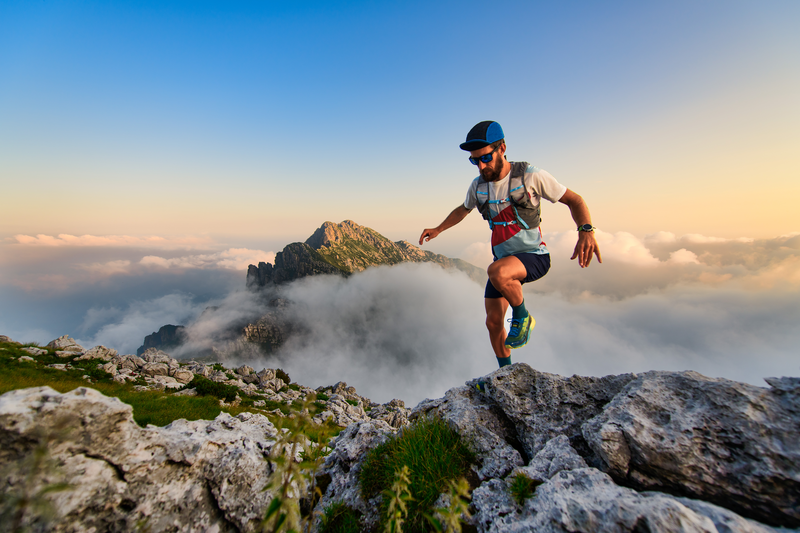
95% of researchers rate our articles as excellent or good
Learn more about the work of our research integrity team to safeguard the quality of each article we publish.
Find out more
ORIGINAL RESEARCH article
Front. Nutr. , 14 August 2023
Sec. Nutritional Epidemiology
Volume 10 - 2023 | https://doi.org/10.3389/fnut.2023.1167372
This article is part of the Research Topic Dietary Patterns in Cancer Prevention and Survival View all 17 articles
Background: Sulfur microbial diet (SMD), related to the enrichment of sulfur-metabolizing gut bacteria, has been confirmed to be linked to an elevated risk of early-onset colorectal adenoma in young females. However, it remains unclear whether SMD is associated with the risk of colorectal adenoma in older people, who are at greater risk for colorectal cancer.
Methods: All data on participants in this study were retrieved from the intervention arm of the Prostate, Lung, Colorectal, and Ovarian (PLCO) cancer screening test. Participants’ adherence to this dietary pattern was assessed using SMD score. Hazard ratios (HR) and 95% confidence intervals (CI) were adopted in Cox proportional hazards regression models to assess the link between SMD score and the incidence of colorectal adenoma in participants included in the study. Specific stratified analyses were constructed to assess whether this association changed in different conditions, whereas the robustness of the association was examined through sensitivity analyses.
Results: The mean baseline age of participants was 62.1 (SD 5.2) years (range 54.0–75.0 years). During 19,468,589 person-years of follow-up, 992 colorectal adenoma cases were documented in a total of 17,627 included participants. In a fully adjusted model, an increased risk of colorectal adenoma was determined in participants in the highest quartile of SMD score in comparison with those in the lowest quartile (HRquartile4 vs. HRquartile1 = 1.23; 95% CI: 1.02, 1.47; p = 0.017 for trend). This positive association between SMD score and adenoma risk was more evident in participants who were current or former smokers (p = 0.029 for interaction).
Conclusion: In this study, our results support a role for the SMD in the carcinogenicity of colorectal cancer precursors among older adults. Nevertheless, these results require validation through more research.
The second most prevalent cause of cancer mortality in the United States is colorectal cancer (CRC), which is considered to be the fourth most frequently diagnosed malignancy (1). Around 41,000 fatalities and 147,000 new cases of colorectal cancer are expected to be diagnosed by 2040 in the United States (2). Recently, research has increasingly indicated that the incidence of early-onset colorectal cancer is increasing rapidly, while the incidence of colorectal cancer after 50 years is gradually decreasing (3). However, it is undeniable that colorectal cancer diagnosed after the age of 50 years still constitutes the majority of newly diagnosed colorectal cancer. Approximately 90% of all colorectal cancer patients are diagnosed after 50 years of age (4). Identifying the high-risk factors for colorectal cancer in the elderly is still the focus of attention.
Colorectal traditional adenoma, as one of the recognized precursors of colorectal cancer, accounts for about 60–80% of sporadic CRC cases (5, 6). Although the majority of traditional adenomas can be removed by colonoscopy, recurrence is observed in nearly 50% of patients at 1 year of follow-up (7). Therefore, early identification of possible risk factors for traditional adenoma is of great significance to reduce the incidence of colorectal cancer. Recently, research has focused on the effects of diet, gut microbiota, and bacterial metabolites on the risk of colorectal cancer (8, 9). A sulfur microbial diet (SMD), which is related to the enrichment of sulfur-metabolizing gut microbiota, was constructed by Nguyen et al. through a large prospective study involving 51,529 U.S. males to investigate its effect on CRC risk (10). Specifically, SMD consists primarily of foods associated with CRC risk (such as decreased legumes and vegetables and an increase in processed meats), and long-term compliance to this diet was linked to a 43% greater risk of distal colon and rectum in this cohort (10). In a study involving a cohort of young female nurses aged 25–42, it was demonstrated that SMD is associated with a 58% increased risk of early-onset colorectal adenoma (11). Given the sex and occupational limitations of the populations included in the above studies, it remains unclear whether SMD is associated with the risk of colorectal adenoma in older individuals, who are at greater risk for colorectal cancer.
Hence, to determine the link between the SMD with the incidence of colorectal adenoma in the population older than 50, a prospective investigation in a large cohort of older adults was executed in the Prostate, Lung, Colorectal, and Ovarian (PLCO) Cancer Screening Trial.
Details of the protocol and statistical analysis plan of the PLCO trial are available on this website,1 the recruitment plan for the study population and the objectives of the study had been thoroughly described in previous literatures (12–14). In brief, the PLCO trial recruited almost 155,000 men and women 55–74 years of age through competitively selected screening centers across the United States during the period of 1993–2001. In subsequent studies, the recruited population was assigned to the intervention or control arms in a 1:1 ratio by a reliable, secure randomized algorithm. The follow-up period continued until 2009–2018 to evaluate the effectiveness of early cancer screening (13). Each participant was required to submit a baseline questionnaire (BQ) covering self-administered risk factors. Additionally, the intervention arm participants were guided to fill in a dietary questionnaire (DQX) documenting daily dietary intake within 1 year and undergo screening programs including 60 cm flexible sigmoidoscopy (15). This trial was approved by the NCI Division of Cancer Prevention and Control, and written informed consent was obtained from the included participants.
The association between SMD and the incidence of colorectal adenoma was determined by executing a detailed nested case–control investigation limited to the intervention arm. Considering the purpose of this study, participants with the following conditions were excluded: (1) did not return complete baseline information; (2) did not return a valid DQX (including the completion date was either missing, or was later to the date of death; at least eight missing frequency responses were available; extreme calorie intake for each gender); (3) confirmed cancer before DQX entry; (4) out of the incident adenoma cohort (the identification: a negative screen at baseline and either a negative screen at T3/T5 or a positive screen at T3/T5 with a left-sided adenoma found on follow-up to the screen); (5) with an inadequate flexible sigmoidoscopy (insertion ≥50 cm with ≥90% of mucosa visualized); (6) received diagnosis of cancer before colorectal adenoma; (7) received a diagnosis of colorectal adenoma before returning a valid DQX; (8) had a history of colon-linked comorbidity (such as Gardner’s syndrome, ulcerative colitis, Crohn’s disease or familial polyposis); (9) had a history of colorectal polyps (Figure 1). Ultimately, the included participants of the study reached 17,627 in total (992 incident colorectal adenoma cases [655 males; 337 females] and 16,635 controls [9,213 males; 7,422 females]). This study was carried out with the permission of the United States NCI (CDAS project “PLCO-1070”).
Figure 1. The flow chart of identifying subjects included in our study. PLCO, prostate, lung, colorectal, and ovarian; BQ, baseline questionnaire; DQX, dietary questionnaire.
This research involved data concerning demographics, lifestyle, and medical history, including age, sex, race, body mass index (BMI), smoking status, smoking pack-years, as well as the history of aspirin consumption, diabetes, hypertension, diverticulitis or diverticulosis, polyps and colonoscopy in the past 3 years retrieved through BQ. Dietary information, including total energy intake, and dietary food or nutrient intake, can be obtained through DQX. The DQX, which counted information on the intake frequency of 137 food items and consisted primarily of 61 Willett FFQ items, has been shown to provide effective, adequate information about the dietary intake of participants over a 1-year period (16–18). The supplemental questionnaire (SQX) was employed to investigate some items not reported in BQ, such as physical activity level, defined as the summarized weekly minutes of self-reported moderate to vigorous activity.
In an initial prospective study of 51,529 male from medical specialties, researchers developed the SMD score by assessing the correlation coefficient of sulfur-metabolizing bacteria abundance in the stools to food groups (10). The detailed components of SMD followed: (1) the positive correlation group included processed meats, liquor and low-calorie drinks; (2) the negative correlation group included beer, fruit juice, legumes, other vegetables and sweets. However, the specific components of SMD developed in another prospective study of 214,797 male and female from medical specialties by the same way were different, compared with the original study (11). In detail, the positive correlation group included low-calorie beverages, French fries, red meats, and processed meats, while negative correlation group included fruits, yellow vegetables, whole grains, legumes, leafy vegetables, and cruciferous vegetables. Considering the contribution of whole grains and sweets to colorectal cancer risk (19–21), we adjusted the specific components of SMD based on previous prospective studies (10, 11, 22). The adjusted SMD score was the sum of the quartile values from 1 to 4 of 8 components, consisting of processed meats, liquor and low-calorie drinks (higher quartiles of intake indicate higher scores); and beer, fruit drinks, legumes, whole grain, other vegetables (higher quartiles of intake indicate lower scores). Thus, the SMD score with a total score ranging from 8 to 32 could be used to assess adherence to this pattern of intake, with higher score indicating greater adherence. Specific food intake and corresponding distribution scores can be found in Supplementary Table S1. In subsequent studies, SMD score were categorized into quartiles.
As required in the PLCO trial, participants in the incident adenoma cohort are required to complete a screening colonoscopy at baseline. Subjects with negative results are allowed to enter a follow-up study and must complete at least one additional screening colonoscopy at T3 or T5. It means that none of the participants in this study had a diagnosis of colorectal adenoma at baseline, and all adenoma identifications during screening colonoscopy were confirmed by biopsied and further histological type. According to the current US guidelines for colonoscopy, conventional adenomas were categorized hierarchically: (1) any adenoma ≥1 cm, with high-grade dysplasia, or with tubulovillous or villous histology should be considered as advanced adenoma; (2) while only for the adenoma <1 cm and lacking advanced histology the diagnosis of non-advanced adenoma is considered (23).
In this study, the data of some covariates were observed to be missing to varying degrees. Hence, for categorical and continuous variables with missing values reported at <5%, namely smoking status, pack-years, as well as the history of colonoscopy, aspirin usage, hypertension, diabetes, family history of colorectal cancer, and BMI, the missing data was imputed utilizing the modal value and the median, respectively (24). As for the variable “physical activity level” with 22.8% missing data, which were assumed to be randomly distributed, multiple imputations were done to complete them (25). The details of imputation values can be found in Supplementary Table S2.
The Cox proportional hazards regression model was constructed with follow-up time as the time variable for estimation of the 95% confidence interval (CIs) and hazard ratios (HRs) of the relationship between SMD score and the risk of colorectal adenoma. It should be emphasized that the main outcome event in this research was the confirmation of adenoma. In this research, the follow-up time was defined as the data from DQX completion to the diagnosis of adenoma, cancer, fatality, loss of follow-up, or end of follow-up (December 31, 2009), whichever came first (Figure 2).
To investigate the existence of a linear trend between quartiles of SMD score and the risk of colorectal adenoma, each participant in the quartile was assigned the median value of the quartile. This was then considered as a continuous variable in cox regression to order to get its p-value, with the reference group considered to be the lowest quartile. As per prior literature review and clinical judgment, sex, race, age, and education levels, total energy intake, BMI, aspirin usage, smoking status, smoking pack-years, as well as the history of hypertension, diverticulitis or diverticulosis, diabetes, colonoscopy, family history of colorectal cancer in past 3 years and physical activity level were adjusted as covariates in multiple regression analyses (11, 22, 26). Meanwhile, 12,916 participants with complete data were selected to test whether the analysis result was influenced by missing data imputation by repeating the same multiple regression analyses. To present colorectal adenoma risk across the full range of SMD score, a restricted cubic spline model with three knots at the 10th, 50th, and 90th was constructed in this study (27).
The influence of various factors on the observed association of SMD score with risk of colorectal adenoma was assessed by means of a series of pre-selected subgroup analyses including age (>65 vs. ≤65 years old), sex (male vs. female), BMI (≤30 vs. >30 kg/m2), smoking status (non-smokers vs. current/former smokers), smoking pack-years (≤median vs. >median years), family history of colorectal cancer (no vs. yes/possible), and history of aspirin consumption (no vs. yes). To verify the robustness of these findings, several sensitivity analyses were carried out: (1) exclusion of participants with a history of diverticulitis or diverticulosis; (2) exclusion of participants with a history of diabetes (more likely to have colorectal adenoma) (28); (3) exclusion of participants with a family history of colorectal cancer; (4) exclusion of colorectal adenoma cases observed within the first two and four years of follow-up to examine the likelihood of the observed association being caused by reverse causation; (5) Further adjusting model 2 for the Healthy Eating Index-2015 (HEI-2015) to determine if the observed link was diet quality-mediated.
All statistical analyses were completed through the software R 4.2.1. Furthermore, a two-tailed p-value less than 0.05 indicated the significance level.
A total of 17,627 participants [9,868 (55.08%) males and 7,759 (44.02%) females] were involved in the current analysis. The mean (standard deviation) baseline age of participants was 62.1 (5.2) years (range 54.0–75.0 years). The primary baseline features of participants per the quarters of the SMD score were depicted in tabular form (Table 1). In contrast with the lowest quartile (Q1), participants in the highest quartile (Q4) of SMD score tended to be younger, with more smoking pack-years, a higher BMI, a history of hypertension and diabetes, lower energy intake from diet, decreased physical activity level, and were less likely to be non-smokers, were regular users of aspirin, and had a family history of colorectal cancer. Additionally, in contrast to Q1, the participants of the Q4 of SMD score had increased intakes of processed meat, liquor, and low-calorie drinks but lower intakes of beer, fruit drinks, legumes, whole grains, and other vegetables.
Table 1. Baseline characteristics of study population according to overall sulfur microbial diet score.
In this study, a total of 992 newly diagnosed conventional colorectal adenomas were documented during 19,468,589 person-years of follow-up, with an overall incidence rate of 0.51 cases per 1,000 person-years. The mean (standard deviation) follow-up length was 11.04 (3.50) years. In univariable analysis, in contrast with Q1, the participants of Q4 of SMD score were found to be at increased risk of colorectal conventional adenoma (HRquartile4: HRquartile1 = 1.28; 95% CI: 1.08, 1.51; p = 0.003 for trend Table 2). Subsequent to thorough adjustment for all possible confounders, the association of SMD score with the risk of conventional adenoma remained a positive one (HRquartile4: HRquartile1 = 1.23; 95% CI: 1.02, 1.47; p = 0.017 for trend Table 2). Notably, the repetition of the aforementioned analysis in a cohort of 12,916 participants with complete data resulted in similar data (HRquartile4: HRquartile1 = 1.24; 95% CI: 1.00, 1.54; p = 0.029 for trend; Supplementary Table S3).
Table 2. Hazard ratios of the association of SMD score with the risk of colorectal cancer precursors.
In the whole study population, the linearity assumptions between SMD score and risk of colorectal conventional adenomas were validated by the restricted cubic spline (p = 0.100 for nonlinearity; Figure 3). The result of subgroup analysis in this study suggested that the status of smoking significantly modified the association between SMD score and incidence of conventional adenoma (p = 0.029 for interaction; Table 3). When compared with the lowest quartile of SMD score, HRs (95%CI) of incidence for the highest quartile of SMD score in the subsets of current or former smoker factors was 1.43 (1.12, 1.83). In addition, the positive association between SMD score and colorectal adenoma risk was depicted as more pronounced in males (HRquartile4: HRquartile1 = 1.28; 95% CI: 1.02, 1.62; Table 3) than in females (HRquartile4: HRquartile1 = 1.07; 95% CI: 0.77, 1.51; Table 3), though the interaction test was not statistically significant (concerning interaction p = 0.192). No other interactions were statistically significant (concerning interaction all p > 0.05; Table 3). The sensitivity analysis showed the initial associations of SMD score with risks of conventional adenoma were not impacted considerably through the exclusion of participants with specific preset conditions or further adjusting Healthy Eating Index-2015 (all p < 0.05 for trend; Table 4), which fully supports the stability of our findings.
Figure 3. Dose–response analysis on the association of SMD score with the risk of colorectal adenoma (including total adenoma, advanced adenoma and non-advanced adenoma). Hazard ratios was adjusted for age, sex, race, education levels, smoking status, pack-years of smoking, BMI, aspirin use, history of hypertension, history of diabetes, family history of colorectal cancer, total energy intake, history of diverticulitis or diverticulosis, history of colonoscopy in past 3 years, and physical activity level.
Table 3. Subgroup analyses on the association of SMD score with the risk of colorectal cancer precursor.
Table 4. Sensitivity analyses on the association of SMD score with the risk of colorectal cancer precursors.
Based on a prospective large cohort study with adequate colonoscopy, the link between the SMD score and colorectal adenoma risk in the older population was assessed. According to the findings, following the SMD for a prolonged period of time was linked to an elevated risk of developing colorectal adenoma. The dose–response analysis also showed a linear trend of increasing the risk of colorectal adenoma with SMD score, suggesting that the risk of adenoma may increase in parallel with the increase in SMD score. The robustness of these findings was confirmed by subsequent sensitivity analysis. Our subgroup analysis showed that the positive association of SMD score with colorectal adenoma risk was only predominantly found in males, but not in females.
To develop a specific dietary pattern related to sulfur-metabolizing bacteria, Nguyen et al. analyzed the correlation of sulfur-metabolizing bacteria in stool samples with respective dietary components from 307 males (10). In his research, two main sulfur-metabolizing bacteria, Erysipelotrichaceae bacterium 21_3 and Bilophila wadsworthia were identified to be associated with dietary (10), which has been previously confirmed to notably increase in the gut of patients with colorectal cancer or adenoma (29–31). Increasingly, research has shown that diet has a substantial influence on gut microbes, leading to an elevated risk of developing colorectal tumors via the colorectal adenoma-carcinoma sequence (32–34). It is well-recognized that sulfur-metabolizing microbes are involved in the conversion of dietary sulfur into hydrogen sulfide (H2S) in the gut, which contributes to the increased prevalence of colorectal tumors (35–38). Specifically, high concentrations of hydrogen sulfide in the intestine may raise the risk of colorectal tumors by damaging DNA in epithelial cells (39), promoting immune cell alterations associated with colorectal cancer (36), and damaging the bilayer of the intestinal mucosa (40, 41).
Notably, SMD score and smoking status depicted a significant interaction concerning the increased colorectal adenoma risk in the subgroup analysis (p = 0.029 for interaction). This means that participants who were former or current smokers would have an elevated risk of colorectal adenoma in comparison to participants who never smoked with the same SMD score, which was consistent with the previous study (22). One of the major risk factors for colorectal adenoma or colorectal cancer is considered to be smoking (42–44). Previous studies have shown that smoking leads to a significant shift in the gut microbiome of humans (45–47), which may be responsible for the increased risk of colorectal adenoma or colorectal cancer. However, microbial species changed by smoking mainly consisted of Prevotella, Veillonella, Bacteroides, and Acidaminococcus (46–48), which were different from those changed by the SMD. This may suggest that smoking may increase colorectal adenoma risk through different mechanisms, compared with a sulfur microbial diet. Recently, Bai et al. explored the mechanism of smoking and gut microbial-mediated colorectal tumorigenesis in mice, the result demonstrating that smoking can promote colonic tumorigenesis by modulating the components of the gut microbiota and inducing dysbiosis of the gut microbiota (49). Smoking may promote colorectal tumorigenesis which results in impairing the gut barrier function, promoting inflammation in colon tumorigenesis, and enhancing oncogenic MAPK/ERK signaling in colonic epithelium (49), which is partially similar to the mechanism of H2S promoting colorectal tumors (36, 40, 41). Smoking may modulate the abundance of microbiota other than sulfur-metabolizing microorganisms in the gut to have some synergistic effect on the increased colorectal adenoma risk induced by the SMD, which may provide a possible explanation for these results. However, this explanation needs to be confirmed by further investigating the interactions between the different microbiota mentioned above.
Intriguingly, our subgroup analysis revealed a more pronounced positive association between adherence to SMD and the risk of colorectal adenomas in males. Several potential explanations can shed light on this observation. Firstly, in our study cohort, males constituted a higher proportion of smokers, comprising approximately 66% of all current or former smokers. Moreover, our subgroup analysis indicated a significant interaction between smoking and SMD adherence in increasing the risk of colorectal adenomas. Given that smoking is a known contributor to colorectal adenoma risk (50), this difference in smoking prevalence between genders may contribute to the sex-specific association observed in colorectal adenoma incidence. On another note, Liu et al. demonstrated that adherence to SMD was linked to an increase risk of obesity (51). Their gender-specific stratified analysis further suggested a more substantial positive association between SMD adherence and obesity risk in males compared to females (51). Considering that obesity is a significant risk factor for colorectal cancer and adenoma (52, 53), the variation in the association between obesity and adherence to the SMD pattern across genders may be the reason why the association between SMD and colorectal adenoma risk is more significant in males.
This study has several strengths. First, unlike previous studies that conducted their study only on health professionals (10, 11, 22), the population in this study was more representative because an almost equal proportion of male and female participants were involved, with no occupational restrictions, who received the same care in different practice settings across the United States. Second, participants with inadequate flexible sigmoidoscopy were excluded, which guaranteed the effectiveness of the colonoscopy. Third, considering the inherent influence of colon-related complications with a genetic predisposition on the incidence of colorectal cancer (such as Crohn’s disease, Gardner’s syndrome, ulcerative colitis, or familial polyposis) (54–57), participants with colon-related complications were excluded to minimize the interference of genetic factors on the study results. Notably, this research confirms for the first time that SMD is linked to an elevated risk of colorectal adenoma in the older individuals. Given the higher risk of colorectal tumors in the elderly population compared to the younger population (4), this research will provide a new dietary guideline for them to minimize the incidence of CRC in this high-risk population.
This research is restricted in some aspects. The microbiota in the stool samples of participants was not analyzed due to some limitations, therefore, the shift in the intestinal microbiota of participants could not be guaranteed to be consistent with previous studies. However, SMD-related analyses have been adequately validated in several various study cohorts (10, 11, 22), making this deficiency acceptable. In addition, the dietary intake of SMD using DQX was calculated only once at baseline, rather than calculating the cumulative mean at long-term follow-up, which may lead to nondifferential bias. However, based on a classical assumption in nutrition, the exposure measured at baseline is more reflective of the daily dietary habits of participants in the years before and following inclusion in the study (24). Hence, these calculations for the dietary intake of participants can be considered valid.
To summarize, our study findings revealed a positive correlation between SMD score and conventional colorectal adenomas risk in an elderly population in the United States, with a median follow-up of 11 years. Furthermore, this positive association is more significant in males. Smoking may have a synergistic effect on the positive association between SMD and colorectal adenoma by modulating intestinal microbiota, which differed from the sulfur-metabolizing bacteria, and the exact mechanism needs to be elucidated by subsequent in-depth studies on the mechanism of intestinal microbiota interactions.
The raw data supporting the conclusions of this article will be made available by the authors, without undue reservation.
The studies involving human participants were reviewed and approved by the Institutional Review Board of the National Cancer Institute. The patients/participants provided their written informed consent to participate in this study.
HH, YW, YJ, and YX contributed to the study design and data analysis. YX and YJ contributed to the data interpretation and writing of the manuscript. YX, LX, ZX, XR, BL, YJ, ZyZ, HZ, YT, HL, QW, ZhZ, HH, and HG contributed to the data collection, and data curation of the present analysis. LP, LX, YW, HH, and HG assisted with statistical analysis and funding acquisition. YJ and HH made significant contributions to the revised manuscript. All of the authors reviewed or revised the manuscript. All authors contributed to the article and approved the submitted version. The work reported in the paper has been performed by the authors, unless clearly specified in the text.
This work was supported by the General Project of Chongqing Natural Science Foundation, Chongqing Science and Technology Commission, China [cstc2021jcyj-msxmX0153 (LP)], [cstc2021jcyj-msxmX0112 (YW)], and [CSTB2022NSCQ-MSX1005 (HG)], and Kuanren Talents Project of the Second Affiliated Hospital of Chongqing Medical University in China [kryc-yq-2110 (HG)]. The funders had no role in the study design or implementation; data collection, management, analysis or interpretation; manuscript preparation, review or approval; or the decision to submit the manuscript for publication.
The authors sincerely appreciate the PLCO study group and PLCO participants. This research has been conducted using the PLCO resource (https://cdas.cancer.gov/plco/) under application number PLCO-1070.
The authors declare that the research was conducted in the absence of any commercial or financial relationships that could be construed as a potential conflict of interest.
All claims expressed in this article are solely those of the authors and do not necessarily represent those of their affiliated organizations, or those of the publisher, the editors and the reviewers. Any product that may be evaluated in this article, or claim that may be made by its manufacturer, is not guaranteed or endorsed by the publisher.
The Supplementary material for this article can be found online at: https://www.frontiersin.org/articles/10.3389/fnut.2023.1167372/full#supplementary-material
1. Siegel, RL, Miller, KD, and Jemal, A. Cancer Statistics, 2018. CA Cancer J Clin. (2018) 68:7–30. doi: 10.3322/caac.21442
2. Rahib, L, Wehner, MR, Matrisian, LM, and Nead, KT. Estimated projection of US cancer incidence and death to 2040. JAMA Netw Open. (2021) 4:e214708. doi: 10.1001/jamanetworkopen.2021.4708
3. Sinicrope, FA. Increasing incidence of early-onset colorectal cancer. N Engl J Med. (2022) 386:1547–58. doi: 10.1056/NEJMra2200869
4. Collaborative, R, Zaborowski, AM, Abdile, A, Adamina, M, Aigner, F, d'Allens, L, et al. Characteristics of early-onset vs late-onset colorectal cancer: a review. JAMA Surg. (2021) 156:865–74. doi: 10.1001/jamasurg.2021.2380
5. Conteduca, V, Sansonno, D, Russi, S, and Dammacco, F. Precancerous colorectal lesions (review). Int J Oncol. (2013) 43:973–84. doi: 10.3892/ijo.2013.2041
6. De Palma, FDE, D'Argenio, V, Pol, J, Kroemer, G, Maiuri, MC, and Salvatore, F. The molecular hallmarks of the serrated pathway in colorectal cancer. Cancers (Basel). (2019) 11:1017. doi: 10.3390/cancers11071017
7. Emami, MH, Salehi, M, Hassanzadeh Keshteli, A, Mansourian, M, Mohammadzadeh, S, and Maghool, F. Calcium and dairy products in the chemoprevention of colorectal adenomas: a systematic review and meta-analysis. Crit Rev Food Sci Nutr. (2022) 62:7168–83. doi: 10.1080/10408398.2021.1911927
8. Loke, YL, Chew, MT, Ngeow, YF, Lim, WWD, and Peh, SC. Colon carcinogenesis: the interplay between diet and gut microbiota. Front Cell Infect Microbiol. (2020) 10:603086. doi: 10.3389/fcimb.2020.603086
9. Konstantinov, SR. Diet, microbiome, and colorectal cancer. Best Pract Res Clin Gastroenterol. (2017) 31:675–81. doi: 10.1016/j.bpg.2017.09.007
10. Nguyen, LH, Ma, W, Wang, DD, Cao, Y, Mallick, H, Gerbaba, TK, et al. Association between sulfur-metabolizing bacterial communities in stool and risk of distal colorectal cancer in men. Gastroenterology. (2020) 158:1313–25. doi: 10.1053/j.gastro.2019.12.029
11. Nguyen, LH, Cao, Y, Hur, J, Mehta, RS, Sikavi, DR, Wang, Y, et al. The sulfur microbial diet is associated with increased risk of early-onset colorectal cancer precursors. Gastroenterology. (2021) 161:1423–1432.e4. doi: 10.1053/j.gastro.2021.07.008
12. Prorok, PC, Andriole, GL, Bresalier, RS, Buys, SS, Chia, D, Crawford, ED, et al. Design of the prostate, lung, colorectal and ovarian (PLCO) cancer screening trial. Control Clin Trials. (2000) 21:273S–309S. doi: 10.1016/s0197-2456(00)00098-2
13. Gohagan, JK, Broski, K, Gren, LH, Fouad, MN, Higgins, D, Lappe, K, et al. Managing multi-center recruitment in the PLCO cancer screening trial. Rev Recent Clin Trials. (2015) 10:187–93. doi: 10.2174/157488711003150928123938
14. Gohagan, JK, Prorok, PC, Greenwald, P, and Kramer, BS. The PLCO cancer screening trial: background, goals, organization, operations. Results Rev Recent Clin Trials. (2015) 10:173–80. doi: 10.2174/1574887110666150730123004
15. Weissfeld, JL, Schoen, RE, Pinsky, PF, Bresalier, RS, Church, T, Yurgalevitch, S, et al. Flexible sigmoidoscopy in the PLCO cancer screening trial: results from the baseline screening examination of a randomized trial. J Natl Cancer Inst. (2005) 97:989–97. doi: 10.1093/jnci/dji175
16. Subar, AF, Ziegler, RG, Thompson, FE, Johnson, CC, Weissfeld, JL, Reding, D, et al. Is shorter always better? relative importance of questionnaire length and cognitive ease on response rates and data quality for two dietary questionnaires. Am J Epidemiol. (2001) 153:404–9. doi: 10.1093/aje/153.4.404
17. Subar, AF, Thompson, FE, Kipnis, V, Midthune, D, Hurwitz, P, McNutt, S, et al. Comparative validation of the block, willett, and national cancer institute food frequency questionnaires: the eating at America's table study. Am J Epidemiol. (2001) 154:1089–99. doi: 10.1093/aje/154.12.1089
18. Willett, WC, Sampson, L, Stampfer, MJ, Rosner, B, Bain, C, Witschi, J, et al. Reproducibility and validity of a semiquantitative food frequency questionnaire. Am J Epidemiol. (1985) 122:51–65. doi: 10.1093/oxfordjournals.aje.a114086
19. Aune, D, Chan, DS, Lau, R, Vieira, R, Greenwood, DC, Kampman, E, et al. Dietary fibre, whole grains, and risk of colorectal cancer: systematic review and dose-response meta-analysis of prospective studies. BMJ. (2011) 343:d6617. doi: 10.1136/bmj.d6617
20. Tabung, FK, Brown, LS, and Fung, TT. Dietary patterns and colorectal cancer risk: a review of 17 years of evidence (2000-2016). Curr Colorectal Cancer Rep. (2017) 13:440–54. doi: 10.1007/s11888-017-0390-5
21. Keum, N, and Giovannucci, E. Global burden of colorectal cancer: emerging trends, risk factors and prevention strategies. Nat Rev Gastroenterol Hepatol. (2019) 16:713–32. doi: 10.1038/s41575-019-0189-8
22. Wang, Y, Nguyen, LH, Mehta, RS, Song, M, Huttenhower, C, and Chan, AT. Association between the sulfur microbial diet and risk of colorectal cancer. JAMA Netw Open. (2021) 4:e2134308. doi: 10.1001/jamanetworkopen.2021.34308
23. Lieberman, DA, Rex, DK, Winawer, SJ, Giardiello, FM, Johnson, DA, and Levin, TR. Guidelines for colonoscopy surveillance after screening and polypectomy: a consensus update by the US multi-society task force on colorectal cancer. Gastroenterology. (2012) 143:844–57. doi: 10.1053/j.gastro.2012.06.001
24. Srour, B, Fezeu, LK, Kesse-Guyot, E, Alles, B, Mejean, C, Andrianasolo, RM, et al. Ultra-processed food intake and risk of cardiovascular disease: prospective cohort study (nutrinet-Sante). BMJ. (2019) 365:1451. doi: 10.1136/bmj.l1451
25. Mainzer, R, Apajee, J, Nguyen, CD, Carlin, JB, and Lee, KJ. A comparison of multiple imputation strategies for handling missing data in multi-item scales: guidance for longitudinal studies. Stat Med. (2021) 40:4660–74. doi: 10.1002/sim.9088
26. Sninsky, JA, Shore, BM, Lupu, GV, and Crockett, SD. Risk factors for colorectal polyps and cancer. Gastrointest Endosc Clin N Am. (2022) 32:195–213. doi: 10.1016/j.giec.2021.12.008
27. Desquilbet, L, and Mariotti, F. Dose-response analyses using restricted cubic spline functions in public health research. Stat Med. (2010) 29:1037–57. doi: 10.1002/sim.3841
28. Ottaviano, LF, Li, X, Murray, M, Frye, JT, Lung, BE, Zhang, YY, et al. Type 2 diabetes impacts colorectal adenoma detection in screening colonoscopy. Sci Rep. (2020) 10:7793. doi: 10.1038/s41598-020-64344-2
29. Chen, W, Liu, F, Ling, Z, Tong, X, and Xiang, C. Human intestinal lumen and mucosa-associated microbiota in patients with colorectal cancer. PLoS One. (2012) 7:e39743. doi: 10.1371/journal.pone.0039743
30. Yazici, C, Wolf, PG, Kim, H, Cross, TL, Vermillion, K, Carroll, T, et al. Race-dependent association of sulfidogenic bacteria with colorectal cancer. Gut. (2017) 66:1983–94. doi: 10.1136/gutjnl-2016-313321
31. Feng, Q, Liang, S, Jia, H, Stadlmayr, A, Tang, L, Lan, Z, et al. Gut microbiome development along the colorectal adenoma-carcinoma sequence. Nat Commun. (2015) 6:6528. doi: 10.1038/ncomms7528
32. David, LA, Maurice, CF, Carmody, RN, Gootenberg, DB, Button, JE, Wolfe, BE, et al. Diet rapidly and reproducibly alters the human gut microbiome. Nature. (2014) 505:559–63. doi: 10.1038/nature12820
33. Desai, MS, Seekatz, AM, Koropatkin, NM, Kamada, N, Hickey, CA, Wolter, M, et al. A dietary fiber-deprived gut microbiota degrades the colonic mucus barrier and enhances pathogen susceptibility. Cells. (2016) 167:1339–53 e21. doi: 10.1016/j.cell.2016.10.043
34. Sanchez-Alcoholado, L, Ramos-Molina, B, Otero, A, Laborda-Illanes, A, Ordonez, R, Medina, JA, et al. The role of the gut microbiome in colorectal cancer development and therapy response. Cancers (Basel). (2020) 12:1046. doi: 10.3390/cancers12061406
35. Guo, FF, Yu, TC, Hong, J, and Fang, JY. Emerging roles of hydrogen sulfide in inflammatory and neoplastic colonic diseases. Front Physiol. (2016) 7:156. doi: 10.3389/fphys.2016.00156
36. Cai, WJ, Wang, MJ, Ju, LH, Wang, C, and Zhu, YC. Hydrogen sulfide induces human colon cancer cell proliferation: role of Akt, Erk and P21. Cell Biol Int. (2010) 34:565–72. doi: 10.1042/CBI20090368
37. Ramasamy, S, Singh, S, Taniere, P, Langman, MJ, and Eggo, MC. Sulfide-detoxifying enzymes in the human colon are decreased in cancer and upregulated in differentiation. Am J Physiol Gastrointest Liver Physiol. (2006) 291:G288–96. doi: 10.1152/ajpgi.00324.2005
38. Blachier, F, Andriamihaja, M, Larraufie, P, Ahn, E, Lan, A, and Kim, E. Production of hydrogen sulfide by the intestinal microbiota and epithelial cells and consequences for the colonic and rectal mucosa. Am J Physiol Gastrointest Liver Physiol. (2021) 320:G125–35. doi: 10.1152/ajpgi.00261.2020
39. Attene-Ramos, MS, Wagner, ED, Gaskins, HR, and Plewa, MJ. Hydrogen sulfide induces direct radical-associated DNA damage. Mol Cancer Res. (2007) 5:455–9. doi: 10.1158/1541-7786.MCR-06-0439
40. Ijssennagger, N, van der Meer, R, and van Mil, SWC. Sulfide as a mucus barrier-breaker in inflammatory bowel disease? Trends Mol Med. (2016) 22:190–9. doi: 10.1016/j.molmed.2016.01.002
41. Schroeder, BO, Birchenough, GMH, Stahlman, M, Arike, L, Johansson, MEV, Hansson, GC, et al. Bifidobacteria or fiber protects against diet-induced microbiota-mediated colonic mucus deterioration. Cell Host Microbe. (2018) 23:27–40e7. doi: 10.1016/j.chom.2017.11.004
42. Giovannucci, E, Colditz, GA, Stampfer, MJ, Hunter, D, Rosner, BA, Willett, WC, et al. A prospective study of cigarette smoking and risk of colorectal adenoma and colorectal cancer in U.S. women. J Natl Cancer Inst. (1994) 86:192–9. doi: 10.1093/jnci/86.3.192
43. Terry, MB, and Neugut, AI. Cigarette smoking and the colorectal adenoma-carcinoma sequence: a hypothesis to explain the paradox. Am J Epidemiol. (1998) 147:903–10. doi: 10.1093/oxfordjournals.aje.a009379
44. Abrams, JA, Terry, MB, and Neugut, AI. Cigarette smoking and the colorectal adenoma-carcinoma sequence. Gastroenterology. (2008) 134:617–9. doi: 10.1053/j.gastro.2007.12.015
45. Biedermann, L, Zeitz, J, Mwinyi, J, Sutter-Minder, E, Rehman, A, Ott, SJ, et al. Smoking cessation induces profound changes in the composition of the intestinal microbiota in humans. PLoS One. (2013) 8:e59260. doi: 10.1371/journal.pone.0059260
46. Lee, SH, Yun, Y, Kim, SJ, Lee, EJ, Chang, Y, Ryu, S, et al. Association between cigarette smoking status and composition of gut microbiota: population-based cross-sectional study. J Clin Med. (2018) 7:282. doi: 10.3390/jcm7090282
47. Prakash, A, Peters, BA, Cobbs, E, Beggs, D, Choi, H, Li, H, et al. Tobacco smoking and the fecal microbiome in a large, multi-ethnic cohort. Cancer Epidemiol Biomarkers Prev. (2021) 30:1328–35. doi: 10.1158/1055-9965.EPI-20-1417
48. Fluhr, L, Mor, U, Kolodziejczyk, AA, Dori-Bachash, M, Leshem, A, Itav, S, et al. Gut microbiota modulates weight gain in mice after discontinued smoke exposure. Nature. (2021) 600:713–9. doi: 10.1038/s41586-021-04194-8
49. Bai, X, Wei, H, Liu, W, Coker, OO, Gou, H, Liu, C, et al. Cigarette smoke promotes colorectal cancer through modulation of gut microbiota and related metabolites. Gut. (2022) 71:2439–50. doi: 10.1136/gutjnl-2021-325021
50. Figueiredo, JC, Crockett, SD, Snover, DC, Morris, CB, McKeown-Eyssen, G, Sandler, RS, et al. Smoking-associated risks of conventional adenomas and serrated polyps in the colorectum. Cancer Causes Control. (2015) 26:377–86. doi: 10.1007/s10552-014-0513-0
51. Liu, X, Wan, X, Zhang, L, Li, Y, Ao, Y, Zhuang, P, et al. The sulfur microbial diet and increased risk of obesity: findings from a population-based prospective cohort study. Clin Nutr. (2023) 42:764–72. doi: 10.1016/j.clnu.2023.03.011
52. Ye, P, Xi, Y, Huang, Z, and Xu, P. Linking obesity with colorectal cancer: epidemiology and mechanistic insights. Cancers (Basel). (2020) 12:1408. doi: 10.3390/cancers12061408
53. Bai, H, Xu, Z, Li, J, Zhang, X, Gao, K, Fei, X, et al. Independent and joint associations of general and abdominal obesity with the risk of conventional adenomas and serrated polyps: a large population-based study in East Asia. Int J Cancer. (2023) 153:54–63. doi: 10.1002/ijc.34503
54. Rogler, G. Chronic ulcerative colitis and colorectal cancer. Cancer Lett. (2014) 345:235–41. doi: 10.1016/j.canlet.2013.07.032
55. Torres, J, Mehandru, S, Colombel, JF, and Peyrin-Biroulet, L. Crohn's disease. Lancet. (2017) 389:1741–55. doi: 10.1016/S0140-6736(16)31711-1
56. Gomez Garcia, EB, and Knoers, NV. Gardner's syndrome (familial adenomatous polyposis): a cilia-related disorder. Lancet Oncol. (2009) 10:727–35. doi: 10.1016/S1470-2045(09)70167-6
Keywords: sulfur microbial diet, colorectal cancer precursors, cancer prevention, epidemiology, dietary pattern
Citation: Xiao Y, He H, Xiang L, Gu H, Xu Z, Luo H, Ren X, Li B, Wei Q, Zhu Z, Zhou H, Tang Y, Zhou Z, Peng L, Wang Y and Jiang Y (2023) Association between sulfur microbial diet and the risk of colorectal cancer precursors in older adults. Front. Nutr. 10:1167372. doi: 10.3389/fnut.2023.1167372
Received: 16 February 2023; Accepted: 31 July 2023;
Published: 14 August 2023.
Edited by:
Raul Zamora-Ros, Institut d'Investigacio Biomedica de Bellvitge (IDIBELL), SpainReviewed by:
Patricia Wolf, University of Illinois Chicago, United StatesCopyright © 2023 Xiao, He, Xiang, Gu, Xu, Luo, Ren, Li, Wei, Zhu, Zhou, Tang, Zhou, Peng, Wang and Jiang. This is an open-access article distributed under the terms of the Creative Commons Attribution License (CC BY). The use, distribution or reproduction in other forums is permitted, provided the original author(s) and the copyright owner(s) are credited and that the original publication in this journal is cited, in accordance with accepted academic practice. No use, distribution or reproduction is permitted which does not comply with these terms.
*Correspondence: Yaxu Wang, MzAwODk3QGhvc3BpdGFsLmNxbXUuZWR1LmNu; Yahui Jiang, MzA0NzkyQGhvc3BpdGFsLmNxbXUuZWR1LmNu
†These authors have contributed equally to this work
Disclaimer: All claims expressed in this article are solely those of the authors and do not necessarily represent those of their affiliated organizations, or those of the publisher, the editors and the reviewers. Any product that may be evaluated in this article or claim that may be made by its manufacturer is not guaranteed or endorsed by the publisher.
Research integrity at Frontiers
Learn more about the work of our research integrity team to safeguard the quality of each article we publish.