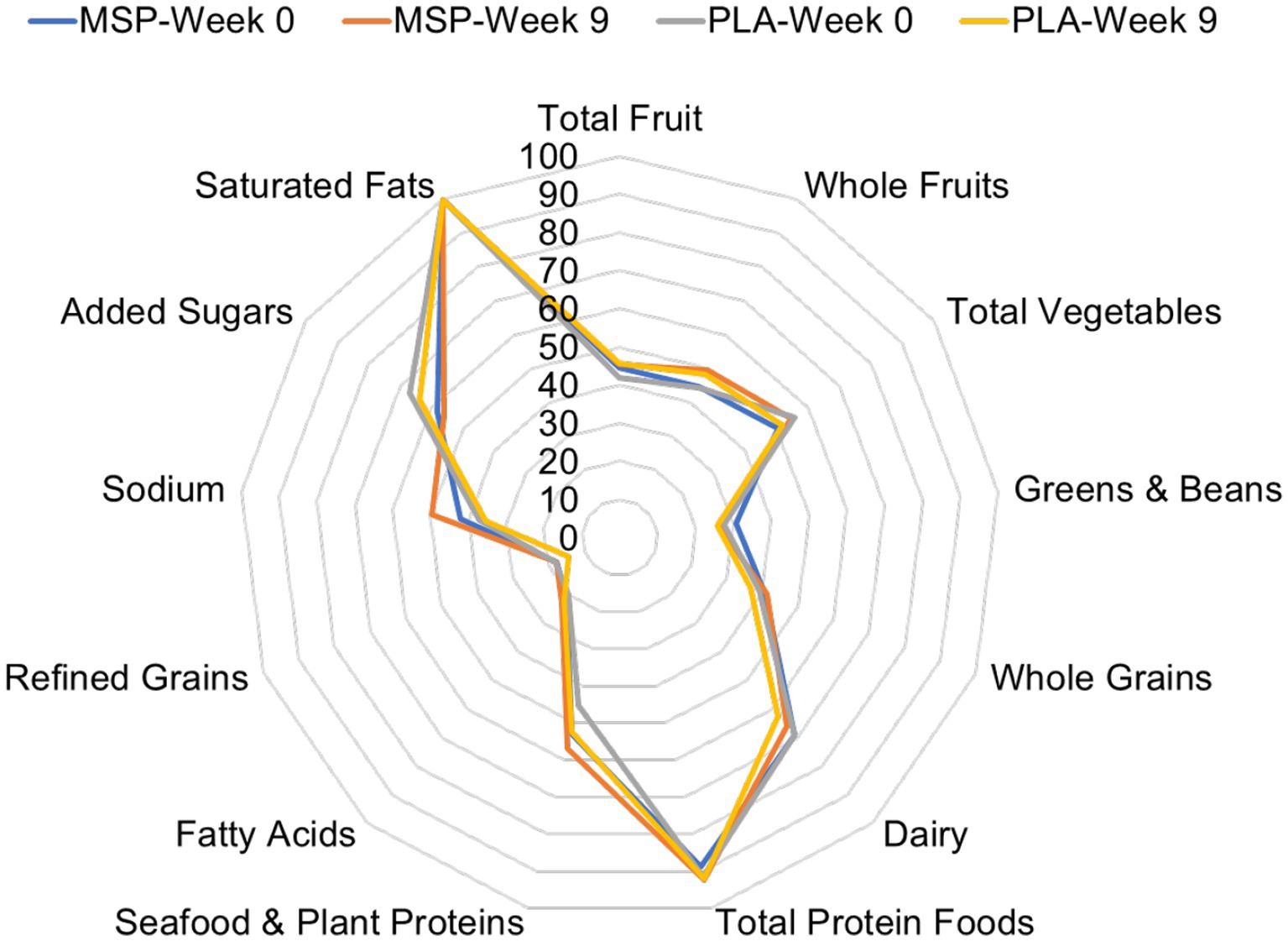
Figure 2. Radar plot of Healthy Eating Index values of MSP and PLA after 0 and 9 weeks of supplementation. MSP, Multi-strain probiotic; PLA, Placebo.
Baseline characteristics
Table 2 provides age, height, body mass, percent body fat, and body mass index values at baseline for all participants (n = 70), men (n = 35), and women (n = 35). No differences between groups were present prior to beginning supplementation.
Questionnaires assessing mood-related constructs
A significant time effect on Beck Depression Inventory (BDI-II) scores was observed in MSP (p < 0.001) as well as PLA (p = 0.007) using the Friedman test (Figure 3; Table 3). Follow-up analysis using Wilcoxon signed-rank tests in MSP indicated that reported scores for week 0 (W0) were different than scores for week 4 (W4, p = 0.001), week 6 (W6, p < 0.001), and 3 weeks after supplementation stopped (W9, p < 0.001). Follow-up analysis using Wilcoxon signed-rank tests in PLA indicated that reported scores for week 0 (W0) were different than scores for W9 (p < 0.001). No between-group differences (Mann–Whitney U) were observed at any of the five time points (p > 0.05). A significant three-way interaction (gender × group × condition) was observed (p = 0.035). Each gender was then analyzed separately. No difference was observed for BDI-II scores in females (Mean difference: 1.23 ± 1.69; 95% CI: −2.21, 4.68, p = 0.742) whereas a significant difference (−3.37 ± 1.31, 95% CI: −6.05, −0.70, p = 0.015) was identified in males. A significant time effect on state anxiety scores as measured in the State-Trait Anxiety Inventory (STAI) was observed in MSP (p < 0.001) and PLA (p = 0.04) using the Friedman test (Table 3). Follow-up analysis using Wilcoxon signed-rank tests with Bonferroni corrections applied for multiple comparison in MSP indicated that reported scores for week 0 (W0) were different than scores for W4 (p = 0.01), W6 (p < 0.001), and W9 (p = 0.001) and tended to be different for W2 (p = 0.02). Additionally, MSP scores were less than PLA (p = 0.04) after 6 weeks of supplementation. A significant time effect on trait anxiety scores was observed in MSP, (p < 0.001) while no difference was observed for PLA (p = 0.80) using the Friedman test (Table 3). Follow-up analysis using Wilcoxon signed-rank tests in MSP indicated that reported scores for week 0 (W0) were different than scores for W6 (p = 0.003) and tended to be different than W9 (p = 0.03). Results from the Mann–Whitney U test indicates that MSP scores were different than PLA at W0 (p = 0.02), W4 (p = 0.004), W6 (p = 0.005), and W9 (p = 0.003), while W2 scores tended (p = 0.06) to be different. No significant gender x group x condition was observed for state anxiety (p = 0.212) and trait anxiety (p = 0.117). Six subscales (hopeless/suicidality, acceptance/coping, aggression, control/perfectionism, risk aversion, and rumination) and a total score were assessed using the Leiden Index of Depress Sensitivity-Revised (LEIDS-R) (Figure 4; Table 3). No significant time effects were observed for the LEIDS-R hopeless/suicidality subscale for MSP (p = 0.10) and PLA (p = 0.10). No within-group differences were observed for either group using Wilcoxon signed-rank tests. Mann–Whitney U results indicated that MSP had significantly lower values than PLA at W0 (p = 0.02), W4 (p = 0.004), W6 (p = 0.006), and W9 (p = 0.003). To account for differences at baseline, each subsequent value was subtracted from its respective baseline value and using this approach no significant differences were found between conditions for the LEIDS-R hopeless subscale at W2 (= 0.811), W4 (p = 0.263), W6 (p = 0.306), and W9 (p = 0.725). No significant time effects were observed for the LEIDS-R acceptance/coping subscale for MSP (p = 0.07) and PLA (p = 0.62). To account for differences at baseline, each subsequent value was subtracted from its respective baseline value and using this approach no significant differences were found between conditions for the LEIDS-R acceptance subscale at W2 (= 0.952), W4 (p = 0.300), W6 (p = 0.194), and W9 (p = 0.168). No within-group differences were observed for either group using Wilcoxon signed-rank tests. No significant between group differences were observed as assessed by Mann–Whitney U tests. No significant time effects were observed for the LEIDS-R aggression subscale for MSP (p = 0.37) and PLA (p = 0.06). No within-group differences were observed for either group using Wilcoxon signed-rank tests. Mann–Whitney U results indicated that MSP had significantly lower values than PLA at W0 (p = 0.02), W2 (p = 0.04), W4 (p = 0.002), W6 (p = 0.03), and W9 (p = 0.02). To account for differences at baseline, each subsequent value was subtracted from its respective baseline value and using this approach no significant differences were found between conditions for the LEIDS-R aggression subscale at W2 (= 0.854), W4 (p = 0.080), W6 (p = 0.887), and W9 (p = 0.896). No significant time effects were observed for the LEIDS-R control/perfectionism subscale for MSP (p = 0.75) and PLA (p = 0.61). No within-group differences were observed (p > 0.05) for either group using Wilcoxon signed-rank tests. No between-group differences were observed (p > 0.05) using Mann–Whitney U at all time points. When Bonferroni corrections were applied for multiple comparisons, no significant time effects were observed for the LEIDS-R risk aversion subscale for MSP (p = 0.04) and PLA (p = 0.04). Wilcoxon signed-rank tests indicated that W9 values were different than W0 in MSP (p = 0.004) and PLA (p = 0.01). No between-group differences were observed (p > 0.05) using Mann–Whitney U at all time points. No significant time effects were observed for the LEIDS-R rumination subscale for MSP (p = 0.27) and PLA (p = 0.38). Wilcoxon signed-rank tests indicated that W6 values were different than W0 in MSP (p = 0.02), but no within-group differences were present in PLA (p > 0.05). Between-group differences were observed at W6 (p = 0.04) and W9 (p = 0.04) while values tended to be different between groups after W2 (p = 0.07) and W4 (p = 0.07). No significant time effects were observed for the LEIDS-R total score for MSP (p = 0.31) and PLA (p = 0.18). No within-group differences were observed (p > 0.05) for either group using Wilcoxon signed-rank tests. Between-group differences were observed at W0 (MSP: 36.3 ± 13.4 vs. PLA: 43.1 ± 14.2, p = 0.04), W2 (MSP: 34.5 ± 13.5 vs. PLA: 41.8 ± 13.4, p = 0.04), W4 (MSP: 35.1 ± 15.2 vs. PLA: 43.3 ± 15.1, p = 0.03), W6 (MSP: 33.5 ± 14.2 vs. PLA: 41.6 ± 15.9, p = 0.03) and W9 (MSP: 31.9 ± 13.6 vs. PLA: 39.7 ± 15.2, p = 0.03). To account for differences at baseline, each subsequent value was subtracted from its respective baseline value and using this approach no significant differences were found between conditions for the LEIDS-R total at W2 (= 0.796), W4 (p = 0.338), W6 (p = 0.424), and W9 (p = 0.981).
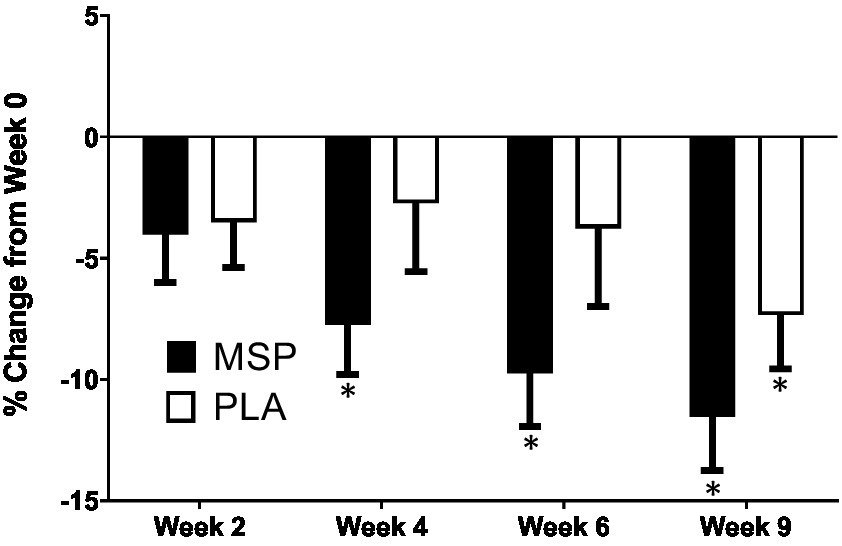
Figure 3. Percent change in Beck Depression Inventory scores. MSP, Multi-strain probiotic; PLA, placebo. *Indicates differences of specified time point from respective W0 (baseline) value using a Wilcoxon Signed-Rank Test (p < 0.05).
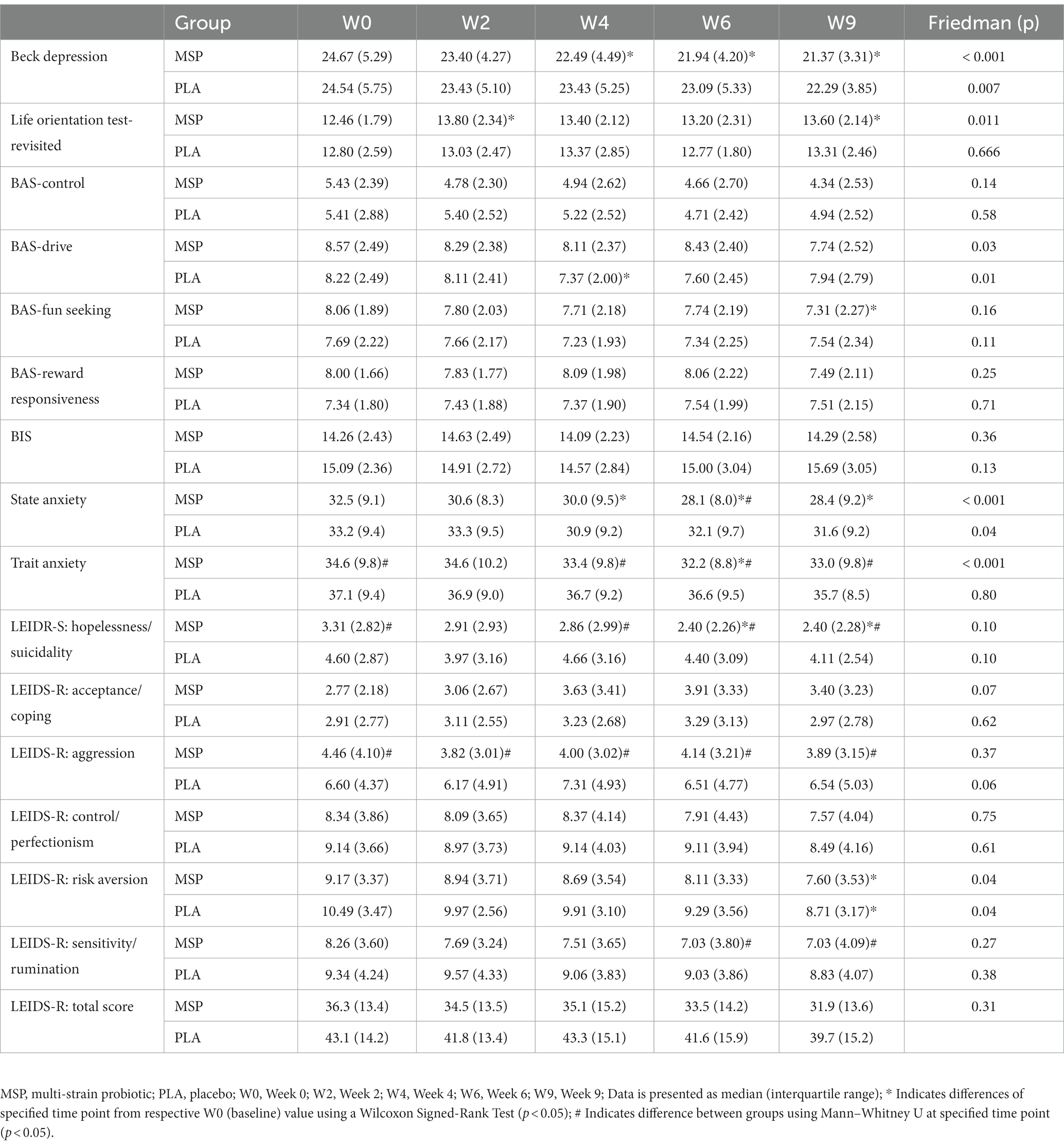
Table 3. Measured outcomes associated with mood-related constructs including the Beck Depression Inventory, State Anxiety, and Trait Anxiety, and the Leiden Index of Depression Sensitivity (LEIDS-R) after 0, 2, 4, and 6 weeks of supplementation as well as 3 weeks after stopping supplementation (week 9).
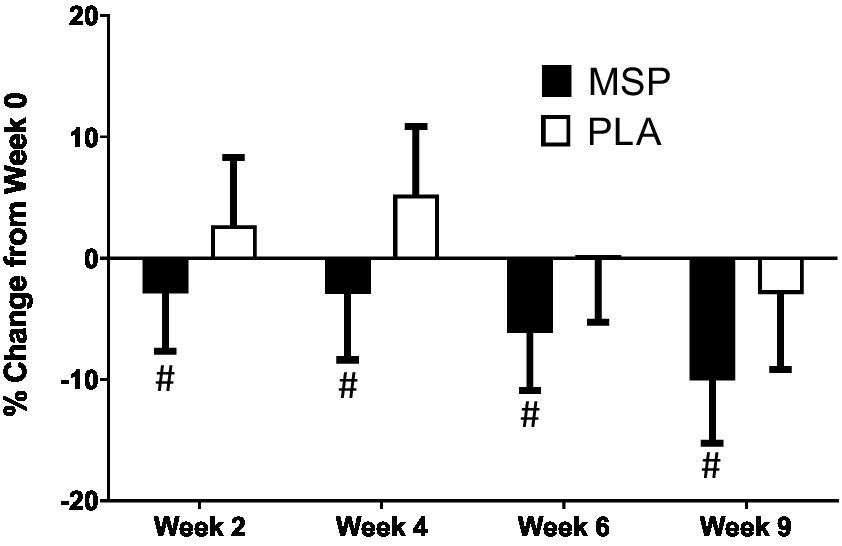
Figure 4. Percent change in Leiden Index of Depression Sensitivity (LEIDS) total score. MSP, Multi-strain probiotic; PLA, placebo. #Indicates difference between groups using Mann–Whitney U at specified time point.
Questionnaires assessing personality-related constructs
A significant time effect on LOT-R scores was observed in MSP (p = 0.011), but not PLA (p = 0.066) using the Friedman test (Table 4). Follow-up analysis using Wilcoxon signed-rank tests in MSP indicated that reported scores for week 0 (W0) were different than scores for week 2 (W2, p = 0.003) and W9 (p = 0.001) and were different from W4 (p = 0.03). No between-group differences (Mann–Whitney U) were observed at any of the five time points (p > 0.05). No significant gender x group x condition was observed for LOT-R total scores (p = 0.128). Four subscales of the behavioral activation scale (control, drive, fun seeking, reward responsiveness) (BAS) and the behavioral inhibition scale (BIS) were evaluated (Table 4). A significant time effect for BAS-Drive scores was observed in MSP (p = 0.03) and PLA (p = 0.01) using the Friedman test (Table 4). Follow-up analysis using Wilcoxon signed-rank tests in PLA indicated that reported scores for W4 (p = 0.003) were different than W2, while no other within-group differences were reported in this condition. No within-group differences between time points were observed in MSP. No significant time effect was observed for MSP or PLA for the control, fun seeking, and rewards responsiveness subscales or BIS. Further, no between-group differences (Mann–Whitney U) were observed at any of the five time points (p > 0.05) for any of the four BAS subscales and for the BIS. A significant time effect was observed for MSP scores for Cope Orientation to the Problems Experienced (COPE) mental disengagement subscale (p = 0.005). For PLA, significant time effects were observed for the COPE behavioral disengagement (p = 0.02), restraint (p = 0.01), use of emotional social support (p = 0.003), and acceptance (p = 0.04) subscales. Within-group changes in MSP were observed between W0 and W4 (p = 0.02), W6 (p = 0.004), and W9 (p = 0.003) for the COPE mental disengagement subscale. Within-group changes in PLA were observed between W0 and W4 for the behavioral disengagement (p = 0.009), use of emotional social support (p = 0.03) and restraint (p = 0.007) subscales, between W0 and W6 for the restraint (p = 0.01) and acceptance (p = 0.01) subscales, and between W0 and W9 for the restraint subscale (p = 0.02). Between-group differences were observed for the COPE behavioral disengagement subscale at W4 (p = 0.03), W6 (p = 0.05), and W9 (p = 0.04).
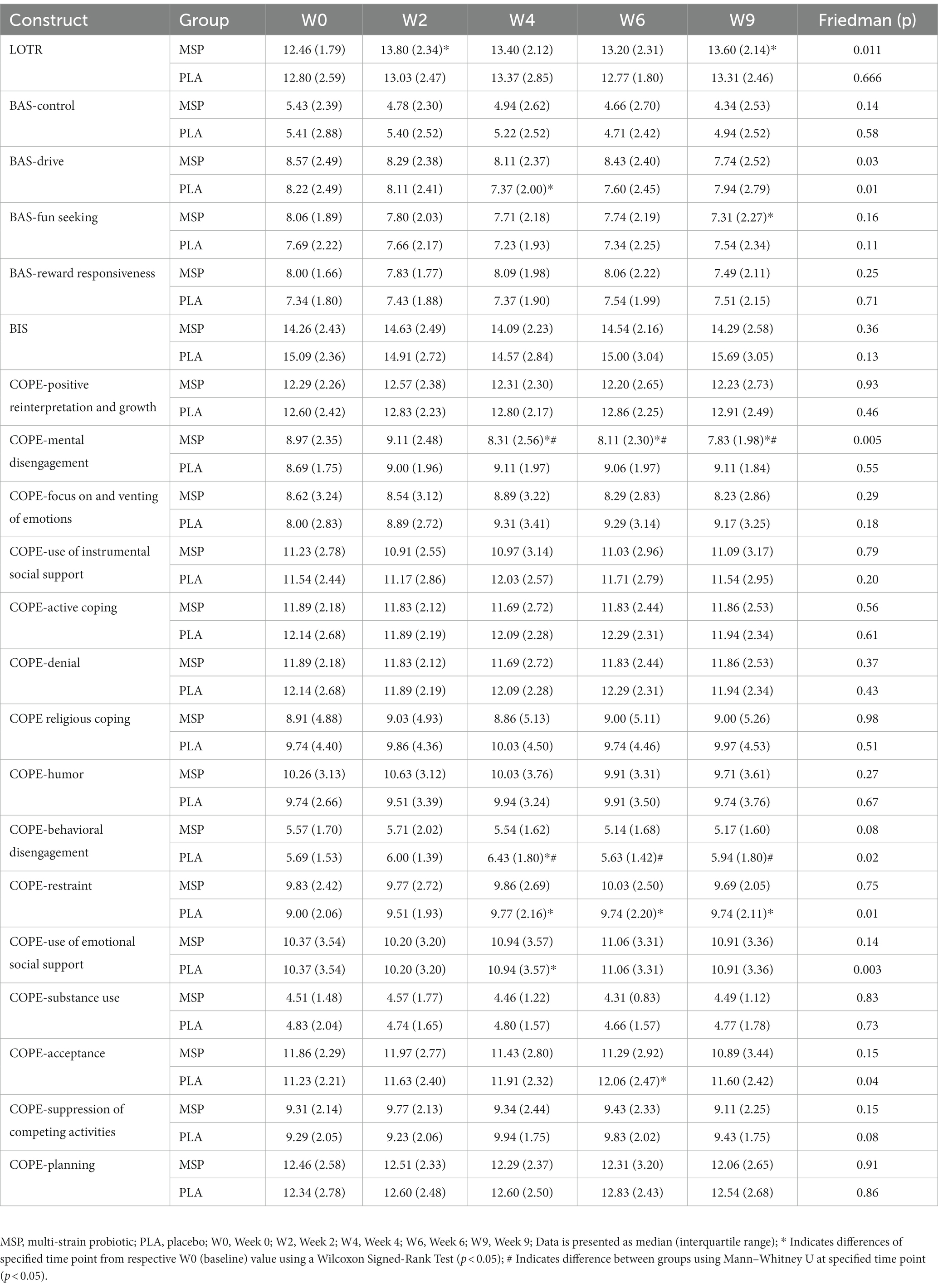
Table 4. Measured outcomes associated with mood-related constructs Life Orientation Test-Revisited (LOTR), Behavioral Activation (BAS), Behavioural Inhibition (BIS), and the Cope Orientation to the Problems Experienced (COPE) after 0, 2, 4, and 6 weeks of supplementation as well as 3 weeks after stopping supplementation (week 9).
Biomarkers assessment
No significant group x time interactions were observed for serotonin (p = 0.77), cortisol (p = 0.48), C-reactive protein (p = 0.45), and dopamine (p = 0.23). A significant time effect was observed for serotonin (p = 0.03) while no significant main effects for time were observed for dopamine (p = 0.71) and cortisol (p = 0.89), although observed changes in C-reactive protein tended to change (p = 0.052) across the study protocol. Simple main effects analysis indicated that serotonin levels significantly increased in MSP after W6 (p = 0.03) and W9 (p = 0.02) while no changes in serotonin were observed in PLA. Independent samples t-tests were completed to evaluate if serotonin responders and non-responders exhibited different changes in reported scores for BDI-II, state anxiety, trait anxiety, LEIDS-R total score, and LOT-R total. In all situations, no significant differences were found between the changes in these questionnaires and serotonin (p = 0.301–0.540). No significant correlations were found between the changes in serotonin and the observed changes in BDI-II Inventory (r = 0.143, p = 0.412), state anxiety (r = 0.078, p = 0.656), trait anxiety (r = 0.099, p = 0.572), LEIDS-R total score (r = −0.041, p = 0.817), and LOT-R total score (r = −0.101, p = 0.566).
Discussion
The aim of the present study was to evaluate the efficacy of multi-strain probiotic supplementation on circulating biomarkers and associated changes in mood, depression, and anxiety in healthy adults. Our results indicate that probiotic supplementation significantly improved outcomes related to depression, anxiety, and mood, alongside increases in serotonin concentrations, however, the probiotic supplementation had limited or no ability to impact outcomes related to associated coping mechanisms during stressful situations and plasma concentrations of cortisol, C-reactive protein, and dopamine. Previous work in clinical and non-clinical populations have provided evidence that probiotic supplementation can favorably impact mood, depression, and anxiety (29, 52, 53), however these outcomes are not universal (54–57). In particular, Marotta et al. (30) implemented a similar study design and used the same probiotic multi-strain formula as the present study and, reported improvements in the profile of mood states (POMS) questionnaire as well as LEIDS-R scale for acceptance. Results from the present study expands upon these findings whereby scores from three out of the six LEIDS-R subscales (hopeless, aggression, rumination), and the total score were significantly lower than scores observed for PLA (Figure 4). The positive changes in cognitive reactivity after MSP supplementation in the present study are also supported by the previous findings of Steenbergen et al. who supplemented 40 healthy adults with a multi-species probiotic for 4 weeks. In contrast to Marotta et al. (30) and Steenbergen et al. (29), the present investigation demonstrated significant improvements in depression scores as evaluated by the BDI-II (Figure 4). Unlike the studies of Marotta et al. (30) and Steenbergen et al. (29) was, the average values for the BDI-II could qualitatively be interpreted as our participants being mildly depressed (Table 3). In addition to the changes reported in mood and depression, changes in state and trait anxiety from supplementation were also evaluated. Participants supplementing with MSP reported significant reductions in state anxiety when compared to baseline, while no changes were reported across the protocol for PLA. Similarly, trait anxiety scores in MSP were lowered at W6 and tended to remain at W9. When compared to values reported in PLA, the MSP group reported significantly lower trait anxiety scores (Table 3). Of special note, the clinical trial was initiated in early 2020 prior to the outbreak of the COVID-19 global pandemic and was subsequently restarted in September 2020. The extent to which the stress and anxiety associated with the pandemic was impacted by our study design remains to be fully determined, but nonetheless our results were realized when many countries and societies were grappling with the challenges and struggles associated with the pandemic.
Changes in various questionnaires that focused on personality-associated outcomes were examined in the present study with several of these measures suggesting that MSP lacks application for altering these outcomes. For example, of the 15 subscales in the COPE questionnaire (Coping Orientation to Problem Experienced), no changes were identified for ten of the subscales (Table 4). For those subscales that were altered, the observed changes were within only one group, apart from Behavioral Disengagement, whereby PLA indicated they were more likely to use this coping mechanism than MSP at W4, W6, and W9. Similarly, no between-group differences were realized for the Behavioral Activation (BAS) and Inhibition (BIS) scales while limited changes across time were identified for the Drive and Fun Seeking subscales (Table 4). Overall, these results align with the previous work of Marotta et al. (30) where limited changes in the COPE and no changes in BIS/BAS were also reported. Finally, and somewhat in contrast to Marotta et al. (30), the MSP group reported significant changes in LOT-R scores which suggested that participants who supplemented with MSP adopted a more optimistic orientation as the protocol progressed. No such changes were identified in PLA and no differences between groups were found for LOT-R scores.
In response to supplementation, changes in concentrations of serotonin, dopamine, cortisol, and C-reactive protein in the blood were evaluated. No changes were identified for cortisol, C-reactive protein, or dopamine; however, serotonin concentrations were significantly increased in MSP (Table 5), while no changes were observed in PLA. These changes are valuable as they provide a mechanistic link to the changes observed in mood, depression, and anxiety questionnaires, albeit the exact mechanism remains to be further determined. Serotonin is a key neurotransmitter linked to various psychological and psychiatric states (34), while more than 95% of the body’s serotonin is produced in the gut (35). However, one must consider that plasma serotonin is not able to cross the blood–brain barrier and the reader should not interpret our findings to suggest the increased serotonin can directly interact with the brain. Instead, we posit as others have (30) that the administered probiotic regimen altered the intestinal microbiota composition in a manner that impacted the production of neurotransmitter precursors, short-chain fatty acids, or other secondary metabolites (23, 24) that went on to impact the regulation or production of various substances that modulated brain functions resulting in our observed changes in the mood-related questionnaires in the present study. Importantly, our findings support previous investigations demonstrating the ability of a MSP to improve mood and cognitive reactivity (29, 30), while extending these findings to demonstrate that changes may be associated with increases in serotonin due to supplementation. Previous work by Karbownik and colleagues (58), contrasts the findings from the present study, where a decrease in salivary serotonin in 32 healthy medical students supplementing with either a placebo or Saccharomyces boulardii CNCM I-1079 for 30 days prior to a stressful event was reported. However, a higher cohort age, a longer supplementation regimen, and the administration of multiple strains versus one strain in the present investigation, should be considered when comparing these outcomes. With regards to cortisol, several investigations using similar study designs, have similarly reported limited potential for probiotics to impact cortisol levels (54, 59).
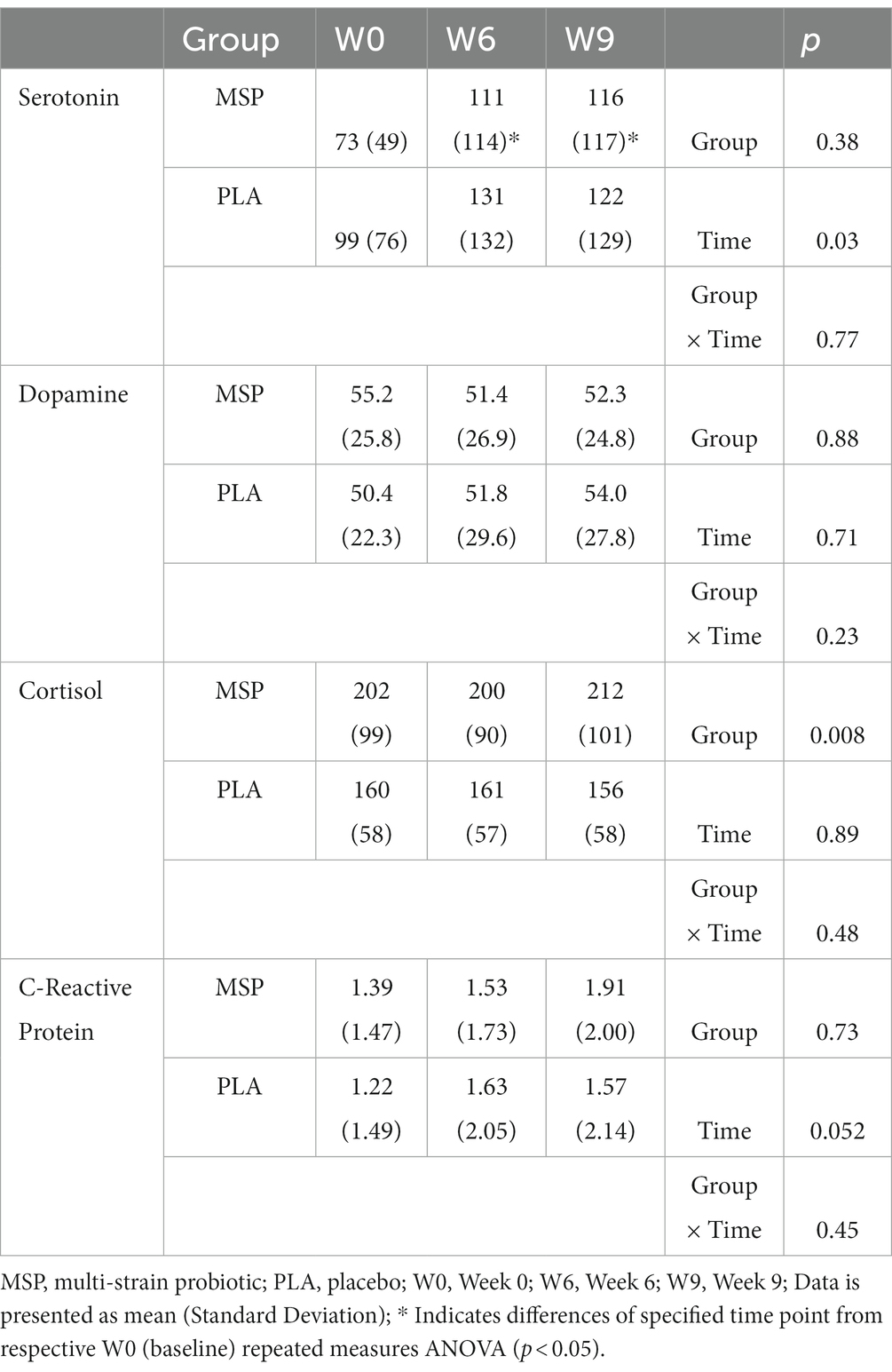
Table 5. Serotonin, dopamine, cortisol, and C-reactive protein after 0 and 6 weeks of supplementation as well as 3 weeks after stopping supplementation (week 9).
A specific strength for our protocol was that it utilized a randomized, double-blind, placebo-controlled approach, with a mixed gender cohort. As seen with Marotta et al. (30), the 6-week supplementation and 3-week washout period allows for additional insight into the impact of the probiotic regimen, including a brief time period after supplementation ceased. Future research should explore the potential impact of longer supplementation and washout periods. Additionally, the combination of applied outcomes from several psychobiological domains in conjunction with systemic biomarkers to evaluate potential relationships between applied outcomes mechanisms should be considered an investigational strength. Some limitations of our study should be acknowledged. First, our protocol was not adequately powered to properly evaluate any potential gender differences, as the a priori analysis was completed with the intent of examining the efficacy of the combination of probiotic strains however, the mixed gender cohort increased external validity. Nevertheless, Beck depression scores between men and women were significantly different. Future investigations should explore this relationship more thoroughly using an adequately powered design. Additionally, a more extensive investigation into the dimensions of cognitive processing and personality could help elucidate areas in which probiotics may exert the greatest impact. We did not control for any dietary considerations in this study protocol except for monitoring compliance to the supplementation regimen. From the food records collected, we were able to determine that each group did not significantly change the amount of energy or macronutrients consumed throughout the study protocol. We also calculated Healthy Eating Index values from our food records and further determined that the amount of various food groups consumed by each group did not change across the study protocol. While it is possible that certain specific foods were consumed that contained prebiotics and synbiotics prior to beginning the study and throughout the study, this seems unlikely considering our evaluation of our dietary data. One of the primary underlying mechanisms to explain the changes we observed in the mood-related questionnaires was the notion that our probiotic supplementation regimen altered the composition of the intestinal microbiota which impacted the production of neurotransmitter precursors that were involved in mood disposition, etc. We did not collect fecal samples throughout our investigation and as a result this suggestion is only based upon speculation from previous findings (5, 23, 24). As such, these samples would have been an effective tool to evaluate changes in the gut microbiome and to potentially demonstrate the supplementation protocol’s ability to alter the presence and richness of candidate bacterial communities. Future investigations should consider examining fecal samples, in addition to systemic blood biomarkers, and applied psychological investigational tools to further explore the potential widespread impact of probiotic supplementation.
Conclusion
In conclusion, the present study determined that in a cohort of 70 healthy men and women, a six-week regimen of a MSP consisting of four probiotic strains: Limosilactobacillus fermentum LF16 (DSM 26956), Lacticaseibacillus rhamnosus LR06 (DSM 21981), Lactiplantibacillus plantarum LP01 (LMG P-21021), and Bifidobacterium longum 04 (DSM 23233) providing a total dosage of 4 × 109 live cells/day favorably impacted reported scores on validated questionnaires assessing depression, anxiety, and mood, while increasing plasma concentrations of serotonin.
Data availability statement
The raw data supporting the conclusions of this article will be made available by the authors, without undue reservation.
Ethics statement
The studies involving humans were approved by Lindenwood University, Protocol IRB-19-212, approval date: July 17, 2019. The studies were conducted in accordance with the local legislation and institutional requirements. The participants provided their written informed consent to participate in this study.
Author contributions
CK, RJ, and MP: conception or design of the study. KW, AH, JK, JM, CG, LA, PM, and CK: acquisition of data and data collection. CK, PM, and AH: analysis and interpretation of data. All authors: drafting work and providing critical edits. All authors approved final version and agree to be held accountable for its contents.
Funding
This study was co-sponsored by Probiotical S.p.A. (Italy) and Ashland (USA). The sponsors had no role in collecting the data, analyzing the data, interpreting the results, or preparing the manuscript.
Acknowledgments
The authors would like to thank Angela Amoruso from Probiotical Research srl, in Novara, Italy for their efforts and support in this project. Your patience and professionalism has helped to make this project a success. In addition, the authors would like to thank the study participants for their commitment to this protocol.
Conflict of interest
MP works for Probiotical srl. MP played no part in conducting, analyzing, interpreting, or preparing the manuscript for publication. RJ is a scientific advisor to Ashland, a co-sponsor with this study and works for Increnovo LLC. He assisted with study design, but played no part in collecting, analyzing, or interpreting the results of this study.
The remaining authors declare that the research was conducted in the absence of any commercial or financial relationships that could be construed as a potential conflict of interest.
Publisher’s note
All claims expressed in this article are solely those of the authors and do not necessarily represent those of their affiliated organizations, or those of the publisher, the editors and the reviewers. Any product that may be evaluated in this article, or claim that may be made by its manufacturer, is not guaranteed or endorsed by the publisher.
Supplementary material
The Supplementary material for this article can be found online at: https://www.frontiersin.org/articles/10.3389/fnut.2023.1219313/full#supplementary-material
Footnotes
1. ^https://clinicaltrials.gov/ct2/show/NCT05343533
2. ^https://epi.grants.cancer.gov/asa24/
3. ^https://epi.grants.cancer.gov/hei/interpret-visualize-hei-scores.html
4. ^https://www.ema.europa.eu/en/ich-q1a-r2-stability-testing-new-drug-substances-drug-products-scientific-guideline
References
1. Grenham, S, Clarke, G, Cryan, JF, and Dinan, TG. Brain-gut-microbe communication in health and disease. Front Physiol. (2011) 2:94. doi: 10.3389/fphys.2011.00094
2. Grossman, MI. Neural and hormonal regulation of gastrointestinal function: an overview. Annu Rev Physiol. (1979) 41:27–33. doi: 10.1146/annurev.ph.41.030179.000331
3. Mayer, EA. Gut feelings: the emerging biology of gut-brain communication. Nat Rev Neurosci. (2011) 12:453–66. doi: 10.1038/nrn3071
4. Mayer, EA, Knight, R, Mazmanian, SK, Cryan, JF, and Tillisch, K. Gut microbes and the brain: paradigm shift in neuroscience. J Neurosci. (2014) 34:15490–6. doi: 10.1523/JNEUROSCI.3299-14.2014
5. Ahmed, H, Leyrolle, Q, Koistinen, V, Karkkainen, O, Laye, S, Delzenne, N, et al. Microbiota-derived metabolites as drivers of gut-brain communication. Gut Microbes. (2022) 14:2102878. doi: 10.1080/19490976.2022.2102878
6. Bravo, JA, Forsythe, P, Chew, MV, Escaravage, E, Savignac, HM, Dinan, TG, et al. Ingestion of Lactobacillus strain regulates emotional behavior and central GABA receptor expression in a mouse via the vagus nerve. Proc Natl Acad Sci U S A. (2011) 108:16050–5. doi: 10.1073/pnas.1102999108
7. Muller, PA, Schneeberger, M, Matheis, F, Wang, P, Kerner, Z, Ilanges, A, et al. Microbiota modulate sympathetic neurons via a gut-brain circuit. Nature. (2020) 583:441–6. doi: 10.1038/s41586-020-2474-7
8. Thion, MS, Ginhoux, F, and Garel, S. Microglia and early brain development: an intimate journey. Science. (2018) 362:185–9. doi: 10.1126/science.aat0474
9. Asano, Y, Hiramoto, T, Nishino, R, Aiba, Y, Kimura, T, Yoshihara, K, et al. Critical role of gut microbiota in the production of biologically active, free catecholamines in the gut lumen of mice. Am J Physiol Gastrointest Liver Physiol. (2012) 303:G1288–95. doi: 10.1152/ajpgi.00341.2012
10. Reigstad, CS, Salmonson, CE, Rainey, JF 3rd, Szurszewski, JH, Linden, DR, Sonnenburg, JL, et al. Gut microbes promote colonic serotonin production through an effect of short-chain fatty acids on enterochromaffin cells. FASEB J. (2015) 29:1395–403. doi: 10.1096/fj.14-259598
11. Yano, JM, Yu, K, Donaldson, GP, Shastri, GG, Ann, P, Ma, L, et al. Indigenous bacteria from the gut microbiota regulate host serotonin biosynthesis. Cells. (2015) 161:264–76. doi: 10.1016/j.cell.2015.02.047
12. Christiansen, CB, Gabe, MBN, Svendsen, B, Dragsted, LO, Rosenkilde, MM, and Holst, JJ. The impact of short-chain fatty acids on GLP-1 and PYY secretion from the isolated perfused rat colon. Am J Physiol Gastrointest Liver Physiol. (2018) 315:G53–65. doi: 10.1152/ajpgi.00346.2017
13. Samuel, BS, Shaito, A, Motoike, T, Rey, FE, Backhed, F, Manchester, JK, et al. Effects of the gut microbiota on host adiposity are modulated by the short-chain fatty-acid binding G protein-coupled receptor, Gpr41. Proc Natl Acad Sci U S A. (2008) 105:16767–72. doi: 10.1073/pnas.0808567105
14. Barrett, E, Ross, RP, O'Toole, PW, Fitzgerald, GF, and Stanton, C. Gamma-aminobutyric acid production by culturable bacteria from the human intestine. J Appl Microbiol. (2012) 113:411–7. doi: 10.1111/j.1365-2672.2012.05344.x
15. Chen, H, Nwe, PK, Yang, Y, Rosen, CE, Bielecka, AA, Kuchroo, M, et al. A forward chemical genetic screen reveals gut microbiota metabolites that modulate host physiology. Cells. (2019) 177:1217–1231.e18. doi: 10.1016/j.cell.2019.03.036
16. Adesso, S, Magnus, T, Cuzzocrea, S, Campolo, M, Rissiek, B, Paciello, O, et al. Indoxyl sulfate affects glial function increasing oxidative stress and neuroinflammation in chronic kidney disease: interaction between astrocytes and microglia. Front Pharmacol. (2017) 8:370. doi: 10.3389/fphar.2017.00370
17. Ren, Z, Zhang, R, Li, Y, Li, Y, Yang, Z, and Yang, H. Ferulic acid exerts neuroprotective effects against cerebral ischemia/reperfusion-induced injury via antioxidant and anti-apoptotic mechanisms in vitro and in vivo. Int J Mol Med. (2017) 40:1444–56. doi: 10.3892/ijmm.2017.3127
18. Verzelloni, E, Pellacani, C, Tagliazucchi, D, Tagliaferri, S, Calani, L, Costa, LG, et al. Antiglycative and neuroprotective activity of colon-derived polyphenol catabolites. Mol Nutr Food Res. (2011) 55:S35–43. doi: 10.1002/mnfr.201000525
19. McLoughlin, RF, Berthon, BS, Jensen, ME, Baines, KJ, and Wood, LG. Short-chain fatty acids, prebiotics, synbiotics, and systemic inflammation: a systematic review and meta-analysis. Am J Clin Nutr. (2017) 106:930–45. doi: 10.3945/ajcn.117.156265
20. Dalile, B, Van Oudenhove, L, Vervliet, B, and Verbeke, K. The role of short-chain fatty acids in microbiota-gut-brain communication. Nat Rev Gastroenterol Hepatol. (2019) 16:461–78. doi: 10.1038/s41575-019-0157-3
21. Mao, JH, Kim, YM, Zhou, YX, Hu, D, Zhong, C, Chang, H, et al. Genetic and metabolic links between the murine microbiome and memory. Microbiome. (2020) 8:53. doi: 10.1186/s40168-020-00817-w
22. Diaz Heijtz, R, Wang, S, Anuar, F, Qian, Y, Bjorkholm, B, Samuelsson, A, et al. Normal gut microbiota modulates brain development and behavior. Proc Natl Acad Sci U S A. (2011) 108:3047–52. doi: 10.1073/pnas.1010529108
23. Desbonnet, L, Garrett, L, Clarke, G, Bienenstock, J, and Dinan, TG. The probiotic Bifidobacteria infantis: an assessment of potential antidepressant properties in the rat. J Psychiatr Res. (2008) 43:164–74. doi: 10.1016/j.jpsychires.2008.03.009
24. Desbonnet, L, Garrett, L, Clarke, G, Kiely, B, Cryan, JF, and Dinan, TG. Effects of the probiotic Bifidobacterium infantis in the maternal separation model of depression. Neuroscience. (2010) 170:1179–88. doi: 10.1016/j.neuroscience.2010.08.005
25. Desbonnet, L, Clarke, G, Traplin, A, O'Sullivan, O, Crispie, F, Moloney, RD, et al. Gut microbiota depletion from early adolescence in mice: implications for brain and behaviour. Brain Behav Immun. (2015) 48:165–73. doi: 10.1016/j.bbi.2015.04.004
26. Hill, C, Guarner, F, Reid, G, Gibson, GR, Merenstein, DJ, Pot, B, et al. Expert consensus document. The International Scientific Association for Probiotics and Prebiotics consensus statement on the scope and appropriate use of the term probiotic. Nat Rev Gastroenterol Hepatol. (2014) 11:506–14. doi: 10.1038/nrgastro.2014.66
27. Li, W, Dowd, SE, Scurlock, B, Acosta-Martinez, V, and Lyte, M. Memory and learning behavior in mice is temporally associated with diet-induced alterations in gut bacteria. Physiol Behav. (2009) 96:557–67. doi: 10.1016/j.physbeh.2008.12.004
28. Tillisch, K, Labus, J, Kilpatrick, L, Jiang, Z, Stains, J, Ebrat, B, et al. Consumption of fermented milk product with probiotic modulates brain activity. Gastroenterology. (2013) 144:1394–1401, 1401 e1391-1394.
29. Steenbergen, L, Sellaro, R, van Hemert, S, Bosch, JA, and Colzato, LS. A randomized controlled trial to test the effect of multispecies probiotics on cognitive reactivity to sad mood. Brain Behav Immun. (2015) 48:258–64. doi: 10.1016/j.bbi.2015.04.003
30. Marotta, A, Sarno, E, Del Casale, A, Pane, M, Mogna, L, Amoruso, A, et al. Effects of probiotics on cognitive reactivity, mood, and sleep quality. Front Psych. (2019) 10:164. doi: 10.3389/fpsyt.2019.00164
31. Nobis, A, Zalewski, D, and Waszkiewicz, N. Peripheral markers of depression. J Clin Med. (2020) 9:3793. doi: 10.3390/jcm9123793
32. Edebol Carlman, HMT, Rode, J, Konig, J, Repsilber, D, Hutchinson, AN, Thunberg, P, et al. Probiotic mixture containing Lactobacillus helveticus, Bifidobacterium longum and Lactiplantibacillus plantarum affects brain responses to an arithmetic stress task in healthy subjects: a randomised clinical trial and proof-of-concept study. Nutrients. (2022) 14:1329. doi: 10.3390/nu14071329
33. Kazemi, A, Noorbala, AA, Azam, K, and Djafarian, K. Effect of prebiotic and probiotic supplementation on circulating pro-inflammatory cytokines and urinary cortisol levels in patients with major depressive disorder: a double-blind, placebo-controlled randomized clinical trial. J Funct Foods. (2019) 52:596–602. doi: 10.1016/j.jff.2018.11.041
34. Olivier, JDA, and Olivier, B. Translational studies in the complex role of neurotransmitter Systems in Anxiety and Anxiety Disorders. Adv Exp Med Biol. (2020) 1191:121–40. doi: 10.1007/978-981-32-9705-0_8
35. Gershon, MD, and Tack, J. The serotonin signaling system: from basic understanding to drug development for functional GI disorders. Gastroenterology. (2007) 132:397–414. doi: 10.1053/j.gastro.2006.11.002
36. Riezzo, G, Chimienti, G, Orlando, A, D'Attoma, B, Clemente, C, and Russo, F. Effects of long-term administration of Lactobacillus reuteri DSM-17938 on circulating levels of 5-HT and BDNF in adults with functional constipation. Benef Microbes. (2019) 10:137–47. doi: 10.3920/BM2018.0050
37. Liu, G, Chong, HX, Chung, FY, Li, Y, and Liong, MT. Lactobacillus plantarum DR7 modulated bowel movement and gut microbiota associated with dopamine and serotonin pathways in stressed adults. Int J Mol Sci. (2020) 21:4608. doi: 10.3390/ijms21134608
38. Bravo, JA, Julio-Pieper, M, Forsythe, P, Kunze, W, Dinan, TG, Bienenstock, J, et al. Communication between gastrointestinal bacteria and the nervous system. Curr Opin Pharmacol. (2012) 12:667–72. doi: 10.1016/j.coph.2012.09.010
39. Cummings, DM, King, DE, Mainous, AG, and Geesey, ME. Combining serum biomarkers: the association of C-reactive protein, insulin sensitivity, and homocysteine with cardiovascular disease history in the general US population. Eur J Cardiovasc Prev Rehabil. (2006) 13:180–5. doi: 10.1097/01.hjr.0000185973.59512.d3
40. Ford, ES, Ajani, UA, Mokdad, AH, National, H, and Nutrition, E. The metabolic syndrome and concentrations of C-reactive protein among U.S. youth. Diabetes Care. (2005) 28:878–81. doi: 10.2337/diacare.28.4.878
41. Ridker, PM, Buring, JE, Shih, J, Matias, M, and Hennekens, CH. Prospective study of C-reactive protein and the risk of future cardiovascular events among apparently healthy women. Circulation. (1998) 98:731–3. doi: 10.1161/01.CIR.98.8.731
42. Ajani, UA, Ford, ES, and Mokdad, AH. Dietary fiber and C-reactive protein: findings from national health and nutrition examination survey data. J Nutr. (2004) 134:1181–5. doi: 10.1093/jn/134.5.1181
43. Ma, Y, Hebert, JR, Li, W, Bertone-Johnson, ER, Olendzki, B, Pagoto, SL, et al. Association between dietary fiber and markers of systemic inflammation in the Women's Health Initiative Observational Study. Nutrition. (2008) 24:941–9. doi: 10.1016/j.nut.2008.04.005
44. Romijn, AR, Rucklidge, JJ, Kuijer, RG, and Frampton, C. A double-blind, randomized, placebo-controlled trial of Lactobacillus helveticus and Bifidobacterium longum for the symptoms of depression. Aust N Z J Psychiatry. (2017) 51:810–21. doi: 10.1177/0004867416686694
45. Akkasheh, G, Kashani-Poor, Z, Tajabadi-Ebrahimi, M, Jafari, P, Akbari, H, Taghizadeh, M, et al. Clinical and metabolic response to probiotic administration in patients with major depressive disorder: a randomized, double-blind, placebo-controlled trial. Nutrition. (2016) 32:315–20. doi: 10.1016/j.nut.2015.09.003
46. van der Does, W, and Williams, J. Leiden index of depression sensitivity - Revised (LEIDS-R). Leiden: Leiden University (2003).
47. Spielberger, C, Gorsuch, R, Lushene, R, Vagg, P, and Jacobs, G. Manual for the state-trait anxiety inventory. Palo Alto, CA: Consulting Psychologists Press (1983).
48. Beck, A, Steer, R, and Brown, G. Manual for Beck depression inventory II (BDI-II). San Antonio, TX: Psychology Corporation (1996).
49. Carver, CS, Scheier, MF, and Weintraub, JK. Assessing coping strategies: a theoretically based approach. J Pers Soc Psychol. (1989) 56:267–83. doi: 10.1037/0022-3514.56.2.267
50. Jorm, A, Christense, H, Henderson, A, Jacomb, P, Korten, A, and Rodgers, B. Using the BIS/BAS scales to measure behavioral inhibition and behavioral activation: factor structure, validity and norms in a large community samples. Peraonal Individ Differ. (1999) 26:49–58.
51. Scheier, MF, Carver, CS, and Bridges, MW. Distinguishing optimism from neuroticism (and trait anxiety, self-mastery, and self-esteem): a reevaluation of the Life Orientation Test. J Pers Soc Psychol. (1994) 67:1063–78. doi: 10.1037/0022-3514.67.6.1063
52. Benton, D, Williams, C, and Brown, A. Impact of consuming a milk drink containing a probiotic on mood and cognition. Eur J Clin Nutr. (2007) 61:355–61. doi: 10.1038/sj.ejcn.1602546
53. Messaoudi, M, Violle, N, Bisson, JF, Desor, D, Javelot, H, and Rougeot, C. Beneficial psychological effects of a probiotic formulation (Lactobacillus helveticus R0052 and Bifidobacterium longum R0175) in healthy human volunteers. Gut Microbes. (2011) 2:256–61. doi: 10.4161/gmic.2.4.16108
54. Kelly, JR, Allen, AP, Temko, A, Hutch, W, Kennedy, PJ, Farid, N, et al. Lost in translation? The potential psychobiotic Lactobacillus rhamnosus (JB-1) fails to modulate stress or cognitive performance in healthy male subjects. Brain Behav Immun. (2017) 61:50–9. doi: 10.1016/j.bbi.2016.11.018
55. Ng, QX, Peters, C, Ho, CYX, Lim, DY, and Yeo, WS. A meta-analysis of the use of probiotics to alleviate depressive symptoms. J Affect Disord. (2018) 228:13–9. doi: 10.1016/j.jad.2017.11.063
56. Nikolova, V, Zaidi, SY, Young, AH, Cleare, AJ, and Stone, JM. Gut feeling: randomized controlled trials of probiotics for the treatment of clinical depression: systematic review and meta-analysis. Ther Adv Psychopharmacol. (2019) 9:2045125319859963. doi: 10.1177/2045125319859963
57. Smith, KS, Greene, MW, Babu, JR, and Fruge, AD. Psychobiotics as treatment for anxiety, depression, and related symptoms: a systematic review. Nutr Neurosci. (2021) 24:963–77. doi: 10.1080/1028415X.2019.1701220
58. Karbownik, MS, Kreczynska, J, Wiktorowska-Owczarek, A, Kwarta, P, Cybula, M, Stilinovic, N, et al. Decrease in salivary serotonin in response to probiotic supplementation with Saccharomyces boulardii in healthy volunteers under psychological stress: secondary analysis of a randomized, double-blind, placebo-controlled trial. Front Endocrinol. (2021) 12:800023
59. Mohammadi, AA, Jazayeri, S, Khosravi-Darani, K, Solati, Z, Mohammadpour, N, Asemi, Z, et al. The effects of probiotics on mental health and hypothalamic-pituitary-adrenal axis: a randomized, double-blind, placebo-controlled trial in petrochemical workers. Nutr Neurosci. (2016) 19:387–95. doi: 10.1179/1476830515Y.0000000023
Keywords: probiotic, gut-brain, quality of life, mood, serotonin
Citation: Walden KE, Moon JM, Hagele AM, Allen LE, Gaige CJ, Krieger JM, Jäger R, Mumford PW, Pane M and Kerksick CM (2023) A randomized controlled trial to examine the impact of a multi-strain probiotic on self-reported indicators of depression, anxiety, mood, and associated biomarkers. Front. Nutr. 10:1219313. doi: 10.3389/fnut.2023.1219313
Edited by:
Yashi Mi, University of Arizona, United StatesReviewed by:
Sadegh Jafarnejad, Kashan University of Medical Sciences, IranRong Fan, University of Massachusetts Amherst, United States
Di Zhu, Chi Forest (Beijing) Food Technology Group Co., Ltd., China
Copyright © 2023 Walden, Moon, Hagele, Allen, Gaige, Krieger, Jäger, Mumford, Pane and Kerksick. This is an open-access article distributed under the terms of the Creative Commons Attribution License (CC BY). The use, distribution or reproduction in other forums is permitted, provided the original author(s) and the copyright owner(s) are credited and that the original publication in this journal is cited, in accordance with accepted academic practice. No use, distribution or reproduction is permitted which does not comply with these terms.
*Correspondence: Chad M. Kerksick, ckerksick@lindenwood.edu
†ORCID: Jessica M. Moon, https://orcid.org/0000-0003-4702-3077
Anthony M. Hagele, https://orcid.org/0000-0002-5575-3670
Chad M. Kerksick, https://orcid.org/0000-0003-0458-7294