- 1Academician Workstation, Changsha Medical University, Changsha, China
- 2Xiangya School of Pharmaceutical Sciences, Central South University, Changsha, Hunan, China
Natural ingredients have many applications in modern medicine and pharmaceutical projects. However, they often have low solubility, poor chemical stability, and low bioavailability in vivo. Spray drying technology can overcome these challenges by enhancing the properties of natural ingredients. Moreover, drug delivery systems can be flexibly designed to optimize the performance of natural ingredients. Among the various drug delivery systems, dry powder inhalation (DPI) has attracted much attention in pharmaceutical research. Therefore, this review will focus on the spray drying of natural ingredients for DPI and discuss their synthesis and application.
1. Introduction
Nowadays, natural ingredients are becoming more and more important in medical research because they have a wide range of clinical benefits. They have rich pharmacological effects, such as anti-cancer, anti-oxidation, lowering blood lipid, inducing autophagy and reducing the side effects of drug therapy (1–4). However, at present, natural ingredients have many shortcomings, such as chemical instability, low solubility and low system bioavailability, which need to be solved by various technical means in order to give full play to their potential in modern medicine (5, 6).
In order to solve these problems, researchers have developed various methods and found that spray drying technology may be a potential solution. Spray drying technology can accurately control the particle size of drugs, improve the dispersion of drugs in the lung, and reduce drug deposition, thus achieving better therapeutic effect. Spray drying technology can transform crystalline drug into an amorphous solid dispersion, which shows a more disordered molecular structure and is more soluble. Amorphous drug has a high energy state, compared with crystalline drug, its saturated solubility and dissolution rate are significantly improved. Spray drying technology can produce dry powder pharmaceutical preparations with good stability. Due to the presence of polymer, amorphous drug can be effectively stabilized, preventing it from crystallizing or degrading during storage. Because of its unique properties, spray-drying is widely used in pharmaceutical preparations. It can accurately control the particle size of drugs, improve the dispersion of drugs in the lung, and reduce drug deposition, so as to achieve better therapeutic effect (7–10). The principle of spray drying is shown in Figure 1. Meanwhile, spray drying technology can significantly improve the solubility, permeability and stability of natural products. For example, the microencapsulation system prepared by spray drying improves the in vitro dissolution and permeation rates of silymarin, enhancing its bioactivity after oral administration. This system effectively preserves the antioxidant activity of silymarin and does not compromise the anti-inflammatory properties of the original extract. It seems that the anthocyanin polyphenols treated by spray drying technology have a higher retention rate and their color characteristics are also maintained. At the same time, it protects phenolic acids and flavonoids from thermal degradation (11, 12). The microencapsulated components of epicatechin and glycosylated quercetin have been proved to protect phenolic acids and flavonoids from thermal degradation in an environment simulating gastrointestinal conditions (13), and the microencapsulated components of epicatechin and glycosylated quercetin remain amorphous after long-term storage, which ensures their long-term solubility and bioavailability in vitro (14). These findings indicate that the use of spray drying techniques can effectively improve the physical properties of natural ingredients and be further applied to clinical practice for the preparation of various formulations, including the development and application of DPI.
The pulmonary drug delivery route provides a direct and effective way to deliver the drug to the systemic circulation, which has many advantages: (1) It can deliver the drug directly to the systemic circulation without passing through the liver, thus avoiding the first-pass effect and improving bioavailability (15); (2) It can take advantage of the anatomical and physiological characteristics of the lung, including large surface area, abundant capillaries and relatively few metabolic reactions, to provide an ideal place for drug transport (Figure 2) (16, 17); (3) It can design suitable DPI pulmonary drug delivery systems according to the drug’s absorption, distribution, metabolism, excretion and toxicity characteristics (ADMET) (18–21); (4) It can target pulmonary diseases, such as asthma, chronic obstructive pulmonary disease (COPD), pulmonary fibrosis and COVID-19, and deliver the drug directly to the lung tissue, achieving local effective results; (5) It can significantly reduce the dosage of the drug and reduce the adverse reactions caused by drug distribution in other parts, compared with other delivery methods (22–26); (6) It can also be used for the treatment and prevention of other systemic diseases, such as diabetes, cancer, osteoporosis and so on. Therefore, for some special pulmonary diseases, the advantages of pulmonary drug delivery are irreplaceable. At the same time, the dry powder inhalation pulmonary drug delivery method has unique advantages in the treatment and prevention of other systemic diseases.
Combined with the characteristics of natural ingredients, this paper mainly introduces the application of spray drying technology in DPIs. Spray drying technology can improve the bioavailability, stability, and solubility of drugs (10, 11, 27–29). Spray drying technology has a broad prospect in the production of dry powder inhalers for natural medicine. Spray drying can not only alleviate the problem of natural ingredients but also greatly improve their efficacy and value. In summary, this paper aims to introduce spray-drying natural ingredients for DPIs and recent research.
2. Composition of the DPIs of natural ingredient
2.1. Composition of natural component DPI
Dry powder inhalation consists of natural ingredients (such as lactose, starch, and cellulose) and natural extracts with therapeutic properties. Its composition may vary depending on the formula of the drugs and the delivery device. Generally, natural ingredients play an important role in the safety and effectiveness of DPI as a pulmonary drug delivery method. The drugs containing natural ingredients differ according to the treatment purpose (30–33).
Natural components are an important part of drug research and development. Since 1981, about half of the small molecule drugs approved by the US Food and Drug Administration (FDA) have come from natural sources. With the progress of science and technology, researchers pay more and more attention to the pharmacological activities of trace, insoluble, and unstable components in natural products, which used to be difficult to obtain. The advances in infrared (IR), ultraviolet-visible (UV), mass spectrometry (MS), and nuclear magnetic resonance (NMR) spectroscopy can determine the structure of active substances in natural components and promote the study of their pharmacological activities. In addition, various chromatographic separation techniques have been used to extract and purify natural components, thus allowing the mass production of trace amounts of these substances and developing them into effective treatment methods for diseases. Natural ingredients are often used in anti-cancer, anti-oxidation, anti-inflammatory, and antibacterial therapy, making their pharmacological activities an indispensable part of modern medicine. Some common natural chemicals are listed in Table 1.
In addition, the research progress of some common pharmacological actions of dry powder inhalers are also listed.
2.1.1. Antitumor effect
In the field of tumor research, natural products and their derivatives play an important role in the research and development of small-molecule anticancer drugs. From the 1940s to the present, 48.6% of 175 small-molecule drugs with anticancer activity were directly derived from natural products (56). Examples of such drugs include vinca alkaloids and taxanes, which target microtubules, inhibit mitosis, lead to cell cycle arrest and tumor cell death, and finally achieve anti-cancer effects. A lot of research shows that the natural ingredients in DPI can effectively treat various kinds of cancer. For example, Adel designed curcumin proliposomes by nano-spray-drying, which improved the delivery concentration of curcumin in lung tissue and overcame its shortcomings such as poor water solubility and low oral bioavailability. Curcumin proliposomes effectively inhibited the growth of lung tumor cells, including 549 cells, and showed a better anticancer effect than oral drugs (57).
2.1.2. Anti-inflammatory effect
Many natural compounds, such as oridonin (58), verbascoside (59), and quercetin (60), have anti-inflammatory activities. For example, resveratrol can regulate inflammatory reactions by inhibiting the synthesis and release of pro-inflammatory mediators, thus showing good anti-inflammatory activity. However, the low bioavailability of resveratrol restricts its clinical application. To solve this problem, researchers developed resveratrol DPI. By spray-drying resveratrol particles to make their diameter less than 5 microns, they can enhance their transport across cell membranes and improve their ability to reach target organs, thus producing more effective anti-inflammatory effects (61, 62).
2.1.3. Antioxidant effect
Resveratrol (63), carotenoids (64), and ginkgolide (65) are the antioxidant substances commonly found in natural active extracts, which can inhibit or prevent the initiation and diffusion of free radical chain reactions, reduce the concentration of free radicals, and slow down cellular aging. A study showed that spray-dried resveratrol has the same antioxidant properties as vitamin C (62). Meanwhile, fluoxetine, a natural antioxidant found in flavonoid plant polyphenols (66), also possesses antioxidant activity. In addition, Mohtar et al. prepared a complex of fluoxetine and cyclodextrin for dry powder inhalation and compared it with ascorbic acid. They found that the IC50 values for free fluoxetine and fluoxetine-cyclodextrin complex solutions were significantly lower (p 0.05) (67), indicating that fluoxetine has excellent antioxidant activity for dry powder inhalation.
2.1.4. Antibacterial effect
From 1981 to 2019, about 50% of all drugs approved by the FDA originated from natural products, and many of them had natural antibacterial functions (68), such as baicalin (69), berberine (70), and evodiamine (71). Besides, research also proved that inhaling andrographolide (AG) dry powder is effective in reducing pro-inflammatory cytokines, regulating the immune response, and downregulating inflammation. AG also showed antibacterial activity and was found to be 10 times more effective than penicillin in the treatment of S. aureus pneumonia. Therefore, it has the potential for pulmonary administration in the treatment of bacterial pneumonia.
2.2. Carriers and additives
Dry powder inhalation drugs are micronized, so they need to add carriers and additives to improve their fluidity and dispersibility (72–75), and lactose is the most commonly used carrier, as well as the only DPI carrier approved by the FDA (76), but it also has drawbacks and limitations, such as not beingsuitable for diabetic patients and binding too tightly with some drugs, affecting release (77–79). Therefore, some studies have explored other alternative carriers, such as mannitol and erythritol (80–83). The functions of carriers and additives are diverse, including increasing stability, enhancing absorption, improving the respiratory tract environment, etc. Table 2 lists some common functions.
3. Spray-dried DPI
Spray drying is used to atomize liquid materials (e.g., solutions, suspensions, emulsions, etc.). By atomizing the liquid into fine particles and then contacting them with a stream of hot air, the liquid is rapidly evaporated to form a dry powder or granule. The process of spray drying usually includes spraying, drying, powder separation, and collection steps. After spraying, the liquid particles enter the preheating drying chamber. In the high-temperature hot air flow, the water quickly evaporates and the particles gradually decrease, reaching a dry state. The temperature and humidity of the drying chamber can be adjusted according to the characteristics of the material and the target powder (98).
Spray drying technology has been used in traditional pharmaceutical industries for a long time to manufacture pharmaceutical products with specific physical and chemical properties. The product is composed of porous, uniform particles (99) with tiny and precise sizes, which is beneficial to the quality control of DPI. In spray drying, the final state of the product can be adjusted by adjusting various parameters of spray drying, which have the greatest impact (100–102): (1) Solvent selection: The choice of solvent has a significant impact on the change in solubility and bioavailability. The appropriate solvent can fully dissolve the target natural extract and obtain a higher proportion of amorphous products. (2) Nozzle diameter selection: nozzle diameter is positively correlated with product diameter; (3) Solid content of feed liquid: It affects the viscosity, surface tension, and drying rate of feed, as well as the yield, particle size, and morphology of powder. Generally speaking, higher solid content leads to larger, more spherical particles and a higher yield. (4) Inlet air temperature and outlet air temperature refer mainly to the temperature of the drying chamber. Generally speaking, it affects the drying rate and efficiency. Higher inlet air temperatures lead to a faster drying rate and shorter residence time, but at the same time, they increase the risk of thermal degradation and the loss of volatile components. Therefore, for target natural components that are easily decomposed or thermally degraded, their inlet air temperature should be strictly controlled or freeze spray drying should be used; outlet air temperature generally refers to the temperature of the dry gas leaving the drying chamber, which reflects the heat and moisture transferred from feed to air during the drying process. It affects the product’s moisture content and stability. Generally speaking, lower temperatures mean significant heat exchange between gas and liquid, low humidity content, and high stability; when target natural extracts are extremely sensitive to high temperatures or humid heat, freeze spray drying can be used instead of conventional spray drying (103, 104). The drying process of this method includes three main steps: droplet formation, freezing, and sublimation drying. The drug and dispersion medium are rapidly cooled and distributed in frozen droplets, then sublimated to form amorphous, porous particles (105–108). (5) Peristaltic pump speed: Also known as feed rate, it is the rate at which feed solution or suspension is delivered to the atomizer. It affects droplet size distribution, drying rate, and product residence time. Generally speaking, a higher feed rate leads to larger, more uneven droplets, a slower drying rate, and a longer residence time, which may affect product quality and yield. (6) Atomization pressure: the pressure used to force feed through the atomizer nozzle. This pressure comes from various sources, such as high-pressure gas, centrifugal force, etc., and directly affects droplet size distribution and atomization efficiency, thus affecting product particle size distribution. Generally speaking, higher atomization pressure leads to smaller, more uniform droplets, resulting in a more uniform particle size distribution with a smaller average particle size.
For natural product DPI preparation, according to its instability, low solubility, and bioavailability characteristics, reasonable adjustment of the above parameters can obtain the best overall performance products.
3.1. Types of DPI powders
Depending on the characteristics of the active pharmaceutical ingredients (API) and the demand for the formulation, we can adapt the spray drying process to prepare granular products in different forms and states, such as common nanoparticles, microspheres, liposomes, and porous nanoparticles (109–111). The different forms of particulate products offer different advantages and have been tailored to the different clinical needs of the products; for example, nanoparticles allow targeted delivery of drugs independent of the physiological environment and facilitate transport across biological barriers (112); microspheres are used to reduce the adhesion of drug carriers, which greatly increases the fraction of fine particles (FPF) (113); and liposomes are used to avoid immune rejection, select drug localization in the lungs, and reduce local or systemic toxicity (114). Three widely used dosage forms are discussed below.
3.1.1. Nanoparticle
Nanoparticle DPIs are made by mixing drug-loaded nanoparticles with a suitable matrix carrier and using spray drying technology to produce inhalable particles. The use of nanospray dryers allows for the production of particles with a more uniform size and a smaller particle size, thus further improving the bioavailability of inhaled powdered drugs. It has been shown that preparing curcumin-loaded casein nanoparticles by spray drying sodium casein (NaCas) and curcumin in an ethanol solution improves the dispersibility of the drug. Curcumin encapsulated in casein nanoparticles has higher bioactivity than pristine curcumin, as assessed by antioxidant and cell proliferation assays (115). The nanoparticles also have good dispersion properties, lung deposition properties, and both slow and controlled release (116). Dimer et al. prepared resveratrol by piezoelectric atomization technique and nanospray drying. They obtained spherical particles with irregular surfaces, low water content (less than 2.0%), low density, high mobility, and good dispersion. This nanoparticle drug deposition is suitable for the bronchial and alveolar regions of the targeted lung, achieving better therapeutic effects (117). Moreover, nanoparticles can be surface-modified so that they are not cleared by macrophages in vivo. This prolongs the contact time of systemic circulation, enhances the therapeutic effect of the drug, and extends the retention time of the drug in the body, thus prolonging the therapeutic effect (118). In addition to this, studies have also been conducted to achieve sustained drug release by preparing nanoparticles as polymers and using the solubilization and adhesion properties of polymers (119).
3.1.2. Microspheres and microcapsule
Microspheres, or microcapsules, are skeletal microspheres formed by dissolving or dispersing a drug in a polymeric material. They both refer to particle dispersion systems formed by dispersing or adsorbing a drug in a polymer. Microspheres are characterized by their large particle size and various morphologies, such as spherical, oval, and rod-shaped. Due to the large specific surface area and pore structure of microspheres, drugs can be embedded and adsorbed inside them, achieving sustained drug release through slow release (120). When manufacturing dosage forms using spray drying technology, drugs are dissolved in an organic solvent to form an emulsion, which is then spray-dried using a spray dryer to obtain microspheres or microcapsules with uniform particle size. Encapsulation of drugs does not lead to loss of activity or denaturation of biologically active substances but rather improves their stability and gives them a sustained release effect. Jovanovic et al. (121) obtained microspheres with higher thermal stability and a higher diffusion coefficient by spray drying. Desai et al. (122) used spray drying to prepare vitamin C-coated chitosan microspheres cross-linked with tripolyphosphate (TPP). Follow-up studies confirmed that vitamin C release from TPP-chitosan microspheres was sustained and that vitamin C was more stable than pure vitamin C. One study prepared anthocyanin-rich microcapsules by spray-freeze-drying a complex condensed double emulsion. The microencapsulation efficiency (MEE) of the microcapsules changed from 84.9 to 94.7%, with higher total anthocyanin and total phenol content. Their sustained release properties were found by testing their in vitro release characteristics, reflecting their good performance in applications. They can provide a good clinical solution for some drugs that have a short half-life in circulation and are easily degraded (123).
3.1.3. Liposome
Liposomes are closed vesicle materials similar to the biological membrane structure formed by encapsulating active substances using liposome technology with a phospholipid bilayer biological membrane structure. Liposomes are composed of amphiphilic molecules, and both water-soluble and fat-soluble drugs can be encapsulated. The fat-soluble drugs are positioned between the bilayer lipid membranes, and the water-soluble drugs are wrapped in the water phase (124). Liposomes can improve the stability of drugs. Because drugs are wrapped in liposomes before entering the target area, they are not decomposed by body enzymes and other factors (111). Curcumin precursor liposomes prepared in a research study using a spray dryer showed enhanced growth inhibition of lung tumor cells A549 and a significant reduction in associated cytokines such as tumor necrosis factor-, interleukin-6, and interleukin-10 compared to the pure drug. Results from pulmonary pharmacokinetic assays confirmed the superiority of curcumin precursor liposomes over curcumin powder in terms of the rate and extent of lung tissue uptake and the mean retention time in lung tissue (5). In contrast, Xu et al. prepared a spray-dried powder of vincristine (VCR) liposomes, and the aerosol had better pharmacokinetic behavior compared to free VCR: Compared to free VCR, its maximum concentration and systemic exposure, respectively, increased by 630.8 and 429.6%, and its elimination half-life was shortened by 81.1%, while the clearance rate was reduced by 83.2%, demonstrating the excellent facilitation of drug bioavailability by spray-dried DPIs (125).
4. DPI principles and advantages
4.1. Principles of DPI
Dry powder inhalers are popular because they offer several advantages over other types of inhalers, such as metered dose inhalers (MDIs). The following describes the operation of dry powder inhalers. Dry powder inhalers can be divided into the following types, depending on the form of the drug and how it is released: (1) Metered-dose dry powder inhalers: These inhalers release a certain amount of drug each time and usually require pressing or twisting the device to activate the drug. Examples include Advair Diskus, Flovent Diskus, and Serevent Diskus; (2) Breath-activated dry powder inhalers: These inhalers release the drug by the user’s breathing force, without the need to press or twist the device. Examples include Symbicort Turbuhaler, Asmanex Twisthaler, and Pulmicort Flexhaler. However, note that this type of inhaler may not be suitable for patients with respiratory dysfunction; (3) Single-dose dry powder inhalers: These inhalers can only be loaded with one dose of medication at a time and require the insertion of a capsule or disc before each use. Examples include Spiriva HandiHaler and Foradil Aerolizer.
All three types of inhalers share a common principle. First, the drug formulation: DPIs use a dry powder formulation containing the active drug. The drug is usually micronized to ensure that the particles are small enough to penetrate the lungs. Then, respiratory drive design: DPIs are usually respiratory drive devices. Drug storage and dose preparation: The DPIs have a drug reservoir containing multiple doses of drug. Each dose is then individually packaged or prepared in the device to ensure proper dosing. Some DPIs use capsules, while others use pre-packaged blisters or spacers that the patient inhales at the same time as the drug is released for therapeutic purposes.
Different types of dry powder inhalers have different advantages and disadvantages and are used in different ways. The researcher or patient may choose the appropriate type of dry powder inhaler based on clinical facts or personal preference.
4.2. Advantages over other traditional delivery methods
The advantages of dry powder inhalers over traditional methods of drug delivery, such as oral administration and injections, are obvious, especially for specific drugs, diseases, and patients. For example (126–131):
(1) Direct action on the lesion: dry powder inhalers can act directly on the lungs through the respiratory tract, delivering the drug directly to the lesion, reducing the metabolism and side effects of the drug in the body.
(2) Rapid onset of action: dry powder inhalers can be quickly absorbed into the alveoli, and then quickly enter the blood circulation, so that the drug effect works quickly.
(3) High patient compliance: especially for some patients with swallowing difficulties, such as the elderly, children, and patients with digestive tract diseases, or some drugs containing bitter or offensive tastes.
(4) Small amount of drug use: Compared to oral drugs, dry powder inhalers require a small amount of drug use, which can reduce the accumulation of drugs in the body and reduce the occurrence of toxic side effects of drugs.
(5) Convenient and easy to use: dry powder inhalers can be used at any time and any place, easy to use and not disturbed by the external environment, especially suitable for long-term treatment of patients with chronic diseases.
(6) Avoid liver first pass effect and enterohepatic circulation, increasing the bioavailability of the drug.
(7) Environmentally friendly and pollution-free: dry powder inhalers do not require solvents or gases as carriers and do not produce waste or harmful substances in the process of use, which is less polluting to the environment and safer and more environmentally friendly than other drug delivery methods.
4.3. Advantages relative to other pulmonary drug delivery methods
And the advantages over other methods of lung drug delivery are also very obvious. DPIs are one of the four main devices used to deliver pharmaceutical preparations to the lungs, along with pressurized metered dose inhalers (PMDIs), nebulizers and soft mist inhalers (SMIs) (23, 132). DPIs also offer several advantages over the other three liquid drug delivery devices:
4.3.1. Environment-friendly
Pressurized metered dose inhalers were once the most widely used inhalers, but they use chlorofluorocarbon (CFC) propellant in the atomization process to deliver to lung tissue, which can lead to depletion of the ozone layer. Dry powder inhalers, on the other hand, are administered as a dry powder and do not require propellant to deliver the drug into the lungs. This makes dry powder inhalers more environmentally friendly and their reusable nature offers significant advantages in terms of resources and energy consumption.
4.3.2. Availability
Nebulizers in in-hospital formulations are compressor driven, which is heavy, bulky, noisy and has limited portability compared to dry powder inhalers (81, 133). On the other hand, dry powder inhalers are portable, do not require an external power source or propellant and can be carried around with the medication according to the patient’s needs, making them more convenient for the patient to use (134, 135).
5. Application and development of natural extracts DPI
Studies have shown that spray drying can significantly improve the efficiency of drug absorption in the lung, improve drug distribution, avoid drug deposition, increase its bioavailability, and allow natural products to exert their target clinical effects (136, 137). For example, in amorphous formulations obtained by spray drying ferulic acid with 10% or 20% PVP-K30, PEG 6000, or Poloxamer-188, free radical scavenging assays confirmed complete antioxidant activity and increased antiplatelet effects.
According to BBC Research, the global pulmonary drug delivery systems market totaled USD 38.1 billion in 2017 and is expected to reach USD 47 billion by 2022, growing at a compound annual growth rate (CAGR) of 4.3% during 2017–2022. The huge market demand is aiding the research on spray drying of natural ingredients for dry powder inhalation. The application of dry powder inhalation has been widely used in formulations for the continuous delivery of atomized particles. Spray drying technology allows the production of free-flowing powders of various sizes, offering unlimited possibilities for the production of dry powder for inhalation.
5.1. Improving the target organ concentration of drugs
Dry powder inhalers exhibit good aerosol properties and can effectively deliver the drug to the lungs and obtain high pulmonary drug concentrations. Hu et al. (138) found that the production of curcumin-DPI by wet milling combined with spray drying resulted in significantly higher plasma curcumin concentrations after inhalation, and most of the curcumin-DPI was deposited in the lungs with high target organ drug concentrations and good efficacy. In addition, Meenach et al. (139), by spray drying an organic solution composed of paclitaxel (PTX) liposomes, found that DPIs with a product median diameter (MMAD) between 1.9 and 2.3 μm, good aerosol dispersion, and a high deposition rate in the lungs were effective in improving the therapeutic effect and achieving better therapeutic goals. DPIs not only increase the local concentration of drugs and achieve targeted distribution of drugs, but also reduce the distribution of drugs in other organs or tissues, reduce or avoid the adverse effects of certain natural ingredients in non-targeted sites, and improve the effectiveness and safety of natural active target substances.
5.2. Improving bioavailability
Since the bioavailability of most natural ingredients is low, an important application of DPI is to improve this problem. DPI is administered directly through the lungs without passing through the enterohepatic circulation, avoiding the first-pass effect and allowing increased entry into the systemic circulation, thus improving bioavailability. Lu et al. (140) prepared salvianolic acid (SMPA) by spray drying and found that, compared to oral administration, pulmonary administration of salvianolic acid B (Sal B) had a bioavailability of up to 19.15%. This strongly suggests that target natural bioactive ingredients with low bioavailability can be effectively applied by spray-drying DPI, which is important for some active ingredients with poor effects in animal experiments but significant pharmacological effects in cellular experiments, such as plant extracts with short half-lives, easy metabolism, and low solubility, which may bring new opportunities for these drugs that would be prematurely eliminated because of these drawbacks.
5.3. Improved stability
In dry powder inhalers, the drug is stored as a dry solid powder; the drug is less susceptible to environmental disturbances during production, transport, and storage; the molecular activity is low; the drug is protected from oxidation and decomposition; and its physical and chemical stability is improved. In addition, stabilizers are usually introduced in the formulation design to ensure that the active ingredients of the drug are not destroyed. One study (112) found that formulations of budesonide (BUD) and colchicine (COL) liposomal DPI were stable for 6 months at 25 ± 2°C, 60% RH, and refrigerated conditions (2–8°C) compared to conventional formulations. The stability of the formulations was greatly improved, and the stability of the active ingredients was effectively ensured. Similarly, DPI is a better choice for natural active ingredients with poor stability.
Dry powder inhalers for inhalation of various natural ingredients are described in Table 3.
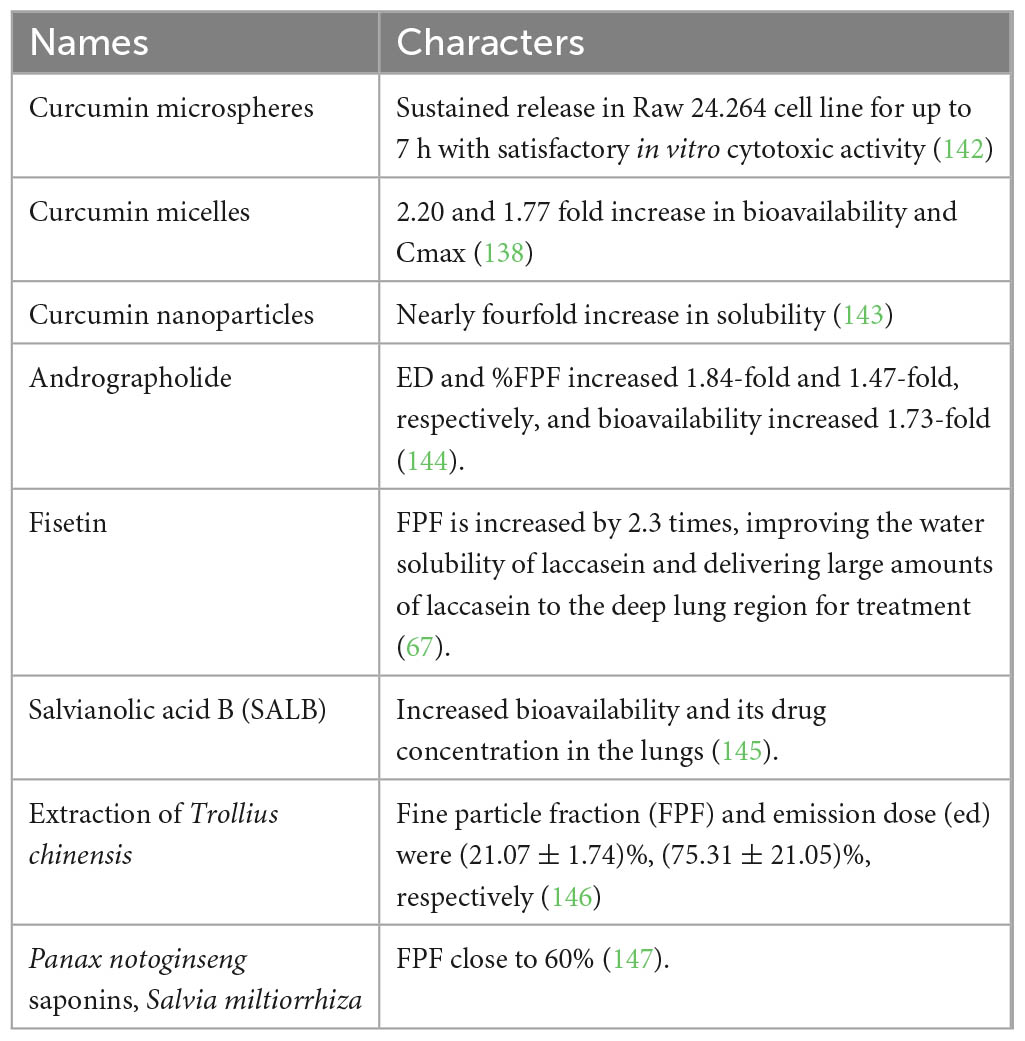
Table 3. Dry powder inhalers with natural ingredients (141).
6. Conclusion and perspective
In contemporary drug formulation design, it is very important to find the appropriate active ingredients, dosing route, and production process for the reasonable application of natural foods with medicinal value. Many natural resources are of rich medicinal value, and natural ingredients are often used as the main raw materials for drugs. However, their unstable chemical properties, low solubility, and low systematic bioavailability often hinder their development and application. Therefore, there is an urgent need to find suitable preparation methods and application means. In this paper, the principle and synthesis of spray drying technology in the preparation of dry powder inhalers of natural products are reviewed, and some successful applications are analyzed. In a word, the use of spray drying technology to produce drugs containing natural ingredients has great prospects and provides the possibility for more drug applications of natural products. In the future, with the increasing incidence of lung diseases such as lung cancer, pneumonia, chronic obstructive pulmonary disease, and pulmonary fibrosis, preparations for pulmonary administration, especially dry powder inhalers, will have great research significance and market prospects. Especially with natural active products, their reasonable and scientific use is also a major breakthrough in pharmaceutical research. Therefore, the research and application of dry powder inhalants with natural active products are important for human health and scientific development.
Author contributions
BW: writing—original draft preparation. JX, ST, and WZ: writing—review and editing. ST and BH: supervision and approval. All authors contributed significantly to the writing of the manuscript.
Funding
This research was supported by Changsha Medical University.
Conflict of interest
The authors declare that the research was conducted in the absence of any commercial or financial relationships that could be construed as a potential conflict of interest.
Publisher’s note
All claims expressed in this article are solely those of the authors and do not necessarily represent those of their affiliated organizations, or those of the publisher, the editors and the reviewers. Any product that may be evaluated in this article, or claim that may be made by its manufacturer, is not guaranteed or endorsed by the publisher.
References
1. Wei S, Sun T, Du J, Zhang B, Xiang D, Li W. Xanthohumol, a prenylated flavonoid from Hops, exerts anticancer effects against gastric cancer in vitro. Oncol Rep. (2018) 40:3213–3222. doi: 10.3892/or.2018.6723
2. Chen X, Liao Y, Long D, Yu T, Shen F, Lin X. The Cdc2/Cdk1 inhibitor, purvalanol A, enhances the cytotoxic effects of taxol through Op18/stathmin in non-small cell lung cancer cells in vitro. Int J Mol Med. (2017) 40:235–242. doi: 10.3892/ijmm.2017.2989
3. Shen F, Long D, Yu T, Chen X, Liao Y, Wu Y, et al. Vinblastine differs from Taxol as it inhibits the malignant phenotypes of NSCLC cells by increasing the phosphorylation of Op18/stathmin. Oncol Rep. (2017) 37:2481–2489. doi: 10.3892/or.2017.5469
4. Dai Z, Zhu B, Yu H, Jian X, Peng J, Fang C, et al. Role of autophagy induced by arecoline in angiogenesis of oral submucous fibrosis. Arch Oral Biol. (2019) 102:7–15. doi: 10.1016/j.archoralbio.2019.03.021
5. Bilia AR, Piazzini V, Guccione C, Risaliti L, Asprea M, Capecchi G, et al. Improving on nature: the role of nanomedicine in the development of clinical natural drugs. Planta Med. (2017) 83:366–381. doi: 10.1055/s-0043-102949
6. Puglia C, Lauro MR, Tirendi GG, Fassari GE, Carbone C, Bonina F, et al. Modern drug delivery strategies applied to natural active compounds. Expert Opin Drug Deliv. (2017) 14:755–768. doi: 10.1080/17425247.2017.1234452
7. Salama AH. Spray drying as an advantageous strategy for enhancing pharmaceuticals bioavailability. Drug Deliv Transl Res. (2020) 10:1–12. doi: 10.1007/s13346-019-00648-9
8. De Mohac LM, Caruana R, Cavallaro G, Giammona G, Licciardi M. Spray-drying, solvent-casting and freeze-drying techniques: a comparative study on their suitability for the enhancement of drug dissolution rates. Pharm Res. (2020) 37:57. doi: 10.1007/s11095-020-2778-1
9. Jermain SV, Brough C, Williams ROIII. Amorphous solid dispersions and nanocrystal technologies for poorly water-soluble drug delivery - An update. Int J Pharm. (2018) 535:379–392. doi: 10.1016/j.ijpharm.2017.10.051
10. Davis M, Walker G. Recent strategies in spray drying for the enhanced bioavailability of poorly water-soluble drugs. J Control Release. (2018) 269:110–127. doi: 10.1016/j.jconrel.2017.11.005
11. Sansone F, Esposito T, Lauro MR, Picerno P, Mencherini T, Gasparri F, et al. Application of spray drying particle engineering to a high-functionality/low-solubility milk thistle extract: powders production and characterization. Molecules. (2018) 23:1716. doi: 10.3390/molecules23071716
12. Zanoni F, Primiterra M, Angeli N, Zoccatelli G. Microencapsulation by spray-drying of polyphenols extracted from red chicory and red cabbage: effects on stability and color properties. Food Chem. (2020) 307:125535. doi: 10.1016/j.foodchem.2019.125535
13. da Costa RS, Teixeira CB, Gabbay Alves TV, Ribeiro-Costa RM, Casazza AA, Aliakbarian B, et al. Optimization of spray drying conditions to microencapsulate cupuassu (Theobroma grandiflorum) seed by-product extract. Nat Prod Res. (2019) 33:2600–2608. doi: 10.1080/14786419.2018.1462178
14. Gavarić A, Vladić J, Ambrus R, Jokić S, Szabó-Révész P, Tomić M, et al. Spray drying of a subcritical extract using marrubium vulgare as a method of choice for obtaining high quality powder. Pharmaceutics. (2019) 11:523. doi: 10.3390/pharmaceutics11100523
15. ElKasabgy NA, Adel IM, Elmeligy MF. Respiratory tract: structure and attractions for drug delivery using dry powder inhalers. AAPS PharmSciTech. (2020) 21:238. doi: 10.1208/s12249-020-01757-2
16. Okamoto H, Danjo K. Application of supercritical fluid to preparation of powders of high-molecular weight drugs for inhalation. Adv Drug Deliv Rev. (2008) 60:433–446. doi: 10.1016/j.addr.2007.02.002
17. Amin R, Ratjen F. Emerging drugs for cystic fibrosis. Expert Opin Emerg Drugs. (2014) 19:143–155. doi: 10.1517/14728214.2014.882316
18. Doryab A, Amoabediny G, Salehi-Najafabadi A. Advances in pulmonary therapy and drug development: lung tissue engineering to lung-on-a-chip. Biotechnol Adv. (2016) 34:588–596. doi: 10.1016/j.biotechadv.2016.02.006
19. Huybrechts KF, Bateman BT, Pawar A, Bessette LG, Mogun H, Levin R, et al. Maternal and fetal outcomes following exposure to duloxetine in pregnancy: cohort study. BMJ. (2020) 368:m237. doi: 10.1136/bmj.m237
20. Roman MA, Rossiter HB, Casaburi R. Exercise, ageing and the lung. Eur Respir J. (2016) 48:1471–1486. doi: 10.1183/13993003.00347-2016
21. Schneider JL, Rowe JH, Garcia-de-Alba C, Kim CF, Sharpe AH, Haigis MC. The aging lung: physiology, disease, and immunity. Cell. (2021) 184:1990–2019. doi: 10.1016/j.cell.2021.03.005
22. Selroos O. Dry-powder inhalers in acute asthma. Ther Deliv. (2014) 5:69–81. doi: 10.4155/tde.13.132
23. Usmani O, Roche N, Marshall J, Danagher H, Price D. An innovative corticosteroid/long-acting β(2)-agonist breath-triggered inhaler: facilitating lung delivery of fluticasone propionate/formoterol fumarate for the treatment of asthma. Expert Opin Drug Deliv. (2019) 16:1367–1380. doi: 10.1080/17425247.2019.1689957
24. Park HS, Yoon D, Lee HY, Ban GY, Wan Yau Ming S, Jie JLZ, et al. Real-life effectiveness of inhaler device switch from dry powder inhalers to pressurized metred-dose inhalers in patients with asthma treated with ICS/LABA. Respirology. (2019) 24:972–979. doi: 10.1111/resp.13559
25. Muralidharan P, Hayes D Jr., Mansour HM. Dry powder inhalers in COPD, lung inflammation and pulmonary infections. Expert Opin Drug Deliv. (2015) 12:947–962. doi: 10.1517/17425247.2015.977783
26. Wang M, Xie Z, Xu J, Feng Z. TWEAK/Fn14 axis in respiratory diseases. Clin Chim Acta. (2020) 509:139–148. doi: 10.1016/j.cca.2020.06.007
27. Assadpour E, Jafari SM. Advances in spray-drying encapsulation of food bioactive ingredients: from microcapsules to nanocapsules. Annu Rev Food Sci Technol. (2019) 10:103–131. doi: 10.1146/annurev-food-032818-121641
28. Eedara B, Alabsi W, Encinas-Basurto D, Polt R, Mansour HM. Spray-Dried Inhalable Powder Formulations of Therapeutic Proteins and Peptides. AAPS PharmSciTech. (2021) 22:185. doi: 10.1208/s12249-021-02043-5
29. Sosnik A, Seremeta KP. Advantages and challenges of the spray-drying technology for the production of pure drug particles and drug-loaded polymeric carriers. Adv Colloid Interface Sci. (2015) 223:40–54. doi: 10.1016/j.cis.2015.05.003
30. Healy AM, Amaro MI, Paluch KJ, Tajber L. Dry powders for oral inhalation free of lactose carrier particles. Adv Drug Deliv Rev. (2014) 75:32–52. doi: 10.1016/j.addr.2014.04.005
31. Hoppentocht M, Hagedoorn P, Frijlink HW, de Boer AH. Technological and practical challenges of dry powder inhalers and formulations. Adv Drug Deliv Rev. (2014) 75:18–31. doi: 10.1016/j.addr.2014.04.004
32. Lavorini F, Pistolesi M, Usmani OS. Recent advances in capsule-based dry powder inhaler technology. Multidiscip Respir Med. (2017) 12:11. doi: 10.4081/mrm.2017.236
33. Shetty N, Cipolla D, Park H, Zhou QT. Physical stability of dry powder inhaler formulations. Expert Opin Drug Deliv. (2020) 17:77–96. doi: 10.1080/17425247.2020.1702643
34. Wicks C, Hudlicky T, Rinner U. Morphine alkaloids: history, biology, and synthesis. Alkaloids Chem Biol. (2021) 86:145–342. doi: 10.1016/bs.alkal.2021.04.001
35. Schubert MM, Irwin C, Seay RF, Clarke HE, Allegro D, Desbrow B. Caffeine, coffee, and appetite control: a review. Int J Food Sci Nutr. (2017) 68:901–912. doi: 10.1080/09637486.2017.1320537
36. Schurman LD, Lu D, Kendall DA, Howlett AC, Lichtman AH. Molecular mechanism and cannabinoid pharmacology. Handb Exp Pharmacol. (2020) 258:323–353. doi: 10.1007/164_2019_298
37. Shara M, Stohs SJ. Efficacy and safety of white willow bark (Salix alba) extracts. Phytother Res. (2015) 29:1112–1116. doi: 10.1002/ptr.5377
38. Wani TA, Bakheit AH, Zargar S, Alanazi ZS, Al-Majed AA. Influence of antioxidant flavonoids quercetin and rutin on the in-vitro binding of neratinib to human serum albumin. Spectrochim Acta A Mol Biomol Spectrosc. (2021) 246:118977. doi: 10.1016/j.saa.2020.118977
39. Kidd PM. Bioavailability and activity of phytosome complexes from botanical polyphenols: the silymarin, curcumin, green tea, and grape seed extracts. Altern Med Rev. (2009) 14:226–246.
40. Riccioni G, Frigiola A, Pasquale S, de Massimo G, D’Orazio N. Vitamin C and E consumption and coronary heart disease in men. Front Biosci (Elite Ed). (2012) 4:373–380. doi: 10.2741/e384
42. Sharifi-Rad M, Mnayer D, Morais-Braga MFB. Echinacea plants as antioxidant and antibacterial agents: from traditional medicine to biotechnological applications. Phytother Res. (2018) 32:1653–1663. doi: 10.1002/ptr.6101
43. Fang X, Hu X. Advances in the synthesis of Lignan natural products. Molecules. (2018) 23:3385. doi: 10.3390/molecules23123385
44. Serafini M, Peluso I, Raguzzini A. Flavonoids as anti-inflammatory agents. Proc Nutr Soc. (2010) 69:273–278. doi: 10.1017/S002966511000162X
45. Güçlü-Ustündağ O, Mazza G. Saponins: properties, applications and processing. Crit Rev Food Sci Nutr. (2007) 47:231–258. doi: 10.1080/10408390600698197
46. Nistor G, Trandafirescu C, Prodea A, Milan A, Cristea A, Ghiulai R, et al. Semisynthetic derivatives of pentacyclic triterpenes bearing heterocyclic moieties with therapeutic potential. Molecules. (2022) 27:6552. doi: 10.3390/molecules27196552
47. Wang XY, Zhang DD, Yin JY, Nie SP, Xie MY. Recent developments in Hericium erinaceus polysaccharides: extraction, purification, structural characteristics and biological activities. Crit Rev Food Sci Nutr. (2019) 59:S96–115. doi: 10.1080/10408398.2018.1521370
48. Wang K, Feng X, Chai L, Cao S, Qiu F. The metabolism of berberine and its contribution to the pharmacological effects. Drug Metab Rev. (2017) 49:139–157. doi: 10.1080/03602532.2017.1306544
49. Wirngo FE, Lambert MN, Jeppesen PB. The physiological effects of dandelion (Taraxacum officinale) in Type 2 diabetes. Rev Diabet Stud. (2016) 13:113–131. doi: 10.1900/RDS.2016.13.113
50. Chanet A, Milenkovic D, Manach C, Mazur A, Morand C. Citrus flavanones: what is their role in cardiovascular protection? J Agric Food Chem. (2012) 60:8809–8822. doi: 10.1021/jf300669s
51. Li AM, Xu J. Cerebrospinal fluid-tissue exchange revealed by phase alternate labeling with null recovery MRI. Magn Reson Med. (2022) 87:1207–1217. doi: 10.1002/mrm.29092
52. Burhani MD, Rasenick MM. Fish oil and depression: The skinny on fats. J Integr Neurosci. (2017) 16:S115–S124. doi: 10.3233/JIN-170072
53. Home P. The evolution of insulin therapy. Diabetes Res Clin Pract. (2021) 175:108816. doi: 10.1016/j.diabres.2021.108816
54. Goulet DR, Atkins WM. Considerations for the design of antibody-based therapeutics. J Pharm Sci. (2020) 109:74–103. doi: 10.1016/j.xphs.2019.05.031
55. Beurskens DMH, Huckriede JP, Schrijver R, Hemker HC, Reutelingsperger CP, Nicolaes GAF. The anticoagulant and nonanticoagulant properties of heparin. Thromb Haemost. (2020) 120:1371–1383. doi: 10.1055/s-0040-1715460
56. Newman DJ, Cragg GM. Natural products as sources of new drugs over the 30 years from 1981 to 2010. J Natural Prod. (2012) 75:311–335. doi: 10.1021/np200906s
57. Adel IM, ElMeligy MF, Abdelrahim MEA, Maged A, Abdelkhalek AA, Abdelmoteleb AMM, et al. Design and characterization of spray-dried proliposomes for the pulmonary delivery of curcumin. Int J Nanomedicine. (2021) 16:2667–2687. doi: 10.2147/IJN.S306831
58. He H, Jiang H, Chen Y, Ye J, Wang A, Wang C, et al. Oridonin is a covalent NLRP3 inhibitor with strong anti-inflammasome activity. Nat Commun. (2018) 9:2550. doi: 10.1038/s41467-018-04947-6
59. Alipieva K, Korkina L, Orhan IE, Georgiev MI. Verbascoside–a review of its occurrence, (bio)synthesis and pharmacological significance. Biotechnol Adv. (2014) 32:1065–1076. doi: 10.1016/j.biotechadv.2014.07.001
60. Carullo G, Cappello AR, Frattaruolo L, Badolato M, Armentano B, Aiello F. Quercetin and derivatives: useful tools in inflammation and pain management. Future Med Chem. (2017) 9:79–93. doi: 10.4155/fmc-2016-0186
61. Dvorakova M, Landa P. Anti-inflammatory activity of natural stilbenoids: a review. Pharmacol Res. (2017) 124:126–145.
62. Trotta V, Lee WH, Loo CY, Haghi M, Young PM, Scalia S, et al. In vitro biological activity of resveratrol using a novel inhalable resveratrol spray-dried formulation. Int J Pharm. (2015) 491:190–197.
63. Prysyazhna O, Wolhuter K, Switzer C, Santos C, Yang X, Lynham S, et al. Blood pressure-lowering by the antioxidant resveratrol is counterintuitively mediated by oxidation of cGMP-dependent protein kinase. Circulation. (2019) 140:126–137.
64. Martin HD, Kock S, Scherrers R, Lutter K, Wagener T, Hundsdörfer C, et al. 3,3’-Dihydroxyisorenieratene, a natural carotenoid with superior antioxidant and photoprotective properties. Angew Chem Int Ed Engl. (2009) 48:400–403. doi: 10.1002/anie.200803668
65. Liu Q, Jin Z, Xu Z, Yang H, Li L, Li G, et al. Antioxidant effects of ginkgolides and bilobalide against cerebral ischemia injury by activating the Akt/Nrf2 pathway in vitro and in vivo. Cell Stress Chaperones. (2019) 24:441–452. doi: 10.1007/s12192-019-00977-1
66. Maurya BK, Trigun SK. Fisetin modulates antioxidant enzymes and inflammatory factors to inhibit aflatoxin-B1 induced hepatocellular carcinoma in rats. Oxid Med Cell Longev. (2016) 2016:1972793. doi: 10.1155/2016/1972793
67. Mohtar N, Taylor KM, Sheikh K, Somavarapu S. Design and development of dry powder sulfobutylether-β-cyclodextrin complex for pulmonary delivery of fisetin. Eur J Pharm Biopharm. (2017) 113:1–10.
68. Porras G, Chassagne F, Lyles JT, Marquez L, Dettweiler M, Salam AM, et al. Ethnobotany and the role of plant natural products in antibiotic drug discovery. Chem Rev. (2021) 121:3495–3560. doi: 10.1021/acs.chemrev.0c00922
69. Tsou LK, Lara-Tejero M, RoseFigura J, Zhang ZJ, Wang YC, Yount JS, et al. Antibacterial flavonoids from medicinal plants covalently inactivate type iii protein secretion substrates. J Am Chem Soc. (2016) 138:2209–2218. doi: 10.1021/jacs.5b11575
70. Jia Y, Hao C, Yang Q, Zhang W, Li G, Liu S, et al. Inhibition of Haemophilus parasuis by berberine and proteomic studies of its mechanism of action. Res Vet Sci. (2021) 138:62–68. doi: 10.1016/j.rvsc.2021.06.004
71. Choi YH, Shin EM, Kim YS, Cai XF, Lee JJ, Kim HP. Anti-inflammatory principles from the fruits of Evodia rutaecarpa and their cellular action mechanisms. Arch Pharm Res. (2006) 29:293–297. doi: 10.1007/BF02968573
72. Li M, Zhang T, Zhu L, Wang R, Jin Y. Liposomal andrographolide dry powder inhalers for treatment of bacterial pneumonia via anti-inflammatory pathway. Int J Pharm. (2017) 528:163–171. doi: 10.1016/j.ijpharm.2017.06.005
73. Lau MSHR. Effect of particle formulation on dry powder inhalation efficiency. Curr Pharm Design. (2010) 16:2377–2387.
74. Peng T, Lin S, Niu B, Wang X, Huang Y, Zhang X, et al. Influence of physical properties of carrier on the performance of dry powder inhalers. Acta Pharm Sin B. (2016) 6:308–318.
75. Pilcer G, Wauthoz N, Amighi K. Lactose characteristics and the generation of the aerosol. Adv Drug Deliv Rev. (2012) 64:233–256.
76. Srichana T, Martin GP, Marriott C. On the relationship between drug and carrier deposition from dry powder inhalers in vitro. Int J Pharm. (1998) 167:13–23.
77. Boer AD. Inhalation characteristics and their effects on in vitro drug delivery from dry powder inhalers Part 1. Inhalation characteristics, work of breathing and volunteers’ preference in dependence of the inhaler resistance. Int J Pharm. (1996) 130:231–244.
78. Zhou, QT, Morton DA. Drug-lactose binding aspects in adhesive mixtures: controlling performance in dry powder inhaler formulations by altering lactose carrier surfaces. Adv Drug Deliv Rev. (2012) 64:275–284. doi: 10.1016/j.addr.2011.07.002
79. Saint-Lorant G, Leterme P, Gayot A, Flament MP. Influence of carrier on the performance of dry powder inhalers. Int J Pharm. (2007) 334:85–91.
80. Hamishehkar H, Emami J, Najafabadi AR, Gilani K, Minaiyan M, Mahdavi H. Influence of carrier particle size, carrier ratio and addition of fine ternary particles on the dry powder inhalation performance of insulin-loaded PLGA microcapsules. Powder Technol. (2010) 201:289–295.
81. Steckel H, Bolzen N. Alternative sugars as potential carriers for dry powder inhalations. Int J Pharm. (2004) 270:297–306.
82. Rattanupatam T, Srichana T. Budesonide dry powder for inhalation: effects of leucine and mannitol on the efficiency of delivery. Drug Deliv. (2014) 21:397–405. doi: 10.3109/10717544.2013.868555
83. Traini D, Young PM, Jones M, Edge S, Price R. Comparative study of erythritol and lactose monohydrate as carriers for inhalation: atomic force microscopy and in vitro correlation. Eur J Pharm Sci. (2006) 27:243–251. doi: 10.1016/j.ejps.2005.10.005
84. Kaialy W, Alhalaweh A, Velaga SP, Nokhodchi A. Effect of carrier particle shape on dry powder inhaler performance. Int J Pharm. (2011) 421:12–23.
85. Kaialy W, Larhrib H, Martin GP, Nokhodchi A. The effect of engineered mannitol-lactose mixture on dry powder inhaler performance. Pharm Res. (2012) 29:2139–2156. doi: 10.1007/s11095-012-0743-3
86. Littringer EM, Mescher A, Schroettner H, Achelis L, Walzel P, Urbanetz NA. Spray dried mannitol carrier particles with tailored surface properties–the influence of carrier surface roughness and shape. Eur J Pharm Biopharm. (2012) 82:194–204. doi: 10.1016/j.ejpb.2012.05.001
87. Tan BM, Chan LW, Heng PW. Improving dry powder inhaler performance by surface roughening of lactose carrier particles. Pharm Res. (2016) 33:1923–1935.
88. Kaialy W, Nokhodchi A. Dry powder inhalers: physicochemical and aerosolization properties of several size-fractions of a promising alterative carrier, freeze-dried mannitol. Eur J Pharm Sci. (2015) 68:56–67. doi: 10.1016/j.ejps.2014.12.005
89. Morton, D, Staniforth J. Dry powder composition comprising co-jet milled particles for pulmonary inhalation. U.S. Patent No. US-8182838-B2. England: Vectura Limited. (2012).
90. Mangal S, Park H, Nour R, Shetty N, Cavallaro A, Zemlyanov D, et al. Correlations between surface composition and aerosolization of jet-milled dry powder inhaler formulations with pharmaceutical lubricants. Int J Pharm. (2019) 568:118504. doi: 10.1016/j.ijpharm.2019.118504
91. Miyazaki Y, Aruga N, Kadota K, Tozuka Y, Takeuchi H. Improved respirable fraction of budesonide powder for dry powder inhaler formulations produced by advanced supercritical CO2 processing and use of a novel additive. Int J Pharm. (2017) 528:118–126. doi: 10.1016/j.ijpharm.2017.06.002
92. Ogáin ON, Li J, Tajber L, Corrigan OI, Healy AM. Particle engineering of materials for oral inhalation by dry powder inhalers. I-Particles of sugar excipients (trehalose and raffinose) for protein delivery. Int J Pharm. (2011) 405:23–35. doi: 10.1016/j.ijpharm.2010.11.039
93. Faghihi H, Vatanara A, Najafabadi AR, Ramezani V, Gilani K. The use of amino acids to prepare physically and conformationally stable spray-dried IgG with enhanced aerosol performance. Int J Pharm. (2014) 466:163–171. doi: 10.1016/j.ijpharm.2014.03.020
94. Chan H-K, Clark AR, Feeley JC, Kuo MC, Lehrman SR, Pikal-Cleland K, et al. Physical stability of salmon calcitonin spray-dried powders for inhalation. J Pharm Sci. (2004) 93:792–804.
95. Mangal S, Nie H, Xu R, Guo R, Cavallaro A, Zemlyanov D, et al. Physico-chemical properties, aerosolization and dissolution of co-spray dried azithromycin particles with L-leucine for inhalation. Pharm Res. (2018) 35:28. doi: 10.1007/s11095-017-2334-9
96. Ogienko AG, Bogdanova EG, Trofimov NA, Myz SA, Ogienko AA, Kolesov BA, et al. Large porous particles for respiratory drug delivery. Glycine-based formulations. Eur J Pharm Sci. (2017) 110:148–156. doi: 10.1016/j.ejps.2017.05.007
97. Vartiainen V, Bimbo LM, Hirvonen J, Kauppinen EI, Raula J. Aerosolization, drug permeation and cellular interaction of dry powder pulmonary formulations of corticosteroids with hydroxypropyl-β-cyclodextrin as a solubilizer. Pharm Res. (2017) 34:25–35.
98. Singh A, Van den Mooter G. Spray drying formulation of amorphous solid dispersions. Adv Drug Deliv Rev. (2016) 100:27–50.
99. Nandiyanto ABD, Okuyama K. Progress in developing spray-drying methods for the production of controlled morphology particles: from the nanometer to submicrometer size ranges. Adv Powder Technol. (2011) 22:1–19.
100. Beck-Broichsitter M, Paulus IE, Greiner A, Kissel T. Modified vibrating-mesh nozzles for advanced spray-drying applications. Eur J Pharm Biopharm. (2015) 92:96–101. doi: 10.1016/j.ejpb.2015.03.001
101. Esposito E, Roncarati R, Cortesi R, Cervellati F, Nastruzzi C. Production of eudragit microparticles by spray-drying technique: influence of experimental parameters on morphological and dimensional characteristics. Pharm Dev Technol. (2000) 5:267–278. doi: 10.1081/pdt-100100541
102. Lechanteur A, Evrard B. Influence of composition and spray-drying process parameters on carrier-free DPI properties and behaviors in the lung: a review. Pharmaceutics. (2020) 12:55. doi: 10.3390/pharmaceutics12010055
103. Gallo L, Bucalá V. A review on influence of spray drying process parameters on the production of medicinal plant powders. Curr Drug Discov Technol. (2019) 16:340–354. doi: 10.2174/1570163815666180801152918
104. Wang B, Liu F, Xiang J, He Y, Zhang Z, Cheng Z, et al. A critical review of spray-dried amorphous pharmaceuticals: synthesis, analysis and application. Int J Pharm. (2021) 594:120165. doi: 10.1016/j.ijpharm.2020.120165
105. Wanning S, Suverkrup R, Lamprecht A. Pharmaceutical spray freeze drying. Int J Pharm. (2015) 488:136–153.
106. Ali ME, Lamprecht A. Spray freeze drying for dry powder inhalation of nanoparticles. Eur J Pharm Biopharm. (2014) 87:510–517.
107. Parthasarathi S, Anandharamakrishnan C. Enhancement of oral bioavailability of vitamin E by spray-freeze drying of whey protein microcapsules. Food Bioprod Process. (2016) 100:469–476.
108. Ziaee A, Albadarin AB, Padrela L, Femmer T, O’Reilly E, Walker G. Spray drying of pharmaceuticals and biopharmaceuticals: critical parameters and experimental process optimization approaches. Eur J Pharm Sci. (2019) 127:300–318. doi: 10.1016/j.ejps.2018.10.026
109. Belbekhouche S, Poostforooshan J, Shaban M, Ferrara B, Alphonse V, Cascone I, et al. Fabrication of large pore mesoporous silica microspheres by salt-assisted spray-drying method for enhanced antibacterial activity and pancreatic cancer treatment. Int J Pharm. (2020) 590:119930. doi: 10.1016/j.ijpharm.2020.119930
110. Bohr A, Ruge CA, Beck-Broichsitter M. Preparation of nanoscale pulmonary drug delivery formulations by spray drying. Adv Exp Med Biol. (2014) 811:183–206.
111. Misra A, Jinturkar K, Patel D, Lalani J, Chougule M. Recent advances in liposomal dry powder formulations: preparation and evaluation. Expert Opin Drug Deliv. (2009) 6:71–89.
112. Chennakesavulu S, Mishra A, Sudheer A, Sowmya C, Suryaprakash Reddy C, Bhargav E. Pulmonary delivery of liposomal dry powder inhaler formulation for effective treatment of idiopathic pulmonary fibrosis. Asian J Pharm Sci. (2018) 13:91–100. doi: 10.1016/j.ajps.2017.08.005
113. Tuli RA, Dargaville TR, George GA, Islam N. Polycaprolactone microspheres as carriers for dry powder inhalers: effect of surface coating on aerosolization of salbutamol sulfate. J Pharm Sci. (2012) 101:733–745.
114. Elhissi A. Liposomes for pulmonary drug delivery: the role of formulation and inhalation device design. Curr Pharm Des. (2017) 23: 362–372.
115. Pan K, Zhong Q, Baek SJ. Enhanced dispersibility and bioactivity of curcumin by encapsulation in casein nanocapsules. J Agric Food Chem. (2013) 61:6036–6043. doi: 10.1021/jf400752a
116. Sverdlov Arzi R, Sosnik A. Electrohydrodynamic atomization and spray-drying for the production of pure drug nanocrystals and co-crystals. Adv Drug Deliv Rev. (2018) 131:79–100. doi: 10.1016/j.addr.2018.07.012
117. Dimer FA, Ortiz M, Pohlmann AR, Guterres SS. Inhalable resveratrol microparticles produced by vibrational atomization spray drying for treating pulmonary arterial hypertension. J Drug Deliv Sci Technol. (2015) 29:152–158.
118. Tang Y, Wang X, Li J, Nie Y, Liao G, Yu Y, et al. Overcoming the reticuloendothelial system barrier to drug delivery with a “Don’t-Eat-Us” strategy. ACS Nano. (2019) 13:13015–13026. doi: 10.1021/acsnano.9b05679
119. Ni R, Zhao J, Liu Q, Liang Z, Muenster U, Mao S. Nanocrystals embedded in chitosan-based respirable swellable microparticles as dry powder for sustained pulmonary drug delivery. Eur J Pharm Sci. (2017) 99:137–146. doi: 10.1016/j.ejps.2016.12.013
120. Dianawati D, Mishra V, Shah NP. Survival of microencapsulated probiotic bacteria after processing and during storage: a review. Crit Rev Food Sci Nutr. (2016) 56:1685–1716. doi: 10.1080/10408398.2013.798779
121. Jovanovic AA, Lević SM, Pavlović VB, Marković SB, Pjanović RV, Ðorđević VB, et al. Freeze vs. spray drying for dry wild thyme (Thymus serpyllum L.) extract formulations: the impact of gelatin as a coating material. Molecules. (2021) 26:3933. doi: 10.3390/molecules26133933
122. Desai KG, Park HJ. Encapsulation of vitamin C in tripolyphosphate cross-linked chitosan microspheres by spray drying. J Microencapsul. (2005) 22:179–192.
123. Kanha N, Regenstein JM, Surawang S, Pitchakarn P, Laokuldilok T. Properties and kinetics of the in vitro release of anthocyanin-rich microcapsules produced through spray and freeze-drying complex coacervated double emulsions. Food Chem. (2021) 340:127950. doi: 10.1016/j.foodchem.2020.127950
124. Mehnert W, Mäder K. Solid lipid nanoparticles: production, characterization and applications. Adv Drug Deliv Rev. (2001) 47:165–196. doi: 10.1016/s0169-409x(01)00105-3
125. Xu J, Lu X, Zhu X, Yang Y, Liu Q, Zhao D, et al. Formulation and characterization of spray-dried powders containing vincristine-liposomes for pulmonary delivery and its pharmacokinetic evaluation from in vitro and in vivo. J Pharm Sci. (2019) 108:3348–3358. doi: 10.1016/j.xphs.2019.05.009
126. Marante T, Viegas C, Duarte I, Macedo AS, Fonte P. An overview on spray-drying of protein-loaded polymeric nanoparticles for dry powder inhalation. Pharmaceutics. (2020) 12:1032. doi: 10.3390/pharmaceutics12111032
127. Zimmermann CM, Baldassi D, Chan K, Adams NBP, Neumann A, Porras-Gonzalez DL, et al. Spray drying siRNA-lipid nanoparticles for dry powder pulmonary delivery. J Control Release. (2022) 351:137–150. doi: 10.1016/j.jconrel.2022.09.021
128. Al-Hallak MH, Sarfraz MK, Azarmi S, Roa WH, Finlay WH, Löbenberg R. Pulmonary delivery of inhalable nanoparticles: dry powder inhalers. Ther Deliv. (2011) 2:1313–1324.
129. Claus S, Weiler C, Schiewe J, Friess W. Optimization of the fine particle fraction of a lyophilized lysozyme formulation for dry powder inhalation. Pharm Res. (2013) 30:1698–1713. doi: 10.1007/s11095-013-1015-6
130. Farkas DR, Hindle M, Longest PW. Characterization of a new high-dose dry powder inhaler (DPI) based on a fluidized bed design. Ann Biomed Eng. (2015) 43:2804–2815. doi: 10.1007/s10439-015-1335-2
131. Hanif SN, Garcia-Contreras L. Pharmaceutical aerosols for the treatment and prevention of tuberculosis. Front Cell Infect Microbiol. (2012) 2:118. doi: 10.3389/fcimb.2012.00118
132. Gaul R, Ramsey JM, Heise A, Cryan SA, Greene CM. Nanotechnology approaches to pulmonary drug delivery. In: Grumezescu AM editor. Design of nanostructures for versatile therapeutic applications. New York, NY: Elsevier (2018). p. 221–53.
133. Martin AR, Finlay WH. Nebulizers for drug delivery to the lungs. Expert Opin Drug Deliv. (2015) 12:889–900. doi: 10.1517/17425247.2015.995087
134. Holland A, Smith F, Penny K, McCrossan G, Veitch L, Nicholson C. Metered dose inhalers versus nebulizers for aerosol bronchodilator delivery for adult patients receiving mechanical ventilation in critical care units. Cochrane Database Syst Rev. (2013) 2013:Cd008863. doi: 10.1002/14651858.CD008863.pub2
135. Waller, DG, Sampson AP. Asthma and chronic obstructive pulmonary disease. Medical pharmacology and therapeutics. Amsterdam: Elsevier (2018). p. 193–209.
136. Velaga SP, Djuris J, Cvijic S, Rozou S, Russo P, Colombo G, et al. Dry powder inhalers: an overview of the in vitro dissolution methodologies and their correlation with the biopharmaceutical aspects of the drug products. Eur J Pharm Sci. (2018) 113:18–28. doi: 10.1016/j.ejps.2017.09.002
137. Nadal JM, Gomes ML, Borsato DM, Almeida MA, Barboza FM, Zawadzki SF, et al. Spray-dried solid dispersions containing ferulic acid: comparative analysis of three carriers, in vitro dissolution, antioxidant potential and in vivo anti-platelet effect. Drug Dev Ind Pharm. (2016) 42:1813–1824. doi: 10.3109/03639045.2016.1173055
138. Hu L, Kong D, Hu Q, Gao N, Pang S. Evaluation of high-performance curcumin nanocrystals for pulmonary drug delivery both in vitro and in vivo. Nanoscale Res Lett. (2015) 10:381. doi: 10.1186/s11671-015-1085-y
139. Meenach SA, Anderson KW, Hilt JZ, McGarry RC, Mansour HM. High-performing dry powder inhalers of paclitaxel DPPC/DPPG lung surfactant-mimic multifunctional particles in lung cancer: physicochemical characterization, in vitro aerosol dispersion, and cellular studies. AAPS PharmSciTech. (2014) 15:1574–1587. doi: 10.1208/s12249-014-0182-z
140. Lu P, Xing Y, Peng H, Liu Z, Zhou QT, Xue Z, et al. Physicochemical and pharmacokinetic evaluation of spray-dried coformulation of Salvia miltiorrhiza polyphenolic acid and l-leucine with improved bioavailability. J Aerosol Med Pulm Drug Deliv. (2020) 33:73–82. doi: 10.1089/jamp.2019.1538
141. Mehta P, Bothiraja C, Mahadik K, Kadam S, Pawar A. Phytoconstituent based dry powder inhalers as biomedicine for the management of pulmonary diseases. Biomed Pharmacother. (2018) 108:828–837. doi: 10.1016/j.biopha.2018.09.094
142. Taki M, Tagami T, Fukushige K, Ozeki T. Fabrication of nanocomposite particles using a two-solution mixing-type spray nozzle for use in an inhaled curcumin formulation. Int. J. Pharm. (2016) 511:104–110. doi: 10.1016/j.ijpharm.2016.06.134
143. Feng T, Wei Y, Lee R, Zhao L. Liposomal curcumin and its application in cancer. Int J Nanomed. (2017) 12:6027–6044.
144. Mali A, Bothiraja C, Purohit RN, Pawar A. In vitro and in vivo performance of novel spray dried andrographolide loaded scleroglucan based formulation for dry powder inhaler. Curr. Drug Deliv. (2017) 14:968–980. doi: 10.2174/1567201814666161109120455
145. Jiang L, Li Y, Yu J, Wang J, Ju J, Dai J. A dry powder inhalable formulation of salvianolic acids for the treatment of pulmonary fibrosis: safety, lung deposition, and pharmacokinetic study. Drug Deliv Transl Res. (2021) 11:1958–1968. doi: 10.1007/s13346-020-00857-7
146. Fan T, Zhu YF, Cao QR, Cui JH. [Preparation, in vitro evaluation of excipient-free dry powder inhalation of extraction of Trollius chinensis]. Zhongguo Zhong Yao Za Zhi. (2013) 38:2096–2100.
Keywords: natural ingredients, spray drying, dry powder inhalation, factors influencing DPI, application of natural extracts
Citation: Wang B, Xiang J, He B, Tan S and Zhou W (2023) Enhancing bioavailability of natural extracts for nutritional applications through dry powder inhalers (DPI) spray drying: technological advancements and future directions. Front. Nutr. 10:1190912. doi: 10.3389/fnut.2023.1190912
Received: 21 March 2023; Accepted: 19 June 2023;
Published: 05 July 2023.
Edited by:
Rosa Perez-Gregorio, University of Vigo, SpainCopyright © 2023 Wang, Xiang, He, Tan and Zhou. This is an open-access article distributed under the terms of the Creative Commons Attribution License (CC BY). The use, distribution or reproduction in other forums is permitted, provided the original author(s) and the copyright owner(s) are credited and that the original publication in this journal is cited, in accordance with accepted academic practice. No use, distribution or reproduction is permitted which does not comply with these terms.
*Correspondence: Binsheng He, hnaios@163.com; Songwen Tan, songwen.tan@csu.edu.cn; Wenhu Zhou, zhouwenhuyaoji@163.com