- 1Guangxi Key Laboratory of Medicinal Resources Protection and Genetic Improvement, Guangxi Botanical Garden of Medicinal Plants, Nanning, China
- 2National Traditional Chinese Medicine Inheritance and Innovation Center, Guangxi Botanical Garden of Medicinal Plants, Nanning, China
- 3College of Pharmacy, Guangxi Medical University, Nanning, China
Platostoma palustre (or Mesona chinensis Benth) is an important medicinal and edible plant in China and Southeast Asian countries. To study the effects of different processing methods on the quality, nutrition, and flavor of P. palustre, we adopted the LC-MS and HS-GC-MS to compare the influences of tedding (S), sweating (M), and drying (H) on the metabolites and volatile substances of P. palustre. Biochemical determinations revealed that the M treatment could promote the accumulation of the contents of total sugar, soluble sugar, and total pectin compared with the H and S treatments but decrease the total flavonoid contents. LC-MS and HS-GC-MS uncovered 98 differential metabolites and 27 differential volatile substances among the three treatments, respectively. Overall, the M treatment facilitated the stabilization and improvement of the quality of polysaccharides and volatile substances, while the H treatment could promote the level of amino acids in P. palustre. The current study provided a theoretical reference for establishing standardized processing methods and sustaining the quality stability of P. palustre in future.
1. Introduction
Platostoma palustre (or Mesona chinensis Benth), also known as “Xiancao,” is an annual herb of the Labiatae family, which is distributed in the provinces of Guangxi, Guangdong, Taiwan, Zhejiang, and Jiangxi in China (1). P. palustre is an important medicinal and edible plant (2, 3), with the above-ground part or the whole plant constituting its medicinal parts. According to the records of “Chinese Materia Medica” and “Dictionary of Traditional Chinese Medicine,” P. palustre is effective in treating thirst and hypertension (4, 5). Modern research reports that P. palustre possesses antioxidation (6), antihypertensive (7), antibiosis (8), and hypolipidemic effects (9). P. palustre is rich in polysaccharides, flavonoids, phenolic acid, volatile oil, and other components (10). Among those, polysaccharides (or xiancao gum) represent one of the most important quality indicators of P. palustre (11), and volatile oil endows the species with a special flavor quality (12). At present, P. palustre is mainly used to produce herbal tea and jelly. With the development of the herbal tea industry, new products made of P. palustre are becoming increasingly popular, thus gradually making it a research hotspot (13).
Generally, the quality of traditional Chinese medicine (TCM) is affected by many factors, such as the variety, origin, harvesting, storage, processing methods, and extraction of the effective components of TCM. Among these, the processing methods include pretreatment, processing, and pulverization of TCM (14). Sweating (micro-fermentation) treatment is the primary processing method for many TCM. Magnolia officinalis has a brown surface, and its cross-section turns purplish-red after sweating treatment and the content of magnolol increases while the content of volatile oil and alkaloids decreases (15). The sweating treatment of Poria cocos after traditional sweating is whiter with better lustre compared with that after steaming, improving the water solubility of its polysaccharides (16). Tedding refers to use the heat emitted by the sun to evaporate water from medicinal materials, while the traditional drying method involves setting up a simple drying room, using outdoor auxiliary equipment to heat the room, and an exhaust fan to extract the generated moisture outside. Both of these methods are necessary steps for the primary processing of many TCM.
In fact, sweating treatment is also the primary processing method in the P. palustre industry. The whole herb medicine of P. palustre is usually harvested before flowering. After harvesting and proper drying, the sweating treatment should be carried out in the production area. Subsequently, through the sweating treatment by stacking, the final herbal medicine of P. palustre would be brownish or brown in color, having a unique aroma (17). Lu (18) reported that there were some differences in the total flavonoids and total sugar content of Mesina chinensis Benth with different (micro-fermentation, shade drying, and oven drying) processing methods, and the content of total flavonoids in M. chinensis treated by micro-fermentation was found to be lower than that in the dried samples. However, there was no obvious difference between the volatile components of the sample of micro-fermentation and the dry samples, but after fermentation was performed two times, the relative content of caryophyllene in the samples decreased significantly (β-caryophyllene 0.85% and α-caryophyllene 0.37%), while fora-selinene and β-selinene had little effect (12, 18).
Up to now, there have been few comprehensive reports on the effects of processing methods on the metabolites and volatile substances of P. palustre. Therefore, in this study, we compared and analyzed the effects of different (tedding, drying, and sweating) processing treatments on the metabolites and volatile substances of P. palustre. Here, we adopted the LC-MS and HS-GC-MS methods to compare the effects of tedding, sweating, and drying on the quality and flavor of P. palustre, thus providing a theoretical reference for establishing standardized processing methods and maintaining the quality stability of P. palustre in future.
2. Materials and methods
2.1. Sample preparation
The P. palustre plants from the same variety (Lingshan) and fields were harvested on 25 July 2022. Subsequently, the fresh plant materials were treated with sweating (M), tedding (S), and drying (H), respectively (Figure 1). Sweating (M) method: Collect the aerial part of P. palustre, remove the roots and redundant soil, and spread and ted the materials in the field. When the materials wither to dark green and lose ~½ of their water content, bundle, stack, and seal the materials with transparent plastic film on the cement ground. Soak the package in the sun for 1 or 2 days, remove the film, spread out, and dry the materials until the stems are fragile, the leaves are slightly flexible, and the water content is ~13–15%. Tedding (S) method: The initial procedure is the same as above. When the materials wither to a dark green color and lose half of their water, transfer them to a cement ground to continue tedding (turn it over every 2 h) until the stems are fragile, the leaves are slightly flexible, and the water content is ~13–15%. Cover them with plastic film in the evening to prevent rain. Drying (H) method: Place the fresh plant materials in the oven and dry at a low temperature of 50°C until the moisture content is ~13–15%. Cut the processed materials into small sections, then grind them into powder, and sieved them with a 200 mesh sieve. All samples were used for subsequent analysis.
2.2. Biochemical determination
The contents of total sugar, soluble sugar, total pectin, and total flavonoid were detected using the total sugar content kit (G0503F), soluble sugar content kit (G0501F), total pectin content kit (G0717F), and total flavonoid kit (G0118F), respectively (Grace Biotechnology, http://geruisi-bio.com/, Suzhou, China). All the kits were measured using a spectrophotometer method.
2.3. UHPLC-MS/MS analysis
A total of six biological replicates were used per treatment. The metabolite extraction was utilized for LC/MS analysis (19), with minor adjustments, and a bioinformatic analysis was carried out at Shanghai Majorbio Co., Ltd. (http://wwwmajorbiocom/) following standard procedures.
2.3.1. Metabolite extraction
In total, 50 mg of powder samples were accurately weighed, and the metabolites were extracted using a 400 μL methanol: water (4:1, v/v) solution with 0.02 mg/mL L-2-chlorophenylalanin as an internal standard. The mixture was allowed to settle at −10°C, treated by high-throughput tissue crusher Wonbio-96c (Shanghai Wanbo Biotechnology Co., LTD) at 50 Hz for 6 min, and then followed by ultrasound at 40 kHz for 30 min at 5°C. The samples were placed at −20°C for 30 min to precipitate proteins. After centrifugation at 13,000 × g at 4°C for 15 min, the supernatant was carefully transferred to sample vials for LC-MS/MS (UHPLC-Q Exactive HF-X system, Thermo Fisher Scientific) analysis.
2.3.2. Quality control sample
As a part of the system conditioning and quality control process, a pooled quality control (QC) sample was prepared by mixing equal volumes of all samples. The QC samples were disposed of and tested in the same manner as the analytic samples, thereby helping to represent the whole sample set, which was injected at regular intervals (every 5–15 samples), in order to monitor the stability of the analysis.
2.3.3. Chromatographic conditions
In total, 2 μL of the sample was separated by an HSS T3 column (100 mm × 2.1 mm i.d., 1.8 μm) and then entered into mass spectrometry detection. The mobile phases consisted of 0.1% formic acid in water:acetonitrile (95:5, v/v; solvent A) and 0.1% formic acid in acetonitrile:isopropanol:water (47.5:47.5:5, v/v; solvent B). The solvent gradient changed according to the following conditions: from 0 to 3.5 min, 0% B to 24.5% B (0.4 mL/min); from 3.5 to 5 min, 24.5% B to 65% B (0.4 mL/min); from 5 to 5.5 min, 65% B to 100% B (0.4 mL/min); from 5.5 to 7.4 min, 100% B to 100% B (0.4 to 0.6 mL/min); from 7.4 to 7.6 min, 100% B to 51.5% B (0.6 mL/min); from 7.6 to 7.8 min, 51.5% B to 0% B (0.6 to 0.5 mL/min); from 7.8 to 9 min, 0% B to 0% B (0.5 to 0.4 mL/min); and from 9 to 10 min, 0% B to 0% B (0.4 mL/min) for equilibrating the systems. The sample injection volume was 2 μL, and the flow rate was set to 0.4 mL/min, with the column temperature maintained at 40°C. During the period of analysis, all these samples were stored at 4°C.
2.3.4. MS conditions
The mass spectrometric data were collected using a Thermo UHPLC -Q Exactive HF-X Mass Spectrometer, equipped with an electrospray ionization (ESI) source operating in either positive or negative ion modes. The optimal conditions were set as followed: heater temperature, 425°C; capillary temperature, 325°C; sheath gas flow rate, 50 arb; aux gas flow rate, 13 arb; ion-spray voltage floating (ISVF), −3,500 V in negative mode, and 3,500 V in positive mode, respectively. Normalized collision energy and 20-40-60V rolling were employed for MS/MS. Full MS resolution was 60,000, and MS/MS resolution was 7,500. Data acquisition was performed using the Data Dependent Acquisition (DDA) mode. The detection was carried out over a mass range of 70–1,050 m/z.
2.3.5. Data preprocessing and annotation
After the mass spectrometry detection was completed, the raw data of LC/MS were preprocessed by Progenesis QI software (Waters Corporation, Milford, USA), and a three-dimensional data matrix in CSV format was exported. The information in this three-dimensional matrix included as follows: sample information, metabolite name, and mass spectral response intensity. Internal standard peaks and any known false positive peaks (including noise, column bleed, and derivatized reagent peaks) were removed from the data matrix, deredundant, and peak pooled. At the same time, the metabolites were searched and identified, with the main databases being the HMDB (http://www.hmdb.ca/), Metlin (https://metlin.scripps.edu/), and Majorbio databases.
The data obtained after the database search were uploaded to the Majorbio cloud platform (https://cloud.majorbio.com) for further analysis. Metabolic features detected in at least 80% of any set of samples were retained. After filtering, minimum metabolite values were imputed for specific samples, in which the metabolite levels fell below the lower limit of quantitation, and each metabolic feature was normalized by sum normalization. To reduce the errors caused by sample preparation and instrument instability, the response intensity of the sample mass spectrum peaks was normalized by the sum normalization method, thereby obtaining the normalized data matrix. At the same time, variables with relative standard deviation (RSD) > 30% of QC samples were removed, and log10 logarithmic transformation was performed to obtain the final data matrix for subsequent analysis.
2.3.6. Differential metabolites analysis
Variance analysis was performed on the matrix file after data preprocessing. The R package ropls (version 1.6.2) was used to perform principal component analysis (PCA) and orthogonal least partial squares discriminant analysis (OPLS-DA), and a seven-cycle interactive validation was used to evaluate the stability of the model. In addition, the Student's t-test and fold difference analysis were carried out. The selection of significantly different metabolites was determined based on the variable importance in the projection (VIP) obtained by the OPLS-DA model and the p-value of the Student's t-test, and the metabolites with VIP > 1 and p < 0.05 were significantly different metabolites (20).
Differential metabolites among the two groups were summarized and mapped into their biochemical pathways through metabolic enrichment and pathway analysis based on a database search (KEGG, http://www.genome.jp/kegg/). Scipy.stats (Python packages; https://docs.scipy.org/doc/scipy/) was exploited to identify statistically significantly enriched pathways using Fisher's exact test.
2.4. HS-GC-MS analysis
In this study, six biological replicates were also employed per treatment. The extraction of volatile substances was used for HS-GC-MS analysis as per the method of Dong et al. (21), with minor modifications, and a bioinformatic analysis was carried out at Shanghai Majorbio Co., Ltd. (http://wwwmajorbiocom/), followed by standard procedures.
2.4.1. Extraction
Accurately, 3 g of the sample was weighed and placed into a 20 ml headspace bottle, and then the headspace bottle was immediately sealed until the analysis.
2.4.2. HS-GC-MS analysis
The analysis was performed using an Agilent 8890B gas chromatography, with a 7697A headspace sampler coupled to an Agilent 7000D mass selective detector with an inert electron impact (EI) ionization source and ionization voltage was 70 eV (Agilent, USA). Analyte compounds were separated with a VF-WAXms (25 m × 0.25 mm × 0.2 μm) capillary column, using 99.999% helium as a carrier gas at a constant flow rate (1 mL/ min). The temperature of the headspace strip heating box was 130°C, the quantitative ring was 150°C, the transmission line was 170°C, the balance time of the sample bottle was 20 min, and the GC cycle time was 35 min. The GC column temperature was programmed to hold at 40°C for 2 min and rose to 100°C at a rate of 5°C per minute, then rose to 230°C at a rate of 15°C per minute, for 5 min. The injection volume of samples was 1 μL and introduced in splitting mode (10:1) with the inlet temperature of 180°C. The ion sources temperature was 230°C, and the quadrupole temperature was 150°C. The scanning mode was full scan mode, the quality scanning range was 30–1,000 m/z, and the scanning frequency was 3.2 scan/s.
2.4.3. Quality control
To evaluate the stability of the analytical system during the run-on process, a quality control (QC) sample was prepared during the experiment. QC samples were prepared by mixing all test samples and were handled in the same way as the formal samples. During instrument testing, a QC sample was inserted every 5–15 samples. The repeatability of QC samples reflects the stability of the instrument in the whole analysis process. At the same time, it can also be used to identify variables with significant variations in the analysis system to ensure the reliability of the results.
2.4.4. Data preprocessing and annotation
After the mass spectrometry detection was completed, the raw data of GC/MS were preprocessed by MassHunter Workstation Quantitative Analysis (v10.0.707.0) software, and a three-dimensional data matrix in CSV format was exported. The internal standard peaks and any known false positive peaks were removed from the data matrix, deredundant, and peak pooled. Meanwhile, the metabolites were searched and identified, and the main database used was the Fiehn and NIST public databases.
The data after being retrieved from the database search were uploaded to the Majorbio cloud platform (https://cloud.majorbio.com) for analysis. Metabolic features detected at least 80% in any set of samples were retained. After filtering, minimum metabolite values were imputed for specific samples, in which the metabolite levels fell below the lower limit of quantitation, and each metabolic feature was normalized by sum. To reduce the errors caused by sample preparation and instrument instability, the response intensity of the sample mass spectrum peaks was normalized by the sum normalization method, thus obtaining a normalized data matrix. At the same time, variables with relative standard deviation (RSD) > 30% of QC samples were removed, and log10 logarithmization was performed to obtain the final data matrix for subsequent analysis.
2.4.5. Differential metabolites analysis
Variance analysis was conducted on the matrix file after data preprocessing. The R package ropls (version 1.6.2) was used to perform principal component analysis (PCA) and orthogonal least partial squares discriminant analysis (OPLS-DA), and a seven-cycle interactive validation was used to evaluate the stability of the model. In addition, Student's t-test and fold difference analysis were performed. The selection of significantly different metabolites was determined based on the variable importance in the projection (VIP), obtained by the OPLS-DA model and the p-value of Student's t-test, and the metabolites with VIP > 1 and p < 0.05 were considered to be significantly different metabolites (20).
2.5. Statistical analysis
SPSS 17.0 software was employed for statistical analysis, and the data means were analyzed using the Duncan test for statistical significance (p < 0.05). Each test was analyzed independently, and the test for homogeneity of variance was performed prior to ANOVA. GraphPad Prism 7 and WPS software were used for data processing and graphic analysis.
3. Results
3.1. Influence of different processing methods on the quality of P. palustre
As shown in Figure 2, compared with the sweating (M) treatment, the total sugar contents significantly decreased by 19.9 and 14.5% in the drying (H) and tedding (S) treatments, respectively. Meanwhile, the soluble sugar contents dramatically reduced by 10.4 and 14.2%, and the total pectin contents significantly decreased by 18.9 and 8.9% in H and S treatments, respectively. However, the total flavonoid contents in H and S treatments significantly increased by 91.2 and 36.5% in comparison with the M treatment.
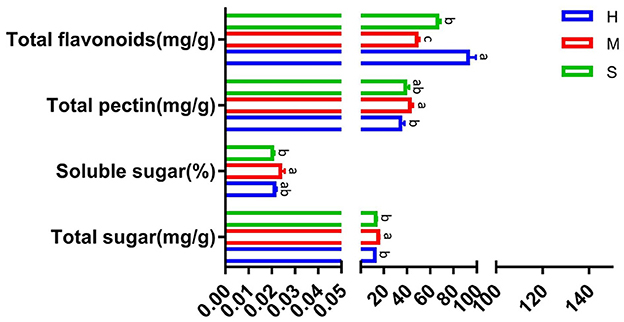
Figure 2. Comparison of total sugar, soluble sugar, total pectin, and total flavonoids under different treatments. The treatments containing the same letters indicated no significant differences (p > 0.05), while the treatments containing different letters indicated significant differences (p < 0.05).
3.2. Metabolites of different treatments based on LC-MS
In this study, the metabolites were extracted from the aerial part of P. palustre plants, with six replicates of each treatment, and analyzed based on LC-MS. A total of 1,591 and 1,595 metabolites were identified under the positive and negative ion scanning modes, respectively (Supplementary Figure 1). Analysis of correlation, PCA, Venn, and PLS-DA showed that the data were reliable (Supplementary Figure 2). Based on these metabolites, the Kyoto Encyclopedia of Genes and Genomes (KEGG) compound classification revealed that a total of 174 metabolites could be categorized as phospholipids, monosaccharides, fatty acids, carboxylic acids, eicosanoids, amino acids, and vitamins (Supplementary Figure 3A; Supplementary Tables 1, 2). Further analysis of the KEGG pathway showed that they were involved in lipid metabolism, carbohydrate metabolism, biosynthesis of other secondary metabolites, amino acid metabolism, and metabolism of cofactors and vitamins (Supplementary Figure 3B).
3.3. Differential metabolites analysis
In this study, the three groups could be better distinguished by OPLS-DA analysis in both positive and negative modes, and the OPLS-DA model was feasible, and the metabolites also differed significantly between the three comparison groups (Figures 3A–C). All the identified metabolites were used for screening differential metabolites according to the VIP > 1 and p < 0.05, and the results of the three comparison groups are presented in Figures 3D–F. A total of 1,594 differential metabolites were identified among the H, M, and S treatments (Supplementary Figure 4). Of these, a total of 1,050, 1,036, and 956 differential metabolites were identified in H vs. M, H vs. S, and S vs. M comparisons, respectively (Supplementary Figures 5A–C). Based on these differential metabolites, the analysis of KEGG compounds classification showed that a total of 98 differential metabolites were detected among the three treatments (Table 1). Among these, there were 62, 53, and 69 differential metabolites in H vs. M, H vs. S, and S vs. M comparisons, respectively, which mainly included phospholipids, carboxylic acids, monosaccharides, amino acids, and vitamins (Supplementary Figures 5D, E). Furthermore, KEGG enrichment analysis revealed that a total of 85, 79, and 46 differential metabolites in H vs. M, H vs. S, and S vs. M comparisons were significantly enriched in different pathways, respectively (p < 0.05; Supplementary Figure 6; Supplementary Table 3). Notably, a total of 28, 51, and 19 differential metabolites presented the highest abundance in H, M, and S treatments, respectively (Table 1).
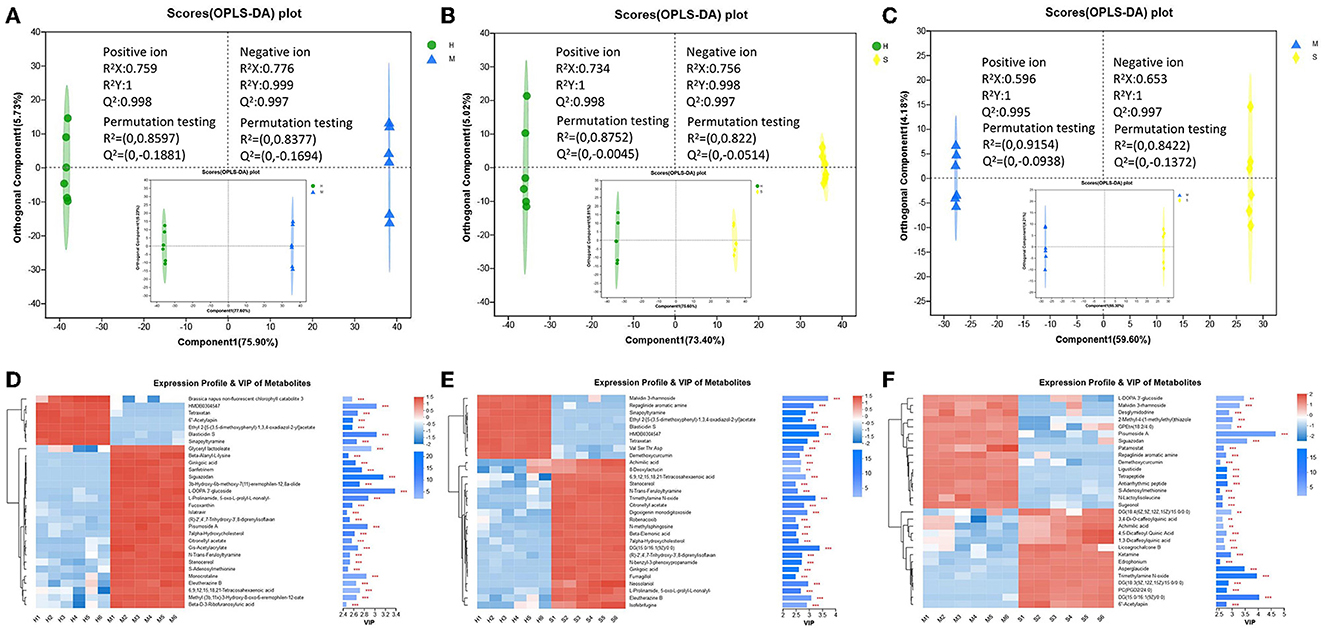
Figure 3. The OPLS-DA plot and expression profile and VIP of metabolites between different comparison groups. (A–C) The OPLS-DA plot of different comparison groups (the larger plot indicated the positive ion modes, and the smaller plot indicated the negative ion modes). (D–F) The expression profile and VIP of metabolites of different comparison groups.
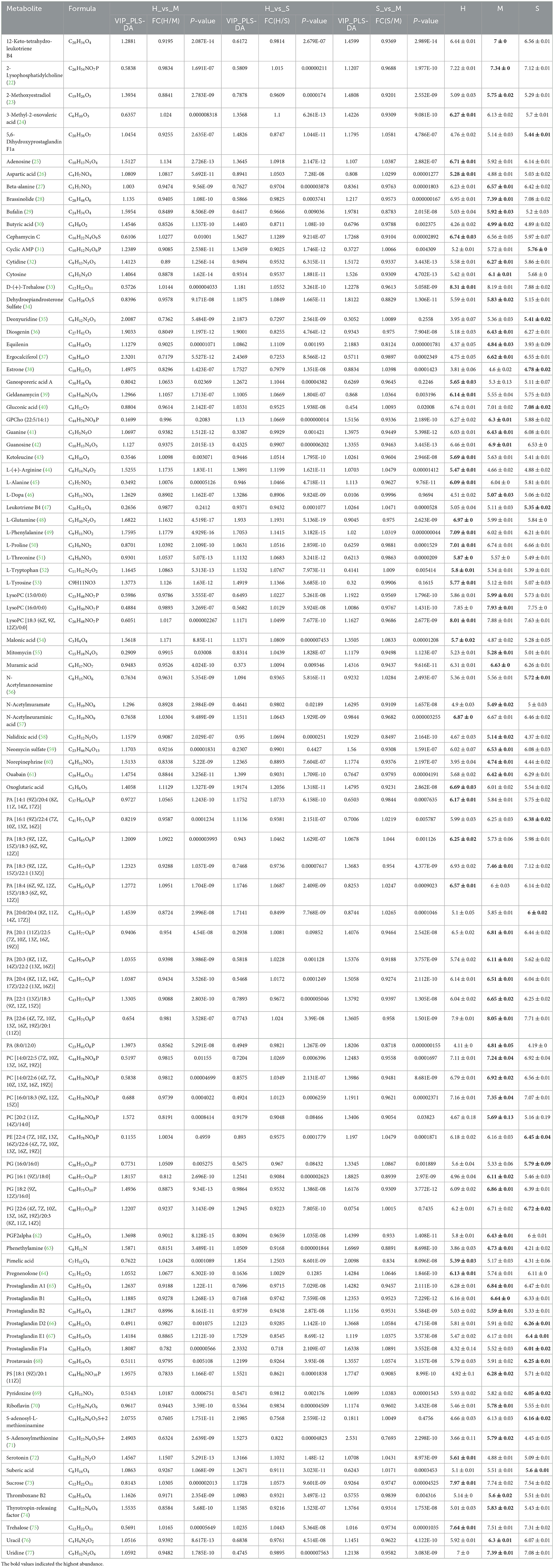
Table 1. Details of 98 differential metabolites identified by LC-MS among the H, M, and S treatments.
3.3.1. Saccharides
As shown in Figures 4A–C, the abundances of sucrose, trehalose, and D-(+)-trehalose were ranked as H>M>S, suggesting that the H and M treatments favored the formation and accumulation of these three substances in comparison with the S treatment. Meanwhile, the abundances of gluconic acid and N-acetylmannosamine under the three treatments were ranked as S>M>H, indicating that the S and M treatments were more conducive to the formation and accumulation of these two substances in comparison to the H treatment (Figures 4D, E).
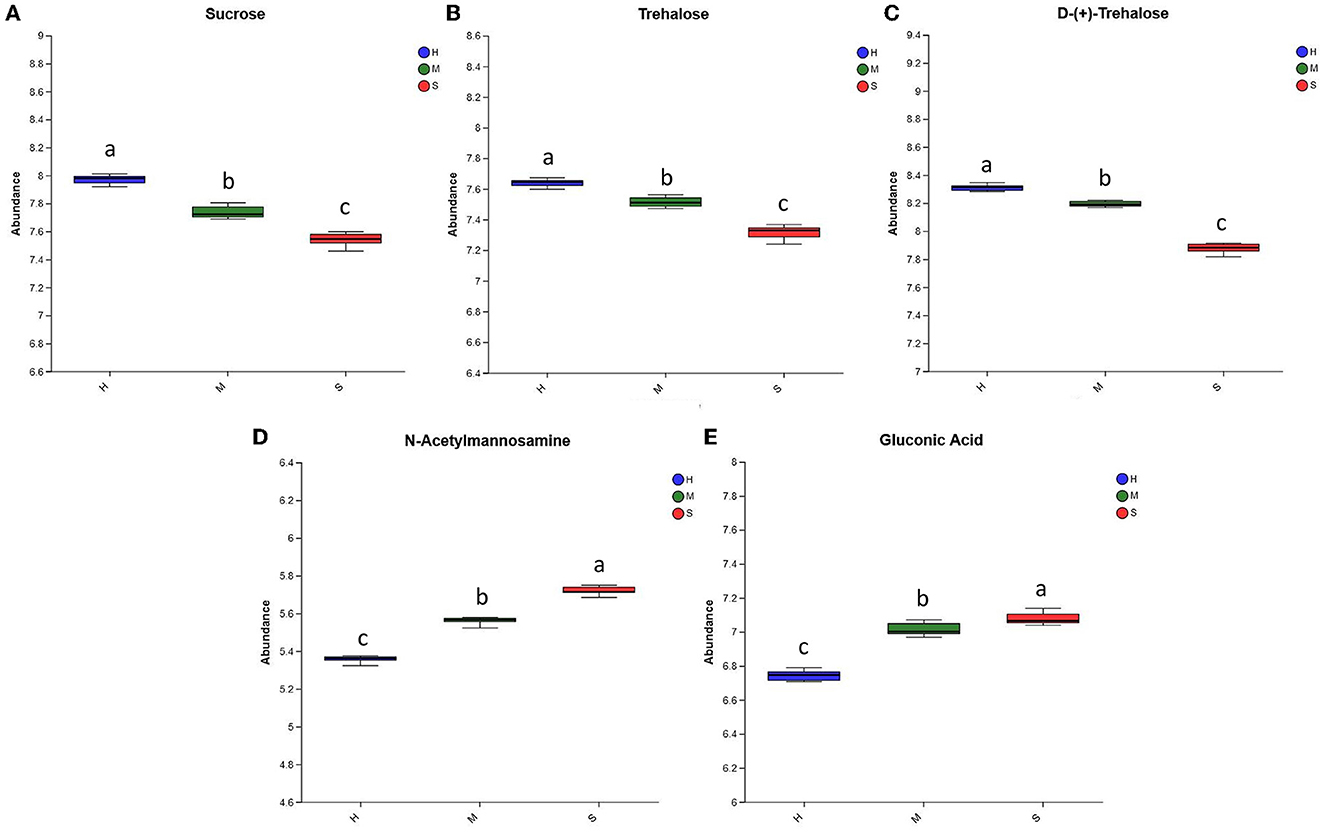
Figure 4. The relative abundance of the differential saccharides among the H, M, and S treatments. (A–E) The abundances of Sucrose, Trehalose, D-(+)-Trehalose, NAcetylmannosamine, and Gluconic Acid among the three treatments, respectively. The treatments containing the same letters indicated no significant differences (p > 0.05), while the treatments containing different letters indicated significant differences (p < 0.05).
3.3.2. Amino acids
Amino acids are important nutrients for P. palustre. Here, we identified 11 differential amino acids, including L-dopa, L-proline, L-alanine, L-glutamine, L-phenylalanine, L-threonine, L-(+)-arginine, beta-alanine, L-tryptophan, L-tyrosine, and aspartic Acid. As shown in Figure 5, the relative abundances of L-dopa and L-alanine in the H treatment were significantly lower than those of the M and S treatments, whereas the relative abundances of the other nine amino acids in the H treatment were dramatically higher than those of the M and S treatments. This suggested that the H treatment could promote the amino acid content of P. palustre. In particular, aspartic acid and glutamic acid are delicious amino acids, and alanine, serine, and glycine are sweet amino acids in P. palustre (22, 78). In our investigation, the abundances of aspartic acid and L-alanine in the H treatment were significantly increased compared with the M and S treatments, while the beta-alanine presented the lowest abundance in the H treatment.
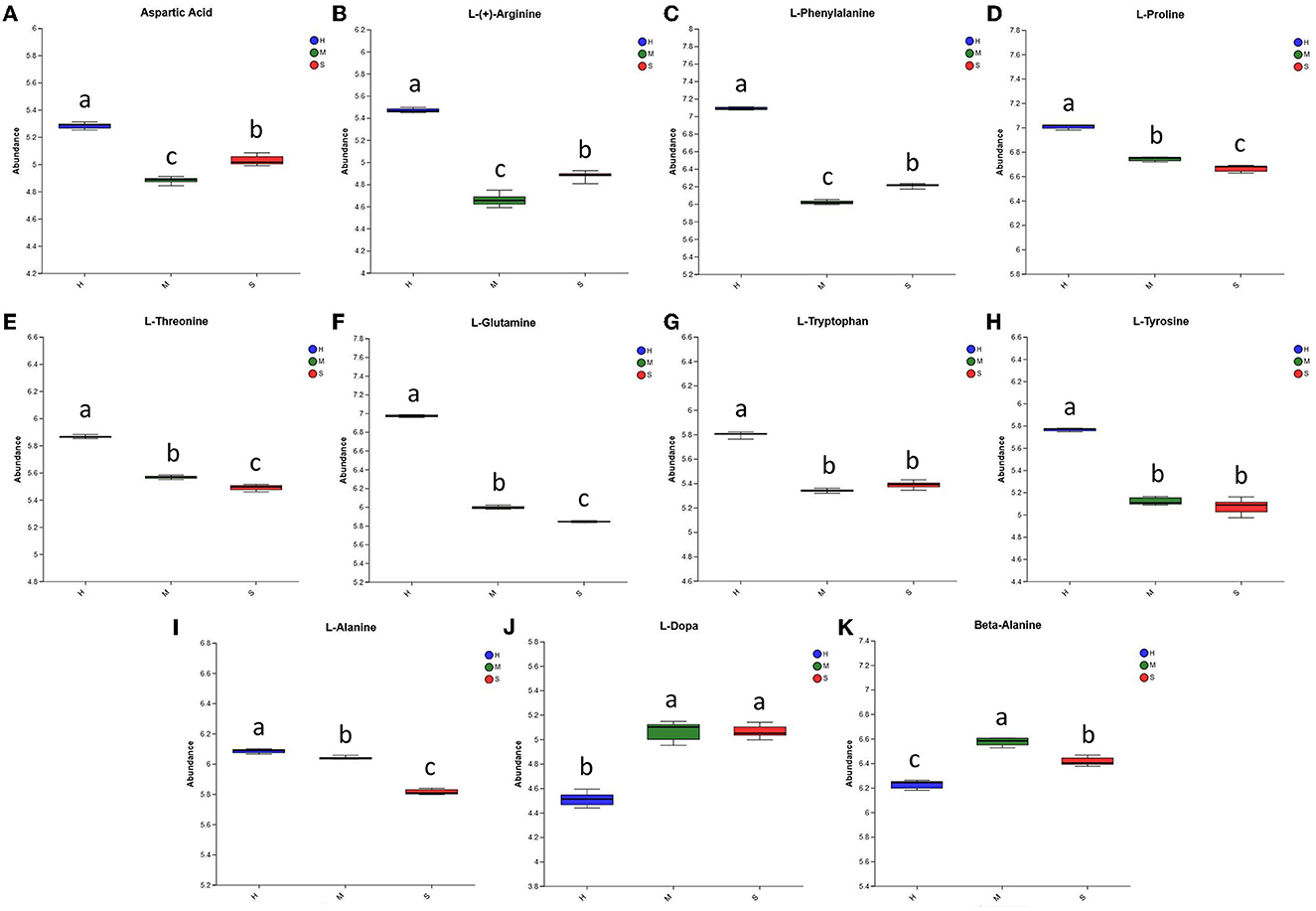
Figure 5. The relative abundance of the differential amino acids among the H, M, and S treatments. (A–K) The abundances of Aspartic Acid, L-(+)-Arginine, L-Phenylalanine, LProline, L-Threonine, L-Glutamine, L-Tryptophan, L-Tyrosine, L-Alanine, L-Dopa, and Beta-Alanine among the three treatments, respectively. The treatments containing the same letters indicated no significant differences (p > 0.05), while the treatments containing different letters indicated significant differences (p < 0.05).
3.4. Volatile compounds analysis based on HS-GC-MS
In this study, we also used the HS-GC-MS technique to investigate the effects of different processing treatments on the volatile components of P. palustre. The results showed that a total of 242 metabolites were identified by HS-GC-MS. The analysis of correlation, PCA, Venn, and PLS-DA indicated that the data were reliable and available (Supplementary Figure 7). Based on these metabolites, in total, 55 volatile substances were identified in HMDB, including organic oxygen compounds (17, 30.91%), lipids and lipid-like molecules (15, 27.27%), organic acids and derivatives (8, 14.55%), organoheterocyclic compounds (8, 14.55%), benzenoids (6, 10.91%), and hydrocarbons (1, 1.82%; Supplementary Figure 8A; Supplementary Table 4). Among these, carbonyl compounds, fatty acids and conjugates, and pyrazines accounted for almost half of the volatile substances (27/55; Supplementary Figure 8B).
3.5. Differential volatile substances among the different treatments
The different groups could be better distinguished by OPLS-DA analysis (Figures 6A–C). All the identified volatile substances were employed for screening differential metabolites according to the VIP > 1 and p < 0.05, and the results of the three comparison groups are shown in Figures 6D–F. The results showed that a total of 109 differential volatile substances were detected among the H, M, and S treatments (Supplementary Figure 8C). Of these, there were 70 (23 upregulated and 47 downregulated), 74 (20 upregulated and 54 downregulated), and 44 (22 upregulated and 22 downregulated) differential volatile substances in H vs. M, H vs. S, and S vs. M treatments, respectively (Supplementary Figures 8D–F). Based on these differential volatile substances, we identified 27 differential volatile substances in HMDB (Table 2), including lipids and lipid-like molecules (10, 37.04%), organic oxygen compounds (7, 25.93%), organoheterocyclic compounds (5, 18.52%), benzenoids (2, 7.41%), organic acids and derivatives (2, 7.41%), and hydrocarbons (1, 3.7%) under superclass classification or carbonyl compounds (6, 22.22%), fatty acids and conjugates (6, 22.22%), pyrazines (4, 14.81%), unknown (3, 11.11%), fatty alcohols (2, 7.41%), monoterpenoids (2, 7.41%), benzoic acids and derivatives (1, 3.7%), carboxylic acid derivatives (1, 3.7%), carboxylic acids (1, 3.7%), and olefins (1, 3.7%), under subclass classification (Supplementary Figure 9; Supplementary Table 5). Of these, there were 18, 17, and 11 differential volatile substances in H vs. M, H vs. S, and S vs. M comparisons, respectively. In addition, among the three treatments, there were 11, 9, and 7 differential volatile substances showing the highest abundance in the H, M, and S treatments, respectively (Table 2).
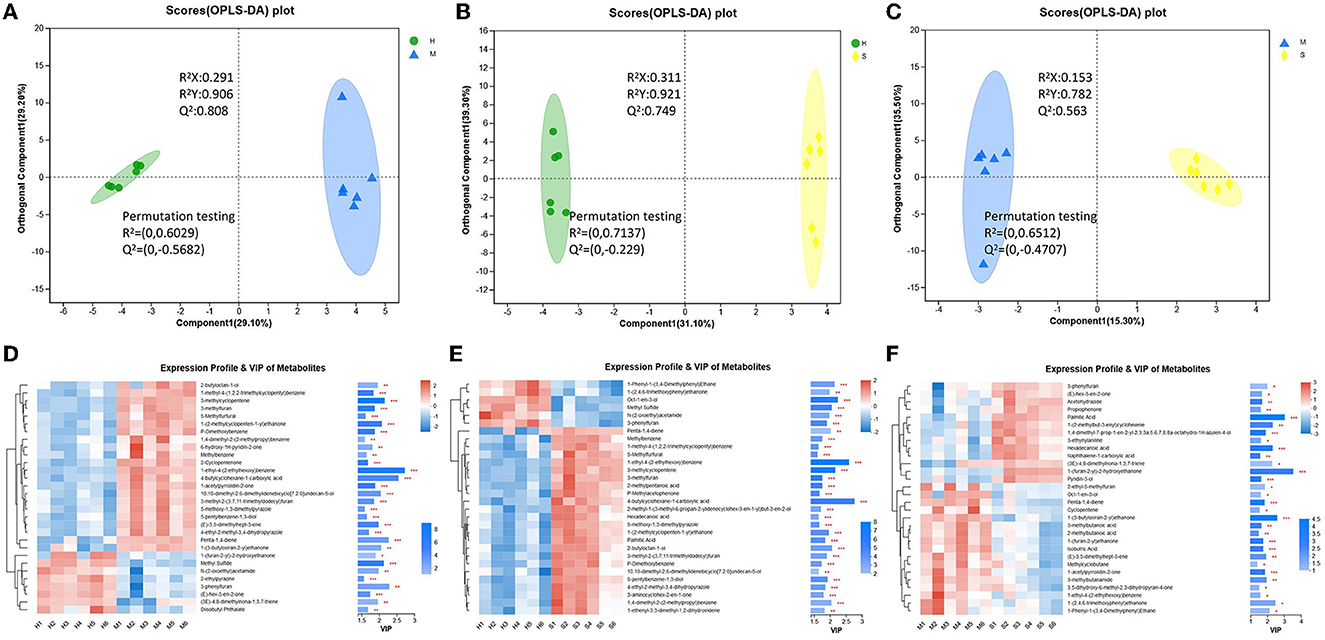
Figure 6. The OPLS-DA plot and expression profile and VIP of volatile substances. (A–C) The OPLSDA plot of different comparison groups. (D–F) The expression profile and VIP of volatile substances of different comparison groups.
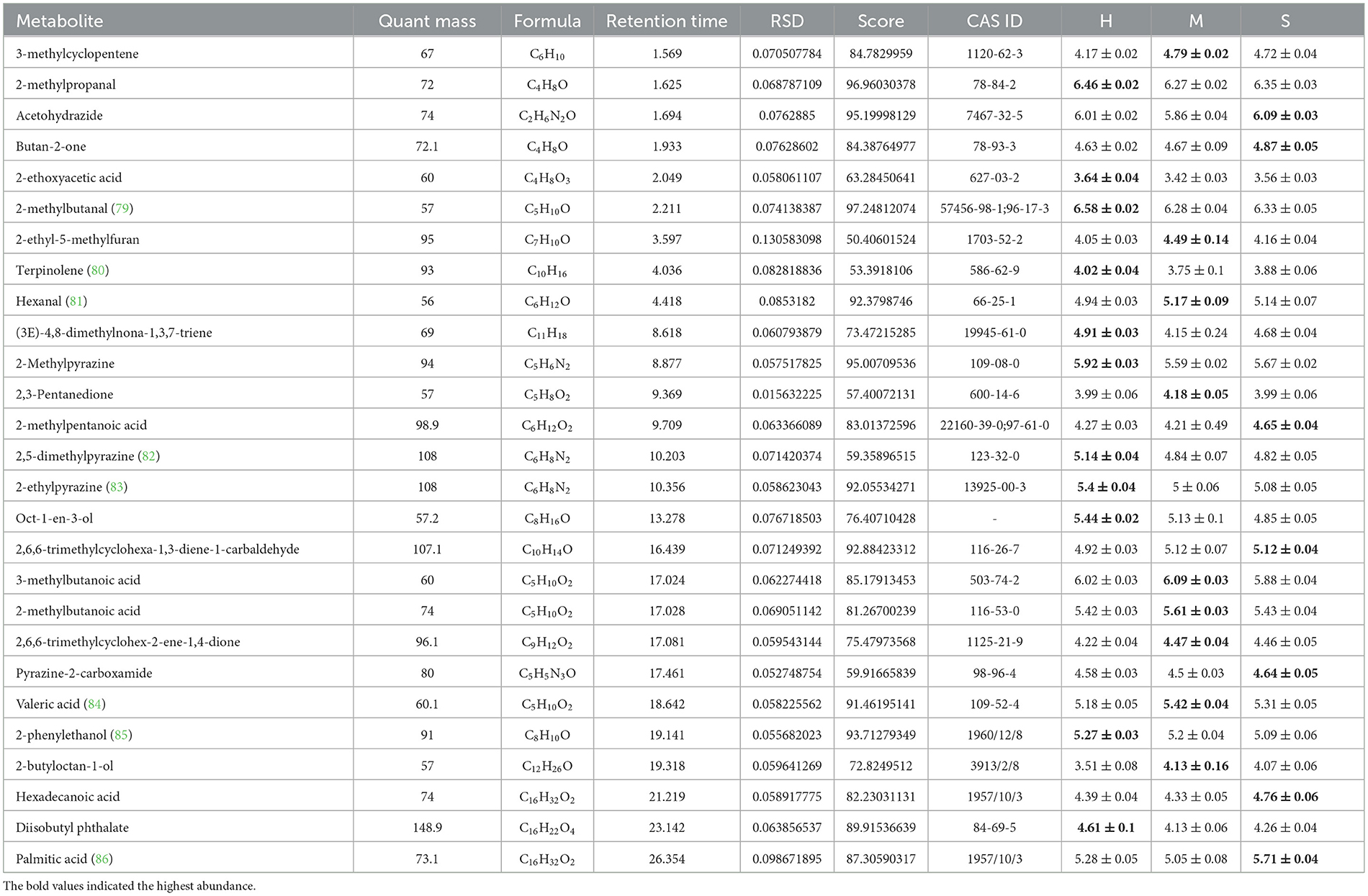
Table 2. Details of 27 differential metabolites identified by HS-GC-MS among the H, M, and S treatments.
4. Discussion
P. palustre mainly contains polysaccharides, flavonoids, triterpenoids, phenolic acid, etc. (11, 87). For evaluating the impact of different processing methods on the quality of P. palustre, we determined the contents of total sugar, soluble sugar, total pectin, and total flavonoids. In this study, we adopted three processing methods including sweating (M), tedding (S), and drying (H) to study the influences of different processing methods on the quality of P. palustre. The results indicated that the M treatment promoted the accumulation of the contents of total sugar, soluble sugar, and total pectin when compared with the H and S treatments (Figure 2). It was inferred that the M treatment could promote the quality of P. palustre. It was also similar to the conclusion of Zhang et al. (16). During the sweating process, the external nutrient supply of P. palustre was cut off, and the tissue cells used nutrients such as polysaccharides stored internally for life activities. The polysaccharides might undergo hydrolysis reactions under the catalysis of hydrolytic enzymes, resulting in reduced molecular weight and improved water solubility of polysaccharides, which were manifested by an increase in the water-soluble polysaccharides. However, the total flavonoid content in the M treatment significantly decreased in comparison with the H and S treatments. It was inferred that the M treatment was not favorable to the improvement of total flavonoids. It was consistent with the results of Lu (18). Overall, the M treatment favored the improvement of the polysaccharide quality of P. palustre.
The metabolites of each treatment were also analyzed based on LC-MS. The results revealed that a total of 174 metabolites included phospholipids, monosaccharides, fatty acids, carboxylic acids, eicosanoids, amino acids, and vitamins were presented (Supplementary Table 1). Tang et al. (2) identified 184 metabolites in M. chinensis Benth by using LC-MS detection, which contained carbohydrates and carbohydrate conjugates, fatty acids and conjugates, eicosanoids, and so on. It was inferred that the number and type of metabolites identified in the present study were similar to the study by Tang et al. (2). This indicated that LC-MS technology was stable and suitable for the identification of P. palustre metabolites. Furthermore, we identified 98 differential metabolites among the three treatments. Notably, a total of 28, 51, and 19 metabolites presented the highest abundance in the H, M, and S treatments, respectively (Table 1). We could observe that the M treatment could promote the abundance of more than half of the differential metabolites (51 out of 98) in comparison with the H and S treatments. It was indicated that the M treatment could significantly change the type and abundance of metabolites of P. palustre. These changes might have significant impacts on the quality and flavor of this herbal medicine.
The content of polysaccharides is an important index for evaluating the quality of P. palustre (11). Zhang et al. (88) found that P. palustre polysaccharides consisted of eight monosaccharides, including galacturonic acid, glucose, galactose, xylose, mannose, rhamnose, ribose, and glucuronic acid. In this study, the relative abundances of sucrose, gluconic acid, D-(+)-trehalose, trehalose, and N-acetylmannosamine among the H, M, and S treatments were identified and analyzed. The abundances of gluconic acid and N-acetylmannosamine under three treatments were ranked as S>M>H, and the abundances of D-(+)-trehalose, sucrose, and trehalose were ranked as H>M>S (Figure 4). This suggested that the M treatment was beneficial to the stability of the polysaccharide quality of P. palustre. Combined with the results in Figures 2, 4, the M treatment seemed to be more favorable for the stability and improvement of the quality of P. palustre polysaccharides, although it decreased the content of total flavonoids.
P. palustre is also rich in amino acids, and at least 18 types of amino acids including aspartic acid (Asp), threonine (Thr), serine (Ser), glutamic acid (Glu), glycine (Gly), cysteine (Cys), methionine (Met), valine (Val), isoleucine (Ile), tyrosine (Tyr), phenylalanine (Phe), lysine (Lys), histidine (His), arginine (Arg), proline (Pro), alanine (Ala), leucine (Leu), and tryptophan (Trp) were determined by Liu and Chen (89). Similarly, Su et al. (78) reported 17 types of amino acids in P. palustre from different production areas, which were almost the same with the results of Liu and Chen (89). In this study, we identified 11 differential amino acids, such as L-dopa, L-proline, L-alanine, L-glutamine, L-phenylalanine, L-threonine, L-(+)-arginine, beta-alanine, L-tryptophan, L-tyrosine, and aspartic Acid, and the abundances of nine amino acids significantly increased in the H treatment (Figure 5). It was indicated that the H treatments could significantly change the abundances of the most differential amino acids in P. palustre. This might be because that the relatively high-temperature condition (50°C) in the H treatment promoted the degradation of protein into amino acids, thereby increasing the expression abundance of amino acids, thus improving their nutritional levels. In addition, since Asp and Glu are delicious amino acids, and Ala, Ser, and Gly are sweet amino acids in P. palustre (78). The abundances of aspartic acid and L-alanine in the H treatment were significantly increased compared with the M and S treatments, indicating that the H treatment could promote the flavor level of P. palustre. Therefore, in general, the H treatment could improve the nutrition and flavor level of P. palustre.
Volatile oil, or essential oil, is a general term used for oily liquids that are volatile and can be distilled with steam (90), and has various effects such as antibacterial (91), anti-inflammatory (92), and antitumor (93). Volatile oil is widely found in herbal medicines such as P. palustre (17). Lu et al. (12) identified 24 types of volatile constituents from Taiwan Mesona chinensis Benth, while Xu and Wei (94) found 56, 54, and 58 types of volatile flavor compounds in Vietnam, Thailand, and Indonesia, respectively. Wei et al. (95) extracted and detected 59 volatile components from the water-soluble extract of Mesona Benth prepared by the solvent extraction method, while Chen et al. (96) identified 40 and 34 volatile components using supercritical CO2 extraction and hydrodistillation methods, respectively. Kung et al. (97) determined 56 and 108 volatile components using headspace solid-phase microextraction (HS-SPME) and simultaneous distillation–extraction (SDE), respectively. In this study, a total of 55 volatile substances were identified in HMDB by HS-GC-MS (Supplementary Table 4). It was inferred that different volatile components of P. palustre could be obtained from different varieties (or regional sources) and different extraction methods and technologies. Furthermore, the main volatile compounds identified in P. palustre were alkenes, alcohols, ketones, aldehydes, and phenols (95) or alkenes, ketones, alkanes, and fatty acids (98), or terpenoids and carbonyl compounds (94). In the present study, the volatile substances identified mainly included organic oxygen compounds (17, 30.91%), lipids and lipid-like molecules (15, 27.27%), organic acids and derivatives (8, 14.55%), organoheterocyclic compounds (8, 14.55%), benzenoids (6, 10.91%), and hydrocarbons (1, 1.82%; Supplementary Figure 8; Supplementary Table 4). In general, the volatile components identified in this study were similar to those previously reported, although there were some differences. This might be caused by the different experimental materials, different experimental treatments, and different sample pretreatments. In particular, we identified 27 differential volatile substances among the H, M, and S treatments, and there were 11, 9, and 7 differential volatile substances showing the highest abundance in the H, M, and S treatments, respectively (Table 2). It was inferred that different processing methods had a great impact on the volatile substances in P. palustre, especially the M treatment. The fermentation in the H treatment, the constant temperature (50°C) in the H treatment, and the variable temperature in the S treatment might account for the differences in volatile substances in P. palustre.
5. Conclusion
To study the effects of different processing methods on the quality, nutrition, and flavor of Platostoma palustre, we adopted the LC-MS and HS-GC-MS methods to compare the effects of tedding (S), sweating (M), and drying (H) on the metabolites and volatile substances of P. palustre. The results showed that the M treatment facilitated the stabilization and improvement of the polysaccharide and volatile substances quality of P. palustre, while the H treatment could promote the nutrition level of P. palustre. Considering the quality of polysaccharides and volatile substances, the M treatment was the optimal processing method for P. palustre. This study provides a theoretical reference for establishing standardized processing methods and maintaining the quality stability of P. palustre in future.
Data availability statement
The original contributions presented in the study are included in the article/Supplementary material, further inquiries can be directed to the corresponding author.
Author contributions
DT: conceptualization, methodology, data curation, formal analysis, writing—original draft, writing—reviewing and editing, and funding acquisition. FW: supervision, methodology, writing—reviewing and editing, and funding acquisition. CQ and SH: software, data curation, and formal analysis. All authors contributed to the article and approved the submitted version.
Funding
This study was supported by the Fund Projects of the Central Government in Guidance of Local Science and Technology Development (GuiKeZY22096020), the National Natural Science Foundation of China (82260750 and 82260749), the Appropriate Technology Development and Promotion Project of Guangxi Traditional Chinese Medicine Administration (GZSY23-07), Guangxi Elite Team of Medicinal Mlant Conservation (2023), and the Scientific Research Funding Project of Guangxi Botanical Garden of Medicinal Plants (GuiYaoJi202011).
Acknowledgments
The authors thank Guangxi Elite Team of Medicinal Plant Conservation (2023).
Conflict of interest
The authors declare that the research was conducted in the absence of any commercial or financial relationships that could be construed as a potential conflict of interest.
Publisher's note
All claims expressed in this article are solely those of the authors and do not necessarily represent those of their affiliated organizations, or those of the publisher, the editors and the reviewers. Any product that may be evaluated in this article, or claim that may be made by its manufacturer, is not guaranteed or endorsed by the publisher.
Supplementary material
The Supplementary Material for this article can be found online at: https://www.frontiersin.org/articles/10.3389/fnut.2023.1181942/full#supplementary-material
References
1. Tang DF, Lin Y, Wei F, Quan CQ, Wei KH, Wei YY, et al. Characteristics and comparative analysis of Mesona chinensis Benth chloroplast genome reveal DNA barcode regions for species identification. Funct Integr Genomics. (2022) 22:467–79. doi: 10.1007/s10142-022-00846-8
2. Tang DF, Huang QF, Wei KH, Yang XN, Wei F, Miao JH. Identification of differentially expressed genes and pathways involved in growth and development of Mesona chinensis Benth under red and blue light conditions. Front Plant Sci. (2021) 12:761068. doi: 10.3389/fpls.2021.761068
3. Tang DF, Quan CQ, Wei KH, Lin Y, Huang QF, Wei F, et al. Physio-morphological, biochemical and transcriptomic analyses provide insights into drought stress responses in Mesona chinensis Benth. Front Plant Sci. (2022) 13:809723. doi: 10.3389/fpls.2022.809723
4. Chinese Materia Medica. Editorial Board of Chinese Materia Medica of the State Administration of Traditional Chinese Medicine. Volume 19. Shanghai: Shanghai Science and Technology Press. (1999). p. 421.
5. Dictionary of Traditional Chinese Medicine. Nanjing University of Traditional Chinese Medicine. Shanghai: Shanghai Science and Technology Press. (2006).
6. Yen GC, Hung CY. Effects of alkaline and heat treatment on antioxidative activity and total henolics of extract from Hsian-tsao (Mesona procumbens Hemsl). Food ResInt. (2000) 33:487–92. doi: 10.1016/S0963-9969(00)00073-9
7. Yeha CT, Huang WH, Yen GC. Antihypertensive effects of Hsian-tsao and its active compound in spontaneously hypertensive rats. J Nutrit Biochem. (2009) 20:866–75. doi: 10.1016/j.jnutbio.2008.07.015
8. Liu FL, Feng CL. In vitro antibacterial test of Hsian-tsao (Mesona chinensis Benth) against avian Escherichia coli. Guangdong J Anim Vet Sci. (2008) 33:17–43.
9. Li DY, Lu G, Wang DD, Wang M. The influence of Xiancao hypolipidemic tea on the TC and TG metabolism of the experimental rabbits. Chin General Practice. (2010) 13:9–10.
10. Lin LH, Huang LC, Xie JH. Review on main chemical constituents and biological activities of Mesona chinensis. Sci Technol Food Industry. (2016) 37:356–9. doi: 10.13386/j.issn1002-0306.2016.20.063
11. Lin LS, Zhang S, Chan YL, Wang ZK, Lin HT. A review of chemical constituents and medicinal function of Mesona chinensis Benth. Current Biotechnology. (2013) 3:448–52. doi: 10.3969/j.issn.20952341.2013.06.14
12. Lu XY, Lin CQ, Lai ZM, Jiang XX, Yan P, Zhan RT. Analysis of volatile constituents from Mesona chinensis Benth. by HS- GC-MS. J Guangzhou Univ Trad Chin Med. (2021) 38:1025–31. doi: 10.13359/j.cnki.gzxbtcm.2021.05.029
13. Tang DF, Wei F, Quan CQ, Huang SH, Huang Y, Wei KH, et al. Codon usage bias and evolution analysis in the mitochondrial genome of Mesona chinensis Benth. Acta Physiologiae Plantarum. (2022) 44:118. doi: 10.1007/s11738-022-03453-z
14. Shi ML, Liu A, Chen YW. Study on the influence of Chinese medicinal materials and its processing on the efficacy of traditional Chinese drugs. Guid J Trad Chin Med Pharm. (2015) 21:26–8. doi: 10.13862/j.cnki.cn43-1446/r.2015.08.008
15. Hu HL, Wei YF, Ma XW, Pan L, Yan J, Lu JR. Effect of different processing methods on the contents of the main chemical constituents of Magnolia officinalis Cortex. Chin Trad Pat Med. (2011) 33:834–7.
16. Zhang P, Zheng ZA, Jiang QW. Effects of different pretreatment methods on quality and drying characteristics of Poria cocos after harvest. Trans Chin Soc Agri Eng. (2018) 34:294–304.
17. Liu BC, Chen JY, Huang YZ, Zhao YQ, Cai SZ. Current status and future development of Mesona chinensis Benth agriculture. Fujian J Agri Sci. (2015) 30:718–25. doi: 10.13651/j.cnki.fjnykj.2015.05.019
18. Lu XY. Study on Effects of Processing Methods on the Quality of Mesona chinensis Benth and Its Lipid-Lowering Activity in vitro. Guangzhou: Guangzhou University of Chinese Medicine (2020).
19. Xie M, Chen W, Lai X, Dai H, Sun H, Zhou X, et al. Metabolic responses and their correlations with phytochelatins in Amaranthus hypochondriacus under cadmium stress. Environ Pollut. (2019) 252:1791–800. doi: 10.1016/j.envpol.2019.06.103
20. Kong XG, Guo ZA, Yao Y, Xia LC, Liu RX, Song HF, et al. Acetic acid alters rhizosphere microbes and metabolic composition to improve willows drought resistance. Sci Tot Environ. (2022) 844:157132. doi: 10.1016/j.scitotenv.2022.157132
21. Dong XY, Mu QX, Song LL, Liu BY, Zhang LH, Wang YF. Study on discrimination of Decoction pieces from Rutaceae by HS-GC-MS. J Chin Med Mater. (2022) 45:2920–5.
22. Andreesen R, Osterholz J, Luckenbach GA, Costabel U, Schulz A, Speth V, et al. Tumor cytotoxicity of human macrophages after incubation with synthetic analogues of 2-lysophosphatidylcholine. J Natl Cancer I. (1984) 72:53–9. doi: 10.1093/jnci/72.1.53
23. Gorska-Ponikowska M, Kuban-Jankowska A, Daca A, Nussberger S. 2-Methoxyestradiol reverses the pro-carcinogenic effect of L-Lactate in Osteosarcoma 143B cells. Cancer Genom Proteom. (2017) 14:483–93. doi: 10.21873/cgp.20058
24. Whitehead A, Krause FN, Moran A, Maccannell A, Roberts LD. Brown and beige adipose tissue regulate systemic metabolism through a metabolite interorgan signaling axis. Nat Commun. (2021) 12:1905. doi: 10.1038/s41467-021-22272-3
25. Yang J, Zheng X, Haugen F, Darè E, Lvdahl C, Schulte G, et al. Adenosine increases LPS-induced nuclear factor kappa B activation in smooth muscle cells via an intracellular mechanism and modulates it via actions on adenosine receptors. Acta Physiol. (2013) 210:590–9. doi: 10.1111/apha.12176
26. Yamaguchi Y, Yamamoto K, Sato Y, Inoue S, Morinaga T, Hirano E. Combination of aspartic acid and glutamic acid inhibits tumor cell proliferation. Biomed Res-Tokyo. (2016) 37:153–9. doi: 10.2220/biomedres.37.153
27. Lee SY, Kim YC. Effect of beta-alanine administration on carbon tetrachloride-induced acute hepatotoxicity. Amino Acids. (2006) 33:543–6. doi: 10.1007/s00726-006-0450-7
28. Nakagawa Y, Nishikawa B, Miyagawa H. Effects of brassinolide on the growing of rice plants. J Pestic Sci. (2021) 46:274–7. doi: 10.1584/jpestics.D21-024
29. Fang K, Zhan Y, Zhu R, Wang YQ, Wu CQ, Sun M, et al. Bufalin suppresses tumour microenvironment-mediated angiogenesis by inhibiting the STAT3 signalling pathway. J Transl Med. (2021) 19:383. doi: 10.1186/s12967-021-03058-z
30. Manrique-Vergara D, González-Sánchez ME. Short chain fatty acids (butyric acid) and intestinal diseases. Nutr Hosp. (2017) 34:58–61. doi: 10.20960/nh.1573
31. Newman WH, Castresana MR, Webb JG, Wang Z. Cyclic AMP inhibits production of interleukin-6 and migration in human vascular smooth muscle cells. J Surg Res. (2003) 109:57–61. doi: 10.1016/S0022-4804(02)00038-0
32. Baswan SM, Leverett J, Pawelek J. Clinical evaluation of the lightening effect of cytidine on hyperpigmented skin. J Cosmet Dermatol-US. (2018) 18:278–85. doi: 10.1111/jocd.12784
33. Nazari-Robati M, Akbari M, Khaksari M, Mirzaee M. Trehalose attenuates spinal cord injury through the regulation of oxidative stress, inflammation and GFAP expression in rats. J Spinal Cord Med. (2018) 42:387–94. doi: 10.1080/10790268.2018.1527077
34. Jiménez MC, Sun Q, Schürks M, Chiuve S, Hu FB, Manson JE, et al. Low dehydroepiandrosterone sulfate is associated with increased risk of ischemic stroke among women. Stroke. (2013) 44:1784–9. doi: 10.1161/STROKEAHA.111.000485
35. Martiniova L, Field MS, Finkelstein JL, Perry CA, Stover PJ. Maternal dietary uridine causes, and deoxyuridine prevents, neural tube closure defects in a mouse model of folate-responsive neural tube defects. Am J Clin Nutr. (2015) 101:860–9. doi: 10.3945/ajcn.114.097279
36. Jiang S, Fan J, Wang Q, Ju DW, Feng MQ, Li JY, et al. Diosgenin induces ROS-dependent autophagy and cytotoxicity via mTOR signaling pathway in chronic myeloid leukemia cells. Phytomedicine. (2016) 23:243–52. doi: 10.1016/j.phymed.2016.01.010
37. Dreyer G, Kieswich J, Harwood S, Ahluwalia A, Yaqoob MM. Ergocalciferol improves endothelial vasodilatory and vasoconstrictor function in an in vivo model of mild uraemia. Bioscience Rep. (2019) 39:BSR20190711. doi: 10.1042/BSR20190711
38. Crescitelli MC, Rauschemberger MB, Cepeda S, Sandoval M, Massheimer VL. Role of estrone on the regulation of osteoblastogenesis. Mol Cell Endocrinol. (2019) 498:110582. doi: 10.1016/j.mce.2019.110582
39. Manaenko A, Fathali N, Chen H, Suzuki H, Williams S, Zhang JH, et al. Heat shock protein 70 upregulation by geldanamycin reduces brain injury in a mouse model of intracerebral hemorrhage. Neurochem Int. (2010) 57:844–50. doi: 10.1016/j.neuint.2010.09.001
40. Michiels J, Truffin D, Majdeddin M, Van Poucke M, Van Liefferinge E, Van Noten N, et al. Gluconic acid improves performance of newly weaned piglets associated with alterations in gut microbiome and fermentation. Porcine Health Manag. (2023) 9:10. doi: 10.1186/s40813-023-00305-1
41. de Oliveira ED, Schallenberger C, Böhmer AE, Hansel G, Schmidt AP. Mechanisms involved in the antinociception induced by spinal administration of inosine or guanine in mice. Eur J Pharmacol. (2015) 772:71–82. doi: 10.1016/j.ejphar.2015.12.034
42. Camargo A, Dalmagro AP, Fraga DB, Rosa JM, Zeni ALB, Kaster MP, et al. Low doses of ketamine and guanosine abrogate corticosterone-induced anxiety-related behavior, but not disturbances in the hippocampal NLRP3 inflammasome pathway. Psychopharmacology. (2021) 238:2555–68. doi: 10.1007/s00213-021-05879-8
43. Hörl WH, Kittel R, Heidland A. Effects of high doses of leucine and ketoleucine on glycogen and protein metabolism in acute uremia. Am J Clin Nutr. (1980) 33:1468–75. doi: 10.1093/ajcn/33.7.1468
44. Akar E, Emon ST, Uslu S, Orakdogen M, Somay H. Effect of L-Arginine therapy on vasospasm: Experimental study in rats. World Neurosurg. (2019) 132:e443–6. doi: 10.1016/j.wneu.2019.08.119
45. Dandare SU, Ezeonwumelu IJ, Shinkafi TS, Magaji UF, Adio AAI, Ahmad K. L-alanine supplementation improves blood glucose level and biochemical indices in alloxan-induced diabetic rats. J Food Biochem. (2020) 45:e13590. doi: 10.1111/jfbc.13590
46. Schmitt I, Kaut O, Khazneh H, deBoni L, Ahmad A, Berg D, et al. L-dopa increases α-synuclein DNA methylation in Parkinson's disease patients in vivo and in vitro. Movement Disord. (2015) 30:1794–801. doi: 10.1002/mds.26319
47. Rabe T, Przylipiak A, Grunwald K, Parta S, Przylipiak M, Runnebaum B. Action of leukotriene B4 in human granulosa cells in vitro. Hum Reprod. (1995) 10:1881–4. doi: 10.1093/oxfordjournals.humrep.a136201
48. Shao H, Dong L, Feng Y, Wang CH, Tong HX. The protective effect of L-glutamine against acute Cantharidin-induced Cardiotoxicity in the mice. BMC Pharmacol Toxicol. (2020) 21:71. doi: 10.1186/s40360-020-00449-8
49. Amin A, Frampton J, Liu Z, Franco-Becker G, Norton M, Alaa A, et al. Differential effects of L- and D-phenylalanine on pancreatic and gastrointestinal hormone release in humans: A randomized crossover study. Diabetes Obes Metab. (2020) 23:147–57. doi: 10.1111/dom.14204
50. Moradi M, Moradi B, Hashemian AH, Bakhtiari M, Khazaei M, Esmaeili F, et al. Beneficial effect of L-Proline supplementation on the quality of human spermatozoa. Andrologia. (2022) 54:e14486. doi: 10.1111/and.14486
51. Zhang H, Chen Y, Li Y, Zhang T, Ying ZX, Su WP, et al. l-Threonine improves intestinal mucin synthesis and immune function of intrauterine growth-retarded weanling piglets. Nutrition. (2018) 59:182–7. doi: 10.1016/j.nut.2018.07.114
52. van Zyl LT, Chung SA, Shahid A. L-tryptophan as treatment for pediatric non-rapid eye movement parasomnia. J Child Adol Psychop. (2018) 28:395–401. doi: 10.1089/cap.2017.0164
53. Hung CH, Chiu CC, Liu KS, Chen YW, Wang JJ. Subcutaneous L-tyrosine elicits cutaneous analgesia in response to local skin pinprick in rats. Eur J Pharmacol. (2015) 765:457–62. doi: 10.1016/j.ejphar.2015.09.010
54. Lee H, Jang JH, Kim SJ. Malonic acid suppresses lipopolysaccharide-induced BV2 microglia cell activation by inhibiting the p38 MAPK/NF-κB pathway. Anim Cells Syst. (2021) 25:110–8. doi: 10.1080/19768354.2021.1901781
55. Xu X, Sun X, Wan X, Chen X, Jiang X. Mitomycin induces alveolar epithelial cell senescence by down-regulating GSK3β signaling. Toxicol Lett. (2021) 352:61–9. doi: 10.1016/j.toxlet.2021.09.015
56. Kuwahara M, Ito K, Hayakawa K, Yagi S, Shiota K. N-Acetylmannosamine improves sleep-wake quality in middle-aged mice: Relevance to autonomic nervous function. Auton Neurosci-Basic. (2014) 187:56–62. doi: 10.1016/j.autneu.2014.11.005
57. Hwang J, Kim YR, Park JY, Nam WH, Kim J, Cho J, et al. Selective anticancer materials by self-assembly of synthetic amphiphiles based on N-Acetylneuraminic acid. ACS Appl Mater Inter. (2022) 14:16100–7. doi: 10.1021/acsami.2c02922
58. Cook TM, Brown KG, Boyle JV, Goss WA. Bactericidal action of nalidixic acid on Bacillus subtilis. J Bacteriol. (1966) 92:1510–4. doi: 10.1128/jb.92.5.1510-1514.1966
59. Blanchard C, Brooks L, Beckley A, Colquhoun J, Dewhurst S. Neomycin sulfate improves the antimicrobial activity of mupirocin-based antibacterial ointments. Antimicrob Agents Ch. (2015) 60:862–72. doi: 10.1128/AAC.02083-15
60. Levy B, Clere-Jehl R, Legras A, Morichau-Beauchant T, Leone M, Frederique G, et al. Epinephrine versus norepinephrine for cardiogenic shock after acute myocardial infarction. J Am Coll Cardiol. (2018) 72:173–82. doi: 10.1016/j.jacc.2018.04.051
61. Valvassori SS, Dal-Pont GC, Varela RB, Resende WR, Quevedo J. Ouabain induces memory impairment and alter the BDNF signaling pathway in an animal model of bipolar disorder: Cognitive and neurochemical alterations in BD model. J Affect Disord. (2020) 282:1195–202. doi: 10.1016/j.jad.2020.12.190
62. Callegari EA, Ferguson-Gottschall S, Gibori G. PGF2alpha induced differential expression of genes involved in turnover of extracellular matrix in rat decidual cells. Reprod Biol Endocrinol. (2005) 3:3. doi: 10.1186/1477-7827-3-3
63. Zheng Y, Martin-Morales A, Wang J, Fujishima M, Okumura E, Sato K. Phenethylamine in chlorella alleviates high-fat diet-induced mouse liver damage by regulating generation of methylglyoxal. NPJ Sci Food. (2021) 5:22. doi: 10.1038/s41538-021-00105-3
64. Negintaji K, Ghanbari A, Frozanfar M, Jafarinia M, Zibara K. Pregnenolone enhances the proliferation of mouse neural stem cells and promotes oligodendrogenesis, together with Sox10, and neurogenesis, along with Notch1 and Pax6. Neurochem Int. (2023) 163:105489. doi: 10.1016/j.neuint.2023.105489
65. Toppozada MK, Darwish E, Barakat AA. Management of severe preeclampsia detected in early labor by prostaglandin A1 or dihydralazine infusions. Am J Obstet Gynecol. (1991) 164:1229–32. doi: 10.1016/0002-9378(91)90688-N
66. Honma Y, Arai I, Hashimoto Y, Futaki N, Sugimoto M, Tanaka M, et al. Prostaglandin D2 and prostaglandin E2 accelerate the recovery of cutaneous barrier disruption induced by mechanical scratching in mice. Eur J Pharmacol. (2005) 518:56–62. doi: 10.1016/j.ejphar.2005.06.006
67. Najafpour A, Mohammadi R, Faraji D, Amini K. Local administration of prostaglandin E1 combined with silicone chamber improves peripheral nerve regeneration. Int J Surg. (2013) 11:1010–5. doi: 10.1016/j.ijsu.2013.05.034
68. Salera D, Argalia G, Giuseppetti GM. Hemodynamic effects of a prostacyclin analog (Prostavasin) in systemic scleroderma patients. Radiol Med. (2005) 110:106–14.
69. Mahmoud A, Tabassum S, Al Enazi S, Lubbad N, Benini R. Amelioration of levetiracetam-induced behavioral side effects by pyridoxine. A randomized double blind controlled study. Pediatr Neurol. (2021) 119:15–21. doi: 10.1016/j.pediatrneurol.2021.02.010
70. Das R, Qubty W. Retrospective observational study on riboflavin prophylaxis in child and adolescent migraine. Pediatr Neurol. (2020) 114:5–8. doi: 10.1016/j.pediatrneurol.2020.09.009
71. Beauchamp LC, Liu XM, Sedjahtera A, Bogeski M, Vella LJ, Bush AI, et al. S-Adenosylmethionine rescues cognitive deficits in the rTg4510 animal model by stabilizing protein phosphatase 2A and reducing phosphorylated Tau. J Alzheimers Dis. (2020) 77:1705–15. doi: 10.3233/JAD-200756
72. Diniz DA, Petrocchi JA, Navarro LC, Souza TC, Castor MGME, Duarte IDG, et al. Serotonin induces peripheral antinociception via the opioidergic system. Biomed Pharmacother. (2017) 97:1434–7. doi: 10.1016/j.biopha.2017.11.048
73. Gao HX, Gao HJ, Xu GH, Li M, Du SZ, Li F, et al. Efficacy and safety of repeated oral sucrose for repeated procedural pain in neonates: A systematic review. Int J Nurs Stud. (2016) 62:118–25. doi: 10.1016/j.ijnurstu.2016.07.015
74. Guerrero JG, Ishida E, Shibusawa N, Lei X, Yamada S, Horiguchi K, et al. Role of thyrotropin-releasing hormone in regulating fibroblast growth factor 21 in mouse pancreatic β Cells. Thyroid. (2023) 33:251–60. doi: 10.1089/thy.2022.0144
75. Yan ZY, Zhao MR, Huang CY, Zhang LJ, Zhang JX. Trehalose alleviates high-temperature stress in Pleurotus ostreatus by affecting central carbon metabolism. Microb Cell Fact. (2021) 20:82. doi: 10.1186/s12934-021-01572-9
76. Ramesh D, Vijayakumar BG, Kannan T. Therapeutic potential of uracil and its derivatives in countering pathogenic and physiological disorders. Eur J Med Chem. (2020) 207:112801. doi: 10.1016/j.ejmech.2020.112801
77. Al N, Çakir A, Koç C, Cansev M, Alkan T. Antioxidative effects of uridine in a neonatal rat model of hyperoxic brain injury. Turk J Med Sci. (2020) 50:2059–66. doi: 10.3906/sag-2002-14
78. Su HL, Chen JY, Huang YZ. Quantitative analysis of amino acids in different areas of Mesona Chinensis. In: Proceedings and Abstracts of the 9th National Symposium on Natural Medicinal Material Resources. Guangzhou; Huazhou (2010). p. 523–6.
79. Aarthi R, Saranya R, Sankaran K. 2-methylbutanal, a volatile biomarker, for non-invasive surveillance of Proteus. Appl Microbiol Biotechnol. (2013) 98:445–54. doi: 10.1007/s00253-013-5393-9
80. Ito K, Ito M. The sedative effect of inhaled terpinolene in mice and its structure-activity relationships. J Nat Med. (2013) 67:833–7. doi: 10.1007/s11418-012-0732-1
81. Kaur K, Kaur G, Brar JS. Pre-harvest application of hexanal formulations for improving post-harvest life and quality of mango (Mangifera indica L) cv Dashehari. J Food Sci Technol. (2020) 57:4257–64. doi: 10.1007/s13197-020-04464-9
82. Yamada K, Sano M, Fujihara H, Ohta A. Effect of 2,5-dimethylpyrazine on uterine contraction in late stage of pregnant female rats. Biol Pharm Bull. (2003) 26:1614–7. doi: 10.1248/bpb.26.1614
83. Ashigai H, Ikeshima E, Koizumi K, Nakashima K, Mizutani M, Yajima H. 2-Ethylpyrazine induces vasodilatation by releasing nitric oxide in the Endothelium. Biol Pharm Bull. (2017) 40:2153–7. doi: 10.1248/bpb.b17-00551
84. Han R, Nusbaum O, Chen X, Zhu Y. Valeric acid suppresses liver cancer development by acting as a novel HDAC inhibitor. Mol Ther Oncolytics. (2020) 19:8–18. doi: 10.1016/j.omto.2020.08.017
85. Majdabadi N, Falahati M, Heidarie-Kohan F, Farahyar S, Rahimi-Moghaddam P, Ashrafi-Khozani M, et al. Effect of 2-Phenylethanol as antifungal agent and common antifungals (Amphotericin B, Fluconazole, and Itraconazole) on candida ppecies isolated from chronic and recurrent cases of Candidal Vulvovaginitis. ASSAY Drug Dev Techn. (2018) 16:141–9. doi: 10.1089/adt.2017.837
86. Vazquez-Jimenez JG, Chavez-Reyes J, Romero-Garcia T, Zarain-Herzberg A, Valdes-Flores J, Galindo-Rosales JM, et al. Palmitic acid but not palmitoleic acid induces insulin resistance in a human endothelial cell line by decreasing SERCA pump expression. Cell Signal. (2015) 28:53–9. doi: 10.1016/j.cellsig.2015.10.001
87. Zhao ZG, Shi YP, Huang NZ, Fu CM, Tang FL, Jiang QY. The research advances on Mesona chinensis Benth in China. J Southern Agri. (2011) 42:657–60. doi: 10.16213/j.cnki.scjas.2011.04.036
88. Zhang WB, Wang ZC, Zhang LY, Qian JH. Analysis of monosaccharide composition and content in Mesona Chinensis Benth polysaccharides by precolumn derivatization ultra-high performance liquid chromatography-tandem quadrupole mass spectrometry. J Instrument Anal. (2013) 32:143–9.
89. Liu XG, Chen MM. Research on the exploitation and utilization of Mesona blume in China. Food Res Dev. (2004) 5:109–14. doi: 10.3969/j.issn.1005-6521.2004.05.040
90. Huang LS, Gu YF, Li H. Advances in herbal volatile oil and aromatic herbs. China J Chin Materia Medica. (2009) 34:1605–11.
91. Zhu MF, Tang Y, Zheng Q, Tang DF, Luo J, Hu PY, et al. Effects of different extraction methods on composition and antibacterial activity of volatile oil from Forsythiae Fructus, Schinzonepetae Herba, and Menthae Haplocalycis Herba. Chin Trad Herbal Drugs. (2018) 49:2845–54. doi: 10.7501/j.issn.0253-2670.2018.12.017
92. Li SM, Zeng BY, Ye Q, Ao H, Li HX. Correlation analysis between GC-MS fingerprint of essential oil of Amomi Fructus and anti-inflammatory activity. Chin J Exp Trad Med Formulae. (2015) 21:133–6. doi: 10.13422/j.cnki.syfjx.2015090133
93. Han F, Zeng L, Wu ZF, Shu JC, Zhao ZD, Xiong W, et al. Analysis of the application and current situation of anti-tumor Chinese medicine volatile oils by searching “The compilation of Chinese Medicine Standard” database. Chin Pharmaceut J. (2017) 15:1376–80. doi: 10.11669/cpj.2017.15.019
94. Xu CH, Wei J. Comparative analysis of the volatile aroma compounds of Mesona Benth in the different regions. Modern Food. (2016) 11:1–5. doi: 10.16736/j.cnki.cn41-1434/ts.2016.11.001
95. Wei J, Zheng EL, Cai XK, Ji XD, Xu CH. Preparation of water-soluble extracts from Mesona Benth and analysis of the volatile aroma components by GC-MS. Food Sci Technol. (2014) 39:190–2. doi: 10.13684/j.cnki.spkj.2014.05.044
96. Chen FL, Xin XF, Tang QF. Extraction and GC-MS analysis of essential oil from Mesona chinensis Benth by Supercritical CO2 extraction and hydrodistillation methods. J Chin Med Mater. (2012) 8:1270–3.
97. Kung TL, Chen YJ, Chao LK, Wu CS, Lin LY, Chen HC. Analysis of volatile constituents in Platostoma palustre (Blume) using headspace solid-phase microextraction and simultaneous distillation-extraction. Foods. (2019) 8:415. doi: 10.3390/foods8090415
Keywords: Platostoma palustre, processing methods, LC-MS, HS-GC-MS, metabolites, volatile substances
Citation: Tang D, Quan C, Huang S and Wei F (2023) Integrating LC-MS and HS-GC-MS for the metabolite characterization of the Chinese medicinal plant Platostoma palustre under different processing methods. Front. Nutr. 10:1181942. doi: 10.3389/fnut.2023.1181942
Received: 08 March 2023; Accepted: 18 April 2023;
Published: 19 May 2023.
Edited by:
Leandro De Morais Cardoso, Juiz de Fora Federal University, BrazilReviewed by:
Nannan Zhang, Guangdong Academy of Sciences, ChinaNguyen Cong-Hau, Nguyen Tat Thanh University, Vietnam
Mohamed Ashour, National Institute of Oceanography and Fisheries (NIOF), Egypt
Copyright © 2023 Tang, Quan, Huang and Wei. This is an open-access article distributed under the terms of the Creative Commons Attribution License (CC BY). The use, distribution or reproduction in other forums is permitted, provided the original author(s) and the copyright owner(s) are credited and that the original publication in this journal is cited, in accordance with accepted academic practice. No use, distribution or reproduction is permitted which does not comply with these terms.
*Correspondence: Fan Wei, d2ZtYW51c2NyaXB0QDE2My5jb20=