- 1Key Laboratory of Plant Resource Conservation and Germplasm Innovation in Mountainous Region (Ministry of Education), Collaborative Innovation Center for Mountain Ecology & Agro-Bioengineering (CICMEAB), Institute of Agro-Bioengineering, College of Life Sciences, Guizhou University, Guiyang, Guizhou, China
- 2Biotechnology and Food Engineering Program, Guangdong Technion-Israel Institute of Technology, Shantou, China
- 3Faculty of Biotechnology and Food Engineering, Technion-Israel Institute of Technology, Haifa, Israel
Introduction: Starch is major component in the big seeds of Cycas revoluta, however the characteristics of Cycas revoluta remain unknown.
Methods: In this study, the physicochemical and structural properties of two starch samples extracted from Cycad revoluta seeds were systematically investigated, using various techniques.
Results: The amylose contents of the two samples were 34.3 % and 35.5%, respectively. The spherical-truncated shaped starch granules possessed A-type crystallinity, and had an average diameter less than 15 μm. Compared to most commonly consumed cereal and potato starch, Cycad revoluta starch showed distinctive characteristics. For physicochemical properties, in the process of gelatinization, the Cycad revoluta starch showed similar viscosity profile to starches of some potato varieties, but Cycad revoluta starch had higher gelatinization temperature. Upon cooling, Cycad revoluta starch formed harder gels than rice starch. For structure, the molecular weight (indexed by Mw, Mn and Rz values), branching degree and the branch chain length distribution were determined.
Discussion: The results suggested that Cycad revoluta starch were different in structure from the main-stream starches. Notable differences in some starch traits between the two samples were recorded, which could be attributed to environmental factors. In general, this study provides useful information on the utilization of Cycad revoluta starch in both food and non-food industries.
1. Introduction
Cycas plants belong to the family Cycadaceae, which consists of 11 genera of plants. Among them, Cycas revoluta, the “Sago palm,” is the most cultivated species and mainly planted for uses in ornamental horticulture (1). Cycas revoluta produces terminal oblong cones containing orange-yellow big seeds, which are potential of nutritional significance for humans. However, study on the nutritional components in seeds of Cycas revoluta is very limited. Starch is usually the major nutritional component in plant seeds, characterization of the seed starch of Cycas revoluta is of importance, as we might be able to find novel starch with unique quality that could be used in food and non-food industry.
Starch is mainly composed of two types of polysaccharides: amylose with few branches and highly branched amylopectin. Starch quality is determined by its characteristics including amylose content, fine structure of amylopectin, and the shape and size of starch granules (2, 3). These characteristics also determine starch utilization, for example, starch with low amylose content is preferred in making fermented cake, while those with intermediate amylose content would be the best material for making porridge (4). Besides, when starch is used in biopolymer film production, the properties of biopolymer film is also influenced by above mentioned starch characteristics (5). Several indicators of starch characteristics are established, and these indicators are known as starch physicochemical and structural properties (4, 6).
A fundamental knowledge of the physicochemical and structural characteristics of a starch is necessary for further developing its utilization. Therefore, in this study, starch samples were extracted from the seeds of two Cycas revoluta grown in different environments. Then, the structural and physicochemical properties of these samples were systematically investigated. The objective of the current study is to reveal the characteristics of starch in seeds of Cycas revoluta, hence to provide useful information for later exploring its utilization in food and non-food industry.
2. Materials and methods
2.1. Materials
Two starch samples were used in this study. One (T1) was extracted from the seeds of Cycad revoluta grown in Shanghai City, China (121.4°E, 30.0°N); and the other (T2) was extracted from the seeds of Cycad revoluta grown in Guiyang City, Guizhou Province, China (106.7°E, 26.4°N).
2.2. Scanning electron microscopy (SEM)
The morphology of the starch granules was observed using an SEM (Scanning Electron Microscope VEGA3, TESCAN, Brno, Czech Republic).
2.3. Apparent amylose content (AAC)
Apparent amylose content was measured according to the method of Li et al. (4).
2.4. Particle size distribution
Particle size distribution of the starch granules was determined using a laser diffraction particle size analyzer (S3500, Microtrac, Montgomeryville, PA, United States).
2.5. Crystal properties
The diffraction pattern of starch samples was determined by an X-ray diffractometer (3 kW/*D8 ADVANCE Da Vinci, Bruker, Karlsruhe, Germany). An X-ray tube Cu-Kα (nickel filter) at 40 kV and 40 mA was used as the X-ray source. The diffraction angle (2θ) was 5°-40° with a step interval of 0.02°.
2.6. Pasting properties
The pasting properties were measured by a rapid visco analyzer (RVA4500, Perten Instruments, Hägersten, Sweden), and the data was recorded and processed by the Thermocline for Windows software. Sample preparation and measurement were based on the procedure described previously (6).
2.7. Textural properties
The starch gel formed after RVA test was further analyzed by a TA-XT2i Texture Analyzer (Stable Micro Systems, Godalming, United Kingdom) to determine the textural properties, following the previously published procedure (6).
2.8. Thermal properties
Thermal properties were measured by differential scanning calorimetry (Discovery DSC 25, TA Instruments, New Castle, DE, United States), based on the previously published procedure (6).
2.9. Fourier transform infrared (FTIR) spectrum analysis
Fourier transform infrared spectra was obtained via scanning starch samples using a Nicolet iZ-10 FTIR instrument (Thermo Fisher Scientific, Waltham, MA, United States). Starch sample (5 mg) was mixed with potassium bromide (250 mg) and pressed into a film-coated tablet. Wavenumbers from 400 to 4,000 cm−1 were measured at 4 cm−1 spectral resolution over 32 scans.
2.10. Branch chain-length distribution
High-performance anion-exchange chromatography (HPAEC) was performed to determine the branch chain-length distribution of amylopectin. Samples was analyzed by a CarboPac PA-100 anion-exchange column (4.0*250 mm; Dionex) using a pulsed amperometric detector (PAD; Dionex ICS 5000 system). Data were collected on the ICS5000 (Thermo Fisher Scientific, Waltham, MA, United States), and processed using chromeleon 7.2 CDS (Thermo Fisher Scientific, Waltham, MA, United States).
2.11. Molecular weight distribution analysis
Gel permeation chromatography-refractive index-multiangle laser light scattering detector (GPC-RI-MALLS) was used to measure the starch molecular weight. The differential refractive index detector (Optilab T-rEX, Wyatt Technology Co., Santa Barbara, CA, United States) was equipped with two tandem columns (300 × 8 mm, Shodex OH-pak SB-805 and 803; Showa Denko K.K., Tokyo, Japan). The data was recorded and processed using ASTRA6.1 software (Wyatt, Santa Barbara, CA, United States).
2.12. Average degree of branching
The Bruker BioSpin GmbH NMR spectrometer equipped with a tempering unit was used to measure the average degree of branching. Starch sample (10 mg) was mixed with 1 mL of deuterated dimethyl sulfoxide-d6 (DMSO-d6) and the solution was thoroughly mixed at 80°C overnight. The mixture was centrifuged at 12,000 rpm. Then, the supernatant was taken and transferred into an NMR tube. The 1H NMR scanning was performed 32 times, at a Larmor frequency of 500.23 MHz. Data were acquired and analyzed using MestReNova. Average DB was calculated with the following equation: DB (%) = (I-1,6) / (I-1,6 + I-1,4)*100, where I-1,4 is the integrated signal at 5.12 ppm and I-1,6 is the integrated signal at 4.77 ppm, respectively.
2.13. Statistical analysis
All traits were measured in duplicate. Data analysis was conducted with SPSS 25.0 statistical software program. Significance of differences between the means were determined by the independent t-test analysis (p < 0.05).
3. Results and discussion
3.1. Morphology of starch granules
The morphology of Cycad revoluta starch granules observed by scanning electron microscope are shown in Figure 1. The Cycad revoluta starch granules showed unique morphology, they were spherical-truncated in shape with smooth surface, which is similar to the shape reported for Lithocarpus dealbatus and cassava (Manihot esculenta) starch (7–9). In contrast, rice and maize starch granules are polygonal, and potato starch granules are oval-shaped (10, 11).
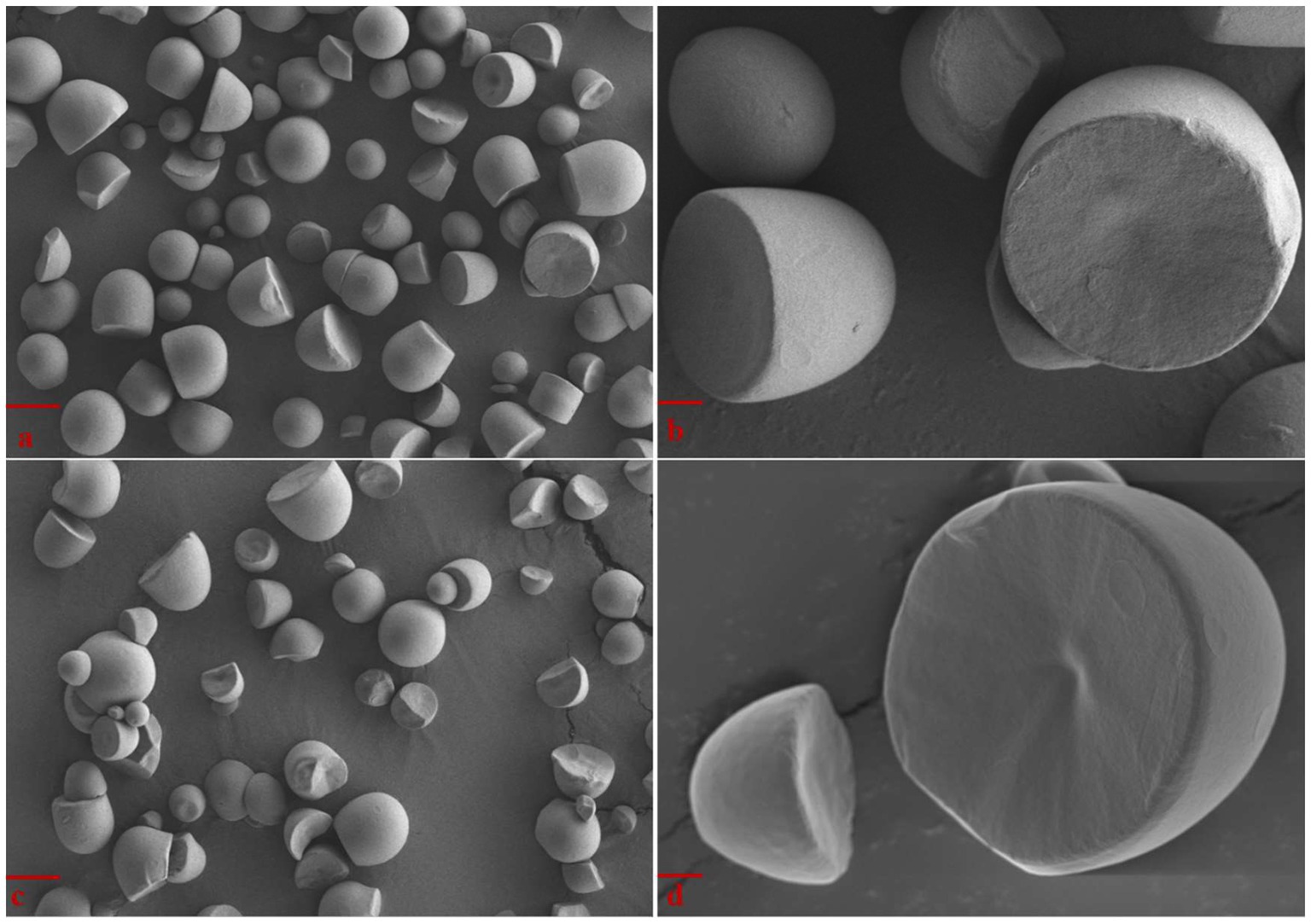
Figure 1. SEM images of Cycad revoluta starch granules (A) Sample T1, Bar = 10 μm; (B) Sample T1, Bar = 2 μm; (C) Sample T2, Bar = 10 μm; (D) Sample T2, Bar = 2 μm.
3.2. Particle size distribution
Particle size distribution of Cycad revoluta starch samples were presented in Table 1. D (4,3) and D (3,2) represent volume and area diameters, respectively. D (0.1), D (0.5), and D (0.9) indicate 10, 50 and 90% of the starch granules were smaller than the values. The two Cycad revoluta starches were significantly different in D (3,2), D (4,3), D (0.9), D (0.5) (p<0.05), with mean values of these parameters of T2 were higher than those of T1. D (0.1) of T1 was higher than that of T2, but the difference was insignificant.
The Cycad revoluta starch granules had an average area diameter smaller than 15 μm, and volume diameter less than 20 μm. The T1 and T2 samples had mean area diameter (D (3,2)) of 12.8 μm and 14.5 μm, respectively. D (0.9) were 26.0 μm and 27.3 μm for T1 and T2, respectively. Generally, judging on the value of mean area diameter, the Cycad revoluta starch granules sized similarly to maize, cassava, and sweet potato starch, and smaller than potato starch, but larger than rice starch (8, 12–16).
3.3. Apparent amylose content
The two Cycad revoluta starches had significantly different apparent amylose content. AAC was 34.3% for T1 sample while 35.5% for T2 sample (Table 1). In rice, AAC could be divided into five groups: waxy (0–2%), very low (5–12%), low (12–20%), intermediate (20–25%) and high (25–33%) (17). As reported previously, AAC ranged from 18.3 to 25.3% in 34 foxtail millet genotypes (4), 2.09 to 35.25% in 192 maize landraces (18), and from 18.9 to 29.4% in 29 potato accessions (19). Therefore, the Cycad revoluta starch had much higher AAC than most commonly consumed starches.
3.4. Pasting properties
All parameters of pasting properties determined by RVA are presented in Table 1. T1 and T2 varied significantly in all parameters except SB (Table 1). The sample (T2) with higher AAC also had higher PV, HPV, CPV. According to previous reports, AAC do have significantly positive correlation to HPV and CPV in some cases [(19–21)], however, AAC usually have negative rather than positive correlation to PV (19, 20, 22). Nevertheless, PV is not only affected by amylose content, but also determined by amylopectin structure. Singh et al. (23) reported that the greater DP of amylopectin chains led to higher values of viscosity. In this respect, the differences on viscosity values between the two Cycad revoluta starch samples could attribute to a complex variation in starch characteristics.
PV, HPV, CPV, BD, SB, and CS were 5,700 and 5,927 cP, 2,413 and 2,807 cP, 4,364 and 4,688 cP, 3,288 and 3,121 cP, −1,336 and − 1,239 cP, 1952 and 1882 cP for the two samples. Since viscosity values can be substantially affected by starch concentration, we compared our result specifically to those using same starch concentration in RVA test. The viscosity values of Cycad revoluta starches were within the range of rice (6), and were higher than that of most of cassava genotypes (24). The viscosity values of Cycad revoluta starches should be lower than that of most potato cultivars, as the latter ones exhibited similar or higher viscosity values, even under a lower starch concentration (19).
3.5. Gel textural properties
Gel textural properties are summarized in Table 1. HD, ADH and COH of the two samples were 77.0 and 106.9 g, −222.3 to −173.1 g.s, 0.469 to 0.427, respectively (Table 1). All the three parameters varied significantly between the two samples, and Cycad revoluta starches can form harder gels than rice starch, under same concentration. All of this could be due to the variance in starch composition. As suggested by previous reports, amylose content is positively correlated to gel hardness and stickiness, and the structure of amylopectin also affects the texture of starch gel (25–27).
3.6. Thermal properties
Gelatinization temperatures and transition enthalpies of Cycad revoluta starches are presented in Table 1 and DSC profiles are shown in Figure 2. A single endothermic conversion was observed in the DSC profile for each sample (Figure 2). Onset (To), peak (Tp), conclusion (Tc) and gelatinization enthalpy (∆Hg) were 73.7 and 69.8°C, 78.8 and 74.6°C, 85.6 and 81.2°C, and 15.1 and 15.3 J/g, respectively. The two samples varied significantly (p<0.05) in all thermal parameters except ΔHg.
The values of thermal properties of Cycad revoluta starches are within the range of that reported for 163 rice accessions, wherein To, Tp, and Tc ranging from 59.2 to 76.6°C, 66.7 to 81°C, and 71.8 to 87.8°C, respectively (6). In seven maize samples, gelatinization temperature (Tp) varied from 72.5 to 75.7°C (28). Ahmed et al. (19) reported a 66.1°C-71.1°C range of Tp variation in 29 potato genotypes. To conclude, the gelatinization temperatures of Cycad revoluta starches are similar to that of commonly consumed cereal starchers, but higher than potato starch.
3.7. Crystallinity
There are three main types of crystallinity in starch based on XRD patterns: A-type starch has peaks at about 15°, 17°, 18°and 23°, and usually exists in cereal starch. B-type having peaks at approximately 5°, 6°, 15°, 17°, 18° and 23° are usually found in tuber starch. C-type is a combination of A-and B-type, and is usually found in bean starches (29, 30).
XRD patterns of Cycad revoluta starches were shown in Figure 3. Peaks of moderate intensity were observed at 10°and 11.5°, followed by a single peak with high intensity at 15°, an unresolved double peak at 17° and 18°, and a broad peak at 23°. This XRD pattern is previously reported in maize and cassava starches and was regarded as A-type crystalline structure (Poto et al., 2018; (31)).
3.8. FT-IR analysis
The FT-IR was conducted to determine the short range order of the Cycad revoluta starches. The crystalline region, amorphous region and hydrated carbohydrate helices of the starch molecule are associated to the absorption peaks at 1047 cm−1, 1,022 cm−1 and 995 cm−1, respectively. Therefore, 1,047/1022 ratio is an intensity indicator of crystalline and amorphous regions while 1022/995 ratio indicates the formation of double helix of starch molecules (32). As presented in Table 1, the two samples had similar absorption peaks, and did not differ significantly in neither of 1047/1022 and 1022/995. However, the 1047/1022 ratio of Cycad revoluta was substantially higher than that of cereal and potato starches (33, 34), suggesting a clear difference in starch structure between Cycad revoluta and traditional common starches.
3.9. Molecular weight distribution
The z-radius of gyration (Rz), number-average molecular weight (Mn), weight-average molecular weight (Mw) and the degree of the dispersion of the molecular weight distribution (Mw/Mn) of Cycad revoluta starches are summarized in Table 1. Rz, Mn, Mw, Mw/Mn in each sample were 177 nm, 13,682 KDa, 62,213 KDa, 4.56 for T1, and 170 nm, 11,852 KDa, 52,240 KDa, 4.48 for T2. The two samples only differed significantly in Mw/Mn (p<0.05).
The high Mw is an indicator for highly polymerized amylopectin, and the high ratio of Mw/Mn indicates that the molecular weight distribution of starch is highly dispersed (35). Higher Rz value indicates higher branching degree, as Rz means the theoretical probability of finding a molecule at a given distance from the center (36). Compared to conventional main-stream starches, Cycad revoluta starches showed similar Mw, but smaller Mn and higher Rz values than rice starches (37), and much higher Mw and Mn than both maize and cassava starch (38, 39). The branching degree of T1 was significantly higher than that of T2 (p<0.05), this is in consistent with the variation pattern in Mw, Mn, Mw/Mn, and Rz between the two samples (Table 1).
3.10. Relative molecular weight distribution
Based on the relative molecular weight distribution analysis, conducted by gel performance chromatography (GPC), branching patterns of the isoamylase-debranched Cycad revoluta starches were determined. The results are shown in Figure 4 and Table 1. The three peaks in Figure 4 represented amylopectin with short-branch chains (AP1), amylopectin with long-branch chains (AP2) and amylose molecules (AM), respectively (40).
The ratios of the three peaks were 46.5 and 53.8%, 27.8 and 26.7%, 25.7 and 19.5%, respectively, in the two Cycad revoluta starch samples. T2 had more proportions of short-branch chains (53.8% VS 46.5%) but less long-branch chains (27.8% VS 26.7%) and amylose chains (19.5% VS 25.7%) than T1 (p<0.05). Peng et al. (41) concluded in rice study that higher proportion of amylopectin short chains associated with higher peak viscosity (PV) and breakdown value (BD), and a softer and stickier texture (HD). This is consistent with the data of the current study. As shown in Table 1, the Cycad revoluta starch sample with higher proportion of amylopectin short chains (T2) did have higher PV, BD and lower HD values than that of sample with lower proportion of amylopectin short chains (T1).
3.11. Chain length distribution of the debranched amylopectin
High-performance anion-exchange chromatography-pulsed amperometric detection (HPAEC-PAD) was used to determine the chain length distribution of amylopectin in this study. The results are shown in Figure 5 and Table 1. According to the degree of polymerization (DP) and the model of amylopectin cluster, branched chains can be grouped into four classes: A (DP 6–12), B1 (DP 13–24), B2 (DP 25–36), and B3 (DP ≥ 37) (42, 43).
As presented in Table 1, the proportions of A, B1, B2, and B3 chains of the two samples were 21.3, 47.9, 13.6, 17.3 and 22.3%, 48.0, 13.6, 16.2%, respectively. The two Cycad revoluta starches only varied significantly in proportion of A chain.
Compared to cereal starches, the patterns of amylopectin chain distribution of Cycad revoluta starches were very different from that of wheat and corn, but similar to some rice varieties (44–46). Generally, the Cycad revoluta amylopectin showed much higher proportion of long chains (B2 + B3 chains), but much lower proportion of short chains (A chain) than that of wheat starch (46), and contained much higher proportion of A and B1 chains, but much lower proportion of B2 and B3 chains than that of corn starch (44). Compared to potato starch, Cycad revoluta starch had more B1 chains, but less B2 and B3 chains, and similar proportion of A chain (47).
4. Conclusion
The structural and physicochemical properties of two Cycad revoluta starch samples were systematically investigated in this study. The Cycad revoluta starch granules were spherical-truncated in shape with smooth surface, and had a small size (mean area diameter < 15 μm). XRD analysis revealed that the Cycad revoluta starches had A-type crystallinity. During gelatinization, the Cycad revoluta starches showed similar viscosity profile with that of some rice and potato varieties, but higher gelatinization temperature than potato starch. The branch chain length distribution analysis revealed that the Cycad revoluta starches were structurally similar to some rice varieties, but totally different from that of corn, wheat and potato starches. In general, the Cycad revoluta starches had distinctive characteristics and its application remained explored in later studies, and the current study provides fundamental information for application of Cycad revoluta starches in food and non-food industries.
Data availability statement
The raw data supporting the conclusions of this article will be made available by the authors, without undue reservation.
Author contributions
KL: conceptualization, resources, supervision, data curation, writing-original draft, and writing-review & editing. TZ: data curation, methodology, and investigation. HR, WZ, and SH: software. YG: methodology. HC: writing-review & editing and funding acquisition. All authors contributed to the article and approved the submitted version.
Funding
The authors would like to thank Shantou Science and Technology Bureau (grant no. STKJ2021024), Guizhou University Natural Science Project (2020-23), Guizhou University Seed Program (2020-26), and The Opening Foundation of National Laboratory of Hazard Factors and Risk Prevention of Agricultural Product Quality and Safety (2021DG700024-KF202209) for financial support.
Conflict of interest
The authors declare that the research was conducted in the absence of any commercial or financial relationships that could be construed as a potential conflict of interest.
Publisher’s note
All claims expressed in this article are solely those of the authors and do not necessarily represent those of their affiliated organizations, or those of the publisher, the editors and the reviewers. Any product that may be evaluated in this article, or claim that may be made by its manufacturer, is not guaranteed or endorsed by the publisher.
References
1. Ballhorn, DJ. Cyanogenic glycosides in nuts and seeds. Nuts Seeds Health Dis Prevention. (2011):129–36. doi: 10.1016/B978-0-12-375688-6.10014-3
2. Lin, JH, Singh, H, Chang, YT, and Chang, YO. Factor analysis of the functional properties of the flours from mutant genotypes. Food Chem. (2011) 126:1108–14. doi: 10.1016/j.foodchem.2010.11.140
3. Yu, S, Ma, Y, Menager, L, and Sun, D. Physicochemical properties of starch and flour from different rice cultivars. Food Bioproc Tech. (2012) 5:626–37. doi: 10.1007/s11947-010-0330-8
4. Li, K, Zhang, T, Sui, Z, Narayanamoorthy, S, Jin, C, Li, S, et al. Genetic variation in starch physicochemical properties of Chinese foxtail millet (Setaria italica Beauv.). Int J Biol Macromol. (2019) 133:337–45. doi: 10.1016/j.ijbiomac.2019.04.022
5. Agarwal, S. Major factors affecting the characteristics of starch based biopolymer films. Eur Polym J. (2021) 160:110788. doi: 10.1016/j.eurpolymj.2021.110788
6. Li, KH, Bao, JS, Corke, H, and Sun, M. Genotypic diversity and environmental stability of starch physicochemical properties in the USDA rice mini-core collection. Food Chem. (2017) 221:1186–96. doi: 10.1016/j.foodchem.2016.11.040
7. Charoenkul, N, Uttapap, D, Pathipanawat, W, and Takeda, Y. Physicochemical characteristics of starches and flours from cassava varieties having different cooked root textures. LWT-Food Sci Technol. (2011) 44:1774–81. doi: 10.1016/j.lwt.2011.03.009
8. Mishra, S, and Rai, T. Morphology and functional properties of corn, potato and tapioca starches. Food Hydrocolloid. (2006) 20:557–66. doi: 10.1016/j.foodhyd.2005.01.001
9. Yu, T, Yang, Q, Deng, M, Cheng, N, Yao, K, Yang, W, et al. Morphological characteristics of seed starch granules of Fagaceae in South China and their implication in paleodiet. Front Plant Sci. (2022) 13:977152. doi: 10.3389/fpls.2022.977152
10. Ashogbon, AO, and Akintayo, TE. Isolation, composition, morphological and pasting properties of starches from rice cultivars grown in Nigeria. Starch/Stärke. (2012) 64:181–7. doi: 10.1002/star.201100044
11. Yan, H, Yayuan, Z, Ling, Z, and Zhengbiao, G. Study on physicochemical characteristics of waxy potato starch in comparison with other waxy starches. Starch/Stärke. (2011) 63:754–9. doi: 10.1002/star.201100013
12. Ali, A, Wani, TA, Wani, IA, and Masoodi, FA. Comparative study of the physico-chemical properties of rice and corn starches grown in Indian temperate climate. J Saudi Soc Agric Sci. (2016) 15:75–82. doi: 10.1016/j.jssas.2014.04.002
13. Chel-Guerrero, L, Cruz-Cervera, G, Betancur-Ancona, D, and Solorza-Feria, J. Chemical composition, thermal and viscoelastic characterization of tuber starches growing in the Yucatan peninsula of Mexico. J Food Process Eng. (2011) 34:363–82. doi: 10.1111/j.1745-4530.2009.00362.x
14. Dhital, S, Butardo, VM, Jobling, SA, and Gidley, MJ. Rice starch granule amylolysis –differentiating effects of particle size, morphology, thermal properties and crystalline polymorph. Carbohyd Polym. (2015) 115:305–16. doi: 10.1016/j.carbpol.2014.08.091
15. Wu, K, Dai, S, Gan, R, Corke, H, and Zhu, F. Thermal and rheological properties of mung bean starch blends with potato, sweet potato, rice, and sorghum starches. Food Bioprocess Tech. (2016) 9:1408–21. doi: 10.1007/s11947-016-1730-1
16. Zhang, J, Murphy, A, Liu, G, Bizimungu, B, Liu, Q, Leclerc, Y, et al. Starch granule size variation and relationship with tuber dry matter content in heritage potato varieties. Sci Hortic-Amsterdam. (2011) 130:503–9. doi: 10.1016/j.scienta.2011.08.006
17. Juliano, BO, Perez, CM, Blakeney, AB, Castillo, DT, Kongseree, N, Laignelet, B, et al. International cooperative testing on the amylose content of milled rice. Starch-Stärke. (1981) 33:157–62. doi: 10.1002/star.19810330504
18. Kahrman, F, Akbulut, U, Düz, G, Songur, U, and Egesel, C. Screening of genetic variability in Turkish maize landraces for protein and starch related traits. Maydica. (2020) 65:M3.
19. Ahmed, S, Zhou, X, Pang, Y, Xu, Y, Tong, C, and Bao, J. Genetic diversity of potato genotypes estimated by starch physicochemical properties and microsatellite markers. Food Chem. (2018) 257:368–75. doi: 10.1016/j.foodchem.2018.03.029
20. Li, K, Zhang, T, Narayanamoorthy, S, Jin, C, and Corke, H. Diversity analysis of starch physicochemical properties in 95 proso millet (Panicum miliaceum L.) accessions. Food Chem. (2020) 324:126863. doi: 10.1016/j.foodchem.2020.126863
21. Narayanamoorthy, S, Zhang, CC, Xu, ZK, Ma, MT, Sui, ZQ, Li, KH, et al. Genetic diversity and inter-relationships of common bean (Phaseolus vulgaris L.) starch traits. Starch-Stärke. (2021) 74:2100189. doi: 10.1002/star.202100189
22. Zaidul, ISM, Yamauchi, H, Takigawa, S, Matsuura-Endo, C, Suzuki, T, and Noda, T. Correlation between the compositional and pasting properties of various potato starches. Food Chem. (2007) 105:164–72. doi: 10.1016/j.foodchem.2007.03.061
23. Singh, N, Isono, N, Srichuwong, S, Noda, T, and Nishinari, K. Structural, thermal and viscoselastic properties of potato starches. Food Hydrocolloid. (2008) 22:979–88. doi: 10.1016/j.foodhyd.2007.05.010
24. Niba, LL, Bokanga, MM, Jackson, FL, Schlimme, DS, and Li, BW. Physicochemical properties and starch granular characteristics of flour from various Manihot Esculenta (cassava) genotypes. J Food Sci. (2002) 67:1701–5. doi: 10.1111/j.1365-2621.2002.tb08709.x
25. Bertoft, E, Annor, GA, Shen, X, Rumpagaporn, P, Seetharaman, K, and Hamaker, BR. Small differences in amylopectin fine structure may explain large functional differences of starch. Carbohyd Polym. (2016) 140:113–21. doi: 10.1016/j.carbpol.2015.12.025
26. Cameron, DK, and Wang, YJ. A better understanding of factors that affect the hardness and stickiness of long-grain rice. Cereal Chem. (2005) 82:113–9. doi: 10.1094/CC-82-0113
27. Zhu, F, and Xie, Q. Rheological and thermal properties in relation to molecular structure of New Zealand sweetpotato starch. Food Hydrocolloid. (2018) 83:165–72. doi: 10.1016/j.foodhyd.2018.05.004
28. Alejandro, AS, Guadalupe, MM, Javier, SF, and Luis, ABP. Thermal and viscoelastic properties of starch gels from maize varieties. J Sci Food Agr. (2006) 86:1078–86. doi: 10.1002/jsfa.2461
29. Cheetham, NWH, and Tao, L. Variation in crystalline type with amylose content in maize starch granules: an X-ray powder diffraction study. Carbohyd. Polym. (1998) 36:277–84. doi: 10.1016/S0144-8617(98)00007-1
30. Colonna, P, Buleon, A, and Mercier, C. Pisum sativum and Vicia faba carbohydrates: part II. Structural studies of starches. J Food Sci. (1981) 46:88–93. doi: 10.1111/j.1365-2621.1981.tb14537.x
31. Hornung, PS, de Oliveira, CS, Lazzarotto, M, da Silveira Lazzarotto, SR, and Schnitzler, E. Investigation of the photo-oxidation of cassava starch granules. J Therm Anal Calorim. (2016) 123:2129–37. doi: 10.1007/s10973-015-4706-x
32. Van Soest, JJG, De Wit, D, Tournois, H, and Vliegenthart, JFG. Retrogradation of potato starch as studied by fourier transform infrared spectroscopy. Starch-Stärke. (1994) 46:453–7. doi: 10.1002/star.19940461202
33. Chung, HJ, Liu, Q, Huang, R, Yin, Y, and Li, A. Physicochemical properties and in vitro starch digestibility of cooked rice from commercially available cultivars in Canada. Cereal Chem. (2010) 87:297–304. doi: 10.1094/CCHEM-87-4-0297
34. Wang, M, Sun, M, Zhang, Y, Chen, Y, Wu, Y, and Ouyang, J. Effect of microwave irradiation-retrogradation treatment on the digestive and physicochemical properties of starches with different crystallinity. Food Chem. (2019) 298:125015. doi: 10.1016/j.foodchem.2019.125015
35. Ma, M, Wang, Y, Wang, M, Jane, J, and Du, S. Physicochemical properties and in vitro digestibility of legume starches. Food Hydrocolloid. (2017) 63:249–55. doi: 10.1016/j.foodhyd.2016.09.004
36. Yang, Q, Qi, L, Luo, Z, Kong, X, Xiao, Z, Wang, P, et al. Effect of microwave irradiation on internal molecular structure and physical properties of waxy maize starch. Food Hydrocolloid. (2017) 69:473–82. doi: 10.1016/j.foodhyd.2017.03.011
37. Zhang, X, Wang, C, Liu, Z, Xue, Y, Zhao, Q, and Shen, Q. Four stages of multi-scale structural changes in rice starch during the entire high hydrostatic pressure treatment. Food Hydrocolloid. (2023) 134:108012. doi: 10.1016/j.foodhyd.2022.108012
38. Wang, B, Dong, YQ, Fang, YX, Gao, W, Kang, XM, Liu, PF, et al. Effects of different moisture contents on the structure and properties of corn starch during extrusion. Food Chem. (2022) 368:130804. doi: 10.1016/j.foodchem.2021.130804
39. Zeng, L, Mao, W, Hao, J, Ye, G, Song, X, Zeng, L, et al. Structure and functionality of cassava starch in different deep eutectic solvents/water mixtures: a comparative study. Ind Crop Prod. (2022) 177:114453. doi: 10.1016/j.indcrop.2021.114453
40. Song, Y, and Jane, J. Characterization of barley starches of waxy, normal, and high amylose varieties. Carbohyd Polym. (2000) 41:365–77. doi: 10.1016/S0144-8617(99)00098-3
41. Peng, Y, Mao, BG, Zhang, CQ, Shao, Y, Wu, TH, Hu, LM, et al. Influence of physicochemical properties and starch fine structure on the eating quality of hybrid rice with similar apparent amylose content. Food Chem. (2021) 353:129461. doi: 10.1016/j.foodchem.2021.129461
42. Hanashiro, I, Abe, J, and Hizukuri, S. A periodic distribution of the chain length of amylopectin as revealed by high-performance anion-exchange chromatography. Carbohydr Res. (1996) 283:151–9. doi: 10.1016/0008-6215(95)00408-4
43. Li, C, and Gong, B. Insights into chain-length distributions of amylopectin and amylose molecules on the gelatinizaition property of rice starches. Int J Biol Macromol. (2020) 155:721–9. doi: 10.1016/j.ijbiomac.2020.04.006
44. Hasjim, J, Srichuwong, S, Scott, MP, and Jane, J. Kernel composition, starch structure, and enzyme digestibility of opaque-2 maize and quality protein maize. J Agric Food Chem. (2009) 57:2049–55. doi: 10.1021/jf803406y
45. Kong, XL, Chen, YL, Zhu, P, Sui, ZQ, Corke, H, and Bao, JS. Relationships among genetic, structural, and functional properties of rice starch. J Agric Food Chem. (2015) 63:6241–8. doi: 10.1021/acs.jafc.5b02143
46. Singh, N, Singh, S, Isono, N, Noda, T, and Singh, AM. Diversity in amylopectin structure, thermal and pasting properties of starches from wheat varieties/lines. Int J Biol Macromol. (2009) 45:298–304. doi: 10.1016/j.ijbiomac.2009.06.005
47. Yang, LP, Xia, YS, Junejo, SA, and Zhou, YB. Composition, structure and physicochemical properties of three coloured potato starches. Int J Food Sci Tech. (2018) 53:2325–34. doi: 10.1111/ijfs.13824
Keywords: Cycad revoluta, starch, physicochemical properties, amylopectin structure, food bioresources
Citation: Li K, Zhang T, Zhao W, Ren H, Hong S, Ge Y and Corke H (2023) Characterization of starch extracted from seeds of Cycas revoluta. Front. Nutr. 10:1159554. doi: 10.3389/fnut.2023.1159554
Edited by:
Yang Yuan, Guangzhou University, ChinaReviewed by:
Zhongquan Sui, Shanghai Jiao Tong University, ChinaKao Wu, Hubei University of Technology, China
Copyright © 2023 Li, Zhang, Zhao, Ren, Hong, Ge and Corke. This is an open-access article distributed under the terms of the Creative Commons Attribution License (CC BY). The use, distribution or reproduction in other forums is permitted, provided the original author(s) and the copyright owner(s) are credited and that the original publication in this journal is cited, in accordance with accepted academic practice. No use, distribution or reproduction is permitted which does not comply with these terms.
*Correspondence: Kehu Li, a2hsaUBnenUuZWR1LmNu
†These authors share first authorship