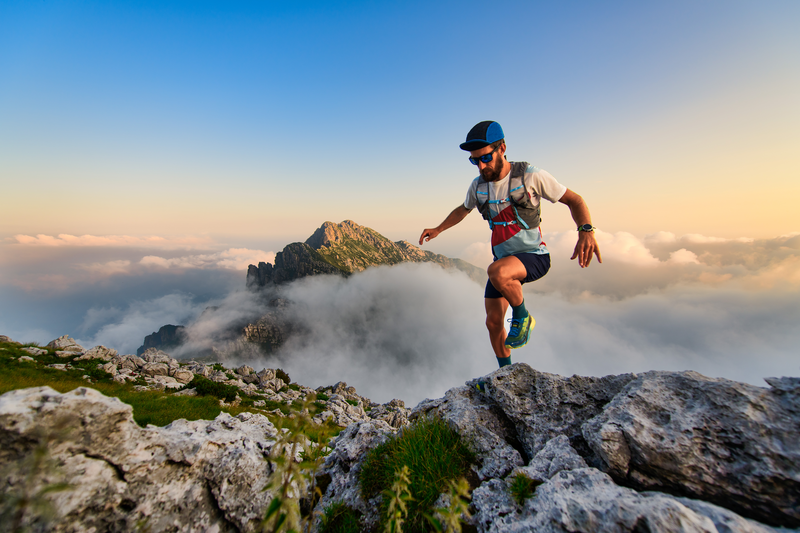
95% of researchers rate our articles as excellent or good
Learn more about the work of our research integrity team to safeguard the quality of each article we publish.
Find out more
ORIGINAL RESEARCH article
Front. Nutr. , 13 September 2023
Sec. Nutrition and Metabolism
Volume 10 - 2023 | https://doi.org/10.3389/fnut.2023.1207394
This article is part of the Research Topic Dietary Influences on White and Brown Adipose Tissue: Mechanisms and Health Implications View all 3 articles
Introduction: Brown adipose tissue (BAT) dissipates energy in the form of heat majorly via the mitochondrial uncoupling protein 1 (UCP1). The activation of BAT, which is enriched in the neck area and contains brown and beige adipocytes in humans, was considered as a potential therapeutic target to treat obesity. Therefore, finding novel agents that can stimulate the differentiation and recruitment of brown or beige thermogenic adipocytes are important subjects for investigation. The current study investigated how the availability of extracellular thiamine (vitamin B1), an essential cofactor of mitochondrial enzyme complexes that catalyze key steps in the catabolism of nutrients, affects the expression of thermogenic marker genes and proteins and subsequent functional parameters during ex vivo adipocyte differentiation.
Methods: We differentiated primary human adipogenic progenitors that were cultivated from subcutaneous (SC) or deep neck (DN) adipose tissues in the presence of gradually increasing thiamine concentrations during their 14-day differentiation program. mRNA and protein expression of thermogenic genes were analyzed by RT-qPCR and western blot, respectively. Cellular respiration including stimulated maximal and proton-leak respiration was measured by Seahorse analysis.
Results: Higher thiamine levels resulted in increased expression of thiamine transporter 1 and 2 both at mRNA and protein levels in human neck area-derived adipocytes. Gradually increasing concentrations of thiamine led to increased basal, cAMP-stimulated, and proton-leak respiration along with elevated mitochondrial biogenesis of the differentiated adipocytes. The extracellular thiamine availability during adipogenesis determined the expression levels of UCP1, PGC1a, CKMT2, and other browning-related genes and proteins in primary SC and DN-derived adipocytes in a concentration-dependent manner. Providing abundant amounts of thiamine further increased the thermogenic competency of the adipocytes.
Discussion: Case studies in humans reported that thiamine deficiency was found in patients with type 2 diabetes and obesity. Our study raises the possibility of a novel strategy with long-term thiamine supplementation, which can enhance the thermogenic competency of differentiating neck area-derived adipocytes for preventing or combating obesity.
Obesity is defined as the abnormal or excessive accumulation of fat, which has a long-term negative impact on health, as it contributes to the development of a number of chronic conditions and diseases (1), such as type 2 diabetes mellitus (DM), cardiovascular diseases, hypercholesterolemia, asthma, or certain type of cancers (2). World Health Organization (WHO) reported that since 1975, the prevalence of obesity has almost tripled worldwide (3).
Two types of adipose tissue have been identified: white adipose tissue (WAT), which is majorly responsible for energy storage, and brown adipose tissue (BAT), which maintains core body temperature through non-shivering thermogenesis (4). White adipocytes contain one large unilocular lipid droplet and few mitochondria, whereas brown adipocytes possess multilocular lipid droplets and large amounts of mitochondria that express uncoupling protein 1 (UCP1) which is referred as the major molecular marker of thermogenic fat cells (5, 6). Another, inducible form of thermogenic adipocytes is called beige (also known as brite) adipocytes. Beige adipocytes possess a transitional phenotype between white and brown adipocytes. They are considered as the subset of WAT but have several properties of brown adipocytes, including increased UCP1 expression, multilocular triglyceride storage, mitochondria density as well as vascular supply, all of which promote the process of adaptive thermogenesis (7). The differentiation of beige adipocytes can be induced, e.g., by cold, physical exercise, peroxisome proliferator-activated receptor (PPAR) γ, or ß3-receptor agonists which drive the process termed “browning” (5, 6).
Human BAT was mainly considered as a tissue type that can only be found in newborns in the axillary, cervical, perirenal, and periadrenal regions maintaining the proper core body temperature without shivering (8). Because the amount of BAT decreases shortly after birth, it has been considered for a long period of time to be functionally insignificant in adults. However, several studies using positron emission tomography (PET) provided evidence that adults have significant amounts of BAT and its activity can be increased by cold exposure. The most common location for BAT in adult humans is the cervical-supraclavicular depot marked by high labeled glucose uptake in that region (9, 10). Assessing the morphology of the biopsies’ specimen from the supraclavicular depot, adipocytes with numerous multilocular and intracellular lipid droplets were detected. A more recent study improved the PET-computed tomography (CT) method to localize brown/beige adipose depots in humans. Using a refined technique of the PET-CT method, Leitner et al. (11) identified the whole-body BAT distribution and estimated its thermogenic capacity. BAT and brownable adipose depots were found interspersed in several areas, such as cervical, supraclavicular, axillary, mediastinal, paraspinal, and abdominal. Intriguingly, individuals with obesity possessed a higher amount of brownable adipose tissue than lean individuals. However, the amount of active BAT in people with obesity was found to be lower than in lean individuals (11).
Research on brown and beige adipocytes has gained an increased attraction in the recent years as they can provide a potential new therapeutic target in the treatment of obesity and various metabolic disorders (12–14). A strong negative correlation was shown between the activity of BAT and the body mass index or the percentage of body fat in humans (15). Targeting BAT by enhancing its thermogenic activity or by recruiting new beige adipocytes through the “browning” process can improve glucose utilization and lipid clearance, leading to an improved metabolic balance. With this regard, possible strategies have been raised including central stimulation of the sympathetic innervation of BAT, transplantation of the existing BAT, as well as stem cell therapy (16).
Previously, we reported the global expression profile and thermogenic capacity of adipocytes derived from subcutaneous (SC) and deep neck (DN) biopsies (17). Based on the RNA-sequencing analysis, 1,049 differentially expressed genes (DEGs) were found in the comparison of SC and DN-derived adipocytes. Among these DEGs, we found 21 solute carrier (SLC) transporters and revealed the importance of alanine-serine-cysteine transporter 1 (ASC1, encoded by SLC7A10) (18) and thiamine transporter 2 (ThTr2, encoded by SLC19A3) (19) during thermogenic activation. One of the strongest upregulated SLC transporters in thermogenic DN-derived adipocytes was ThTr2, whose pharmacological inhibition attenuated cAMP-mediated thermogenic activation of human neck area-derived adipocytes (19).
Vitamin B1, also known as thiamine, is a water-soluble vitamin that plays a crucial role in carbohydrate, lipid, and protein metabolism (20). Intracellular thiamine is mainly present in its active form, thiamine pyrophosphate (TPP) which is formed by TPP kinase. TPP acts as a coenzyme for several enzymes, such as α-ketoglutarate dehydrogenase or pyruvate dehydrogenase (PDH) therefore contributing to the activity of the tricarboxylic acid cycle. The presence of TPP is also required for transketolase (shifts excess fructose-6-phosphate to glyceraldehyde-3-phosphate) enzyme acting in the pentose phosphate pathway (21). The level of thiamine in the human body is entirely dependent on dietary intake; there is no known endogenous synthesis, although some types of bacteria in the gut can produce small amounts of thiamine (22). The half-life of thiamine is notably short (1–12 h) and its storage capacity in the body is limited; therefore, continuous dietary supplementation is necessary to maintain tissue thiamine levels at a constant level (23). The total thiamine concentration in human blood plasma ranges around 10–20 nM, which is remarkably lower as compared to rodents, where it can reach 100 nM (24).
Our current study presents a novel approach to facilitate human adipocyte browning ex vivo by providing either gradually increasing or abundant amounts of thiamine during the differentiation of human neck area-derived adipocytes. The presence of gradually increasing concentrations of thiamine in a thiamine-free medium led to elevated basal, cAMP-stimulated, and proton-leak respiration along with increased mitochondrial biogenesis of the differentiated adipocytes. Long-term thiamine treatment also upregulated thermogenesis-related genes and proteins in primary SC and DN-derived adipocytes in an increased proportion to the available thiamine concentration. These results suggest that thiamine availability determines the efficiency of differentiation towards thermogenic cells.
The commercially available culture media contain a much higher thiamine concentration (8.2 μM) than that is found in human blood plasma. Providing excess (25 μM or 50 μM) thiamine further increased the thermogenic competency of SC and DN-derived adipocytes. Our results raise the possibility of a novel strategy of long-term thiamine supplementation, which can elevate the thermogenic competency of differentiating neck area-derived adipocytes for combating obesity.
All chemicals were acquired from Sigma-Aldrich (Munich, Germany) unless otherwise stated.
Tissue collection was approved by the Medical Research Council of Hungary (20571-2/2017/EKU) followed by the EU Member States’ Directive 2004/23/EC on presumed consent practice for tissue collection. All experiments were carried out in accordance with the guidelines of the Helsinki Declaration. Written informed consent was obtained from all participants before the surgical procedure. During thyroid surgeries, pairs of DN and SC adipose tissue biopsy samples were obtained to rule out inter-individual variations. Patients with known DM, malignant tumor, or abnormal thyroid hormone levels at the time of surgery were excluded. Human adipose-derived stromal cells (hASCs) were isolated from the stromal-vascular fraction (SVF) of SC and DN fat biopsies as described previously (17, 25). The absence of mycoplasma contamination was confirmed by PCR analysis (PCR Mycoplasma Test Kit I/C, Promocell, Heidelberg, Germany).
Human primary adipocytes from SVF of adipose tissue containing hASCs (or preadipocytes) were seeded in 6-well plates, eight-well Ibidi μ-chambers (Ibidi GmbH, Gräfelfing, Germany), or XF96 assay plates (Seahorse Biosciences, North Billerica, MA, United States) and cultured in Dulbecco’s Modified Eagle’s Medium/Nutrient F-12 Ham (DMEM-F12) medium containing 33 μM biotin, 17 μM pantothenic acid, 100 U/mL penicillin/streptomycin, and 10% fetal bovine serum (Thermo Fisher Scientific, Waltham, MA, United States) at 37°C in 5% CO2 until they reached complete confluence.
The adipocytes were differentiated by regular adipogenic differentiation medium (ADIP) which was induced for 3 days with serum-free DMEM-F12 medium or thiamine-free medium (Gibco, Waltham, MA, United States, cat# ME2107367) supplemented with 33 μM biotin, 17 μM pantothenic acid, 100 U/mL penicillin/streptomycin, 2 μM rosiglitazone (Cayman Chemicals, Ann Arbor, MI, United States), 25 nM dexamethasone, 0.25 mM 3-isobutyl-l-methylxantine, 0.1 μM cortisol, 0.01 mg/mL human apo-transferrin, 0.2 nM triiodothyronine, and 20 nM human insulin. After the third day, rosiglitazone, dexamethasone, and 3-isobutyl-l-methylxantine were removed from the medium for the remaining 11 days of the differentiation (26).
The differentiation of adipocytes with higher browning capacity (B-ADIP) was induced for 3 days with serum-free DMEM-F12 medium or thiamine-free medium supplemented with 33 μM biotin, 17 μM pantothenic acid, 100 U/mL penicillin/streptomycin, 1 μM dexamethasone, 0.5 mM 3-isobutyl-l-methylxantine, 0.01 mg/mL human apo-transferrin, 0.2 nM triiodothyronine, and 0.85 μM human insulin. After the third day, dexamethasone and 3-isobutyl-l-methylxantine were removed and 500 nM rosiglitazone was added to the medium for the remaining 11 days of differentiation (17, 27).
Abundant and gradually increasing concentrations of thiamine (cat# 731188) were added from the third day of the differentiation until the fourteenth day. In thiamine concentration-dependence experiments, we used a custom-made thiamine-free culture fluid for differentiating the cells supplemented with gradually increasing concentrations (0.04 μM, 0.2 μM, 1 μM, 5 μM, or 25 μM) of thiamine throughout the last 11 days of differentiation. In these experiments, the control (Ctrl) cells were differentiated in the absence of thiamine throughout the entire differentiation process. In the experiments with excess thiamine, hASCs were differentiated using DMEM-F12 medium with or without the addition of excess (25 μM or 50 μM) thiamine throughout the last 11 days of differentiation. In these experiments, the Ctrl cells were differentiated only in the presence of thiamine found in the DMEM-F12, which was 8.2 μM, throughout the entire differentiation process. Media were changed every 3 days and cells were lysed or analyzed after 14 days of differentiation. In every repetition, Ctrl samples were obtained from the same donor. Cells were incubated at 5% CO2 and 37°C. Adipogenic differentiation ratio was quantified using laser-scanning cytometry as previously described (26, 28).
Cells were collected in TRIzol (Molecular Research Centre, Cincinnati, OH, United States, cat#TR 118), total RNA was isolated by chloroform-isopropanol extraction, and quantitative real time PCR (RT-qPCR) was performed as described previously (19, 29, 30). Custom-made gene primers and probes were designed and supplied by Thermo Fisher Scientific as listed in Supplementary Table S1.
Immunoblotting was carried out as described previously (19, 31). Antibodies and working dilutions are listed in Supplementary Table S2. FIJI ImageJ software (National Institutes of Health, Bethesda, MD, United States) was used for densitometry analysis, where the density value of the target protein of interest was divided by the density value of the tubulin household protein, thus obtaining the normalized optical density.
Oxygen consumption and extracellular acidification rates (OCR and ECAR) of adipocytes, which were differentiated as described in 2.3, were measured using an XF96 oximeter (Seahorse Biosciences) according to previously optimized protocols (19, 29, 30). When the adipocytes were differentiated in the presence of excess thiamine, cells were pre-incubated for 1 h and treated in Agilent Seahorse XF Base Medium (Agilent Technologies, Santa Clara, CA, United States, cat#103334-100), which is optimized for extracellular flux assays and contains thiamine at 8.2 μM concentration. When the adipocytes were differentiated at thiamine concentrations close to the physiological blood plasma levels, the measurement was performed in thiamine-free medium to carry out the assay with the previously obtained thiamine pool of the cells which was gained during differentiation. Since thiamine-free medium was used instead of the buffer-free Agilent Seahorse XF Base Medium during the measurement, it was not possible to determine ECAR in this setting. In both types of experimental settings, after recording the baseline OCR, 500 μM dibutyril-cAMP (cat#D0627) was injected to mimic an adrenergic cue; then stimulated OCR was recorded every 20 min. Proton-leak respiration was monitored after injecting oligomycin (cat#495455) at 2 μM concentration. Finally, adipocytes received a single bolus of Antimycin A (cat#A8674) at 10 μM concentration for baseline correction (measuring non-mitochondrial respiration). The OCR was normalized to protein content.
The results are expressed as mean ± SD. The normality of the distribution of the data was tested by Shapiro–Wilk test. For multiple comparisons of each group, one-way or two-way ANOVA and Tukey’s post hoc test were used. The data were visualized and analyzed by using GraphPad Prism 8 (GraphPad Software, San Diego, CA, United States).
In our previous study, we found that high expression of ThTrs and high thiamine influx contributed to the effective thermogenic activation of neck area-derived adipocytes (19). Therefore, we primarily investigated how the expression of the ThTrs is affected by the presence of excess (25 μM or 50 μM) or gradually increasing (0.04 μM, 0.2 μM, 1 μM, or 5 μM) concentrations of thiamine during the differentiation of human SC and DN-derived adipocytes differentiated either by regular adipogenic differentiation medium (ADIP) or long-term rosiglitazone treatment with enhanced thermogenic capacity (B-ADIP) (19). In the first experimental setup, adipocytes were differentiated to ADIPs or B-ADIPs at regular culture conditions; in the presence of 8.2 μM thiamine found in DMEM-F12 medium, or when excess thiamine was administered to the differentiation regimen (Figure 1A). The amount of extracellular thiamine did not affect the differentiation ratio of ADIPs (Figure 1B). We reported recently that ThTr2, but not ThTr1, was expressed at a higher level in DN-derived ADIPs, compared with SC ones, both at mRNA and protein levels (19). Focusing on the different applied thiamine concentrations, 25 μM thiamine treatment significantly increased the expression of ThTr1 in SC-derived B-ADIPs, while in DN-derived B-ADIPs, both 25 μM and 50 μM treatments resulted in an increasing effect (Figure 1C). The 50 μM excess thiamine significantly increased the expression of ThTr2 in DN-derived B-ADIPs at mRNA level (Figure 1D), while ThTr2 protein expression was significantly upregulated when SC-derived B-ADIPs were differentiated in the presence of 25 μM thiamine (Figure 1E and Supplementary Figure S1A). The expression of ThTr1 at protein level was not affected by the presence of abundant amounts of thiamine in the adipogenic media (Figure 1E and Supplementary Figure S1A).
Figure 1. Effect of excess (25 μM and 50 μM) concentrations of thiamine (Th) on the expression of Th transporters (ThTrs) in human subcutaneous (SC) and deep neck (DN)-derived adipocytes (ADIPs) and brown differentiated adipocytes (B-ADIPs). ADIPs and B-ADIPs were differentiated at regular culture conditions (Ctrl, 8.2 μM Th, white bars) or in the presence of 25 μM (Th25) or 50 μM (Th50) of excess Th (orange bars). (A) Schematic illustration of the differentiation protocol. (B) Quantification of differentiation ratio by laser-scanning cytometry, n = 4. (C,D) mRNA expression of SLC19A2 and SLC19A3 assessed by RT-qPCR, n = 6. (E) ThTr1 (SLC19A2) and ThTr2 (SLC19A3) protein expression detected by immunoblotting, n = 4. Uncropped images are shown in Supplementary Figure S1A. Statistical analysis was performed by two-way ANOVA, *p < 0.05, **p < 0.01.
In the second experimental setup, we studied the importance of thiamine availability even at ranges corresponding to physiological levels during adipocyte differentiation, where SC or DN-derived hASCs were differentiated to ADIPs or B-ADIPs in thiamine-free medium (Th 0) or when thiamine was re-administered at gradually increasing concentrations (Figure 2A). The proportion of differentiated ADIPs was not affected by the availability of thiamine in the differentiation regimen (Figure 2B). The mRNA expression of both ThTrs was upregulated in direct proportion to increasing thiamine concentrations, where the presence of 1 μM or 5 μM thiamine resulted in a significant effect compared to Th 0 condition or to the 0.04 μM concentration, which is close to the physiological concentration of the vitamin found in human blood plasma (Figures 2C,D). A similar trend was observed when the protein expression of the ThTrs was investigated (Figure 2E and Supplementary Figure S1B). Similarly to our observations in ADIPs, thiamine potentiated the mRNA expression of both ThTrs in B-ADIPs, especially in DN-derived ones, in a concentration-dependent manner (Supplementary Figures S2A,B). In summary, extracellular thiamine levels during the differentiation of neck area-derived adipocytes positively associate with the expression of ThTrs, and adipocytes differentiated at regular culture conditions are fully equipped with ThTrs. The expression of SLC25A19 that encodes the mitochondrial TPP transporter was not significantly affected by the availability of thiamine at physiological (Supplementary Figure S2C) or at abundant levels (Supplementary Figure S2D) in the differentiation media of adipocytes.
Figure 2. Effect of gradually increasing (0.04 μM, 0.2 μM, 1 μM, 5 μM) concentrations of thiamine (Th) on the expression of Th transporters (ThTrs) in human subcutaneous (SC) and deep neck (DN)-derived adipocytes (ADIPs). SC or DN-derived preadipocytes were differentiated into ADIPs in Th-free medium (Th 0) or in the presence of gradually increasing concentrations of Th. (A) Schematic illustration of the differentiation protocol. (B) Quantification of differentiation ratio by laser-scanning cytometry, n = 3. (C,D) mRNA expression of SLC19A2 and SLC19A3 assessed by RT-qPCR, n = 3. (E) ThTr1 (SLC19A2) and ThTr2 (SLC19A3) protein expression detected by immunoblotting, n = 3. Uncropped images are shown in Supplementary Figure S1B. Statistical analysis was performed by one-way ANOVA, *#p < 0.05, **##p < 0.01, ***###p < 0.001, *comparing data at each concentration of Th to the lack of Th or # comparing the indicated groups.
Next, we studied the importance of thiamine availability in the thermogenesis of ADIPs which were differentiated either in Th 0 medium or in the presence of gradually increasing concentrations of thiamine (0.04 μM, 0.2 μM, 1 μM, 5 μM, or 25 μM) re-administered to the Th 0 medium. After recording basal OCR, dibutyryl-cAMP, a cell-permeable cAMP analog that imitates adrenergic stimulation of thermogenesis, was injected, which strongly increased mitochondrial respiration, as reported previously (18). Then proton-leak respiration, an indicator of UCP1-dependent thermogenesis, was measured by the administration of oligomycin, which inhibits ATP synthase. Basal OCR, the maximal respiration rate after the injection of the cAMP analog, and stimulated proton-leak OCR tended to elevate in proportion to the increasing thiamine concentrations within the differentiation media from 0.04 μM up to 25 μM in both SC and DN-derived ADIPs compared to the Th 0 condition. In the case of SC-derived ADIPs, statistically significant differences were observed from 0.2 μM thiamine availability (Figure 3A), while in DN-derived ADIPs, only the differences between 25 μM and 0.04 μM thiamine treatments or Th 0 controls were statistically significant, respectively (Figure 3B). The stimulated oligomycin-sensitive ATP-dependent OCR was not affected by the availability of thiamine in the adipogenic differentiation media of both SC and DN-derived ADIPs (Figures 3A,B). Of note, the extracellular flux assay was carried out in Th 0 medium to measure OCR with the previously obtained thiamine pool of the adipocytes which was gained during differentiation, therefore we could not assess ECAR in this experimental setup. However, the expression of SLC2A1 and SLC2A4 that encode GLUT1 and GLUT4 glucose transporters, respectively, was not significantly affected by the availability of thiamine at physiological levels in the differentiation media of adipocytes (Supplementary Figure S3).
Figure 3. Gradually increasing (0.04 μM, 0.2 μM, 1 μM, 5 μM, 25 μM) thiamine (Th) concentrations during differentiation increases oxygen consumption rate (OCR) and the expression of OXPHOS complex subunits of human subcutaneous (SC) and deep neck (DN)-derived adipocytes (ADIPs). (A) SC or (B) DN-derived preadipocytes were differentiated into ADIPs in Th-free medium (Th 0) or in the presence of gradually increasing concentrations of Th, then OCR was detected at basal conditions, following the injection of 500 μM dibutyryl-cAMP for 4 h, and after 2 μM oligomycin administration (stimulated FIGURE 3 (Continued)oligomycin-sensitive ATP-dependent and oligomycin-resistant proton-leak respiration). Each left panel shows representative curves of 3 measurements with independent donor samples. OCR at basal, maximal stimulation post cAMP injection, and after oligomycin administration (right panels) were quantified in ADIPs derived from three independent donors. (C) Total OXPHOS protein subunit expression detected by immunoblotting, n = 3. Statistical analysis was performed by one-way ANOVA, *#p < 0.05, **#p < 0.01, *comparing data at each concentration of thiamine to the lack of Th (Th 0) or # comparing the indicated groups.
The protein expression of mitochondrial complex subunits I, II, and III showed an increasing tendency in SC-derived ADIPs differentiated in the presence of gradually increasing concentrations of thiamine, compared to the Th 0 control. A statistically significant increase was observed in the case of 1 μM and 5 μM thiamine administration compared to 0.04 μM thiamine treatment or Th 0, respectively. The amount of complex subunits IV and V was not affected by thiamine availability up to 5 μM in SC-derived ADIPs. Only the expression of complex subunit II was influenced by the extracellular thiamine levels in DN-derived ADIPs, which already expressed these proteins at large amounts (Figure 3C). These results suggest that thiamine availability during adipocyte differentiation has a significant effect on the thermogenic competency of neck-derived ADIPs.
As we observed that increasing concentrations of thiamine in the adipogenic differentiation media positively associated with the mitochondrial respiration, especially with stimulated proton-leak OCR, of neck area-derived ADIPs, we also investigated how the availability of thiamine affects the expression of thermogenesis-related genes. UCP1 and PGC1A mRNA expression increased in direct proportion to the increasing thiamine concentrations in both SC and DN-derived ADIPs (Figures 4A,B), statistically significant effects were observed from 0.04 μM or 1 μM thiamine availability as compared to Th 0, respectively. In the case of B-ADIPs, the same trend was found, with statistically significant changes from 0.2 μM thiamine administration as compared to Th 0 with regard to both genes (Supplementary Figures S4A,B). In SC-derived ADIPs, UCP1 but not PGC1A protein expression was significantly affected by thiamine availability in a concentration-dependent manner (Figure 4C and Supplementary Figure S5A). In SC-derived B-ADIPs, DN-derived ADIPs, and DN-derived B-ADIPs, the expression of both investigated proteins was increased when the thiamine levels were consecutively raised during adipogenesis. In these cases, the effect of thiamine availability was statistically significant even at concentrations corresponding to physiological human blood plasma thiamine levels (Figure 4C and Supplementary Figures S4C, S5A).
Figure 4. Effect of gradually increasing (0.04 μM, 0.2 μM, 1 μM, 5 μM) concentrations of thiamine (Th) on thermogenic gene and protein expression in human subcutaneous (SC) and deep neck (DN)-derived adipocytes (ADIPs). ADIPs were differentiated and treated as in Figure 2. (A,B) mRNA expression of UCP1 and PGC1A assessed by RT-qPCR, n = 3. (C) UCP1 and PGC1A protein expression detected by immunoblotting, n = 3. Uncropped images are shown in Supplementary Figure S5A. Statistical analysis was performed by one-way ANOVA, *#p < 0.05, **#p < 0.01, *comparing data at each concentration of Th to the lack of Th (Th 0) or # comparing the indicated groups.
We also investigated the effect of thiamine availability on the mRNA expression of several thermogenesis-related genes and the white marker LEP in SC and DN-derived ADIPs (Figure 5) and B-ADIPs (Supplementary Figure S6). Increased amounts of extracellular thiamine upregulated the expression of DIO2 (32), TBX1 (14), CKMT2 (33), and CIDEA (34) in a concentration-dependent manner in ADIPs and B-ADIPs of both origins. CITED1 (13) was expressed at the highest level when both types of adipocytes were differentiated in the presence of 0.04 μM thiamine. In SC and DN-derived ADIPs, Th 0 condition and higher availability of thiamine tended to decrease CITED1 mRNA expression, while in the case of B-ADIPs, the beige marker gene was expressed significantly lower in these conditions. LEP mRNA expression showed a similar trend, however, we observed suppressive effects at statistically significant levels of higher doses of thiamine in DN ADIPs as well. We were unable to detect TMEM26 and TNFRSF9 (encoding CD137) expression, which were proposed as beige markers in mice, in the aforementioned samples (data not shown). Altogether, our data suggest that thiamine availability during adipocyte differentiation, even at concentrations found in human blood plasma, has a significant impact on the expression of thermogenesis-related genes.
Figure 5. Effect of gradually increasing concentrations of thiamine (Th) on thermogenic gene induction in human subcutaneous (SC) and deep neck (DN)-derived adipocytes (ADIPs). ADIPs were differentiated and treated as in Figures 2, 4. (A–F) mRNA expression of DIO2, TBX1, CKMT2, CIDEA, CITED1, and LEP assessed by RT-qPCR, n = 3. Statistical analysis was performed by one-way ANOVA, *#p < 0.05, **##p < 0.01, *comparing data at each concentration of Th to the lack of Th (Th 0) or # comparing the indicated groups.
Next, we investigated how the administration of abundant amounts of thiamine to the regular adipocyte differentiation medium (that already contained 8.2 μM of thiamine) affected the thermogenesis of SC and DN-derived ADIPs. SC-derived ADIPs differentiated in the presence of 25 μM excess thiamine had significantly higher basal OCR compared to those differentiated with regular differentiation medium (Figure 6A, left and middle panels). In the case of DN-derived ADIPs, a similar trend was observed without statistical significance (Figure 6B, left and middle panels). The presence of excess thiamine in the adipogenic differentiation cocktail resulted in higher maximal cAMP-stimulated respiration in ADIPs of both SC and DN origins. Both SC and DN-derived unstimulated ADIPs had higher proton-leak and ATP-dependent OCR when the hASCs were differentiated in the presence of excess thiamine. As expected, thermogenic stimulation with dibutyryl-cAMP elevated proton-leak and ATP-dependent respiration, especially in DN-derived ADIPs. In the case of stimulated ADIPs, the difference in proton-leak OCR caused by excess thiamine availability during differentiation was observed only in SC but not in DN-derived cells at a statistically significant level (Figures 6A,B, left and middle panels). DN-derived ADIPs differentiated in the presence of excess thiamine had higher stimulated ATP-dependent respiration compared to those ones that were differentiated with the regular medium (Figures 6A,B, left and middle panels). The ECARs were also elevated in response to stimulation in both SC and DN-derived ADIPs, however, were not affected by the abundant thiamine concentrations applied during adipogenesis (Figures 6A,B, right panels).
Figure 6. Excess thiamine provided at adipogenic differentiation increases oxygen consumption rate (OCR) and the expression of OXPHOS complex subunits of human subcutaneous (SC) and deep neck (DN)-derived adipocytes (ADIPs). (A) SC or (B) DN-derived preadipocytes were differentiated into ADIPs at regular culture conditions (8.2 μM thiamine, white bars) and in the presence of excess thiamine (25 μM, orange bars), then OCR was detected at basal conditions, following the injection of 500 μM dibutyryl-cAMP for 4 h, and after 2 μM oligomycin administration (oligomycin-sensitive ATP-dependent and oligomycin-resistant proton-leak respiration). Each left panel shows representative curves of 4 measurements with independent donor samples. OCR at basal, maximal stimulation post cAMP injection, and after oligomycin administration (middle panels), and extracellular acidification rate (ECAR) (right panels) were quantified in ADIPs derived from 4 independent donors. (C) Total OXPHOS protein subunit expression detected by immunoblotting, n = 4. Statistical analysis was performed by one-way ANOVA, *p < 0.05, **p < 0.01.
The protein expression of mitochondrial complex subunits I, II, and IV was significantly increased upon 25 μM or 50 μM of thiamine treatment in SC-derived differentiating ADIPs, while 50 μM thiamine administration resulted in higher complex I subunit amount in DN-derived ADIPs (Figure 6C). These results demonstrate that excess thiamine present in large amounts during adipocyte differentiation further increases the thermogenic competency and mitochondrial biogenesis of neck area-derived ADIPs.
After observing an elevation in the proton-leak respiration in the presence of excess thiamine, we hypothesized that abundant thiamine during adipocyte differentiation can further elevate the expression of thermogenic and mitochondrial biogenesis-related genes. We found that at mRNA levels, in the case of both UCP1 (Figure 7A) and PGC1A (Figure 7B), the presence of excess thiamine increased their expression compared to the control ADIPs or B-ADIPs, except for UCP1 in the case of DN-derived ADIPs in which the observed difference was not statistically significant. 50 μM of thiamine resulted in stronger effects in several cases in both ADIPs and B-ADIPs derived from both anatomical origins (Figures 7A,B). At protein levels, we observed that UCP1 expression was increased in the presence of excess thiamine (both 25 μM and 50 μM) compared to the controls, which was in association with the mRNA expression. These differences were statistically significant in the case of B-ADIPs. With regard to PGC1A, we observed a significant difference caused by the PPARγ-driven B-ADIP differentiation in SC-derived cells, and 50 μM abundant thiamine administration further increased the protein expression of the mitochondrial biogenesis master regulator in SC-derived B-ADIPs (Figure 7C and Supplementary Figure S5B).
Figure 7. Excess thiamine (Th) during adipogenic differentiation increases the expression of thermogenesis markers in human subcutaneous (SC) and deep neck (DN)-derived adipocytes (ADIPs) and brown differentiated adipocytes (B-ADIPs). ADIPs and B-ADIPs were differentiated and treated as in Figure 1. (A,B) mRNA expression of UCP1 and PGC1A assessed by RT-qPCR, n = 6. (C) UCP1 and PGC1A protein expression detected by immunoblotting, n = 4. Uncropped images are shown in Supplementary Figure S5B. Statistical analysis was performed by two-way ANOVA, *p < 0.05, **p < 0.01.
We also investigated the effect of excess thiamine administered to the differentiation cocktail on the mRNA levels of CKMT2, CIDEA, DIO2, TBX1, CITED1, and LEP and found that – with the exception of the white adipogenic marker, LEP – thiamine potentiated the expression of these genes in both SC and DN-derived B-ADIPs (Figure 8). In SC-derived ADIPs, the expression of CKMT2 and TBX1 was significantly increased when the differentiation was carried out in the presence of excess thiamine, while DIO2, TBX1, and CITED1 expression was upregulated in response to abundant thiamine levels in DN-derived ADIPs, respectively (Figure 8). These results suggest that excess thiamine provided during differentiation of neck area-derived adipocytes potentiates the expression of thermogenesis-related and brown/beige marker genes.
Figure 8. Excess thiamine (Th) during adipogenic differentiation elevates the expression of thermogenesis marker genes in human subcutaneous (SC) and deep neck (DN)-derived adipocytes (ADIPs) and brown differentiated adipocytes (B-ADIPs). ADIPs and B-ADIPs were differentiated and treated as in Figures 1, 7. (A–F) mRNA expression of CKMT2, CIDEA, DIO2, TBX1, CITED1, and LEP was assessed by RT-qPCR, n = 6. Statistical analysis was performed by two-way ANOVA, *p < 0.05, **p < 0.01.
To understand the different molecular mechanisms involved in the regulation of thermogenesis, Tóth et al. (17) previously studied differentiated adipocyte populations derived from the SVFs of paired DN and SC adipose tissue sections of nine independent donors and compared their global gene expression patterns using RNA-sequencing. Adipocytes were differentiated by using a standard adipogenic differentiation medium (ADIP) or long-term rosiglitazone treatment (B-ADIP), which enhanced the rate of adipocyte browning. Brown adipocyte content and browning capacity, which were estimated by BATLAS (35) and ProFAT (36) open access webtools, respectively, were higher in DN as compared to SC-derived adipocytes irrespective of the applied differentiation protocols (17). Among these DEGs, we found 21 SLC transporters, which mediate nutrient uptake and are known to play role in various biological processes. We have reported that pharmacological inhibition of ThTrs attenuated the efficiency of thermogenic activation in human neck area-derived adipocytes. On the other hand, abundant thiamine levels were required for the effective adrenergic-driven thermogenic stimulation of those cells (19). In this study, we addressed the question of how extracellular thiamine availability during differentiation affects the thermogenic capacity of human neck area-derived adipocytes.
Certain types of meals are rich in thiamine including brown rice, whole grains, legumes, and meats (37). The recommended daily allowance (RDA) of thiamine for adults is 1.2 mg/day for males and 1.1 mg/day for females (RDA increases for pregnant women) (38). Although thiamine is abundant in several food ingredients commonly consumed diets can be low in micronutrients. Thiamine deficiency, which mostly affects the nervous system, heart, and gastrointestinal tract, usually occurs when the RDA is not maintained. It can be developed because of impaired intestinal absorption or elevated excretion rates of thiamine caused by alcohol dependency or malnutrition (39, 40). The most common diseases resulting from thiamine deficiency are Beriberi disorder and Wernicke-Korsakoff syndrome (WKS) (41). The main symptoms of WKS are memory impairment, ataxia, and hypothermia, which is frequently reported as a secondary symptom. It has been reported that thiamine deficiency leads to the lesion of the hypothalamus, which is the main regulatory site of body temperature and appetite (42). Human case studies have reported that the hypothermic condition can be treated by thiamine administration for 2 days (43). Our presented data showed that the absence of thiamine during adipogenic differentiation led to a reduced thermogenic capacity of human neck area-derived adipocytes marked by lower cellular respiration, proton-leak respiration reflecting UCP1-dependent heat production, and expression of thermogenic markers. Gradually increasing concentrations of thiamine during differentiation elevated the thermogenic capacity and expression of thermogenic markers in a concentration-dependent manner suggesting the beneficial effect of thiamine in directly boosting the browning capacity of human neck area-derived adipocytes.
Thiamine deficiency is rarely found in developed countries, however, it has become common among people with alcoholism in Europe, North America, and Australia (44). Thiamine deficiency was also found in 15.5–29% of patients with obesity prior to bariatric surgery (45, 46). The complications of thiamine deficiency are also found in patients with DM contributing to hyperglycemia-induced cellular damage, endothelial dysfunction, and elevated oxidative stress (44). Reduced activity of erythrocyte transketolase was found in 17–79% of patients with DM (47, 48). Another study involving 120 adults with type 2 DM (out of which 46 individuals had microalbuminuria) reported that thiamine deficiency occurred in 98% and 100% of total involved subjects with or without microalbuminuria, respectively (49). Significantly lower levels of thiamine were also found in 76% or 75% of patients with type 1 or type 2 DM, respectively (50). Thiamine supplementation (100 mg, 3×100 mg daily which is approximately 100x higher than the RDA) for 6 weeks improved glucose tolerance in individuals with hyperglycemia (51). In drug-naïve patients with type 2 DM, a daily dose of 150 mg of thiamine for 1 month led to a significant decrease in plasma fasting glucose concentration (52). It has also been reported that thiamine supplementation may provide beneficial effects in patients with type 2 DM by improving lipid and creatinine profiles (53). Other risk factors of thiamine deficiency are immune diseases, hyperthyroidism, heart failure, and renal failure requiring dialysis (44).
Thiamine, which is converted to TPP, plays an important role in the proper function of mitochondrial enzyme complexes, including PDH. Tanaka et al. (54) reported that male Otsuka Long-Evans Tokushima-Fatty (OLETF) rats treated with water containing 0.2% of thiamine had higher hepatic PDH activity and decreased lipid oxidation. In addition, thiamine treatment prevented obesity and metabolic disorders in OLETF rats by decreasing the body weight and visceral WAT mass and alleviated hypertrophy of epididymal white adipocytes (54).
Hitherto, the effects of micronutrient availability on the differentiation of human adipocytes remain elusive. Hirata et al. (55) reported that thiamine promotes the differentiation of T lymphocytes via TGF-β superfamily production in thymic stromal cells suggesting the role of thiamine as a key nutritional regulator for immune cell development. A randomized controlled trial involving 100 individuals with obesity or overweight reported that a high protein diet combined with high thiamine administration (2.8 mg/day) led to reduced body weight up to 11 kg after 16 weeks and without affecting erythrocyte thiamine levels (56).
The concentration of thiamine in the regular cell culture medium is close to 10 μM, whereas thiamine concentration in human blood plasma is in the nM range. Our current data revealed that 25 μM thiamine addition to regular cell culture medium during the differentiation of the same adipocyte types elevated basal, maximal, and proton-leak respiration that reflects heat production. Our results also indicated that thiamine supplementation during adipogenesis augmented the expression of thermogenic markers in the thermogenically prone neck area-derived adipocytes ex vivo. Brown/beige adipocytes have been proposed as a potential target to treat obesity and DM, mainly due to their high capability as glucose and fatty acid sink (9, 57). Transplantation of hASCs, which are differentiated into brown/beige adipocytes, into recipient mice exerted beneficial effects, such as significant glucose consumption and increased energy expenditure (58). Despite the successful differentiation of hASCs into beige adipocytes, increasing the thermogenic capacity of these beige cells to be sufficient to drive heat production in vivo remains challenging. Our presented results showed that the addition of extracellular thiamine during the differentiation stage increased proton-leak respiration reflecting high heat production even at basal conditions, which may provide an approach to elevate the thermogenesis of beige adipocytes before transplanting them into the recipient. Therefore, our results highlight that extracellular thiamine availability should be optimized when thermogenic adipocytes are differentiated for transplantation approaches. On the other hand, constant supplementation with thiamine might open up a possibility for a safe and cost-effective nutritional approach for the prevention or treatment of obesity and related metabolic disorders. Although our data demonstrated the beneficial effects of thiamine in a primary human ex vivo adipocyte differentiation model, our study has indeed several limitations. Future studies involving in vivo experiments will be required to investigate the long-term effects of thiamine on adipocyte differentiation especially with respect to thermogenesis. More research is needed to explore whether thiamine affects the classical brown adipogenesis or the beigeing of WAT, therefore, the application of thiamine in anti-obesity therapy can be more accurate and efficient.
The original contributions presented in the study are included in the article/Supplementary material, further inquiries can be directed to the corresponding author.
The studies involving human participants were reviewed and approved by Medical Research Council of Hungary (20571-2/2017/EKU). The patients/participants provided their written informed consent to participate in this study.
BV and RA performed the experiments and wrote the original draft of the manuscript. EK, RA, and BV conceptualized the research, with inputs from LF. FG provided adipose tissue samples. ZB analyzed adipocyte differentiation rate. EK and LF supervised the research, acquired funding, and edited the final version of the manuscript. All authors contributed to the article and approved the submitted version.
This research was funded by the National Research, Development and Innovation Office (NKFIH-FK131424 and K129139) of Hungary. BV was supported by the ÚNKP-22-3-I New National Excellence Program of the Ministry for Culture and Innovation from the source of the National Research, Development and Innovation Fund.
We thank Éva Csősz for her exceptional help in reviewing the manuscript before its submission and Jennifer Nagy for technical assistance.
The authors declare that the research was conducted in the absence of any commercial or financial relationships that could be construed as a potential conflict of interest.
All claims expressed in this article are solely those of the authors and do not necessarily represent those of their affiliated organizations, or those of the publisher, the editors and the reviewers. Any product that may be evaluated in this article, or claim that may be made by its manufacturer, is not guaranteed or endorsed by the publisher.
The Supplementary material for this article can be found online at: https://www.frontiersin.org/articles/10.3389/fnut.2023.1207394/full#supplementary-material
1. Panuganti, KK, Nguyen, M, and Kshirsagar, RK. Obesity (2023). Treasure Island, FL, United States: StatPearls Publishing.
2. Safaei, M, Sundararajan, EA, Driss, M, Boulila, W, and Shapi'i, A. A systematic literature review on obesity: understanding the causes & consequences of obesity and reviewing various machine learning approaches used to predict obesity. Comput Biol Med. (2021) 136:104754. doi: 10.1016/j.compbiomed.2021.104754
3. World Health Organization . (2021). Obesity and overweight. Available at: www.who.int/health-topics/obesity.
4. Ikeda, K, Maretich, P, and Kajimura, S. The common and distinct features of brown and beige adipocytes. Trends Endocrinol Metab. (2018) 29:191–200. doi: 10.1016/j.tem.2018.01.001
5. Cohen, P, and Kajimura, S. The cellular and functional complexity of thermogenic fat. Nat Rev Mol Cell Biol. (2021) 22:393–409. doi: 10.1038/s41580-021-00350-0
6. Dragoo, JL, Shapiro, SA, Bradsell, H, and Frank, RM. The essential roles of human adipose tissue: metabolic, thermoregulatory, cellular, and paracrine effects. J Cartilage Joint Preserv. (2021) 1:100023. doi: 10.1016/j.jcjp.2021.100023
7. Cheng, L, Wang, J, Dai, H, Duan, Y, An, Y, Shi, L, et al. Brown and beige adipose tissue: a novel therapeutic strategy for obesity and type 2 diabetes mellitus. Adipocyte. (2021) 10:48–65. doi: 10.1080/21623945.2020.1870060
8. Wu, J, Cohen, P, and Spiegelman, BM. Adaptive thermogenesis in adipocytes: is beige the new brown? Genes Dev. (2013) 27:234–50. doi: 10.1101/gad.211649.112
9. Cypess, AM, Lehman, S, Williams, G, Tal, I, Rodman, D, Goldfine, AB, et al. Identification and importance of brown adipose tissue in adult humans. N Engl J Med. (2009) 360:1509–17. doi: 10.1056/NEJMoa0810780
10. Virtanen, KA, Lidell, ME, Orava, J, Heglind, M, Westergren, R, Niemi, T, et al. Functional brown adipose tissue in healthy adults. N Engl J Med. (2009) 360:1518–25. doi: 10.1056/NEJMoa0808949
11. Leitner, BP, Huang, S, Brychta, RJ, Duckworth, CJ, Baskin, AS, McGehee, S, et al. Mapping of human brown adipose tissue in lean and obese young men. Proc Natl Acad Sci U S A. (2017) 114:8649–54. doi: 10.1073/pnas.1705287114
12. Kajimura, S, Spiegelman, BM, and Seale, P. Brown and beige fat: physiological roles beyond heat generation. Cell Metab. (2015) 22:546–59. doi: 10.1016/j.cmet.2015.09.007
13. Sharp, LZ, Shinoda, K, Ohno, H, Scheel, DW, Tomoda, E, Ruiz, L, et al. Human BAT possesses molecular signatures that resemble beige/brite cells. PLoS One. (2012) 7:e49452. doi: 10.1371/journal.pone.0049452
14. Wu, J, Boström, P, Sparks, LM, Ye, L, Choi, JH, Giang, AH, et al. Beige adipocytes are a distinct type of thermogenic fat cell in mouse and human. Cell. (2012) 150:366–76. doi: 10.1016/j.cell.2012.05.016
15. van Marken Lichtenbelt, WD, and Schrauwen, P. Implications of nonshivering thermogenesis for energy balance regulation in humans. Am J Physiol Regul Integr Comp Physiol. (2011) 301:R285–96. doi: 10.1152/ajpregu.00652.2010
16. Trayhurn, P . Brown adipose tissue-a therapeutic target in obesity? Front Physiol. (2018) 9:1672. doi: 10.3389/fphys.2018.01672
17. Tóth, BB, Arianti, R, Shaw, A, Vámos, A, Veréb, Z, Póliska, S, et al. FTO intronic SNP strongly influences human neck adipocyte browning determined by tissue and PPARγ specific regulation: a transcriptome analysis. Cells. (2020) 9:987. doi: 10.3390/cells9040987
18. Arianti, R, Vinnai, BÁ, Tóth, BB, Shaw, A, Csősz, É, Vámos, A, et al. ASC-1 transporter-dependent amino acid uptake is required for the efficient thermogenic response of human adipocytes to adrenergic stimulation. FEBS Lett. (2021) 595:2085–98. doi: 10.1002/1873-3468.14155
19. Arianti, R, Vinnai, BÁ, Győry, F, Guba, A, Csősz, É, Kristóf, E, et al. Availability of abundant thiamine determines efficiency of thermogenic activation in human neck area derived adipocytes. J Nutr Biochem. (2023) 119:109385. doi: 10.1016/j.jnutbio.2023.109385
20. Sriram, K, Manzanares, W, and Joseph, K. Thiamine in nutrition therapy. Nutr Clin Pract. (2012) 27:41–50. doi: 10.1177/0884533611426149
22. Teran, MDM, de Moreno de LeBlanc, A, Savoy de Giori, G, and LeBlanc, JG. Thiamine-producing lactic acid bacteria and their potential use in the prevention of neurodegenerative diseases. Appl Microbiol Biotechnol. (2021) 105:2097–107. doi: 10.1007/s00253-021-11148-7
23. Whitfield, KC, Bourassa, MW, Adamolekun, B, Bergeron, G, Bettendorff, L, Brown, KH, et al. Thiamine deficiency disorders: diagnosis, prevalence, and a roadmap for global control programs. Ann N Y Acad Sci. (2018) 1430:3–43. doi: 10.1111/nyas.13919
24. Gangolf, M, Czerniecki, J, Radermecker, M, Detry, O, Nisolle, M, Jouan, C, et al. Thiamine status in humans and content of phosphorylated thiamine derivatives in biopsies and cultured cells. PLoS One. (2010) 5:e13616. doi: 10.1371/journal.pone.0013616
25. Kristóf, E, Klusóczki, Á, Veress, R, Shaw, A, Combi, ZS, Varga, K, et al. Interleukin-6 released from differentiating human beige adipocytes improves browning. Exp Cell Res. (2019) 377:47–55. doi: 10.1016/j.yexcr.2019.02.015
26. Kristóf, E, Doan-Xuan, QM, Bai, P, Bacso, Z, and Fésüs, L. Laser-scanning cytometry can quantify human adipocyte browning and proves effectiveness of irisin. Sci Rep. (2015) 5:12540. doi: 10.1038/srep12540
27. Elabd, C, Chiellini, C, Carmona, M, Galitzky, J, Cochet, O, Petersen, R, et al. Human multipotent adipose-derived stem cells differentiate into functional brown adipocytes. Stem Cells. (2009) 27:2753–60. doi: 10.1002/stem.200
28. Doan-Xuan, QM, Sarvari, AK, Fischer-Posovszky, P, Wabitsch, M, Balajthy, Z, Fesus, L, et al. High content analysis of differentiation and cell death in human adipocytes. Cytometry A. (2013) 83:933–43. doi: 10.1002/cyto.a.22333
29. Klusóczki, Á, Veréb, Z, Vámos, A, Fischer-Posovszky, P, Wabitsch, M, Bacso, Z, et al. Differentiating SGBS adipocytes respond to PPARγ stimulation, irisin and BMP7 by functional browning and beige characteristics. Sci Rep. (2019) 9:5823. doi: 10.1038/s41598-019-42256-0
30. Kristóf, E, Doan-Xuan, QM, Sárvári, AK, Klusóczki, Á, Fischer-Posovszky, P, Wabitsch, M, et al. Clozapine modifies the differentiation program of human adipocytes inducing browning. Transl Psychiatry. (2016) 6:e963. doi: 10.1038/tp.2016.230
31. Szatmári-Tóth, M, Shaw, A, Csomós, I, Mocsár, G, Fischer-Posovszky, P, Wabitsch, M, et al. Thermogenic activation downregulates high mitophagy rate in human masked and mature beige adipocytes. Int J Mol Sci. (2020) 21:6640. doi: 10.3390/ijms21186640
32. Cannon, B, and Nedergaard, J. Brown adipose tissue: function and physiological significance. Physiol Rev. (2004) 84:277–359. doi: 10.1152/physrev.00015.2003
33. Kazak, L, Chouchani, ET, Jedrychowski, MP, Erickson, BK, Shinoda, K, Cohen, P, et al. A creatine-driven substrate cycle enhances energy expenditure and thermogenesis in beige fat. Cell. (2015) 163:643–55. doi: 10.1016/j.cell.2015.09.035
34. Garcia, RA, Roemmich, JN, and Claycombe, KJ. Evaluation of markers of beige adipocytes in white adipose tissue of the mouse. Nutr Metab. (2016) 13:24. doi: 10.1186/s12986-016-0081-2
35. Perdikari, A, Leparc, GG, Balaz, M, Pires, ND, Lidell, ME, Sun, W, et al. BATLAS: deconvoluting brown adipose tissue. Cell Rep. (2018) 25:784–797.e4. doi: 10.1016/j.celrep.2018.09.044
36. Cheng, Y, Jiang, L, Keipert, S, Zhang, S, Hauser, A, Graf, E, et al. Prediction of adipose browning capacity by systematic integration of transcriptional profiles. Cell Rep. (2018) 23:3112–25. doi: 10.1016/j.celrep.2018.05.021
37. Sharma, S, Sheehy, T, and Kolonel, LN. Ethnic differences in grains consumption and their contribution to intake of B-vitamins: results of the multiethnic cohort study. Nutr J. (2013) 12:65. doi: 10.1186/1475-2891-12-65
38. Turck, D, Bresson, JL, Burlingame, B, Dean, T, Fairweather-Tait, S, Heinonen, M, et al. Dietary reference values for thiamin. EFSA J. (2016) 14:e04653. doi: 10.2903/j.efsa.2016.4653
39. Fouarge, E, and Maquet, P. Conséquences neurologiques centrales et périphériques de l’alcoolisme [Neurological consequences of alcoholism]. Rev Med Liege. (2019) 74:310–3.
40. Thomson, AD, Baker, H, and Leevy, CM. Patterns of 35S-thiamine hydrochloride absorption in the malnourished alcoholic patient. J Lab Clin Med. (1970) 76:34–45.
41. Mateos-Díaz, AM, Marcos, M, and Chamorro, AJ. Wernicke-Korsakoff syndrome and other diseases associated with thyamine deficiency. Síndrome de Wernicke-Korsakoff y otras patologías asociadas al déficit de tiamina. Med Clin. (2022) 158:431–6. doi: 10.1016/j.medcli.2021.11.015
42. Tanev, KS, Roether, M, and Yang, C. Alcohol dementia and thermal dysregulation: a case report and review of the literature. Am J Alzheimers Dis Other Dement. (2008) 23:563–70. doi: 10.1177/1533317508323479
43. Hansen, B, Larsson, C, Wirén, J, and Hallgren, J. Hypothermia and infection in Wernicke's encephalopathy. Acta Med Scand. (1984) 215:185–7. doi: 10.1111/j.0954-6820.1984.tb04991.x
44. DiNicolantonio, JJ, Liu, J, and O'Keefe, JH. Thiamine and cardiovascular disease: a literature review. Prog Cardiovasc Dis. (2018) 61:27–32. doi: 10.1016/j.pcad.2018.01.009
45. Carrodeguas, L, Kaidar-Person, O, Szomstein, S, Antozzi, P, and Rosenthal, R. Preoperative thiamine deficiency in obese population undergoing laparoscopic bariatric surgery. Surg Obes Relat Dis. (2005) 1:517–22. doi: 10.1016/j.soard.2005.08.003
46. Kerns, JC, Arundel, C, and Chawla, LS. Thiamin deficiency in people with obesity. Adv Nutr. (2015) 6:147–53. doi: 10.3945/an.114.007526
47. Jermendy, G . Evaluating thiamine deficiency in patients with diabetes. Diab Vasc Dis Res. (2006) 3:120–1. doi: 10.3132/dvdr.2006.014
48. Page, GL, Laight, D, and Cummings, MH. Thiamine deficiency in diabetes mellitus and the impact of thiamine replacement on glucose metabolism and vascular disease. Int J Clin Pract. (2011) 65:684–90. doi: 10.1111/j.1742-1241.2011.02680.x
49. Nix, WA, Zirwes, R, Bangert, V, Kaiser, RP, Schilling, M, Hostalek, U, et al. Vitamin B status in patients with type 2 diabetes mellitus with and without incipient nephropathy. Diabetes Res Clin Pract. (2015) 107:157–65. doi: 10.1016/j.diabres.2014.09.058
50. Thornalley, PJ, Babaei-Jadidi, R, Al Ali, H, Rabbani, N, Antonysunil, A, Larkin, J, et al. High prevalence of low plasma thiamine concentration in diabetes linked to a marker of vascular disease. Diabetologia. (2007) 50:2164–70. doi: 10.1007/s00125-007-0771-4
51. Alaei Shahmiri, F, Soares, MJ, Zhao, Y, and Sherriff, J. High-dose thiamine supplementation improves glucose tolerance in hyperglycemic individuals: a randomized, double-blind cross-over trial. Eur J Nutr. (2013) 52:1821–4. doi: 10.1007/s00394-013-0534-6
52. González-Ortiz, M, Martínez-Abundis, E, Robles-Cervantes, JA, Ramírez-Ramírez, V, and Ramos-Zavala, MG. Effect of thiamine administration on metabolic profile, cytokines and inflammatory markers in drug-naïve patients with type 2 diabetes. Eur J Nutr. (2011) 50:145–9. doi: 10.1007/s00394-010-0123-x
53. Al-Attas, O, Al-Daghri, N, Alokail, M, Abd-Alrahman, S, Vinodson, B, and Sabico, S. Metabolic benefits of six-month thiamine supplementation in patients with and without diabetes mellitus type 2. Clin Med Insights Endocrinol Diabetes. (2014) 7:1–6. doi: 10.4137/CMED.S13573
54. Tanaka, T, Kono, T, Terasaki, F, Yasui, K, Soyama, A, Otsuka, K, et al. Thiamine prevents obesity and obesity-associated metabolic disorders in OLETF rats. J Nutr Sci Vitaminol. (2010) 56:335–46. doi: 10.3177/jnsv.56.335
55. Hirata, SI, Sawane, K, Adachi, J, Isoyama, J, Sugiura, Y, Matsunaga, A, et al. Vitamin B1 supports the differentiation of T cells through TGF-β superfamily production in thymic stromal cells. iScience. (2020) 23:101426. doi: 10.1016/j.isci.2020.101426
56. Keogh, JB, Cleanthous, X, Wycherley, TP, Brinkworth, GD, Noakes, M, and Clifton, PM. Increased thiamine intake may be required to maintain thiamine status during weight loss in patients with type 2 diabetes. Diabetes Res Clin Pract. (2012) 98:e40–2. doi: 10.1016/j.diabres.2012.09.032
57. Wu, Q, Kazantzis, M, Doege, H, Ortegon, AM, Tsang, B, Falcon, A, et al. Fatty acid transport protein 1 is required for nonshivering thermogenesis in brown adipose tissue. Diabetes. (2006) 55:3229–37. doi: 10.2337/db06-0749
Keywords: obesity, adipocytes, beige, thermogenesis, thiamine, UCP1
Citation: Vinnai BÁ, Arianti R, Győry F, Bacso Z, Fésüs L and Kristóf E (2023) Extracellular thiamine concentration influences thermogenic competency of differentiating neck area-derived human adipocytes. Front. Nutr. 10:1207394. doi: 10.3389/fnut.2023.1207394
Received: 17 April 2023; Accepted: 14 August 2023;
Published: 13 September 2023.
Edited by:
Ellen E. Blaak, Maastricht University, NetherlandsReviewed by:
Marie Sophie Isidor, University of Massachusetts Medical School, United StatesCopyright © 2023 Vinnai, Arianti, Győry, Bacso, Fésüs and Kristóf. This is an open-access article distributed under the terms of the Creative Commons Attribution License (CC BY). The use, distribution or reproduction in other forums is permitted, provided the original author(s) and the copyright owner(s) are credited and that the original publication in this journal is cited, in accordance with accepted academic practice. No use, distribution or reproduction is permitted which does not comply with these terms.
*Correspondence: Endre Kristóf, a3Jpc3RvZi5lbmRyZUBtZWQudW5pZGViLmh1
†These authors have contributed equally to this work and share first authorship
Disclaimer: All claims expressed in this article are solely those of the authors and do not necessarily represent those of their affiliated organizations, or those of the publisher, the editors and the reviewers. Any product that may be evaluated in this article or claim that may be made by its manufacturer is not guaranteed or endorsed by the publisher.
Research integrity at Frontiers
Learn more about the work of our research integrity team to safeguard the quality of each article we publish.