- 1School of Life Sciences, Guangzhou University, Guangzhou, China
- 2School of Pharmacy, Guangxi University of Chinese Medicine, Nanning, Guangxi, China
Cyclocarya paliurus (C. paliurus), a nutritional and nutraceutical resource for human and animal diets, has been constantly explored. The available biological components of C. paliurus were triterpenoids, polysaccharides, and flavonoids. Recent studies in phytochemical-phytochemistry; pharmacological-pharmacology has shown that C. paliurus performed medicinal value, such as antihypertensive, antioxidant, anticancer, antimicrobial, anti-inflammatory and immunological activities. Furthermore, C. paliurus and its extracts added to drinks would help to prevent and mitigate chronic diseases. This review provides an overview of the nutritional composition and functional applications of C. paliurus, summarizing the research progress on the extraction methods, structural characteristics, and biological activities. Therefore, it may be a promising candidate for developing functional ingredients in traditional Chinese medicine. However, a more profound understanding of its active compounds and active mechanisms through which they perform biological activities is required. As a result, the plant needs further investigation in vitro and in vivo.
1. Introduction
With the improvement of modern life quality and the rapid development of science and technology, more and more diseases of modern civilization come along with it. These diseases include cardiovascular and cerebrovascular diseases, hypertension, hyperglycemia, hyperlipidemia, etc. (1–3). Plants provide the wealthiest resource of functional natural compounds, which directly or indirectly aroused a wide range of applications for the well-being of the human population and domestic animals (4). With global attention to health, there is more and more research on preventing and treating civilized diseases. Many herbal plants with health benefits have been developed, and C. paliurus is one of them.
Cyclocarya paliurus (Batalin) Iljinskaja, as shown in Figures 1A–C, is a deciduous tree belonging to the genus Cyclocarya Iljinskaja (Juglangdaceae) (5), which is called “sweet tea” in China. As a nutritional and nutraceutical resource for human and animal diets, C. paliurus is widely distributed in south China, such as Hunan, Hubei, Sichuan, Guangdong, and other southern cities (6). The medicinal virtues of C. paliurus have been well-known for their uses in several diseases and disorders since ancient times. As a traditional Chinese medicine, C. paliurus contains rich biological active substances, such as flavonoids, polysaccharides, triterpenic acids, and other essential human trace elements (7–9). In addition, C. paliurus leaves have been widely used as a therapeutic drug in traditional Chinese medicine to treat hyperlipidemia, coronary heart disease and hyperglycemia or to improve immunity (10–13). In 2013, it was approved as a new food ingredient by the National Health and Family Planning Commission of China (14). Recently, people have used C. paliurus leaves for tea because of their diverse biological and physiological activities (15). Cyclocarya paliurus tea became the first health tea approved by the Food and Drug Administration (FDA) in China in 1999 Due to its potential to develop healthy food which can treat diseases, improve work efficiency, and help people recover from mental fatigue (16). According to the Web of Science database statistics, the research articles about C. paliurus have been recorded since 1992 with 2,778 cited frequencies, as shown in Figure 1E.
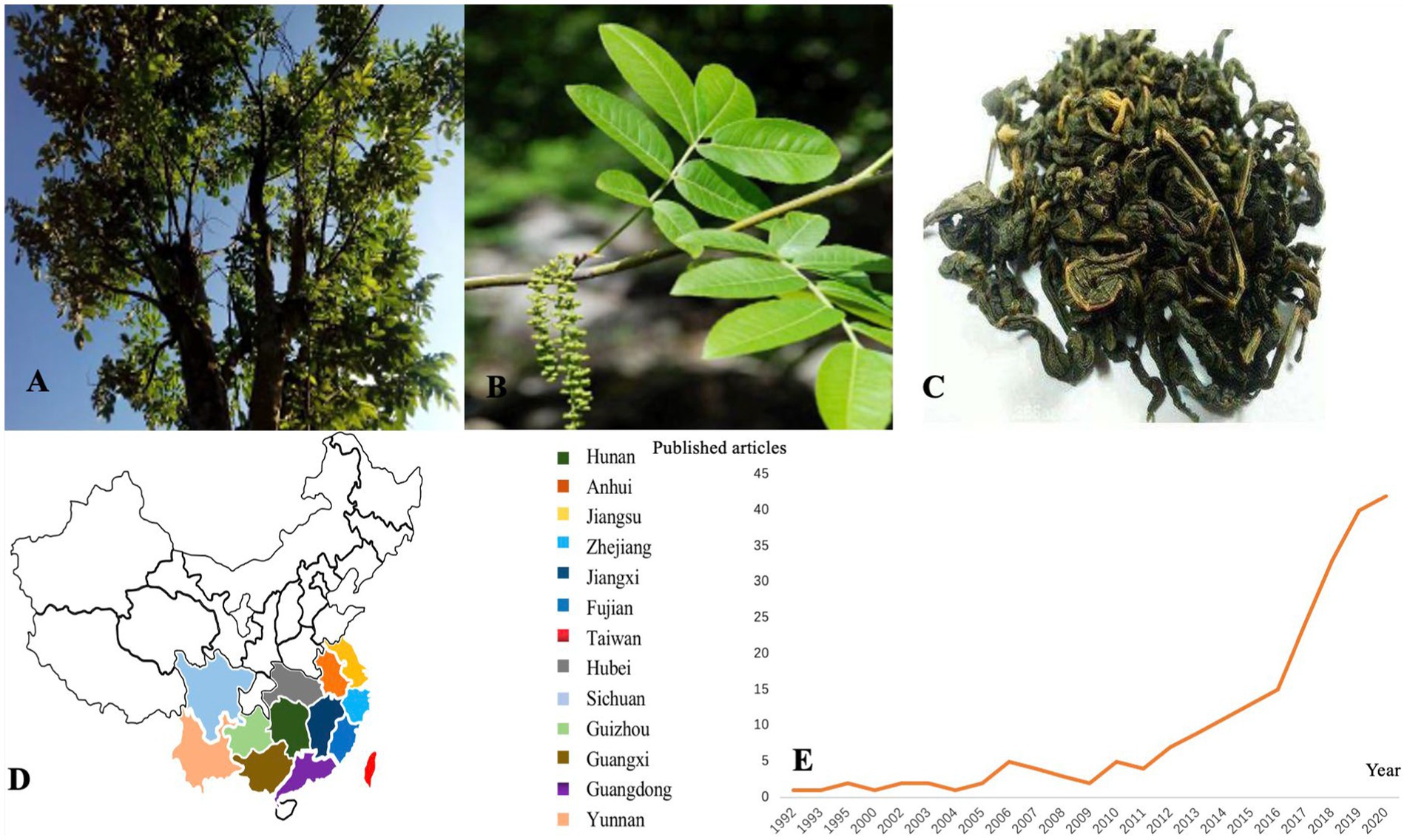
Figure 1. (A) Cyclocarya paliurus tree; (B) C. paliurus leaves; (C) C. paliurus tea; (D) C. paliurus distribution in China; (E) Publication of C. paliurus articles from 1992 to 2020.
Cyclocarya paliurus has a long history in China, and its active components and physiological functions are gradually being developed. However, there needs to be a systematic description of the chemical composition, bioactivity, and applications of C. paliurus. In order to elucidate the importance of C. paliurus to human health, we discussed its phytochemical composition and health effects through the previous research results. This review also aims to provide researchers with background and the latest research progress. This review analyzes the problems and development prospects of C. paliurus research and provides a scientific basis for utilization and further development.
2. Phytochemicals types
2.1. Polysaccharides
Cyclocarya paliurus polysaccharides (CP) is a bioactive component mixture with various physiological functional ingredients, which has aroused great interest and widespread concern (17, 18). As a result, more research has been done on developing CP and corresponding functional foods in recent years. Extraction of plant polysaccharides requires pretreatment of raw materials, such as washing, drying, grinding, sieving, degreasing with petroleum ether, and then refluxing with 80% ethanol to remove various impurities, such as monosaccharides, disaccharides, glycosides, polyphenols, etc. Numerous studies have shown that the polysaccharides of C. paliurus are composed of arabinose, glucose, xylose, mannose, rhamnose, and galactose (19–21). Currently, the mainstream methods for extracting CP are the traditional hot water extraction method, ultrasonic-assisted extraction method (UAE), and microwave-assisted extraction method. Yang et al. extracted polysaccharides from C. paliurus using distilled water as the extraction solvent. The optimum conditions include extraction temperature of 100°C, extraction time of 4 h, a material/water ratio of 1:20 and two rounds of extraction, which provide a final crude polysaccharides yield of 4.37% (22). In another study, ultrasonic-assisted extraction, microwave-assisted extraction, and heat reflux extraction (HRE) were used to compare the extraction rates of CP. The results showed that the ideal extraction technique is microwave-assisted extraction, which had the highest yield of polysaccharides (5.07 ± 0.02%), and the HRE method and UAE method were 3.89 ± 0.04% and 4.82 ± 0.03%, respectively (23). The extracted crude polysaccharides were then precipitated, deproteinated and dialyzed, followed by column purification with DEAE-Sephadex A-25 column, AB-8 macroporous anion-exchange column, DEAE-cellulose chromatography, and D301R chromatography column (22, 24–26). The structural characteristics of the polysaccharides of C. paliurus are shown in Table 1.
Several factors would determine the biological activity of polysaccharides, such as the structure, molecular weight, functional groups, the sugar unit of the backbone, the type and polymerization degree of the branch, the glycosidic bond, and the conformation of polysaccharides (29, 30). Some modifications, such as sulfation, carboxymethylation, and acetylation, would change its biological activity. The modification of C. paliurus polysaccharides was then studied in different ways. Xie et al. extracted polysaccharides from C. paliurus and chemically modified them to obtain sulphated polysaccharides(S-CP). They found that DS (degree of substitution), Mw (molecular weight), sulfate content, protein, and uric acid content would be the main factors affecting the sulfated polysaccharides’ antioxidant activity. It was found that the physicochemical properties, monosaccharide composition, molar ratio, molecular weight, and immunomodulatory activity of the polysaccharides were changed after the sulfate modification (31). According to Yu’s research (Figure 2), SCP5(with a DS of 0.13) had better immunomodulatory activity than SCP3(with a DS of 0.45). So, we can conclude that a proper degree of sulfation may enhance the immunomodulatory activity of CCPs in a dose-dependent way. Among these sulfates, polysaccharides with different DS, S-CP1–4 (0.42 ± 0.04) and S-CP1–8 (0.06 ± 0.01) with relatively low DS had a better antioxidant effect, and their antioxidant activity was significantly enhanced with increasing concentration (19). Besides, based on the H2O2-induced RAW264.7 cells models, S-CP1–4 (400 μg/mL) treatment increased superoxide dismutase (SOD) activity was 1.29 ± 0.20 units, with CP (1.12 ± 0.18 units) and S-CP1–8 (1.08 ± 0.11 units). In addition, compared to S-CP1–4 and CP, S-CP1–8 performed a significantly higher antioxidant capacity (p < 0.05) and inhibited lipid oxidation (11). Another study through further in-depth chemical characterization revealed that S-CP1–8 consisted of Ara, Rha, Gal, Glc, Xyl, Man, GalA, and GlcA with a molar ratio of 1.0:0.1:1.8:1.1:0.2:0.6:0.4:0.1 and its molecular weight (Mw) was 970 kDa. Compared with CP, S-CP1–8 had immune-enhancing effects by significantly increasing the secretion levels of cytokines (TNF-α (Tumor Necrosis Factor-α), IL-1β (Interleukin-1β) and IL-6 (Interleukin-6)) in a dose-dependent manner, and enhancing Nitric Oxide (NO) release and phagocytic activity of macrophages (32). In addition, they successfully prepared the acetylated C. paliurus polysaccharides (Ac-CP). They found that its monosaccharide composition was Ara, Gal, Glc, Rha, Man and GalA with a molar ratio of 1.00: 1.67: 1.07: 0.15: 0.34: 1.58: 0.1, and its molecular weight was 1.05 × 106 Da. Compared with the total polysaccharides, Ac-CP stimulated the production of pro-inflammatory cytokines by RAW264.7 cells. Acetylation modification could further enhance its activity to improve the immune activity of CP (33). Furthermore, they found acetyl groups were substituted at the O-2 and O-6 positions of 3)-β-D-Galp-(1 residue of the acetylated C. paliurus polysaccharides (Ac-CPP0.1). Ac-CPP0.1 treatment significantly increased the levels of SOD, Glutathione peroxidase (GSH-Px), and catalase in H2O2-treated dendritic cells. The molecular-level study revealed that the mechanism might be related to the activation of the Nrf2-Keap1 signaling pathway (34). Similarly, carboxymethylated C. paliurus polysaccharides (CM-CPs) affected the content of carbohydrates, proteins, and glyoxylates and changed the Mw and the molar ratio of monosaccharides. The result showed that the CM-CPs had higher ratios of Gal, Rha, and Glc and relatively high DS and Mw compared with native polysaccharides (35).
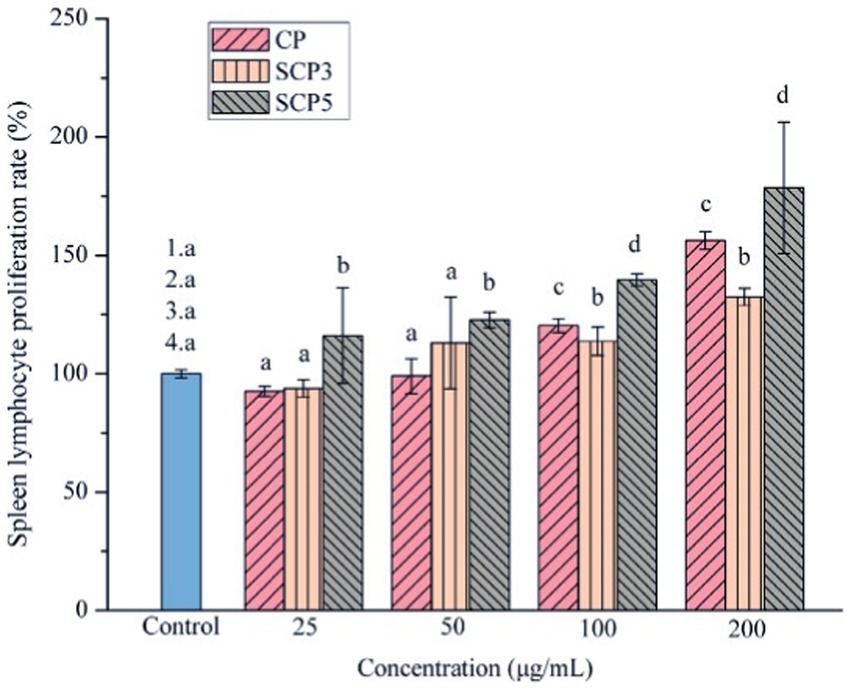
Figure 2. The effect of sulfate modification on CPP’s immunomodulatory enhancement (31).
2.2. Triterpenoid saponins
Triterpenoid saponins are composed of oleanolic acid, ursolic acid, maslinic acid, corosolic acid, 23-hydroxyursolic acid, arjunolic acid, echinocystic acid, and other organic acids (36). Triterpenoid saponins are widely distributed in plants, such as Glycyrrhiza uralensis Fisch, Ilex pubescens Hook, Panax ginseng, and C. paliurus, among which C. paliurus has specific triterpenoid saponins. However, it has a few kinds. Different sources of triterpenoid saponins exhibit different bio functions, such as anticancer activity, anti-AIDS activity, hypoglycemic activity, and anti-cardiovascular activity. However, their mechanisms depend on the antioxidant activity resulting from the isoprene structure. A previous study found that ethanol extract of C. paliurus leaves was abundant in triterpenic acids (37). With different eluents, 13 saponins were obtained in C. paliurus leaves. Shu et al. isolated two secodammarane triterpenoid saponins from C. paliurus leave (38). Another study isolated new triterpenoid saponins from the ethanol extract of C. paliurus, and the structure was identified by spectroscopy (39). Similarly, Shang et al. successfully quantified four triterpenoids in the leaves of C. paliurus by HPLC (40).
2.3. Polyphenols/flavonoids
The polyphenols in C. paliurus are extracted with a 1% hydrochloric acid solution. There are mainly two polyphenols (catechin and procyanidin B1) in C. paliurus. From 21 natural populations, the total polyphenol content of C. paliurus was 20.80–52.69 mg/g using the Folin–Ciocalteu, suggesting high phenolic content and antioxidant capacity may come from the low-temperature pressure (41, 42). Flavonoids are a type of polyphenol which exist in most plants. In recent years, the research on flavonoids of C. paliurus has increased gradually, which can provide a theoretical reference for developing potential value. Many flavonoids, such as isoquercetin, quercetin, and kaempferol, were identified from C. paliurus (7, 43) (Table 2). The extraction of flavonoids from C. paliurus included ethanol extraction (44), ultrasonic extraction (47), Enzymolysis-ultrasonic assisted extraction (43) (Table 3), and purification using AB-8 resin (7), HPD-600 macroporous resin (43), anion exchange, Sephadex G-100, and Sepharose CL-6B column. Liquid Chromatograph Mass Spectrometer (LC–MS) (17), GC, and high-performance liquid chromatography (HPLC) (43), ultra-performance liquid chromatography-quadrupole-time of flight mass spectrometry (UPLC-Q-TOF-MS) (44) are commonly used to identify flavonoids.
Hu et al. used the colorimetric method to determine the total flavonoid content of CPF (C. paliurus flavonoids), which was calculated to be 752.60 ± 10.64 mg/g. Afterwards, using the UPLC-Q-TOF-MS analysis found CPF contained five main flavonoids, including quercetin-3-O-glycoside (47.38 ± 2.32 mg/g), quercetin-3-O-rhamnoside (38.83 ± 2.02 mg/g), kaempferol-3-O-rhamnoside (315.42 ± 12.23 mg/g), quercetin (97.23 ± 3.89 mg/g) and kaempferol(52.78 ± 3.21 mg/g) (44). Similar results were obtained by Lei et al. (43). Different purification methods and concentrations of ethanol solutions result in different flavonoid compositions and contents in C. paliurus. A study found that seven flavonoids of C. paliurus leaves were composed of 3-O-caffeoylquinic acid (0.04–1.38 mg/g), quercetin-3-O-glucuronide (0.30–2.38 mg/g), kaempferol-3-O-glucuronide (0.17–1.88 mg/g), kaempferol-3-O-rhamnoside (0.05–2.30 mg/g), arjunolic acid (0.02–2.70 mg/g), cyclocaric acid B (0.04–0.98 mg/g), pterocaryoside B (0.01–2.46 mg/g), and pterocaryoside A (1.07–2.48 mg/g) (45). Another study found that Kaempferol-3-O-glucuronide was the main flavonoid in C. paliurus leaves, and the content of kaempferol-3-O-rhamnoside was 256.82 ± 9.53 mg/g, Kaempferol-3-O-𝛽-glucuronide was 472.47 ± 11.79, Isoquercitrin was 151.47 ± 3.74 mg/g, and Quercetin was 86.33 ± 1.55 mg/g (7). It is reported quercetin-3-O-b-D-glucuronide, quercetin, kaempferol-3-O-b-D-glucuronide, kaempferol-7-O-a-L-rhamnoside and kaempferol were main constituents in the C. paliurus leaves using LC–MS (17). It is also reported that Kaempferol-3-O-glucuronide was the main flavonoid in C. paliurus leaves whose content was 472.47 ± 11.79 mg/g. Meanwhile, the content of kaempferol-3-O-rhamnoside was 256.82 ± 9.53 mg/g, the content of Isoquercitrin was 151.47 ± 3.74 mg/g, and the content of Quercetin was 86.33 ± 1.55 mg/g (7). By comparing the adsorption effects of several macroporous resins and polyamide for separating flavonoids from C. paliurus, the influence of light and province on the flavone content is different in diverse regions. The flavone content in C. paliurus is also different due to the diversity of light and temperature (50).
3. Health benefits
The biological activity and many health properties of C. paliurus have been the focus of research in vitro and in vivo. As a result, there is a growing interest in the commercial application of C. paliurus. However, evidence from human epidemiological and interventional studies is still lacking. It is reported that C. Palinurus’s biological activities include antioxidant, hypoglycaemic, hypotensive, anti-inflammatory, antitumour, anti-ageing, cardioprotective effects, etc.
3.1. Antioxidant activity
The antioxidant activities of C. paliurus have been widely studied. The extract of C. paliurus, such as polysaccharides, flavonoids, and triterpenoid saponins, are closely correlated with apparent antioxidant effects. Several studies have focused on the antioxidant activity of C. paliurus, and their results are presented in Table 4. The trace elements and vitamin E in C. paliurus also help prevent oxidation. For example, selenium is involved in the formation of glutathione peroxidase in the body, which scavenges excess free radicals in the body (17). Xie et al. evaluated the antioxidant activity of CPP, compared with the DPPH free radical scavenging rate of BHT (91.2%) and ascorbic acid (98.9%). The results showed that CPP was 91.4% (24). After the antioxidant activity of CPP has been purified with a further step by DEAE-Cellulose, it can also significantly reduce MDA (Malondialdehyde) levels, increase the activity of SOD (Superoxide dismutase), T-AOC (Total antioxidant capacity,) and CAT (Catalase) (26). The antioxidant activity of CPP differs by different extraction methods. Research shows that ultrasonic treatment can improve the antioxidant activity of CPP by changing the monosaccharide composition. The difference may be caused by the change in monosaccharide composition, but the relationship between the enhancement of antioxidant activity and the change needs further investigation (19).
Moreover, the derivatization of CPP can improve antioxidant function, including sulfation, phosphorylation, carboxymethylation, and acetylation. In the carotenoid-linoleic acid assay, the highest substituted carboxymethylated CPP showed high antioxidant activity. However, the ability to scavenge hydroxyl and superoxide radicals decreased (35). Sulfated CPP scavenged hydroxyl radicals in a dose-dependent manner. Among them, sulfate groups are considered to play an important role. The antioxidant activity of these sulfated polysaccharides may depend on the sulfated derivatives’ chemical composition, molecular weight, and specific structural units (14). Further, an oxidative stress model was prepared in the RAW264.7 cell line using hydrogen peroxide (H2O2). Compared with unmodified CPP, it can significantly enhance the proliferation and phagocytosis activity, inhibit lipid oxidation as determined by the level of MDA, and promote the production of nitric oxide (NO) in RAW264.7 cells, which suggests that the sulfated CPP has particular immunological activity (11). Similar experimental results were obtained for phosphorylated CPP. It can reduce oxidative stress and cell oxidative damage by increasing intracellular antioxidant enzyme SOD content and decreasing MDA content while decreasing apoptotic capacity, improving cell viability and achieving intracellular antioxidant activity. According to Xie’s research, phosphorylated CPP decreased the MDA content to 34.8% compared with the model group (51). Moreover, acetylated CPP achieves antioxidant activity by regulating the Nrf2-Keap1 signaling pathway and improving the activity of antioxidant enzymes (34).
The phenolic compounds of quercetin and kaempferol glycosides are the main antioxidant components in the ethanol extracts of palm leaves (52). The content of phenolic compounds in the extracts from the leaves of C. paliurus was positively correlated with antioxidant activity. Cao et al. found that extracts with higher phenolic compound content had higher antioxidant activity (53). The Zhou et al. study found that the total polyphenol contributed most significantly to the antioxidant activity of 15 natural populations among flavonoids, phenolics and triterpenoids (54). In addition, the antioxidant capacity of phenolic compounds was related to the number and configuration of H-donating hydroxyl groups (53). Xie et al. isolated and purified flavonoids from the leaves of C. paliurus. The result showed that the DPPH radical scavenging rate of CPF was lower than that of VC but higher than that of BHT. The antioxidant activity of CPF was evaluated in vitro by DPPH radical-scavenging activity and showed higher antioxidant activity depending on the dose with higher concentrations (17). Xiong et al. extracted CPF by enzymatic digestion-assisted ultrasound. They employed three agents to analyze the antioxidant activity: DPPH radical scavenging activity, superoxide radical scavenging activity, and ABTS+ radical scavenging activity. To this end, Purified CPF showed significant scavenging activity against DPPH, superoxide and ABTS+ radicals, showing a dose-dependent scavenging activity (43). In vivo study was conducted on the beneficial effects of polysaccharides from the leaves of C. paliurus. It was found that CPP-treated worms had a robust antioxidant defense system, with an 81% reduction in ROS accumulation and reduced content of peroxidation products (MDA, NEFAs (Nonest esterified fatty acid) and GSSG (Glutathione)), while increasing antioxidant-active enzymes (SOD, CAT and GSH-Px (Glutathione peroxidase)) and GSH Glutathione, r-glutamyl cysteinyl +glycine) (52). Lin et al. developed a beverage which contains Momordica saponins and CPP. The in vivo study showed that it could promote nuclear localization of DAF-16 and translation of SOD-3 to activate the antioxidant system, reduce the levels of ROS (Reactive oxygen species), MDA, and NEFAs, and alleviate age-related pigmentation and damage caused by neurodegenerative diseases. In contrast, inhibiting of fat accumulation provides antioxidant protection and reduces oxidative damage (55). CPP alleviates ROS-induced oxidative stress mechanisms in vitro and in vivo, possibly by enhancing the scavenging activity of antioxidant enzymes on liver and kidney free radicals and inhibiting CYP2E1 expression, thereby improving oxidative stability (8). Further studies revealed that induction analysis of stress resistance indicated that the CPP-mediated response CPP-mediated stress response is dependent on skn-1 and hsf-1, which may act together with genes responsible for stress induction, including sod-3, sod-5 hsp-16.1, hsp-16.2, ctl-1, and ctl-2 (18).
3.2. Hypoglycemic activities
The role of C. paliurus in regulating diabetes has also been demonstrated. Hyperglycemia is one of the main symptoms of diabetes. Due to the apparent hypoglycemic effect of C. paliurus, it has aroused broad interest among researchers. Several researchers have investigated the hypoglycemic activity of C. paliurus through in vivo studies (Table 5). Zhang et al. reported the hypoglycemic bioactivity of triterpenic acid of C. paliurus through in vivo and in vitro experiments. A kidney injury model was established in vitro using HG-induced HK-2 cells, and CPT treatment significantly ameliorated HG-induced apoptosis in kidney cells. In vivo studies showed that triterpenic acid with different concentrations significantly reduced blood glucose in STZ (Streptozocin)-induced diabetic rats and showed a significant increase in body weight.
In addition, significant urinary protein levels in the urine improved renal meningeal stromal accumulation and glomerular fibrosis. CPT (C. paliurus triterpenoids) treatment significantly increased the phosphorylation of AMPK (Adenosine 5′-monophosphate (AMP)-activated protein kinase) and decreased the phosphorylation of its downstream effector mTOR (mammalian target of rapamycin). It effectively improved kidney damage caused by diabetes (59). Xiao et al. found that CPE (CP extract) significantly reduced the proportion of apoptotic cells, inhibited apoptosis in pancreatic β-cells, and inhibited STZ-induced upregulation of caspase-3 and caspase-9 in a dose-dependent manner. CPE effectively inhibited the STZ-induced increase in ERK (extracellular regulated protein kinases), JNK (c-Jun N-terminal kinase), and p38 phosphorylation in NIT-1 cells and increased in a dose-dependent manner reduction of Akt (protein kinase B) phosphorylation. Bodyweight, food intake and plasma glucose levels were significantly decreased in mice treated with CPE. The wet pancreas/body weight ratio, plasma insulin levels, β-cell area, and insulin-secreting β-cells were significantly increased (60). These findings suggest that CPE protects pancreatic β-cells from apoptosis by affecting MAPK (mitogen-activated protein kinase) and Akt signaling pathways. Another related study found that CPE enhanced tyrosine phosphorylation of insulin receptor substrates in C2C12 cells and activated phosphatidylinositol 3-kinase and Akt via sirtuin1.
Furthermore, oral administration of CPE increased the expression of Glut4 in skeletal muscle membranes. It enhanced the phosphorylation of Akt in STZ-induced hyperglycemic mice with reduced plasma glucose levels, and the signaling pathway is shown in Figure 3 (58). Jiang et al. also found that CPE can regulate adipokine expression and improve insulin resistance by suppressing inflammation in mice (61). Li et al. established a diabetes model. Their results showed that Various fractions of C. paliurus bark significantly reduced blood glucose levels in diabetic rats, especially the chloroform fraction (56). Their biological activities determined the phenolic compounds from C. paliurus leaves through PTP1B inhibition experiments. Compared with the control group (HD0518), the study found that the naphthoquinone derivatives and phenolic compounds in the extracts inhibit the activity of PTPIB as a potential functional food ingredient to prevent and treat diabetes and obesity (62).
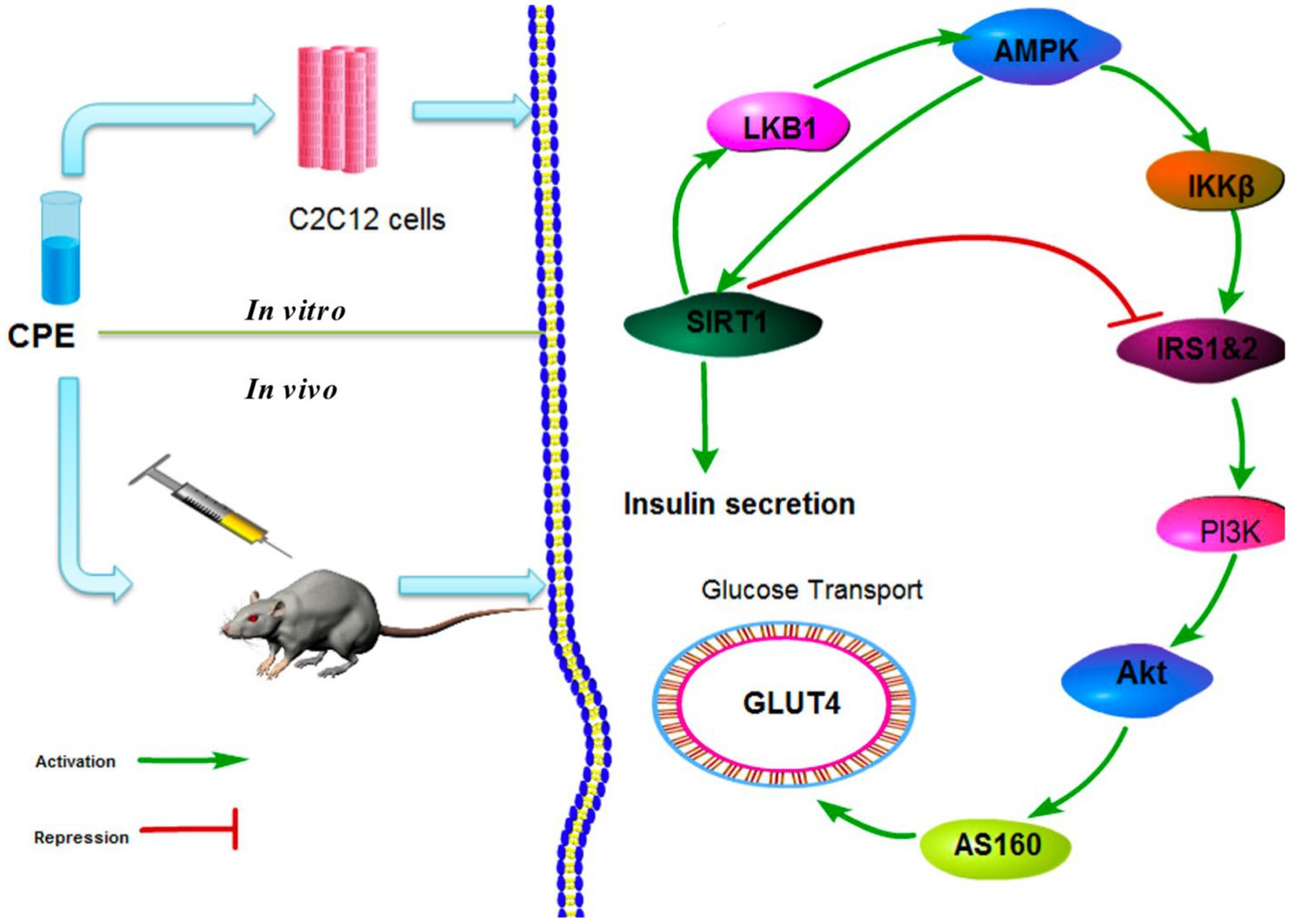
Figure 3. CPE in vivo and in vitro hypoglycemic mechanism (58).
3.3. Hypolipidemic activities
Several research groups have investigated the hypoglycemic activity of C. paliurus in animal models. The results are presented in Table 6. The hypoglycemic activity of CPP was investigated by high-fat emulsion (HFE)-induced hyperlipidemic rats for 8 weeks. The addition of CPP to the diet caused a significant decrease in plasma TC (total cholesterol), TG (Triglyceride), and LDL-C (Low-Density Lipoprotein Cholesterol) levels. It increased HDL-C (High-density Lipoprotein Cholesterol) levels in HFE diet rats. CPP significantly increased ATGL (Adipose Triglyceride Lipase) and PPAR (peroxisome proliferators-activated receptors) expression in the liver group, while hepatic FAS (Fatty acid synthase) and HMG-CoA (3-hydroxy-3-methyl glutaryl coenzyme A reductase) expression were relatively decreased (22). They demonstrated for the first time that CPP regulates the promoter expression levels of leptin and MTTP (Microsomal triglyceride transfer protein) by altering DNA methylation in the promoter (63). The team used CPP-2 purified by a DEAE-cellulose column as a raw material for further investigation. Similar experimental results were obtained. Compared with the model control group, CPP-2 significantly decreased TG, TC and LDL-C levels and increased HDL-C levels in mice. Also, CPP-2 significantly counteracted the increased oxidative stress by lowering LPO (Lipid peroxide) and MDA levels in serum and liver and increasing the activity of SOD, GSH-PX, GPT and T-AOC (12). While in another study, Lin et al. elucidated the lipid-lowering effect and mechanism of CPP on Caenorhabditis elegans. The result showed that CPP regulates lipid metabolic pathways through the MUFA biosynthetic pathway, mdt-15/sbp-1 and NHR-49/MDT-15 signaling pathways to reduce fat accumulation in C. elegans (64). Zhu et al. found that CPP can protect islets by reducing oxidative stress and pro-inflammatory cytokines. It also reduced dyslipidemia, hepatic steatosis, and liver diseases while CPP down-regulated lipid-induced metabolism, redox, and apoptosis-related genes and up-regulated miR-199a/31a gene, indicating it can help prevent liver damage (21). Yao et al. found that the preventive effect of CPE on hyperlipidemia and obesity was partly due to blocking fat absorption from the intestine by inhibiting the activity of apoB48. It is hypothesized that it may lower serum and liver cholesterol by inhibiting the activity of 3-hydroxy-3-methylglutaryl coenzyme A reductase while enhancing the activity of cholesterol 7α-hydroxylase to promote cholesterol excretion (10). In addition, triterpenoids were isolated from cyanobacteria and found to inhibit lipoprotein B48 secretion in the cells in a Caco-2 cell model (36).
3.4. Immunomodulatory activity
The inflammatory response is a defensive response of the body when it is invaded or infected by pathogens. Macrophages are important to the immune system members, and macrophages’ activation is a critical factor in the inflammatory response. Table 7 lists the immunological activities of the active substances of C. paliurus. CPP-3 was studied in the RAW264.7 cell assay and significantly promoted the release of TNF-α, IL-1 and PGE2 from cells when CPP-3 concentrations ranged from 12.5 to 200 μg/ mg (p < 0.01). In LPS -stimulated RAW264.7 cells, CPP-3 inhibited the cellular release of NO (Nitric Oxide), TNF-α, IL-1, and PGE2 (Prostaglandin E2) (p < 0.01) and had a synergistic effect on NO production (29). Meanwhile, CPP-3 up-regulated the expression levels of iNOS (Inducible Nitric Oxide Synthase), IFN-γ (Interferon-γ), TNF-α, IL-12 and IL-18 mRNA in RAW264.7 cells (65). Furthermore, modified CPP was non-toxic to RAW264.7 cells and enhanced the immunomodulatory effect on RAW264.7 macrophages compared to CPP (32, 33). Further studies have shown that the effect of sulfated CPP with the degree of substitution on cell proliferation is different. However, all of them can promote the secretion of Th1 cytokines, IL-2 and TNF-α, and Th2 cytokine IL-4 (31), which exerts immune mechanisms related to MAPK and NF-κB (nuclear factor kappa-B) pathways (66).
3.5. Liver protection
Nowadays, liver injury caused by various chronic diseases has become one of the most common diseases in the world. Carbon tetrachloride (CCl4) is a typical environmental poison often used to establish animal models of acute and chronic liver and kidney diseases (67). Table 8 lists some studies on the hepatoprotective effects of C. paliurus substances. Xie et al. found that CPF significantly inhibited the increase of AST (Aspartate aminotransferase) and ALT (Alanine aminotransferase) activities in CCl4-induced liver injury serum in mice. Simultaneously, CPF would significantly reduce the content of MDA in liver tissues and enhance SOD and T-AOC activities in liver tissues (68). Wang et al. verified the protective effect of CPF against LPS/D-GalN-induced acute liver failure. The mechanism might be related to inhibiting NF-κB activation and activating the Nrf2/HO-1 pathway (44). Furthermore, CPT-regulated palmitic acid-induced hepatic steatosis in HepG2 cells showed that CPT could significantly reduce the number of lipid droplets and intracellular TG content PA-induced HepG2 cells and primary hepatocytes. The molecular mechanism studies showed that CPT regulates the pathway of the PI3K (phosphoinositide 3-kinase) /Akt/GSK3β (phosphorylation of glycogen synthase-3β). Similar experimental results were obtained in animal models, increasing PI3K, Akt and GSK3β, and the PI3K inhibitor was replaced by LY294002 (69). In another study, CP may protect the liver by improving oxidative stress to protect the liver and kidney from CCl4 damage (8). LPS (Lipopolysaccharide) stimulated the cells to secrete the opposite pro-inflammatory cytokines, and D-GalN (D-galactosamine) inhibited the mRNA synthesis of anti-apoptotic genes and induced liver necrosis.
3.6. Other activities
In addition to antioxidant, hypoglycemic, and lipid-lowering effects, other aspects of C. paliurus are also being developed. Polysaccharides from C. paliurus leaves have antibacterial activity. It was found that CPP (1 mg/mL) had good antibacterial activity against Saccharomyces cerevisiae and Candida, and the inhibition zone was 9.72 ± 0.16 mm and 10.21 ± 0.37 mm, respectively. It also had specific antibacterial activity against Escherichia coli, Staphylococcus aureus, and Bacillus subtilis. The diameter of the inhibition zone was 6.54 ± 0.23, 6.57 ± 0.11, and 6.93 ± 0.45 mm, respectively. However, at the concentration of 1 mg/ml, the polysaccharides had no inhibitory effect on three kinds of fungi (Aspergillus niger, Mucor, and Penicillium) (23). In another study, the antibacterial activity of CPP was concentration-dependent. When the concentration of polysaccharides was 80 μg/ml, it had an apparent inhibitory effect on Staphylococcus aureus, Salmonella, Escherichia coli, and other microorganisms. The inhibition zone diameter was 21.5 ± 0.45, 17.5 ± 0.35, and 13.5 ± 0.25 mm, respectively. The antibacterial mechanism of CPP needs to be further studied. Experimental research by Lei et al. showed that when the concentration of flavonoids in C. paliurus is higher than 40 mg/mL, the antibacterial and bactericidal effects are better. The purified flavonoids in C. paliurus had the most substantial inhibitory ability against Staphylococcus aureus, followed by salmonella, and the weakest ability inhibitory capacity against E.coli (43). These results indicate that C. paliurus can be used as an antibacterial agent for some bacteria.
CPP has been proven to have antitumor activity, and its mechanism was explored by evaluating its antitumor activity in vitro. Different concentrations of CPP (50, 100, 200, and 400 μg/mL) were used to treat HeLa cancer cells. In the concentration range of 50–200 μg/mL with the increase of CPP concentration, the inhibitory effect on HeLa cells was significantly enhanced in a dose-dependent manner. The results showed that CPP could directly inhibit the proliferation of HeLa cancer cells, suggesting that CPP can inhibit the growth of HeLa cancer cells through S-phase cell cycle arrest and induce apoptosis (70). Moreover, the team continued to study CPP’s effect on the composition of intestinal flora. The results showed that CPP could significantly increase the short-chain fatty acids (acetic acid, propionic acid, butyric acid, and valeric acid) in the faeces of healthy mice in a dose-dependent manner.
Furthermore, 16S rRNA showed that CPP could effectively increase the diversity of intestinal microflora in healthy mice and affect the relative abundance of Chlamydia, Clostridium and Clostridium. In addition, PICRUSt2 showed that the phenotype of high-dose mice was metabolized by the KEGG pathway (48). Thus, CPP can enhance the metabolic function of intestinal microbiota by increasing the release of SCFAs and changing the composition of intestinal microbiota.
4. Conclusion and commercial value
This mini-review summarizes the extraction techniques, chemical structures, and biological activities of some of the polysaccharides, flavonoids, and saponins found in C. paliurus, suggesting that C. paliurus has potential medicinal and dietary value. In the last few years, C. paliurus has gained the attention of the scientific and business communities due to its versatility in food, pharmaceuticals, waste management, and sustainable agricultural practices. More recently, research interest has shifted toward incorporating C. paliurus trees into beverages and beverage products to improve physicochemical properties, shelf life, and nutritional value. Jiangxi Xiushui has developed a health drink from C. paliurus, which uses C. paliurus leaves as primary raw material for functional species of health drinks such as hypoglycemia, and is the first health tea to receive FDA quality approval. Beijing Orient Kewen has also developed a series of health products from C. paliurus, including tablets, granules, capsules, and lozenges, all of which have received the “Health Food Approval Certificate” issued by the state. In addition, the C. paliurus leaves are ground into flour and mixed with wheat flour to make biscuits. Compared with ordinary biscuits, C. paliurus biscuits have a hypoglycemic effect and suit people prone to obesity and high blood sugar. C. paliurus has a unique sweet flavour and is commonly used in folklore for sweet biscuits and sweets and is a natural food additive that can be added directly to food. Its main components are caryophyllene A and nucleoside I, which are more than 200 times sweeter than sucrose.
Cyclocarya paliurus is a plant with multidimensional utility and versatile applications in human nutrition. There is still a need for in-depth research into C. paliurus, through in vivo studies in animals and epidemiological studies, to better understand the effects on human health. Cyclocarya paliurus can be an excellent reserve for pharmaceutical and herbal preparations. The wealth of phytochemical information and advances in biotechnology have led to the creation of new avenues aimed at increasing the overall commercial value of the tree.
Author contributions
JW and DY proposed the concept of the review. YS, YP, XZ, HL, and FK collected and analyzed the literature. YS, YP, and JW drafted the manuscript. JW, YS, DY, and LZ critically revised the manuscript. All authors have read and approved the final version of the manuscript.
Conflict of interest
The authors declare that the research was conducted in the absence of any commercial or financial relationships that could be construed as a potential conflict of interest.
Publisher’s note
All claims expressed in this article are solely those of the authors and do not necessarily represent those of their affiliated organizations, or those of the publisher, the editors and the reviewers. Any product that may be evaluated in this article, or claim that may be made by its manufacturer, is not guaranteed or endorsed by the publisher.
Funding
This work was supported by China Postdoctoral Science Foundation (621312-2), Talent Project of Guangzhou University (Nos RP2020078 and RD2020052), the National Natural Science Foundation of China (32060576) and ‘Gui-pai Xinglin young talent’ from Guangxi University of Chinese Medicine (2022C026).
References
1. Pearson, TA, Mensah, GA, Alexander, RW, Anderson, JL, Cannon, RO, Criqui, M, et al. Markers of inflammation and cardiovascular disease application to clinical and public health practice – a statement for healthcare professionals from the centers for disease control and prevention and the American Heart Association. Circulation. (2003) 107:499–511. doi: 10.1161/01.Cir.0000052939.59093.45
2. Townsend, N, Wilson, L, Bhatnagar, P, Wickramasinghe, K, Rayner, M, and Nichols, M. Corrigendum to: Cardiovascular disease in Europe: epidemiological update 2016. Eur Heart J. (2019) 40:189–9. doi: 10.1093/eurheartj/ehy342
3. Qin, D-W, Gu, Z, and Guo, J-Y. Medicinal mushroom for prevention of disease of modern civilization. Evid Based Complement Alternat Med. (2015) 2015:1–2. doi: 10.1155/2015/812725
4. Khanuja, SPS. Functional diversity of plant metabolome and microbiome in health services to the human life. Proc Natl Acad Sci India B. (2012) 82:291–4. doi: 10.1007/s40011-012-0105-2
5. Fang, S, Sun, D, Shang, X, Fu, X, and Yang, W. Variation in radial growth and wood density of Cyclocarya paliurus across its natural distribution. New For. (2020) 51:453–67. doi: 10.1007/s11056-019-09742-9
6. Fang, S, Yang, W, Chu, X, Shang, X, She, C, and Fu, X. Provenance and temporal variations in selected flavonoids in leaves of Cyclocarya paliurus. Food Chem. (2011) 124:1382–6. doi: 10.1016/j.foodchem.2010.07.095
7. Cheng, L, Chen, Y, Zhang, X, Zheng, X, Cao, J, Wu, Z, et al. A metagenomic analysis of the modulatory effect of Cyclocarya paliurus flavonoids on the intestinal microbiome in a high-fat diet-induced obesity mouse model. J Sci Food Agric. (2019) 99:3967–75. doi: 10.1002/jsfa.9622
8. Wu, T, Shen, M, Liu, S, Yu, Q, Chen, Y, and Xie, J. Ameliorative effect of Cyclocarya paliurus polysaccharides against carbon tetrachloride induced oxidative stress in liver and kidney of mice. Food Chem Toxicol. (2020) 135:111014. doi: 10.1016/j.fct.2019.111014
9. Jiang, ZY, Zhang, XM, Zhou, J, Qiu, SX, and Chen, JJ. Two new triterpenoid glycosides from Cyclocarya paliurus. J Asian Nat Prod Res. (2006) 8:93–8. doi: 10.1080/10286020500480217
10. Yao, X, Lin, Z, Jiang, C, Gao, M, Wang, Q, Yao, N, et al. Cyclocarya paliurus prevents high fat diet induced hyperlipidemia and obesity in Sprague-Dawley rats. Can J Physiol Pharmacol. (2015) 93:677–86. doi: 10.1139/cjpp-2014-0477
11. Wang, ZJ, Xie, JH, Kan, LJ, Wang, JQ, Shen, MY, Li, WJ, et al. Sulfated polysaccharides from Cyclocarya paliurus reduce H2O2-induced oxidative stress in RAW264.7 cells. Int J Biol Macromol. (2015) 80:410–7. doi: 10.1016/j.ijbiomac.2015.06.031
12. Yang, Z, Wang, J, Li, J, Xiong, L, Chen, H, Liu, X, et al. Antihyperlipidemic and hepatoprotective activities of polysaccharide fraction from Cyclocarya paliurus in high-fat emulsion-induced hyperlipidaemic mice. Carbohydr Polym. (2018) 183:11–20. doi: 10.1016/j.carbpol.2017.11.033
13. Lei, X, Ke-Hui, O, Yan, J, Zhan-Wei, Y, Wen-Bing, H, Hui, C, et al. Chemical composition of Cyclocarya paliurus polysaccharide and inflammatory effects in lipopolysaccharide-stimulated RAW264.7 macrophage. Int J Biol Macromol. (2018) 107:1898–907. doi: 10.1016/j.ijbiomac.2017.10.055
14. Xie, J-H, Wang, Z-J, Shen, M-Y, Nie, S-P, Gong, B, Li, H-S, et al. Sulfated modification, characterization and antioxidant activities of polysaccharide from Cyclocarya paliurus. Food Hydrocoll. (2016) 53:7–15. doi: 10.1016/j.foodhyd.2015.02.018
15. Wang, Q, Jiang, C, Fang, S, Wang, J, Ji, Y, Shang, X, et al. Antihyperglycemic, antihyperlipidemic and antioxidant effects of ethanol and aqueous extracts of Cyclocarya paliurus leaves in type 2 diabetic rats. J Ethnopharmacol. (2013) 150:1119–27. doi: 10.1016/j.jep.2013.10.040
16. Wang, J, and Wang, K. Fatigue-alleviating effect of polysaccharides from Cyclocarya paliurus (Batal) Iljinskaja in mice. Afr J Microbiol Res. (2012) 6:5243–8. doi: 10.5897/ajmr12.655
17. Xie, JH, Dong, CJ, Nie, SP, Li, F, Wang, ZJ, Shen, MY, et al. Extraction, chemical composition and antioxidant activity of flavonoids from Cyclocarya paliurus (Batal.) Iljinskaja leaves. Food Chem. (2015) 186:97–105. doi: 10.1016/j.foodchem.2014.06.106
18. Lin, C, Su, Z, Luo, J, Jiang, L, Shen, S, Zheng, W, et al. Polysaccharide extracted from the leaves of Cyclocarya paliurus (Batal.) Iljinskaja enhanced stress resistance in Caenorhabditis elegans via skn-1 and hsf-1. Int J Biol Macromol. (2020) 143:243–54. doi: 10.1016/j.ijbiomac.2019.12.023
19. Tang, W, Lin, L, Xie, J, Wang, Z, Wang, H, Dong, Y, et al. Effect of ultrasonic treatment on the physicochemical properties and antioxidant activities of polysaccharide from Cyclocarya paliurus. Carbohydr Polym. (2016) 151:305–12. doi: 10.1016/j.carbpol.2016.05.078
20. Hu, W-B, Zhao, J, Chen, H, Xiong, L, and Wang, W-J. Polysaccharides from Cyclocarya paliurus: chemical composition and lipid-lowering effect on rats challenged with high-fat diet. J Funct Foods. (2017) 36:262–73. doi: 10.1016/j.jff.2017.07.020
21. Li, J, Luo, M, Luo, Z, Guo, A-Y, Yang, X, Hu, M, et al. Transcriptome profiling reveals the anti-diabetic molecular mechanism of Cyclocarya paliurus polysaccharides. J Funct Foods. (2019) 55:1–8. doi: 10.1016/j.jff.2018.12.039
22. Yang, ZW, Ouyang, KH, Zhao, J, Chen, H, Xiong, L, and Wang, WJ. Structural characterization and hypolipidemic effect of Cyclocarya paliurus polysaccharide in rat. Int J Biol Macromol. (2016) 91:1073–80. doi: 10.1016/j.ijbiomac.2016.06.063
23. Xie, JH, Shen, MY, Xie, MY, Nie, SP, Chen, Y, Li, C, et al. Ultrasonic-assisted extraction, antimicrobial and antioxidant activities of Cyclocarya paliurus (Batal.) Iljinskaja polysaccharides. Carbohydr Polym. (2012) 89:177–84. doi: 10.1016/j.carbpol.2012.02.068
24. Xie, J-H, Xie, M-Y, Nie, S-P, Shen, M-Y, Wang, Y-X, and Li, C. Isolation, chemical composition and antioxidant activities of a water-soluble polysaccharide from Cyclocarya paliurus (Batal.) Iljinskaja. Food Chem. (2010) 119:1626–32. doi: 10.1016/j.foodchem.2009.09.055
25. Xie, JH, Shen, MY, Nie, SP, Liu, X, Zhang, H, and Xie, MY. Analysis of monosaccharide composition of Cyclocarya paliurus polysaccharide with anion exchange chromatography. Carbohydr Polym. (2013) 98:976–81. doi: 10.1016/j.carbpol.2013.07.011
26. An, Q, Ye, X, Han, Y, Zhao, M, Chen, S, Liu, X, et al. Structure analysis of polysaccharides purified from Cyclocarya paliurus with DEAE-Cellulose and its antioxidant activity in RAW264.7 cells. Int J Biol Macromol. (2020) 157:604–15. doi: 10.1016/j.ijbiomac.2019.11.212
27. Xie, J-H, Shen, M-Y, Nie, S-P, Li, C, and Xie, M-Y. Decolorization of polysaccharides solution from Cyclocarya paliurus (Batal.) Iljinskaja using ultrasound/H2O2 process. Carbohydr Polym. (2011) 84:255–61. doi: 10.1016/j.carbpol.2010.11.030
28. Xie, JH, Shen, MY, Nie, SP, Zhao, Q, Li, C, and Xie, MY. Separation of water-soluble polysaccharides from Cyclocarya paliurus by ultrafiltration process. Carbohydr Polym. (2014) 101:479–83. doi: 10.1016/j.carbpol.2013.09.075
29. Xiong, L, Ouyang, KH, Jiang, Y, Yang, ZW, Hu, WB, Chen, H, et al. Chemical composition of Cyclocarya paliurus polysaccharide and inflammatory effects in lipopolysaccharide-stimulated RAW264.7 macrophage. Int J Biol Macromol. (2018) 107:1898–907. doi: 10.1016/j.ijbiomac.2017.10.055
30. Zhang, L, Hu, Y, Duan, X, Tang, T, Shen, Y, Hu, B, et al. Characterization and antioxidant activities of polysaccharides from thirteen boletus mushrooms. Int J Biol Macromol. (2018) 113:1–7. doi: 10.1016/j.ijbiomac.2018.02.084
31. Yu, Y, Song, Q, Huang, L, Shen, M, Yu, Q, Chen, Y, et al. Immunomodulatory activities of sulfated Cyclocarya paliurus polysaccharides with different degrees of substitution on mouse spleen lymphocytes. J Funct Foods. (2020) 64:103706. doi: 10.1016/j.jff.2019.103706
32. Yu, Y, Shen, M, Wang, Z, Wang, Y, Xie, M, and Xie, J. Sulfated polysaccharide from Cyclocarya paliurus enhances the immunomodulatory activity of macrophages. Carbohydr Polym. (2017) 174:669–76. doi: 10.1016/j.carbpol.2017.07.009
33. Liu, X, Xie, J, Jia, S, Huang, L, Wang, Z, Li, C, et al. Immunomodulatory effects of an acetylated Cyclocarya paliurus polysaccharide on murine macrophages RAW264.7. Int J Biol Macromol. (2017) 98:576–81. doi: 10.1016/j.ijbiomac.2017.02.028
34. Meng-Zhao,, Yi-Han,, Li, J, Qi-An,, Ye, X, Xiang-Li,, et al. Structural characterization and antioxidant activity of an acetylated Cyclocarya paliurus polysaccharide (Ac-CPP0.1). Int J Biol Macromol. (2021) 171:112–22. doi: 10.1016/j.ijbiomac.2020.12.201
35. Wang, ZJ, Xie, JH, Shen, MY, Tang, W, Wang, H, Nie, SP, et al. Carboxymethylation of polysaccharide from Cyclocarya paliurus and their characterization and antioxidant properties evaluation. Carbohydr Polym. (2016) 136:988–94. doi: 10.1016/j.carbpol.2015.10.017
36. Wu, ZF, Meng, FC, Cao, LJ, Jiang, CH, Zhao, MG, Shang, XL, et al. Triterpenoids from Cyclocarya paliurus and their inhibitory effect on the secretion of apoliprotein B48 in Caco-2 cells. Phytochemistry. (2017) 142:76–84. doi: 10.1016/j.phytochem.2017.06.015
37. Ma, Y, Jiang, C, Yao, N, Li, Y, Wang, Q, Fang, S, et al. Antihyperlipidemic effect of Cyclocarya paliurus (Batal.) Iljinskaja extract and inhibition of apolipoprotein B48 overproduction in hyperlipidemic mice. J Ethnopharmacol. (2015) 166:286–96. doi: 10.1016/j.jep.2015.03.030
38. Shu, R-G, Xu, C-R, Li, L-N, and Yu, Z-L. Cyclocariosides II and III: two Secodammarane Triterpenoid Saponins from Cyclocarya paliurus. Planta Med. (1995) 61:551–3. doi: 10.1055/s-2006-959369
39. Cui, B-S, and Li, S. New triterpenoid saponins from the leaves of Cyclocarya paliurus. Chin Chem Lett. (2015) 26:585–9. doi: 10.1016/j.cclet.2014.11.033
40. Shang, XL, Wu, ZF, Yin, ZQ, Zhang, J, Liu, ZJ, and Fang, SZ. Shang and co-authors describe advances in complementary and alternative medicine (Simultaneous determination of flavonoids and triterpenoids in Cyclocarya paliurus leaves using high-performance liquid). Technol Bus J. (2015) 12
41. Zhou, M, Lin, Y, Fang, S, Liu, Y, and Shang, X. Phytochemical content and antioxidant activity in aqueous extracts of Cyclocarya paliurus leaves collected from different populations. PeerJ. (2019) 7:e6492. doi: 10.7717/peerj.6492
42. Zhao, W, Tang, D, Yuan, E, Wang, M, Zhang, Q, Liu, Y, et al. Inducement and cultivation of novel red Cyclocarya paliurus callus and its unique morphological and metabolic characteristics. Ind Crop Prod. (2020) 147:112266. doi: 10.1016/j.indcrop.2020.112266
43. Lei, X, Hu, W-B, Yang, Z-W, Hui, C, Wang, N, Liu, X, et al. Enzymolysis-ultrasonic assisted extraction of flavanoid from Cyclocarya paliurus (Batal) Iljinskaja:HPLC profile, antimicrobial and antioxidant activity. Ind Crop Prod. (2019) 130:615–26. doi: 10.1016/j.indcrop.2019.01.027
44. Hu, W-B, Ouyang, K-H, Wu, G-Q, Chen, H, Xiong, L, Liu, X, et al. Hepatoprotective effect of flavonoid-enriched fraction from Cyclocarya paliurus leaves on LPS/D-GalN-induced acute liver failure. J Funct Foods. (2018) 48:337–50. doi: 10.1016/j.jff.2018.07.031
45. Liu, Y, Cao, Y, Fang, S, Wang, T, Yin, Z, Shang, X, et al. Antidiabetic effect of Cyclocarya paliurus leaves depends on the contents of antihyperglycemic flavonoids and antihyperlipidemic triterpenoids. Molecules. (2018) 23:1042. doi: 10.3390/molecules23051042
46. Li, J, Liu, X, Gao, Y, Zong, G, Wang, D, Liu, M, et al. Identification of a UDP-Glucosyltransferase favouring substrate- and regio-specific biosynthesis of flavonoid glucosides in Cyclocarya paliurus. Phytochemistry. (2019) 163:75–88. doi: 10.1016/j.phytochem.2019.04.004
47. Yang, SX, Liu, B, Tang, M, Yang, J, Kuang, Y, Zhang, MZ, et al. Extraction of flavonoids from Cyclocarya paliurus (Juglandaceae) leaves using ethanol/salt aqueous two-phase system coupled with ultrasonic. J Food Proc Preservat. (2020) 44:e14469. doi: 10.1111/jfpp.14469
48. Wu, T, Shen, M, Yu, Q, Chen, Y, Chen, X, Yang, J, et al. Cyclocarya paliurus polysaccharide improves metabolic function of gut microbiota by regulating short-chain fatty acids and gut microbiota composition. Food Res Int. (2021) 141:110119. doi: 10.1016/j.foodres.2021.110119
49. Jawhari, FZ, Moussaoui, AEL, Bourhia, M, Imtara, H, Saghrouchni, H, Ammor, K, et al. Anacyclus pyrethrum var. pyrethrum (L.) and Anacyclus pyrethrum var. depressus (Ball) Maire: correlation between total phenolic and flavonoid contents with antioxidant and antimicrobial activities of chemically characterized extracts. Plant. (2021) 10:149. doi: 10.3390/plants10010149
50. Bo, D, Xulan, S, Shengzuo, F, Qiongqiong, L, Xiangxiang, F, and Jun, S. Integrated effects of light intensity and fertilization on growth and flavonoid accumulation in Cyclocarya paliurus. J Agric Food Chem. (2012) 60:6286–92. doi: 10.1021/jf301525s
51. Xie, L, Shen, M, Wen, P, Hong, Y, Liu, X, and Xie, J. Preparation, characterization, antioxidant activity and protective effect against cellular oxidative stress of phosphorylated polysaccharide from Cyclocarya paliurus. Food Chem Toxicol. (2020) 145:111754–4. doi: 10.1016/j.fct.2020.111754
52. Liu, Y, Chen, P, Zhou, M, Wang, T, Fang, S, Shang, X, et al. Geographic variation in the chemical composition and antioxidant properties of phenolic compounds from Cyclocarya paliurus (Batal) Iljinskaja leaves. Molecules. (2018) 23:2440. doi: 10.3390/molecules23102440
53. Cao, Y, Fang, S, Fu, X, Shang, X, and Yang, W. Seasonal variation in phenolic compounds and antioxidant activity in leaves of Cyclocarya paliurus (Batal.) Iljinskaja. Forests. (2019) 10:624. doi: 10.3390/f10080624
54. Zhou, M, Chen, P, Lin, Y, Fang, S, and Shang, X. A comprehensive assessment of bioactive metabolites, antioxidant and antiproliferative activities of Cyclocarya paliurus (Batal.) Iljinskaja leaves. Forests. (2019) 10:625. doi: 10.3390/f10080625
55. Lin, C, Lin, Y, Xiao, J, Lan, Y, Cao, Y, and Chen, Y. Effect of Momordica saponin- and Cyclocarya paliurus polysaccharide-enriched beverages on oxidative stress and fat accumulation in Caenorhabditis elegans. J Sci Food Agric. (2020) 101:3366–75. doi: 10.1002/jsfa.10966
56. Li, S, Li, J, Guan, X-L, Li, J, Deng, S-P, Li, L-Q, et al. Hypoglycemic effects and constituents of the barks of Cyclocarya paliurus and their inhibiting activities to glucosidase and glycogen phosphorylase. Fitoterapia. (2011) 82:1081–5. doi: 10.1016/j.fitote.2011.07.002
57. Wang, X, Li, W, and Kong, D. Cyclocarya paliurus extract alleviates diabetic nephropathy by inhibiting oxidative stress and aldose reductase. Ren Fail. (2016) 38:678–85. doi: 10.3109/0886022X.2016.1155394
58. Yoshitomi, H, Tsuru, R, Li, L, Zhou, J, Kudo, M, Liu, T, et al. Cyclocarya paliurus extract activates insulin signaling via Sirtuin1 in C2C12 myotubes and decreases blood glucose level in mice with impaired insulin secretion. PLoS One. (2017) 12:e0183988. doi: 10.1371/journal.pone.0183988
59. Zhang, XX, Jiang, CH, Liu, Y, Lou, DX, Huang, YP, Gao, M, et al. Cyclocarya paliurus triterpenic acids fraction attenuates kidney injury via AMPK-mTOR-regulated autophagy pathway in diabetic rats. Phytomedicine. (2019) 64:153060. doi: 10.1016/j.phymed.2019.153060
60. Hai-Tao, X, Bo, W, Zi-Wan, N, Li-Xiang, Z, Cheng-Hui, L, Cheng-Yuan, L, et al. Cyclocarya paliurus tea leaves enhances pancreatic β cell preservation through inhibition of apoptosis. Sci Rep. (2017) 7:9155. doi: 10.1038/s41598-017-09641-z
61. Jiang, C, Yao, N, Wang, Q, Zhang, J, Sun, Y, Xiao, N, et al. Cyclocarya paliurus extract modulates adipokine expression and improves insulin sensitivity by inhibition of inflammation in mice. J Ethnopharmacol. (2014) 153:344–51. doi: 10.1016/j.jep.2014.02.003
62. Zhang, J, Shen, Q, Lu, J-C, Li, J-Y, Liu, W-Y, Yang, J-J, et al. Phenolic compounds from the leaves of Cyclocarya paliurus (Batal.) Ijinskaja and their inhibitory activity against PTP1B. Food Chem. (2010) 119:1491–6. doi: 10.1016/j.foodchem.2009.09.031
63. Yang, Z, Zhao, J, Wang, J, Li, J, Ouyang, K, and Wang, W. Effects of Cyclocarya paliurus polysaccharide on lipid metabolism-related genes DNA methylation in rats. Int J Biol Macromol. (2019) 123:343–9. doi: 10.1016/j.ijbiomac.2018.11.110
64. Lin, C, Lin, Y, Meng, T, Lian, J, Liang, Y, Kuang, Y, et al. Anti-fat effect and mechanism of polysaccharide-enriched extract from Cyclocarya paliurus (Batal.) Iljinskaja in Caenorhabditis elegans. Food Funct. (2020) 11:5320–32. doi: 10.1039/c9fo03058a
65. Xiong, L, Ouyang, K-H, Chen, H, Yang, Z-W, Hu, W-B, Wang, N, et al. Immunomodulatory effect of Cyclocarya paliurus polysaccharide in cyclophosphamide induced immunocompromised mice. Bioact Carbohydr Diet Fibre. (2020) 24:100224. doi: 10.1016/j.bcdf.2020.100224
66. Han, Y, Ouyang, K, Li, J, Liu, X, An, Q, Zhao, M, et al. Sulfated modification, characterization, immunomodulatory activities and mechanism of the polysaccharides from Cyclocarya paliurus on dendritic cells. Int J Biol Macromol. (2020) 159:108–16. doi: 10.1016/j.ijbiomac.2020.04.265
67. Bo, Z, Yongzhe, L, Tao, H, and Yan, Z. The hepatoprotective effect of polysaccharides from Pleurotus ostreatus on carbon tetrachloride-induced acute liver injury rats. Int J Biol Macromol. (2019) 131:1–9. doi: 10.1016/j.ijbiomac.2019.03.043
68. Xie, J, Wang, W, Dong, C, Huang, L, Wang, H, Li, C, et al. Protective effect of flavonoids from Cyclocarya paliurus leaves against carbon tetrachloride-induced acute liver injury in mice. Food Chem Toxicol. (2018) 119:392–9. doi: 10.1016/j.fct.2018.01.016
69. Zheng, X, Zhao, M-G, Jiang, C-H, Sheng, X-P, Yang, H-M, Liu, Y, et al. Triterpenic acids-enriched fraction from Cyclocarya paliurus attenuates insulin resistance and hepatic steatosis via PI3K/Akt/GSK3β pathway. Phytomedicine. (2020) 66:153130. doi: 10.1016/j.phymed.2019.153130
Keywords: Cyclocarya paliurus, nutritional composition, extraction methods, biological activities, functional applications
Citation: Shen Y, Peng Y, Zhu X, Li H, Zhang L, Kong F, Wang J and Yu D (2023) The phytochemicals and health benefits of Cyclocarya paliurus (Batalin) Iljinskaja. Front. Nutr. 10:1158158. doi: 10.3389/fnut.2023.1158158
Edited by:
Jie Zhang, Jilin University, ChinaReviewed by:
Xin Zhang, Ningbo University, ChinaJinyan Gong, Zhejiang University of Science and Technology, China
Copyright © 2023 Shen, Peng, Zhu, Li, Zhang, Kong, Wang and Yu. This is an open-access article distributed under the terms of the Creative Commons Attribution License (CC BY). The use, distribution or reproduction in other forums is permitted, provided the original author(s) and the copyright owner(s) are credited and that the original publication in this journal is cited, in accordance with accepted academic practice. No use, distribution or reproduction is permitted which does not comply with these terms.
*Correspondence: Jia Wang, ginie1000@foxmail.com; Di Yu, yudi1982@126.com
†These authors have contributed equally to this work and share first authorship