- 1College of Pharmaceutical Science, Dali University, Dali, China
- 2Formula-Pattern Research Center, School of Traditional Chinese Medicine, Jinan University, Guangzhou, Guangdong, China
Lotus is a famous plant of the food and medicine continuum for millennia, which possesses unique nutritional and medicinal values. Polysaccharides are the main bioactive component of lotus and have been widely used as health nutritional supplements and therapeutic agents. However, the industrial production and application of lotus polysaccharides (LPs) are hindered by the lack of a deeper understanding of the structure–activity relationship (SAR), structural modification, applications, and safety of LPs. This review comprehensively comments on the extraction and purification methods and structural characteristics of LPs. The SARs, bioactivities, and mechanisms involved are further evaluated. The potential application and safety issues of LPs are discussed. This review provides valuable updated information and inspires deeper insights for the large scale development and application of LPs.
1. Introduction
Lotus, also known as Kamala, water lily, and sacred lotus, is a well-known traditional edible and medicinal aquatic monocotyledonous plant (1), which is broadly distributed in Asia, Oceania, and America (Figure 1C). Its edible parts mainly include seed, root, and leaf (Figure 1A). Owing to its nutritional characteristics, lotus has been used in food for 7,000 years in Asia (2). Since ancient times, various parts of the lotus have been utilized as dietary supplements and herbal medicine in China (3, 4). The medicinal values of lotus can be traced back to the ‘Shen Nong Ben Cao Jing (Shen Nong’s Classic of the Materia Medica),’ written between AD 200–300, which stated that “long-lasting intake of the lotus may lead to agility and longevity” (5). Numerous studies have demonstrated that lotus can be used for many symptoms, such as hypertension, depression, insomnia, cancer, cardiac ailments, and diarrhea (6).
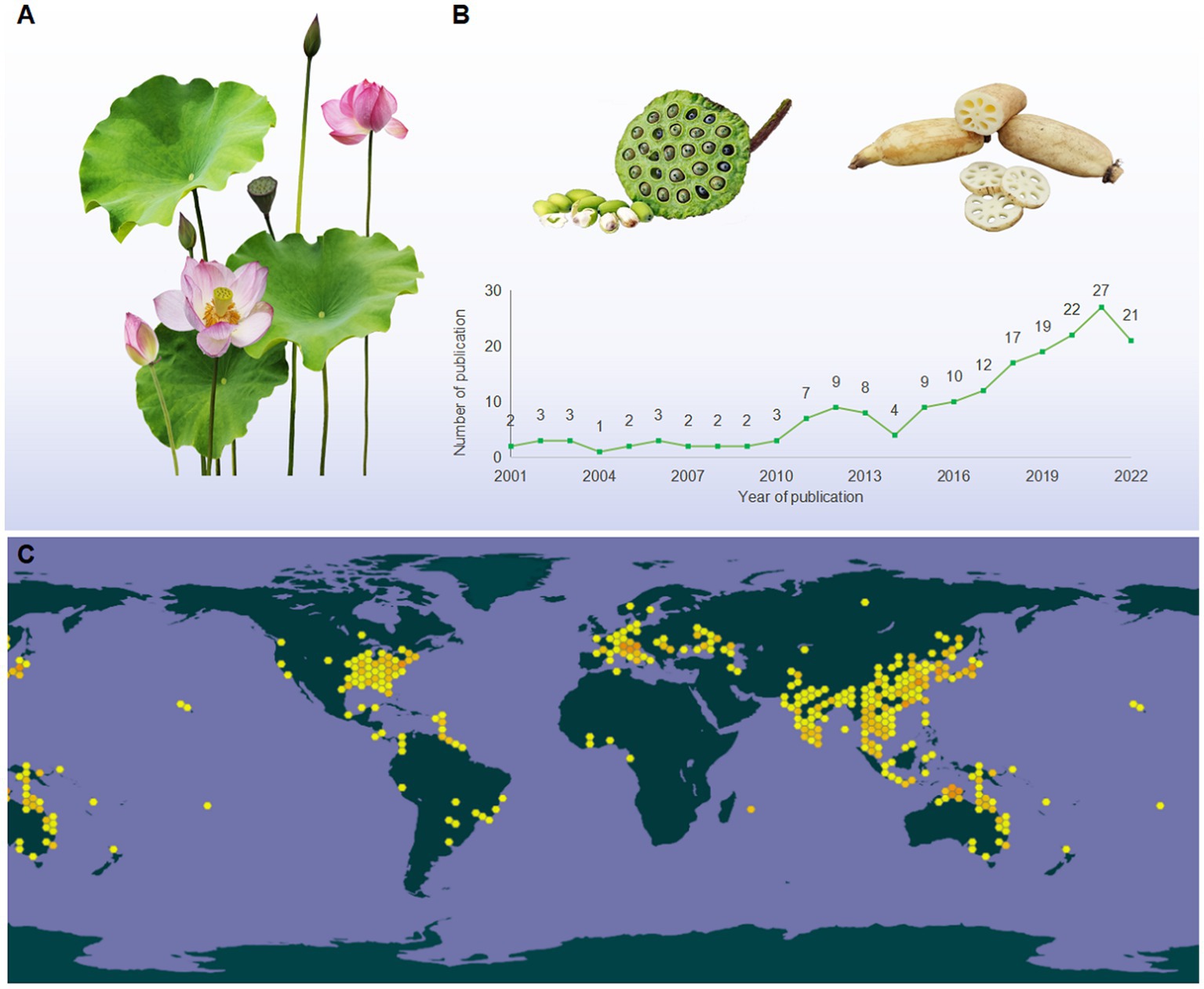
Figure 1. (A) The morphological characteristics of lotus; (B) the tendency in the number of publications relevant to LPs (2001 from 2022), taken from the database of the web of science (http://apps.webofknowledge.com), topics entered: “lotus polysaccharides” and “Nelumbo nucifera polysaccharides”; (C) the global distribution map of lotus (https://www.gbif.org/species/2520108).
The bioactivities of lotus are closely associated with their phytochemical compositions, which mainly referring to polysaccharides, alkaloids, saponins, and phenolic compounds (1, 6). Among these, polysaccharides have been considered as one of the major bioactive constituents of lotus (6). Previous studies have shown that lotus polysaccharides (LPs) was typical acidic heteropolysaccharide composed mainly of Fuc, Glc, Ara, Gal, Man, Xyl Rha, and GalA with small amounts of Rib, Fru, and GlcA (7). Within the past two decades, various potential bioactivities of LPs have been widely evaluated in vitro and in vivo, such as antioxidant, immunomodulatory, anti-inflammatory, antidiabetes, antitumor, antibacterial, antiobesity, and antiosteoporosis (8–10). The health benefits associated with LPs, there is a growing interest in their applications in industries focused on functional foods, dietary supplements, pharmaceuticals, and medical products (1).
According to the Web of Science database, a total of 188 articles on LPs have been published during 2001 and 2022 (Figure 1B). These studies primarily focused on extraction, purification, structural characterization, and bioactivity (9, 11, 12). To our knowledge, the most recent review articles regarding the extraction, purification, structures, and bioactivities of LPs have been summarized (7, 13). However, the detailed information on safety issues, structural modification, and structure–activity relationships (SARs) of LPs remain unclear. Moreover, the molecular mechanisms underlying the bioactivities of LPs have not been elaborated, particularly their anti-inflammatory mechanisms and immune regulation. These shortages may hinder the further utilization of LPs. Herein, an overview of the extraction, purification methods, and structural characteristics of LPs are conceived. Besides, the safety issues and structural modification that have never been emphasized are highlighted. Finally, the impact of chemical modification on the biological activity of LPs was comparatively discoursed, and the molecular mechanisms of bioactivities were summarized and discussed.
2. Extraction
Extraction is the initial and critical step in preparing active polysaccharides (14). Typically, the extraction procedure of crude LPs is as follows. Firstly, the dried plant materials are crushed into powder and sieved, then colored substances, lipids, and small molecules of the powder are removed by refluxing extraction with petroleum ether or ethanol. Then residues are extracted using water or organic solvents at different temperatures, followed by filtrating. Finally, the filtrates are collected, concentrated, deproteinized, decolorized, dialyzed, and lyophilized.
Conventional methods, including hot water extraction (HWE) (15–20), acidic extraction (21), and alkali extraction (22), have been applied to extract LPs. HWE is the most commonly utilized technique due to its simplicity, safety, and ease of implementation (23). However, this method has several drawbacks, such as low extraction efficiency, significant energy consumption, and extensive time requirements, etc. (24). In contrast, acidic/alkali extraction can enhance extraction efficiency by destroying the structure of plant cell walls structures (23). Nevertheless, adding a dilute acidic or alkali solution can easily cause degradation and reduction of polysaccharides activities (25). In short, although the traditional extraction methods have definite advantages, they also have significant disadvantages.
Novel and effective extraction techniques have been developed to overcome the limitations of these methods. Specifically, ultrasonic-assisted extraction (UAE) (26–28), microwave-assisted extraction (MAE) (29), ultrasound-MAE (UMAE) (30), dynamic high-pressure micro fluidization-assisted extraction (DHPMAE) (31, 32), enzyme-assisted extraction (EAE) (33–35), and deep eutectic solvent-assisted extraction (DESAE) (12, 36) have been implemented to extraction LPs. UAE utilizes the cavitation effect and strong shear forces by applying ultrasound to enhance the extraction ability of LPs (37). This technique leads to shorter extraction time, lower extraction temperatures, and reduced environmental contamination (38). However, applying high-energy ultrasound during extraction can cause permanently alter the structures and activities of LPs due to rapidly forming and collapsing cavitation bubbles within the liquid medium (25).
MAE utilizes microwaves to penetrate plant cells, increase intracellular temperature, and disrupt cell wall structures, thereby improving extraction efficiency. This method offers the advantages of efficiency, energy-conserving, and eco-friendly (39). However, MAE requires expensive equipment and may be unsuitable for large-scale LPs extraction (40). DHPMAE employs collective forces such as shear, high-frequency vibration, cavitation, instantaneous pressure drops, and high pressure (up to 200 MPa) to enhance extraction yield and efficiency (41), which provides mild extraction conditions, a high extraction rate, and reduced impurities. However, it consumes a significant amount of energy (42).
EAE has gained considerable popularity in LPs extraction due to its efficiency, energy-conserving features, and eco-friendly nature. The addition of enzymes promotes the degradation of cell walls and facilitates the release of LPs, resulting in excellent extraction efficiency (43). However, strict control of extraction conditions, including temperature, pH, extraction time, and enzyme dosage, is necessary to implement this method (25). In recent years, DESAE has attracted attention for its low economic cost, safety, biodegradability, and high solvent dissolution ability, which enhances extraction rates by increasing the solubility of LPs in solvents (36). However, it is challenging to separate LPs from solvents, limiting the industrial applications of DESAE (44).
In addition to the extraction method, conditions, such as solid–liquid ratio, extraction temperatures, and extraction times, also influence the extraction rate of LPs (45). Single-factor and orthogonal experiments have indicated that the order of extraction rate is extraction temperature > solid–liquid ratio > extraction time (21). Notably, there are distinct differences in the extraction methods and conditions for different plant parts. HWE has an extraction volume that follows the order: lotus leaf > lotus root > lotus seed > lotus plumula (46, 47). Moreover, the acid/alkali extraction method is exclusively applied to extract LPs from seeds, leaves, and roots, which may be attributed to the presence of acidic or alkaline groups in these polysaccharides (21, 22).
In addition, the yield and bioactivity of LPs are intimately related to their raw materials, extraction methods, and extraction conditions (45). For example, Peng et al. (30) extracted three seed LPs using different methods (HWE, UAE, and UMAE). The results revealed that UMAE exhibited the highest extraction yield (9.78%), followed by HWE (8.13%) and UAE (1.68%), indicating that the choice of extraction method could influence the yield of LPs. Moreover, the extraction methods and conditions can also influence the bioactivities of polysaccharides. Xing et al. (48) extracted four leaf LPs through water, ultrasonic, enzymatic, and alkaline extraction, and subsequently assessed their antioxidant activity. They found that the polysaccharide obtained by water extraction showed the most potent antioxidant activity. Song et al. (16) used amylase, cellulose, pectinase, and protease to extract four leaf LPs (LLEP-A, LLEP-C, LLEP-P, and LLEP-PR) and found that LLEP-P from pectinase extraction significantly improved the immune responses of macrophages in vitro. Thus, the selection of an appropriate extraction approach is crucial for obtaining polysaccharides that align with the specific objectives of the experiment.
3. Purification
During the process of LPs extraction, impurities, such as proteins, pigments, and other small molecules, are co-extracted (49). Thus, removing co-existing impurities is required before the separation of LPs. Generally, the proteins in LPs were commonly removed by Sevag, trichloroacetic acid, and enzymatic methods (50–52). And the first two approaches have been commonly used to remove the free protein of LPs. However, these two approaches have several shortcomings, such as inefficiency, complicated operation, and a substantial decrease in the content of LPs. Sevag combined with enzymatic methods can overcome these weaknesses (30, 32, 53). Besides, pigments can oxidize LPs, influencing chromatographic and structural analysis. Thus, removing pigments is a critical step in the process of purification. So far, various methods, such as activated carbon adsorption (50), hydrogen peroxide (H2O2) (54), and microporous resins (29), have been developed to remove the pigments of LPs. H2O2 and microporous resins were the most common approaches to remove the pigments of LPs, whereas the activated carbon adsorption method is not routinely applied because of the low efficiency and residual effect.
Crude polysaccharides are complex mixtures composed of different degrees of polymerized polysaccharides. Thus, additional purification and separation steps are crucial for investigating their structural characteristics (45). The purification and separation techniques commonly employed for LPs include membrane separation, ethanol precipitation, and column chromatography. In membrane separation, different membranes, such as microfiltration, nanofiltration, ultrafiltration, and classical osmosis membranes, were utilized to separate LPs (55). Ethanol precipitation is the most commonly used method, especially suitable for separating polysaccharides with significant differences in molecular weight (Mw) and solubility (56). Column chromatography is one of the most efficient approaches for separating polysaccharides (57). Ion exchange chromatography (IEC) and gel filtration chromatography (GFC) are commonly utilized to purify LPs. Water, different concentrations of sodium chloride (NaCl) solution, or phosphate buffer are frequently employed as eluents in both methods. In general, IEC is employed for purifying neutral or acidic polysaccharides (58), with diethylaminoethyl (DEAE)-cellulose 52, DEAE-Sephadex A-25, and DEAE Sepharose FF being commonly used chromatographic media (9, 52, 59). GFC is commonly used to purify polysaccharides of different Mw with Sephadex G-200, Sephadex G-150, or Sephadex G-100 as chromatographic media. Generally, obtaining pure polysaccharides using a single method is challenging. Thus, combining IEC and GFC is often employed to achieve superior purification (40). In summary, the schematic representation of the extraction and purification processes of LPs is shown in Figure 2.
4. Structural characteristics
Polysaccharide is one of the vital bioactivity constituents of lotus, which shows diversified and complicated structural characteristics (60). To better understand the LPs, it is necessary to explain their structural characteristics, including Mw, monosaccharide composition, and chemical structures. Commonly, LPs’ structural characteristics can be detected by a series of analysis methods, such as high-performance liquid chromatography (HPLC), Fourier-transform infrared spectroscopy (FT-IR), gas chromatography (GC), nuclear magnetic resonance (NMR), methylation analysis, etc. (9, 61, 62). The structural features of LPs from different parts are reviewed, and the relevant information is listed in Table 1.
4.1. Monosaccharide composition
Conventionally, the monosaccharide composition of LPs is determined by HPLC and GC (11, 78). Among newly utilized techniques, high-performance anion-exchange chromatography combined with a pulsed amperometric detector (HPAEC-PAD) is one of the convenient techniques to detect the monosaccharide composition without complex derivatization steps (60). Song et al. (76) used the HPAEC-PAD to determine the monosaccharide composition of two leaf LPs (LLWP-3 and LLWP-1). LLWP-1 consisted of Ara, Glu, Gal, Rha, and GalA (24.8: 6.0: 28.0: 7.0: 26.4), and LLWP-3 consisted of Man, Rha, Ara, Glu, Gal, and GalA (6.2: 6.6: 9.8: 8.9: 15.0: 47.2). As shown in Table 1, LPs mainly consist of Fuc, Glc, Ara, Gal, Man, Xyl, Rha, and GalA (9, 31). Other monosaccharides such as Rib, Fru, and GlcA are also identified in LPs (17, 64, 69). Interestingly, Rib was only detected in the polysaccharides of lotus seed and root (17, 69), while Fru and GlcA were only found in the lotus seed and root, respectively (64, 69). These suggest that LPs exhibited different monosaccharides in different plant parts (i.e., leaf, seed, root, etc.), which leads to differences in polysaccharide properties.
4.2. Molecular weight
Mw reflects the polymerization of polysaccharides, whose detailed elucidation is crucial to study the physiological properties of polysaccharides (79). Conventionally, size exclusion chromatography (SEC), GFC, and gel permeation chromatography (GPC) were used to determine the Mw of LPs (52, 59, 62, 74). Presently, GPC is most frequently used to detect the Mw of LPs. Compared to GPC, SEC-MALLs-RI was more efficient and can be applied to measure the polydispersity coefficient, number-average Mw, and weight-average Mw of polysaccharides (80). For instance, Yan et al. (69) used the SEC-MALLs-RI to determine the Mw of the root LPs (LRP-1). The number-average Mw and weight-average Mw of LRP1 were 251,783 g/mol and 10,236 g/mol, respectively. The LPs were roughly grouped into neutral and acidic fractions, and the Mw distribution of LPs was relatively broad, ranging from 1.095 kDa to 578.09 kDa (32, 74). In particular, the Mw of root LPs is relatively low. The detailed characteristics are listed in Table 1.
4.3. Chemical structure
Currently, the chemical structures of polysaccharides have been detected by FT-IR, GC–MS, and NMR (60). Several studies have isolated LPs with different chemical structures by various separation and purification methods. For instance, Yu et al. (56) obtained homogeneous LPs (ELPS-VII) from the plumula. Its backbone was characterized as α and β-pyranose and (1 → 6)-linked glycosyl residues. Zheng et al. (66) isolated homogeneous LPs (PNP) from plumula. Based on methylation and NMR results, its primary glycosidic linkage was determined as →5)-α-L-Araf-(1→, →3)-β-D-Galp-(1→, β-D-Xylp-(→1, →3,4)-β-D-Rhap-(1→, →4)-β-D-GalpA-(1→. Deng et al. (17) separated three LPs (SN1, SN2, and SN3) from seed and found that SN1 consisted of the α-D-glucopyranosyl ring, SN2 mainly consisted of glucan, while SN3 consisted of 1 → 3, 1 → 2, or 1 → 4 glycosidic bonds. Another LPs (LSPS-1) was isolated and purified from seed, whose backbone was mainly composed of α-pyranose configuration (59), which is consistent with Gao et al. (81).
Moreover, an oligosaccharide from lotus seed was found to contain four glycosidic linkages: α (1 → 6)-Manp, α (1 → 6)-Glup, Galp-(1→), and Manp-(1→) (11). Lei et al. (65) prepared three oligosaccharides, LOS3-1, LOS3-2, and LOS4, from lotus seed. According to 1D, 2D NMR, and FT-IR, they had a linear structure comprising of (1 → 6)-α-D-glucopyranosyl and (1 → 6)-α-D-mannopyranosyl with terminal (1 → 2)-α-D-fucopyranosyl and (1 → 6)-α/β-D-glucopyranosyl (Figure 3A). The backbone of a root LPs (LRPs) primarily composed of Glc-(1→, →6)-Glc-(1→, →6)-Gal-(1→, →4,6)-Gal-(1 → and →3,6)-Glc-(1 → in the molar ratio of 1.00: 4.33: 0.83: 0.13: 1.14 (62). Another root LPs might be [α-D-Glc (1-4)-]n (67). Another study revealed that root LPs (LRP) was composed of Glc and connected by α-glycosidic bonds (Figure 3D) (47). The root LPs (LRP) obtained by Hu et al. (8) consisted of α-D-(1 → 4)-linked glucopyranosyl moieties with branches attached to O-6 of α-D-Glcp residues.
Besides, the structures of pectic polysaccharides from lotus have been elucidated. For instance, Li et al. (78) separated a pectic polysaccharide, WNPP-2-RG, from lotus bee pollen. GC–MS and NMR analysis indicated that WNPP-2-RG comprised a rhamnogalacturonan I (RG-I) region, with the primary linkage consisting of t-D-Gal (12.0%), 1,6-D-Gal (18.3%), and 1,5-L-Ara (25.6%), and side chains were primarily composed of arabinogalactan type I (AG-I) and type II (AG-II). Huang et al. (77) isolated a pectic polysaccharide (NNLP) from lotus leaf, which was predominantly composed of RG-I and homogalacturonan (HG) backbone, including an extended HG fraction and short RG-I fraction with side chains of AG-II and 1–3 linked rhamnose. Recently, She et al. (9) separated a pectic polysaccharide (LPWF) from lotus plumula and further fractionated it into three fractions (LPWF1, LPWF2, and LPWF3) by acid hydrolysis. Methylation and NMR analyses revealed that LPWF represented a unique pectin composition, consisting of xylogalacturonan (XGA, LPWF3) and RGI (RGI, LPWF1-2). LPWF1 was identified as an arabinan peeled from the RGI fraction with a 1,5-linked backbone branching at the O-2 position (Figure 3B). LPWF2 was the residual fraction of RGI consisting of GalA (43.7%), Rha (36.1%), and Gal (17.8%), while LPWF3 was the XGA fraction with a backbone of α-1,4-linked GalA and branches of mono-xylose substitutions attached to O-3 of GalA (Figure 3C).
5. Biological activities
Recent studies revealed that LPs and their derivatives possess multiple bioactivities, including immunomodulatory, antioxidant, anti-inflammatory, antitumor, antidiabetic, and prebiotic activities, which are mediated through interactions with various receptors (7). A comprehensive summary of the bioactivities of LPs is presented in Table 2 and Figure 4, and the mechanisms of immunomodulatory and anti-inflammatory activities are summarized in Figures 5, 6, respectively.
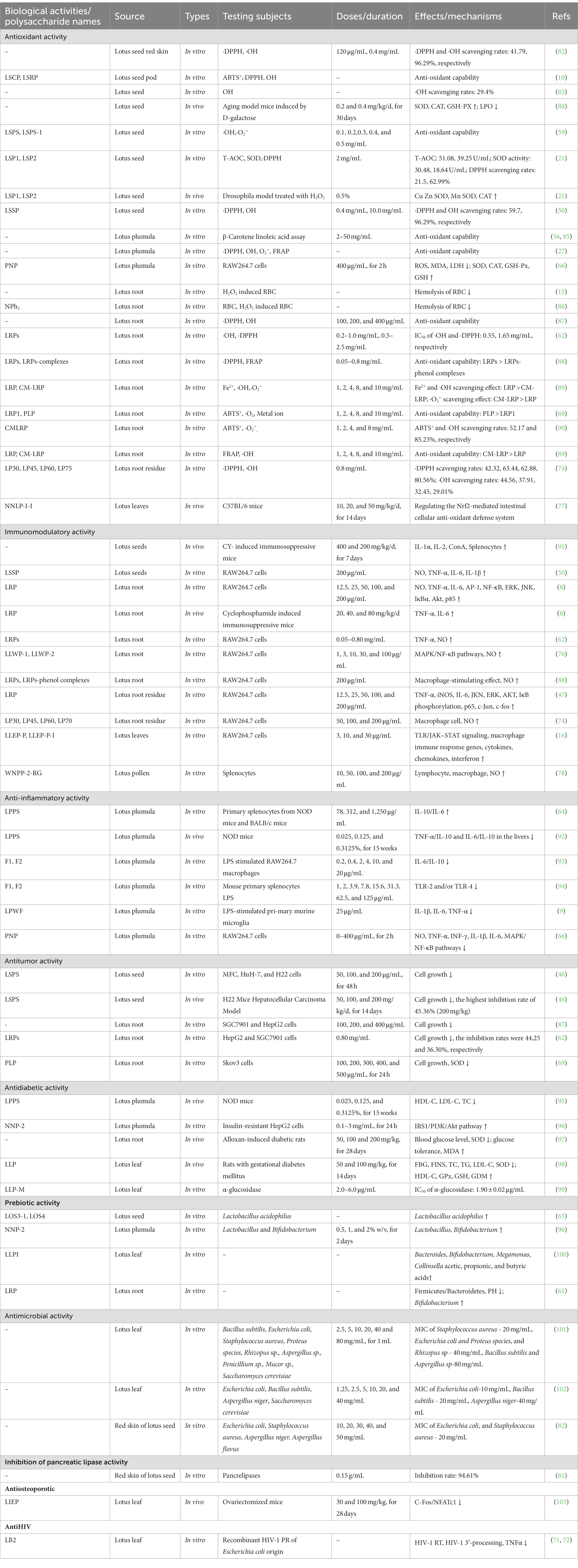
Table 2. Summary of biological activities of LPs from the different parts (↓: decrease; ↑: increase).
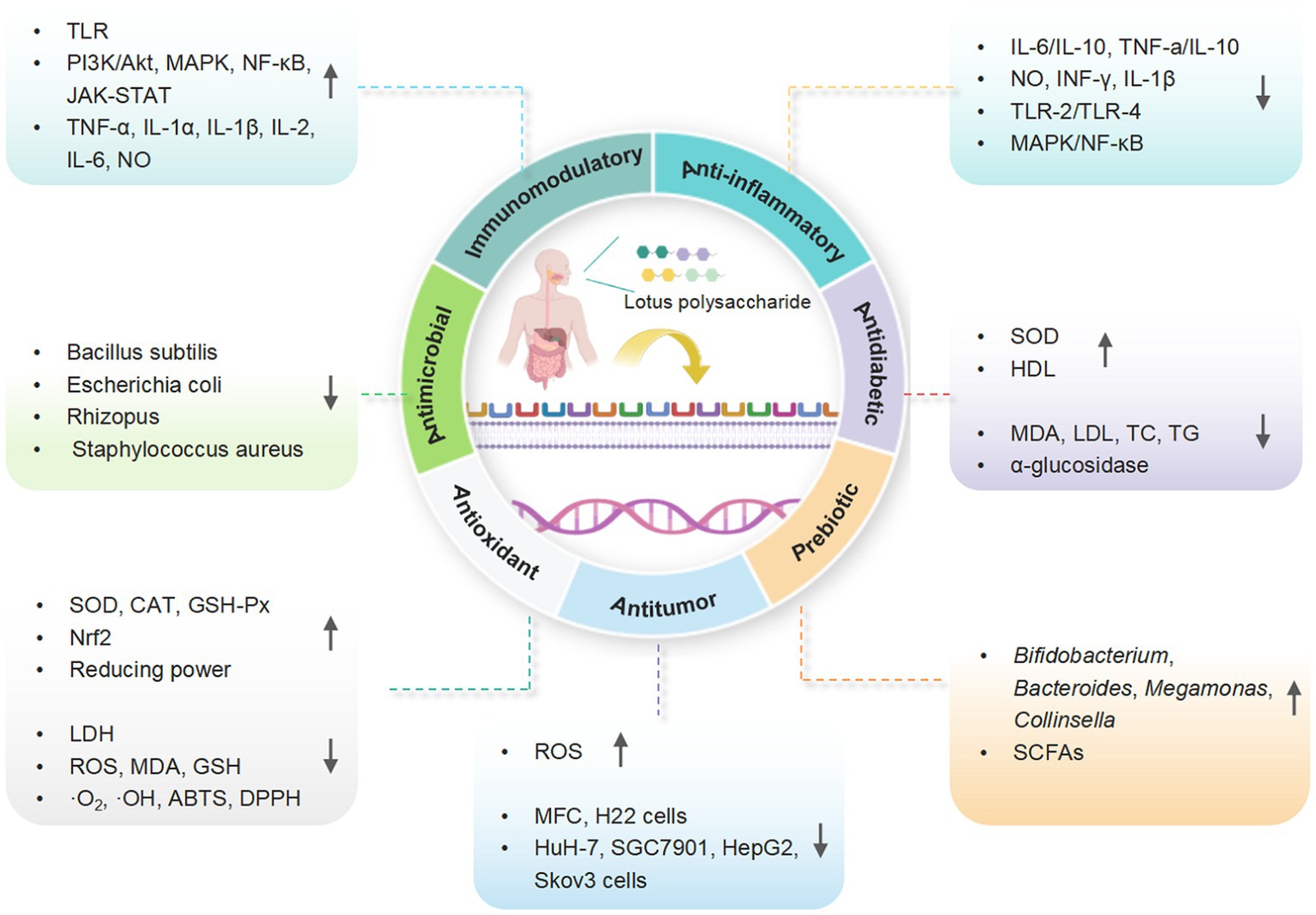
Figure 4. A schematic representation for the biological functions of LPs (created with BioRender.com).
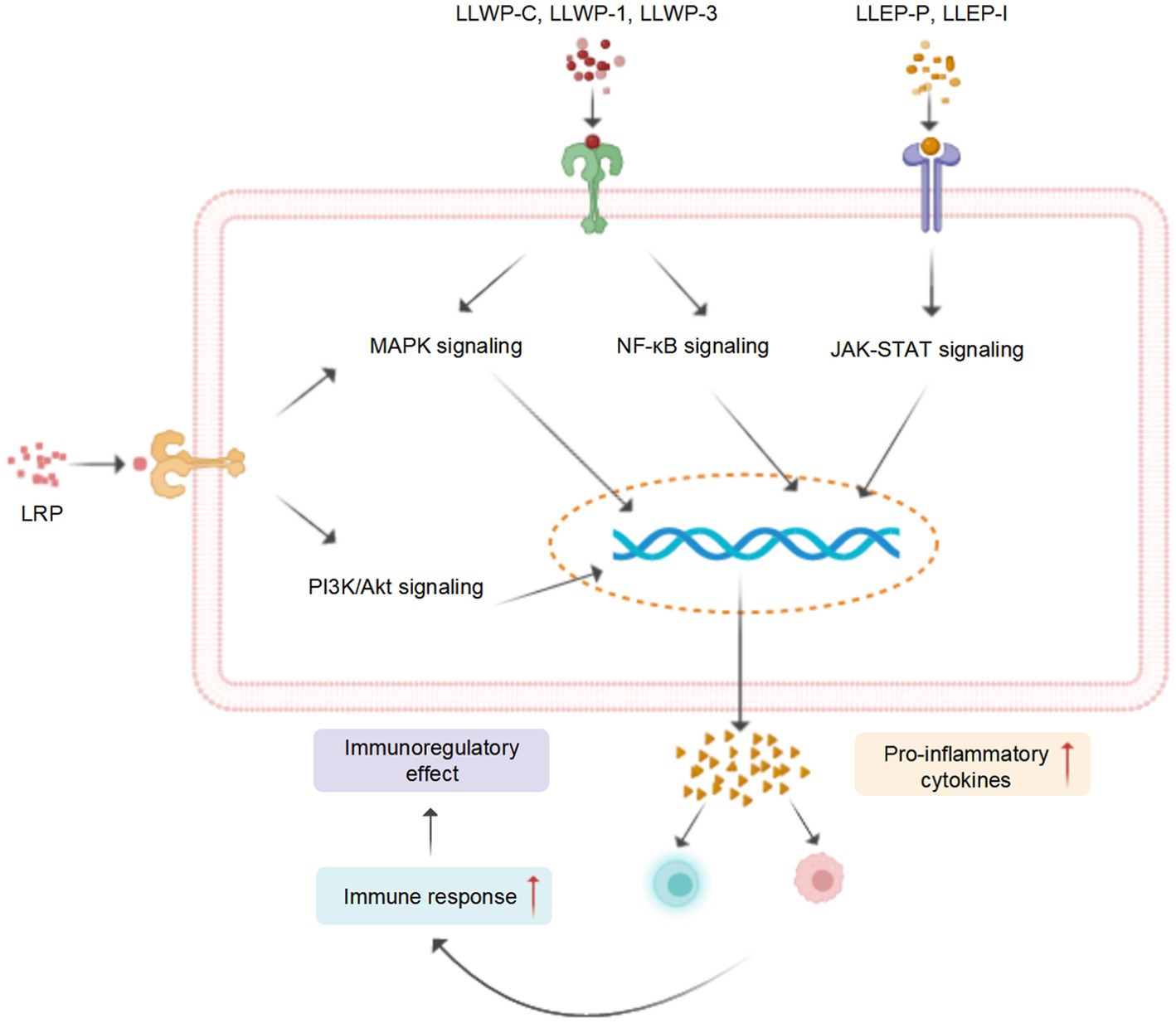
Figure 5. A tentative schematic representation for the proposed mechanisms of immunostimulatory activity of LPs (created with BioRender.com).
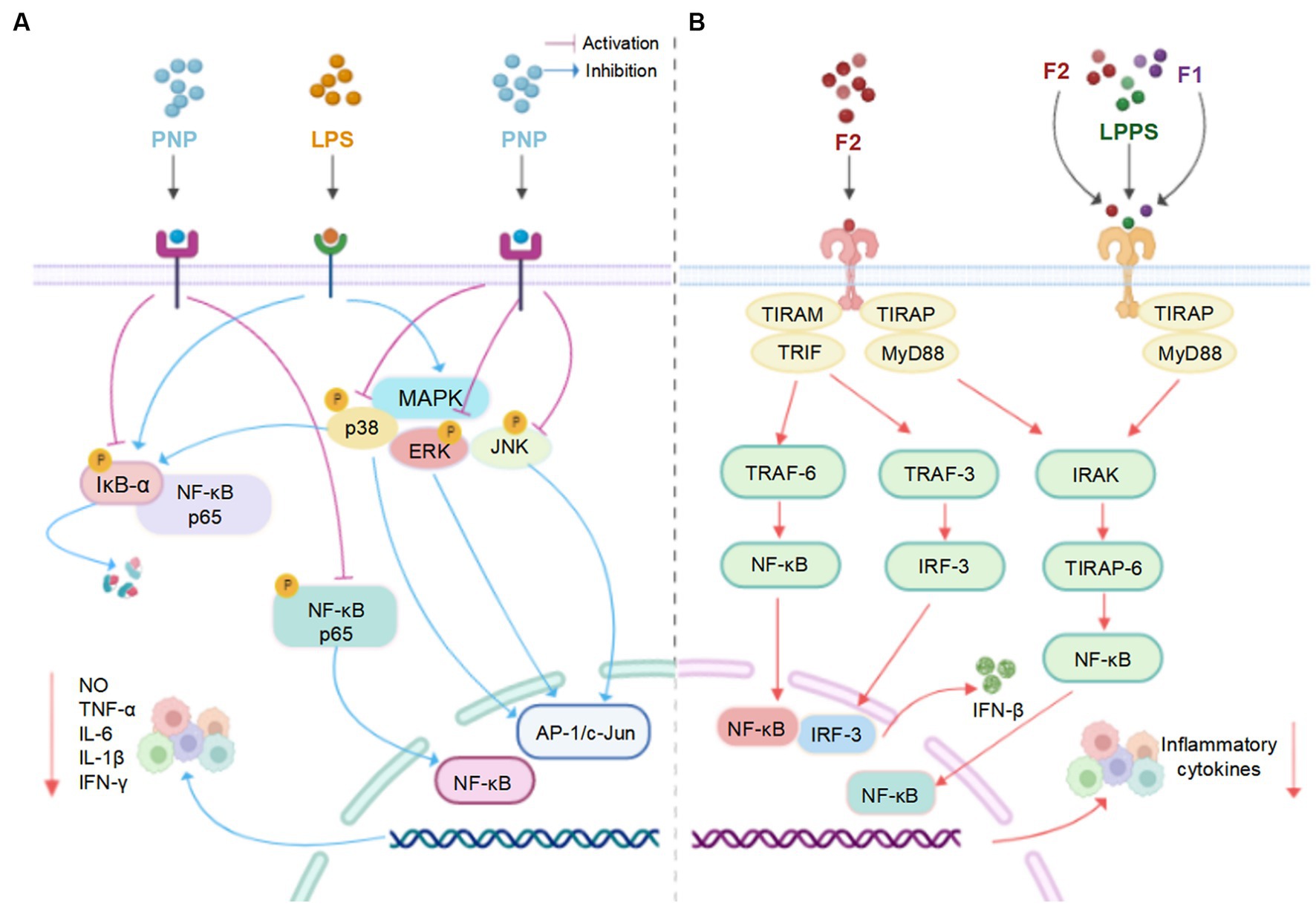
Figure 6. (A) The possible mechanism of PNP and LPs inhibiting inflammation in RAW 264.7 cells (66). (B) The possible mechanism of LPPS, F1 and F2 inhibiting inflammation in mouse primary splenocytes (94) (created with BioRender.com).
5.1. Antioxidant activity
As representative plant polysaccharides, LPs exhibited excellent antioxidant activities in β-carotene linoleic acid, superoxide anion (·O2-), hydroxyl (·OH), 2, 2-azinobis-6-s-(3-ethyl benzothiazoline sulfonic acid) (ABTS), and 1,1-dipheny1-2-picrylhydrazyl (·DPPH) assays (10, 50, 59, 62). LPs also exhibited significant reducing power and anti-lipid peroxidation activity (27, 56, 69). Moreover, the root LPs effectively prevented the oxidative damage of red blood cells induced by H2O2 (15), which is consistent with Yan et al. (86). A plumula LPs (PNP) effectively prevented oxidative damage in RAW264.7 cells by reducing the production of lactate dehydrogenase (LDH), reactive oxygen species (ROS), and malondialdehyde (MDA), improving the activity of glutathione peroxidase (GSH-Px), superoxide dismutase (SOD), and catalase (CAT), and stimulating the production of GSH (66).
The antioxidant activity of LPs has also been investigated in vivo. In a study conducted on D-galactose-induced aging mice, oral administration of seed LPs (PLPs) (0.2 and 0.4 g/kg/d) for 30 days resulted in a significant increase in the activity of SOD, CAT, and GSH-Px in blood, Concurrently, it led to a decrease in the levels of lipid peroxide (LPO) in the plasma, liver, and heart (84). Seed LPs extended the lifespan of Drosophila melanogaster via upregulating the expression level of CuZnSOD, CAT, and MnSOD genes (21). Additionally, leaf LPs (NNLP-I-I) exhibited excellent antioxidant properties by regulating the nuclear factor erythroid 2-related factor (Nrf2) and improving the intestinal cellular antioxidant defense system (77). Although multiple studies have provided evidence of the antioxidant actions of LPs in vitro, there is a scarcity of in vivo investigations. Thus, it is imperative to conduct further comprehensive research to more thoroughly understand the antioxidant mechanism of LPs.
5.2. Immunomodulatory activity
The immune response is recognized as the primary defense mechanism against cancer and infections (104). For instance, the seed LPs (LSSP) exhibit significant immunomodulatory activity by stimulating the production of nitrous oxide (NO), interleukin-1β (IL-1β), IL-6, and tumor necrosis factor-α (TNF-α), in activated RAW 264.7 cells (50). Four root PLs (LP30, LP45, LP30, and LP60) induced the release of NO and enhanced the activity of macrophages (74). Song et al. (76) investigated the mechanism of macrophage activation of crude leaf LPs (LLWP-C) and two derived ingredients (LLWP-1 and LLWP-3), which effectively improved the phagocytic and secretory effects of macrophages via upregulating the mitogen-activated protein kinase (MAPK) and nuclear factor κB (NF-κB) pathways. Another leaf LPs (LLEP-P-I) launched the immune responses of macrophages via upregulating the Janus tyrosine kinase/toll-like receptor (JAK–STAT) and toll-like receptor (TLR) (16).
In the cyclophosphamide (CY)-induced immunosuppressed mice, the seed LPs enhanced the immunocompetence by promoting the secretion of IL-1α and IL-2 and reducing the production of soluble interleukin-2 receptor (SIL-2R) (91). A root LPs (LRP) promoted the production of IL-2 and TNF-α in CY-induced immunosuppressive mice, possibly by activating the MAPK and phosphoinositide 3-kinase/protein kinase (PI3K/Akt) signaling pathways (8), which agrees with Sun et al. (47). These studies indicated that LPs possess potent immunomodulatory activity and could be developed as potential dietary supplements or immunomodulators.
5.3. Anti-inflammatory activity
Accumulated studies reveal that LPs exhibited anti-inflammation effects by regulating pro−/anti-inflammatory cytokine levels. In a study conducted on non-obese diabetics (NOD) mice, the oral administration of plumula LPs (LPPS) for 15 weeks markedly decreased the secretion ratios of IL-6/IL-10 cytokines in splenocytes, and dose-dependently decreased absolute weights of the enlarged spleens (64). Furthermore, LPPS exhibited a marked downregulation of IL-6/IL-10 and TNF-a/IL-10 expression levels in the livers of NOD mice (92).
In vitro, the anti-inflammatory activities of two plumula LPs (F1 and F2) were assessed in splenocytes and lipopolysaccharide (LPS)-stimulated RAW264.7 cells. The secretion ratios of IL-10/IL-6 cytokine were remarkably increased after the treatment of F1 and F2, especially the latter, in a dose-dependent manner. The mechanisms of action could be decreasing the production of TLR-4 or TLR-2 (93, 94). Moreover, plumula LPs (PNP) effectively decreased the secretion of IL-1β, IL-6, INF-γ, TNF-α, and NO in RAW264.7 cells, possibly through the inhibition of the MAPK/NF-κB signaling pathways (66). In addition, a specific type of plumula LPs (LPWF) significantly inhibited the production of TNF-α, and IL-6, IL-1β in the primary murine microglia stimulated by LPS (9).
In brief, LPs demonstrate considerable anti-inflammatory effects both in vivo and in vitro, suggesting their potential as therapeutic agents for inflammation. However, existing literature on the mechanism and SARs underlying the anti-inflammatory activity of LPs is limited. Thereby, it is necessary to further examine these SARs and their specific reaction mechanism.
5.4. Antidiabetic activity
Diabetes mellitus (DM) is a prevalent metabolic disease induced by an abnormal increase in blood glucose levels, which has become the third leading cause of mortality worldwide (104). LPs exhibited great potential in treating DM. Luo et al. (97) studied the hypoglycemic activity of root LPs using an alloxan-induced diabetic mice model. The oral administration of root LPs for 28 days reduced the level of glucose lipid, improved glucose tolerance, and increased the body weight of diabetic mice. Additionally, root LPs enhanced the SOD activity and decreased MDA levels in the kidney, liver, and pancreas of diabetic mice. In NOD female mice, after 15 weeks of administration of plumula LPs (LPPS), the number of pancreatic islet cells increased, the level of low-density lipoprotein cholesterol (LDL-C) and total cholesterol (TC) significantly decreased, and the level of high-density lipoprotein cholesterol (HDL-C) improved (95). Furthermore, Zeng et al. (98) studied the hypoglycemic effect of the leaf LPs (LLP) in pregnant rats with gestational DM (GDM). Oral administration of LLP effectively improved the lipid profile of GDM rats, as evidenced by significantly decreased serum levels of LDL-C, TC, and triglyceride, except for HDL-C.
Le et al. (96) isolated a plumula LPs (NNP-2), which inhibited α-glucosidase with IC50 97.32 μg/mL. Protein expression analysis and real-time PCR showed that NNP-2 could ameliorate insulin resistance in HepG2 cells by regulating the IRS1/PI3K/Akt pathway. Four fractions of leaf LPs (LLP-RF, LLP-V, LLP-M, and LLP-H) demonstrated remarkable antiglycation and α-glucosidase inhibitory activity in vitro (99). These findings suggest that LPs possess potent hypoglycemic activity and are potential dietary supplements or hypoglycemic agents.
5.5. Antitumor activity
LPs have demonstrated remarkable inhibitory effects on cell proliferation in various cancers, e.g., ovarian carcinoma, gastric and liver cancer, in vivo and in vitro. The administration of seed LPs (LSPS) effectively inhibited the growth of mouse hepatocarcinoma H22 cells, human liver cancer HuH-7 cells, and mouse gastric cancer MFC cells. Moreover, in vivo experiments showed that LSPS significantly suppressed tumor cell proliferation in H22-bearing mice with inhibition rates of 17.9.%, 39.60, and 45.36%, respectively (50, 100, and 200 mg/kg/d, 14 days) (46). In another study, Yi et al. (87) isolated 39 LPs from peels, fleshes, and nodes of 13 lotus roots and found that all LPs can notably inhibit the growth of HepG2 and SGC7901 cells. Furthermore, a specific type of LPs derived from the root (LRP) significantly suppressed the proliferation of HepG2 and SGC7901 cells in a dose-dependent manner at 0.05–0.80 mg/mL (62). Yan et al. (69) evaluated the anticancer activity of phosphorylated root LPs (LRPs) in human ovarian cancer Skov3 cells, and found that LRPs effectively inhibited Skov3 proliferation and induced reactive ROS production. Despite significant advances in understanding the antitumor activity of LPs, the underlying mechanism remains limited. As a result, further research is needed to comprehensively assess their potential therapeutic applications.
5.6. Prebiotic activity
LPs have garnered interest as potential prebiotics due to their ability to modulate the intestinal flora by facilitating the fermentation and growth of beneficial bacteria, such as Bacteroides, Bifidobacterium, Megamonas, and Collinsella, consequently enhancing the production of short-chain fatty acids (SCFAs) (105). Studies have shown that plumula LPs (NNP-2) improved the relative abundance of probiotics, specifically Lactobacillus acidophilus and Bifidobacterium adolescentis (96). Additionally, two lotus seed oligosaccharides (LOS3-1 and LOS4) effectively increased the survival rate of L. acidophilus (65). Recently, Guan et al. (60) explored the prebiotic effect of the root LPs (LRP) utilizing an in vitro fermentation model. LRP demonstrated the capacity to modulate intestinal microbiota by promoting Bifidobacterium’s growth and altering the ratio of Firmicutes/Bacteroidetes, thereby promoting the generation of SCFAs (butyric, propionic, and acetic) (61). Besides, leaf LPs (LLPI) selectively improved the abundance of beneficial microbes, including Megamonas, Bacteroides, Bifidobacterium, and Collinsella, and effectively promoted the production of SCFAs (100). These findings underscore the potential of LPs to improve the intestinal micro-environment and highlight their prebiotic properties. Further research is required to assess the digestive and glycolytic characteristics of LRPs in future studies.
5.7. Antimicrobial activity
Leaf LPs had an antimicrobial effect against Escherichia coli and Rhizopus with minimal inhibitory concentration (MIC) of 20 and 40 mg/mL, respectively (101). An in vitro antimicrobial screening assay revealed the growth inhibitory efficiency of the seed LPs (20 mg/mL) on the growth of Staphylococcus aureus and E. coli (82). Moreover, the leaf LPs effectively reduced the growth of E. coli and Bacillus subtilis, although they had little inhibition on Yeast and Aspergillus niger (102). However, there are a limited amount of studies on the antimicrobial activity of PLPs in vivo, and more studies are warranted.
5.8. Other bioactivities
LPs have demonstrated various additional biological activities. Specifically, three types of root LPs (LB2, L2f-2, and L2f-3) exhibited significant inhibition of HIV-1 reverse transcriptase and integrase L2f-3. Notably, LB2 directly inhibited HIV-1 via reducing the expression of TNF-α (71, 72). At 0.15 g/mL, the inhibition rate of the seed LPs on pancreatic lipase reached 94.61%, and its inhibition constant was 0.0736 g/mL (81). Furthermore, leaf LPs (LLEP) demonstrated antiosteoporotic effects in ovariectomized mice. Oral administration of LLEP at doses of 30 and 50 mg/kg/d for 4 weeks remarkably ameliorated the estrogen deficiency-induced bone loss, potentially through the down-regulation of c-Fos/NFATc1 expression (103).
6. Structure-activity relationships
The activity of plant polysaccharides is strongly associated with their structural feature (45). Some studies showed that the monosaccharide, Mw, composition, glycosidic linkage, chemical conformation, and structural modification are critical influencing factors for the bioactivities of LPs (38).
6.1. Monosaccharide composition
Several studies have demonstrated that the monosaccharide composition of LPs is closely related to their biological activity, particularly in terms of Gal, Ara, and Man (62, 78, 87). For instance, Yi et al. found that the immunostimulatory activity of root LPs (LRPs) is associated with the presence of Gal and Man side chains, while the antitumor activity of LRPs may be related to the branched Man residues (62). Moreover, Ara content exhibited a positive correlation with the ferric-reducing antioxidant power (FRAP) and DPPH radical scavenging activity of LRPs, and the Gal content had a positive effect on the ·OH scavenging effect (87). Furthermore, Li et al. reported that hydrolyzed LPs (RG-8H-P) exhibited increased phagocytic activity within macrophages compared to the original LPs. This can be attributed to alterations in the spatial structure and conformation of Gal side chain residues subsequent to the hydrolysis of the original polysaccharides (78). These alterations likely enhance the binding affinity between RG-8H-P and its receptors, thereby facilitating the phagocytosis, digestion, and metabolism of foreign materials by macrophages (106). Similar observations were documented for the polysaccharides derived from Solieria chordalis (107). These findings indicate the significance of monosaccharide composition in understanding the biological activity of LPs.
6.2. Molecular weight
Numerous studies have demonstrated a correlation between the Mw of LPs and their biological activity (60). Specifically, it has been observed that low-Mw F2 (25.7 kDa) displays more potent anti-inflammatory effects than F1 (> 2000 kDa) (94), presumably owing to the improved permeability of low-Mw LPs, facilitating their penetration through the cell membrane (23). Additionally, LPs derived from leaves with low-Mw exhibit a more pronounced activation effect on RAW264.7 macrophages (76) as well as superior antioxidant activity, α-amylase inhibition, and α-glucosidase inhibition (12), aligning with previous findings regarding the polysaccharides of Polygonatum (108). Notably, LPs with low-Mw (LOS3-1 and LOS4) display significant prebiotic activity (65), likely due to their enhanced absorption and utilization by L. acidophilus (109). However, the exact mechanism underlying this discrepancy remains elusive, warranting further studies.
6.3. Structural modifications
Appropriate chemical modifications have been shown to enhance the efficacy of LPs and broaden their potential applications (39). Recent studies showed that phosphorylation and carboxymethylation could significantly improve the scavenging ability of LRPs on ABTS, ·OH radicals, and ferrous ions (69, 89). This enhancement is likely attributed to the introduction of carboxymethyl and phosphate groups, which alter the water solubility and spatial conformation of LRPs, thereby influencing their biological activity (110). Furthermore, Yi et al. (88) observed a significant enhancement in the FRAP capacity and ·DPPH radical scavenging effect of phenolic compound-modified LRPs. The extent of enhancement was positively correlated with the binding ratio of phenolic compounds, possibly due to the presence of multiple hydroxyl groups with unpaired electrons in these compounds (110). Yuan et al. (111) found that the binding of Fe (III) with LRPs can significantly enhance the antioxidant activities of LRPs. It can also alleviate the symptoms of iron deficiency anemia in mice by impacting the gut microbiome and regulating the biosynthesis of steroid hormones.
Due to the scarcity of literature on the chemical structures and structural modifications of LPs, it is challenging to explain the SARs of LPs. Undoubtedly, comprehensively understanding these aspects would propel the development of LPs-based dietary supplements and therapeutic medications. Therefore, it is urgently necessary to expand current scientific research in this field.
7. Applications
In recent years, LPs and their derivatives have not only been used to treat and prevent disease but also used to develop nutritional enhancers and dietary supplements. LPs have gained widespread utilization as natural active ingredients in health products. For instance, a composite functional beverage was prepared using roots LPs, vitamin C, sucrose, oligosaccharide maltose, citric acid, NaCl, food color, and essence, which has a significant antifatigue effect (112). Due to the ability to promote the growth of beneficial bacteria and stimulate the production of SCFAs (61, 65, 100), LPs hold promising potential as prebiotics in the food industry. Additionally, LPs exhibit significant immunomodulatory activity (16, 74), making them a favorable food supplement for individuals with compromised immune function. Recent studies showed that lotus pectic polysaccharides possess characteristics that contribute to satiety and hinder digestion, rendering them suitable for the development of weight loss products (13).
In addition, LPs can also be used as additives in cosmetics because of their anti-oxidant and anti-senescence activities (21, 91). Besides, LPs can be applied in the research and development of new drugs because of their biological activity, such as anti-inflammatory, antidiabetic, and antiosteoporotic activities. LRPs could be used as a potential drug carrier to develop new systems for drug release, particularly colon-delivery systems (113).
8. Safety assessment
It is critical to evaluate the cytotoxicity and adverse effects before developing any product for food and medical treatments. To date, various beneficial effects of LPs have been extensively studied. However, studies investigating the safety and toxicity of LPs are lacking. In H22 tumor-bearing mice, oral administration of seed LPs (LSPS) (50, 100, and 200 mg/kg) for 14 days did not alter the blood parameters, e.g., platelets, hemoglobin, and red blood cells (46). Zeng et al. (98) studied the toxicity of leaf LPs (LLP) in rats and found that the neurologic and behavioral alterations of LLP-treated rats were regular, and no death or harmful effect was observed during the experiment. In vitro studies have shown that the plumula LPs (PNP) of 25–400 μg/mL had no toxicity on RAW264.7 cells (66). While these findings suggest the potential safety of LPs, it is essential to note that their toxicity studies remain underdeveloped. Consequently, additional research involving clinical trials and animal experiments is needed to further verify their safety and toxicity.
9. Conclusion and future perspective
Numerous studies have focused on the extraction, separation, purification, structural identification, and pharmacological effects of LPs. The most common extraction method for LPs is HWE, while UAE and MAE improve the extraction rate of LPs. LPs are classified as heteropolysaccharides, comprising monosaccharide units such as Glc, Gal, Ara, Xyl, Man, and Rha. These polysaccharides possess diverse bioactivities, including immunoregulatory, anti-inflammatory, antioxidant, antidiabetic, antitumor, prebiotic, and antimicrobial, and can be used as promising functional food supplements or therapeutic agents. It is worth noting that the biological activities of LPs are influenced by their structural characteristics, especially the Mw, monosaccharide composition, and glycosidic bonds, among other factors.
Despite the opulent results of LPs, there are still some critical issues that need to be addressed. Firstly, new methods have been used for the extraction of LPs. However, industrial production needs more simple, efficient, and less expensive methods to produce high-quality LPs. Different extraction methods should be combined to develop methods suitable for industrial production. Secondly, the current focus of structural analyses of LPs predominantly revolves around their primary structure, such as Mw, monosaccharide composition, glycosidic bonds, etc. The advanced structure (spatial conformation) of LPs must be elucidated by circular dichroism, scanning tunneling microscopy, and X-ray diffraction. Thirdly, exploring SARs of LPs is still in its preliminary stages. The relationships between the structure (including Mw, monosaccharide composition, type of glycosidic linkages, and chemical conformation) and the activity of LPs remain unclear and require further exploration. Fourthly, it is worth noting that there are remarkable differences between the different sources and medicinal parts of lotus in the biological activity, structural features, and content of LPs. It is necessary to systematically investigate the different parts and kinds of LPs, which is critical for quality control. Fifthly, the action mechanism, dosage, usage, course, and safety of LPs are poorly understood, and more pharmacological experiments and acute/chronic toxicity studies should be conducted to explore the underlying mechanisms, optimal dosage, reliability, and effectiveness. Lastly, exploring specific structural modifications, such as hydroxylation, selenization, sulfation, and olefination, should be pursued to enhance the bioactivity of LPs, which is one of the critical directions for future research.
Author contributions
GD, JW, and JZ: writing – original draft. CX, YW, and BD: conceptualization, supervision, project administration. All authors contributed to the article and approved the submitted version.
Funding
This work was supported by the Yunnan academician expert workstation [202105AF150053, 202205AF150026], the key technology projects in the Yunnan Province of China [202002AA100007], and the Yunnan Xingdian talent support plan [YNWR-QNBJ-2020251].
Conflict of interest
The authors declare that the research was conducted in the absence of any commercial or financial relationships that could be construed as a potential conflict of interest.
Publisher’s note
All claims expressed in this article are solely those of the authors and do not necessarily represent those of their affiliated organizations, or those of the publisher, the editors and the reviewers. Any product that may be evaluated in this article, or claim that may be made by its manufacturer, is not guaranteed or endorsed by the publisher.
References
1. Wang, Z, Cheng, Y, Zeng, M, Wang, Z, Qin, F, Wang, Y, et al. Lotus (Nelumbo nucifera Gaertn.) leaf: a narrative review of its phytoconstituents, health benefits and food industry applications. Trends Food Sci Tech. (2021) 112:631–50. doi: 10.1016/j.tifs.2021.04.033
2. Arooj, M, Imran, S, Inam-Ur-Raheem, M, Rajoka, M, Sameen, A, Siddique, R, et al. Lotus seeds (Nelumbinis semen) as an emerging therapeutic seed: a comprehensive review. Food Sci Nutrition. (2021) 9:3971–87. doi: 10.1002/fsn3.2313
3. Cho, W, Yang, HJ, and Ma, JY. Lotus (Nelumbo nucifera Gaertn.) leaf water extracts suppress influenza a viral infection via inhibition of neuraminidase and hemagglutinin. J Funct Foods. (2022) 91:105019. doi: 10.1016/j.jff.2022.105019
4. Chen, C, Li, G, and Zhu, F. A novel starch from lotus (Nelumbo nucifera) seeds: composition, structure, properties and modifications. Food Hydrocolloid. (2021) 120:106899. doi: 10.1016/j.foodhyd.2021.106899
5. Xiong, W, Chen, X, Lv, G, Hu, D, Zhao, J, and Li, S. Optimization of microwave-assisted extraction of bioactive alkaloids from lotus plumule using response surface methodology. J Pharm Anal. (2016) 6:382–8. doi: 10.1016/j.jpha.2016.05.007
6. Punia, BS, Dunno, K, Kumar, M, Mostafa, H, and Maqsood, S. A comprehensive review on lotus seeds (Nelumbo nucifera Gaertn.): nutritional composition, health-related bioactive properties, and industrial applications. J Funct Foods. (2022) 89:104937. doi: 10.1016/j.jff.2022.104937
7. Liang, T, Li, J, Wang, Y, and Wang, X. Extraction and purification, structural feature and biological activity of polysaccharides in different parts of lotus (Nelumbo nucifera Gaertn.): a review. Food Sci. (2022) 7:1–18. doi: 10.7506/spkx1002-6630-20220514-185
8. Hu, W, Jiang, Y, Xue, Q, Sun, F, Zhang, J, Zhou, J, et al. Structural characterisation and immunomodulatory activity of a polysaccharide isolated from lotus (Nelumbo nucifera Gaertn.) root residues. J Funct Foods. (2019) 60:103457. doi: 10.1016/j.jff.2019.103457
9. Shen, L, Chu, X, Zhang, Z, and Wu, T. Structural characterization and in vitro anti-inflammatory estimation of an unusual pectin linked by rhamnogalacturonan I and xylogalacturonan from lotus plumule. Int J Biol Macromol. (2022) 194:100–9. doi: 10.1016/j.ijbiomac.2021.11.178
10. Wu, H, Shu, L, Liang, T, Li, Y, Liu, Y, Zhong, X, et al. Extraction optimization, physicochemical property, antioxidant activity, and α-glucosidase inhibitory effect of polysaccharides from lotus seedpods. J Sci Food Agr. (2022) 102:4065–78. doi: 10.1002/jsfa.11755
11. Lu, X, Zhang, Y, and Wu, X. Effect of specific structure of lotus seed oligosaccharides on the production of short-chain fatty acids by Bifidobacterium adolescentis. Chin J Struc Chem. (2015) 34:510–22. doi: 10.14102/j.cnki.0254-5861.2011-0521
12. Feng, K, Huang, L, Wu, D, Li, F, Gan, R, Qin, W, et al. Physicochemical properties and in vitro bioactivities of polysaccharides from lotus leaves extracted by different techniques and solvents. J Food Meas Charact. (2022) 16:1583–94. doi: 10.1007/s11694-021-01256-3
13. Wang, M, Hu, W, Wang, Q, Yang, B, and Kuang, H. Extraction, purification, structural characteristics, biological activities, and application of the polysaccharides from Nelumbo nucifera Gaertn. (lotus): a review. Int J Biol Macromol. (2023) 226:562–79. doi: 10.1016/j.ijbiomac.2022.12.072
14. Tang, Z, and Huang, G. Extraction, structure, and activity of polysaccharide from Radix astragali. Biomed Pharmacother. (2022) 150:113015. doi: 10.1016/j.biopha.2022.113015
15. Wang, Y, Gao, P, Jiang, L, Boxun, Z, Liang, X, Tong, L, et al. Extraction of the lotus roots polysaccharides and their biological activity. Food Sci Technol. (2007) 5:113–6. doi: 10.13684/j.cnki.spkj.2007.05.035
16. Song, Y, Han, A, Park, S, Cho, C, Rhee, Y, and Hong, H. Effect of enzyme-assisted extraction on the physicochemical properties and bioactive potential of lotus leaf polysaccharides. Int J Biol Macromol. (2020) 153:169–79. doi: 10.1016/j.ijbiomac.2020.02.252
17. Deng, T. Effect of Ultrasonic Radiation on Structural Characterization of Polysaccharides from Poria cocos and Lotus Seeds (Master) Shangxi: Shaanxi Normal University (2012).
18. Liu, Y. Study on extraction and antioxidation of water soluble polysaccharides from lotus leaves. Chin J Ethnomed Ethnopharmacy. (2009) 18:10.
19. Wang, Z, and Sun, G. Extraction and purification of soluble polysaccharides from lotus leaves. China Feed. (2008) 19:39–41. doi: 10.15906/j.cnki.cn11-2975/s.2008.19.002
20. Dan, B. Separation of Sync of Flavonoids, Alkaloids and Polysaccharides & Researches of Futuction of Alkaloids in Lotus Leaves (Master) Nanchang: Nanchang University (2008).
21. Zhang, Y. Anti-Aging and Molecular Mechanisms of Lotus Seed Polysaccharide (Master) Fuzhou: Fujian Agriculture and Forestry University (2013).
22. Chen, L, Zhang, G, You, L, Hu, W, and Yuan, Q. Effects of different extraction methods on polysaccharide components of lotus root residue. Guangdong Chem Ind. (2019) 46:55–7.
23. Sharma, K, Kumar, M, Waghmare, R, Suhag, R, Gupta, OP, Lorenzo, JM, et al. Moringa (Moringa oleifera Lam.) polysaccharides: extraction, characterization, bioactivities, and industrial application. Int J Biol Macromol. (2022) 209:763–8. doi: 10.1016/j.ijbiomac.2022.04.047
24. Li, X, Rao, Z, Xie, Z, Qi, H, and Zeng, N. Isolation, structure and bioactivity of polysaccharides from Atractylodes macrocephala: a review. J Ethnopharmacol. (2022) 296:115506. doi: 10.1016/j.jep.2022.115506
25. Wang, W, Tan, J, Nima, L, Sang, Y, Cai, X, and Xue, H. Polysaccharides from fungi: a review on their extraction, purification, structural features, and biological activities. Food Chem X. (2022) 15:100414. doi: 10.1016/j.fochx.2022.100414
26. Li, Y, Zhou, J, Wan, Y, and Chen, X. Optimization of ultrasonic-assisted extraction of polysaccharide from lotus seed skins. Cereal Feed Ind. (2011) 2:42–4. doi: 10.3969/j.issn.1003-6202.2011.02.013
27. Zhang, L, Cheng, Z, Zhao, Q, and Wang, M. Green and efficient PEG-based ultrasound-assisted extraction of polysaccharides from superfine ground lotus plumule to investigate their antioxidant activities. Ind Crop Prod. (2017) 109:320–6. doi: 10.1016/j.indcrop.2017.08.018
28. Tu, Z, Kou, Y, Wang, H, Zhang, Q, Liu, J, and Chen, Z. Ultrasonic-assisted exyraction and antioxidant activity of polysaccharides from lotus leaves. Food Sci. (2013) 34:108–12. doi: 10.7506/spkx1002-6630-201316022
29. Wang, C, and Zhang, X. Study on microwave assisted extraction and purification of lotus seed polysaccharides. Food Nutr China. (2009) 4:36–8. doi: 10.3969/j.issn.1006-9577.2009.04.012
30. Peng, X. Ultrasonic Synergistric Preparation of Polysaccharides from Lotus Seeds and Tis Effect on the Proliferation of Lactobacillus (Master) Fuzhou: Fujian Agriculture and Forestry University (2017).
31. Zhang, L, Tu, Z, Wang, H, Kou, Y, Wen, Q, Fu, Z, et al. Response surface optimization and physicochemical properties of polysaccharides from Nelumbo nucifera leaves. Int J Biol Macromol. (2015) 74:103–10. doi: 10.1016/j.ijbiomac.2014.11.020
32. Kou, Y. Influence of Dynamic High-Pressure Microfluidization on Extraction, Structure and Anti-Oxidant Activities of Polysaccharides from Lotus Leaf (Master) Nanchang: Nanchang University (2013).
33. Xu, H, Zhu, Y, Cao, Y, and Song, H. Study on extraction technology of polysaccharides from red skin of lotus seed. Sci Technol Food Ind. (2011) 32:266–8. doi: 10.13386/j.issn1002-0306.2011.02.063
34. Wang, Z, Zhao, J, Zhu, T, Hu, J, Zhao, C, and Xiang, L. Cellulase-assisted extraction process optimization, kinetics and thermodynamics of polysaccharides from Nelumbinis Rhizomatis Nodus. Chin Tradit Pat Med. (2020) 42:836–42. doi: 10.3969/j.issn.1001-1528.2020.04.003
35. Liu, J, Zou, L, and Zhao, Y. Polysaccharides extraction technology from lotus leaves by enzyme-assisted technique. Food Res Dev. (2013) 34:74–6. doi: 10.3969/j.issn.1005-6521.2013.24.021
36. Wu, D, Feng, K, Huang, L, Gan, R, Hu, Y, and Zou, L. Deep eutectic solvent-assisted extraction, partially structural characterization, and bioactivities of acidic polysaccharides from lotus leaves. Foods. (2021) 10:2330. doi: 10.3390/foods10102330
37. Li, B, and Huang, G. Preparation, structure-function relationship and application of Grifola umbellate polysaccharides. Ind Crop Prod. (2022) 115:221–6. doi: 10.1016/j.indcrop.2022.115282
38. Qin, L, Chen, S, Xie, L, Yu, Q, Chen, Y, and Xie, J. Recent advances in mung bean polysaccharides: extraction, physicochemical properties and biological activities. Process Biochem. (2022) 121:248–56. doi: 10.1016/j.procbio.2022.07.014
39. Jia, X, Ma, L, Li, P, Chen, M, and He, C. Prospects of Poria cocos polysaccharides: isolation process, structural features and bioactivities. Trends Food Sci Tech. (2016) 54:52–62. doi: 10.1016/j.tifs.2016.05.021
40. Ji, K, Xing, J, Wang, N, Meng, X, Shuang, S, Wu, S, et al. Insights into the structure-bioactivity relationships of marine sulfated polysaccharides: a review. Food Hydrocolloid. (2021) 123:107049. doi: 10.1016/j.foodhyd.2021.107049
41. Feijoo, S, Hayes, W, Watson, C, and Martin, J. Effects of microfluidizer® technology on Bacillus licheniformis spores in ice cream mix1. J Dairy Sci. (1997) 80:2184–7. doi: 10.3168/jds.S0022-0302(97)76166-6
42. Zhang, S, Bi, H, and Liu, C. Extraction of bio-active components from Rhodiola sachalinensis under ultrahigh hydrostatic pressure. Sep Purif Technol. (2007) 57:277–82. doi: 10.1016/j.seppur.2007.04.022
43. Luan, F, Peng, X, Zhao, G, Zeng, J, Zou, J, Rao, Z, et al. Structural diversity and bioactivity of polysaccharides from medicinal mushroom Phellinus spp.: a review. Food Chem. (2022) 397:133731. doi: 10.1016/j.foodchem.2022.133731
44. Zdanowicz, M, Wilpiszewska, K, and Spychaj, T. Deep eutectic solvents for polysaccharides processing. A review. Carbohyd Polym. (2018) 200:361–80. doi: 10.1016/j.carbpol.2018.07.078
45. Zou, Y, Zhang, Y, Paulsen, BS, Fu, Y, Huang, C, Feng, B, et al. Prospects of Codonopsis pilosula polysaccharides: structural features and bioactivities diversity. Trends Food Sci Tech. (2020) 103:1–11. doi: 10.1016/j.tifs.2020.06.012
46. Zheng, Y, Wang, Q, Zhuang, W, Xu, L, Anca, M, Chai, TT, et al. Cytotoxic, antitumor and immunomodulatory effects of the water-soluble polysaccharides from lotus (Nelumbo nucifera Gaertn.) seeds. Molecules. (2016) 21:1465. doi: 10.3390/molecules21111465
47. Sun, F. Structure and Immunological Activity of Polysaccharides from Lotus Root Residue and Preparation of Polysaccharides Selenium Nanoparticles (Master) Yangzhou: Yangzhou University (2019).
48. Xing, Y, and Jing, Y. Effect of different extraction methods on yield and antioxidant activity of lotus leaves polysaccharide. Food Mach. (2020) 36:166–169+175. doi: 10.13652/j.issn.1003-5788.2020.06.030
49. Zeng, F, Yao, Y, Wang, L, and Li, W. Polysaccharides as antioxidants and prooxidants in managing the double-edged sword of reactive oxygen species. Biomed Pharmacother. (2023) 159:114221. doi: 10.1016/j.biopha.2023.114221
50. Wu, L, Wu, J, Hu, J, Xiong, W, Gu, Z, and Wang, H. Physicochemical properties and immunomodulatory activities of polysaccharides from white lotus seed skin. Mod Food Sci Technol. (2021) 37:100–108+129. doi: 10.13982/j.mfst.1673-9078.2021.5.1008
51. Gao, H, Gao, Y, and Xu, H. Separation, purification and structural characteristics of polysaccharides from red skin of lotus seeds. Food Sci. (2016) 37:94–9. doi: 10.7506/spkx1002-6630-201615016
52. Wu, C. Studies on extraction, purification and chemical of the polysaccharides from embryo of the seeds of Nelumbo nucifera Gaerth (Master) Fuzhou: Fujian Agriculture and Forestry University (2010).
53. Chang, Q, and Peng, X. Study on separation and purification of polysaccharides from lotus seeds. Light Text Ind Fujian. (2018) 8:14–20. doi: 10.3969/j.issn.1007-550X.2018.08.001
54. Zhong, X. Studies on Extraction, Purification and Structure of the Polysaccharides of Lotus Leaf snd Extraction of Its Flavonoid (Master) Nanchang: Nanchang University (2005).
55. Chen, C. Study on the Extraction Technology Structure and Properties of the Lotus Seed Polysaccharide (Master) Fuzhou: Fujian Agriculture and Forestry University (2007).
56. Yu, Y. Purification and Antioxidant Activity of Polysaccharides from Lotus Plumule (Master) Hangzhou: Zhejiang University (2006).
57. Zhang, R, Zhang, X, Tang, Y, and Mao, J. Composition, isolation, purification and biological activities of Sargassum fusiforme polysaccharides: a review. Carbohyd Polym. (2020) 228:115381. doi: 10.1016/j.carbpol.2019.115381
58. Tang, W, Liu, D, Yin, J, and Nie, S. Consecutive and progressive purification of food-derived natural polysaccharide: based on material, extraction process and crude polysaccharide. Trends Food Sci Tech. (2020) 99:76–87. doi: 10.1016/j.tifs.2020.02.015
59. Tian, Y, Zheng, B, Chen, C, and Zheng, Y. Ultrasound-assisted extraction, preliminary characterization, and antioxidant activity of a novel water-soluble polysaccharide from lotus (Nelumbo nucifera Gaertn.) seeds. Separat Sci Technol. (2012) 47:2408–16. doi: 10.1080/01496395.2012.665118
60. Zhang, J, Liu, D, Wen, C, Liu, J, Xu, X, Liu, G, et al. New light on Grifola frondosa polysaccharides as biological response modifiers. Trends Food Sci Tech. (2022) 119:566–78. doi: 10.1016/j.tifs.2021.11.017
61. Guan, X, Feng, Y, Jiang, Y, Hu, Y, Zhang, J, Li, Z, et al. Simulated digestion and in vitro fermentation of a polysaccharide from lotus (Nelumbo nucifera Gaertn.) root residue by the human gut microbiota. Food Res Int. (2022) 155:111074. doi: 10.1016/j.foodres.2022.111074
62. Yi, Y, Huang, X, Zhong, Z, Huang, F, Li, S, Wang, L, et al. Structural and biological properties of polysaccharides from lotus root. Int J Biol Macromol. (2019) 130:454–61. doi: 10.1016/j.ijbiomac.2019.02.146
63. Deng, T, Zhang, J, Huang, D, and Sun, R. Semen nelumbinis polysaccharide structure and antioxidant activity. Chin J Bioproc E. (2012) 10:47–50. doi: 10.3969/j.issn.1672-3678.2012.06.010
64. Liao, C, Guo, S, and Lin, J. Characterisation of the chemical composition and in vitro anti-inflammation assessment of a novel lotus (Nelumbo nucifera Gaertn) plumule polysaccharide. Food Chem. (2011) 125:930–5. doi: 10.1016/j.foodchem.2010.09.082
65. Lei, Y, Zhang, Y, Wang, Q, Zheng, B, Miao, S, and Lu, X. Structural characterization and in vitro analysis of the prebiotic activity of oligosaccharides from lotus (Nelumbo nucifera Gaertn.) seeds. Food Chem. (2022) 388:133045. doi: 10.1016/j.foodchem.2022.133045
66. Zheng, Q, Chen, J, Yuan, Y, Zhang, X, Li, L, Zhai, Y, et al. Structural characterization, antioxidant, and anti-inflammatory activity of polysaccharides from plumula nelumbinis. Int J Biol Macromol. (2022) 212:111–22. doi: 10.1016/j.ijbiomac.2022.05.097
67. Zhong, Z. Isolating and Charactering Polyphenols and Polysaccharides from Lotus Root for the Preparation of Blended Liposomes (Master) Wuhan: Wuhan Polytechnic University (2018).
68. Das, S, Ray, B, and Ghosal, PK. Structural studies of a polysaccharide from the seeds of Nelumbo nucifera. Carbohydr Res. (1992) 224:331–5. doi: 10.1016/0008-6215(92)84121-8
69. Yan, Y, Yuan, S, Zhao, S, Xu, C, and Zhang, X. Preparation and application of phosphorylated lotus root polysaccharide. Food Sci Tech-Brazil. (2022) 42:82121. doi: 10.1590/fst.82121
70. Liu, E, Huang, X, Sun, J, Min, T, and Yi, Y. Optimization of monosaccharide composition analysis methods of lotus root polysaccharides. Food Sci Technol. (2018) 43:292–6. doi: 10.13684/j.cnki.spkj.2018.06.053
71. Jiang, J, Wang, C, Li, N, Chen, Z, Zhang, D, Lv, P, et al. Isolation of a polysaccharide with antioxidant and HIV-1 integrase inhibitory activities from Nulumbo nuciferra Gaertn. Acta Sci Nat Univ Nankaiensis. (2010) 43:42–7.
72. Jiang, Y, Ng, TB, Liu, Z, Wang, C, Li, N, Qiao, W, et al. Immunoregulatory and anti-HIV-1 enzyme activities of antioxidant components from lotus (Nelumbo nucifera Gaertn.) rhizome. Biosci Rep. (2011) 31:381–90. doi: 10.1042/BSR20100062
73. Sun, F, Sun, F, and Li, W. Determination of monosaccharide composition of lotus root residue polysaccharide. Chin Cond. (2018) 43:144–6. doi: 10.3969/j.issn.1000-9973.2018.09.03
74. Zhang, M, Yang, Y, Ting, M, Wang, L, and Sun, Y. Composition analysis and in vitro evaluation of some activities of RAW264.7 of lotus root polysaccharide extracted by ethanol precipitation with different concentrations. Food Sci Technol. (2021) 46:162–70. doi: 10.13684/j.cnki.spkj.2021.08.025
75. Yan, L, Zhang, S, Zhang, F, Shi, B, Li, S, and Li, Q. Extractation and characteristics of polysaccharides from lotus root pomace. Food Sci. (2007) 28:226–30. doi: 10.3321/j.issn:1002-6630.2007.12.052
76. Song, Y, Han, A, Lim, T, Lee, E, and Hong, H. Isolation, purification, and characterization of novel polysaccharides from lotus (Nelumbo nucifera) leaves and their immunostimulatory effects. Int J Biol Macromol. (2019) 128:546–55. doi: 10.1016/j.ijbiomac.2019.01.131
77. Huang, C, Peng, X, Pang, D, Li, J, Paulsen, BS, Rise, F, et al. Pectic polysaccharide from Nelumbo nucifera leaves promotes intestinal antioxidant defense in vitro and in vivo. Food Funct. (2021) 12:10828–41. doi: 10.1039/d1fo02354c
78. Li, S, Yang, G, Yan, J, Wu, D, Hou, Y, Diao, Q, et al. Polysaccharide structure and immunological relationships of RG-I pectin from the bee pollen of Nelumbo nucifera. Int J Biol Macromol. (2018) 111:660–6. doi: 10.1016/j.ijbiomac.2018.01.015
79. Pak, S, Chen, F, Ma, L, Hu, X, and Ji, J. Functional perspective of black fungi (Auricularia auricula): major bioactive components, health benefits and potential mechanisms. Trends Food Sci Tech. (2021) 114:245–61. doi: 10.1016/j.tifs.2021.05.013
80. Wu, D, Guo, H, Lin, S, Lam, S, Zhao, L, Lin, D, et al. Review of the structural characterization, quality evaluation, and industrial application of Lycium barbarum polysaccharides. Trends Food Sci Tech. (2018) 79:171–83. doi: 10.1016/j.tifs.2018.07.016
81. Gao, H, Wu, N, Gao, Y, and Xu, H. Inhibitory effect on pancreatic lipase activity of polysaccharide from lotus seed skin. Cereals Oils. (2017) 30:87–91. doi: 10.3969/j.issn.1008-9578.2017.05.024
82. Xue, S, Yang, D, Li, L, Jianjun, H, Guan, J, Zeng, G, et al. Antioxidant and antimicrobial activities of the polysaccharides from red skin of lotus seed. Hubei Agr Sci. (2015) 54:6342–6345+6350. doi: 10.14088/j.cnki.issn0439-8114.2015.24.064
83. Ding, L, Zhou, G, and Han, S. Study on extraction of water soluble polysaccharide from lotus seed and its scavenging ability to free radicals. Food Sci. (2002) 23:252–4. doi: 10.3321/j.issn:1002-6630.2002.08.077
84. Miao, M, Xu, Y, and Fang, X. Antioxidation of lotus seed polysaccharide on aging mice. Chin J Mod Appl Pharm. (2005) 22:11–2. doi: 10.3969/j.issn.1007-7693.2005.01.004
85. Yu, Y, Wu, Y, and Pan, Y. Extraction-isolation and antioxidant activity of the polysaccharides from embryo of the seeds of Nelumbo nucifera Gaertn. J Zhejiang Univ. (2008) 35:48–51.
86. Yan, L, Shi, B, and Li, Q. Antioxidant properties of polysaccharide extracted and purified from lotus roots pomace. Food Sci. (2008) 29:66–9. doi: 10.3321/j.issn:1002-6630.2008.04.007
87. Yi, Y, Lamikanra, O, Sun, J, Wang, L, Min, T, and Wang, H. Activity diversity structure-activity relationship of polysaccharides from lotus root varieties. Carbohyd Polym. (2018) 190:67–76. doi: 10.1016/j.carbpol.2017.11.090
88. Yi, Y, Tang, H, Sun, Y, Xu, W, Min, T, and Wang, H. Comprehensive characterization of lotus root polysaccharide-phenol complexes. Food Chem. (2022) 366:130693. doi: 10.1016/j.foodchem.2021.130693
89. Yan, Y, Wang, Q, Sun, L, and Zhang, X. Extraction, preparation, and carboxymethyl of polysaccharide from lotus root. Food Sci Tech-Brazil. (2022) 42:17822. doi: 10.1590/fst.17822
90. Zhang, H, Wang, Q, Shi, L, Zhang, X, and Zhang, H. Synthesis and antioxidant activity of selenium polysaccharide from lotus root. Food Sci Tech-Brazil. (2022):42. doi: 10.1590/fst.40522
91. Miao, M, Yang, Y, and Fang, X. Effect of semen nelumbinis polysaccharide on the immune function of cyclophosphamide induced immunosuppressed mice. Chin J Tissue E Res. (2008) 12:10477–80. doi: 10.3321/j.issn:1673-8225.2008.53.027
92. Liao, C, and Lin, J. Lotus (Nelumbo nucifera Gaertn) plumule polysaccharide protects the spleen and liver from spontaneous inflammation in non-obese diabetic mice by modulating pro−/anti-inflammatory cytokine gene expression. Food Chem. (2011) 129:245–52. doi: 10.1016/j.foodchem.2011.03.103
93. Liao, C, and Lin, J. Purification, partial characterization and anti-inflammatory characteristics of lotus (Nelumbo nucifera Gaertn) plumule polysaccharides. Food Chem. (2012) 135:1818–27. doi: 10.1016/j.foodchem.2012.06.063
94. Liao, C, and Lin, J. Purified active lotus plumule (Nelumbo nucifera Gaertn) polysaccharides exert anti-inflammatory activity through decreasing toll-like receptor-2 and -4 expressions using mouse primary splenocytes. J Ethnopharmacol. (2013) 147:164–73. doi: 10.1016/j.jep.2013.02.028
95. Liao, C, and Lin, J. Lotus (Nelumbo nucifera Gaertn) plumule polysaccharide ameliorates pancreatic islets loss and serum lipid profiles in non-obese diabetic mice. Food Chem Toxicol. (2013) 58:416–22. doi: 10.1016/j.fct.2013.05.018
96. Le, B, Anh, P, and Yang, S. Polysaccharide derived from Nelumbo nucifera lotus plumule shows potential prebiotic activity and ameliorates insulin resistance in HepG2 cells. Polymers-Basel. (2021) 13:1780. doi: 10.3390/polym13111780
97. Luo, D, Zhou, T, Yuan, Z, and Cui, D. Hypoglyce mice activity of (Rhaizoma Nelumbo nucifera) polysaccharide and its effect in vivo antioxidant activity. J Anhui Agr Sci. (2011) 39:3334–3335+3385. doi: 10.3969/j.issn.0517-6611.2011.06.072
98. Zeng, Z, Xu, Y, and Zhang, B. Antidiabetic activity of a lotus leaf selenium (se)-polysaccharide in rats with gestational diabetes mellitus. Biol Trace Elem Res. (2017) 176:321–7. doi: 10.1007/s12011-016-0829-6
99. Li, W, Wu, D, Li, F, Gan, R, and Zou, L. Structural and biological properties of water soluble polysaccharides from lotus leaves: effects of drying techniques. Molecules. (2021) 26:4395. doi: 10.3390/molecules26154395
100. Wu, D, Feng, K, Li, F, Hu, Y, Wang, S, Gan, R, et al. In vitro digestive characteristics and microbial degradation of polysaccharides from lotus leaves and related effects on the modulation of intestinal microbiota. Curr Res Food Sci. (2022) 5:752–62. doi: 10.1016/j.crfs.2022.04.004
101. Tang, L. Stydies on Isolation, Purification, Structure and Function of Polysaccharides from Lotus Leaves (Master) Nanchang: Nanchang University (2007).
102. Xiong, J, Xu, K, Chen, M, Zhuo, X, and Xu, J. Studied on extraction and functional of the lotus leaf polysaccharides. Food Res Dev. (2014) 35:31–6. doi: 10.3969/j.issn.1005-6521.2014.21.008
103. Hwang, Y, Jang, S, Lee, A, Cho, C, Song, Y, Hong, H, et al. Polysaccharides isolated from lotus leaves (LLEP) exert anti-osteoporotic effects by inhibiting osteoclastogenesis. Int J Biol Macromol. (2020) 161:449–56. doi: 10.1016/j.ijbiomac.2020.06.059
104. Du, Y, Wan, H, Huang, P, Yang, J, and He, Y. A critical review of Astragalus polysaccharides: from therapeutic mechanisms to pharmaceutics. Biomed Pharmacother. (2022) 147:112654. doi: 10.1016/j.biopha.2022.112654
105. Zhang, S, Hu, J, Sun, Y, Tan, H, Yin, J, Geng, F, et al. Review of structure and bioactivity of the Plantago (Plantaginaceae) polysaccharides. Food Chem X. (2021) 12:100158. doi: 10.1016/j.fochx.2021.100158
106. Qiang, W, Hongzhi, L, and Kui, Z. Review on the relationship of polysaccharide molecular chain comformation changes and bioactivities. Curr Biotechnol. (2011) 1:318–26.
107. Stephanie, B, Eric, D, Sophie, FM, Christian, B, and Yu, G. Carrageenan from Solieria chordalis (Gigartinales): structural analysis and immunological activities of the low molecular weight fractions. Carbohyd Polym. (2010) 81:448–60. doi: 10.1016/j.carbpol.2010.02.046
108. Gong, H, Gan, X, Li, Y, Chen, J, Xu, Y, Shi, S, et al. Review on the genus Polygonatum polysaccharides: extraction, purification, structural characteristics and bioactivities. Int J Biol Macromol. (2023) 229:909–30. doi: 10.1016/j.ijbiomac.2022.12.320
109. Lin, B, and Huang, G. Extraction, isolation, purification, derivatization, bioactivity, structure-activity relationship, and application of polysaccharides from white jellyfungus. Biotechnol Bioeng. (2022) 119:1359–79. doi: 10.1002/bit.28064
110. Fernandes, PAR, and Coimbra, MA. The antioxidant activity of polysaccharides: a structure-function relationship overview. Carbohyd Polym. (2023) 314:120965. doi: 10.1016/j.carbpol.2023.120965
111. Yuan, S, Dong, P, Ma, H, Liang, S, Li, L, and Zhang, X. Antioxidant and biological activities of the lotus root polysaccharide-iron (III) complex. Molecules. (2022) 27:7106. doi: 10.3390/molecules27207106
112. Zhou, T, Yuan, Z, and Cui, D. Experimental study on anti fatigue activity of lotus root polysaccharide health drink. Jiangsu Agr Sci. (2011) 1:329–30. doi: 10.15889/j.issn.1002-1302.2011.01.018
113. Braz, R, Hechenleitner, AAW, and Cavalcanti, OA. Extraction, structural modification and characterization of lotus roots polysaccharides (Nelumbo nucifera Gaertn). Excipient with potential application in modified drug delivery systems. Lat Am J Pharm. (2007) 26:706–10. doi: 10.1007/s10118-013-1300-1
Keywords: lotus, polysaccharides, separation, structural features, biological activities, applications, SAR
Citation: Dai G, Wang J, Zheng J, Xia C, Wang Y and Duan B (2023) Bioactive polysaccharides from lotus as potent food supplements: a review of their preparation, structures, biological features and application prospects. Front. Nutr. 10:1171004. doi: 10.3389/fnut.2023.1171004
Edited by:
Lisa He, Harvard Medical School, United StatesReviewed by:
Ya-Fang Shang, Hefei University of Technology, ChinaHongliang Zeng, Fujian Agriculture and Forestry University, China
Copyright © 2023 Dai, Wang, Zheng, Xia, Wang and Duan. This is an open-access article distributed under the terms of the Creative Commons Attribution License (CC BY). The use, distribution or reproduction in other forums is permitted, provided the original author(s) and the copyright owner(s) are credited and that the original publication in this journal is cited, in accordance with accepted academic practice. No use, distribution or reproduction is permitted which does not comply with these terms.
*Correspondence: Baozhong Duan, YnpkdWFuQDEyNi5jb20=; Yaping Wang, eXB3YW5nMzBAMTYzLmNvbQ==