- 1Institute of Bast Fiber Crops, Chinese Academy of Agricultural Sciences, Changsha, China
- 2College of Life Sciences, Hunan Normal University, Changsha, China
- 3Hunan Engineering Laboratory for Good Agricultural Practice and Comprehensive Utilization of Famous-Region Medicinal Plants, College of Bioscience and Biotechnology of Hunan Agricultural University, Changsha, China
- 4Key Laboratory for Enzyme and Enzyme-Like Material Engineering of Heilongjiang, College of Life Science, Northeast Forestry University, Harbin, Heilongjiang, China
This study aimed to evaluate the effect of solid-state fermentation (SSF) with Aspergillus niger on the total phenolic content (TPC), the total flavonoid content (TFC), individual phenolic contents, and antioxidant and inhibitory activities against metabolic syndrome-associated enzymes in an ethanol extract from Apocynum venetum L. (AVL). TPC, TFC, and the contents of quercetin and kaempferol during SSF were 1.52-, 1.33-, 3.64-, and 2.22-fold higher than those of native AVL in the ethyl acetate (EA) subfraction of the ethanol extract. The ABTS·+, DPPH· scavenging, and inhibitory activities against α-glucosidase and pancreatic lipase were found to be highest in the EA subfraction. Fermentation significantly increased the ABTS radical cation, DPPH radical scavenging, and pancreatic lipase inhibitory activities by 1.33, 1.39, and 1.28 times, respectively. TPC showed a significantly positive correlation with antioxidant activities or inhibition against metabolic syndrome-associated enzymes. This study provides a theoretical basis for producing tea products with enhanced antioxidant, antidiabetic, and antihyperlipidemic activities.
1. Introduction
Apocynum venetum L. (AVL) has been used in traditional Chinese medicine and is widely grown in a saline–alkaline desert and on river banks (1). Due to the flavonoid-rich content in its leaves, it has the potential capacity to cure angiocardiopathies by lowering blood pressure, preventing hyperlipidemia, treating depression, and calming nerves (2–4). AVL-based medicines and tea beverages have attracted great interest in China, Japan, and the USA (2).
In previous studies, the main active fractions of AVL are phenolic acid, flavonoid (hyperoside and isoquercitrin), and flavan-3-ol components (5, 6). Phenolics exist in both soluble and insoluble-bound forms (7). Several previously published literature studies reported that the content of insoluble phenolic compounds in some agricultural by-products is listed in increasing order: 16% in pomegranate peel, 25% in pomegranate seed, 47% in chestnut shell, 53% in black carrot pomace, 57% in blueberry seed meal, 61% in blackberry seed meal, 63% in black raspberry seed meal, 63% in watermelon peel, 67% in melon peel, 68% in mango seed, 70% in pear peel, 79% in kiwi peel, 80% in orange peel, 82% in banana peel, and 88% in apple peel (8–11). Soluble phenolic compounds (PC) in plants can be extracted using organic solvents, such as ethanol, acetone, and ethyl acetate (EA) (12). However, insoluble phenolic components are bound to the cell structure via ester or glycosidic linkages and cannot be effectively extracted (11). This leads to low or limited utilization efficiency of these bioactive substances in materials (13). Thus, fermentation technologies can effectively improve their bioavailability. Nowadays, microbial fermentation with α-amylase, cellulase, β-glucosidase, and xylanase secreted by microorganisms can release phenolics (14). Monascus anka, Bacillus sp., and Aspergillus oryzae have been widely applied in oats (M. anka), black rice bran (A. oryzae), and guava leaves (M. anka and Bacillus sp.) on the release of PC and enhancement of bioactivities (15–17). Aspergillus niger (A. niger) enhanced the antioxidant activity of food or tartary buckwheat leaves (18, 19). Fermentation with A. niger was found to increase the total phenolic content (TPC) and antioxidant properties of oats (20).
Currently, few reports are available on the result of solid-state fermentation (SSF) in terms of polyphenolic composition, antioxidant properties, and inhibition activities against metabolic syndrome-associated enzymes in AVL. In this study, Monascus purpureus, A. oryzae, and A. niger were used to investigate their influence on TPC and the total flavonoid content (TFC). Then, further research was done to optimize the conditions of fermentation of the best strain. In an ethanol extract and its three subfractions [EA, petroleum ether (PE), and water], the composition of individual phenolics, TPC and TFC, and the antioxidant and inhibitory activities against metabolic syndrome-associated enzymes in fermented or native AVL leaves were also discussed. Moreover, the correlation between TPC and TFC with antioxidant and inhibitory activities against metabolic syndrome-associated enzymes was investigated. This study provides a theoretical foundation for AVL fermented tea products with a stronger bioactivity by increasing the content of phenolics, especially quercetin and kaempferol.
2. Materials and methods
2.1. Microorganisms
In this research, A. oryzae (CICC40934, China Center of Industrial Culture Collection), M. purpureus (bio-67015, the inquiry network for microbial strains in China), and A. niger (CGMCC5.0809, China General Microbiological Culture Collection Center) were used to ferment AVL leaves. Before the experiment, these fungal strains were incubated on potato dextrose agar (PDA) plates at 28°C for 7 days for later use. To collect spores from these strains, the PDA surface was rinsed with 0.9% NaCl to obtain a spore suspension, which was then stored at 4°C for further experiments.
2.2. Substrate and SSF
Apocynum venetum L. leaves (30%, w/w, air-dried) and seed suspensions of A. oryzae, M. purpureus, and A. niger (8 log CFU/ml, 30%, v/w) with a water content of 40% (w/w) were used as substrate media for SSF. Before fermentation, the sterilized AVL leaf was obtained via autoclaving at 121°C for 20 min. Each group was divided into three parts. TPC and TFC of AVL were monitored at 3, 6, 9, and 12 days during fermentation.
2.3. Preparation of extracts
Approximately 1 g of dried AVL leaves (40 meshes) were extracted using 70% ethanol (1:20, v/v) in a water bath at 50°C for 1 h. The residue was extracted three times. Supernatants were evaporated in a rotary evaporator (45°C) until dry; then, the dry matter was dissolved again in 20 ml of the supernatant with 70% ethanol (v/v) to acquire the ethanol fractions. As was described in Figure 1, it was then dissolved in water and extracted with 30 ml of PE, and 30 ml of EA using a liquid–liquid partition of 1:1 (v/v). The ethanol, PE, EA, and water extracts were redissolved in dimethyl sulfoxide (DMSO) to yield the different phenolic fractions.
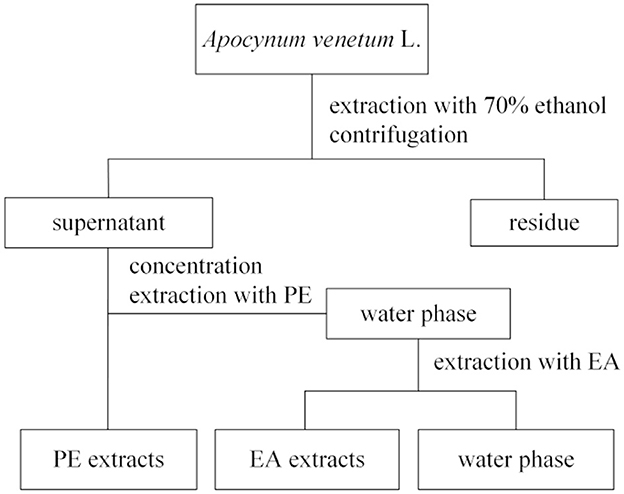
Figure 1. A procedure for ethanol extraction and separation of the petroleum ether (PE), ethyl acetate (EA), and water subfractions.
2.4. Determination of TPC and TFC
Samples (1 ml) were mixed with 1.5 ml of the Folin–Ciocalteu reagent at room temperature for ~3–8 min. Subsequently, 1 ml of Na2CO3 (20%, w/v) was added, and the total volume of 10 ml was filled with pure water. After incubation for 1 h, the optical density (OD) at 765 nm was measured. TPC was expressed as milligrams of gallic acid equivalents (GAE) per gram of dry weight (DW) or milliliters. These results were replicated at least three times with identical results.
The aluminum nitrate colormetric method was used to determine TFC, and rutin was used as standard. NaNO2 (1 ml, 5%, w/v) was added to samples (1 ml) and gently swirled for 5 min. Approximately 1 ml of Al(NO3)3 (10%, w/v) was added and maintained for 5 min, and 10 ml of NaOH (0.5 M) was added and allowed to react for 15 min. OD at 510 nm was measured. Experiments were performed three times. The TFC value was expressed as DW in milligrams of rutin equivalents (RTE) per gram or sample volume in milliliter.
2.5. Enzymatic assay
For the enzymatic assay, AVL fermented with A. niger was collected at different times (3, 6, 9, and 12 days). Wet weight substrate (3 g) was mixed with citric acid buffer (60 ml, pH 4.8) for the extraction of extracellular enzymes. The mixture was stirred in an ice bath at 120 revolutions per minute (rpm) for 6 h. The activities of carboxymethyl cellulase (CMCase), xylanase, and β-glucosidase were assayed, as mentioned in previous works (21, 22).
2.6. High-performance liquid chromatography analysis of EA subfractions
The samples were purified through a 0.22-μm syringe filter (Biosharp, China) for further high-performance liquid chromatography (HPLC). The components and concentration of PC in AVL were determined on an UltiMate™ 3000 RSLCnano System consisting of a Supersil AQ-C18 column (250 × 4.6 mm, 5 μm, Waters, China) as well as an RS variable wavelength detector (UltiMate™ 3000, DIONEX, USA). Acetonitrile solution and 0.2% phosphoric acid aqueous solution (v/v) were used as mobile phases A and B at a flow rate of 0.8 ml/min. The elution gradient concentration was set with 0–8 min, 5–12% solution A; 8–20 min, 12–18% solution A; 20–45 min, 18–22% solution A; 45–50 min, 22–35% solution A; and 50–60 min, 35–50% solution A. The other parameters of HPLC were operated: wavelength scanning detection (256 nm), a column temperature (35°C), and an injection volume (10 μl). The content of each standard in every group was calculated according to the standard curve (DW in mg/g). A standard curve was established using 100 μg/ml of chlorogenic acid, rutin, quercetin-3-O-galactoside, isoquercitrin, and kaempferol-3-O-glucose and 50 μg/ml of quercetin and kaempferol in methanol.
2.7. Assay of antioxidant activities and ferric reducing power
2.7.1. ABTS·+ scavenging activity
The stable ABTS·+ (1:1, v/v) was generated by mixing the solution of 2.6 mM K2S2O8 and 7.4 mM ABTS·+, which was then reacted with antioxidants in samples. ABTS·+ and K2S2O8 mixed solution was reacted in a dark place at 12 h and diluted with ethanol (95%, v/v). The working solution was diluted with ethanol (95%, v/v) until the absorbance reached ~0.7. The final reaction mixture was combined with samples (200 μl) and 800 μl of ABTS·+ working solution. The mixture was incubated at 25°C for 6 min. The reaction was detected at 734 nm. Ascorbic acid was used as a positive control. The scavenging capacity was calculated:
where A1 and A0 are the ODs of the test sample and blank, respectively.
2.7.2. DPPH· radical scavenging activity
Approximately 1 ml of samples and 1 ml of DPPH (0.2 mM) were mixed and maintained at 25°C for 10 min under darkness. Absorbance at 517 nm was measured. Ascorbic acid served as the positive control. The scavenging activity was calculated as follows:
where A0, A1, and A2 are the OD values of the blank control, the test sample, and the sample control, respectively.
2.7.3. Hydroxyl free radical (·OH) scavenging activity
Samples were mixed with FeSO4 (6 mM) and H2O2 (6 mM) solution (2 ml, 1:1:1, v/v/v) at room temperature for 10 min. Then, 2 ml of salicylic acid was added, and the absorbance at 510 nm was measured. Ascorbic acid served as a positive control. Percentage (%) inhibition of OH was used to express the results according to the following equation:
where A1 and A0 are the ODs of the reacted mixture with the test sample and blank control.
2.7.4. Ferric reducing power
Approximately 1 ml of samples, 2.5 ml of polybutylene succinate (PBS) (0.2 M, pH 6.6), and 2.5 ml of K3[Fe(CN)6] (1%, m/v) were mixed in a sequence. After incubation for 20 min at 50°C, 1 ml of C2HCl3O2 (10%, m/v) was added and mixed. Then, 2.5 ml of the mentioned reaction mixture, 2.5 ml of pure water, and 0.5 ml of FeCl3 (1%, m/v) were mixed and kept for 30 min. Absorbance read at 700 nm was expressed as the ferric reducing power.
2.8. Inhibitory activity against metabolic syndrome-associated enzymes
2.8.1. α-glucosidase inhibitory assay
Polybutylene succinate (25 mM, pH 6.9) was used to dissolve samples to 100 μg/ml. Approximately 0.2 ml of samples and 1 ml of α-glucosidase (1 × 10−3 mg/ml) were mixed and incubated at 37°C for 10 min. Then, 0.5 ml of p-nitrophenyl-α-D-glucopyranoside (p-NPG, 5 mM) was used to initiate the reaction for 10 min. Approximately 1 ml of Na2CO3 (0.1 M) was mixed to stop the reaction. The absorbance was measured at 405 nm. Samples were assayed three times. Acarbose served as the positive control. The inhibition ratio of α-glucosidase was calculated as follows:
where A4, A3, A2, and A1 are the ODs of the tested group, the blank tested group, the control group, and the blank control group, respectively.
2.8.2. Porcine pancreatic lipase inhibitory assay
Pure water was used to distill porcine pancreatic lipase type II to 5 mg/ml enzyme suspension. The supernatant was centrifuged at 6,000 × g at 4°C for 10 min, and it was recovered for further use. Pancreatic lipase solution, PBS (pH 7.4), and diluted samples (50 μl, 1:1:1, v/v/v) were blended and incubated at 37°C for 10 min. Then, 50 μl of p-nitrophenyl palmitate (p-NPB, 11.2 mM) was added at 37°C for 20 min, and the absorbance at 405 nm was measured. Samples were assayed in three duplicates. Orlistat served as the reference compound. The calculation formula is as follows:
where A4, A3, A2, and A1 represent the ODs of the tested group, blank tested group, control group, and blank control group, respectively.
2.9. Statistical analysis
In this study, all data were reported as the mean ± standard deviation (SD) of three replications. One-way analysis of variance (ANOVA) was used to determine the difference analysis between values, and a p-value of < 0.05 was considered statistically significant. Correlation analysis was performed using a two-tailed Pearson's correlation test of GraphPad Prism version 8.0 software package.
3. Results
3.1. TPC and TFC of AVL fermented with A. oryzae, M. purpureus, and A. niger
The key step for the fermentation of AVL is to select suitable microorganisms. The effect of different fungi on fermentation was investigated by measuring TPC and TFC. In the present study, AVL was fermented with A. oryzae, M. purpureus, and A. niger for 6 days. Table 1 shows the observation of the highest TPC (1.67 mg GAE/ml) and TFC (0.44 mg RTE/ml) during fermentation with A. niger. Thus, A. niger was selected as the optimal fermentation fungus for subsequent experiments.
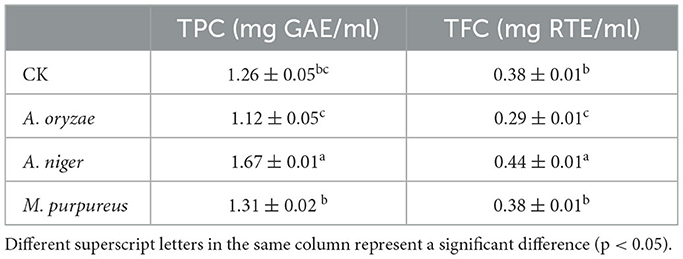
Table 1. Changes in the total phenolic content (TPC) and total flavonoid content (TFC) after fermentation of different strains.
3.2. Determination of the optimal fermentation time of AVL with A. niger
To evaluate the optimal fermentation time, AVL was fermented with A. niger in four different stages (3, 6, 9, and 12 days). Table 2 shows a gradual increase in TPC and TFC with the extension of time before 9 days. On the 6th day, TPC and TFC of the A. niger treatment were 1.47 mg GAE/ml and 0.46 mg RTE/ml, respectively. Over 6 days, TPC and TFC were slightly decreased. Thus, the 6th day was the optimal fermentation period.
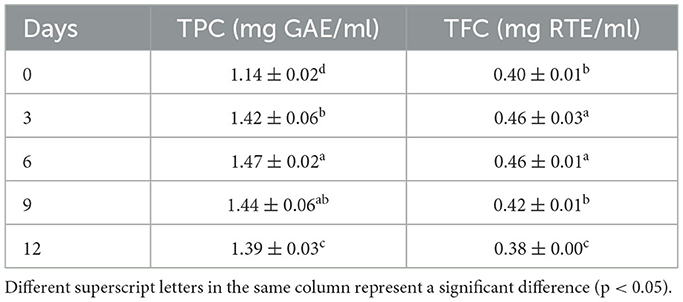
Table 2. Changes in TPC and TFC of Apocynum venetum L. (AVL) fermented with Aspergillus niger for 0, 3, 6, 9, and 12 days.
3.3. CMCase, xylanase, and β-glucosidase activities of A. niger during the fermentation of AVL
To elucidate the relationship between enzyme activities produced by A. niger and bioactivity release of AVL during fermentation, we tracked the enzymatic activities of CMCase, xylanase, and β-glucosidase produced by A. niger during the fermentation process. Table 3 shows that CMCase (0.05 U/ml), xylanase (0.07 U/ml), and β-glucosidase (0.05 U/ml) activities were relatively low on the 3rd day of fermentation. On the 6th day of fermentation, the activities of CMCase and xylanase were significantly increased, and the activity of xylanase (0.21 U/ml) was relatively higher than CMCase (0.18 U/ml). On the 9th day of fermentation, the activity of xylanase (0.19 U/ml) had a slight decline, and the activity of CMCase (0.08 U/ml) was remarkably decreased. The β-glucosidase activity of A. niger was lower during the entire incubation period (0.05 U/ml at 3 days, 0.06 U/ml at 6 days, 0.08 U/ml at 9 days, and 0.05 U/ml at 12 days) as compared to CMCase and xylanase.
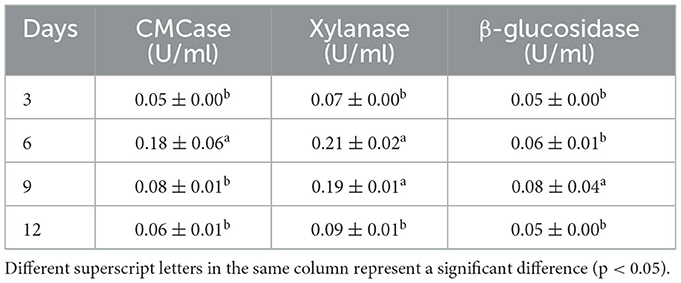
Table 3. Carboxymethyl cellulase (CMCase), xylanase, and β-glucosidase activities of A. niger during the AVL fermentation process at 3, 6, 9, and 12 days.
3.4. TPC and TFC of the ethanol extract, PE, EA, and water subfraction of native (unfermented) and fermented AVL
The total phenolic content of ethanol extract, PE, EA, and water subfractions from native AVL is shown in Figure 2A. The EA subfraction showed higher TPC (206.84 mg GAE/g DM) than the water subfraction (87.41 mg GAE/g DM), and the PE subfraction had the lowest value (22.71 mg GAE/g DM). It was shown that fermentation significantly increased TPC in ethanol extract, EA, and water subfractions of AVL (Figure 2A). The EA subfraction of AVL fermented with A. niger (314.58 mg GAE/g DM) showed a more significant increase in TPC than that of native AVL (206.84 mg GAE/g DM). Only TPC of PE subfractions did not show any statistically significant differences between fermented and native AVL.
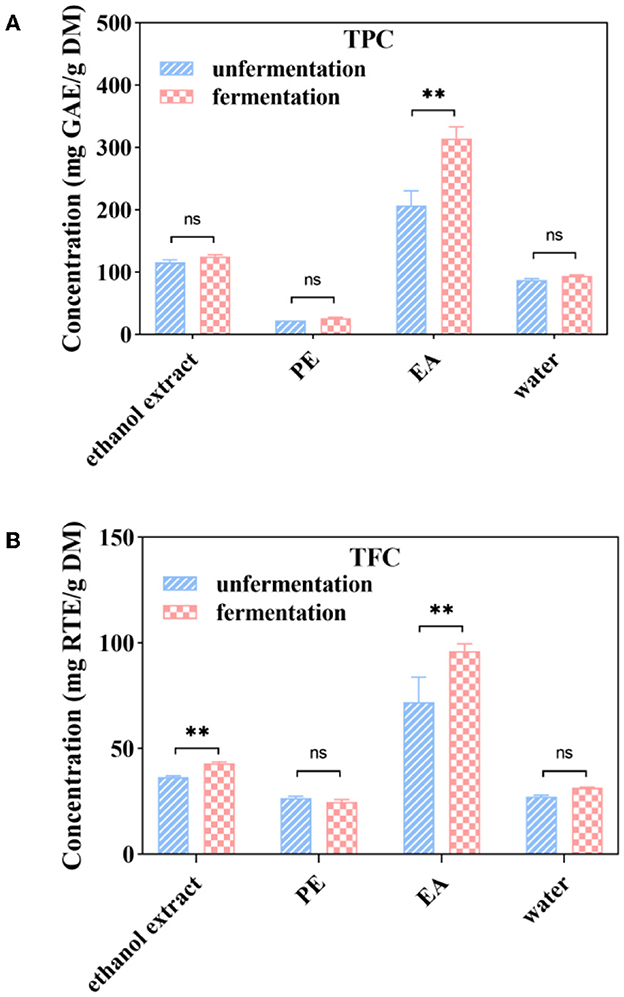
Figure 2. Soluble phenolic contents (A), soluble flavonoid contents (B) and soluble phenolic extracts at different subfractions from Apocynum venetum L. (AVL) fermented with A. niger. ** P < 0.05.
As shown in Figure 2B, the EA subfraction of native AVL showed the highest TFC of 71.93 mg RTE/g DM, followed by the water subfraction of 27.13 mg RTE/g DM, while TFC was not detected in PE subfractions (Figure 2). Compared with native AVL, TFC in the EA subfractions fermented with A. niger showed a remarkable increase of 33.49%. TFC in the water subfraction of native and fermented AVL showed no significant differences. In addition, TFC was not detected in PE subfractions of fermented AVL.
3.5. Comparison of antioxidant activities of ethanol extract, PE, EA, and water subfractions of native and fermented AVL
In this study, DPPH·, ABTS·+, hydroxyl radical scavenging activity, and ferric reducing power were used to measure the total antioxidant activities of ethanol extract, PE, EA, and water subfractions from native and fermented AVL.
3.5.1. ABTS·+ radical scavenging activity
The scavenging ratio of the different subfractions from native and fermented AVL is shown in Figure 3A. Among the subfractions of unfermented AVL, the EA subfraction showed the highest ABTS·+ radical scavenging activity (87.81% with 100 μg/ml), followed by the water subfraction (24.44% with 100 μg/ml). The PE subfraction had the lowest ABTS·+ radical scavenging activity (5.28% with 100 μg/ml). Compared with a native AVL extract, the ABTS·+ radical scavenging ratio of the ethanol extract remarkably increased by 24.53% after A. niger fermentation, but the scavenging activity of EA subfractions showed no significant difference compared with the values of their native AVL.
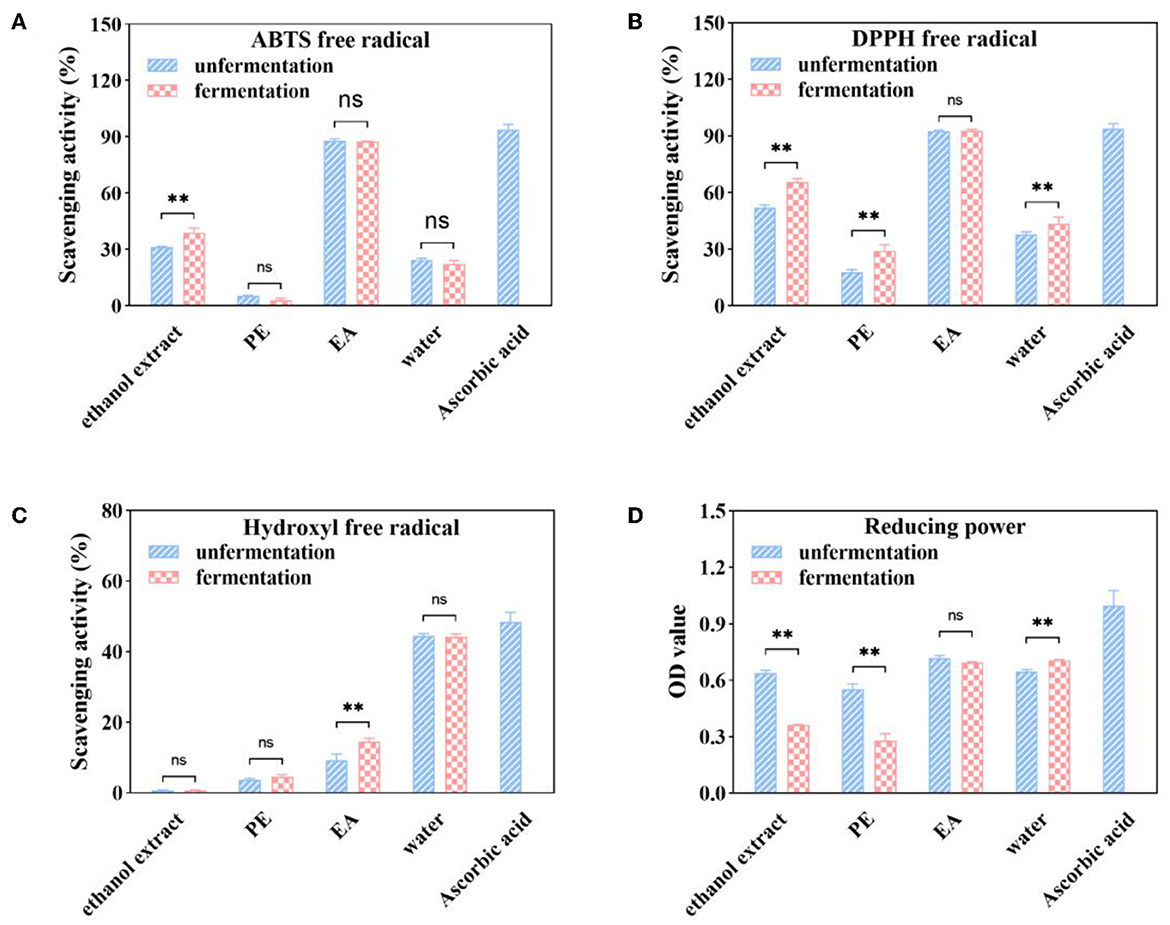
Figure 3. ABTS·+ (A), DPPH (B), hydroxyl (C), and free radical scavenging activities and reducing power (D) of soluble phenolic extracts at different subfractions from AVL fermented with A. niger (100 μg/ml of ascorbic acid as a positive control). ** P < 0.05.
3.5.2. DPPH· radical scavenging activity
As shown in Figure 3B, the scavenging activity of DPPH· radical in untreated AVL was as follows: EA (92.67%) > water (37.88%) > PE (17.89%), which was consistent with TPC and ABTS·+ radical scavenging activity. Fermentation with A. niger was also found to have increased the DPPH·radical scavenging ratio in all of these four subfractions. The DPPH·radical scavenging ratio of the PE subfraction was improved by 63.17% over that of the untreated group. However, EA subfractions fermented with A. niger showed no differences with native AVL.
3.5.3. Hydroxyl free radical (·OH) scavenging activity
As shown in Figure 3C, the water subfraction in untreated AVL had the highest (OH) scavenging activity (44.53% at a sample concentration of 1 mg/ml), followed by the (OH) scavenging activity of the EA subfraction (9.28%). The PE subfraction had the lowest value (3.76%). However, the TPC of water subfraction was lower than that of the EA subfraction (Figure 2A). It is indicated that·OH scavenging activity may not be related to the content of polyphenol. However, compared with native AVL, the·OH scavenging activity in EA subfractions was also increased by 56.27% after fermentation (Figure 3C).
3.5.4. Ferric reducing power
Figure 3D shows that the EA subfraction has the best ferric-reducing activity (OD700 value = 0.6501 ± 0.012) followed by water subfraction (OD700 value = 0.6031 ± 0.011). In addition, we noted that ferric-reducing power is very low for the petroleum ether extract, which is the least polar extract (OD700 value = 0.5412 ± 0.018). The ethanol extract and PE subfraction of AVL have a reducing activity of ferric reducing power with a lower activity for fermentation with A. niger in comparison with a native group. However, the EA and water subfractions have no statistically significant differences compared to the fermented group in ferric-reducing power activity (p < 0.05).
3.6. Inhibitory activities of metabolic syndrome-associated enzymes
As shown in Figure 4A, inhibitory activities of α-glucosidase enzyme were 55.45, 97.48, and 61.27% for the PE, EA, and water subfractions at a sample concentration of 1 mg/ml, respectively. Inhibitory activities of PE and water subfractions were significantly increased by 29.64 and 16.00% after fermentation with A. niger. However, the inhibition of α-glucosidase was not significantly changed after fermentation in the EA subfraction.
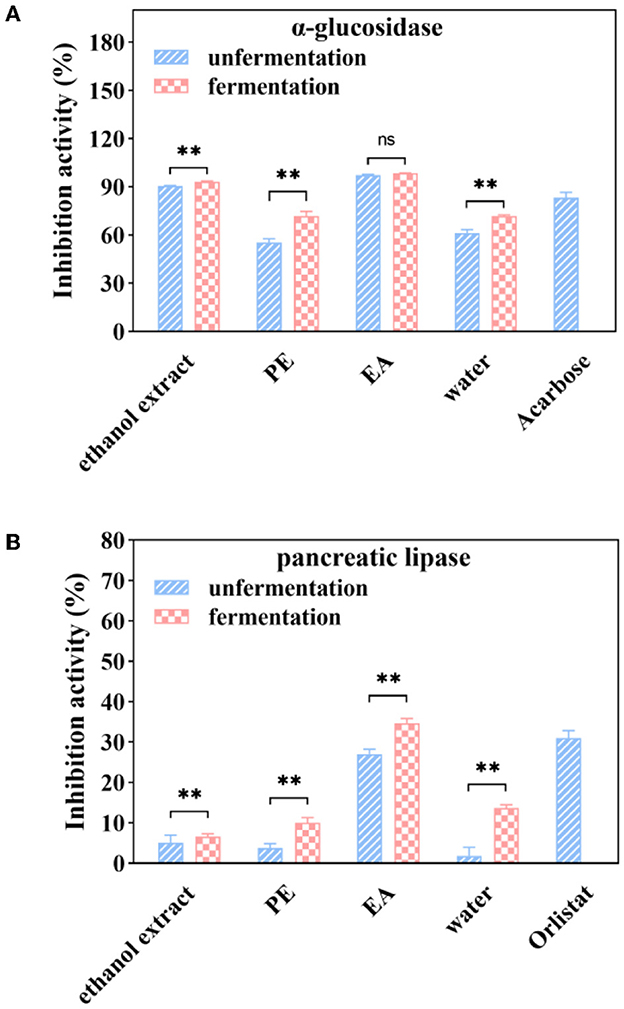
Figure 4. α-glucosidase (A) and pancreatic lipase (B) inhibition activities of soluble phenolic extracts at different subfractions from AVL fermented with A. niger (100 μg/ml of acarbose and 1 mg/ml of orlistat as positive controls). ** P < 0.05.
Further experiment was performed to study the effect of fermentation on pancreatic lipase inhibition efficacy. It was shown that PE, EA, and water subfractions of pancreatic lipase inhibition activities were 4, 26, and 2%, respectively, at a sample concentration of 1 mg/ml (Figure 4B). Fermentation with A. niger enhanced the inhibitory efficiency of all subfractions on the inhibitory activities against porcine pancreatic lipase. After 6 days of fermentation, a significant increase (28%) in the inhibitory activities against pancreatic lipase was observed in the EA subfraction.
3.7. Changes in soluble phenolics in the EA subfraction of AVL fermented with A. niger
The effect of AVL fermented with A. niger on soluble phenolics was investigated. HPLC analyses were applied to the EA fraction, as this fraction had a higher TPC, TFC, DPPH, and ABTS·+ radical scavenging activity and ferric reducing power when compared to the ethanol, PE, and water subfractions. Quantities of seven compounds, including chlorogenic acid, rutin, quercetin-3-O-galactoside, isoquercitrin, kaempferol, quercetin, and kaempferol-3-O-glucose, were measured (Table 4; Figure 5). According to HPLC analyses, the composition of soluble phenolics was similar after A. niger fermentation, but their contents were significantly different. The soluble contents of most of the phenolics from AVL extracts were slightly increased due to fermentation. As shown in Figures 5A–C and Table 4, increases in phenolic components after fermentation were as follows: chlorogenic acid, 18%; rutin, 28%; quercetin-3-O-galactoside, 101%; isoquercitrin, 17%; kaempferol-3-O-glucose, 10%; quercetin 264%; and kaempferol 122%.
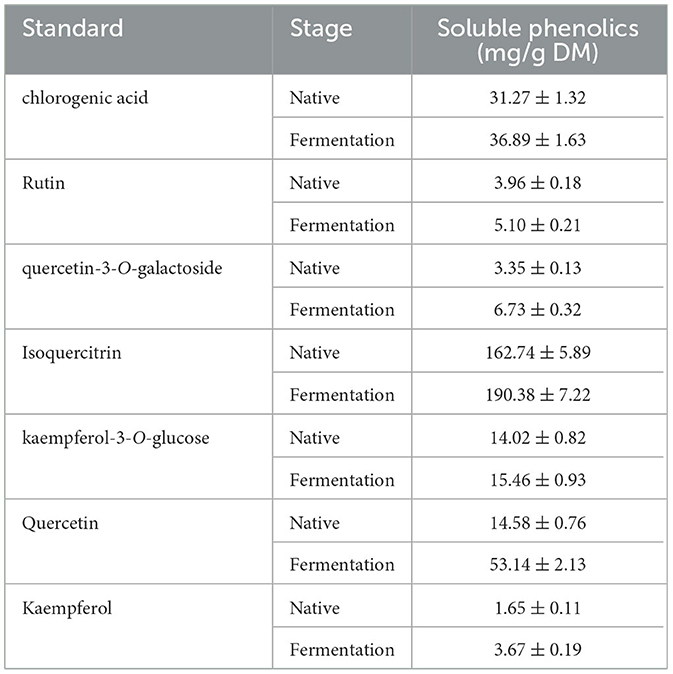
Table 4. Changes in individuals of soluble phenolics in the EA subfraction of AVL fermented with A. niger.
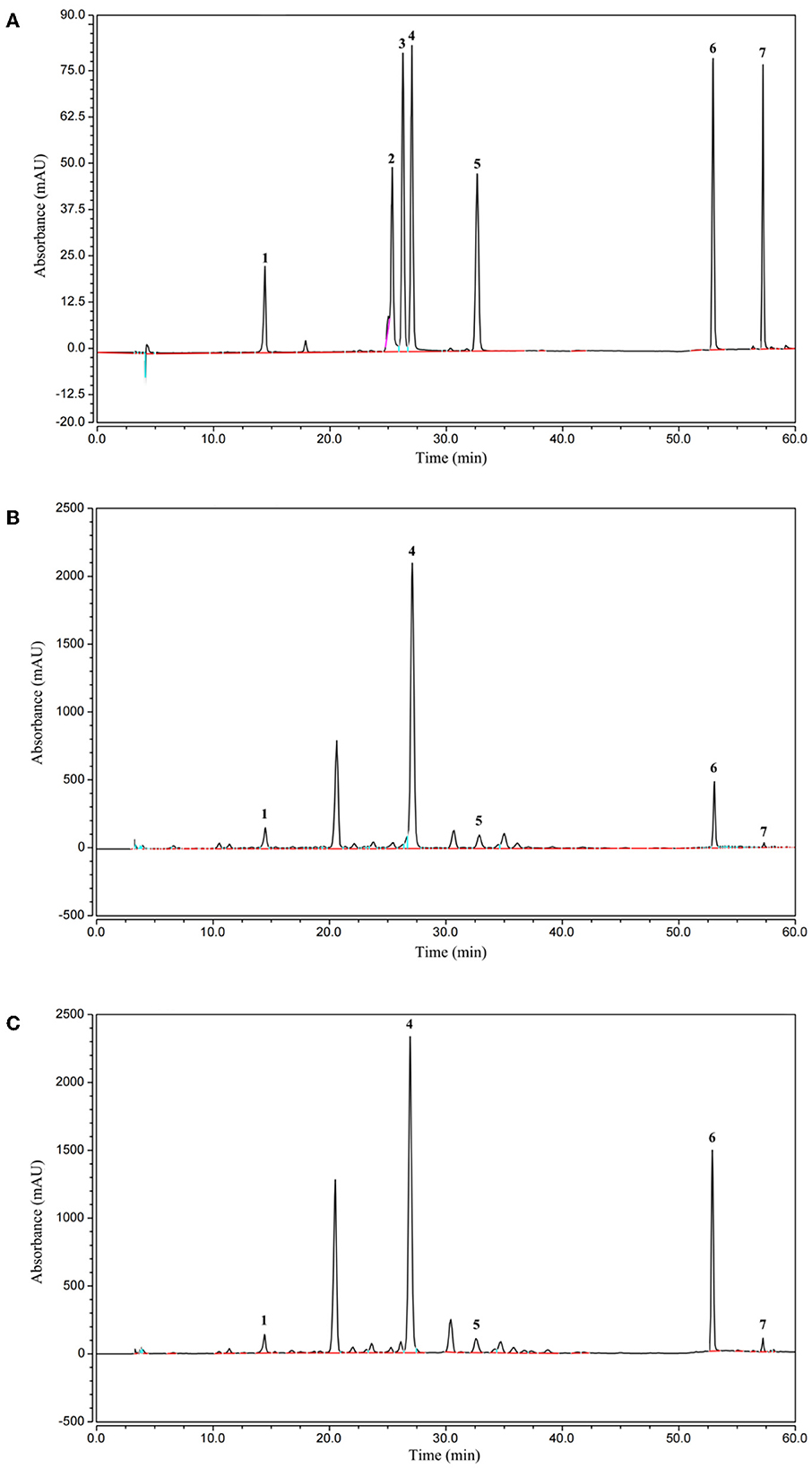
Figure 5. High-performance liquid chromatography (HPLC) chromatogram of the standard compounds (A), native (B), and fermented phenolics (C) in the EA subfraction of AVL, Peaks: 1, Chlorogenic acid; 2, Rutin; 3, quercetin-3-O-galactoside; 4, Isoquercitrin; 5, kaempferol-3-O-glucose; 6, Quercetin; 7, Kaempferol.
3.8. Correlation analysis
A Pearson's test was carried out to evaluate correlations between TPC and TFC as well as bioactivities in the AVL extract. As shown in the correlation analysis results (Table 5), DPPH· and ABTS·+ scavenging activities were significantly positively correlated with TPC (R2 = 0.8926, p < 0.05 and R2 = 0.8612, p < 0.05) and TFC (R2 = 0.8175, p < 0.05 and R2 = 0.8778, p < 0.05). However, Table 4 displays no correlation between OH scavenging activity and TPC (R2 = 0.0003, p = 0.92) or TFC (R2 = 0.0245, p = 0.39). Positive correlations were observed between α-glucosidase inhibitory activity and TPC (R2 = 0.6181, p < 0.05) or TFC (R2 = 0.5750, p < 0.05). The pancreatic lipase inhibitory activity showed a stronger positive relationship with TFC (R2 = 0.8249, p < 0.05) than with TPC (R2 = 0.7334, p < 0.05).
Correlations between antioxidant activities and inhibitory activities against metabolic syndrome-associated enzymes in an AVL extract are presented in Table 6. The results indicated a positive correlation between antioxidant activities (DPPH· and ABTS·+ scavenging activity) and α-glucosidase (R2 = 0.8093, p < 0.05 and R2 = 0.6503, p < 0.05) and pancreatic lipase inhibitory activities (R2 = 0.6621, p < 0.05 and R2 = 0.7214, p < 0.05). The results also demonstrated no significantly positive correlation between antioxidant activities (OH scavenging activity and ferric reducing power) and inhibitory activities against metabolic syndrome-associated enzymes.
4. Discussion
Fermentation with filamentous fungi has been widely reported for the release of diverse polyphenolic compounds. A. oryzae, M. purpureus, and A. niger were recognized as safe fungi and employed in the preparation of foods (17, 20, 23). For example, Zhang et al. (19) used A. niger CJ-1 to ferment TBL. In the EA subfraction of A. oryzae fermented oats, caffeic and ferulic acids were increased by 2.7- and 5.5-fold, respectively, and chlorogenic and p-Coumaric acids also increased about two times (20). Fermentation with M. anka GIM 3.592 and S. cerevisiae GIM 2.139 enhanced the release of chlorogenic acid, rutin, and quercetin in guava leaves (23).
Based on the limitation of the type of enzymes secreted by microorganisms and the complexity of plant cell structures, suitable microorganisms should be selected for the fermentation of AVL. In the present study, A. oryzae, M. purpureus, and A. niger were applied in the fermentation of AVL leaves. Fermentation with A. niger showed a higher level of TPC than that with M. purpureus, while TPC fermented with A. oryzae had a lower value than that fermented with A. niger and M. purpureus. The data showed that an increase in TPC was related to the types of fungi. However, the biotransformation and degradation mechanism of polyphenol components of AVL fermented with A. niger has not been sufficiently clarified.
In the present study, one polyphenol conversion pathway was found by analyzing the differential metabolites in AVL fermented with A. niger. A. niger can induce β-glucosidase to hydrolyze 3-glycosidic linkage in isoquercitrin and kaempferol-3-O-glucoside, liberating quercetin and kaempferol. Compared with untreated AVL, the contents of quercetin and kaempferol were increased 3.64 and 2.22 times, respectively. In addition, after fermentation with A. niger, the contents of chlorogenic acid, rutin, quercetin-3-O-galactoside, isoquercitrin, kaempferol-3-O-glucoside, quercetin, and kaempferol were significantly increased due to fermentation with A. niger, which might attribute to the conversion of conjugated PC into a free polyphenol by A. niger. This microorganism could produce cellulase and xylanase to release PC conjugated with xylan, cellulose, or lignin (20, 24–26). Previous studies showed that there was a positive correlation between cellulase or xylanase activities and TPC (27–29).
It was reported that the major bioactive components of AVL mainly consisted of phenolic acids (chlorogenic acid and caffeic acid) and flavonoids (rutin, hyperin, isoquercitrin, quercetin, kaempferol quercetin-3-O-β-D-xylopyranoside, quercetin-3-O-α-L-arabinoside, and kaempferol-3-O-glucoside) (5, 30, 31). Phenolics are important native antioxidants in AVL. The present study showed that fermentation with A. niger significantly increased the DPPH·, ABTS·+, and OH radical scavenging activities of AVL. Meanwhile, fermentation with A. niger can greatly convert flavonoids (isoquercitrin and kaempferol-3-O-glucoside) into quercitrin and kaempferol. Previous reports confirmed that aglycone exhibited much higher antioxidant capacity than its glycoside derivatives (32, 33). For example, aglycone corresponding to quercetin showed a higher antioxidant activity than rutin (32). Compared with those lacking neutralizing free radicals, quercetin contained more hydroxyl groups and has a comparatively greater antioxidant potential (33). Hence, fermentation with A. niger cannot only further enhance the release of soluble phenolics from AVL but also transform glycoside compounds into aglycones with stronger antioxidant capacity.
In addition to antioxidant activities, AVL has been reported to have antihypertensive effects, cholesterol-lowering activity, and antidiabetic activity (2). In the present study, fermentation with A. niger showed stronger inhibition activities against α-glucosidase and pancreatic lipase than native AVL. Phenolic compounds were shown to perform in vitro inhibition activities against metabolic syndrome-associated enzymes like lipases, α-amylase, and α-glucosidase (34). The specific structural features of polyphenols had an effect on their inhibitory activities against these enzymes (35). For example, flavonoids had more hydroxyl groups than phenolic acids, and they showed higher inhibitory activities compared with phenolic acids (36). As mentioned earlier, increased contents of soluble phenolics, quercetin, and kaempferol in AVL fermented with A. niger could enhance their inhibitory activities against metabolic syndrome-associated enzymes.
5. Conclusion
A. niger, M. purpureus, and A. oryzae were used in the fermentation of AVL. AVL fermented with A. niger not only releases more free phenolics but also converts glycoside derivatives into aglycone. Compared with native AVL, fermentation with A. niger significantly increases free phenolics (particularly, quercetin and kaempferol). Among phenolics, isoquercitrin showed the highest level. Additionally, ethanol extracts from AVL following fermentation exhibited a much higher antioxidant activity and inhibitory efficacy toward α-glucosidase and pancreatic lipase. The present study provided guidance for obtaining tea processing methods with higher antioxidant, antidiabetic, and antihyperlipidemic activities.
Data availability statement
The raw data supporting the conclusions of this article will be made available by the authors, without undue reservation.
Author contributions
CC and DL: data curation, writing—original draft, and writing—review and editing. CX: software, data curation, and critical content review. YZ, WG, and ZZ: visualization and investigation. NL and YW: software and validation. CL, LY, and ZH: conceptualization, methodology, and software. CX and YP: supervision. All authors contributed to the article and approved the submitted version.
Funding
This work was supported by a grant from the Young Scientists of Hunan Province (2022RC1151), the China Agriculture Research System for Bast and Leaf Fiber Crops (CARS-16-E25), the Training Program for Excellent Young Innovators of Changsha (KQ2106095), and the Agricultural Science and Technology Innovation Program of China (ASTIP-IBFC-06).
Conflict of interest
The authors declare that the research was conducted in the absence of any commercial or financial relationships that could be construed as a potential conflict of interest.
Publisher's note
All claims expressed in this article are solely those of the authors and do not necessarily represent those of their affiliated organizations, or those of the publisher, the editors and the reviewers. Any product that may be evaluated in this article, or claim that may be made by its manufacturer, is not guaranteed or endorsed by the publisher.
References
1. Xu Z, Zhou J, Zhang C, Li Y. Review of current research and utilization status of Apocynum venetum germplasm in China. Chin Bull Bot. (2018) 53:382–90. doi: 10.11983/CBB1802
2. Xie W, Zhang X, Wang T, Hu J. Botany, traditional uses, phytochemistry and pharmacology of Apocynum venetum L. (Luobuma): a review. J Ethnopharmacol. (2012) 141:1–8. doi: 10.1016/j.jep.2012.02.003
3. Zhang Y, Liu C, Zhang Z, Wang J, Wu G, Li S. Comprehensive separation and identification of chemical constituents from Apocynum venetum leaves by high-performance counter-current chromatography and high performance liquid chromatography coupled with mass spectrometry. J Chromatogr B. (2010) 878:3149–55. doi: 10.1016/j.jchromb.2010.09.027
4. Li C, Huang G, Tan F, Zhou X, Mu J, Zhao X. In vitro analysis of antioxidant, anticancer, and bioactive components of Apocynum venetum tea extracts. J Food Quality. (2019) 1–13. doi: 10.1155/2019/2465341
5. An H, Wang H, Lan Y, Hashi Y, Chen S. Simultaneous qualitative and quantitative analysis of phenolic acids and flavonoids for the quality control of Apocynum venetum L. Leaves by Hplc-Dad-Esi-It-Tof-Ms and Hplc-Dad. J Pharm Biomed Anal. (2013) 85:295–304. doi: 10.1016/j.jpba.2013.07.005
6. Shen J, Yang K, Jiang C, Ma XQ, Zheng MX, Sun CH. Development and application of a rapid hplc method for simultaneous determination of hyperoside, isoquercitrin and Eleutheroside E in Apocynum venetum L. and Eleutherococcus senticosus. BMC Chem. (2020) 14:35. doi: 10.1186/s13065-020-00687-1
7. Zhang B, Zhang Y, Li H, Deng Z, Tsao R. A review on insoluble-bound phenolics in plant-based food matrix and their contribution to human health with future perspectives. Trends Food Sci Technol. (2020) 105:347–62. doi: 10.1016/j.tifs.2020.09.029
8. Torres-Leon C, Ramirez-Guzman N, Ascacio-Valdes J, Serna-Cock L, dos Santos Correia MT, Contreras-Esquivel JC, et al. Solid-state fermentation with Aspergillus niger to enhance the phenolic contents and antioxidative activity of mexican mango seed: a promising source of natural antioxidants. Lwt-Food Science and Technology. (2019) 112:108236. doi: 10.1016/j.lwt.2019.06.003
9. Perez-Jimenez J, Saura-Calixto F. Fruit peels as sources of non-extractable polyphenols or macromolecular antioxidants: analysis and nutritional implications. Food Res Int. (2018) 111:148–52. doi: 10.1016/j.foodres.2018.05.023
10. Gulsunoglu Z, Karbancioglu-Guler F, Raes K, Kilic-Akyilmaz M. Soluble and insoluble-bound phenolics and antioxidant activity of various industrial plant wastes. Int J Food Prop. (2019) 22:1501–10. doi: 10.1080/10942912.2019.1656233
11. Ayoub M, de Camargo AC, Shahidi F. Antioxidants and bioactivities of free, esterified and insoluble-bound phenolics from berry seed meals. Food Chem. (2016) 197:221–32. doi: 10.1016/j.foodchem.2015.10.107
12. Wu S, Shen D, Wang R, Li Q, Mo R, Zheng Y, et al. Phenolic profiles and antioxidant activities of free, esterified and bound phenolic compounds in Walnut kernel. Food Chem. (2021) 350:129217. doi: 10.1016/j.foodchem.2021.129217
13. Wang C, Li P, Zhang B, Yu X, Li X, Han G, et al. Combining transcriptomics and polyphenol profiling to provide insights into phenolics transformation of the fermented Chinese jujube. Foods. (2022) 11:2546. doi: 10.3390/foods11172546
14. Bei Q, Chen G, Lu F, Wu S. Wu Z. Enzymatic action mechanism of phenolic mobilization in oats (Avena sativa L) during solid-state fermentation with Monascus anka. Food Chem. (2018) 245:297–304. doi: 10.1016/j.foodchem.2017.10.086
15. Shin HY, Kim SM, Lee JH, Lim ST. Solid-state fermentation of black rice bran with Aspergillus awamori and Aspergillus oryzae: effects on phenolic acid composition and antioxidant activity of bran extracts. Food Chem. (2019) 272:235–41. doi: 10.1016/j.foodchem.2018.07.174
16. Wang L, Luo Y, Wu Y, Wu Z. Impact of fermentation degree on phenolic compositions and bioactivities during the fermentation of guava leaves with Monascus anka and Bacillus sp. J Funct Foods. (2018) 41:183–90. doi: 10.1016/j.jff.2017.12.044
17. Bei Q, Liu Y, Wang L, Chen G, Wu Z. Improving free, conjugated, and bound phenolic fractions in fermented oats (Avena sativa l) with Monascus anka and their antioxidant activity. J Funct Foods. (2017) 32:185–94. doi: 10.1016/j.jff.2017.02.028
18. Dulf FV, Vodnar DC, Dulf E-H, Tosa MI. Total phenolic contents, antioxidant activities, and lipid fractions from berry pomaces obtained by solid-state fermentation of two Sambucus species with Aspergillus niger. J Agr Food Chem. (2015) 63:3489–500. doi: 10.1021/acs.jafc.5b00520
19. Zhang X-Y, Chen J, Li X-L, Yi K, Ye Y, Liu G, et al. Dynamic changes in antioxidant activity and biochemical composition of tartary buckwheat leaves during Aspergillus niger fermentation. J Funct Foods. (2017) 32:375–81. doi: 10.1016/j.jff.2017.03.022
20. Cai S, Wang O, Wu W, Zhu S, Zhou F, Ji B, et al. Comparative study of the effects of solid-state fermentation with three filamentous fungi on the total phenolics content (tpc), flavonoids, and antioxidant activities of subfractions from oats (Avena sativa L). J Agr Food Chem. (2012) 60:507–13. doi: 10.1021/jf204163a
21. Azadian F, Badoei-dalfard A, Namaki-Shoushtari A, Hassanshahian M. Purification and biochemical properties of a thermostable, haloalkaline cellulase from bacillus licheniformis amf-07 and its application for hydrolysis of different cellulosic substrates to bioethanol production. Mol Biol Res Commun. (2016) 5:143–55.
22. Shi X, Xie J, Liao S, Wu T, Zhao LG, Ding G, et al. High-level expression of recombinant thermostable β-glucosidase in Escherichia coli by regulating acetic acid. Bioresour Technol. (2017) 241:795–801. doi: 10.1016/j.biortech.2017.05.105
23. Wang L, Bei Q, Wu Y, Liao W, Wu Z. Characterization of soluble and insoluble-bound polyphenols from Psidium guajava l. leaves co-fermented with Monascus anka and Bacillus sp and their bio-activities. J Funct Foods. (2017) 32:149–59. doi: 10.1016/j.jff.2017.02.029
24. Cavka A, Alriksson B, Rose SH, van Zyl WH, Jonsson LJ. Production of cellulosic ethanol and enzyme from waste fiber sludge using ssf, recycling of hydrolytic enzymes and yeast, and recombinant cellulase-producing Aspergillus niger. J Ind Microbiol Biot. (2014) 41:1191–200. doi: 10.1007/s10295-014-1457-9
25. Lubbers RJM, de Vries RP. Production of protocatechuic acid from p-hydroxyphenyl (h) units and related aromatic compounds using an Aspergillus niger cell factory. Mbio. (2021) 12:e0039121. doi: 10.1128/mBio.00391-21
26. Tang PL, Hassan O. Bioconversion of ferulic acid attained from pineapple peels and pineapple crown leaves into vanillic acid and vanillin by Aspergillus niger I-1472. BMC Chem. (2020) 14:7. doi: 10.1186/s13065-020-0663-y
27. Chen Y, Wang Y, Chen J, Tang H, Wang C, Li Z, et al. Bioprocessing of soybeans (glycine max l) by solid-state fermentation with Eurotium cristatum yl-1 improves total phenolic content, isoflavone aglycones, and antioxidant activity. Rsc Adv. (2020) 10:16928–41. doi: 10.1039/C9RA10344A
28. Zhu Y-F, Chen J-J, Ji X-M, Hu X, Ling T-J, Zhang Z-Z, et al. Changes of major tea polyphenols and production of four new b-ring fission metabolites of catechins from post-fermented jing-wei fu brick tea. Food Chem. (2015) 170:110–7. doi: 10.1016/j.foodchem.2014.08.075
29. Rocchetti G, Bhumireddy SR, Giuberti G, Mandal R, Lucini L, Wishart DS. Edible nuts deliver polyphenols and their transformation products to the large intestine: an in vitro fermentation model combining targeted/untargeted metabolomics. Food Res Int. (2019) 116:786–94. doi: 10.1016/j.foodres.2018.09.012
30. Li Z, Wang C, Zhang S, Wang J, Zhang X, He Q. Effect of total flavonoids in Apocynum venetum leaves on rat hypertension induced by high fat and high salt and its molecular mechanism. Chin Tradit Herb Drugs. (2012) 43:540–5. doi: 10.7501/j.issn.0253-2670
31. Gao G, Chen P, Chen J, Chen K, Wang X, Abubakar AS, et al. Genomic survey, transcriptome, and metabolome analysis of Apocynum venetum and Apocynum hendersonii to reveal major flavonoid biosynthesis pathways. Metabolites. (2019) 9:296. doi: 10.3390/metabo9120296
32. Erlund I, Kosonen T, Alfthan G, Mäenpää J, Perttunen K, Kenraali J, et al. Pharmacokinetics of quercetin from quercetin aglycone and rutin in healthy volunteers. Eur J Clin Pharmacol. (2000) 56:545–53. doi: 10.1007/s002280000197
33. Lesjak M, Beara I, Simin N, Pintac D, Majkic T, Bekvalac K, et al. Antioxidant and anti-inflammatory activities of quercetin and its derivatives. J Funct Foods. (2018) 40:68–75. doi: 10.1016/j.jff.2017.10.047
34. Nagaraj S, Suriyamoorthy P, Subrhamanian H, Kanakasabapathi D. Phytochemical analysis, anti inflammatory activity, in vitro antidiabetic activity and gc-ms profile of Erythrina variegata l. bark. J Appl Pharm Sci. (2016) 6:147–55. doi: 10.7324/JAPS.2016.60722
35. Naoi M, Wu Y, Shamoto-Nagai M, Maruyama W. Mitochondria in neuroprotection by phytochemicals: bioactive polyphenols modulate mitochondrial apoptosis system, function and structure. Int J Mol Sci. (2019) 20:2451. doi: 10.3390/ijms20102451
36. Szewczyk K, Pietrzak W, Klimek K, Miazga-Karska M, Firlej A, Flisiński M, et al. Flavonoid and phenolic acids content and in vitro study of the potential anti-aging properties of Eutrema japonicum (miq.) koidz cultivated in wasabi farm poland. Int J Mol Sci. (2021) 22:6219. doi: 10.3390/ijms22126219
Keywords: biotransformation, Aspergillus niger, Apocynum venetum L., antioxidant activities, metabolic syndrome-associated enzymes, phenolic compounds
Citation: Cao C, Lin D, Zhou Y, Li N, Wang Y, Gong W, Zhu Z, Liu C, Yan L, Hu Z, Peng Y and Xie C (2023) Solid-state fermentation of Apocynum venetum L. by Aspergillus niger: Effect on phenolic compounds, antioxidant activities and metabolic syndrome-associated enzymes. Front. Nutr. 10:1125746. doi: 10.3389/fnut.2023.1125746
Received: 16 December 2022; Accepted: 30 January 2023;
Published: 27 February 2023.
Edited by:
Uroš M. Gašić, Siniša Stanković Institute for Biological Research, University of Belgrade, SerbiaReviewed by:
Jelena BoŽunović, Siniša Stanković Institute for Biological Research, University of Belgrade, SerbiaAleksandar Ž. Kostic, Faculty of Agriculture, University of Belgrade, Serbia
Milica Sredojevic, Faculty of Chemistry, University of Belgrade, Serbia
Copyright © 2023 Cao, Lin, Zhou, Li, Wang, Gong, Zhu, Liu, Yan, Hu, Peng and Xie. This is an open-access article distributed under the terms of the Creative Commons Attribution License (CC BY). The use, distribution or reproduction in other forums is permitted, provided the original author(s) and the copyright owner(s) are credited and that the original publication in this journal is cited, in accordance with accepted academic practice. No use, distribution or reproduction is permitted which does not comply with these terms.
*Correspondence: Chunliang Xie, eGllY2h1bmxpYW5nJiN4MDAwNDA7Y2Fhcy5jbg==; Yuande Peng,
aWJmY3B5ZDMxMyYjeDAwMDQwOzEyNi5jb20=
†These authors have contributed equally to this work and share first authorship