- 1Department of Nursing, Chinese Academy of Medical Sciences-Peking Union Medical College Hospital, Beijing, China
- 2Department of Medical Intensive Care Unit, Chinese Academy of Medical Sciences-Peking Union Medical College Hospital, Beijing, China
- 3Department of Urology, Chinese Academy of Medical Sciences-Peking Union Medical College Hospital, Beijing, China
Background: Patients with critical illness often develop low skeletal muscle mass (LSMM) for multiple reasons. Numerous studies have explored the association between LSMM and mortality. The prevalence of LSMM and its association with mortality are unclear. This systematic review and meta-analysis was performed to examine the prevalence and mortality risk of LSMM among critically ill patients.
Methods: Three internet databases (Embase, PubMed, and Web of Science) were searched by two independent investigators to identify relevant studies. A random-effects model was used to pool the prevalence of LSMM and its association with mortality. The GRADE assessment tool was used to assess the overall quality of evidence.
Results: In total, 1,582 records were initially identified in our search, and 38 studies involving 6,891 patients were included in the final quantitative analysis. The pooled prevalence of LSMM was 51.0% [95% confidence interval (CI), 44.5–57.5%]. The subgroup analysis showed that the prevalence of LSMM in patients with and without mechanical ventilation was 53.4% (95% CI, 43.2–63.6%) and 48.9% (95% CI, 39.7–58.1%), respectively (P-value for difference = 0.44). The pooled results showed that critically ill patients with LSMM had a higher risk of mortality than those without LSMM, with a pooled odds ratio of 2.35 (95% CI, 1.91–2.89). The subgroup analysis based on the muscle mass assessment tool showed that critically ill patients with LSMM had a higher risk of mortality than those with normal skeletal muscle mass regardless of the different assessment tools used. In addition, the association between LSMM and mortality was statistically significant, independent of the different types of mortality.
Conclusion: Our study revealed that critically ill patients had a high prevalence of LSMM and that critically ill patients with LSMM had a higher risk of mortality than those without LSMM. However, large-scale and high-quality prospective cohort studies, especially those based on muscle ultrasound, are required to validate these findings.
Systematic review registration: http://www.crd.york.ac.uk/PROSPERO/, identifier: CRD42022379200.
1. Introduction
The intensive care unit (ICU), created in 1953, has become an integral part of the health care system worldwide for patients with critical illness (1). The survival rates of patients with critical illness have improved because of major progress in medical technology, greater understanding of disease pathophysiology, and use of multidisciplinary approaches to care (2). However, improving the prognosis of critically ill patients remains an important issue for critical care departments. Numerous risk factors are closely related to the mortality of critically ill patients, such as undernutrition (3), multiple organ failure (4), advanced age (5), sepsis (6), muscle wasting, frailty (7), and other factors. Among these factors, muscle wasting has drawn increasing attention from intensive care physicians.
Muscle wasting, also termed myopenia, is defined as wasting or thinning of muscle mass (8). Muscle wasting is assessed using a semiautomatic method of measuring the amount of muscle area on a computed tomography (CT) scan using predefined Hounsfield units (9). The skeletal muscle index is then computed by dividing the estimated muscle area by the body height. A reasonable threshold for prediction of low skeletal muscle mass (LSMM) has been suggested to be a skeletal muscle index in the fifth age-matched percentile (10). Previous studies have confirmed that older people with muscle wasting have a high risk of falls (11), mortality (12), fractures (13), and functional decline (14), which lead to adverse impacts on the economy and society as well as increased healthcare expenditures (15). Therefore, management of LSMM has become an important issue. Numerous methods have been adopted to assess muscle mass, including magnetic resonance imaging, CT, ultrasound, dual-energy X-ray, bioelectrical impedance, and anthropometric methods (16). The most commonly used method in critically ill patients is CT.
In recent decades, LSMM has become a focus of research in critical care. Critically ill patients can easily develop LSMM secondary to malnutrition, inactivity, and inflammatory reactions (17). Several studies have explored the association between LSMM and adverse outcomes among critically ill patients (18–20). These studies showed that the presence of LSMM based on CT scans was associated with a high risk of all-cause death among critically ill patients (18, 21) and that measurement of the total psoas muscle area can improve the prediction of mortality (22). In addition, many studies have shown that the prevalence of LSMM among critically ill people is higher than that among older people (23, 24). A recent systematic review revealed an LSMM prevalence of 50.9% (25). However, this review consisted of only 9 studies involving 1,563 patients, and the studies used only CT to assess muscle mass. Some studies have detected LSMM by newer technologies such as ultrasound (26, 27). Most importantly, the above-mentioned systematic review did not perform a subgroup analysis. Moreover, many new articles have explored the impact of LSMM on mortality (18, 19, 21, 28–35). Therefore, we considered it very important to perform an updated systematic review to summarize the prevalence and mortality risk of LSMM in critically ill patients. The aim of our study was to systematically summarize the prevalence of muscle wasting among critically ill patients and identify whether critical illness with LSMM can increase the risk of mortality.
2. Methods
2.1. Search strategy
This systematic review is reported in accordance with the PRISMA guidelines and was preregistered in the PROSPERO database (CRD42022379200). Two authors searched for relevant articles in three internet databases (PubMed, Embase, and Web of Science) from database inception to 1 September 2022. We used the following keywords and Medical Subject Headings (MeSH) terms to identify relevant studies: “muscle mass” or “muscle wasting” or “low skeletal muscle” and (“mortality” or “death” or “survival”) and “critically ill patient”. The detailed search strategy is shown in Supplementary File 1.
2.2. Inclusion and exclusion criteria
The patients, intervention, comparison, outcomes, and study design (PICOS) principle was adopted to confirm study eligibility. The inclusion criteria were as follows: (1) the patients involved in the study were critically ill (i.e., adult patients treated in the ICU); (2) as the exposure, LSMM was definitively diagnosed based on CT scans, anthropometric methods, and ultrasound; (3) the article presented the prevalence of LSMM, or the prevalence could be calculated using the data available within the article; and (4) the study design was observational (cohort study or cross-sectional study). Reviews, case reports, comments, correspondence articles, letters, and abstracts were excluded because complete quality assessment of such reports could not be performed.
2.3. Study selection and data extraction
Two authors independently formulated the search strategy and screened the articles. First, the results of the relevant studies from the three databases were imported into EndNote X9 software, and duplicates were deleted. Next, the authors screened the title and abstract based on the PICOS principle, checked the abstract for potential relevance, and screened the full text. The final studies were confirmed after careful review of the full text. During this process, disagreements were resolved by discussion with a third reviewer. Two authors also independently extracted the data based on standardized forms consisting of author, year, country, main diagnosis, age, sample size, prevalence of muscle wasting, number of female/male participants, prevalence of muscle wasting by sex, muscle wasting assessment tool used, and effect size of the association between LSMM and mortality.
2.4. Assessment of study quality
We used the Newcastle–Ottawa Scale to assess the quality and methodology of the included studies. The assessing item including selection, comparability, and outcome. To minimize the potential for bias, we had two reviewers independently evaluate each included study using the Newcastle-Ottawa Scale, and we resolved any discrepancies through discussion and consensus. The total score of the included studies ranged from 0 to 9 points, and the quality of the study was defined as poor, moderate, or high with corresponding scores of 0–4, 5–6, and 7–9 points, respectively.
2.5. The quality of evidence
We used GRADE tool to assess the overall quality of the evidence. This tool consisted of five items including risk of bias, inconsistency, indirectness, imprecision and publication bias.
2.6. Outcome measures
The primary outcome of this systematic review was the prevalence of LSMM, and the secondary outcome was all-cause mortality.
2.7. Statistical analysis
All statistical analyses were performed with Stata Version 14 (StataCorp, College Station, TX, USA). Metaprop, a Stata command, was used to pool the prevalence of muscle wasting from each included study, and the metan command was used to combine the results regarding the association between LSMM and mortality risk of all studies. A random-effects model was used because of the high heterogeneity (I2 > 50%) across studies caused by differences in countries, definitions, sample sizes, and reasons for ICU admission. In addition, to detect the original cause of heterogeneity, different subgroup analyses based on country, sex, sample size (<100 vs. ≥100), age group, main diagnosis for ICU admission, mechanical ventilation, and type of outcome were performed if there were more than two studies within each stratum. Finally, a sensitivity analysis and test of publication bias were performed.
3. Results
3.1. Study selection
In total, 1,582 records were identified from 3 databases (PubMed, n = 615; Embase, n = 743; and Web of Science, n = 224). After deleting duplicates, 1,357 studies remained to be screened. Two authors deleted 1,269 studies after checking the title and abstract, resulting in 88 studies for full-text review. Of these, 38 studies were included in the final quantitative analysis based on the inclusion and exclusion criteria (18–21, 24, 26–58). The main reasons for exclusion were an ineligible study design and irrelevant studies exploring the association between LSMM and other clinical outcomes (Figure 1).
3.2. Characteristics of included studies
Thirty-eight studies involving 6,891 participants met the eligibility criteria. The participants' mean or median age ranged from 41.4 to 79 years. Most of the studies were conducted in the United States (n = 9), followed by Korea (n = 7) and Japan (n = 6). Four studies were performed in the Netherlands, three in China, and two in Italy. Only one study each was conducted in Brazil, India, Germany, and Malaysia. The main diagnosis among the participants in the included studies was sepsis (14 studies), trauma (7 studies), surgical diseases (3 studies), and COVID-19 (1 study). Thirteen studies involved patients with mixed diagnoses collectively referred to as “critical illness”. The highest prevalence of LSMM was 90%, and the lowest prevalence was 25%. The largest sample size of the included studies was 905 (33), and the smallest sample size was 37 (31). A total of 89.4% of studies used CT scans to assess muscle mass, whereas only two studies used ultrasonography (26, 27) and two used anthropometric methods (19, 50). Thirty studies explored the association between LSMM and mortality among critically ill patients (Table 1). Thirteen studies considered the in-hospital mortality as the main outcome and six studies reported 30-day mortality, followed 6 studies for 1-year mortality. Supplementary Table 1 displayed the time for assessing muscle mass.
3.3. The diagnosis criteria and cut-off points for LSMM of each study
Based on the information provided, the diagnosis criteria and cut-off points for LSMM among the included studies were summarized in Supplementary Table 2. For CT-scans, the majority of studies used skeletal muscle mass index (SMI) to define LSMM. Some studies used skeletal muscle area (SMA), and only two studies adopted total psoas area (TPA). In addition, ultrasonography was used in some studies to assess the Femoris Muscle for confirming LSMM. Whereas, the cut-off value for confirming LSMM were varied across these studies.
3.4. Meta-analysis of prevalence of LSMM in critically ill patients
In total, 37 studies reported the prevalence of LSMM among critically ill patients (24, 27–33, 36–52). The prevalence ranged from 25 to 90%, and the pooled prevalence of LSMM was 51.0% [95% confidence interval (CI), 44.5–57.5%] (Figure 2).
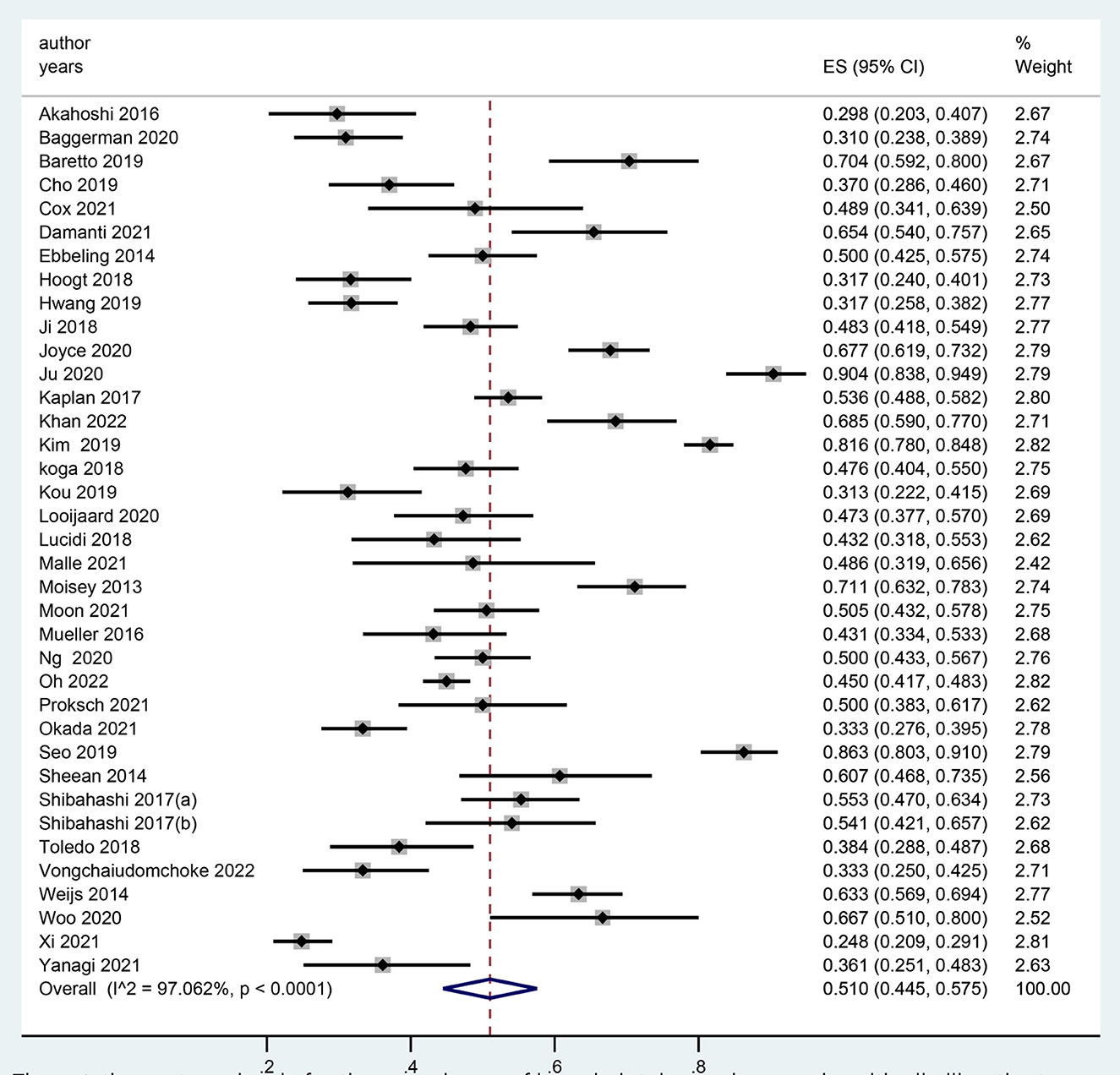
Figure 2. The meta-anlaysis for the prevalence of low skeletal muscle mass in critically ill patients.
3.5. Subgroup analyses of pooled prevalence by different variables
3.5.1. Subgroup analysis by region
The results of the subgroup analysis of the pooled prevalence of LSMM based on region showed that the prevalence of LSMM was 51.1% (95% CI, 40.6–61.6%) among Asians, 46.9% (33.9–60.0%) among Europeans, 51.7% (43.2–60.2%) among Americans, and 65.8% (60.6–70.9%) among Oceanians (Table 2).
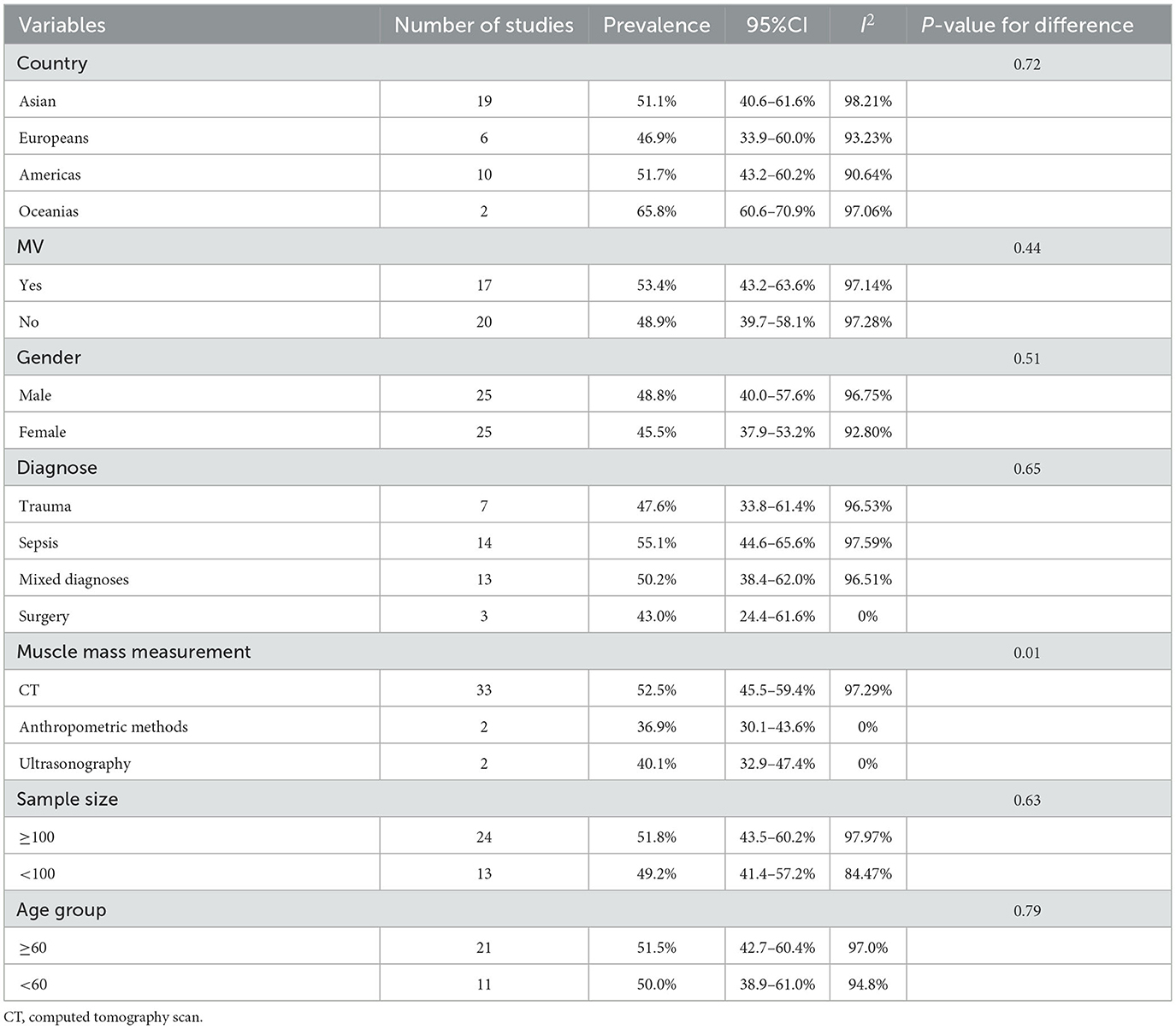
Table 2. Subgroup analyses for the meta-analysis of prevalence of LSMM among critically ill patients.
3.5.2. Subgroup analysis by sex
Twenty-five studies provided the prevalence of LSMM by sex. The results showed no statistically significant difference in the prevalence of LSMM based on sex. The prevalence of LSMM was 48.8% (95% CI, 40.0–57.6%) among men and 45.5% (37.9–53.2%) among women (Table 2).
3.5.3. Subgroup analysis by mechanical ventilation
Seventeen studies included critically ill patients who underwent mechanical ventilation during the hospitalization period. The results of this subgroup analysis showed that the prevalence of LSMM was slightly higher in patients with than without mechanical ventilation, at 53.4% (43.2–63.6%) and 48.9% (39.7–58.1%), respectively. However, there was no significant difference between these two groups (P = 0.44; Table 2).
3.5.4. Subgroup analysis by diagnosis
We categorized the studies into four classifications based on the main diagnosis. Fourteen studies focused on sepsis, and the prevalence of LSMM among these participants was 55.1% (95% CI, 44.6–65.6%). Seven studies focused on critically ill patients with trauma, and the prevalence of LSMM was 47.6% (33.8–61.4%). Only three studies focused on surgical patients, among whom the prevalence of LSMM was 43.0% (24.4–61.6%). The remaining 13 studies involved patients with mixed diagnoses, and the pooled prevalence of LSMM was 50.2% (38.4–62.0%) (Table 2).
3.5.5. Other subgroup analyses of prevalence of LSMM
We split the sample size into two groups (<100 vs. ≥100), and the prevalence of LSMM was similar between the two groups at 49.2% (41.4–57.2%) and 51.8% (43.5–60.2%), respectively. Additionally, a subgroup analysis between age groups split by 60 years showed no statistically significant difference between these two age groups (51.5%, 95%CI: 42.7–60.4%; vs. 50.0%, 95%CI: 38.9–61.0%) (Table 2).
3.6. Meta-analysis of association between LSMM and mortality risk
Thirty studies explored the association between LSMM and mortality risk (19, 20, 24, 26–37, 39, 41–44, 46–48, 50–53, 55, 57, 58). The pooled odds ratio (OR) for the association between LSMM and mortality risk was 2.35 (95% CI, 1.91–2.89), which indicated that critically ill patients with LSMM had an approximately 2.35 higher likelihood of mortality than those without LSMM (Figure 3).
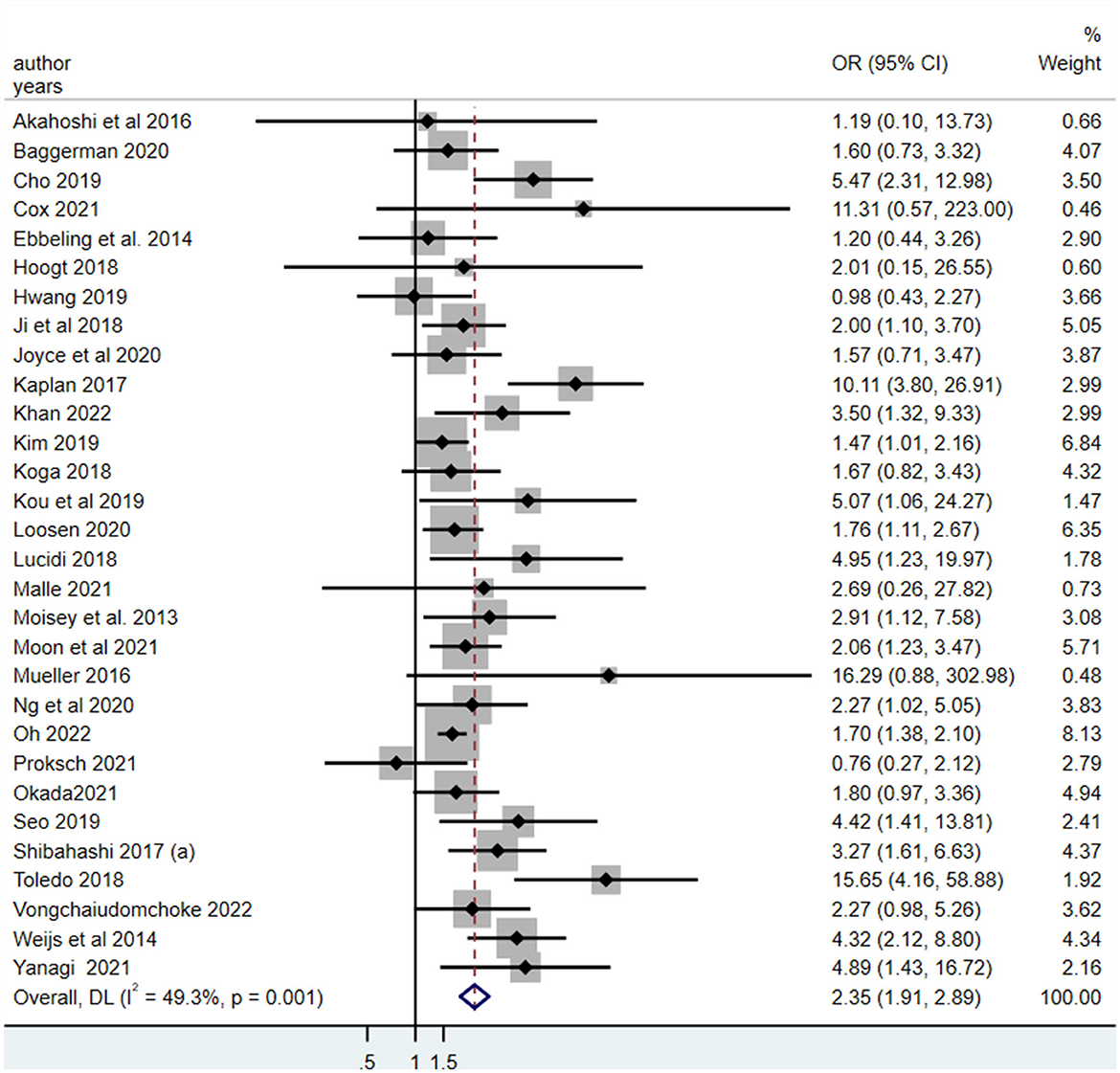
Figure 3. The meta-analysis for the association between the low skeletal muscle mass and all-cause mortality in critically ill patients.
3.7. Subgroup analysis of association between LSMM and mortality risk by different variables
3.7.1. Subgroup analysis based on mechanical ventilation
For critically ill patients who were mechanically ventilated, the pooled OR for the association between LSMM and mortality risk was 2.32 (95% CI, 1.85–2.90). Critically ill patients with LSMM who were not ventilated also had a high risk of mortality, with a pooled OR of 2.42 (95% CI, 1.74–3.36) (Table 3).
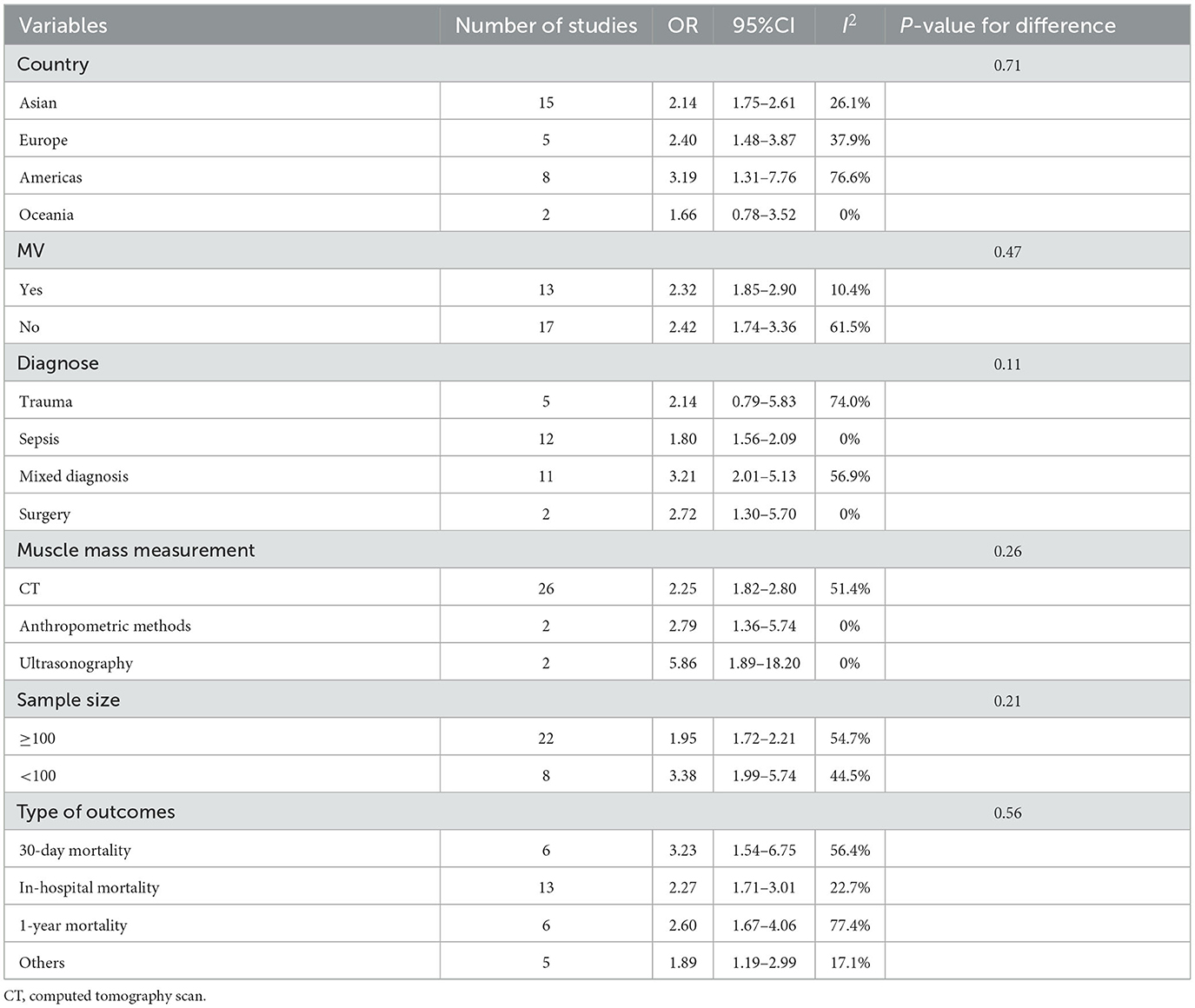
Table 3. Subgroup analyses of the association between low skeletal muscle mass and mortality in critically ill patients.
3.7.2. Subgroup analysis based on outcomes
Critically ill patients with LSMM had a higher risk of mortality than those with normal skeletal muscle mass regardless of the type of outcome (in-hospital mortality, 30-day mortality, or 1-year mortality) with an OR of 2.27 (1.71–3.01), 3.23 (1.54–6.75), and 2.60 (1.67–4.06), respectively.
3.7.3. Subgroup analysis based on assessment tools
We also performed a subgroup analysis based on the assessment tools used. The majority of the studies used CT for assessment of LSMM, and a few studies used anthropometric methods for defining LSMM; only two studies applied ultrasonography. The results showed that critically ill patients with LSMM had a higher mortality risk than those without LSMM when using CT or anthropometric methods; the pooled ORs were 2.25 (95% CI, 1.82–2.80) and 2.79 (1.36–5.74), respectively. We also found an independent association between LSMM and mortality risk among critically ill patients when using ultrasonography for confirmation (pooled OR,5.86; 95% CI, 1.89–18.20) (Table 3).
3.7.4. Subgroup analysis based on diagnosis
Critically ill patients with LSMM had a higher mortality risk than those without LSMM among participants with sepsis (pooled OR, 1.80; 95% CI, 1.56–2.09), surgery (pooled OR, 2.72; 95% CI, 1.30–5.70), or mixed diagnosis (pooled OR, 3.21; 95% CI, 2.01–5.13). However, this association was not statistically significant among trauma patients (pooled OR, 2.14; 95% CI, 0.79–5.83) (Table 3).
3.7.5. Other subgroup analyses of association between LSMM and mortality risk by country and sample size
We also performed subgroup analyses based on country and sample size. These results also showed that critically ill patients with LSMM had a higher mortality risk than their counterparts without LSMM, and the results were independent of country and sample size (Table 3).
3.8. Publication bias
We tested the publication bias for the outcomes of the prevalence of LSMM and mortality. The results showed no potential publication bias when pooling the prevalence of LSMM as indicated by Begg's test (P-value = 0.27) (Supplementary Figure 1a). However, the results of Begg's test (P-value = 0.05) showed potential publication bias when the results for the association between LSMM and mortality risk were pooled (Supplementary Figure 1b).
3.9. Quality assessment
The Newcastle–Ottawa Scale score ranged from 5 to 8 points, and most of the studies had 7 points. This result indicated that the quality of the included studies was relatively good (Supplementary Table 3).
3.10. The results of overall quality of evidence
The summary results of GRADE were showed in Supplementary Table 4, indicating that the evidence was low because there were few inconsistencies in some included studies and they were some publication bias across these studies.
3.11. Sensitivity analysis
A sensitivity analysis was performed by omitting one study and pooling the remaining studies to determine whether the pooled results showed major changes. The results of the sensitivity analysis regarding prevalence or mortality showed no significant changes (Supplementary Figure 2). In addition, we also conducted sensitivity analysis by omitting the studies that used anthropometric methods to define LSMM and the results was almost not changed, which indicated the results was stable (Supplementary Figure 3).
4. Discussion
Our study showed that the prevalence of LSMM among critically ill patients was very high at 51.0% (95% CI, 44.5–57.5%), meaning that more than half of critically ill patients had LSMM. This study also indicated that critically ill patients with LSMM had an approximately 2.35-fold higher mortality risk than those without LSMM. This systematic review and meta-analysis suggests that greater attention to LSMM, early screening of patients at high risk of LSMM, and timely interventions such as comprehensive treatments consisting of exercise and nutrition programs must be performed to slow down the process of muscle wasting. These efforts might reduce the mortality rate among critically ill patients.
Critically ill individuals often lose muscle mass for multiple reasons (17), such as extended time of inactivity, nutrient deficits, and impaired equilibrium between muscle protein synthesis and breakdown. In 2021, a systematic review of nine studies explored the association between LSMM defined by CT and mortality among critically ill patients (25). That study indicated that LSMM based on CT was associated with high short-term mortality. Notably, the study had two main limitations. First, their search strategy was relatively old studies, resulting in only 9 studies involving 1,563 patients, which might have influenced the representativeness of their findings. Second, the authors did not perform subgroup analyses based on different variables; such analyses are very important. Given that more researchers and clinicians are paying attention to LSMM in critically ill patients, increasing numbers of studies are focusing on this topic. Therefore, an updated meta-analysis that can provide an overall picture of the impact of LSMM on critical illness is required. Our study has overcome these limitations.
The pooled prevalence of LSMM in our study was very high at 51.0%. A previous systematic review showed that the pooled prevalence of LSMM was 46.2% (95% CI, 43.95–48.45%) among patients with metastasized colorectal cancer, which was very close to our results (59). We speculate that malnutrition, anorexia, and inflammatory reactions are common characteristics among patients with cancer and critically ill patients, leading to a high prevalence of LSMM (60). In addition, inactivity due to disease and treatment procedures places critically ill patients at high risk for LSMM. Therefore, early screening for LSMM among these select patients is very important.
The present study showed that critically ill patients undergoing mechanical ventilation might have a higher prevalence of LSMM than those without this treatment procedure, although there was no statistically significant difference. We speculate that LSMM may be worse in critically ill inpatients with than without mechanical ventilation, but this requires further study. Patients who require mechanical ventilation often have major lung disease. Mechanical ventilation prevents oral ingestion of food (61); instead, these patients require nasogastric tube feeding or other methods for nutrition and energy, which might not provide sufficient nutrition, thus reducing their protein intake. In addition, mechanical ventilation makes critically ill inpatients immobile. For these two main reasons, critical illness may be associated with a high prevalence of LSMM. Moreover, our study showed that the prevalence of LSMM among patients with sepsis was higher than that among patients with other diagnoses. The reason for this difference might be that patients with sepsis often have a high inflammatory response that leads to multiple organ disorders and malnutrition, resulting in a high risk of LSMM (62, 63). In addition, a previous study revealed that the mechanisms by which sepsis leads to LSMM are disorganized sarcomeres and myofibril dysfunction (64).
Our study also showed that patients with LSMM had a higher risk of mortality than those with normal muscle mass, and this result was in line with a previous study (25). LSMM is the main element in muscle wasting and has been widely confirmed to increase the risk of mortality across different populations (65–68). The mechanisms underlying the association between LSMM and mortality were elucidated in a previous study. In general, most critically ill patients with LSMM had serious comorbidities that increased their mortality risk. In addition, patients with LSMM may have decreased immune system function, which reduces their ability to resist the wide-ranging adverse effects of many treatment procedures such as mechanical ventilation, polypharmacy, and pulse index continuous cardiac output monitoring. Therefore, LSMM complicated by worsening of the patient's primary disease can become a vicious circle that results in a high likelihood of mortality.
Ultrasound measurement is a convenient, widely applied method with which to determine whether patients with critical illness have LSMM (69, 70). Multiple studies have compared the performance between B-mode ultrasound images and typical methods of defining LSMM (71–73). One study indicated that B-mode ultrasound imaging has great potential as a surrogate diagnostic tool for LSMM. Our study also showed a statistically significant association between LSMM and mortality, which is consistent with a previous study (74). One study in which ultrasound was used to detect muscle psoas indices showed that the psoas muscle index was associated with mortality, with a hazard ratio of 0.93 (95% CI, 0.876–0.987) (71). In addition, a study conducted in 2022 revealed that muscle thickness tested by ultrasound was independently associated with death among patients undergoing hemodialysis (73). Therefore, the association between LSMM based on ultrasound and mortality among critically ill patients should be further explored. Given the simplicity, practicality, and convenience of ultrasound for assessing muscle mass, we expect that this technique will provide prognostic value for predicting mortality among critically ill patients.
Our study had several strengths and limitations. First, compared with previous studies, this updated meta-analysis provided a more in-depth subgroup analysis of the pooled prevalence of LSMM and pooled the association between LSMM and mortality. The subgroup analysis based on diagnosis and measurement assessment for LSMM provides more valuable information that can guide clinical practice. Second, this meta-analysis included multiple studies involving 6,891 participants, thus providing a highly representative sample of this special population. Third, a comprehensive statistical analysis was used to test the robustness of our study. However, our meta-analysis also had some limitations. First, there was potential publication bias regarding the association between LSMM and mortality. Some non-English-language studies might have been excluded from the meta-analysis. Second, some studies used the results of a univariate analysis to assess the association between LSMM and mortality, potentially resulting in overestimation of the effect by confounding factors. Third, we considered the effect size by the hazard ratio to be equal to the OR in our study, and caution is therefore required when interpreting our main findings. Fourth, the overall quality of GRADE assessment was “low”, more large-scale and high-quality prospective cohort studies, especially those based on muscle ultrasound, are required to validate these findings.
5. Conclusion
Our study showed a high prevalence of LSMM among critically ill patients and revealed that critically ill patients with LSMM had an 2.35-fold higher mortality risk than those with normal muscle mass. This study indicates that the importance of muscle mass as a potentially important prognostic factor in critically ill patients. Healthcare providers were encouraged to carefully consider the risks and benefits of using CT for muscle mass measurement in critically ill patients, and to consider alternative methods such as ultrasound or bioelectrical impedance analysis (BIA) where appropriate. Early interventions, such as mobilizing patients and providing nutritional support, after confirming LSMM may reduce the mortality rate in this particular population.
Data availability statement
The original contributions presented in the study are included in the article/Supplementary material, further inquiries can be directed to the corresponding authors.
Author contributions
HY and X-XW were responsible for screening and extracting the studies. HM and ZL were responsible for study quality assessment. HY, LW, and X-MZ were responsible for statistical analysis. YX and X-MZ were responsible for the study design and review. HY, X-XW, and X-MZ drafted the manuscript. All authors contributed to the article and approved the submitted version.
Funding
This study was funded by National High Level Hospital Clinical Research Funding (2022-PUMCH-B-031).
Acknowledgments
The authors thank the staff of Peking Union Medical Hospital. The authors also thank Angela Morben, DVM, ELS, from Liwen Bianji (Edanz) (www.liwenbianji.cn), for editing the English text of a draft of this manuscript.
Conflict of interest
The authors declare that the research was conducted in the absence of any commercial or financial relationships that could be construed as a potential conflict of interest.
Publisher's note
All claims expressed in this article are solely those of the authors and do not necessarily represent those of their affiliated organizations, or those of the publisher, the editors and the reviewers. Any product that may be evaluated in this article, or claim that may be made by its manufacturer, is not guaranteed or endorsed by the publisher.
Supplementary material
The Supplementary Material for this article can be found online at: https://www.frontiersin.org/articles/10.3389/fnut.2023.1117558/full#supplementary-material
References
1. Marshall JC, Bosco L, Adhikari NK, Connolly B, Diaz JV, et al. What is an intensive care unit? A report of the task force of the World Federation of Societies of Intensive and Critical Care Medicine. J Crit Care. (2017) 37:270–6. doi: 10.1016/j.jcrc.2016.07.015
2. Prevedello D, Fiore M, Creteur J, Preiser JC. Intensive care units follow-up: a scoping review protocol. BMJ Open. (2020) 10:e037725. doi: 10.1136/bmjopen-2020-037725
3. Lew CCH, Wong GJY, Cheung KP, Chua AP, Chong MFF, Miller M. Association between Malnutrition and 28-day mortality and intensive care length-of-stay in the critically ill: a prospective cohort study. Nutrients. (2017) 10:10. doi: 10.3390/nu10010010
4. Lone NI, Walsh TS. Impact of intensive care unit organ failures on mortality during the five years after a critical illness. Am J Respir Crit Care Med. (2012) 186:640–7. doi: 10.1164/rccm.201201-0059OC
5. Martin-Loeches I, Guia MC, Vallecoccia MS, Suarez D, Ibarz M, Irazabal M, et al. Risk factors for mortality in elderly and very elderly critically ill patients with sepsis: a prospective, observational, multicenter cohort study. Ann Intensive Care. (2019) 9:26. doi: 10.1186/s13613-019-0495-x
6. Biason L, Teixeira C, Haas JS, Cabral Cd, Friedman G. Effects of sepsis on morbidity and mortality in critically ill patients 2 years after intensive care unit discharge. Am J Crit Care. (2019) 28:424–32. doi: 10.4037/ajcc2019638
7. Muscedere J, Waters B, Varambally A, Bagshaw SM, Boyd JG, Maslove D, et al. The impact of frailty on intensive care unit outcomes: a systematic review and meta-analysis. Intensive Care Med. (2017) 43:1105–22. doi: 10.1007/s00134-017-4867-0
8. Cruz-Jentoft AJ, Sayer AA. Sarcopenia. Lancet. (2019) 393:2636–46. doi: 10.1016/S0140-6736(19)31138-9
9. Albano D, Messina C, Vitale J, Sconfienza LM. Imaging of sarcopenia: old evidence and new insights. Eur Radiol. (2020) 30:2199–208. doi: 10.1007/s00330-019-06573-2
10. Cruz-Jentoft AJ, Bahat G, Bauer J, Boirie Y, Bruyère O, Cederholm T, et al. Sarcopenia: revised European consensus on definition and diagnosis. Age Ageing. (2019) 48:601. doi: 10.1093/ageing/afz046
11. Zhang X, Huang P, Dou Q, Wang C, Zhang W, Yang Y, et al. Falls among older adults with sarcopenia dwelling in nursing home or community: a meta-analysis. Clin Nutr. (2020) 39:33–9. doi: 10.1016/j.clnu.2019.01.002
12. Xu J, Wan CS, Ktoris K, Reijnierse EM, Maier AB. Sarcopenia is associated with mortality in adults: a systematic review and meta-analysis. Gerontology. (2022) 68:361–76. doi: 10.1159/000517099
13. Yeung SSY, Reijnierse EM, Pham VK, Trappenburg MC, Lim WK, Meskers CGM, et al. Sarcopenia and its association with falls and fractures in older adults: a systematic review and meta-analysis. J Cachexia Sarcopenia Muscle. (2019) 10:485–500. doi: 10.1002/jcsm.12411
14. Xia L, Zhao R, Wan Q, Wu Y, Zhou Y, Wang Y, et al. Sarcopenia and adverse health-related outcomes: an umbrella review of meta-analyses of observational studies. Cancer Med. (2020) 9:7964–78. doi: 10.1002/cam4.3428
15. Ali S, Garcia JM. Sarcopenia, cachexia and aging: diagnosis, mechanisms and therapeutic options - a mini-review. Gerontology. (2014) 60:294–305. doi: 10.1159/000356760
16. Sizoo D, de Heide LJ, Emous M, van Zutphen T, Navis G, van Beek AP. Measuring muscle mass and strength in obesity: a review of various methods. Obesity Surg. (2021) 31:384–93. doi: 10.1007/s11695-020-05082-2
17. Chapple LS, Parry SM, Schaller SJ. Attenuating muscle mass loss in critical illness: the role of nutrition and exercise. Curr Osteoporos Rep. (2022) 20:290–308. doi: 10.1007/s11914-022-00746-7
18. Woo HY, Oh S-Y, Lee H, Ryu HG. Evaluation of the association between decreased skeletal muscle mass and extubation failure after long-term mechanical ventilation. Clin Nutr. (2020) 39:2764–70. doi: 10.1016/j.clnu.2019.12.002
19. Vongchaiudomchoke W, Sathitkarnmanee B, Thanakiattiwibun C, Jarungjitaree S, Chaiwat O. The association between sarcopenia and functional outcomes after hospital discharge among critically ill surgical patients. Asian J Surg. (2022) 45:1408–13. doi: 10.1016/j.asjsur.2021.09.012
20. Toledo DO, Carvalho AM, Oliveira AMRR, Toloi JM, Silva AC, Farah JF, et al. The use of computed tomography images as a prognostic marker in critically ill cancer patients. Clin Nutr ESPEN. (2018) 25:114–20. doi: 10.1016/j.clnesp.2018.03.122
21. Xi F, Tan S, Gao T, Ding W, Sun J, Wei C, et al. Low skeletal muscle mass predicts poor clinical outcomes in patients with abdominal trauma. Nutrition. (2021) 89:111229. doi: 10.1016/j.nut.2021.111229
22. Zhang X-M, Zhang W-W, Yu X-Z, Dou Q-L, Cheng AS. Comparing the performance of SOFA, TPA combined with SOFA and APACHE-II for predicting ICU mortality in critically ill surgical patients: a secondary analysis. Clin Nutr. (2020) 39:2902–9. doi: 10.1016/j.clnu.2019.12.026
23. Tey SL, Chew STH, How CH, Yalawar M, Baggs G, Chow WL, et al. Factors associated with muscle mass in community-dwelling older people in Singapore: Findings from the SHIELD study. PLoS ONE. (2019) 14:e0223222. doi: 10.1371/journal.pone.0223222
24. Joyce PR, O'Dempsey R, Kirby G, Anstey C. A retrospective observational study of sarcopenia and outcomes in critically ill patients. Anaesth Intensive Care. (2020) 48:229–35. doi: 10.1177/0310057X20922234
25. Meyer HJ, Wienke A, Surov A. Computed tomography-defined low skeletal muscle mass as a prognostic marker for short-term mortality in critically ill patients: a systematic review and meta-analysis. Nutrition. (2021) 91–92:111417. doi: 10.1016/j.nut.2021.111417
26. Yanagi N, Koike T, Kamiya K, Hamazaki N, Nozaki K, Ichikawa T, et al. Assessment of sarcopenia in the intensive care unit and 1-year mortality in survivors of critical illness. Nutrients. (2021) 13:726. doi: 10.3390/nu13082726
27. Mueller N, Murthy S, Tainter CR, Lee J, Riddell K, Fintelmann FJ, et al. Can sarcopenia quantified by ultrasound of the rectus femoris muscle predict adverse outcome of surgical intensive care unit patients as well as frailty? A prospective, observational cohort study. Annal Surg. (2016) 264:1116–24. doi: 10.1097/SLA.0000000000001546
28. Cox MC, Booth M, Ghita G, Wang Z, Gardner A, Hawkins RB, et al. The impact of sarcopenia and acute muscle mass loss on long-term outcomes in critically ill patients with intra-abdominal sepsis. J Cachexia Sarcopenia Muscle. (2021) 12:1203–13. doi: 10.1002/jcsm.12752
29. Khan S, Benjamin J, Maiwall R, Tripathi H, Kapoor PB, Shasthry V, et al. Sarcopenia is the independent predictor of mortality in critically ill patients with cirrhosis. J Clin Transl Res. (2022) 8:200–8.
30. Kim T, Huh S, Kim S-Y, Han J, Lee SE, Cho WH, et al. ICU rehabilitation is associated with reduced long-term mortality from sepsis in patients with low skeletal muscle mass: a case control study. Ann Transl Med. (2019) 7:430. doi: 10.21037/atm.2019.08.117
31. Malle O, Maurer D, Wagner D, Schnedl C, Amrein S, Pieber T, et al. Morphometric parameters of muscle and bone in critically ill patients: Post hoc analysis of the VITdAL-ICU trial. Wien Klin Wochenschr. (2021) 133:529–35. doi: 10.1007/s00508-020-01736-4
32. Moon SW, Kim SY, Choi JS, Leem AY, Lee SH, Park MS, et al. Thoracic skeletal muscle quantification using computed tomography and prognosis of elderly ICU patients. Sci Rep. (2021) 11:23461. doi: 10.1038/s41598-021-02853-4
33. Oh HJ, Kim JH, Kim HR, Ahn JY, Jeong SJ, Ku NS, et al. The impact of sarcopenia on short-term and long-term mortality in patients with septic shock. J Cachexia Sarcopenia Muscle. (2022) 13:2054–63. doi: 10.1002/jcsm.12995
34. Okada Y, Kiguchi T, Okada A, Iizuka R, Iwami T, Ohtsuru S. Predictive value of sarcopenic findings in the psoas muscle on CT imaging among patients with sepsis. Am J Emerg Med. (2021) 47:180–6. doi: 10.1016/j.ajem.2021.04.011
35. Proksch DM, Kelley KM, White S, Burgess JR. Identification of sarcopenia in elderly trauma patients: the value of clinical competency and experience. Am Surg. (2022) 88:339–42. doi: 10.1177/0003134820982854
36. Akahoshi T, Yasuda M, Momii K, Kubota K, Shono Y, Kaku N, et al. Sarcopenia is a predictive factor for prolonged intensive care unit stays in high-energy blunt trauma patients. Acute Med Surg. (2016) 3:326–31. doi: 10.1002/ams2.195
37. Baggerman MR, van Dijk DPJ, Winkens B, van Gassel RJJ, Bol ME, Schnabel RM, et al. Muscle wasting associated co-morbidities, rather than sarcopenia are risk factors for hospital mortality in critical illness. J Crit Care. (2020) 56:31–6. doi: 10.1016/j.jcrc.2019.11.016
38. Barreto EF. Validation of the sarcopenia index to assess muscle mass in the critically ill: A novel application of kidney function markers. Clin Nutr. (2019) 38:1362–7. doi: 10.1016/j.clnu.2018.05.031
39. Cho WH, Choi YY, Byun KS, Lee SE, Jeon D, Kim YS, et al. Prognostic value of sarcopenia for long-term mortality in extracorporeal membrane oxygenation for acute respiratory failure. ASAIO Journal. (2020) 66:367–72. doi: 10.1097/MAT.0000000000001006
40. Damanti S, Cristel G, Ramirez GA, Bozzolo EP, Da Prat V, Gobbi A, et al. Influence of reduced muscle mass and quality on ventilator weaning and complications during intensive care unit stay in COVID-19 patients. Clin Nutr. (2022) 41:2965–72. doi: 10.1016/j.clnu.2021.08.004
41. de Hoogt, PA, Reisinger, KW, Tegels, JJW, Bosmans, JWAM, Tijssen, F, Stoot, JHMB. Functional compromise cohort study (FCCS): sarcopenia is a strong predictor of mortality in the intensive care unit. World J Surg. (2018) 42:1733–41. doi: 10.1007/s00268-017-4386-8
42. Ebbeling L, Grabo DJ, Shashaty M, Dua R, Sonnad SS, Sims CA, et al. Psoas:lumbar vertebra index: central sarcopenia independently predicts morbidity in elderly trauma patients. Eur J Trauma Emerg Surg. (2014) 40:57–65. doi: 10.1007/s00068-013-0313-3
43. Hwang F, McGreevy CM, Pentakota SR, Verde D, Park JH, Berlin A, et al. Sarcopenia is predictive of functional outcomes in older trauma patients. Cureus. (2019) 11:e6154. doi: 10.7759/cureus.6154
44. Ji Y, Cheng B, Xu Z, Ye H, Lu W, Luo X, et al. Impact of sarcopenic obesity on 30-day mortality in critically ill patients with intra-abdominal sepsis. J Crit Care. (2018) 46:50–4. doi: 10.1016/j.jcrc.2018.03.019
45. Ju S, Choi SM, Park YS, Lee C-H, Lee S-M, Yoo C-G, et al. Rapid muscle loss negatively impacts survival in critically ill patients with cirrhosis. J Intensive Care Med. (2020) 35:663–71. doi: 10.1177/0885066618775706
46. Kaplan SJ, Pham TN, Arbabi S, Gross JA, Damodarasamy M, Bentov I, et al. Association of radiologic indicators of frailty with 1-year mortality in older trauma patients: opportunistic screening for sarcopenia and osteopenia. JAMA Surg. (2017) 152:e164604. doi: 10.1001/jamasurg.2016.4604
47. Koga Y, Fujita M, Yagi T, Todani M, Nakahara T, Kawamura Y, et al Early enteral nutrition is associated with reduced in-hospital mortality from sepsis in patients with sarcopenia. J Crit Care. (2018) 47:153–8. doi: 10.1016/j.jcrc.2018.06.026
48. Kou H-W, Yeh C-H, Tsai H-I, Hsu C-C, Hsieh Y-C, Chen W-T, et al. Sarcopenia is an effective predictor of difficult-to-wean and mortality among critically ill surgical patients. PLoS ONE. (2019) 14:e0220699. doi: 10.1371/journal.pone.0220699
49. Looijaard WGPM, Stapel SN, Dekker IM, Rusticus H, Remmelzwaal S, Girbes ARJ, et al. Identifying critically ill patients with low muscle mass: agreement between bioelectrical impedance analysis and computed tomography. Clin Nutr. (2020) 39:1809–17. doi: 10.1016/j.clnu.2019.07.020
50. Lucidi C, Lattanzi B, Di Gregorio V, Incicco S, D'Ambrosio D, Venditti M, et al. A low muscle mass increases mortality in compensated cirrhotic patients with sepsis. Liver Int. (2018) 38:851–7. doi: 10.1111/liv.13691
51. Moisey LL, Mourtzakis M, Cotton BA, Premji T, Heyland DK, Wade CE, et al. Skeletal muscle predicts ventilator-free days, ICU-free days, and mortality in elderly ICU patients. Crit Care. (2013) 17:R206. doi: 10.1186/cc12901
52. Ng CC, Lee Z-Y, Chan WY, Jamaluddin MF, Tan LJ, Sitaram PN, et al. Low muscularity as assessed by abdominal computed tomography on intensive care unit admission is associated with mortality in a critically ill Asian population. J Parent Enteral Nutr. (2020) 44:425–33. doi: 10.1002/jpen.1666
53. Seo D-W, Kim KW, Sohn CH, Ryoo SM, Kim Y-J, Shin A, et al. Progressive loss of muscle mass could be an adverse prognostic factor of 28-day mortality in septic shock patients. Sci Rep. (2019) 9:16471. doi: 10.1038/s41598-019-52819-w
54. Sheean PM, Peterson SJ, Perez SG, Troy KL, Patel A, Sclamberg JS, et al. The prevalence of sarcopenia in patients with respiratory failure classified as normally nourished using computed tomography and subjective global assessment. J Parent Enteral Nutr. (2014) 38:873–9. doi: 10.1177/0148607113500308
55. Shibahashi K, Sugiyama K, Kashiura M, Hamabe Y. Decreasing skeletal muscle as a risk factor for mortality in elderly patients with sepsis: a retrospective cohort study. J Inten Care. (2017) 5:8. doi: 10.1186/s40560-016-0205-9
56. Shibahashi K, Sugiyama K, Hoda H, Hamabe Y. Skeletal muscle as a factor contributing to better stratification of older patients with traumatic brain injury: a retrospective cohort study. World Neurosurg. (2017) 106:589–94. doi: 10.1016/j.wneu.2017.07.025
57. Weijs PJM, Looijaard WGPM, Dekker IM, Stapel SN, Girbes AR, Oudemans-van Straaten HM, et al. Low skeletal muscle area is a risk factor for mortality in mechanically ventilated critically ill patients. Crit Care. (2014) 18:R12. doi: 10.1186/cc13189
58. Loosen SH, Schulze-Hagen M, Püngel T, Bündgens L, Wirtz T, Kather JN, et al. Skeletal muscle composition predicts outcome in critically ill patients. Crit Care Explor. (2020) 2:e0171. doi: 10.1097/CCE.0000000000000171
59. Meyer H-J, Strobel A, Wienke A, Surov A. Prognostic role of low-skeletal muscle mass on staging computed tomography in metastasized colorectal cancer: a systematic review and meta-analysis. Clin Colorectal Cancer. (2022) 21:e213–25. doi: 10.1016/j.clcc.2022.03.005
60. Fairman CM, Lønbro S, Cardaci TD, VanderVeen BN, Nilsen TS, Murphy AE. Muscle wasting in cancer: opportunities and challenges for exercise in clinical cancer trials. JCSM Rapid Commun. (2022) 5:52–67. doi: 10.1002/rco2.56
61. Allen K, Hoffman L. Enteral nutrition in the mechanically ventilated patient. Nutr Clin Pract. (2019) 34:540–57. doi: 10.1002/ncp.10242
62. Hotchkiss RS, Monneret G, Payen D. Sepsis-induced immunosuppression: from cellular dysfunctions to immunotherapy. Nat Rev Immunol. (2013) 13:862–74. doi: 10.1038/nri3552
63. Kosałka K, Wachowska E, Słotwiński R. Disorders of nutritional status in sepsis - facts and myths. Przeglad Gastroenterol. (2017) 12:73–82. doi: 10.5114/pg.2017.68165
64. Goossens C, Weckx R, Derde S, Van Helleputte L, Schneidereit D, Haug M, et al. Impact of prolonged sepsis on neural and muscular components of muscle contractions in a mouse model. J Cachexia Sarcopenia Muscle. (2021) 12:443–55. doi: 10.1002/jcsm.12668
65. de Santana FM, Premaor MO, Tanigava NY, Pereira RMR. Low muscle mass in older adults and mortality: a systematic review and meta-analysis. Exp Gerontol. (2021) 152:111461. doi: 10.1016/j.exger.2021.111461
66. Pierobon ES, Moletta L, Zampieri S, Sartori R, Brazzale AR, Zanchettin G, et al. The prognostic value of low muscle mass in pancreatic cancer patients: a systematic review and meta-analysis. J Clin Med. (2021) 10:33. doi: 10.3390/jcm10143033
67. Ribeiro, HS, Neri, SGR, Oliveira, JS, Bennett, PN, Viana, JL, Lima, RM. Association between sarcopenia and clinical outcomes in chronic kidney disease patients: a systematic review and meta-analysis. Clin Nutr. (2022) 41:1131–40. doi: 10.1016/j.clnu.2022.03.025
68. Weerink LBM, van der Hoorn A, van Leeuwen BL, de Bock GH. Low skeletal muscle mass and postoperative morbidity in surgical oncology: a systematic review and meta-analysis. J Cachexia Sarcopenia Muscle. (2020) 11:636–49. doi: 10.1002/jcsm.12529
69. Leigheb M, de Sire A, Colangelo M, Zagaria D, Grassi FA, Rena O, et al. Sarcopenia diagnosis: reliability of the ultrasound assessment of the tibialis anterior muscle as an alternative evaluation tool. Diagnostics. (2021) 11:2158. doi: 10.3390/diagnostics11112158
70. Sconfienza LM. Sarcopenia: ultrasound today, smartphones tomorrow? Eur Radiol. (2019) 29:1–2. doi: 10.1007/s00330-018-5833-0
71. Hari A, Berzigotti A, Štabuc B, Caglevič N. Muscle psoas indices measured by ultrasound in cirrhosis - Preliminary evaluation of sarcopenia assessment and prediction of liver decompensation and mortality. Dig Liver Dis. (2019) 51:1502–7. doi: 10.1016/j.dld.2019.08.017
72. Lee Z-Y, Ong SP, Ng CC, Yap CSL, Engkasan JP, Barakatun-Nisak MY, et al. Association between ultrasound quadriceps muscle status with premorbid functional status and 60-day mortality in mechanically ventilated critically ill patient: a single-center prospective observational study. Clin Nutr. (2021) 40:1338–47. doi: 10.1016/j.clnu.2020.08.022
73. Sabatino A, Kooman JP, Di Motta T, Cantarelli C, Gregorini M, Bianchi S, et al. Quadriceps muscle thickness assessed by ultrasound is independently associated with mortality in hemodialysis patients. Eur J Clin Nutr. (2022) 76:1719–26. doi: 10.1038/s41430-022-01166-7
Keywords: prevalence, mortality, low skeletal muscle mass, critically ill patients, systematic review and meta-analysis
Citation: Yang H, Wan X-X, Ma H, Li Z, Weng L, Xia Y and Zhang X-M (2023) Prevalence and mortality risk of low skeletal muscle mass in critically ill patients: an updated systematic review and meta-analysis. Front. Nutr. 10:1117558. doi: 10.3389/fnut.2023.1117558
Received: 06 December 2022; Accepted: 11 April 2023;
Published: 12 May 2023.
Edited by:
Nathan A. Berger, Case Western Reserve University, United StatesReviewed by:
Shao-Yuan Chuang, National Health Research Institutes, TaiwanNobuto Nakanishi, Kobe University, Japan
Copyright © 2023 Yang, Wan, Ma, Li, Weng, Xia and Zhang. This is an open-access article distributed under the terms of the Creative Commons Attribution License (CC BY). The use, distribution or reproduction in other forums is permitted, provided the original author(s) and the copyright owner(s) are credited and that the original publication in this journal is cited, in accordance with accepted academic practice. No use, distribution or reproduction is permitted which does not comply with these terms.
*Correspondence: Ying Xia, eGlheWluZzg4MSYjeDAwMDQwO3NpbmEuY29t; Xiao-Ming Zhang, emhhbmdtdXhpMDMxMCYjeDAwMDQwOzE2My5jb20=
†These authors share first authorship